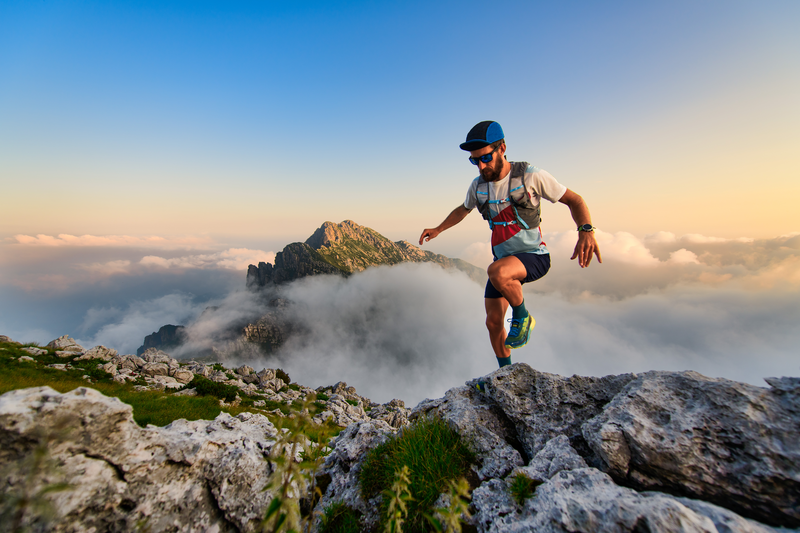
95% of researchers rate our articles as excellent or good
Learn more about the work of our research integrity team to safeguard the quality of each article we publish.
Find out more
ORIGINAL RESEARCH article
Front. Environ. Sci. , 22 July 2021
Sec. Soil Processes
Volume 9 - 2021 | https://doi.org/10.3389/fenvs.2021.712301
This article is part of the Research Topic Climate Change and Anthropogenic Impacts on Soil Organic Matter View all 5 articles
Assessing the response of soil heterotrophic and autotrophic respiration to climate change is critical for forecasting terrestrial carbon cycle behavior in the future. In the present study, we conducted a drought experiment in Vitexnegundo var. heterophylla shrub ecosystem of the Middle Taihang Mountain. Three precipitation manipulation treatments (natural conditions/ambient precipitation (CK), reduced precipitation by 30% (PE30), and reduced precipitation by 60% (PE60)) were used to study the impact of different levels of precipitation exclusion on total soil respiration (Rs) and its heterotrophic (Rh) and autotrophic (Ra) components. Our results showed that the rates of Rs and its components were significantly decreased under the precipitation exclusion treatments. The proportion of Rh in Rs reduced from 72.6% for CK to 71.9% under PE60. The annual cumulative C fluxes of Rs decreased by 47.8 g C m−2 in PE30 and 106.0 g C m−2 in PE60, respectively. An exponential relationship was observed between the rate of each soil respiration component and soil temperature in all treatments ( p < 0.01). Moreover, each soil respiration component rate was better represented by a quadratic model which included soil moisture ( p < 0.01). However, including both of soil temperature and soil moisture did not explain more variation in soil respiration components compared than the regression model with soil moisture only. In addition, excluding precipitation increased the temperature sensitivity (Q10 values) of Rs and its Ra and Rh components compared to the control. Collectively, our findings suggest that increased drought will inhibit the release of carbon from the soil to the atmosphere, and will likely decrease the contribution of Rh to Rs in this semiarid shrubland ecosystem.
In future climate scenarios, the intensity and frequency of precipitation is forecasted to decrease in many areas (IPCC, 2013; Sun et al., 2019). Reductions in precipitation induced by climate change will greatly impact terrestrial carbon (C) cycling, including soil respiration (Rs), especially in arid and semiarid regions (Ahlström et al., 2015; van der Molen et al., 2011). Terrestrial ecosystems release approximately 58 Pg CO2 per year, which is 10 times more than fossil fuels emissions (Schlesinger and Andrews, 2000; Huang et al., 2018). Accordingly, even small changes in Rs may influence inter-annual fluctuations in C cycling, with subsequent feedbacks on climate change (Bond-Lamberty and Thomson, 2010; Liu et al., 2021).
Responses of Rs to decreasing precipitation have been studied in various ecosystems, but considerable uncertainty remains about the directions and magnitude of the response (Hinko-Najera et al., 2015; Sotta et al., 2007; van Straaten et al., 2010; Wei et al., 2016). Precipitation reduction was reported to suppress Rs in arid and semiarid ecosystems (Talmon et al., 2011) but enhance it in tropical rainforests (Cleveland et al., 2010; Zhang et al., 2015). These contradictory results have been attributed to differences in vegetation types, climatic conditions, and soil microbial activities (Li et al., 2020; Liu et al., 2016). Rs is comprised of autotrophic respiration (Ra), produced by the activities of vegetation roots and associated organisms, and heterotrophic respiration (Rh), associated with decomposition of soil organic matter (SOM) through soil biota (Luo and Zhou, 2006). These two components represent different biological and ecological processes and respond differently to changes in environmental factors including soil temperature (Ts) and soil moisture (Ms) (Liu et al., 2016). For example, reduced precipitation strongly suppressed Ra, but had no effect on Rh in a temperate broadleaved evergreen eucalypt forest (Hinko-Najera et al., 2015). Therefore, identifying differences between Ra and Rh and the factors that control them in different precipitation treatments could help to reduce some of uncertainties associated with climate-carbon feedback forecasts (Liu et al., 2016; Huang et al., 2018; Zhang et al., 2015). However, very few studies have combined manipulating precipitation with partitioning Rs into its component fluxes in semiarid shrublands.
