- 1State Key Laboratory of Freshwater Ecology and Biotechnology, Institute of Hydrobiology, Chinese Academy of Sciences, Wuhan, China
- 2University of Chinese Academy of Sciences, Beijing, China
- 3Meteorological Bureau of Dali Prefecture, Dali, China
- 4Key Laboratory of Aquatic Botany and Watershed Ecology, Chinese Academy of Sciences, Wuhan, China
- 5Institute of Eastern-Himalaya Biodiversity Research, Dali University, Dali, China
- 6Ecology Department, College of Bioscience and Biotechnology, Hunan Provincial Key Laboratory of Rural Ecosystem Health in Dongting Lake Area, Hunan Agricultural University, Changsha, China
Water level plays a crucial role in the function and social services of lakes. Studies on historical changes in water level and its eco-social function can give insights into future water conservation and management. In this study, interannual and seasonal changes in the water level of Erhai Lake were analyzed from 1952 to 2019 to explore water level responses to human activities and climate change. The time series was divided into three distinct periods, i.e., 1952–1971, 1972–2003, and 2004–2019. Results showed that the water level and fluctuation amplitude differed among the different time periods, i.e., 1965.8 and 1.3 m (1952–1971), 1964.4 and 1.9 m (1972–2003), and 1965.2 and 1.2 m (1972–2003), respectively. The construction and operation of a hydroelectric power plant along the outlet river significantly decreased the water level and increased fluctuation amplitude in the 1972–2003 period. Since 2004, due to the implementation of local government water level management laws for Erhai Lake, the water level has remained relatively high, with moderate fluctuation amplitude. In addition, compared to the increase in water level amplitude in response to increased wet season (May–October) precipitation in the 1952–1971 period, response sensitivity increased in the 1972–2003 period, but became non-significant in the 2004–2019 period. In regard to the multi-timescale relationship between water level and precipitation, precipitation decreased by 89 mm in the 2004–2019 period compared with that from 1952 to 1971, and artificial water-level regulation resulted in a time-lag of 2, 3–3.5, and 4 months between water level and precipitation during the 1952–1971, 1972–2003, and 2004–2019 periods, respectively. The eco-social aspects of changes in water level are discussed below, and water level regulation from an ecological perspective is recommended to gain economic returns in the future.
Introduction
The hydrological regime plays a vital role in regulating the structure and function of aquatic ecosystems (Poff et al., 1997; Bunn and Arthington 2002; Coops et al., 2003). It affects water flow, water level, nutrients, and temperature, which are closely related to biological activities and behaviors, such as spawning, rearing, and recruitment in fish (Copp 1989; Junk et al., 1989; Sparks 1995; Humphries et al., 1999) and growth and colonization of aquatic plants (Quennerstedt 1958; Zhao et al., 2012; Evtimova and Donohue 2016). In addition, lakes, reservoirs, and rivers are widely used for drinking water, irrigation, fish farming, hydropower, and recreation through artificial adjustments of the hydrological regime (Riis and Hawes, 2002; Coops et al., 2003; Cott et al., 2008; Reid et al., 2013).
Climate change and human activities have altered the distribution of water resources and the hydrological regime of many aquatic systems on a global scale over the past decades (Leira and Cantonati 2008; Haddeland et al., 2014; Tornqvist et al., 2014; Kong et al., 2017), with implications for ecosystem services. For example, the Mediterranean basin has been experiencing longer and warmer summers, with shifts in seasonal and annual precipitation since 1950, resulting in water resource shortages and water quality deterioration (Cramer et al., 2018). Therefore, increasing conflicts have emerged in view of water resource utilization between human welfare and ecological demands, particularly in developing countries that rely on water resources for irrigation, fisheries, and hydropower. In developed countries, however, increasing attention has been paid to the ecological aspects of water resources. For example, in the United States, many dams had been removed to restore natural hydrological regimes for aquatic ecosystems (Born et al., 1998; Pohl 2002). In China, water resource management has begun to focus more on ecological concerns, as shown by the debate on whether to build a dam between the Yangtze River and Poyang Lake, the largest lake in its basin (Xie 2018).
Human activity, especially artificial water level regulation, can have serious impacts on lake ecosystem services, including changes in water supply, water depuration services, flood control, freshwater biodiversity, climate regulation, and recreation (Coops and Hosper 2002; Riis and Hawes 2002; Fang et al., 2006). Water level has emerged as a dynamic reflection of hydrological processes and water balance of lake ecosystems and can have a dominant effect on lake structure and function (Coops et al., 2003; Leira and Cantonati 2008; Gownaris et al., 2018). The amplitude, timing, and frequency of water level plays crucial roles in determining macrophyte depth distribution (Liu et al., 2018; Liu et al., 2020; Zheng et al., 2020), water trophic states (Zohary and Ostrovsky 2011; Bakker and Hilt 2016; da Costa et al., 2016; Kong et al., 2017), and invasion of aquatic organisms in lake ecosystems (Van Geest et al., 2005). Climate change and human activities are two major driving forces affecting water level variation (Paillisson and Marion 2011; Tao et al., 2019). Climate change can impact water level via temperature, precipitation, evaporation, and glacier melting (Ludwig et al., 2014). However, the driving factors of water level change differ among regions, and vary in different types of lakes, leading to distinct seasonal and spatial characteristics of water level change (Qin, 1999; Tao et al., 2019). The water levels of flood plain lakes are usually affected by the water level of their main rivers, and the operation of upstream hydrological projects (Van Geest et al., 2005; Yuan et al., 2015; Xu et al., 2020). In addition, given the usually well-developed river networks in these catchment and high wet season precipitation, severe seasonal water level fluctuations can occur over the yearly cycle. Nevertheless, in plateau lakes, which are generally deep-water tectonic lakes, the temperature is relatively low and water levels are subject to smaller variations than flood plain lakes. In Yunnan, the plateau lakes located in the upper reaches of the Yangtze and Pearl rivers provide significant ecosystem services, including water provision for the plateau region and maintenance of biological diversity, as well as important areas for tourism and ethnic culture. Erhai Lake is the second largest plateau lake in the northwest Yunnan-Guizhou plateau. To the best of our knowledge, however, limited research has been conducted on the characteristics of historical variations in the water levels of plateau lakes in Yunnan (Zhu and Yao 2012; He et al., 2019), and no previous related work has been reported on Erhai Lake.
