- 1School of Science, University of Waikato, Hamilton, New Zealand
- 2School of Life and Environmental Sciences, Deakin University, Geelong, VIC, Australia
- 3Harry Butler Institute, Murdoch University, Murdoch, WA, Australia
Terrestrial sources of marine debris on beaches are substantial, increasing, and are primarily a result of mismanaged waste on land. The scale, source, and composition of beached marine debris in New Zealand was determined by surveying 41 beaches, with triplicate belt transects, across the North and South Islands. Results demonstrated a significant spatial variance, with the South Island showing a significantly higher mean density than the more populated North Island by count as well as by weight. The majority of all anthropogenic marine debris detected was plastic and arrived through the water. Explanations for regional variances in debris presence are difficult to ascertain with certainty but could not be explained by population density and proximity. These findings contribute to the understudied field of marine debris research in New Zealand and the Southern Hemisphere and provide a starting point for evidence-based mitigation. Recommended changes to future monitoring programs are made. This first national baseline study of marine debris in New Zealand serves as a reference for follow-up studies, including research at other locations.
Introduction
Globally, about 80% of anthropogenic marine debris (AMD) is derived from land-based sources (Derraik, 2002; Sheavly and Register, 2007); a large proportion of which results from mismanaged terrestrial waste (Barnes et al., 2009; Jambeck et al., 2015; Lau et al., 2020). Coastal marine environments are a known accumulation zone for AMD (Galgani et al., 2015; Sherman and Sebille, 2016), and beach surveys are an often-applied tool to better understand the scope and current nature of the problem of AMD (Slavin et al., 2012; Jang et al., 2018). Most coastal AMD studies occur on sandy beaches (Browne et al., 2015; Serra-Gonçalves et al., 2019) due to easy access and generally require no specialized equipment (GESAMP, 2019). In addition to obtaining scientific data, beach surveys are also performed by volunteers (citizen scientists) to support scientists, raise public awareness, and as education and community outreach programs (Ribic et al., 2010; Hidalgo-Ruz and Thiel, 2015; Murray et al., 2018). There are many different methods to obtain data and/or measure the spatial and temporal distribution of debris (Velander and Mocogni, 1999; Browne et al., 2015; GESAMP, 2019), and many variations exist.
Such variations occur, for example, in measurement units (e.g., transect, quadrant, line), placement of sampling unit (horizontally or vertically relating to the waterline), location of sampling unit on the beach (anywhere between the waterline and into the backshore), sample replication, and reporting units (e.g., total item count, litter density (Kordella et al., 2013; Kaladharan et al., 2017; Rangel-Buitrago et al., 2017; Schmuck et al., 2017). Due to these differences, results are often not comparable, rendering an evaluation and comparison of associated mitigation actions at a regional, national, or global scale challenging (Serra-Gonçalves et al., 2019).
Debris studies often classify the origin of AMD either from ocean-based, land-based, or unknown sources (e.g., Coe and Rogers 1997; Sheavly and Register 2007) or through a likely origin (Whiting, 1998; Tudor and Williams, 2004; Pieper et al., 2019; Verlis and Wilson, 2020). However, in New Zealand (NZ), it is not always clear whether the origin of an item is freshwater or ocean. Land-based sourced items can have multiple pathways: entering the aquatic environment through stormwater drains, lakes and waterways, washed or blown out onto the beaches and into the ocean (McCormick and Hoellein, 2016; Boucher and Friot, 2017; Lebreton et al., 2017). Hence, most debris in the marine environment may have had a land origin.
In NZ, boundaries of regional management areas are roughly based on catchment areas. The respective administrative agencies, “Regional Authorities”, are responsible for, amongst others, the region’s environmental management. The underlying local municipalities, “Territorial Authorities”, are among other things, responsible for waste management and the control of the effects of land use. In 2017, the year of this study, NZ was estimated to have produced 740.3 kg of waste per capita, 218.7 kg more (per capita) than the average (521.6 kg) of all other OECD countries combined (OECD, 2021). Furthermore, waste creation in NZ continued to increase, as evidenced by the 2018 per capita amount of 781.1 kg versus a 538.3 kg average of all OECD countries. The NZ waste and recycling industry is listed as one of the least efficient of all developed countries (Hoornweg and Bhada-Tata, 2012; OECD, 2017). All of NZ’s residual waste goes to landfills, creating an increased potential for waste loss to the (marine) environment (Office of the Prime Minister’s Chief Science Advisor, 2019). One example of such waste loss is the Fox Glacier landfill (SI) rupture in 2019, which released decades of previously landfilled household rubbish onto 1,313 ha of sensitive riverbeds and banks, and 64 km of coastline (Office of the Prime Minister’s Chief Science Advisor 2019, page 194).
Previous NZ studies measured marine debris on beaches in the Coromandel Peninsula on the North Island (NI) (Campbell et al., 2017), from the coasts of the Canterbury region on the South Island (SI) (Clunies-Ross et al., 2016), and around Auckland (NI; Bridson et al., 2020). Earlier beach surveys were decades old (Gregory, 1978), and all studies were localized. Currently, no nationwide research study of AMD exists that contemporaneously covers both the NI and SI beaches. Hence, it is difficult to accurately determine the national status of AMD and its environmental effects and relationship to local or national mitigation actions to reduce waste loss to the marine environment. Understanding the actual AMD problem across both islands and the relevant management areas is an important gap to fill. Therefore, this study aims to understand further the scale and composition of AMD loads at a local, regional, and national level. A second aim is to develop a baseline of AMD on NZ beaches against which future changes in AMD can be measured and the efficacy of mitigating actions assessed. The three research questions supporting these goals are:
• What is the distribution of AMD on NZ beaches by count, weight, type, and source?
• Are there significant differences in the results of the AMD distribution by island (north versus south)?
• How is AMD geographically distributed across regional management scales?
Materials and Methods
Regions and Sample Sites
The human population is unevenly distributed over the islands, with the NI and SI having 3,642,900 and 1,149,576 inhabitants, respectively (Stats, 2019). NZ is an archipelago bordered by the Tasman Sea and the South Pacific Ocean with approximately 18,200 km of shoreline (Hutching, 1998). A subtropical, southern current flows along the NI’s east coast that joins the northern current by the East Cape (NI) (Chiswell et al., 2015). The SI has sub-Antarctic surface water moving northwards along the east coast (Hayward et al., 2003). Between Gisborne (NI) and Otago (SI), waves usually arrive from the south and east (NIWA, 2017). The daily changing weather is a result of weather systems, the maritime position, and orography, causing predominantly westerly winds and an average annual rainfall of 800 – 1,500 mm y-1 (Pickrill and Mitchell, 1979; Tomlinson, 1992).