Shrublands are a widely distributed biome type in China (Piao et al., 2009), covering approximately 1.23 million km2 of China (Yang et al., 2016). The arid and semi-arid shrublands are important land-cover types in northern China, which are affected by increasing temperatures and changing precipitation patterns (Jia et al., 2016). Moreover, the region of arid and semi-arid shrublands is projected to be characterized by large fluctuations in precipitation and frequent drought periods under future climate change (Liu et al., 2012). Thus, it is important to better understand the response of soil respiration components to changing precipitation by using an artificial precipitation manipulation experiment. In the present study, we established precipitation shelters in mountain shrublands of northern China to identify: 1) the impacts of precipitation exclusion on the different soil respiration component rates and their temperature sensitivity values, and 2) the relation between soil respiration components and soil temperature/moisture.
This research was carried out at the Hilly Ecosystem Experimental Station of Taihang Mountain, Chinese Academy of Science (114°15′50″ E, 37°52′44″ N, 350 m a.s.l.) in the Hebei Province of northern China. This region has a semi-arid continental climate with an annual mean atmospheric temperature of 13°C. The lowest average temperature in January is −4°C, and the highest average air temperature in July is 26°C (Zeng et al., 2014). Annual mean precipitation is about 560 mm concentrated from June to September (Shen et al., 2014). Monthly accumulated precipitation and average air temperature during the experimental period are shown in Figure 1. The soil is categorized as Cinnamon soil under the Chinese soil taxonomy, which is equal to Ustalf in the USDA Soil Taxonomy (Zeng et al., 2014). The most abundant shrub is Vitex negundo var. heterophylla, which forms a relatively closed canopy (Shen et al., 2014).
The experiment was carried out in June 2015. Based on a randomized block design, three replicate treatments were used to create precipitation gradients, including natural conditions/ambient precipitation (CK), reduced precipitation by 30% (PE30), and reduced precipitation by 60% (PE60). This design resulted in a total of nine plots. Each experimental plot of 10 m × 10 m was established with 10 m spacing between plots. All measurements were carried out at the center (8 m × 8 m) to avoid edge effects. In PE30 and PE60 treatments, precipitation was reduced by 30 and 60%, respectively, by using plastic rainout shelters as described by Sherman et al. (2012). Although the shelters minimally intercepted incoming light, past work has shown that the interception has little effect on plant responses (Zhang et al., 2017).
A mini-trenching method was adopted to measure Rh in the subplots. In each plot, three trenches were excavated (0.5 m high and 0.4 m in diameter) and then the roots were removed. Subsequently, the soil was put into nylon mesh bags (mesh size of 0.038 mm) to avoid roots expanding into the subplots and to allow the movement of soil organic nutrients, microbes, and soil water (Zhang et al., 2014). Finally, the soil was placed back into the trenches. Vegetation was restricted from the area inside each trench by manually cutting plant growth throughout the study period.
In each plot, three PVC collars (inner diameter of 10.4 cm and height of 8 cm) were installed in the soil at 5 cm depth to monitor Rs in the untrenched area. In addition, another three PVC collars were inserted at the center of the trenching areas to measure Rh. In order to remove the impacts of soil collar installation on Rs or Rh measurements, the collars were installed one year before measurements were collected (July 2015). Moreover, living vegetation inside the PVC collars was removed before monitoring (Fang et al., 2018). Rs and Rh were monitored on clear days once every month between August 2016 and July 2017 using a LI-COR 8100 infrared gas analyzer. All measurements of Rs and Rh were carried out from 8:00 to 11:00 a.m. Ra was calculated by subtracting Rh from Rs (Sun et al., 2019). The soil temperature and humidity sensors equipped with the LI-COR 8100 system were used to record Ts and Ms at a soil depth of 10 cm (Shen et al., 2014).
An exponential model was used to calculate the relationship between monthly mean Rs, Ra, and Rh and monthly mean Ts (°C) from three replicates (Sun et al., 2019):
where R stands for Rs, Ra, or Rh (μmol m−2 s−1); Ts is the soil temperature (°C); a and b are regression parameters; Q10 is the temperature sensitivity of different soil respiration components.
A polynomial function (Sun et al., 2019) was established to analyze the variation between monthly mean Rs, Ra, or Rh and monthly mean Ms (%) as follows:
where R stands Rs, Ra, or Rh (μmol m−2 s−1); c, d, and e are functional parameters; Ms is the soil moisture (%).
To consider the combined impacts of Ts and Ms on soil respiration components, we also fitted soil respiration components using a two-factor regression model (Zhang et al., 2015) as follows:
Where R represents Rs, Ra, or Rh (μmol m−2 s−1); f, g, and h are regression parameters; Ts and Ms are the soil temperature (°C) and soil moisture (%) at 10 cm depth, respectively.
According to the method described by Shen et al. (2014), Rs and Rh measurements between respective sampling dates were interpolated and summed to estimate the annual cumulative fluxes for different treatments.
Repeated measures ANOVA analysis was used to examine the significant differences in mean Ts, Ms, Rs, Ra, Rh and Rh/Rs between the CK and treatment plots for various periods. ANOVA was also performed to test the effect of precipitation treatment on the mean soil respiration components, environmental factors, and cumulative soil respiration component fluxes. All analyses were performed using SPSS 13.0 software (SPSS for Windows, Chicago, IL). Significant differences were indicated at the level of p < 0.05. Exponential model 1) and polynomial model 2) and their corresponding coefficients were performed using Sigmplot 12 (Systat Software Inc., CA, United States of America); non-linear regression model 3) and its regression parameters were performed using R 4.1.0 for Windows (https://www.r-project.org).