In this study, we investigated long-term changes in the water level of Erhai Lake from 1952 to 2019 under a warming climate with lower precipitation and in response to rapid social development over the past half century (Duan and Tao 2012; Li et al., 2017; Chen et al., 2018). During the 1952–1971 period, the lake had a natural water level fluctuation regime. In 1972, however, a new hydropower station was constructed, and water levels were regulated artificially for hydropower until 2003 (Yuan and Wang 1985). In 2004, emphasis shifted to the ecological management of water level fluctuation, limited the operation range of water level between 1964.3 and 1966.0 m according to the promulgated “Amendment to Regulations on Erhai lake” (Li et al., 2011). Thus, the aims of this study were to: 1) Compare the interannual and seasonal variation characteristics of water level in three periods over the past 68 years; 2) Compare the sensitivity of water level increase in response to accumulated wet season precipitation over the three periods; and 3) Compare the multi-timescale relationship between water level and precipitation in the three periods.
Materials and Methods
Study Area
Erhai Lake (25°36′–25°58′N and 100°15′–100°18′E), located in the Yunnan-Guizhou plateau in southwestern China (Figure 1), is a typical fault lake with a maximum depth of 21 m (mean depth of 10.5 m), area of 252 km2, and volume of 29.59 × 108 m³ (Chen et al., 2018). The region has a subtropical monsoon climate, with an annual mean temperature of 15°C, wet season precipitation of 870 mm (May–October), and dry season precipitation of 170 mm (November–May). Moist air comes from the western Pacific and Indian oceans and temperature and precipitation respond to changes in global climate. The catchment covers an area of 2,565 km2 (Hu et al., 2018; Lin et al., 2020). Water supply mainly originates from precipitation but also partially from groundwater and snowmelt from the Cangshan Mountains. In total, 117 rivers and streams ultimately drain into Erhai Lake. The only outlet river (Xi’er River) is located in the south, with a total length of 23 km and total depth drop of 610 m, eventually running into a branch of the Mekong River (Yuan and Wang, 1985; Guo et al., 2001).
Data Sources
Daily water level data (average water level over 24 h) from 1952 to 2019 were obtained from the Erhai Hydrological Station. Daily climate data (exact precipitation) were obtained from the Dali Meteorological Bureau.
Data Analysis
For analysis of long-term trends in interannual water levels, the 68-year study period was divided into three periods, i.e., 1952–1971, 1972–2003, and 2004–2019, corresponding to phases with natural water levels, artificial water level adjustment for hydropower, and ecological water level management, respectively. The mean, maximum, and minimum water levels each year were averaged for the three periods, together with the occurrence times of the maximum and minimum water levels. The durations of increasing water levels were calculated based on the occurrence time of the minimum and maximum water level each year and averaged for the three periods. For analysis of seasonal water levels within a year, mean monthly water levels were averaged for the three periods. Precipitation data were analyzed following the same methods as that of water levels. Seasonal precipitation was divided into a wet season (May–October) and dry season (November–April). Regression analysis was used to explore the relationship between the magnitude of water level fluctuations and cumulative precipitation in the wet season.
Cross-wavelet analysis, which combines cross-spectrum analysis and wavelet transforms (Hudgins et al., 1993; Torrence and Compo 1998), was applied to explore the multi-timescale relationship between water level and precipitation. Cross-wavelet analysis can compare the frequencies of two time series and identify asynchrony of two time series over certain periods and across certain time ranges (Grinsted et al., 2004). According to Veleda et al. (2012), two cross-wavelet transforms, i.e., time series
The modulus can be understood as the cross-wavelet power, which is used to assess the similarity of wavelet power of two series in the time-frequency (or time-scale) domain:
In a geometric sense, cross-wavelet power can be interpreted as local covariance between the time series at each time and frequency.
Instantaneous or local phase advance of any periodic component of (
When converted to a certain angle in the interval [−π,π], this is equal to the difference of each phase, that is, Phase x—Phase y (Ge 2008; Aguiarconraria-Conraria and Joana Soares, 2011). An angle less than (or greater than) π/2 indicates that the two sequences are moving in-phase (or anti-phase). Thus, instantaneous time is the origin and frequency (or period) in question. The sign of the phase difference indicates which series is the leading one in the relationship.
Monte Carlo simulation, with red noise as the standard spectrum, was used to test if synchronization was statistically significant (Torrence and Compo 1998). In the application of cross-wavelet analysis, as some water level data between 1986 and 1989 were missing, we divided the 1972–2003 period into 1972–1985 and 1990–2003. Statistical analyses were performed using R 3.4.2 (R Core Team, 2017).
Results
Interannual and Seasonal Changes in Water Levels in Erhai Lake From 1952 to 2019
The water levels of Erhai Lake changed substantially from 1952 to 2019. Over the last 68 years, the mean annual water level was 1965.0 m, with the lowest (1962.7 m) and highest (1966.0 m) water levels in 1982 and 1961, respectively. The annual water levels remained relatively stable (1965.5–1966.0 m) during the period from 1952 to 1974, then decreased sharply from 1975 to 1982 (1965.3–1962.7 m), and finally increased gradually from 1983 to 2019 (1963.0–1964.9 m) (Figure 2).
The annual water levels were highest (mean 1965.8 m) and fluctuated naturally during the 1952–1971 period. The annual water levels were lowest (mean 1964.4 m) during the 1972–2003 period when the water level was regulated for hydroelectric power, and mid-range (mean 1965.2 m) during the 2004–2019 period when the water level was adjusted with an emphasis on ecological management (Figure 3A). The variations in interannual and seasonal water levels were 0.5 and 1.3 m during the 1952–1971 period, 3.0 and 1.9 m during the 1972–2003 period, and 1.0 and 1.2 m during the 2004–2019 period, respectively, (Figure 2, 3B).
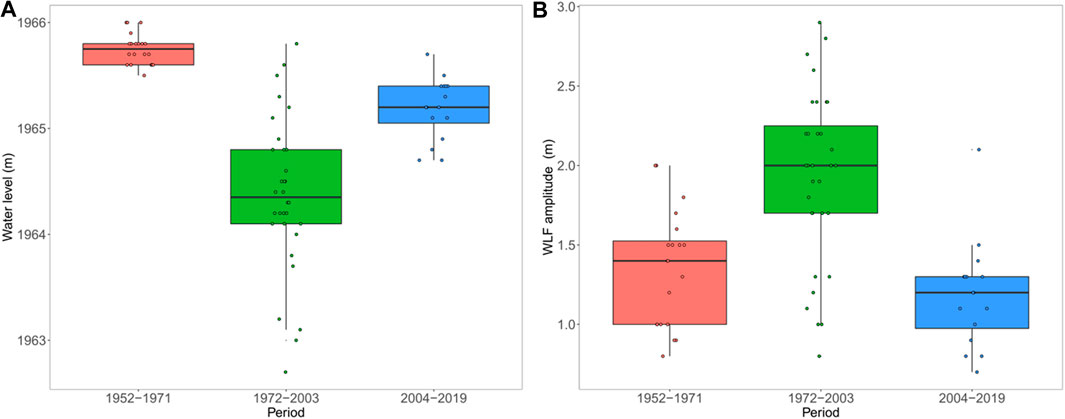
FIGURE 3. Box plots on water level (A) and its fluctuation (WLF) (B) in Erhai Lake in 1952–1971, 1972–2003, and 2004–2019 periods. Lower, middle, and upper lines of box indicate first quantile, median, and third quantile of data, respectively. Vertical line indicates values within 1.5 interquartile range below the lower quartile and above the upper quartile. Dots are yearly data.
