- 1Key Laboratory of Genetics and Germplasm Innovation of Tropical Special Forest Trees and Ornamental Plants (Hainan University), Ministry of Education, College of Forestry, Hainan University, Haikou, China
- 2Sanya Emergency Management Bureau, Sanya, China
- 3Sanya Huasheng Tianya Cement Co., Ltd, Sanya, China
- 4Guangzhou Shimen National Forest Park Management Office, Guangzhou, China
- 5Key Laboratory of Vegetation Restoration and Management of Degraded Ecosystem, South China Botanical Garden, Chinese Academy of Sciences, Guangzhou, China
Reforestation is an effective way to alleviate deforestation and its negative impacts on ecosystem services. In tropical rainforest ecosystem, however, frequent typhoons and heavy rainfall can result in landslides and uprooting of many seedlings, making reforestation efforts very difficult, especially within extremely degraded sites where soil conditions cannot support any plant life. Here, we described a reforestation protocol which is based on tropical rainforest successional processes to not only prevent landslides and tree uprooting due to frequent typhoon and heavy rain, but also accelerate tropical forest succession. This protocol first used the slope and soil layer of the undisturbed old-growth tropical rainforest as a reference to reconstruct slope and soil layers. Then multiple tropical tree species with high growth and survival rate were separately monocultured in the reconstructed soil layers. In the year of 2015 and 2016, we tested the effectiveness of this protocol to recover a 0.2 km2 extremely degraded tropical rainforest which consists of bare rock and thus does not support any plant life, in Sanya city, China. Our results showed that, both typhoons and heavy rains did not result in landslide or any tree damages in the area this reforestation protocol was used. Moreover, our separately monocultured eight fast-growing tree species have much higher fast-growing related functional traits than those for tree species in the adjacent undisturbed tropical seasonal forest, which in turn resulted in large soil water and nutrient loss within 3 years. This seemed to simulate a quick transition from primary succession (consist of bare rock and cannot support any plant life) to mid-stage of secondary tropical rainforest succession (many fast-growing pioneer tree species induced high soil water and nutrient loss). Thus, mixing the late-successional tropical tree species with each of the separately monocultured eight fast-growing tree species can accelerate recovery to the undisturbed tropical rainforest as soon as possible. Overall, based on tropical rainforest successional processes, our research provides an effective protocol for quickly and effectively restoring an extremely degraded tropical rainforest ecosystem. We expect that this work will be important for the future recovery of other extremely degraded tropical rainforest ecosystems.
Introduction
Tropical rain forests are highly important ecosystems due to their high biodiversity and ability to store large amount of carbon thereby to play a key role in regulating global climate change (Houghton et al., 2000; Mitchard 2018). Human disturbances in tropical rain forests, including mining, and agricultural use have resulted in heavy deforestation and degradation of these systems and it has been predicted that tropics will have the most deforestation in the near future (Laurance et al., 2014). This degradation, in turn, has led to a global loss in tropical biodiversity, increased carbon emissions, and excessive water loss (Aleman et al., 2018; Symes et al., 2018; Taubert et al., 2018). As a result, it is urgent to develop some effective way to reduce deforestation and its native influences on tropical rain forests.
Reforestation has been assumed to be an effective way to alleviate deforestation and its negative impacts on ecosystem services (Griscom et al., 2017; Taubert et al., 2018). However, frequent typhoons and heavy rains in tropical rainforest can result in landslides and uprooting of many trees (Chang et al., 2008; Yumul et al., 2012; Acosta et al., 2016; Villamayor et al., 2016), making reforestation very difficult. In extremely degraded tropical rainforest (i.e., long ore mining induced extremely degraded tropical rainforest), only a bare rock environment can be found and any plant life can be supported. This is another challenge for performing reforestation that must be overcome. Extremely degraded tropical rainforest (e.g., the ore mining induced degraded tropical rainforest) can be comparable to the initial stage of primary forest succession which originates from the bare rock environment. Thus, an effective approach to reforestation in such conditions is to follow the natural forest successional trajectory (Funk et al., 2008; Johnson and Miyanishi, 2008). However, primary succession usually requires more than 200 years to change the bare rock into the appropriate soil environments to sustain the colonization and survival of tree species (Chapin et al., 1994). Thus, it is highly needed to develop a quick and effective reforestation protocol to not only prevent the landslide resulting from the frequent typhoon and heavy rain, but also facilitate quick forest restoration.
The final target for reforestation in the extremely degraded tropical rainforest is restoring to the undisturbed old-growth tropical rainforest. Usually, frequent typhoons and heavy rains are less disruptive in undisturbed old-growth tropical rain forests. Thus, undisturbed old-growth rainforest in the similar but adjacent locations to the ore mining sites can be a reference to reconstruct slope and soil layers to perform reforestation. It has been found that planting monocultures of fast-growing tree species with high survival rate can help prevent landslide (Stokes et al., 2009; Walker et al., 2009; Pang et al., 2018), thereby to facilitate successfully performing reforestation in tropics. Additionally, based on the tropical rainforest successional processes, fast-growing species usually dominate early succession which in turn may lead to gradually reduced soil water and nutrients loss (Lohbeck et al., 2014). Planting monocultures of fast-growing species can quickly cause high soil nutrient and water loss (Lamb et al., 2005; Wingfield et al., 2015), and thus it can quickly accelerate tropical rainforest succession (Lugo, 1997). Here we develop a simple reforestation protocol, which first uses the slope and soil layer of the undisturbed old-growth tropical rainforest as a reference to reconstruct slope and soil layers. Then separately monoculture multiple tropical tree species with high growth and survival rate in the reconstructed soil layers to not only prevent landslides and tree uprooting due to frequent typhoon and heavy rain, but also accelerate tropical rainforest restoration.
We hypothesized that our protocol could prevent landslides and tree uprooting due to frequent typhoon and heavy rain, while at the same time accelerated tropical rainforest succession. Thus, it can successfully perform reforestation to quickly and effectively recover extremely degraded tropical rain forests. To test this hypothesis, we used our reforestation protocol in a 0.2 km2 extremely degraded tropical rainforest in the Baopoling hill (BPL) in Sanya city, Hainan island. The site has been extremely degraded due to 20 years of limestone mining associated with the cement industry and now consists of bare rock that does not support any plant life. In this study we aim to quantify whether using our reforestation protocol to recover this 0.2 km2 extremely degraded tropical rainforest can prevent landslides resulting from frequent typhoon and heavy rain while also simulating a quick transition from primary succession to mid-stage of the secondary succession and thereby accelerate tropical rainforest succession.