Soil temperature varied seasonally, from the lowest of −2.8°C in January 2017 to the highest of 26.9°C in July 2017 (Figure 2A). Mean monthly Ts depth for CK, PE30 and PE60 were 14.1, 13.9 and 13.8°C, respectively, and there was not a significant difference among the three treatments throughout the study period (Figure 2B).
FIGURE 2. Seasonal variation in (A) soil temperature (Ts) at 10 cm depth, and (C) soil moisture (Ms) at 10 cm depth from August 2016 to July 2017. Data are the mean ± SD (n = 3). Monthly mean values of (B)Ts and (D)Ms throughout the study period. Letters on the top of the bars indicate significant difference among treatments at level of p < 0.05. CK: ambient precipitation; PE30: 30% reduced precipitation; PE60: 60% reduced precipitation.
Soil moisture in the three precipitation gradients displayed similar seasonal variation (Figure 2C). On average, Ms was 10.2% in CK and decreased by 5.0 and 17.8% in PE30 and PE60, respectively (Figure 2D). In addition, soil in PE60 was significantly drier than the soil in the control (p < 0.05).
During the study period, Rs, Rh, and Ra showed similar seasonal variation to Ts and Ms, with maximum values occurring during summer (July) and minimum values occurring in the winter (Figure 3A,C,E). However, the ratio of Rh/Rs showed an opposite pattern with the lowest value (approximately 60%) during the peak season (Figure 3G). The average Rs was 1.48 ± 0.14 μmol m−2 s−1 in CK, and was reduced by 8.5 and 18.8% in PE30 and PE60, respectively; the reduction in Rs was significant for PE60 (p < 0.05) (Figure 3B). The average Rh was 1.07 ± 0.09, 0.98 ± 0.08, and 0.88 ± 0.04 μmol m−2 s−1 in CK, PE30, and PE60, respectively. The mean Ra was 0.41 ± 0.08 μmol m−2 s−1 in CK, with a reduction of 7.5% in PE30 and 19.9% in PE60, respectively (Figure 3D,F). The one-way ANOVA showed that the average Rh was significantly higher in CK than in PE60 (Figure 3D). In contrast, Ra was not significantly altered by changing precipitation (Figure 3F). In addition, precipitation exclusion did not significantly alter the ratio of Rh/Rs (Figure 3H). Rh was significantly and positively correlated to Rs under the three precipitation manipulation treatments (Figure 4). The model implied that Rh approached zero with Rs, which made sense biologically as Rh occurred in the soil when Rs > 0 (Bond-Lamberty et al., 2004). Annually, reducing precipitation significantly decreased the cumulative Rs by 47.8 g C m−2 in PE30 and 106.0 g C m−2 in PE60, respectively (Figure 5). Moreover, the contribution of Rh to Rs was altered by different precipitation treatments, with a larger Rh/Rs ratio in CK than in PE30 and PE60.
FIGURE 3. Seasonal variations in (A) total soil respiration (Rs) and its (C) heterotrophic (Rh) and (E) autotrophic (Ra) components and (G) the ratio of Rh/Rs (%) from August 2016 to July 2017. Data are the mean ± SD (n = 3). Monthly mean values of (B)Rs, (D)Rh, (F)Ra and (H) ratio of Rh/Rs (%) throughout the study period. Letters on the top of the bars indicate significant difference among treatments at level of p < 0.05. CK: ambient precipitation; PE30: 30% reduced precipitation; PE60: 60% reduced precipitation.
FIGURE 4. Relationship between soil respiration (Rs) and its heterotrophic (Rh) component during the study period. (A) CK: ambient precipitation; (B) PE30: 30% reduced precipitation; (C) PE60: 60% reduced precipitation.
FIGURE 5. The ratio of heterotrophic respiration (Rh) and autotrophic respiration (Ra) to cumulative soil respiration components fluxes (g C m−2) from August 2016 to July 2017. Letters on the top of the bars indicate significant difference among treatments at level of p < 0.05. CK: ambient precipitation; PE30: 30% reduced precipitation; PE60: 60% reduced precipitation.
Both Rs and its Rh and Ra components increased exponentially with Ts (p < 0.01) for the three precipitation treatments (Table 1). Ts interpreted 68.2–78.9% of the variations in Rs, Rh, and Ra (Table 1). The Q10 values of Rs, Rh, and Ra varied from 3.35 to 4.57, 2.12 to 2.20, and 3.60 to 4.35, respectively. Moreover, the Q10 of Rs and Ra both significantly increased with reduced precipitation, while the Q10 of Rh did not. A significant quadratic relationship was observed between soil respiration components and Ms (p < 0.01) measured at 10 cm depth (Table 2). Ms explained 76.1–78.6% of the variation in Rs, 71.8–74.5% in Rh, and 76.9–81.6% in Ra, respectively (Table 2); thus, Ms was a better predictor of soil respiration components in the three precipitation treatments. Moreover, simultaneously considering both Ts and Ms explained 56.6–77.9% of the variation in soil respiration components (p < 0.01 or p < 0.05) (Table 3), indicating that the inclusion of Ts did not improve the explanation of soil respiration components compared to the model based on Ms only.
TABLE 1. Impacts of soil temperature (Ts, °C) on the variation of different soil respiration components (R, μmol m−2 s−1). R2, p, and Q10 values are reported.
