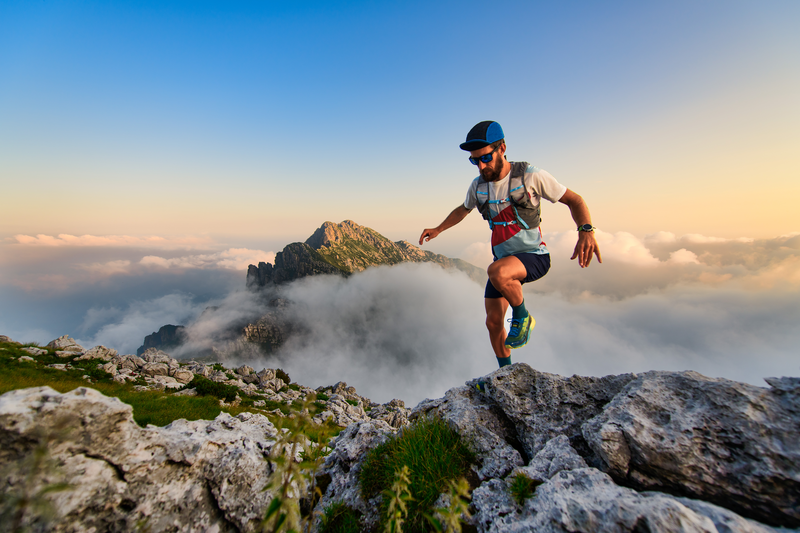
94% of researchers rate our articles as excellent or good
Learn more about the work of our research integrity team to safeguard the quality of each article we publish.
Find out more
ORIGINAL RESEARCH article
Front. Environ. Sci. , 23 June 2021
Sec. Toxicology, Pollution and the Environment
Volume 9 - 2021 | https://doi.org/10.3389/fenvs.2021.693112
This article is part of the Research Topic Environmental Behavior and Control of Emerging Chemical Pollutants View all 5 articles
The formation of leachate is one of the inevitable consequences associated with the operation of landfills. In addition to the study of physicochemical composition, toxicity is an important parameter taken into account, among others because of the need to subject leachate to treatment before discharge into the environment. The aim of this study was to determine the degree of leachate contamination using LPI and toxicity index. An analysis of the relationship between the toxic effect on plants and the calculated LPI values was also carried out to assess whether they could provide a measure of leachate toxicity when selecting a treatment method. The research conducted was to determine the degree of leachate contamination using LPI and toxicity index. An analysis of the relationship between the toxic effect on plants and the calculated LPI values was also carried out to assess whether they could provide a measure of leachate toxicity when selecting a treatment method. Calculated values of the leachate pollution index showed that leachates from selected Polish landfills are characterized by low and medium levels of LPI values. The variation in index values obtained was particularly evident between active and closed landfills and was related to the physicochemical composition of leachates. In closed landfills this value ranged from 7.4 to 11.1, while in active landfills from 12.9 to 15.9. The variation in index values obtained was particularly evident between active and inactive landfills and was related to the physicochemical composition of leachates. Phytotoxicity tests showed that leachate at low concentrations can promote plant growth. At higher concentrations (50 and 100%), leachates caused inhibition of root and shoot growth, which correlated with high LPI values. The results confirmed the relationship between the toxic effect on plants and the LPI values, so it can be considered as a reliable indicator of leachate toxicity.