Study sites were predetermined based on a stratified random sampling design. The sampling frame spanned the east coasts of both islands, starting at the top of the NI (34.4°S) to the bottom of the SI (46.5°S). Three random numbers were generated per latitude to select a location from where the nearest sampling site was determined. From this random starting point, the coastline was followed to the right (when facing the water), until the following criteria were met for a site:
1) East-facing (when facing the water, compass direction was either NE, E, or SE)
2) Length was > 1 km
3) Faced open ocean
4) Away from (> 500 m) obstructions (such as headlands, breakwaters, jetties)
5) Accessible from the land through public roads
These site criteria were applied to reduce potential confounding factors such as fluctuations in oceanic and climatic influences or localized effects (e.g. urban marine structures; Campbell et al., (2017). Furthermore, a large part of the SI’s west coast is inaccessible from land.
Forty-one beaches (20 on the NI and 21 on the SI) were surveyed between latitudes 34.7°S and 46.3°S, covering a total of 11.6° in latitude (Figure 1). Study sites covered 11 of NZ’s 16 regions (8 on NI and 3 on SI), with the remaining regions not sampled because they did not border the east coast. One exception was Southland, which was excluded because of random sampling and the criteria (i.e., east facing). Sites were distributed over 25 out of 66 territorial authorities (14 on the NI and 11 on the SI).
Sampling Method
A one-time-only, standing stock survey at each beach provided a temporal snapshot of the AMD distribution by count, weight, type, and source. We note that standing stock surveys are subject to limitations in comparison to an accumulation study (Smith and Markic, 2013; Ryan et al., 2014). In addition, sampling occurred during the austral spring (between 28th September and November 3, 2017) to avoid localized effects of the busier (with tourists) austral summer and autumn seasons.
The adapted research method was based on Cheshire et al. (2009) and sampled the highest visible wrack line using triplicate belt transects (10 m long by 2 m wide). Instead of sampling the entire beach surface between the waterline and backshore, the wrack line was selected, as it can be sampled irrespective of tides and is a known accumulation zone of AMD (Velander and Mocogni, 1999). In addition, prior studies indicate that AMD is rarely detected between the waterline and wrack line (Williams and Tudor, 2001; Hidalgo-Ruz et al., 2018), and the wrack line was, therefore, our focus to determine the AMD standing stock.
A starting point at the beach was placed 50 strides (about 1 m each) to the left (when facing the water). Randomly generated distances (between 21 and 40 strides) determined the distance of the starting point for each belt transect. A 10 m long tape measure was placed on the middle of the wrack line, and 1 m on each side of the tape was examined (creating a 2 m wide transect). All visible items (> 2 mm) from the surface of each transect were collected by a visual survey without raking or digging. Items were bagged and labeled after which the (detangled) debris was counted, weighed, and classified based on the type of material (plastic, foamed plastic, rubber, metal, glass and ceramic, cloth, paper and cardboard, wood, and other) (Cheshire et al., 2009). Large items (> 1 m) were counted but not weighed. Other variables recorded during sampling included the gradient of the beach, substrate, backshore type, beach shape, aspect, wind speed, wind direction, number of people on the beach, number of rubbish bins, and number of parking spaces (adapted from Lippiatt et al., 2013; Schuyler et al., 2018).
In this study, a subjective distinction between the source of an item was based on visual evidence of an item having been in water (whether the ocean or freshwater) and will hereafter be called “waterborne”. Debris characteristics indicating a waterborne source include:
• A weathered appearance-porous look/feel, faded colors, bite marks, smoothened edges.
• Items from a foreign source that are typically not sold in NZ.
• The presence of biofouling, entanglement in other marine organisms.
In contrast, “land-based” items lacked a weathered appearance (were pristine and intact) and lacked biofouling. If a decision on origin could not be determined, then the item was labeled “unknown”.
Analysis
Differences between AMD distribution by island (north versus south) were calculated through mean density and weight and were analyzed with Welch’s t-test (Welch, 1947). This adaptation of the Student’s t-test is better suited for testing the means of two populations with unequal variances (Ruxton, 2006). Results are given in t[degrees of freedom] = t, p (T ≤ t).
Significant differences in AMD composition and sources between islands were determined using Pearson’s Chi-Square test of independence (Pearson, 1900). Results are reported with (degrees of freedom and sample size) the Pearson chi-square value and the significance level. All statistical tests were performed in Microsoft ExcelTM (version 2105) with a significance level of 0.05.
Mean densities and weights for each region were calculated and reported as Mean ± SE items m-2 and g m-2, respectively, to determine distribution at a regional scale. AMD composition was compared at a regional level by percentages of types by count density. The resulting exploratory data is represented in a graph illustrating beach numbers from north to south (increasing latitudes, with no overlapping longitudes).
Results
Baseline AMD Beach Data on Density, Mass, Composition, and Source
The mean AMD density detected across 41 beaches on both islands of NZ ranged from zero to 0.82 items m-2 per beach, with an overall mean of 0.16 ± 0.02 items m-2. Overall, the highest AMD density was detected at Karitane Beach (site 37, SI, Figure 2A) with 0.82 ± 0.02 items m-2, more than five times the national mean. In contrast, three beaches [sites 6 (NI), 24 and 26 (SI)] had zero items >2 mm recorded (Figure 2B).