TABLE 2. Impacts of soil moisture (Ms, %) on the variation of different soil respiration components (R, μmol m−2 s−1). R2 and p values are reported.
TABLE 3. Combined impacts of soil temperature (Ts, °C) and soil moisture (Ms, %) on the variation of different soil respiration components (R, μmol m−2 s−1). R2 and p values are reported.
The average Rs in this study ranged from 1.20 ± 0.09 μmol m−2 s−1 to 1.48 ± 0.14 μmol m−2 s−1, which was in the range of values reported for other shrubland ecosystems (de Dato et al., 2010; Shi et al., 2020; Talmon et al., 2011). The relative contribution of Rh to Rs was 72.6, 72.3 and 71.9% in CK, PE30 and PE60, respectively. These were consistent with the values reported by Cheng et al. (2015) and Huang et al. (2018), but were higher than those reported in other ecosystems (Comstedt et al., 2011; Huang et al., 2016; Saiz et al., 2005). The trenching method has been widely utilized to distinguish Rh from Rs in many ecosystems (Hanson et al., 2000; Kukumägi et al., 2017; Liu et al., 2016). Nevertheless, it should be noted that a long period of time (i.e., more than 6 months) might be needed to completely remove the influence of dead roots on Rh (Xu et al., 2015; Lei et al., 2017). In order to eliminate the impacts of dead root decomposition on Rh measurements, we inserted collars into the trenches almost one year before the measurement of soil respiration components. However, the trenching method may lead to low estimates of Rh due to removal of inputs from root exudates and dead roots (Yi et al., 2007; Fang et al., 2018).
Precipitation can affect Rs and its components by changing soil humidity, which directly influences the substrates for heterotrophic respiration as well as the autotrophic respiration of roots and microorganisms (Wang et al., 2014b; Liu et al., 2018; Sun et al., 2019). Consistent with several previous works (Balogh et al., 2016; Borken et al., 2006; Suseela et al., 2012), a decrease in Rs and its Rh and Ra components was also observed under the precipitation exclusion treatments in the present study, and this response can be explained by a number of abiotic and biotic mechanisms. First, reductions in Rh, and hence Rs, were possibly caused by lower soil moisture due to decreased precipitation (Yang et al., 2020). Reduced precipitation might inhibit Rh and Rs by impeding the diffusion of unstable substrates, and thus decreasing the rates of soluble substrates absorption by microorganisms (Yan et al., 2011). Second, lower fine root growth caused by lower soil moisture levels might also explain the effect of decreased precipitation on Rs and its components (Hinko-Najera et al., 2015). Third, lower soil moisture in decreased precipitation treatments might increase the amount of CO2 that accumulates in soil pores (Liu et al., 2019).
However, many other studies have reported varying responses of soil respiration to reduced precipitation (Deng et al., 2018; Zhang et al., 2015). Davidson et al. (2008) found no effect of reduced precipitation on soil respiration, indicating that belowground carbon allocation may not have been significantly impacted by reduced precipitation. In addition, Cleveland et al. (2010) and Zhang et al. (2015) reported that reducing precipitation increased soil respiration in tropical rainforest experiments because the soils responded to the increase of dissolved organic matter concentrations or soil O2 availability. The reasons for these inconsistent results may be due to the fact that they were conducted in diverse ecosystems and measurements were conducted on different temporal scales (Wang et al., 2014b). Therefore, it is necessary to quantitatively assess the changes of Rs and its components under different intensities and frequencies of precipitation exclusion in different ecosystems.
Soil temperature exhibited seasonal variation, which primarily accounted for the temporal variation of soil respiration components (Fang et al., 2018). In this study, both Rs and its components rose exponentially with the increase of Ts in the three precipitation treatments, consistent with previous findings from shrublands (Lellei-Kovács et al., 2016; Sun et al., 2021) and other ecosystems (Rey et al., 2002; Zhang et al., 2015). Mechanisms underlying the response of soil respiration components to changes in Ts may include the availability of nutrients and substrates, the adaptation of roots to different soil environments, and the alteration of the microbial community (Wei et al., 2016). In addition to Ts, Ms has also been considered an important variable that controls the variation of Rs and its components (Saiz et al., 2005; Sun et al., 2019). Our results provided evidence that the precipitation manipulation treatments significantly decreased Ms and had a much stronger effect on both Rs and its components. Ms might limit soil respiration components by stressing the distribution of assimilates in the plant-soil system, microorganisms, and enzymatic activities in the rhizosphere (Escolar et al., 2015; Sanaullah et al., 2011). According to the Ms-based quadratic function, both Rs and its components may become depressed when Ms becomes either too high or too low (Liu et al., 2018). We simulated the soil respiration components with a two-factor model (Eq. 3) (Table 3) which turned out to be weaker than the Ms-based model, suggesting that precipitation exclusion amplified the effects of soil water limitation on soil respiration (Sun et al., 2019).