Materials and Methods
Study Sites
BPL (300 m elevation), is a limestone mountain in Sanya City, Hainan island, China (110°58′01′′E, 19°38′48′′N) (Figure 1). The climate of the region is a tropical monsoon oceanic climate with a mean annual temperature of 28°C. The average annual precipitation in Sanya city is 1,500 mm, with approximately 91% of the precipitation occurring between June to October (Luo et al., 2018). The vegetation is described as broad-leaf tropical rainforest (Guo et al., 2013). Limestone mining associated with the cement industry lasted from 1995 till 2015, resulting in a 0.2 km2 extremely degraded area covered by bare rock (Figure 1). Outside of this degraded area is undisturbed old-growth tropical rainforest (Figure 1).
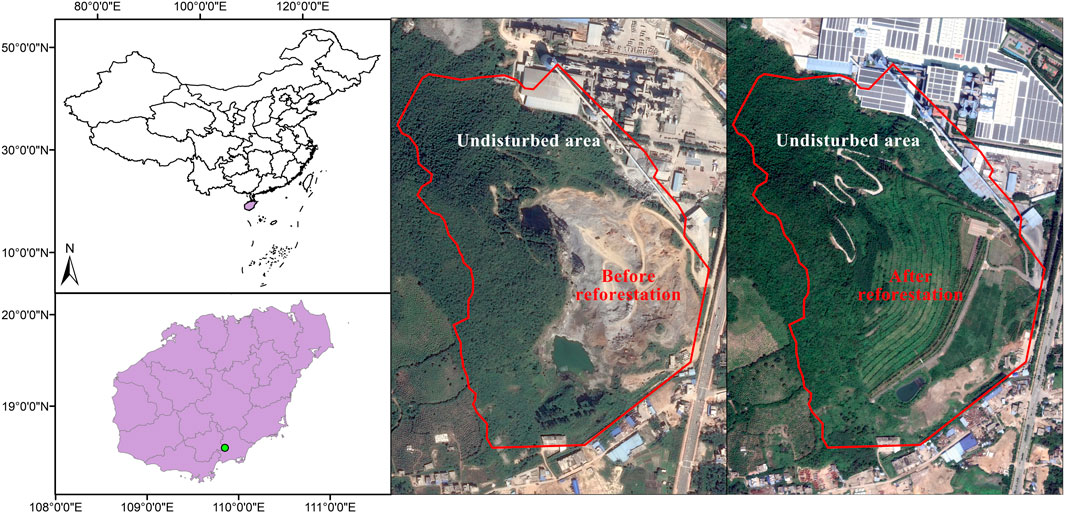
FIGURE 1. Map of the study site (Baopoling hill, Sanya city, Hainan island, China) and the surrounding landscape of the 0.2 km2 highly degraded tropical bare hill, both before (A) and after (B) reforestation efforts.
Description of Our Reforestation Method on BPL
We followed our protocol to use the slope and soil layer of the undisturbed area as a reference to reconstruct slope and soil layers to plant tree seedlings. Thus, the first step of our reforestation project is to analyze the slope and soil depth of the adjacent undisturbed areas of BPL. The slope and soil depth of the adjacent undisturbed areas were measure by theodolite and soil compactness measuring instrument (Spectrum SC900, The United States). Second, the original slope of the highly degraded 0.2 km2 area was cut so as to match the slope from the adjacent undisturbed area (Figure 2A). Third, excavators were used to dig a lot of holes that approximated the soil depth of the adjacent undisturbed area to refill the local high nutrient soils to form eight planted areas form the top to the foot of the 0.2 km2 area (Figure 2B). Finally, eight fast-growing tropical tree species (Terminalia neotaliala, Bombax malabarica, Cleistanthus sumatranus, Ficus microcarpa, Muntingia colabura, Acacia mangium, Syzygium hainanense and Bougainvillea spectabilis) are separately mono-planted in the reconstructed soil layers to quickly perform the reforestation (Figure 2C). These eight fast-growing tree species are proven good afforestation tropical trees in Hainan island and they can have high growth rate and surviving rate after reforestation (Lin et al., 2006; Liu et al., 2017). Moreover, their seedlings are very easy to get in the local market. Specifically, the initial height and a diameter at breast height (DBH) for seedlings of the eight separately monocultured fast-growing tropical tree species ranged from 2 to 3 m and 3–5 cm respectively. Planting density was kept at 80–100 stems per hectare. The entire project was finished in 2016 (Figure 2D) and we recorded their survival rates from 2016 to 2019 as:
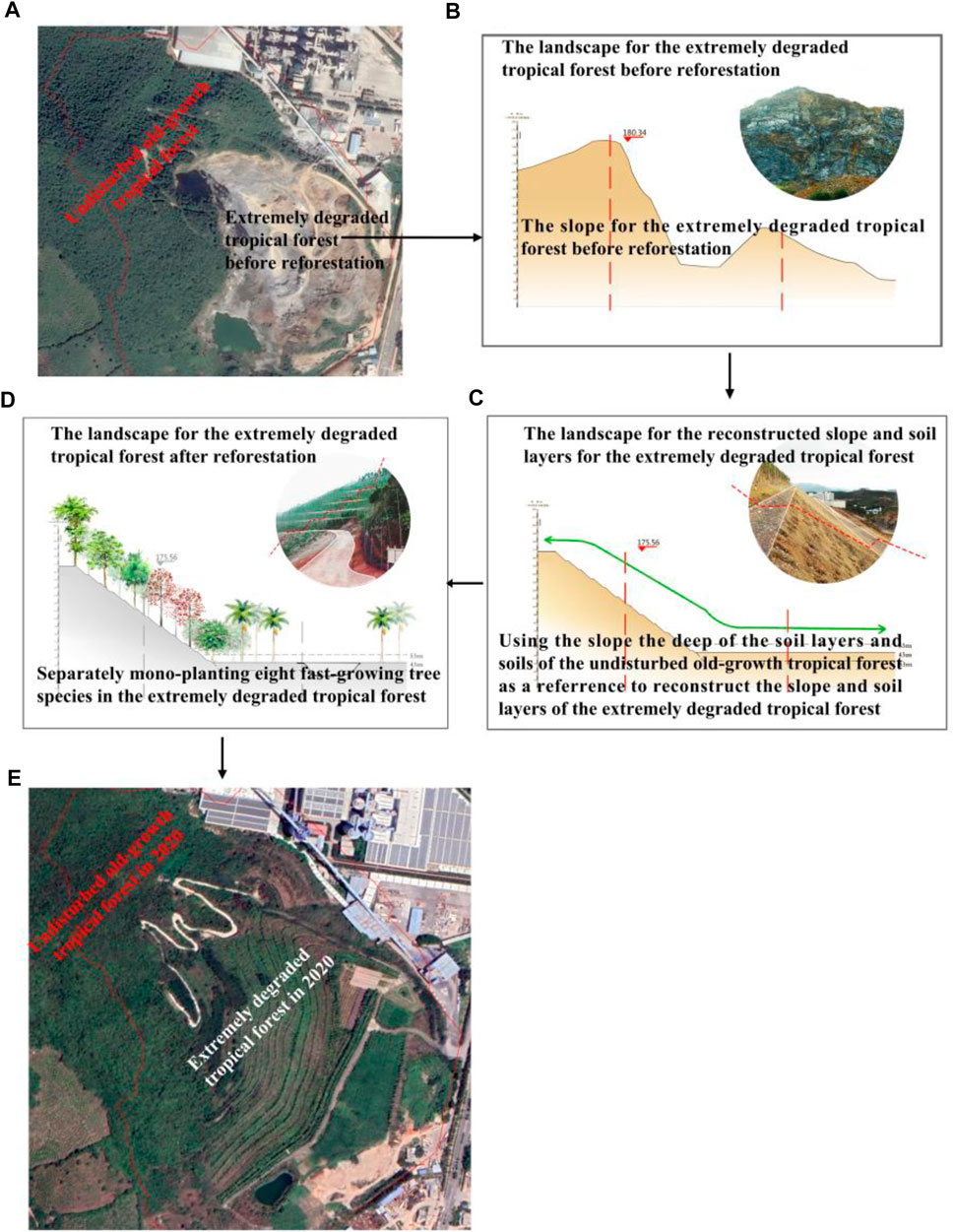
FIGURE 2. The surrounding landscape and detailed procedures regarding the reforestation project in the 0.2 km2 extremely degraded tropical rain forest in Baopoling mountain (BPL). (A) BPL and the surrounding landscape including the 0.2 km2 area of extremely degraded tropical rain forest and the adjacent undisturbed old-growth tropical rain forests. (B) the original landscape and slope of the 0.2 km2 extremely degraded tropical rain forest; (C) using the slope, the deep of soil layers as a reference to reconstructing slope and soil layers, and to refill soils from the undisturbed old-growth tropical forests to form planting area in the extremely degraded tropical rain forest; (D) separately mono-planting eight fast-growing tropical tree species; (E) the landscape of undisturbed old-growth tropical rain forest and the 0.2 km2 extremely degraded tropical rain forest after reforestation efforts in 2020.