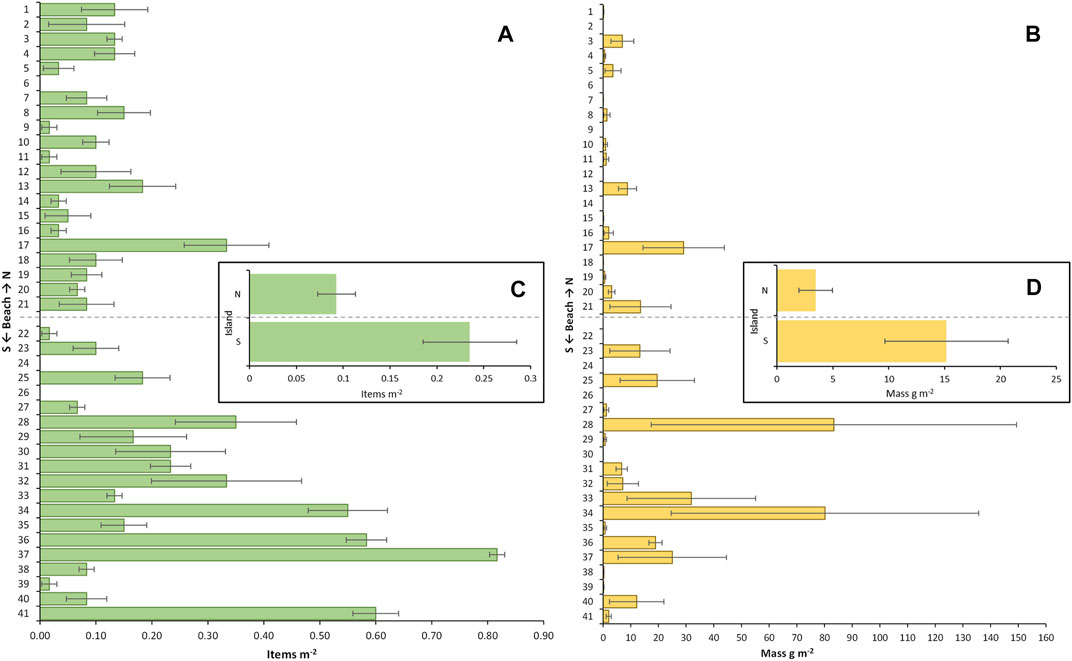
FIGURE 2. Mean Anthropogenic Marine Debris (> 2 mm) Across New Zealand Beaches by (A) Density (± SE), and (B) Weight (± SE); and Across Islands by (C) Density (± SE), and (D) Weight (± SE).
The mean weight of AMD items ranged from zero to 83.38 g m-2 per beach, with an overall mean AMD weight of 9.17 ± 2.91 g m-2 per beach. Overall, the highest AMD weight was detected at Ashworths Beach (site 28, SI) with 83.38 ± 80.82 g m-2, more than nine times the national mean. In contrast, six beaches [sites 2, 6, 14 (NI) and 22, 24, 26 (SI)] recorded no AMD weight, albeit three of those did record items by count, but their weight was < 1 gr.
By count, the most prevalent type of AMD was plastic (n = 261 items), followed by foamed plastic (n = 44 items), metal (n = 41 items), wood (n = 19 items), glass and ceramic (n = 9 items), cloth (n = 7 items), rubber (n = 6 items), other (n = 2 items), and paper and cardboard (n = 1 item).
By weight, the most prevalent type of AMD was metal (6739 g), followed by wood (6723 g), rubber (4011 g), plastic (2513 g), cloth (1416 g), glass and ceramic (1154 g), paper and cardboard (6 g), other (6 g), and foamed plastic (0 g). Of the 399 AMD items (including large items) detected, 306 (77%) were waterborne, 56 (14%) were land-based, and 37 items (9%) had an unknown source.
Differences Between North and South Island
When comparing the islands by AMD density, there was a significant difference between the amount (number of items) of AMD detected, between the NI and SI (t[23] = -2.60; p < .02) (Figure 2C). There was a higher mean density of AMD items on the SI (M = 0.24 ± 0.05 m-2) than the NI (M = 0.09 ± 0.02 m-2). Similarly, the mean weight of AMD differed significantly between islands (t[22] = -2.05; p = 0.05), with significantly higher mean AMD weight on the SI (M = 15.18 ± 5.52 g m-2) compared to the NI (M = 3.45 ± 1.50 g m-2) (Figure 2D).
The composition of AMD, however, was similar between islands (χ2 (3, N = 399) = 6.13, p < 11). These results were based upon comparing four AMD types only (i.e., plastic, foamed plastic, metal, and wood) due to a lack of sufficient count data in the other categories. Overall, the sources of the AMD found between the islands were significantly different (χ2 (2, N = 399) = 23.03, p < .01); NI and SI showed a similar proportion of waterborne items (74 and 78%, respectively). The NI had more land-based items than the SI, with 26 and 9%, respectively. The SI showed 13% of unknown items, of which the NI had none.
Comparison of Debris by Regional Management Level
The Otago region (SI) had the highest mean density of AMD (M = 0.36 ± 0.06 m-2), and the Waikato region (NI) had the least mean density (M = 0.02 ± 0.02 m-2) (Figure 3A). The Otago region also had the highest mean weight (M = 17.45 ± 9.28 g m-2), and the Auckland region (NI) the least mean weight (M = 0.57 ± 0.47 g m-2) (Figure 3B).
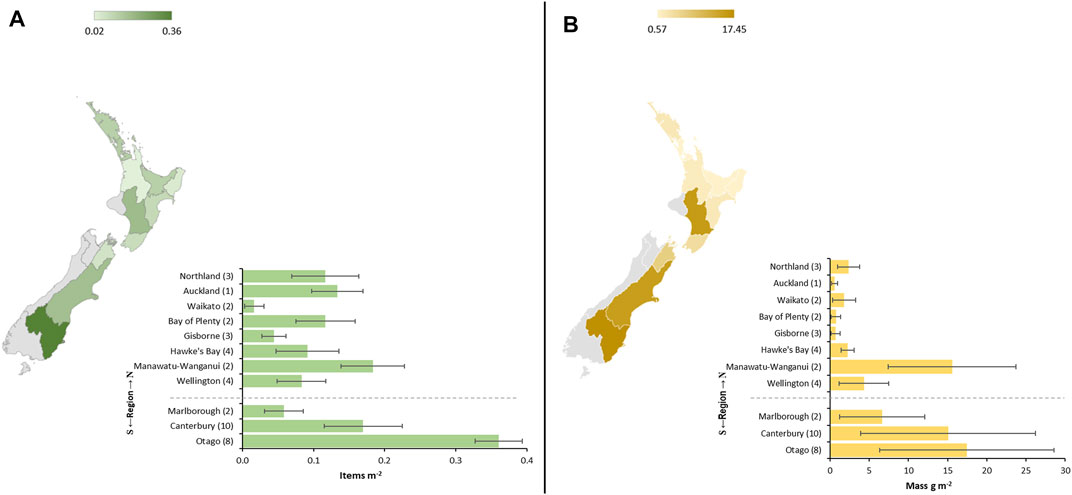
FIGURE 3. New Zealand Beach Anthropogenic Marine Debris (> 2 mm) per Region (Number of Beaches) in Spring 2017 by (A) Mean Density (± SE) and (B) Mean Weight (± SE).
The Waikato region (NI) stood out as only one AMD category (glass and ceramic) was detected here by count (n = 2). When considering the weight of AMD categories, metal debris was not found on a substantial scale in the four most northern regions (Northland, Auckland, Waikato, and Bay of Plenty), which was otherwise detected in significant amounts (>1 g) in all other regions.
Discussion
The etiology of beached AMD results from multiple and often interwoven factors (Hardesty et al., 2017; Willis et al., 2018; Schuyler et al., 2021). This study contributes to the understanding of AMD on NZ beaches by providing a first national baseline. We found that on NZ beaches, population density does not explain variations in AMD between islands and regions.