Q10 is recognized as an important parameter to evaluate temperature adaptation of Rs (Luo and Zhou, 2006; Fang et al., 2018). In our work, the values of Q10 ranged from 2.12 to 4.57, which was consistent with the range (0.65–5.18) of other ecosystems (Rey et al., 2002; Zou et al., 2018; Sun et al., 2021). Previous studies have suggested that drought might change the sensitivity of Rs to temperature and disrupt the coupling between temperature and humidity (Selsted et al., 2012; Wang et al., 2014b). Soil-water deficit adequately weakened the sensitivity of Rs to Ts, leading to the decrease of Q10 (Rey et al., 2002; Wang et al., 2014b; Liu et al., 2016). In contrast, our study found that reducing precipitation increased the Q10 values of different soil respiration components, which was consistent with previous results in a grassland ecosystem (Sun et al., 2019). The Q10 values of Ra were higher than those of Rh, reflecting a tighter relationship between Q10 and plant root activities (Sun et al., 2019; Zou et al., 2018). In addition, the results also suggested that Rh was less sensitive than Ra to the precipitation exclusion treatments, indicating that drought might have a weaker feedback mechanism of SOM decomposition on climate change (Sun et al., 2018).
The present study provided unique data for exploring the impacts of precipitation exclusion on Rs and its components in a semiarid mountain shrubland of northern China. Precipitation exclusion significantly depressed Rs and its Rh and Ra components. Rs and its components were all exponentially related with Ts and quadratically related with Ms. The temperature sensitivity (Q10) of Rs and Ra were both significantly increased by decreased precipitation. In addition, decreasing the intensity of precipitation decreased the contribution of Rh to Rs. We estimated an annual C reduction release of 47.8 and 106.0 g C m−2 in response to treatments that decreased precipitation by 30 and 60%, respectively. Our findings are critical for understanding and forecasting possible changes in the release of carbon by semiarid shrublands in response to climate change. Further work with long-term experiments is necessary to evaluate the influence of precipitation manipulation treatments on soil respiration components and how the responses may vary along under future drought events.
The raw data supporting the conclusions of this article will be made available by the authors, without undue reservation.
HS was involved with experiment plan, data collection and analysis, manuscript formation and manuscript editing. LZ, HM, and TZ collected the data. ZZ and YZ edited the manuscript.
The present study was funded by the Natural Science Foundation of Hebei Province (Grant No. C2019302018), the Natural Science Foundation of China (Grant No. 31500448), the Youth Talent Project of Hebei Province, and the Talent Training Program of Hebei Academy of Sciences (Grant No. 2020G16).
The authors declare that the research was conducted in the absence of any commercial or financial relationships that could be construed as a potential conflict of interest.
The authors would like to thank Elizabeth Tokarz at Yale University for her assistance with polishing up the manuscript.
Ahlström, A., Raupach, M. R., Schurgers, G., Smith, B., Arneth, A., Jung, M., et al. (2015). The Dominant Role of Semi-arid Ecosystems in the Trend and Variability of the Land CO2 Sink. Science 348 (6237), 895–899. doi:10.1126/science.aaa1668
Balogh, J., Papp, M., Pintér, K., Fóti, S., Posta, K., Eugster, W., et al. (2016). Autotrophic Component of Soil Respiration Is Repressed by Drought More Than the Heterotrophic One in Dry Grasslands. Biogeosciences 13, 5171–5182. doi:10.5194/bg-13-5171-2016
Bond-Lamberty, B., and Thomson, A. (2010). Temperature-associated Increases in the Global Soil Respiration Record. Nature 464 (7288), 579–582. doi:10.1038/nature08930
Bond-Lamberty, B., Wang, C. K., and Gower, S. T. (2004). A Global Relationship between the Heterotrophic and Autotrophic Components of Soil Respiration?. Glob. Chang. Biol. 10, 1756–1766. doi:10.1111/j.1365-2486.2004.00816.x
Borken, W., Savage, K., Davidson, E. A., and Trumbore, S. E. (2006). Effects of Experimental Drought on Soil Respiration and Radiocarbon Efflux from a Temperate forest Soil. Glob. Chang. Biol. 12, 177–193. doi:10.1111/j.1365-2486.2005.001058.x
Cheng, X. Q., Kang, F. F., Han, H. R., Liu, H. W., and Zhang, Y. L. (2015). Effect of Thinning on Partitioned Soil Respiration in a Young Pinus Tabulaeformis Plantation during Growing Season. Agric. For. Meteorol. 214-215, 473–482. doi:10.1016/j.agrformet.2015.09.016