In general, the seasonal water level was lowest from May to June and highest from September to November (Figure 4). During the 1952–1971, 1972–2003, and 2004–2019 periods, the lowest and highest seasonal water levels occurred on 9 May and 12 September, 24 May and 21 October, and 27 May and 3 November, respectively, indicating sequential time-lags among the three periods and prolonged duration from the lowest to highest water levels (Table 1).
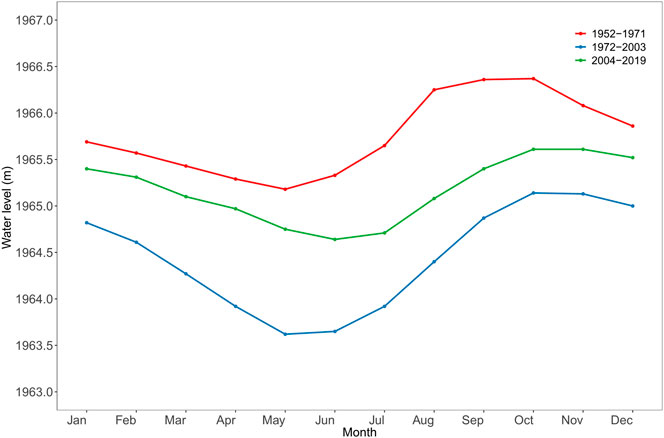
FIGURE 4. Seasonal changes in water levels in Erhai Lake in 1952–1971, 1972–2003, and 2004–2019 periods.
Interannual and Seasonal Changes in Precipitation in Erhai Lake Basin From 1952 to 2019
Annual precipitation from 1952 to 2019 ranged from 650 to 1,391 mm, respectively, with a mean of 1,040 mm (Figure 5). Whole year, wet season (May–October), and dry season (November–April) precipitation amounts were 1,068, 917, and 148 mm during the 1952–1971 period, 1,053, 875, and 185 mm during the 1972–2003 period, and 979, 804, and 172 during the 1952–1971 period, respectively (Figure 6). Precipitation also changed seasonally, with the maximum (218 mm) and minimum (13 mm) in August and December, respectively (Figure 7). Compared with the 1952–1971 period, a reduction (97 mm) in precipitation in May and October largely contributed to the decrease in annual precipitation during the 2004–2019 period (Figure 6).
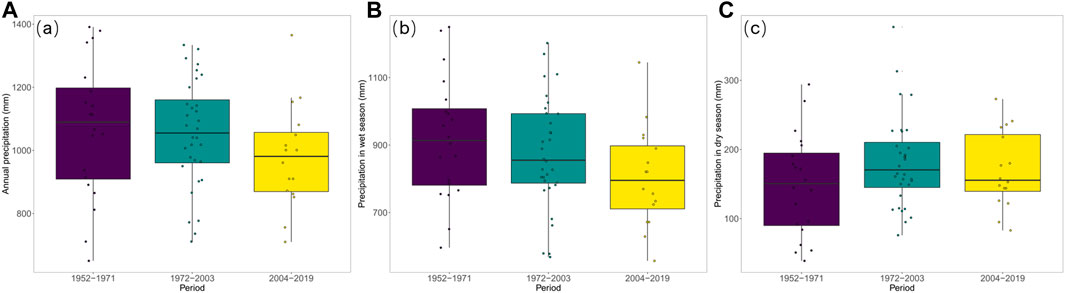
FIGURE 6. Box plots for annual (A), wet season (B), and dry season (C) precipitation in 1952–1971, 1972–2003, and 2004–2019 periods. Lower, middle, and upper lines of box indicate first quantile, median, and third quantile of the data, respectively. Vertical line indicates values within 1.5 interquartile range below the lower quartile and above the upper quartile. Dots are yearly data.
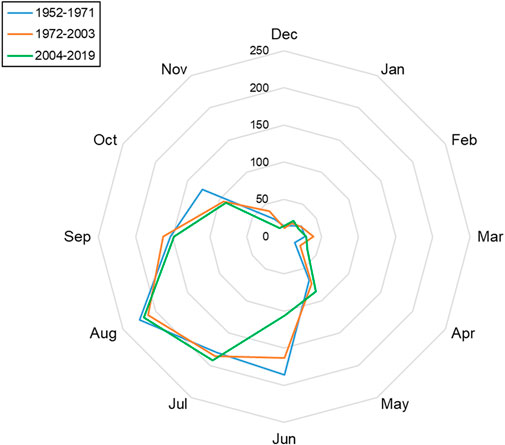
FIGURE 7. Radar chart for monthly precipitation (mm) in Erhai Lake catchment in 1952–1971, 1972–2003, and 2004–2019 periods.
Relationship Between Water Level and Precipitation From 1952 to 2019
In the wet season (May–October) in the 1952–1971 and 1972–2003 periods, increased water levels were positively correlated with the amount of precipitation. Both the slope and coefficient of determination of the relationship were higher in the 1972–2003 period (slope = 0.003, R2 = 0.59, p < 0.001) than in the 1952–1971 period (slope = 0.001, R2 = 0.40, p = 0.003; Figure 8). No significant relationship was found between the increase in water level and amount of precipitation in the 2004–2019 period (Figure 8).
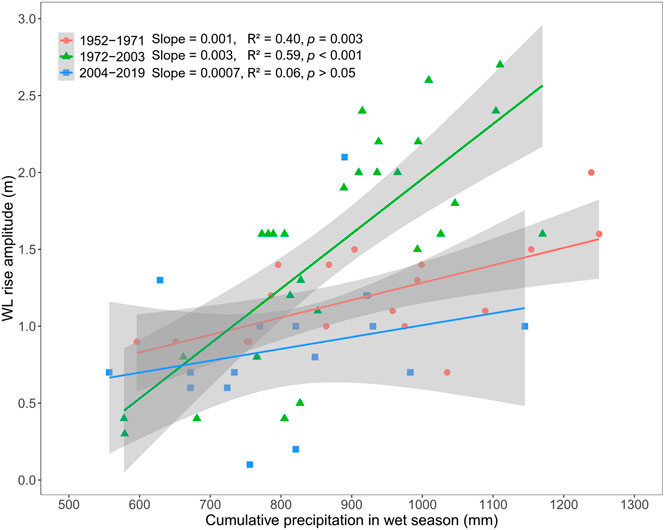
FIGURE 8. Increase in water level (WL) plots against amount of cumulative precipitation in same wet season (May–October) in 1952–1971, 1972–2003, and 2004–2019 periods. Dots are yearly data.