Patterns of AMD Distribution
This study showed a similar mean AMD density to a prior NZ study on 27 beaches in one region (Waikato, NI), however, measurements were made on sample sites with a different aspect, smaller size, and on a different part of the beach (Campbell et al., 2017). Other NZ debris studies have reported results in different units and measured fewer beaches, making comparisons challenging (Gregory, 1999; Hayward, 1984, 1999). When comparing to standing stock studies of more than 20 beaches in other locations, the overall mean density as determined in this study is similar to results from Australia, but less than surveys from Turkey, Sri Lanka, and Portugal (Azores) (Table 1). Although these studies did report results in an equivalent format (items m-2), not all studies measured the same part of the beach, thus making results incomparable. Specifically, the studies in Australia, Turkey, and the Azores, measured from the waterline into the backshore, whereas in Sri Lanka, quadrats were used with an unknown location on the beach (Aydin et al., 2016; Hardesty et al., 2017; Jang et al., 2018; Ríos et al., 2018). To address this universal challenge of incomparable results of beach surveys, international recommendations to harmonize beach study methods have been made (GESAMP, 2019).
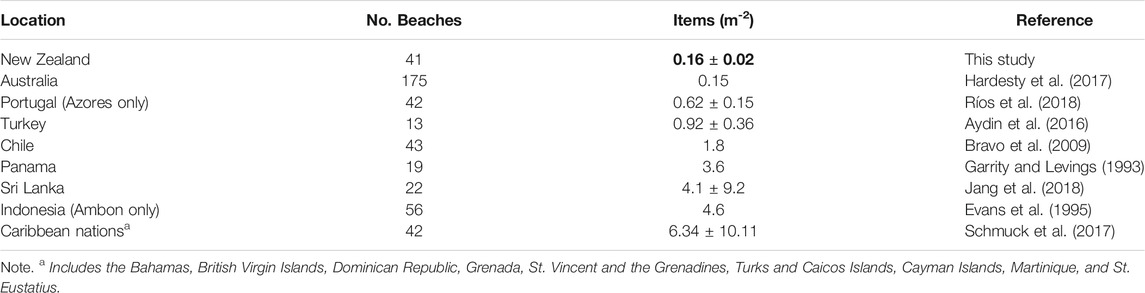
TABLE 1. New Zealand Beach Debris Density Results from This Study (Spring 2017; numbers in bold) Compared With Other Multi Regional Studies.
Studies from Malaysia and Japan show that the types of recreational activities performed on a beach may cause a similar amount of litter to become buried in the top layer of the beach sediment (Fauziah et al., 2015) as observed on the surface (Kusui and Noda, 2003). Moreover, on a remote Pacific Island, up to 68% of AMD items were found buried (Lavers and Bond, 2017), leading some to conclude that all AMD surveys should include measurement of items buried in the top layer (5 cm) of the beach (Serra-Gonçalves et al., 2019). In the present study, the sand was not raked nor sieved. Thus, it is likely that the actual density of AMD is higher than is represented in the results.
While debris count and weight on the SI were significantly higher, they also showed greater variation in density, weight, and material type. We did not detect a significant difference in the composition of AMD between the islands, but the SI had two additional types of debris: rubber and cloth. The overall proportion of plastics by count in this study is similar to other studies both in NZ and in Australia (Campbell et al., 2017; Hardesty et al., 2017), and plastic debris was present on all beaches where AMD was detected. Whereas the proportion of waterborne sources of AMD were comparable between the NI and SI, the NI had more land-based sources and AMD with an unknown source was only found on the SI.
Some variations between the islands and regions might be because the SI has more rivers discharging on the east coast as these are known pathways for land-based AMD (Lebreton et al., 2017; Schmidt et al., 2017; Meijer et al., 2021). Alternatively, variations could be related to circulation and currents in coastal waters, and AMD washing on and off the beach (Nagelkerken et al., 2001; Critchell and Lambrechts, 2016). The difference in currents and wind waves between the islands (Chiswell et al., 2015) may also result in mixed effects on the different types and sources of materials (Pieper et al., 2015). The circulation and currents in coastal waters which cause AMD to wash on and off the beach (Nagelkerken et al., 2001; Critchell and Lambrechts, 2016), also suggests a correlated abundance between certain types of AMD found on the beach and in the adjacent coastal waters (Thiel et al., 2013). On the beach, AMD can be buried and exhumed based on geophysical and environmental factors (Orr et al., 2005; Thiel et al., 2013), including tidal cycles and wind waves (Orr et al., 2005). Together, such variables cause AMD to move constantly, creating variability based on the specific local environmental circumstances at a particular time.
Regional Variations
This study did not find a relation of AMD density with population density as the highest mean density and weight was found in a region (Otago, SI) with a population of 239,313 (Stats, 2019) (Figure 3). In contrast, the most populated region (Auckland, NI) with a population of 1,590,261, showed the least AMD by weight and the lowest mean density by count was in a region (Waikato, NI) with a population of 466,110 (Stats, 2019). Waikato beaches are popular tourist destinations with many holiday homes close to the beach. Studies in Australia and Easter Island (Chile) showed less debris at beaches in proximity (< 5 km) to homes (Hardesty et al., 2017; Kiessling et al., 2017), perhaps a result of local stewardship. It is likely that debris on those beaches, specifically when frequented by tourists, are cleaned up more regularly than others (e.g., in Israel; Pasternak et al., 2017).
Similar to the differences between the islands, the contrast between the three regions on the SI (Figure 3) might also be due to geophysical and oceanic factors. Marlborough is located on the Northern part of the South Island, and its coast is exposed to different current and wind wave patterns. Marlborough is also located further away from (larger) watersheds, possibly being less affected by waterborne sources. Alternatively, the large proportion of rubber, cloth, and wood in Canterbury might be partially explained by local use factors, as beaches in Canterbury had more vehicles and horses on the beach than the other regions at the time of sampling.
A better understanding, of the combined reasons for the regional variations in AMD density, weight, composition, and source, is needed to develop tailored mitigative action. Therefore, the establishment of monitoring programs is critical to facilitate the understanding of variability in AMD (Schuyler et al., 2021). Since the conclusion of this baseline study, a nationwide citizen science beach monitoring project has started collecting seasonal AMD data on more than 100 NZ beaches1. The data collection is based on similar categorizations as this study and is according to international guidelines on the monitoring and assessment of litter in the ocean (GESAMP 2019), rendering future comparisons feasible. Based on the results of this study, we recommend measuring the effects of beach cleaning, watersheds, buried debris, ocean currents, and the type of activity on the beach.