Cleveland, C. C., Wieder, W. R., Reed, S. C., and Townsend, A. R. (2010). Experimental Drought in a Tropical Rain forest Increases Soil Carbon Dioxide Losses to the Atmosphere. Ecology 91, 2313–2323. doi:10.1890/09-1582.1
Comstedt, D., Boström, B., and Ekblad, A. (2011). Autotrophic and Heterotrophic Soil Respiration in a Norway spruce forest: Estimating the Root Decomposition and Soil Moisture Effects in a Trenching experiment. Biogeochemistry 104, 121–132. doi:10.1007/s10533-010-9491-9
Davidson, E. A., Nepstad, D. C., Ishida, F. Y., and Brando, P. M. (2008). Effects of an Experimental Drought and Recovery on Soil Emissions of Carbon Dioxide, Methane, Nitrous Oxide, and Nitric Oxide in a Moist Tropical forest. Glob. Chang. Biol. 14, 2582–2590. doi:10.1111/j.1365-2486.2008.01694.x
de Dato, G. D., De Angelis, P., Sirca, C., and Beier, C. (2010). Impact of Drought and Increasing Temperatures on Soil CO2 Emissions in a Mediterranean Shrubland (Gariga). Plant Soil 327, 153–166. doi:10.1007/s11104-009-0041-y
Deng, Q., Zhang, D. Q., Han, X., Chu, G. W., Zhang, Q. F., and Hui, D. F. (2018). Changing Rainfall Frequency rather Than Drought Rapidly Alters Annual Soil Respiration in a Tropical forest. Soil Biol. Biochem. 121, 8–15. doi:10.1016/j.soilbio.2018.02.023
Escolar, C., Maestre, F. T., and Rey, A. (2015). Biocrusts Modulate Warming and Rainfall Exclusion Effects on Soil Respiration in a Semi-arid Grassland. Soil Biol. Biochem. 80, 9–17. doi:10.1016/j.soilbio.2014.09.019
Fang, C., Li, F. M., Pei, J. Y., Ren, J., Gong, Y. H., Yuan, Z. Q., et al. (2018). Impacts of Warming and Nitrogen Addition on Soil Autotrophic and Heterotrophic Respiration in a Semi-arid Environment. Agric. For. Meteorol. 248, 449–457. doi:10.1016/j.agrformet.2017.10.032
Hanson, P. J., Edwards, N. T., Garten, C. T., and Andrews, J. A. (2000). Separating Root and Soil Microbial Contributions to Soil Respiration: a Review of Methods and Observations. Biogeochemistry 48 (1), 115–146. doi:10.1023/A:1006244819642
Hinko-Najera, N., Fest, B., Liversley, S. J., and Arndt, S. K. (2015). Reduced Throughfall Decreases Autotrophic Respiration, but Not Heterotrophic Respiration in a Dry Temperate Broadleaved evergreen forest. Agric. For. Meteorol. 200, 66–77. doi:10.1016/j.agrformet.2014.09.013
Huang, W. J., Han, T. F., Liu, J. X., Wang, G. S., and Zhou, G. Y. (2016). Changes in Soil Respiration Components and Their Specific Respiration along Three Successional Forests in the Subtropics. Funct. Ecol. 30 (8), 1466–1474. doi:10.1111/1365-2435.12624
Huang, S. D., Ye, G. F., Lin, J., Chen, K. T., Xu, X., Ruan, H. H., et al. (2018). Autotrophic and Heterotrophic Soil Respiration Responds Asymmetrically to Drought in a Subtropical forest in the Southeast China. Soil Biol. Biochem. 123, 242–249. doi:10.1016/j.soilbio.2018.04.029
IPCC (2013). The Physical Science Basis. Contribution of Working Group I to the Fifth Assessment Report of the Intergovernmental Panel on Climate Change. Cambridge: Cambridge University Press.
Jia, X., Zha, T. S., Gong, J. N., Wu, B., Zhang, Y. Q., Qin, S. G., et al. (2016). Energy Partitioning over a Shrubland in Northern China. Hydrol. Process. 30 (6), 972–985. doi:10.1002/hyp.10685
Kukumägi, M., Ostonen, I., Uri, V., Helmisaari, H., Kanal, A., Kull, O., et al. (2017). Variation of Soil Respiration and its Components in Hemiboreal Norway spruce Stands of Different Ages. Plant Soil 414, 265–280. doi:10.1007/s11104-016-3133-5
Lei, L., Xiao, W. F., Zeng, L. X., Zhu, J. H., Huang, Z. L., Cheng, R. M., et al. (2017). Thinning but Not Understory Removal Increased Heterotrophic Respiration and Total Soil Respiration in Pinus Massoniana Stands. Sci. Total Environ. 621, 1360–1369. doi:10.1016/j.scitotenv.2017.10.092
Lellei-Kovács, E., Botta-Dukát, Z., de Dato, G., Estiarte, M., Guidolotti, G., Kopittke, G. R., et al. (2016). Temperature Dependence of Soil Respiration Modulated by Thresholds in Soil Water Availability across European Shrubland Ecosystems. Ecosystems 19, 1460–1477. doi:10.1007/s10021-016-0016-9
Li, Y., Zhou, Z. X., Lei, L. J., Ru, J. Y., Song, J., Zhou, M. X., et al. (2020). Asymmetric Responses of Soil Respiration in Three Temperate Steppes along a Precipitation Gradient in Northern China Revealed by Soil-Monolith Transplanting experiment. Agric. For. Meteorol. 294, 108126. doi:10.1016/j.agrformet.2020.108126
Liu, R., Pan, L. P., Jenerette, G. D., Wang, Q. X., Cieraad, E., and Li, Y. (2012). High Efficiency in Water Use and Carbon Gain in a Wet Year for a Desert Halophyte Community. Agric. For. Meteorol. 162-163, 127–135. doi:10.1016/j.agrformet.2012.04.015
Liu, Y. C., Liu, S. R., Wan, S. Q., Wang, J. X., Luan, J. W., and Wang, H. (2016). Differential Responses of Soil Respiration to Soil Warming and Experimental Throughfall Reduction in a Transitional oak forest in central China. Agric. For. Meteorol. 226-227, 186–198. doi:10.1016/j.agrformet.2016.06.003