Plant Leaf Sampling and Functional Traits Measurement
In 2018, 30 plots, each of 20 × 20 m2 (an area of 400 m2 for each plot) that were at least 100–300 m apart from one another, were randomly sampled across the adjacent undisturbed old-growth forest. Within each plot, all freestanding trees with diameter of ≥1 cm at breast height (DBH) were measured and identified to species. We finally found 80 tree species in the undisturbed old-growth forest and we select the eight tree species (200–300 stems per hectare) (Bridelia tomentosa, Radermachera frondosa, Lepisanthes rubiginosa, Rhaphiolepis indica, Pterospermum heterophyllum, Fissistigma oldhamii, Psychotria rubra and Cudrania cochinchinensis) as our candidate dominant slow-growing species. In the maximum growth season (August) of 2019, we measured plant height and DBH for these eight separately monocultured fast-growing tropical tree species and the eight dominant old-growth tropical rainforest tree species found in the undisturbed site. We also sampled 20 fully expanded and healthy leaves from five individuals for each of the eight separately monocultured fast-growing tropical tree species and dominant old-growth tropical tree species in the undisturbed site, and only individuals with DBH close to the species mean value were selected (Supplementary Table S1). Leaf samples were used to measure ten functional traits related to plant growth, including leaf anatomy traits [palisade tissue (μm), spongy tissue (μm), leaf thickness (μm), leaf tightness (%), and stomatal density (numbers mm−2)], specific leaf area (g cm−2), leaf hydraulic conductivity (kleaf; mmol m−2 s−1 MPa−1), maximum photosynthesis rate (Amass; μmol m−2 s−1), stomatal conductance (mmol m−2 s−1), and transpiration rate (μmol m−2 s−1). Traits were measured following the methods described in Hua et al. (2017) and Zhang et al. (2018a).
Soil Sampling and Properties Measurements
In the peak of growing season (August) of 2015, before planting the seedlings of the eight separately monocultured fast-growing tropical tree species, we followed the methods described in Burt (2009) to determine soil available nitrogen (ammonium and nitrate nitrogen; mg kg−1), soil available phosphorus (mg kg−1), and soil water content (g kg−1) in randomly collected 24 soil samples at a depth of 0–20 cm of the high nutrient local soils that are used for reforestation and soils in the adjacent undisturbed old-growth tropical rain forest. After reforestation, in the peak of growing season (August) of 2019, three soil samples were collected randomly at a depth of 0–20 cm around each of the eight dominant old-growth tropical tree species and monocultured fast-growing tropical tree species to measure soil available nitrogen, soil available phosphorus, and soil water content in the undisturbed old-growth forests. Detailed procedures for measuring both functional traits and soil properties can be found in the Supplementary Material.
Statistical Analysis
Since we did not know the detail distribution of all of our data, we first utilized non-parametric analysis (Wilcoxon signed-rank test) to quantify whether there were any important differences in plant height, DBH, and ten growth traits between the eight separately monocultured fast-growing tropical tree species and eight old-growth tropical tree species. Then, we used principal component analysis (PCA) to evaluate which of the ten growth traits best discriminated between the eight separately monocultured fast-growing tropical tree species and eight old-growth tropical tree species. Finally, we also compared differences in soil water content, available nitrogen, and phosphorus content among the initial local high nutrient soil before reforestation sampled in July, 2015 (reforestation soil in 2015), soils in undisturbed old growth forest sampled in July, 2015 (undisturbed soil in 2015), soils in reforestation area sampled in July, 2019 (reforestation soil in 2019) and soils in undisturbed old growth forest sampled in July, 2019 (undisturbed soil in 2019).
Results
So far both typhoons and heavy rains have not resulted in landslide or tree damages in this reforestation project (Figure 1). Moreover, all of the eight separately mono-planting fast-growing have survived and have had positive growth rates after reforestation. The survival rates among all separately mono-planting fast-growing tree seedlings were greater than 92% after 3 years’ reforestation (Figure 1; Table 1). Furthermore, the height and DBH for the eight separately mono-planting fast-growing tree seedlings increased 2–6 times in the 3 years’ plantation (from 2 to 3 m and 3–5–13 m and 10 cm). In addition, the height and DBH for the eight monocultured fast-growing tree species were also 1.6–2 times larger than the eight dominant old-growth tree species (p < 0.001, based on Wilcoxon signed-rank test, Supplementary Figure S1).
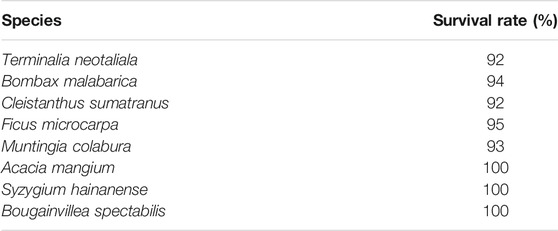
TABLE 1. Survival rates for the eight fast-growing tree species (Terminalia neotaliala (Combretaceae), Bombax malabarica (Bombacaceae), Cleistanthus sumatranus (Euphorbiaceae), Ficus microcarpa (Moraceae), Muntingia colabura (Elaeocarpaceae), Acacia mangium (Leguminosae), Syzygium hainanense (Myrtaceae) and Bougainvillea spectabilis (Nyctaginaceae)) after 3°years of reforestation.