In a meta-analysis of studies in the Southern Hemisphere, Barnes (2005) found that AMD density diminished on a latitudinal gradient from the equator towards the pole, with higher concentrations of AMD at the equator. Based on a review of 47 studies, this trend was explained by population density, which reduced further away from the equator (Barnes, 2005). Other studies have also shown that the proximity of population centers can result in higher debris loads on beaches (Santos et al., 2005), with even further intensification as the population density increases (Araújo et al., 2018). However, other studies show that the vicinity to population on increased AMD densities is not universally evident (Martinez-Ribes et al., 2007; Ribic et al., 2010; Lavers and Bond, 2017). Similarly, a comparison of AMD data from seven countries, although not all in the Southern Hemisphere, found that the determining drivers for AMD variability did not include population density (Schuyler et al., 2021). The results presented here, albeit on a subset of latitudes, also contradict Barnes findings by showing increasing AMD densities in higher latitudes with less population (SI). Thus, AMD density on NZ beaches cannot be explained by latitude nor population density.
Conclusion
A comprehensive and reproducible (standing crop) survey of AMD on beaches along the east coast of NZ provided a robust and reproducible baseline. This study indicated a substantial spatial variation in AMD density, mass, and source with a concomitant variation per island and region, requiring targeted mitigation efforts. Both AMD density and weight were significantly higher on the SI than on the more densely populated NI, showing increasing AMD densities with increasing latitudes. Debris on the NI showed significantly more land-based sources and the SI, more unknown sources, possibly resulting from inland sources through freshwater inputs. Further research explaining regional variances is needed and we recommend adding in monitoring the effects of beach cleaning, ocean currents, buried debris, watersheds, and type of activity on the beach. These results can be used as an evidence base for mitigative actions and can be compared against similar research in the future (e.g., NZ’s west coast and other countries).
Data Availability Statement
The raw data supporting the conclusions of this article will be made available by the authors, without undue reservation.
Author Contributions
EV, MC, and CH contributed to the conception and design of this study. EV collected the data, prepared figures, and wrote the manuscript. EV, MC, and CH analysed the data and all authors reviewed the manuscript.
Funding
This work was made possible through a University of Waikato Doctoral Scholarship and the Faculty of Science and Engineering Student Trust Fund (2017).
Conflict of Interest
The authors declare that the research was conducted in the absence of any commercial or financial relationships that could be construed as a potential conflict of interest.
Publisher’s Note
All claims expressed in this article are solely those of the authors and do not necessarily represent those of their affiliated organizations, or those of the publisher, the editors and the reviewers. Any product that may be evaluated in this article, or claim that may be made by its manufacturer, is not guaranteed or endorsed by the publisher.
Acknowledgments
We thank Charlie Watt for his assistance during the fieldwork.
Abbreviations
AMD, Anthropogenic Marine Debris; NZ, New Zealand; NI, North Island; SI, South Island.
Footnotes
1https://litterintelligence.org/
References
Araújo, M. C. B., Silva-Cavalcanti, J. S., and Costa, M. F. (2018). Anthropogenic Litter on Beaches with Different Levels of Development and Use: A Snapshot of a Coast in Pernambuco (Brazil). Front. Mar. Sci. 5, 233. doi:10.3389/fmars.2018.00233
Aydin, C., Guven, O., Salihoglu, B., and Kideys, A. E. (2016). The Influence of Land Use on Coastal Litter: An Approach to Identify Abundance and Sources in the Coastal Area of Cilician Basin, Turkey. Turk. J. Fisher. Aquatic Sci. 16 (1). doi:10.4194/1303-2712-v16_1_04
Barnes, D. K. A., Galgani, F., Thompson, R. C., and Barlaz, M. (2009). Accumulation and Fragmentation of Plastic Debris in Global Environments. Phil. Trans. R. Soc. B. 364 (1526), 1985–1998. doi:10.1098/rstb.2008.0205
Barnes, D. K. A. (2005). Remote Islands Reveal Rapid Rise of Southern Hemisphere Sea Debris. Scientific World J. 5, 915–921. doi:10.1100/tsw.2005.120
Boucher, J., and Friot, D. (2017). Primary Microplastics in the Oceans: A Global Evaluation of Sources. Gland: IUCN, Global Marine and Polar Programme, 43. doi:10.2305/iucn.ch.2017.01.en
Bravo, M., de los Ángeles Gallardo, M., Luna-Jorquera, G., Núñez, P., Vásquez, N., and Thiel, M. (2009). Anthropogenic Debris on Beaches in the SE Pacific (Chile): Results From a National Survey Supported by Volunteers. Mar. Pollut. Bull. 58 (11), 1718–1726. doi:10.1016/j.marpolbul.2009.06.017
Bridson, J. H., Patel, M., Lewis, A., Gaw, S., and Parker, K. (2020). Microplastic Contamination in Auckland (New Zealand) Beach Sediments. Mar. Pollut. Bull. 151, 110867. doi:10.1016/j.marpolbul.2019.110867
Browne, M. A., Chapman, M. G., Thompson, R. C., Amaral Zettler, L. A., Jambeck, J., and Mallos, N. J. (2015). Spatial and Temporal Patterns of Stranded Intertidal marine Debris: Is There a Picture of Global Change? Environ. Sci. Technol. 49 (12), 7082–7094. doi:10.1021/es5060572
Campbell, M. L., King, S., Heppenstall, L. D., van Gool, E., Martin, R., and Hewitt, C. L. (2017). Aquaculture and Urban marine Structures Facilitate Native and Non-indigenous Species Transfer Through Generation and Accumulation of Marine Debris. Mar. Pollut. Bull. 123 (1), 304–312. doi:10.1016/j.marpolbul.2017.08.040
Cheshire, A., Adler, E., Barbière, J., Cohen, Y., Evans, S., Jarayabhand, , et al. (2009). UNEP/IOC Guidelines on Survey and Monitoring of Marine Litter (Regional Seas Reports and Studies No. 186; IOC Technical Series No. 83). Nairobi: United Nations Environment Programme, Regional Seas Programme; Intergovernmental Oceanographic Commission, Integrated Coastal Area Management and Regional Programme, 83.