Over the past 68 years, water level and precipitation have varied seasonally (but not synchronically) over a periodic 12-month scale (Figure 9). The changes in water level lagged precipitation by 2 months during the 1952–1971 period, by 3 months during the 1972–1985 period, 3.5 months during the 1990–2003 period, and 4 months during the 2004–2019 period (Figure 9).
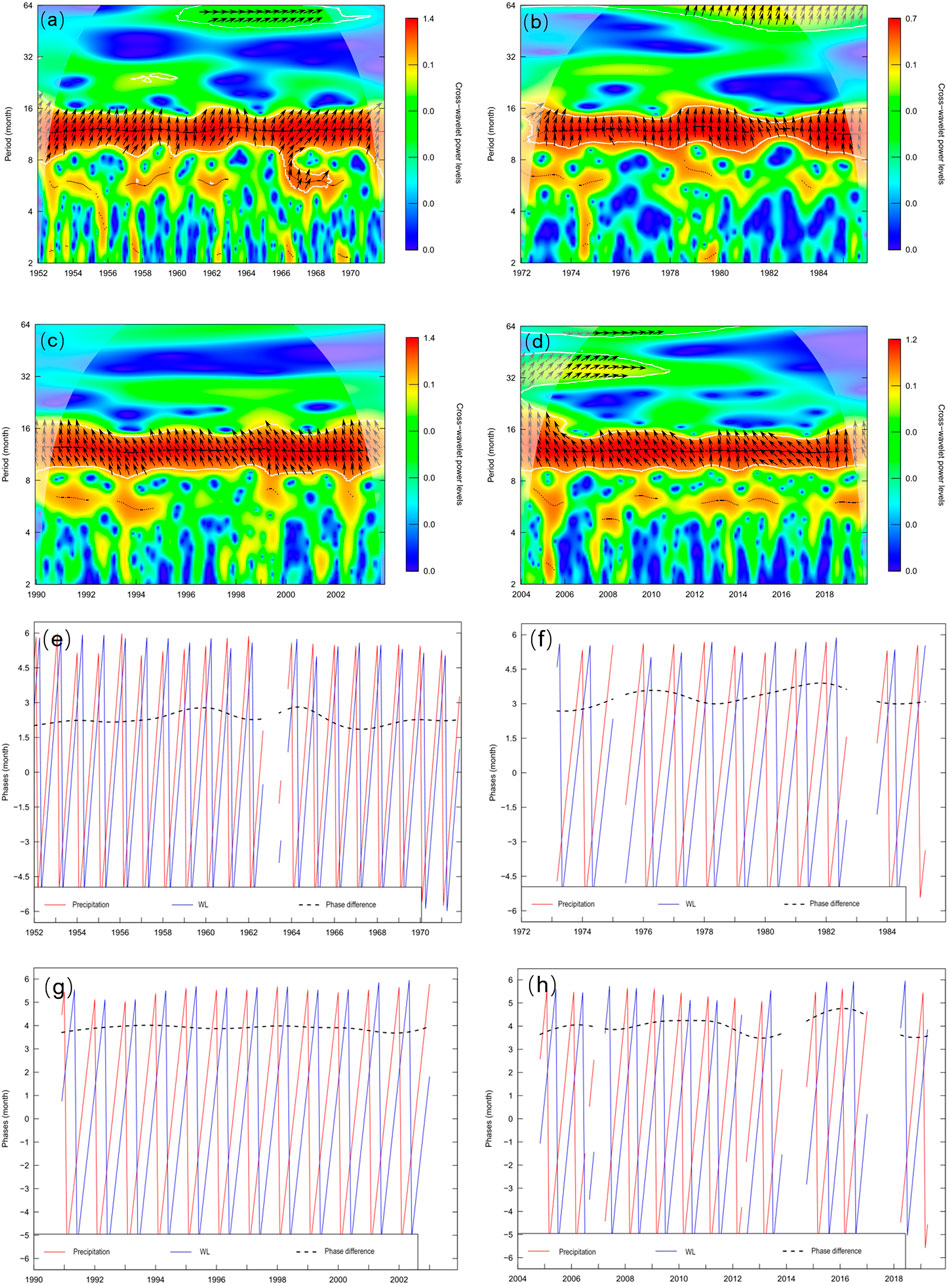
FIGURE 9. Wavelet power analysis of precipitation and water level (WL) in different periods. (A–D) Wavelet coherency-image colors represent wavelet power spectrum. Blue U-shaped line is cone of influence, below which edge effects limit confidence in results. Horizontal arrows on right indicate precipitation is in-phase with water level, on left indicate that two series are in antiphase, upward indicates that precipitation is 1/4 cycle ahead of water level, otherwise, 1/4 cycle behind. Arrows are plotted only with white contour lines indicating significance (concerning null hypothesis of white noise process) at the 1% level. Darker colors indicate stronger coherence between two series. (E–H) Phases and phase differences. Red line represents WL phase, blue line represents precipitation phase, and black dashed line represents phase differences.
The relationship between water level and precipitation was primarily reflected in two aspects. First, during the wet seasons, the sensitivity of the water level increase response to cumulative precipitation in the 2004–2019 period declined compared to the other two periods (Figure 8). Secondly, the time delay between water level and precipitation became increasingly obvious with time (Figure 9).
Discussion
Our results revealed distinct characteristics of water-level fluctuations in Erhai Lake over the last seven decades in conjunction with climate change and human activities. The water level from 1952 to 2019 was divided into three periods, namely 1952–1971, 1972–2003, and 2004–2019. Human activities have changed in magnitude and occurring dates of the rise and fall of water level and led to the asynchrony of water-level fluctuation and seasonal precipitation.
In the 1952–1971 period, Erhai Lake had the highest mean annual water level, with a natural seasonal fluctuation of 1.3 m. In the wet season (May–October), the increase in water level was closely related to the amount of precipitation, implying that water-level fluctuations followed a natural process. The high water levels in the wet season often caused flooding in the villages near the lakeshore, and many villages and urban areas were located at elevations below the maximum water level (1967 m) during these years. In the Yunnan-Guizhou Plateau, many lakes are surrounded by high mountains, and thus there is very limited land for human activities. Therefore, during the wet season, these areas were prone to flood disasters. About 25.46 km2 of lakeside farmland located at an elevation of 1965.69–1967.19 m was frequently inundated, resulting in a reduction in production, especially in large areas of farmland north of the lake, which flooded every year during the wet season (Li et al., 1986). Higher water levels are also unfavorable for aquatic vegetation growth in many lakes (Engel and Nichols 1994; O’Farrell et al., 2010). The high water levels in Erhai Lake resulted in highly turbid water in the littoral zone and a colonization depth of submerged vegetation of only 3 m (Li et al., 1963).