We also found all ten growth trait metrics for the monocultured tree species were greater than the dominant old-growth tree species (p < 0.001, based on Wilcoxon signed-rank test, Figure 3). However, results from the PCA show that five of the traits were best at discriminating between the mono-planting tree species and the old-growth tree species. These include stomatal conductance, stomatal density, leaf water conductance, maximum photosynthesis rate, and transpiration rate (Figure 4; Table 2). Thus, stomatal conductance, stomatal density, leaf water conductance, maximum photosynthesis rate, and transpiration rate are the key characteristics to facilitate the much higher growth rate in the monocultured tree species compared to the dominant old growth tree species.
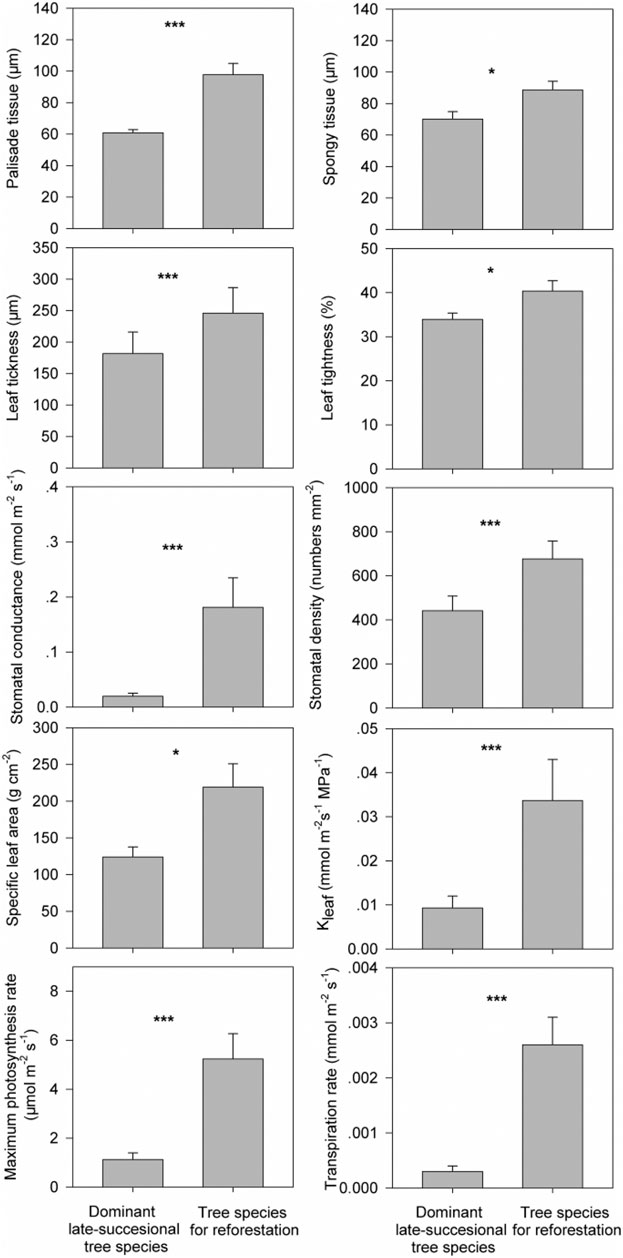
FIGURE 3. Differences in ten functional traits associated with a fast-growth strategy (i.e., palisade tissue (PT; μm), spongy tissue (ST; μm), leaf thickness (LT; μm), leaf tightness (LTI; %), stomatal conductance (SD; mmol m−2 s−1), stomatal density (numbers mm−2), specific leaf area (SLA; g cm−2), leaf hydraulic conductivity (kleaf; mmol m−2s−1 MPa−1), maximum photosynthesis rate (Amass; μmol m−2 s−1) and transpiration rate (μmol m−2 s−1)) between the eight separately monocultured fast-growing tropical tree species used for reforestation (tree species for reforestation) and the eight dominant tropical tree species in the undisturbed old-growth tropical rainforest (dominant late-successional tree species). *** indicates p < 0.001 based on Wilcoxon signed-rank tests.
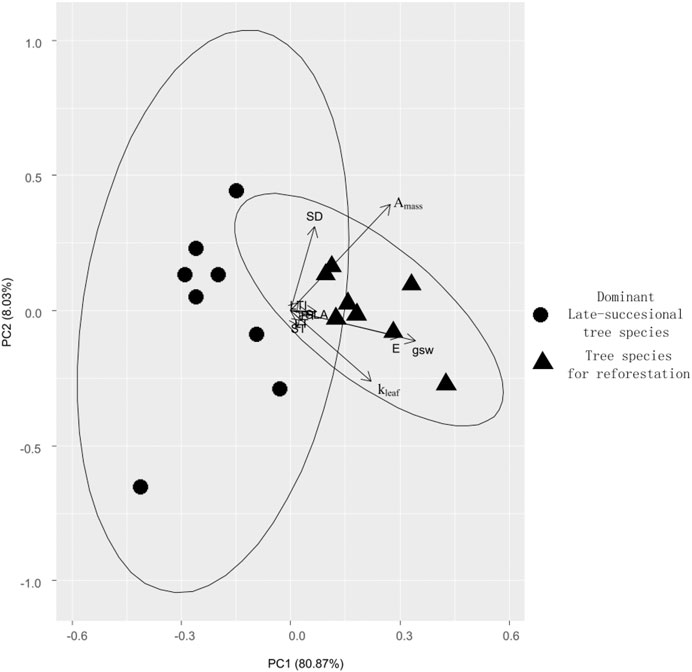
FIGURE 4. Principal component analysis (PCA) of the ten functional traits associated with fast growth between the eight separately monocultured fast-growing tropical tree species (tree species for reforestation) and the eight dominant tropical tree species in the undisturbed old-growth tropical rain forest (dominant late-successional tree species).
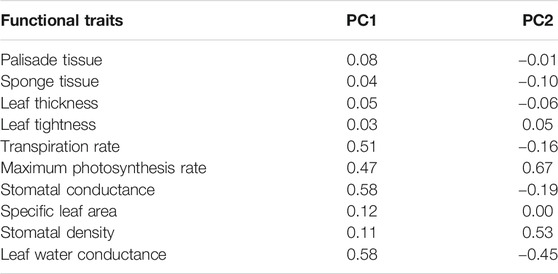
TABLE 2. The first two axes of a principal component analysis (PCA) for the eight fast-growing tree species and eight dominant old-growth tree species, based on 10 plant traits. These include palisade tissue (μm), spongy tissue (μm), leaf thickness (μm), leaf tightness (%), stomatal conductance (mmol m-2 s-1), stomatal density (numbers mm-2), specific leaf area (g cm-2), leaf hydraulic conductivity (kleaf; mmol m-2 s-1 MPa-1), maximum photosynthesis rate (Amass; μmol m-2 s-1) and transpiration rate (μmol m-2 s-1).