Liu, Y. T., Li, J., Jin, Y. Q., Zhang, Y. P., Sha, L. Q., Grace, J., et al. (2018). The Influence of Drought Strength on Soil Respiration in a Woody savanna Ecosystem, Southwest China. Plant Soil. 428, 321–333. doi:10.1007/s11104-018-3678-6
Liu, Y. C., Zhao, C. C., Shang, Q., Su, L., and Wang, L. (2019). Responses of Soil Respiration to spring Drought and Precipitation Pulse in a Temperate oak forest. Agric. For. Meteorol. 268, 289–298. doi:10.1016/j.agrformet.2019.01.029
Liu, Y. Z., Zhao, C. C., Guo, J. W., Zhang, L. N., Xuan, J., Chen, A. Q., et al. (2021). Short-term Phosphorus Addition Augments the Effects of Nitrogen Addition on Soil Respiration in a Typical Steppe. Sci. Total Environ. 761, 143211. doi:10.1016/j.scitotenv.2020.143211
Luo, Y. Q., and Zhou, X. H. (2006). Soil Respiration and the Environment. New York: Academic Press. doi:10.1016/B978-012088782-8/50012-7
Piao, S. L., Fang, J. Y., Ciais, P., Peylin, P., Huang, Y., Sitch, S., et al. (2009). The Carbon Balance of Terrestrial Ecosystems in China. Nature 458, 1009–1013. doi:10.1038/nature07944
Rey, A., Pegoraro, E., Tedeschi, V., Parri, I. D., Jarvis, P. G., and Valentini, R. (2002). Annual Variation in Soil Respiration and its Components in a Coppice oak forest in Central Italy. Glob. Chang. Biol. 8 (9), 851–866. doi:10.1046/j.1365-2486.2002.00521.x
Saiz, G., Byrne, K. A., Butterbach-bahl, K., Kiese, R., Blujdea, V., and Farrell, E. P. (2005). Stand Age-Related Effects on Soil Respiration in a First Rotation Sitka Spruce Chronosequence in Central Ireland. Glob. Chang. Biol. 12 (6), 1007–1020. doi:10.1111/j.1365-2486.2006.01145.x
Sanaullah, M., Blagodatskaya, E., Chabbi, A., Rumpel, C., and Kuzyakov, Y. (2011). Drought Effects on Microbial Biomass and Enzyme Activities in the Rhizosphere of Grasses Depend on Plant Community Composition. Appl. Soil Ecol. 48, 38–44. doi:10.1016/j.apsoil.2011.02.004
Schlesinger, W. H., and Andrews, J. A. (2000). Soil Respiration and the Global Carbon Cycle. Biogeochemistry 48, 7–20. doi:10.1023/A:1006247623877
Selsted, M. B., Linden, L., Ibrom, A., Michelsen, A., Larsen, K. S., Pedersen, J. K., et al. (2012). Soil Respiration Is Stimulated by Elevated CO2 and Reduced by Summer Drought: Three Years of Measurements in a Multifactor Ecosystem Manipulation experiment in a Temperate Heathland (CLIMAITE). Glob. Chang. Biol. 18, 1216–1230. doi:10.1111/j.1365-2486.2011.02634.x
Shen, H. T., Cao, J. S., Zhang, W. J., Zeng, X. H., and Wang, H. R. (2014). Winter Soil CO2 Flux from Different Mid-latitude Sites from Middle Taihang Mountain in North China. PLoS ONE. 9 (3), e91589. doi:10.1371/journal.pone.0091589
Sherman, C., Sternberg, M., and Sternberger, Y. (2012). Effect of climate change on soil respiration and carbon processing in Mediterranean and semi-arid regions: An experimental approach. Eur. J. Soil Boil. 52, 48–58. doi:10.1016/j.ejsobi.2012.06.001
Shi, P., Qin, Y. L., Liu, Q., Zhu, T. T., Li, Z. B., Li, P., et al. (2020). Soil Respiration and Response of Carbon Source Changes to Vegetation Restoration in the Loess Plateau. China. Sci. Total Environ. 707, 135507. doi:10.1016/j.scitotenv.2019.135507
Sotta, E. D., Veldkamp, E., Schwendenmann, L., Guimaraes, B. R., Paixao, R. K., Ruivo, M., et al. (2007). Effects of an Induced Drought on Soil Carbon Dioxide (CO2) Efflux and Soil CO2 Production in an Eastern Amazonian Rainforest, Brazil. Glob. Chang. Biol. 13, 2218–2229. doi:10.1111/j.1365-2486.2007.01416.x
Sun, S. Q., Lei, H. Q., and Chang, S. X. (2019). Drought Differentially Affects Autotrophic and Heterotrophic Soil Respiration Rates and Their Temperature Sensitivity. Biol. Fertil. Soils. 55, 275–283. doi:10.1007/s00374-019-01347-w
Sun, W. H., Zhao, X. N., Gao, X. D., Shi, W. Y., Ling, Q., Siddique, K. H. M., et al. (2021). Impacts of Land Use Conversion on the Response of Soil Respiration to Precipitation in Drylands: A Case Study with Four-Yearlong Observations. Agric. For. Meteorol. 304-305, 108426. doi:10.1016/j.agrformet.2021.108426
Suseela, V., Conant, R. T., Wallenstein, M. D., and Dukes, J. S. (2012). Effects of Soil Moisture on the Temperature Sensitivity of Heterotrophic Respiration Vary Seasonally in an Old-Field Climate Change experiment. Glob. Chang. Biol. 18, 336–348. doi:10.1111/j.1365-2486.2011.02516.x
Talmon, Y., Sternberg, M., and Grünzweig, J. M. (2011). Impact of Rainfall Manipulations and Biotic Controls on Soil Respiration in Mediterranean and Desert Ecosystems along an Aridity Gradient. Glob. Chang. Biol. 17 (2), 1108–1118. doi:10.1111/j.1365-2486.2010.02285.x
van der Molen, M. K., Dolman, A. J., Ciais, P., Eglin, T., Gobron, N., Law, B. E., et al. (2011). Drought and Ecosystem Carbon Cycling. Agric. For. Meteorol. 151 (7), 765–773. doi:10.1016/j.agrformet.2011.01.018