In the 1972–2003 period, the water level of Erhai Lake was artificially regulated with the primary purpose to produce hydroelectric power. As such, the outlet river was artificially deepened, and a dam was constructed to control outflow. The lowest annual water level and largest seasonal variation (1.9 m) were observed during this period. During the dry season, the power supply capacity of run-of-river hydroelectric stations in local region decreases, but the cascade power station of Xi’er River compensates for the lack of power supply, resulting in the water level of Erhai Lake dropped sharply (Li et al., 1986). Compared to the 1952–1971 period, the lower mean annual water level in the 1972–2003 period was mainly induced by the artificial control of the Xi’er River outlet, as annual precipitation did not differ significantly between the two periods. The lower water level corresponded to a smaller water surface area according to lake basin morphology, and therefore the same amount of wet season precipitation caused a larger increase in water levels compared to the 1952–1971 period, thus the water level response was more sensitive to precipitation. From a social perspective, the lower water level resulted in a larger precipitation containing capacity, which helped alleviate local village and urban flooding under heavy precipitation conditions. On the other hand, the lower water level led to larger beach areas, allowing further human intrusion along the lakeshore, e.g., fishing, farming, housing construction, which further impacted the local ecosystem. In addition, during the wet season, the decline in water level inevitably led to a decrease in tributary depth and an increase in steep river slopes, and the bridges, channels, and embankments of some tributaries around the lake were damaged, and houses and farmland were threatened (Li et al., 1986). In addition, lower water levels impacted normal hydroelectric power generation as the water level could not reach the minimum water level required (1964.97 m), which also affected agricultural irrigation to a certain extent (Li et al., 1986). From an ecological perspective, the larger fluctuation in water level decreased the retention time for water exchange, and the lower water level resulted in an increase in area suitable for the colonization of submerged vegetation (Riis and Hawes, 2002; Litvinov and Roshchupko, 2007), which are two key processes beneficial for water clarification. Furthermore, the decrease in water level made it difficult for certain endemic fish species, e.g., Barbodes exigua, Barbodes daliensis, and Schizothorax taliensis, to migrate and spawn; notably, various wintering fish holes along the eastern lakeshore became isolated or dry, leaving fish unprotected during severe winters (Du and Li 2001). The increased seasonal variation in water level also caused some coastal gravel beaches to dry up during the spawning period, leading to the loss of spawning grounds, extinction of endemic fish species, reduction in endemic fish resources, and eventual decrease in fishery yield and economy (Li et al., 1986; Wu and Wang 1999). However, the lower water levels promoted submerged vegetation growth, which covered 30–40% of the lake surface and reached a water depth of 7 m (Li 1983; Dai 1984; Ni et al., 2011). This increased water quality in terms of transparency (Chen et al., 2018; Dong et al., 2018; Lin et al., 2020). Beklioglu et al. (2006) reported a similar response to decreased water levels in five shallow lakes in Turkey, i.e., expansion of submerged vegetation and improvement in water quality. Nevertheless, the increase in submerged plants led to an increase in the populations of Cyprinus carpio and Carassius auratus auratus, which prefer to spawn among submerged vegetation, and accounted for 65–80% of total fish production in the 1970s in Erhai Lake (Wu and Wang 1999). Eventually, fish community composition changed, and endemic fish species were seriously impacted.
In the 2004–2019 period, the water level of Erhai Lake was regulated with greater regard to ecological restoration, and water levels were between those observed in the 1952–1971 and 1972–2003 periods, with a water level fluctuation of 1.2 m. From 2004, a large part of the emergent lakeshore was again flooded when the water level increased, and the ecological function of the lakeshore was partly restored, e.g., expansion of wetlands. In the 2004–2019 period, the increase in water level in the wet season was not significantly correlated with precipitation. This was partly due to the decrease in water input caused by the 89-mm reduction in precipitation compared with the 1952–1971 period, as well as local government regulation of the Erhai water level through the Xi’er hydropower station. During this period, the function of the Xi’er hydropower station changed from generating electricity to a tool for regulating the water level. From 2004 to 2008, the water levels rose sharply to dilute nutrient concentrations, and from 2009 to 2019, the water level was regulated to a nearly natural water level fluctuating state. Furthermore, the mid-range water level was controlled artificially with a small fluctuation of 1.2 m, which was beneficial for the function of industrial and agricultural water supply, as well as lakeshore scenery and tourism. Since 2005, local tourism has experienced an unprecedented boom, with tourism revenue increasing from 2.39 billion RMB in 2005 to 29.26 billion RMB in 2017 (Li et al., 2020). In addition, the mid-range water levels and eutrophication induced an increase in emergent and floating plants (e.g., Nelumbium nucifera and Trapa maximowiczii) as well as invasion of pest species such as Eichhornia crassipes and Myriophyllum aquaticum, but a decline in submerged vegetation (Tan et al., 2017; Zhao et al., 2020). The maximum colonization of submerged macrophytes is less than 5 m and coverage has shrunk to 8% (Li et al., 2011; Fu et al., 2013). Changes in hydrological regimes are also reported to induce the invasion of alien species, loss of biodiversity, and alteration in ecological functions (Moyle and Mount 2007).
We observed an even longer time-lag between water levels and precipitation in respect of the multi-timescale relationship, as well as a time-lag in the occurrence of the lowest and highest water levels in the three periods and an extended interval between the lowest and highest water levels. Therefore, the time-lag in the water levels in combination with the reduction in water input and the controlled water levels might disentangle the response of water level to precipitation since 2004. These results indicate that the water level rhythm has changed to a certain extent in the context of human activity (hydroelectric generation), intervention, and climate change (reduced precipitation). In particular, the longer time-lag between water levels and precipitation reflects the effects of human intervention, which may change the pattern and extent of hydrological regime responses to extreme weather events. In addition, altered multi-timescale relationships may change the timing and duration of lakeside and wetland flooding, eventually affecting the ecosystem services of the Erhai Lake catchment.
Conclusion
In this study, interannual and seasonal variations in the water level of Erhai Lake from 1952 to 2019 were examined to assess the responses of water level to climate change and human activities. The time series was divided into three distinct periods, i.e., 1952–1971, 1972–2003, and 2004–2019.
The results showed that the construction and operation of a hydroelectric plant along the only outlet river greatly decreased the water level and increased its fluctuation amplitude during the 1972–2003 period. However, the water level remained relatively high and demonstrated moderate fluctuation from 2004, when local economic development became increasingly dependent on tourism and there was a greater emphasis on ecological restoration. In addition, water level responses to precipitation in the wet season were most sensitive in the 1972–2003 period, and a gradually increasing time-lag was found between them from a multi-time scale perspective.