In addition, we found that our reforestation method could quickly result in soil water and nutrient loss. That was because soil water content, soil available nitrogen (ammonium and nitrate nitrogen), and available phosphorus content in the local high nutrient soils before reforestation were 1.5–2 times higher than those for soils in undisturbed old growth forest sampled in the same time (August, 2015) (Figure 5 and p < 0.05 based on Wilcoxon signed-rank test in Supplementary Table S2). However, in 2019, after 3 years of growth, the reforestation area had merely 1/4 of the available nitrogen (ammonium and nitrate) and available phosphorus content those for the initial local nutrient soils before reforestation (Figure 5 and p < 0.05 based on Wilcoxon signed-rank test in Supplementary Table S2). In contrast, there were no significant variation in soil water content, soil available nitrogen (ammonium and nitrate nitrogen), and available phosphorus content in the undisturbed area from 2015 to 2019 (Figure 5 and p > 0.05 based on Wilcoxon signed-rank test in Supplementary Table S2). Moreover, soil water content, soil available nitrogen (ammonium and nitrate nitrogen), and available phosphorus content in the reforestation area were only 1/2–1/3 of those in the undisturbed area (Figure 5 and p < 0.05 based on Wilcoxon signed-rank test in Supplementary Table S2).
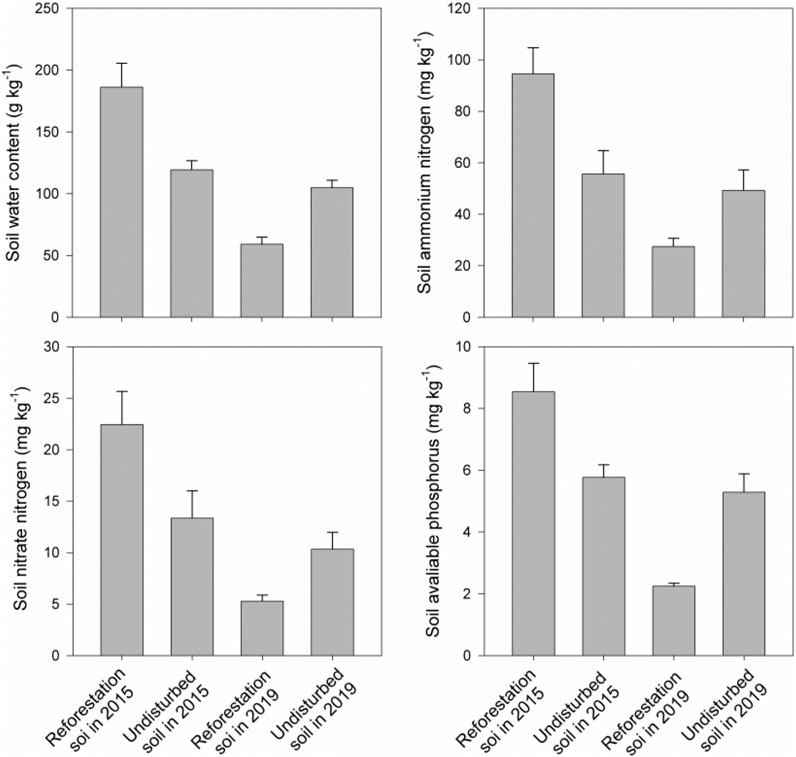
FIGURE 5. Variation in soil water content, soil available nitrogen (ammonium and nitrate N), and soil available phosphorus among the initial local high nutrient soil before reforestation sampled in 2015 (reforestation soil in 2015), soils in undisturbed old-growth tropical rainforest sampled in 2015 (undisturbed soil in 2015), soils in reforestation area sampled in 2019 (reforestation soil in 2019) and soils in undisturbed old-growth tropical rainforest sampled in 2019 (undisturbed soil in 2019).
Discussion
Here we introduce a reforestation protocol which is based on tropical rainforest successional processes to quickly and effectively restore extremely degraded tropical rainforest. Our approach resulted in a successfully restored 0.2 km2 extremely degraded tropical rainforest which consists of bare rock and cannot support any plant life. Importantly this large scale reforestation project helps to solve the challenge of improving forest conditions and the negative outcomes (landslides and tree uprooting) due to frequent typhoon and heavy rain, but also quickly simulates a transition from primary tropical rainforest succession (consisting of merely bare rock and no plant can grow and survive) to mid-stage of secondary tropical rainforest succession (dominant fast-growing tree species have resulted in soil water and nutrient loss). Finding the appropriate tree species in late-stage of secondary tropical rainforest succession can have high potential to quickly simulate late secondary forest succession thereby accelerating the forest trajectory towards undisturbed old-growth tropical rainforest. Ultimately, our reforestation protocol can be treated as a general protocol to perform reforestation to recover extremely degraded tropical rainforest.
The high risk of landslides and uprooting of many trees due to frequent typhoons and heavy rains in tropical rain forests (Chang et al., 2008; Yumul et al., 2012; Acosta et al., 2016; Villamayor et al., 2016) and bare rock environment are two big challenges for performing reforestation in extremely degraded tropical rainforest. Here both typhoons and heavy rains did not result in landslide and any tree damages, when using our reforestation protocol. Moreover, all separately mono-planting multiple fast-growing tropical tree species had a very high survival and growth rate rate (the height and DBH for the eight separately monocultured tree species were 1.6–2 times larger than the dominant tree species in the undisturbed old-growth tropical rain forests after 3 years’ plantation). This confirmed that mono-planting multiple tree species with high survival and growth rate could prevent typhoons and heavy rains induced landslide in tropical rainforest (Stokes et al., 2009; Walker et al., 2009; Pang et al., 2018), but also indicated our reforestation protocol is successful in extremely degraded tropical rainforest.
Photosynthetic rate influences the energy balance of a plant, with higher photosynthetic rates being associated with a fast growth rate (Kirschbaum, 2011; Zhang et al., 2018b). Here, we found that the photosynthetic rates for the eight species were as much as five times larger than the eight native species. This can explain why the height and DBH of the initial seedlings of the species increase 3–5 times merely in 3 years’ plantation (from the year of 2016 to 2019). Higher photosynthetic rates also result in higher leaf anatomy traits (Oguchi et al., 2003; He et al., 2017; Hua et al., 2017), stomatal traits (McAusland et al., 2016; Lawson and Vialet-Chabrand, 2019), transpiration rate and leaf hydraulic conductivity (Maherali et al., 2008; Bucci et al., 2019). Indeed, we found that these traits for the eight fast-growing species were three to five times greater than for native species. These results also indicate that these fast-growing tropical tree species likely developed appropriate leaf traits for maximizing photosynthetic rates and overall growth.