Chiswell, S. M., Bostock, H. C., Sutton, P. J., and Williams, M. J. (2015). Physical Oceanography of the Deep Seas Around New Zealand: A Review. New Zealand J. Mar. Freshw. Res. 49 (2), 286–317. doi:10.1080/00288330.2014.992918
Clunies-Ross, P., Smith, G., Gordon, K., and Gaw, S. (2016). Synthetic Shorelines in New Zealand? Quantification and Characterisation of Microplastic Pollution on Canterbury's Coastlines. New Zealand J. Mar. Freshw. Res. 50 (2), 317–325. doi:10.1080/00288330.2015.1132747
Coe, J. M., and Rogers, D. B. (1997). Marine Debris: Sources, Impacts, and Solutions. New York: Springer-Verlag, 440.
Critchell, K., and Lambrechts, J. (2016). Modelling Accumulation of Marine Plastics in the Coastal Zone; What are the Dominant Physical Processes? Estuarine, Coastal Shelf Sci. 171, 111–122. doi:10.1016/j.ecss.2016.01.036
Derraik, J. G. B. (2002). The Pollution of the marine Environment by Plastic Debris: A Review. Mar. Pollut. Bull. 44 (9), 842–852. doi:10.1016/S0025-326X(02)00220-5
Evans, S. M., Dawson, M., Day, J., Frid, C. L. J., Gill, M. E., Pattisina, L. A., et al. (1995). Domestic Waste and TBT Pollution in Coastal Areas of Ambon Island (Eastern Indonesia). Mar. Pollut. Bull. 30 (2), 109–115. doi:10.1016/0025-326X(94)00182-9
Fauziah, S., Liyana, I., and Agamuthu, P. (2015). Plastic Debris in the Coastal Environment: The Invincible Threat? Abundance of Buried Plastic Debris on Malaysian Beaches. Waste Manag. Res. 33 (9), 812–821. doi:10.1177/0734242X15588587
Galgani, F., Hanke, G., and Maes, T. (2015). “Global Distribution, Composition and Abundance of Marine Litter,” in Marine Anthropogenic Litter. Editors M. Bergman, L. Gutow, and M. Klages (Heidelberg: Springer), 29–56. doi:10.1007/978-3-319-16510-3_2
Garrity, S. D., and Levings, L. C. (1993). Marine Debris Along the Caribbean Coast of Panama. Mar. Pollut. Bull. 26 (6), 317–324. doi:10.1016/0025-326X(93)90574-4
GESAMP (2019). Guidelines for the Monitoring and Assessment of Plastic Litter in the Ocean (No. 99; GESAMP Reports and Studies). IMO/FAO/UNESCO-IOC/UNDIO/WMO/IAEA/UN/UNEP/UNDP/ISA Joint Group of Experts on the Scientific Aspects of Marine Environmental Protection. Available at: http://www.gesamp.org/publications/guidelines-for-the-monitoring-and-assessment-of-plastic-litter-in-the-ocean (Accessed June 1, 2021).
Gregory, M. R. (1978). Accumulation and Distribution of Virgin Plastic Granules on New Zealand Beaches. New Zealand J. Mar. Freshw. Res. 12 (4), 399–414. doi:10.1080/00288330.1978.9515768
Gregory, M. R. (1999). Marine Debris: Notes from Chatham Island, and Mason and Doughboy Bays, Stewart Island. Tane. 37, 201–210.
Hardesty, B. D., Lawson, T., van der Velde, T., Lansdell, M., and Wilcox, C. (2017). Estimating Quantities and Sources of Marine Debris at a continental Scale. Front. Ecol. Environ. 15 (1), 18–25. doi:10.1002/fee.1447
Hayward, B. W. (1984). Rubbish Trends—Beach Litter Surveys at Kawerua, 1974—1982. Tane. 30, 209–217.
Hayward, B. W. (1999). A Load of Old Rubbish—Kawerua beach Litter Surveys, 1974-1997. Tane. 37 (15), 13.
Hayward, B. W., Grenfell, H. R., Sabaa, A., and Hayward, J. J. (2003). Recent Benthic Foraminifera From Offshore Taranaki, New Zealand. New Zealand J. Geology. Geophys. 46 (4), 489–518. doi:10.1080/00288306.2003.9515024
Hidalgo-Ruz, V., Honorato-Zimmer, D., Gatta-Rosemary, M., Nuñez, P., Hinojosa, I. A., and Thiel, M. (2018). Spatio-Temporal Variation of Anthropogenic marine Debris on Chilean Beaches. Mar. Pollut. Bull. 126, 516–524. doi:10.1016/j.marpolbul.2017.11.014
Hidalgo-Ruz, V., and Thiel, M. (2015). “The Contribution of Citizen Scientists to the Monitoring of Marine Litter,” in Marine Anthropogenic Litter. Editors M. Bergmann, L. Gutow, and M. Klages (Heidelberg: Springer), 429–447. doi:10.1007/978-3-319-16510-3_16
Hoornweg, D., and Bhada-Tata, P. (2012). What a Waste. A Global Review of Solid Waste Management. (No. 15; Urban Development Series). Washington, DC: World Bank. Available at: http://hdl.handle.net/10986/17388 (Accessed June 1, 2021).
Jambeck, J. R., Geyer, R., Wilcox, C., Siegler, T. R., Perryman, M., Andrady, A., et al. (2015). Plastic Waste Inputs From Land into the Ocean. Science. 347 (6223), 768–771. doi:10.1126/science.1260352
Jang, Y. C., Ranatunga, R. R. M. K. P., Mok, J. Y., Kim, K. S., Hong, S. Y., Choi, Y. R., et al. (2018). Composition and Abundance of Marine Debris Stranded on the Beaches of Sri Lanka: Results From the First Island-wide Survey. Mar. Pollut. Bull. 128, 126–131. doi:10.1016/j.marpolbul.2018.01.018
Kaladharan, P., Vijayakumaran, K., Vijayakumaran, K., Singh, V. V., Prema, D., Asha, P. S., et al. (2017). Prevalence of Marine Litter along the Indian Beaches : A Preliminary Account on its Status and Composition. J.Mar.Biol.Ass.India. 59 (1), 19–24. doi:10.6024/jmbai.2017.59.1.1953-03
Kiessling, T., Salas, S., Mutafoglu, K., and Thiel, M. (2017). Who Cares about Dirty Beaches? Evaluating Environmental Awareness and Action on Coastal Litter in Chile. Ocean Coastal Management. 137, 82–95. doi:10.1016/j.ocecoaman.2016.11.029
Kordella, S., Geraga, M., Papatheodorou, G., Fakiris, E., and Mitropoulou, I. M. (2013). Litter Composition and Source Contribution for 80 Beaches in Greece, Eastern Mediterranean: A Nationwide Voluntary Clean-Up Campaign. Aquat. Ecosystem Health Management. 16 (1), 111–118. doi:10.1080/14634988.2012.759503
Kusui, T., and Noda, M. (2003). International Survey on the Distribution of Stranded and Buried Litter on Beaches along the Sea of Japan. Mar. Pollut. Bull. 47 (1–6), 175–179. doi:10.1016/S0025-326X(02)00478-2
Lau, W. W. Y., Shiran, Y., Bailey, R. M., Cook, E., Stuchtey, M. R., Koskella, J., et al. (2020). Evaluating Scenarios Toward Zero Plastic Pollution. Science. 369, 1455–1461. doi:10.1126/science.aba9475
Lavers, J. L., and Bond, A. L. (2017). Exceptional and Rapid Accumulation of Anthropogenic Debris on One of the World's Most Remote and Pristine Islands. Proc. Natl. Acad. Sci. USA. 114 (23), 6052–6055. doi:10.1073/pnas.1619818114
Lebreton, L. C. M., van der Zwet, J., Damsteeg, J.-W., Slat, B., Andrady, A., and Reisser, J. (2017). River Plastic Emissions to the World's Oceans. Nat. Commun. 8, 15611. doi:10.1038/ncomms15611
Lippiatt, S., Opfer, S., and Arthur, C. (2013). Marine Debris Monitoring and Assessment. Silver Spring, MD: NOAA Technical Memorandum NOS-OR&R-46, 82.