In summary, the changes in water level and the responses of water level to climate change (precipitation) in Erhai Lake were largely determined by the hydroelectric plant in the 1972–2003 period, and jointly impacted by decreased precipitation and artificial water level regulation in the 2004–2019 period. We also explored the subsequent social and ecological changes in Erhai Lake and recommend that the water level be regulated from an ecological perspective to gain economic returns in the future.
Data Availability Statement
The original contributions presented in the study are included in the article/Supplementary Material, further inquiries can be directed to the corresponding authors.
Author Contributions
All authors listed have made a substantial, direct, and intellectual contribution to the work and approved it for publication.
Funding
This study was supported by the Key Project (Grant No. 31930074) and Normal Project (Grant No. 31870446) of the National Natural Science Foundation of China and State Key Laboratory of Freshwater Ecology and Biotechnology (2019FBZ01).
Conflict of Interest
The authors declare that the research was conducted in the absence of any commercial or financial relationships that could be construed as a potential conflict of interest.
References
Aguiar-Conraria, L. s., and Joana Soares, M. (2011). Business Cycle Synchronization and the Euro: A Wavelet Analysis. J. Macroeconomics 33, 477–489. doi:10.1016/j.jmacro.2011.02.005
Bakker, E. S., and Hilt, S. (2016). Impact of Water-Level Fluctuations on Cyanobacterial Blooms: Options for Management. Aquat. Ecol. 50, 485–498. doi:10.1007/s10452-015-9556-x
Beklioglu, M., Altinayar, G. T., and Tan, C. O. (2006). Water Level Control over Submerged Macrophyte Development in Five Shallow Lakes of Mediterranean Turkey. archiv_hydrobiologie 166, 535–556. doi:10.1127/0003-9136/2006/0166-0535
Born, S. M., Genskow, K. D., Filbert, T. L., Hernandez-Mora, N., Keefer, M. L., and White, K. A. (1998). Socioeconomic and Institutional Dimensions of Dam Removals: The Wisconsin Experience. Environ. Manage. 22, 359–370. doi:10.1007/s002679900111
Bunn, S. E., and Arthington, A. H. (2002). Basic Principles and Ecological Consequences of Altered Flow Regimes for Aquatic Biodiversity. Environ. Manage. 30, 492–507. doi:10.1007/s00267-002-2737-0
Chen, X., Qian, X., Li, X., Wei, Z., and Hu, S. (2018). Long-term Trend of Eutrophication State of Lake Erhai in 1988-2013 and Analyses of its Socio-Economic Drivers. J. Lake Sci. 30, 70–78. doi:10.18307/2018.0107
Coops, H., and Hosper, S. H. (2002). Water-level Management as a Tool for the Restoration of Shallow Lakes in the Netherlands. Lake Reservoir Manage. 18, 293–298. doi:10.1080/07438140209353935
Coops, H., Beklioglu, M., and Crisman, T. L. (2003). The Role of Water-Level Fluctuations in Shallow lake Ecosystems - Workshop Conclusions. Hydrobiologia 506-509, 23–27. doi:10.1023/b:Hydr.0000008595.14393.77
Copp, G. H. (1989). The Habitat Diversity and Fish Reproductive Function of Floodplain Ecosystems. Environ. Biol. Fish. 26, 1–27. doi:10.1007/Bf00002472
Cott, P. A., Sibley, P. K., Somers, W. M., Lilly, M. R., and Gordon, A. M. (2008). A Review of Water Level Fluctuations on Aquatic Biota with an Emphasis on Fishes in Ice-Covered Lakes1. JAWRA 44, 343–359. doi:10.1111/j.1752-1688.2007.00166.x
Cramer, W., Guiot, J., Fader, M., Garrabou, J., Gattuso, J.-P., Iglesias, A., et al. (2018). Climate Change and Interconnected Risks to Sustainable Development in the Mediterranean. Nat. Clim Change 8, 972–980. doi:10.1038/s41558-018-0299-2
da Costa, M. R. A., Attayde, J. L., and Becker, V. (2016). Effects of Water Level Reduction on the Dynamics of Phytoplankton Functional Groups in Tropical Semi-arid Shallow Lakes. Hydrobiologia 778, 75–89. doi:10.1007/s10750-015-2593-6
Dai, Q. (1984). Preliminary Study on Aquatic Vegetation of Erhai Lake in Yunnan. Trans. Oceanol. Limnol. 4, 31–41.
Dong, J., Zhou, Q., Gao, Y., Gu, Q., Li, G., and Song, L. (2018). Long-term Effects of Temperature and Nutrient Concentrations on the Phytoplankton Biomass in three lakes with Differing Trophic Statuses on the Yungui Plateau, China. Ann. Limnol. - Int. J. Lim. 54, 9. doi:10.1051/limn/2017031
Du, B., and Li, Y. (2001). Danger Risk to Fish Diversity in Erhai lake and Proposals to Dispel it. Res. Environ. Sci. 14, 42–44. doi:10.13198/j.res.2001.03.45.dubh.013
Duan, X., and Tao, Y. (2012). The Climate Change of Yunnan over the Last 50 Years. J. Trop. Meteorology 28, 243–250. doi:10.3969/j.issn.1004-4965.2012.02.012
Engel, S., and Nichols, S. A. (1994). Aquatic Macrophyte Growth in a Turbid Windswept Lake. J. Freshw. Ecol. 9, 97–109. doi:10.1080/02705060.1994.9664436
Evtimova, V. V., and Donohue, I. (2016). Water-level Fluctuations Regulate the Structure and Functioning of Natural Lakes. Freshw. Biol. 61, 251–264. doi:10.1111/fwb.12699
Fang, J., Wang, Z., Zhao, S., Li, Y., Tang, Z., Yu, D., et al. (2006). Biodiversity Changes in the Lakes of the Central Yangtze. Front. Ecol. Environ. 4, 369–377. doi:10.1890/1540-9295(2006)004[0369:BCITLO]2.0.CO;2
Fu, H., Yuan, G., Zhong, J., Cao, T., Ni, L., and Xie, P. (2013). Environmental and Ontogenetic Effects on Intraspecific Trait Variation of a Macrophyte Species across Five Ecological Scales. PLoS One 8, e62794. doi:10.1371/journal.pone.0062794
Ge, Z. (2008). Significance Tests for the Wavelet Cross Spectrum and Wavelet Linear Coherence. Ann. Geophys. 26, 3819–3829. doi:10.5194/angeo-26-3819-2008
Gownaris, N. J., Rountos, K. J., Kaufman, L., Kolding, J., Lwiza, K. M. M., and Pikitch, E. K. (2018). Water Level Fluctuations and the Ecosystem Functioning of Lakes. J. Great Lakes Res. 44, 1154–1163. doi:10.1016/j.jglr.2018.08.005
Grinsted, A., Moore, J. C., and Jevrejeva, S. (2004). Application of the Cross Wavelet Transform and Wavelet Coherence to Geophysical Time Series. Nonlin. Process. Geophys. 11, 561–566. doi:10.5194/npg-11-561-2004