It is well known that soil available nitrogen, phosphorus, and soil water content are key constraints on plant maximum photosynthesis rates (Guan et al., 2015; Riley et al., 2018). This suggests that the eight fast-growing species may absorb a large amount of soil water and nutrients needed for maintaining a higher photosynthetic rate. This, in turn, may decrease soil water and nutrient storage. Indeed, we found that soil water, available nitrogen, and phosphorus largely decrease after 3 years’ reforestation. Thus separately monocultures of multiple fast-growing tree species can indeed result in soil water and nutrient loss (Lamb et al., 2005; Wingfield et al., 2015). Generally in the mid-stage (usually more than 40 years) of secondary tropical rainforest succession, the increased species richness and abundances for the fast-growing pioneers tree species will lead to soil water and nutrient loss, and the late-successional slow-growing but highly resource competitive tree species will gradually replace the early successional fast-growing pioneers tree species (Grime, 2006; Mason et al., 2012). As a result, our reforestation protocol can effectively lead to a transition from primary tropical rainforest succession (consist of bare rock and cannot support any plant life) to the mid-stage of secondary tropical rainforest succession (dominant fast-growing pioneer tropical trees lead to soil water and nutrient loss).
Our PCA results revealed that the eight separately mono-planting tree species and the eight dominant old-growth tree species were significantly distinguished by stomatal conductance, stomatal density, leaf water conductance, maximum photosynthesis rate and transpiration rate. This suggests that these five are the key traits related to higher growth rate in the monocultured fast growing species compared to the old-growth trees. Thus, these five traits can be utilized by a recently developed native species screening framework (Wang et al., 2020) in future to select many late-successional tropical tree species that are maximally dissimilar to these eight separately monocultured tropical tree species from all undisturbed old-growth tropical rainforest in whole Sanya City. Based on the limiting similarity theory, species that are functionally dissimilar can stable coexist (MacArthur and Levins, 1967). However, given the low soil water and nutrient and the much higher water and soil nutrient demand due to the eight separately monocultured tropical tree species’ very high growth rate, these eight separately monocultured tropical tree species cannot compete with the late-successional tropical tree species. Thus, mixing these late-successional tropical tree species with each of the eight separately monocultured tropical tree species can quickly simulate late successional forests and thereby accelerate recovery to the undisturbed tropical rainforest as soon as possible.
Conclusion
In summary, based on tropical rainforest successional processes, we developed a reforestation protocol that includes the following steps for successfully performing reforestation to recover extremely degraded tropical rainforest. First, use the slope, the depth of the soil layers and soil of the adjacent undisturbed tropical rainforest as a reference to reconstruct slope and soil layers (Figures 6A,B). Second separately mono-planting multiple tropical tree species with high growth rate and surviving rate to prevent landslide and tree uprooting (Figure 6C). Third, evaluate whether the high fast-growth rate for these fast-growing species have resulted in large soil water and nutrient loss. If this these fast-growing species have indeed resulted in large soil water and nutrient loss, identify key traits that can be used Wang et al. (2020) to select many functionally dissimilar tropical tree species from the undisturbed old-growth tropical rainforest. Finally, we recommend mixing selected late-successional tropical tree species with each of the monocultured tropical tree species to quickly simulate the late succession thereby to recover to the undisturbed old-growth tropical rainforest as soon as possible (Figures 6D,E). We expect that this work will be important for the future recovery of other extremely degraded tropical rainforest ecosystems.
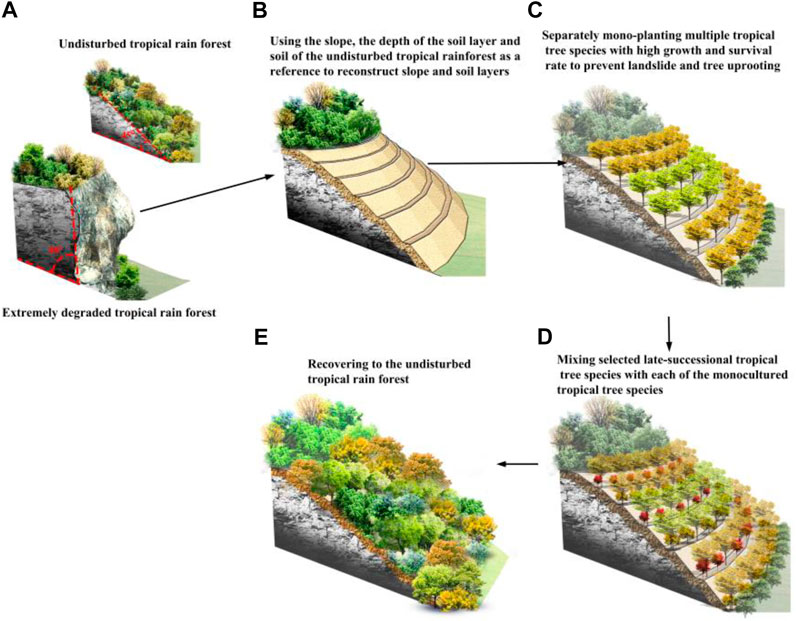
FIGURE 6. A protocol for successfully performing reforestation to recover extremely degraded tropical rainforest. (A) Extremely degraded tropical rain forest and the adjacent undisturbed old-growth tropical rain forests. (B) using the slope, the deep of soil layers as a reference to reconstructing slope and soil layers, and to refill soils from the undisturbed old-growth tropical forests to form planting area in the extremely degraded tropical rain forest; (C) separately mono-planting multiple tropical tree species with high growth rate and survival rate; (D) mixing selected late-successional tropical tree species in the undisturbed tropical rainforest with each of the monocultured tropical tree species; (E) Recovering the extremely degraded tropical rainforest to the undisturbed old-growth tropical rainforest.
Data Availability Statement
The original contributions presented in the study are included in the article/Supplementary Material, further inquiries can be directed to the corresponding authors.
Author Contributions
YaZ, YiZ, LW, RS, HZ, TL, JC, CW, QH, and KJ designed research; YaZ, YiZ, LW, RS, HZ, BC, YX, TL, JC, CW, QH, and KJ performed research; YaZ, YiZ, LW, RS, HZ, TL, JC, CW, QH, and KJ analyzed data; YaZ, HZ, TL, JC, and CW wrote the paper.
Conflict of Interest
Author YX was employed by the company Sanya Huasheng Tianya Cement Co., Ltd.
The remaining authors declare that the research was conducted in the absence of any commercial or financial relationships that could be construed as a potential conflict of interest.