van Straaten, O., Veldkamp, E., Kohler, M., and Anas, I. (2010). Spatial and Temporal Effects of Drought on Soil CO2 Efflux in a Cacao Agroforestry System in Sulawesi, Indonesia. Biogeosciences 7, 1223–1235. doi:10.5194/bg-7-1223-2010
Wang, Y. F., Hao, Y. B., Cui, X. Y., Zhao, H. T., Xu, C. Y., Zhou, X. Q., et al. (2014b). Responses of Soil Respiration and its Components to Drought Stress. J. Soils Sediments 14, 99–109. doi:10.1007/s11368-013-0799-7
Wei, X. R., Zhang, Y. J., Liu, J., Gao, H. L., Fan, J., Jia, X. X., et al. (2016). Response of Soil CO 2 Efflux to Precipitation Manipulation in a Semiarid Grassland. J. Environ. Sci. 45, 207–214. doi:10.1016/j.jes.2016.01.008
Xu, X., Shi, Z., Li, D. J., Zhou, X. H., Sherry, R. A., and Luo, Y. Q. (2015). Plant Community Structure Regulates Responses of Prairie Soil Respiration to Decadal Experimental Warming. Glob. Chang. Biol. 21, 3846–3853. doi:10.1111/gcb.12940
Yan, L., Chen, S., Huang, J., and Lin, G. (2011). Water Regulated Effects of Photosynthetic Substrate Supply on Soil Respiration in a Semiarid Steppe. Glob. Chang. Biol. 17, 1990–2001. doi:10.1111/j.1365-2486.2010.02365.x
Yang, X., Chi, X. L., Ji, C. J., Liu, H. Y., Ma, W. H., Mohhammat, A., et al. (2016). Variations of Leaf N and P Concentrations in Shrubland Biomes across Northern China: Phylogeny, Climate, and Soil. Biogeosciences 13, 4429–4438. doi:10.5194/bg-13-4429-2016
Yang, Z. L., Wei, Y. Y., Fu, G. Y., Song, H. Q., Li, G. Y., and Xiao, R. (2020). Asymmetric Effect of Increased and Decreased Precipitation in Different Periods on Soil and Heterotrophic Respiration in a Semiarid Grassland. Agric. For. Meteorol. 291, 108039. doi:10.1016/j.agrformet.2020.108039
Yi, Z. G., Fu, S. L., Yi, W. M., Zhou, G. Y., Mo, J. M., Zhang, D. Q., et al. (2007). Partitioning Soil Respiration of Subtropical Forests with Different Successional Stages in South China. For. Ecol. Manag. 243 (2-3), 178–186. doi:10.1016/j.foreco.2007.02.022
Zeng, X. H., Zhang, W. J., Shen, H. T., Cao, J. S., and Zhao, X. (2014). Soil Respiration Response in Different Vegetation Types at Mount Taihang, China. Catena 116, 78–85. doi:10.1016/j.catena.2013.12.018
Zhang, C., Niu, D., Hall, S. J., Wen, H., Li, X., Fu, H., et al. (2014). Effects of Simulated Nitrogen Deposition on Soil Respiration Components and Their Temperature Sensitivities in a Semiarid Grassland. Soil Biol. Biochem. 75, 113–123. doi:10.1016/j.soilbio.2014.04.013
Zhang, X., Zhang, Y. P., Sha, L. Q., Wu, C. S., Tan, Z. H., Song, Q. H., et al. (2015). Effects of continuous draught stress on soil respiration in a tropical rainforest in southwest China. Plant Soil 394, 343–353. doi:10.1007/s11104-015-2523-4
Zhang, B., Tan, X. R., Wang, S. S., Chen, M. L., Chen, S. P., Ren, T. T., et al. (2017). Asymmetric Sensitivity of Ecosystem Carbon and Water Processes in Response to Precipitation Change in a Semiarid Steppe. Funct. Ecol. 31 (6), 1301–1311. doi:10.1111/1365-2435.12836
Keywords: climate change, precipitation exclusion, soil respiration, soil moisture, soil temperature
Citation: Shen H, Zhang L, Meng H, Zheng Z, Zhao Y and Zhang T (2021) Response of Soil Respiration and Its Components to Precipitation Exclusion in Vitex negundo Var. Heterophylla Shrubland of the Middle Taihang Mountain in North China. Front. Environ. Sci. 9:712301. doi: 10.3389/fenvs.2021.712301
Received: 20 May 2021; Accepted: 12 July 2021;
Published: 22 July 2021.
Edited by:
Xiao Jun Allen Liu, University of Oklahoma, United StatesReviewed by:
Dafeng Hui, Tennessee State University, United StatesCopyright © 2021 Shen, Zhang, Meng, Zheng, Zhao and Zhang. This is an open-access article distributed under the terms of the Creative Commons Attribution License (CC BY). The use, distribution or reproduction in other forums is permitted, provided the original author(s) and the copyright owner(s) are credited and that the original publication in this journal is cited, in accordance with accepted academic practice. No use, distribution or reproduction is permitted which does not comply with these terms.
*Correspondence: Zhenhua Zheng, enpoNjcxMUBzaW5hLmNvbQ==; Yanxia Zhao, emh5eDg2OThAMTYzLmNvbQ==
Disclaimer: All claims expressed in this article are solely those of the authors and do not necessarily represent those of their affiliated organizations, or those of the publisher, the editors and the reviewers. Any product that may be evaluated in this article or claim that may be made by its manufacturer is not guaranteed or endorsed by the publisher.
Research integrity at Frontiers
Learn more about the work of our research integrity team to safeguard the quality of each article we publish.