Martinez-Ribes, L., Basterretxea, G., Palmer, M., and Tintoré, J. (2007). Origin and Abundance of Beach Debris in the Balearic Islands. Sci. Mar. 71 (2), 305–314. doi:10.3989/scimar.2007.71n2305
McCormick, A. R., and Hoellein, T. J. (2016). Anthropogenic Litter Is Abundant, Diverse, and Mobile in Urban Rivers: Insights from Cross-Ecosystem Analyses Using Ecosystem and Community Ecology Tools. Limnol. Oceanogr. 61 (5), 1718–1734. doi:10.1002/lno.10328
Meijer, L. J. J., van Emmerik, T., van der Ent, R., Schmidt, C., and Lebreton, L. (2021). More Than 1000 Rivers Account for 80% of Global Riverine Plastic Emissions into the Ocean. Sci. Adv. 7 (18), eaaz5803. doi:10.1126/sciadv.aaz5803
Murray, C. C., Maximenko, N., and Lippiatt, S. (2018). The Influx of Marine Debris from the Great Japan Tsunami of 2011 to North American Shorelines. Mar. Pollut. Bull. 132, 26–32. doi:10.1016/j.marpolbul.2018.01.004
Nagelkerken, I., Wiltjer, G. A. M. T., Debrot, A. O., and Pors, L. P. J. J. (2001). Baseline Study of Submerged Marine Debris at Beaches in Curaçao, West Indies. Mar. Pollut. Bull. 42 (9), 786–789. doi:10.1016/s0025-326x(01)00091-1
NIWA (2017). Wave Action. Water & Atmosphere. Available at: https://www.niwa.co.nz/news/wave-action (Accessed February 6, 2017).
OECD (2017). Environmental Performance Reviews—New Zealand 2017 [Environmental Performance Reviews]. Available at: https://read.oecd-ilibrary.org/environment/oecd-environmental-performance-reviews-new-zealand-2017_9789264268203-en#page1 (Accessed June 1, 2021).
OECD (2021). Municipal Waste (Indicator). Available at: http://data.oecd.org/waste/municipal-waste.htm (Accessed June 1, 2021).
Office of the Prime Minister’s Chief Science Advisor (2019). Rethinking Plastics in Aotearoa New Zealand. Available at: https://cpb-ap-se2.wpmucdn.com/blogs.auckland.ac.nz/dist/f/688/files/2020/02/Rethinking-Plastics-in-Aotearoa-New-Zealand_Full-Report_8-Dec-2019-PDF-1.pdf (Accessed June 1, 2021).
Orr, M., Zimmer, M., Jelinski, D. E., and Mews, M. (2005). Wrack Deposition on Different Beach Types: Spatial and Temporal Variation in the Pattern of Subsidy. Ecology. 86 (6), 1496–1507. doi:10.1890/04-1486
Pasternak, G., Zviely, D., Ribic, C. A., Ariel, A., and Spanier, E. (2017). Sources, Composition and Spatial Distribution of Marine Debris Along the Mediterranean Coast of Israel. Mar. Pollut. Bull. 2, 1036–1045. doi:10.1016/j.marpolbul.2016.11.023
Pearson, K. (1900). X. On the Criterion that a Given System of Deviations from the Probable in the Case of a Correlated System of Variables is Such that it Can Be Reasonably Supposed to Have Arisen from Random Sampling. Lond. Edinb. Dublin Philosophical Mag. J. Sci. 50 (302), 157–175. doi:10.1080/14786440009463897
Pickrill, R. A., and Mitchell, J. S. (1979). Ocean Wave Characteristics Around New Zealand. New Zealand J. Mar. Freshw. Res. 13 (4), 501–520. doi:10.1080/00288330.1979.9515827
Pieper, C., Amaral-Zettler, L., Law, K. L., Loureiro, C. M., and Martins, A. (2019). Application of Matrix Scoring Techniques to Evaluate Marine Debris Sources in the Remote Islands of the Azores Archipelago. Environ. Pollut. 249, 666–675. doi:10.1016/j.envpol.2019.03.084
Pieper, C., Ventura, M. A., Martins, A., and Cunha, R. T. (2015). Beach Debris in the Azores (NE Atlantic): Faial Island as a First Case Study. Mar. Pollut. Bull. 101 (2), 575–582. doi:10.1016/j.marpolbul.2015.10.056
Rangel-Buitrago, N., Williams, A., Anfuso, G., Arias, M., and Gracia C., A. (2017). Magnitudes, Sources, and Management of Beach Litter along the Atlantico Department Coastline, Caribbean Coast of Colombia. Ocean Coastal Management. 138, 142–157. doi:10.1016/j.ocecoaman.2017.01.021
Ribic, C. A., Sheavly, S. B., Rugg, D. J., and Erdmann, E. S. (2010). Trends and Drivers of Marine Debris on the Atlantic Coast of the United States 1997-2007. Mar. Pollut. Bull. 60 (8), 1231–1242. doi:10.1016/j.marpolbul.2010.03.021
Ríos, N., Frias, J. P. G. L., Rodríguez, Y., Carriço, R., Garcia, S. M., Juliano, M., et al. (2018). Spatio-Temporal Variability of Beached Macro-Litter on Remote Islands of the North Atlantic. Mar. Pollut. Bull. 133, 304–311. doi:10.1016/j.marpolbul.2018.05.038
Ruxton, G. D. (2006). The Unequal Variance T-Test Is an Underused Alternative to Student's T-Test and the Mann-Whitney U Test. Behav. Ecol. 17 (4), 688–690. doi:10.1093/beheco/ark016
Ryan, P. G., Lamprecht, A., Swanepoel, D., and Moloney, C. L. (2014). The Effect of Fine-Scale Sampling Frequency on Estimates of Beach Litter Accumulation. Mar. Pollut. Bull. 88 (1), 249–254. doi:10.1016/j.marpolbul.2014.08.036
Santos, I. R., Friedrich, A. C., Wallner-Kersanach, M., and Fillmann, G. (2005). Influence of Socio-Economic Characteristics of Beach Users on Litter Generation. Ocean Coastal Management. 48 (9–10), 742–752. doi:10.1016/j.ocecoaman.2005.08.006
Schmidt, C., Krauth, T., and Wagner, S. (2017). Export of Plastic Debris by Rivers into the Sea. Environm. Sci. Technol. 51 (21), 12246–12253. doi:10.1021/acs.est.7b02368
Schmuck, A. M., Lavers, J. L., Stuckenbrock, S., Sharp, P. B., and Bond, A. L. (2017). Geophysical Features Influence the Accumulation of Beach Debris on Caribbean Islands. Mar. Pollut. Bull. 121 (1–2), 45–51. doi:10.1016/j.marpolbul.2017.05.043
Schuyler, Q., Wilcox, C., Lawson, T., Ranatunga, R. R. M. K. P., Hu, C.-S., and Hardesty, B. (2021). Human Population Density is a Poor Predictor of Debris in the Environment. Front. Environ. Sci. 9, 583454. doi:10.3389/fenvs.2021.583454
Schuyler, Q., Willis, K., Lawson, T., Mann, V., Wilcox, C., and Hardesty, B. D. (2018). Handbook of Survey Methodology—Plastics Leakage (1.2). Canberra: CSIRO. Available at: https://research.csiro.au/marinedebris/resources/ (Accessed June 1, 2021).