Guo, H. C., Liu, L., Huang, G. H., Fuller, G. A., Zou, R., and Yin, Y. Y. (2001). A System Dynamics Approach for Regional Environmental Planning and Management: a Study for the Lake Erhai Basin. J. Environ. Manage. 61, 93–111. doi:10.1006/jema.2000.0400
Haddeland, I., Heinke, J., Biemans, H., Eisner, S., Flörke, M., Hanasaki, N., et al. (2014). Global Water Resources Affected by Human Interventions and Climate Change. Proc Natl Acad Sci USA 111, 3251–3256. doi:10.1073/pnas.1222475110
He, K., Gao, W., Duan, C., Zhu, Y., Liu, C., Zhang, W., et al. (2019). Water Level Variation and Its Driving Factors in Lake Dianchi, Fuxian and Yangzong During 1988-2015. J. Lake Sci. 31, 1379–1390. doi:10.18307/2019.0504
Hu, Y. n., Peng, J., Liu, Y., and Tian, L. (2018). Integrating Ecosystem Services Trade-Offs with Paddy Land-To-Dry Land Decisions: A Scenario Approach in Erhai Lake Basin, Southwest China. Sci. Total Environ. 625, 849–860. doi:10.1016/j.scitotenv.2017.12.340
Hudgins, L., Friehe, C. A., and Mayer, M. E. (1993). Wavelet Transforms and Atmopsheric Turbulence. Phys. Rev. Lett. 71, 3279–3282. doi:10.1103/PhysRevLett.71.3279
Humphries, P., King, A. J., and Koehn, J. D. (1999). Fish, Flows and Flood plains: Links between Freshwater Fishes and Their Environment in the Murray-Darling River System, Australia. Environ. Biol. Fishes 56, 129–151. doi:10.1023/A:1007536009916
Junk, W. J., Bayley, P. B., and Sparks, R. E. (1989). The Flood Pulse Concept in River-Floodplain Systems. CAN. J. FISH AQUAT. SCI. 106, 110–127.
Kong, X., He, Q., Yang, B., He, W., Xu, F., Janssen, A. B. G., et al. (2017). Hydrological Regulation Drives Regime Shifts: Evidence from Paleolimnology and Ecosystem Modeling of a Large Shallow Chinese lake. Glob. Change Biol. 23, 737–754. doi:10.1111/gcb.13416
Leira, M., and Cantonati, M. (2008). Effects of Water-Level Fluctuations on Lakes: an Annotated Bibliography[J]. Springer Netherlands, 171–184. doi:10.1007/978-1-4020-9192-6_16
Li, S., Yu, M., Li, G., Zeng, J., Che, J., Gao, B., et al. (1963). Limnological Survey of the Lakes of Yunnan Plateau. Oceanol. Limnol. Sin. 5, 87–114.
Li, J., Wu, L., and Yang, H. (1986). Construction of cascade Power Station in Xier River and Environmental Problems in Erhai Area. Yunnan Water Power 2, 20–28.
Li, E., Wang, X., Cai, X., Wang, X., and Zhao, S. (2011). Features of Aquatic Vegetation and the Influence Factors in Erhai Lakeshore Wetland. Jounal Lake Sci. 23, 738–746. doi:10.18307/2011.0511
Li, Y., Li, B., Zhang, K., Zhu, J., and Yang, Q. (2017). Study on Spatiotemporal Distribution Characteristics of Annual Precipitation of Erhai Basin. J. China Inst. Water Resour. Hydropower Res. 3, 234–240. doi:10.13244/j.cnki.jiwhr.2017.03.012
Li, J., Bai, Y., and Alatalo, J. M. (2020). Impacts of Rural Tourism-Driven Land Use Change on Ecosystems Services Provision in Erhai Lake Basin, China. Ecosystem Serv. 42, 101081. doi:10.1016/j.ecoser.2020.101081
Lin, S.-S., Shen, S.-L., Zhou, A., and Lyu, H.-M. (2020). Sustainable Development and Environmental Restoration in Lake Erhai, China. J. Clean. Prod. 258, 120758. doi:10.1016/j.jclepro.2020.120758
Litvinov, A. S., and Roshchupko, V. F. (2007). Long-term and Seasonal Water Level Fluctuations of the Rybinsk Reservoir and Their Role in the Functioning of its Ecosystem. Water Resour. 34, 27–34. doi:10.1134/S0097807807010034
Liu, L., Guan, Y.-T., Qin, T.-J., Wang, Y.-Y., Li, H.-L., and Zhi, Y.-B. (2018). Effects of Water Regime on the Growth of the Submerged Macrophyte Ceratophyllum Demersum at Different Densities. J. Freshw. Ecol. 33, 45–56. doi:10.1080/02705060.2017.1422043
Liu, Q., Liu, J., Liu, H., Liang, L., Cai, Y., Wang, X., et al. (2020). Vegetation Dynamics under Water-Level Fluctuations: Implications for Wetland Restoration. J. Hydrol. 581, 124418. doi:10.1016/j.jhydrol.2019.124418
Ludwig, F., van Slobbe, E., and Cofino, W. (2014). Climate Change Adaptation and Integrated Water Resource Management in the Water Sector. J. Hydrol. 518, 235–242. doi:10.1016/j.jhydrol.2013.08.010
Moyle, P. B., and Mount, J. F. (2007). Homogenous Rivers, Homogenous Faunas. Proc. Natl. Acad. Sci. 104, 5711–5712. doi:10.1073/pnas.0701457104
Ni, Z., Wang, S., Jin, X., Jiao, L., and Li, Y. (2011). Study on the Evolution and Characteristics of Eutrophication in the Typical Lakes on Yunnan-Guizhou Plateau. Acta Scientiae Circumstantiae 31, 2681–2689. doi:10.13671/j.hjkxxb.2011.12.019
O’Farrell, I., Izaguirre, I., Chaparro, G., Unrein, F., Sinistro, R., Pizarro, H., et al. (2010). Water Level as the Main Driver of the Alternation between a Free-Floating Plant and a Phytoplankton Dominated State: a Long-Term Study in a Floodplain lake. Aquat. Sci. 73, 275–287. doi:10.1007/s00027-010-0175-2
Paillisson, J.-M., and Marion, L. (2011). Water Level Fluctuations for Managing Excessive Plant Biomass in Shallow Lakes. Ecol. Eng. 37, 241–247. doi:10.1016/j.ecoleng.2010.11.017
Poff, N. L., Allan, J. D., Bain, M. B., Karr, J. R., Prestegaard, K. L., Richter, B. D., et al. (1997). The Natural Flow Regime. Bioscience 47, 769–784. doi:10.2307/1313099
Pohl, M. M. (2002). Bringing Down Our Dams: Trends in American Dam Removal Rationales. J. Am. Water Resour. Assoc 38, 1511–1519. doi:10.1111/j.1752-1688.2002.tb04361.x
Qin, B. (1999). A Preliminary Investigation of Lake Evolution in 20-century in Inland Mainland Asia with Relation to the Global Warming. J. Lake Sci. 11, 11–19. doi:10.18307/1999.0102
Quennerstedt, N. (1958). Effect of Water Level Fluctuation on lake Vegetation. 13, 901–906. doi:10.1080/03680770.1956.11895487
R Core Team (2017). R: A Language and Environment for Statistical Computing. Vienna, Austria: R Foundation for Statistical ComputingAvailable at: https://www.R-project.org/.