Acknowledgments
This work was funded by the National Natural Science Foundation of China (31770469, 41905094, 31961143023, 31670453, 41430529), Scientific Research Project of Ecological Restoration of Baopoling Mountain in Sanya, and the start-up fund from Hainan University (KYQD (ZR) 1876), the Strategic Priority Research Program of the Chinese Academy of Sciences (Grant No. XDA23080302).
Supplementary Material
The Supplementary Material for this article can be found online at: https://www.frontiersin.org/articles/10.3389/fenvs.2021.701210/full#supplementary-material
References
Acosta, L. A., Eugenio, E. A., Macandog, P. B. M., Macandog, D. B. M., Lin, E. K. H., Abucay, E. R., et al. (2016). Loss and Damage from Typhoon-Induced Floods and Landslides in the Philippines: Community Perceptions on Climate Impacts and Adaptation Options. Int. J. Glob. Warming 9 (1), 33–65. doi:10.1504/ijgw.2016.074307
Aleman, J. C., Jarzyna, M. A., and Staver, A. C. (2018). Forest Extent and Deforestation in Tropical Africa Since 1900. Nat. Ecol. Evol. 2, 26–33. doi:10.1038/s41559-017-0406-1
Bucci, S. J., Carbonell Silletta, L. M., Garré, A., Cavallaro, A., Efron, S. T., Arias, N. S., et al. (2019). Functional Relationships Between Hydraulic Traits and the Timing of Diurnal Depression of Photosynthesis. Plant Cell Environ. 42 (5), 1603–1614. doi:10.1111/pce.13512
Burt, R. (2009). Soil Survey Field and Laboratory Methods Manual. Washington, DC: National Soil Survey Center, Natural Resources Conservation Service, US Department of Agriculture.
Chang, K. T., Chiang, S. H., and Lei, F. (2008). Analysing the Relationship Between Typhoon‐Triggered Landslides and Critical Rainfall Conditions. Earth Surf. Process. Landforms 33 (8), 1261–1271. doi:10.1002/esp.1611
Chapin, F. S., Walker, L. R., Fastie, C. L., and Sharman, L. C. (1994). Mechanisms of Primary Succession Following Deglaciation at Glacier Bay, Alaska. Ecol. Monogr. 64, 149–175. doi:10.2307/2937039
Funk, J. L., Cleland, E. E., Suding, K. N., and Zavaleta, E. S. (2008). Restoration Through Reassembly: Plant Traits and Invasion Resistance. Trends Ecol. Evol. 23 (12), 695–703. doi:10.1016/j.tree.2008.07.013
Grime, J. P. (2006). Trait Convergence and Trait Divergence in Herbaceous Plant Communities: Mechanisms and Consequences. J. Vegetation Sci. 17 (2), 255–260. doi:10.1111/j.1654-1103.2006.tb02444.x
Griscom, B. W., Adams, J., Ellis, P. W., Houghton, R. A., Lomax, G., Miteva, D. A., et al. (2017). Natural Climate Solutions. Proc. Natl. Acad. Sci. USA 114 (44), 11645–11650. doi:10.1073/pnas.1710465114
Guan, K., Pan, M., Li, H., Wolf, A., Wu, J., Medvigy, D., et al. (2015). Photosynthetic Seasonality of Global Tropical Forests Constrained by Hydroclimate. Nat. Geosci. 8 (4), 284–289. doi:10.1038/ngeo2382
Guo, K. J., Xiong, J. W., Wu, J. Z., Liu, Z. F., Li, Z. G., and Zhang, B. (2013). Scenery Resources Survey and Feature Analysis of forest in Sanya City. Cent. South For. Inventory Plann. 32 (3), 11–17. doi:10.16166/j.cnki.cn43-1095.2013.03.001
He, N., Liu, C., Tian, M., Li, M., Yang, H., Yu, G., et al. (2017). Variation in Leaf Anatomical Traits from Tropical to Cold‐temperate Forests and Linkage to Ecosystem Functions. Funct. Ecol. 32 (8), 10–19. doi:10.1111/1365-2435.12934
Houghton, R. A., Skole, D. L., Nobre, C. A., Hackler, J. L., Lawrence, K. T., and Chomentowski, W. H. (2000). Annual Fluxes of Carbon from Deforestation and Regrowth in the Brazilian Amazon. Nature 403 (6767), 301–304. doi:10.1038/35002062
Hua, L., Chen, Y., Zhang, H., Fu, P., and Fan, Z. (2017). Stronger Cooling Effects of Transpiration and Leaf Physical Traits of Plants from a Hot Dry Habitat Than from a Hot Wet Habitat. Funct. Ecol. 31 (12), 2202–2211. doi:10.1111/1365-2435.12923
Johnson, E. A., and Miyanishi, K. (2008). Testing the Assumptions of Chronosequences in Succession. Ecol. Lett. 11 (5), 419–431. doi:10.1111/j.1461-0248.2008.01173.x
Kirschbaum, M. U. F. (2011). Does Enhanced Photosynthesis Enhance Growth? Lessons Learned from CO2 Enrichment Studies. Plant Physiol. 155 (1), 117–124. doi:10.1104/pp.110.166819
Lamb, D., Erskine, P. D., and Parrotta, J. A. (2005). Restoration of Degraded Tropical Forest Landscapes. Science 310 (5754), 1628–1632. doi:10.1126/science.1111773
Laurance, W. F., Sayer, J., and Cassman, K. G. (2014). Agricultural Expansion and its Impacts on Tropical Nature. Trends Ecol. Evol. 29 (2), 107–116. doi:10.1016/j.tree.2013.12.001
Lawson, T., and Vialet-Chabrand, S. (2019). Speedy Stomata, Photosynthesis and Plant Water Use Efficiency. New Phytol. 221 (1), 93–98. doi:10.1111/nph.15330
Lin, J. Y., Zeng, R., Liu, H. W., Chen, H. Y., and Li, S. X. (2006). The Important Role of Local Tree Species in the Construction of Tropical Characteristic Garden. Trop. For. 4, 1–11. doi:10.3969/j.issn.1672-0938.2006.04.007
Liu, X. Z., Xue, Y., Wang, X. Y., Lin, Z. P., and Su, X. F. (2017). Analysis of Different Reforestation experiment in the Northeast Tropical Coast of Hainan Province. Ecol. Sci. 36, 130–134. doi:10.14108/j.cnki.1008-8873.2017.03.018
Lohbeck, M., Poorter, L., Martínez-Ramos, M., Rodriguez-Velázquez, J., van Breugel, M., and Bongers, F. (2014). Changing Drivers of Species Dominance During Tropical forest Succession. Funct. Ecol. 28 (4), 1052–1058. doi:10.1111/1365-2435.12240
Lugo, A. E. (1997). The Apparent Paradox of Reestablishing Species Richness on Degraded Lands with Tree Monocultures. For. Ecol. Manage. 99 (1), 9–19. doi:10.1016/S0378-1127(97)00191-6