Serra-Gonçalves, C., Lavers, J. L., and Bond, A. L. (2019). Global Review of Beach Debris Monitoring and Future Recommendations. Environ. Sci. Technol. 53 (21), 12158–12167. doi:10.1021/acs.est.9b01424
Sheavly, S. B., and Register, K. M. (2007). Marine Debris & Plastics: Environmental Concerns, Sources, Impacts and Solutions. J. Polym. Environ. 15 (4), 301–305. doi:10.1007/s10924-007-0074-3
Sherman, P., and van Sebille, E. (2016). Modeling Marine Surface Microplastic Transport to Assess Optimal Removal Locations. Environ. Res. Lett. 11 (1), 014006. doi:10.1088/1748-9326/11/1/014006
Slavin, C., Grage, A., and Campbell, M. L. (2012). Linking Social Drivers of Marine Debris With Actual Marine Debris on Beaches. Mar. Pollut. Bull. 64 (8), 1580–1588. doi:10.1016/j.marpolbul.2012.05.018
Smith, S. D. A., and Markic, A. (2013). Estimates of Marine Debris Accumulation on Beaches Are Strongly Affected by the Temporal Scale of Sampling. Plos One. 8 (12), e83694. doi:10.1371/journal.pone.0083694
Stats, N. Z. (2019). 2018 Census Population and Dwelling Counts. Available at: https://www.stats.govt.nz/tools/2018-census-place-summaries/new-zealand#population-counts (Accessed June 1, 2021).
Thiel, M., Hinojosa, I. A., Miranda, L., Pantoja, J. F., Rivadeneira, M. M., and Vásquez, N. (2013). Anthropogenic Marine Debris in the Coastal Environment: A Multi-Year Comparison between Coastal Waters and Local Shores. Mar. Pollut. Bull. 71 (1–2), 307–316. doi:10.1016/j.marpolbul.2013.01.005
Tomlinson, A. I. (1992). “Precipitation and the Atmosphere,” in Waters of New Zealand. Editor M. P. Mosley (Wellington: New Zealand Hydrological Society Inc), 63–74.
Tudor, D. T., and Williams, A. T. (2004). Development of a 'Matrix Scoring Technique' to Determine Litter Sources at a Bristol Channel Beach. J. Coast Conserv. 10 (1–2), 119–127. doi:10.1007/bf02818948
Velander, K., and Mocogni, M. (1999). Beach Litter Sampling Strategies: is There a 'Best' Method? Mar. Pollut. Bull. 38 (12), 1134–1140. doi:10.1016/S0025-326X(99)00143-5
Verlis, K. M., and Wilson, S. P. (2020). Paradise Trashed: Sources and Solutions to marine Litter in a Small Island Developing State. Waste Management. 103, 128–136. doi:10.1016/j.wasman.2019.12.020
Welch, B. L. (1947). The Generalization of `Student's' Problem When Several Different Population Variances are Involved. Biometrika. 34 (1–2), 28–35. doi:10.1093/biomet/34.1-2.28
Whiting, S. D. (1998). Types and Sources of Marine Debris in Fog Bay, Northern Australia. Mar. Pollut. Bull. 36 (11), 904–910. doi:10.1016/S0025-326X(98)00066-6
Williams, A. T., and Tudor, D. T. (2001). Litter Burial and Exhumation: Spatial and Temporal Distribution on a Cobble Pocket beach. Mar. Pollut. Bull. 42 (11), 1031–1039. doi:10.1016/S0025-326X(01)00058-3
Keywords: marine debris, marine litter, marine pollution, New Zealand, mismanaged waste, beach survey, baseline study, plastic pollution
Citation: van Gool E, Campbell M, Wallace P and Hewitt CL (2021) Marine Debris on New Zealand Beaches - Baseline Data to Evaluate Regional Variances. Front. Environ. Sci. 9:700415. doi: 10.3389/fenvs.2021.700415
Received: 26 April 2021; Accepted: 19 July 2021;
Published: 28 July 2021.
Edited by:
Anabela Cachada, University of Porto, PortugalReviewed by:
Danilo Balthazar, Paulista University, BrazilRebeca Castro, Fluminense Federal University, Brazil
Catharina Diogo Pieper, University of the Azores, Portugal
Copyright © 2021 van Gool, Campbell, Wallace and Hewitt. This is an open-access article distributed under the terms of the Creative Commons Attribution License (CC BY). The use, distribution or reproduction in other forums is permitted, provided the original author(s) and the copyright owner(s) are credited and that the original publication in this journal is cited, in accordance with accepted academic practice. No use, distribution or reproduction is permitted which does not comply with these terms.
*Correspondence: Ella van Gool, elladorien@gmail.com