Reid, J. R. W., Colloff, M. J., Arthur, A. D., and McGinness, H. M. (2013). Influence of Catchment Condition and Water Resource Development on Waterbird Assemblages in the Murray-Darling Basin, Australia. Biol. Conservation 165, 25–34. doi:10.1016/j.biocon.2013.05.009
Riis, T., and Hawes, I. (2002). Relationships between Water Level Fluctuations and Vegetation Diversity in Shallow Water of New Zealand Lakes. Aquat. Bot. 74, 133–148. doi:10.1016/s0304-3770(02)00074-8
Sparks, R. E. (1995). Need for Ecosystem Management of Large Rivers and Their Floodplains. Bioscience 45, 168–182. doi:10.2307/1312556
Tan, W., Liu, P., Liu, Y., Yang, S., and Feng, S. (2017). A 30-Year Assessment of Phytoplankton Blooms in Erhai Lake Using Landsat Imagery: 1987 to 2016. Remote Sensing 9, 1265. doi:10.3390/rs9121265
Tao, S., Fang, J., Ma, S., Cai, Q., Xiong, X., Tian, D., et al. (2019). Changes in China's Lakes: Climate and Human Impacts. Natl. Sci. Rev. doi:10.1093/nsr/nwz103
Törnqvist, R., Jarsjö, J., Pietroń, J., Bring, A., Rogberg, P., Asokan, S. M., et al. (2014). Evolution of the Hydro-Climate System in the Lake Baikal basin. J. Hydrol. 519, 1953–1962. doi:10.1016/j.jhydrol.2014.09.074
Torrence, C., and Compo, G. P. (1998). A Practical Guide to Wavelet Analysis. Bull. Amer. Meteorol. Soc. 79, 61–78. doi:10.1175/1520-0477(1998)079<0061:Apgtwa>2.0.Co;2
Van Geest, G. J., Coops, H., Roijackers, R. M. M., Buijse, A. D., and Scheffer, M. (2005). Succession of Aquatic Vegetation Driven by Reduced Water-Level Fluctuations in Floodplain Lakes. J. Appl. Ecol. 42, 251–260. doi:10.1111/j.1365-2664.2005.00995.x
Veleda, D., Montagne, R., and Araujo, M. (2012). Cross-Wavelet Bias Corrected by Normalizing Scales. J. Atmos. Ocean. Technol. 29, 1401–1408. doi:10.1175/jtech-d-11-00140.1
Wu, Q., and Wang, Y. (1999). On the Succession of Aquatic Communities in Erhai Lake. Jounal Lake Sci. 11, 267–273.
Xie, P. (2018). Biodiversity Crisis in the Yangtze River From Point Views of Evolution and Ecology. Beijing: Science Press, 75–87.
Xu, D., Lyon, S. W., Mao, J., Dai, H., and Jarsjö, J. (2020). Impacts of Multi-Purpose Reservoir Construction, Land-Use Change and Climate Change on Runoff Characteristics in the Poyang Lake basin, China. J. Hydrol. Reg. Stud. 29, 100694. doi:10.1016/j.ejrh.2020.100694
Yuan, Y., Zeng, G., Liang, J., Huang, L., Hua, S., Li, F., et al. (2015). Variation of Water Level in Dongting Lake over a 50-year Period: Implications for the Impacts of Anthropogenic and Climatic Factors. J. Hydrol. 525, 450–456. doi:10.1016/j.jhydrol.2015.04.010
Zhao, D., Jiang, H., Cai, Y., and An, S. (2012). Artificial Regulation of Water Level and its Effect on Aquatic Macrophyte Distribution in Taihu Lake. PLoS One 7, e44836. doi:10.1371/journal.pone.0044836
Zhao, H., Kong, D., Fan, Y., Tan, Z., Wang, Z., Wu, W., et al. (2020). Analysis on the Situation and Changing Trend of Aquatic Macrophytes in the Riparian Zone of Erhai Lake. Environ. Sci. Surv. 39, 20–25. doi:10.13623/j.cnki.hkdk.2020.02.003
Zheng, L., Zhan, P., Xu, J., Xu, L., Tan, Z., and Wang, X. (2020). Aquatic Vegetation Dynamics in Two Pit Lakes Related to Interannual Water Level Fluctuation. Hydrological Process. 34, 2645–2659. doi:10.1002/hyp.13757
Zhu, S., and Yao, L. (2012). Analysis on the Variation Trend and Affecting Factors of Water Level in Dianchi Lake. J. Water Resour. Water Eng. 23, 182–184.
Keywords: water level, water resource, climate change, Erhai lake, ecosystem service
Citation: Wen Z, Ma Y, Wang H, Cao Y, Yuan C, Ren W, Ni L, Cai Q, Xiao W, Fu H, Cao T and Zhang X (2021) Water Level Regulation for Eco-social Services Under Climate Change in Erhai Lake Over the Past 68 years in China. Front. Environ. Sci. 9:697694. doi: 10.3389/fenvs.2021.697694
Received: 20 April 2021; Accepted: 21 May 2021;
Published: 07 June 2021.
Edited by:
Peng Bao, Institute of Urban Environment (CAS), ChinaReviewed by:
Xiaodong QU, China Institute of Water Resources and Hydropower Research, ChinaGuoqi WEN, Agriculture and Agri-Food Canada (AAFC), Canada
Copyright © 2021 Wen, Ma, Wang, Cao, Yuan, Ren, Ni, Cai, Xiao, Fu, Cao and Zhang. This is an open-access article distributed under the terms of the Creative Commons Attribution License (CC BY). The use, distribution or reproduction in other forums is permitted, provided the original author(s) and the copyright owner(s) are credited and that the original publication in this journal is cited, in accordance with accepted academic practice. No use, distribution or reproduction is permitted which does not comply with these terms.
*Correspondence: Te Cao, Y2FvdGVAaWhiLmFjLmNu; Xiaolin Zhang, emhhbmd4bEBpaGIuYWMuY24=