Luo, H. X., Dai, S. P., Li, M. F., and Xi, Z. H. (2018). The Climate Characteristics of 302 Hainan Island from 1959-2015. Jiangsu Agric. Sci. 46 (15), 261–268. doi:10.15889/j.issn.1002-1302.2018.15.066
MacArthur, R., and Levins, R. (1967). The Limiting Similarity, Convergence, and Divergence of Coexisting Species. The Am. Naturalist 101 (921), 377–385. doi:10.1086/282505
Maherali, H., Sherrard, M. E., Clifford, M. H., and Latta, R. G. (2008). Leaf Hydraulic Conductivity and Photosynthesis Are Genetically Correlated in an Annual Grass. New Phytol. 180 (1), 240–247. doi:10.1111/j.1469-8137.2008.02548.x
Mason, N. W. H., Richardson, S. J., Peltzer, D. A., de Bello, F., Wardle, D. A., and Allen, R. B. (2012). Changes in Coexistence Mechanisms along a Long-Term Soil Chronosequence Revealed by Functional Trait Diversity. J. Ecol. 100 (3), 678–689. doi:10.1111/j.1365-2745.2012.01965.x
McAusland, L., Vialet‐Chabrand, S., Davey, P., Baker, N. R., Brendel, O., and Lawson, T. (2016). Effects of Kinetics of Light‐induced Stomatal Responses on Photosynthesis and Water‐use Efficiency. New Phytol. 211 (4), 1209–1220. doi:10.1111/nph.14000
Mitchard, E. T. A. (2018). The Tropical Forest Carbon Cycle and Climate Change. Nature 559 (7715), 527–534. doi:10.1038/s41586-018-0300-2
Oguchi, R., Hikosaka, K., and Hirose, T. (2003). Does the Photosynthetic Light-Acclimation Need Change in Leaf Anatomy? Cell Environ. 26 (4), 505–512. doi:10.1046/j.1365-3040.2003.00981.x
Pang, C.-C., Ma, X. K.-K., Lo, J. P.-L., Hung, T. T.-H., and Hau, B. C.-H. (2018). Vegetation Succession on Landslides in Hong Kong: Plant Regeneration, Survivorship and Constraints to Restoration. Glob. Ecol. Conservation 15 (2), e00428. doi:10.1016/j.gecco.2018.e00428
Riley, W. J., Zhu, Q., and Tang, J. Y. (2018). Weaker Land-Climate Feedbacks from Nutrient Uptake During Photosynthesis-Inactive Periods. Nat. Clim. Change 8, 1002–1006. doi:10.1038/s41558-018-0325-4
Stokes, A., Atger, C., Bengough, A. G., Fourcaud, T., and Sidle, R. C. (2009). Desirable Plant Root Traits for Protecting Natural and Engineered Slopes Against Landslides. Plant Soil 324 (1), 1–30. doi:10.1007/s11104-009-0159-y
Symes, W. S., Edwards, D. P., Miettinen, J., Rheindt, F. E., and Carrasco, L. R. (2018). Combined Impacts of Deforestation and Wildlife Trade on Tropical Biodiversity Are Severely Underestimated. Nat. Commun. 9 (1), 4052. doi:10.1038/s41467-018-06579-2
Taubert, F., Fischer, R., Groeneveld, J., Lehmann, S., Müller, M. S., Rödig, E., et al. (2018). Global Patterns of Tropical forest Fragmentation. Nature 554 (7693), 519–522. doi:10.1038/nature25508
Villamayor, B. M. R., Rollon, R. N., Samson, M. S., Albano, G. M. G., and Primavera, J. H. (2016). Impact of Haiyan on Philippine Mangroves: Implications to the Fate of the Widespread Monospecific Rhizophora Plantations Against Strong Typhoons. Ocean Coastal Manage. 132 (2), 1–14. doi:10.1016/j.ocecoaman.2016.07.011
Walker, L. R., Velázquez, E., and Shiels, A. B. (2009). Applying Lessons from Ecological Succession to the Restoration of Landslides. Plant Soil 324 (1), 157–168. doi:10.1007/s11104-008-9864-1
Wang, C., Zhang, H., Liu, H., Jian, S., Yan, J., and Liu, N. (2020). Application of a Trait‐based Species Screening Framework for Vegetation Restoration in a Tropical Coral Island of China. Funct. Ecol. 34 (6), 1193–1204. doi:10.1111/1365-2435.13553
Wingfield, M. J., Brockerhoff, E. G., Wingfield, B. D., and Slippers, B. (2015). Planted Forest Health: The Need for a Global Strategy. Science 349 (6250), 832–836. doi:10.1126/science.aac6674
Yumul, G. P., Servando, N. T., Suerte, L. O., Magarzo, M. Y., Juguan, L. V. V., and Dimalanta, C. B. (2012). Tropical Cyclone-Southwest Monsoon Interaction and the 2008 Floods and Landslides in Panay Island, central Philippines: Meteorological and Geological Factors. Nat. Hazards 62 (3), 827–840. doi:10.1007/s11069-012-0109-5
Zhang, H., Chen, H. Y. H., Lian, J., John, R., Ronghua, L., Liu, H., et al. (2018a). Using Functional Trait Diversity Patterns to Disentangle the Scale‐dependent Ecological Processes in a Subtropical forest. Funct. Ecol. 32 (5), 1379–1389. doi:10.1111/1365-2435.13079
Keywords: deforestation, fast-growing trees, functional traits, primary succession, reforestation, secondary succession
Citation: Zhao Y, Zou Y, Wang L, Su R, He Q, Jiang K, Chen B, Xing Y, Liu T, Zhang H, Cui J and Wang C (2021) Tropical Rainforest Successional Processes can Facilitate Successfully Recovery of Extremely Degraded Tropical Forest Ecosystems Following Intensive Mining Operations. Front. Environ. Sci. 9:701210. doi: 10.3389/fenvs.2021.701210
Received: 27 April 2021; Accepted: 14 May 2021;
Published: 07 June 2021.
Edited by:
Wenxing Long, Hainan University, ChinaCopyright © 2021 Zhao, Zou, Wang, Su, He, Jiang, Chen, Xing, Liu, Zhang, Cui and Wang. This is an open-access article distributed under the terms of the Creative Commons Attribution License (CC BY). The use, distribution or reproduction in other forums is permitted, provided the original author(s) and the copyright owner(s) are credited and that the original publication in this journal is cited, in accordance with accepted academic practice. No use, distribution or reproduction is permitted which does not comply with these terms.
*Correspondence: Tiedong Liu, dGllZG9uZ2xpdUAxNjMuY29t; Hui Zhang, NDQ2MDU2OTYyQHFxLmNvbQ==; Jie Cui, amllX19jdWlAMTYzLmNvbQ==; Chen Wang, Y2hlbi53YW5nQHNjYmcuYWMuY24=
†These authors have contributed equally to this work