In many countries landfilling is still a popular method of municipal waste disposal due to the fact that it is considered easy and cost-effective (Nagarajan et al., 2012; Rana et al., 2018). Poland belongs to the European Union countries, and according to HDI (Human Development Index) is classified as a highly developed country (United Nations, 2019). Despite this, a large part of municipal waste still ends up in landfills. According to Eurostat, on average, 38.7% of municipal waste went to landfills in the European Union countries in 2018. The largest amount of municipal waste (among EU countries) in 2018 was collected in Germany and it was more than 50 million tons, of which only 1% of waste went to landfills. Similarly in Belgium, Denmark, Finland, and Sweden, where 1% of the collected municipal waste went to landfill in 2018. In Switzerland, on the other hand, municipal waste was not landfilled at all (Eurostat, 2014). In Poland, almost 12 million tons of municipal waste was collected in 2018, of which 43% went to landfills. There were also EU countries where the majority of waste in 2018 was landfilled. These included: Malta-83%, Greece-78%, and Romania-74% (Eurostat, 2014).Landfills can pose a serious threat to the environment, especially when they are improperly secured and active (Tałałaj et al., 2016). The formation of leachate is one of the inevitable consequences associated with the operation of landfills. Leachates are generated both during operation and many years after the closure of the landfill as a result of seepage of rainwater through waste layers (Aziz et al., 2010). Leachate formation is one of the most important factors that is considered in the operation and long-term management of municipal landfills (Tränkler et al., 2005)
Leachates contain large amounts of organic pollutants (expressed by chemical oxygen demand (COD), biochemical oxygen demand (BOD), and ammonia content, among others) and inorganic pollutants such as calcium, magnesium, sodium, potassium, iron, sulfates, chlorides, and heavy metals (cadmium, chromium, copper, lead, nickel, and zinc) (Christensen et al., 2001; Jones et al., 2006; Singh et al., 2016). They may contain many other substances and their composition for each landfill may be different (Öman and Junestedt, 2008). This causes difficulties in assessing the actual degree of threat to the environment and selecting an appropriate method of leachate disposal (Gao et al., 2014; Bove et al., 2015). Leachate water quality studies are conducted all over the world, but often the analysis ranges differ, which significantly hinders the comparison of results. One solution to this problem may be the use of leachate pollution index (LPI), proposed by Kumar and Alappat (Kumar and Alappat, 2005a). The LPI makes it possible to determine the degree of leachate contamination from different sites (with different size, age, and waste composition) and to compare them with each other. LPI can also be used to assess the toxicity potential of leachates (Rana et al., 2018), but the significance of the index in assessing their phytotoxic effects is not known (Guerrero-Rodríguez et al., 2014).
The LPI is determined based on 18 variables (parameters), selected by expert judgment. The selected parameters are pH, Total Dissolved Solids (TDS), Biological Oxygen Demand (BOD), Chemical Oxygen Demand (COD), Total Kjeldahl Nitrogen (TKN), Ammonium Nitrogen (AN), Fe, Cu, Ni, Zn, Pb, Cr, Hg, As, Phenolic Compounds (PC), Cl, Cyanide (CN), and Total Coliform Bacteria (TCB). Each of the selected parameters was assigned weights, which were calculated based on the mean scores given by the experts in the questionnaires on a scale of 1–5. A value of “1” indicated the parameter that had the lowest relative importance for leachate contamination, while a value of “5” was assigned to the parameter that had the highest relative importance (Kumar and Alappat, 2004; Kumar and Alappat, 2005b). Weights were determined for the selected variables and relationships were formulated between the concentration ranges most frequently observed during the study and the corresponding individual pollution sub-index (Kumar and Alappat, 2004). When the results of analyses of all 18 pollution indices are available, the LPI is calculated using the formula:
where: LPI-leachate pollution index, wi-weight of the i-th parameter, pi-individual pollution index, read from the curve, made on the basis of experts’ evaluations, n-number of studied variables for 18
In addition to the study of physicochemical composition, toxicity is an important parameter taken into account, among others because of the need to subject leachate to treatment before discharge into the environment. Leachate from landfills can be treated by various methods. One possibility is the use of phytoremediation. This is a technique that uses plants and associated rhizospheric microorganisms to remove, transform, or retain toxic substances from soil, water, sludge, or landfill leachate (Liao and Chang, 2004). Phytoremediation is used to remove petroleum hydrocarbons, pesticides, or heavy metals, among others (McCutcheon et al., 2002). It is a promising technique due to its low cost, self-sufficiency, and ability to remove contaminants at the site of leachate generation, among others (Singer et al., 2007; Akinbile et al., 2012). Phytoremediation has many advantages, but landfill leachate can adversely affect plants and cause leaf damage, reduced photosynthesis, necrosis, and even mortality (Zalesny et al., 2008; Kalčíková et al., 2012). The ability of plants to tolerate the stress caused by leachate and the selection and maintenance of appropriate concentrations within the limits of non-toxic effects on plants determines the effectiveness of phytoremediation (Kalčíková et al., 2012). Phytotoxicity tests are usually performed at the early germination stage due to short duration, ease of execution and low cost (Šourková et al., 2020). Popular parameters considered for phytotoxicity determination are seed germination, root, and shoot length increment (Maiorana et al., 2019), and therefore were used in the present study.
The aim of the study was to determine the degree of leachate contamination using LPI and toxic effect. An analysis of the relationship between the toxic effect on plants and the calculated LPI values was also carried out to assess whether they could provide a measure of leachate toxicity when selecting a treatment method.
The study was conducted at four landfills located in southwestern Poland (Figure 1). Two sites are inactive: landfill in Wrocław (N 51° 10′ 23.784″ E 16° 55′ 40.74″'), landfill in Bielawa (N 51° 9′ 21.485'' E 17° 14′ 18.03″). The landfill in Wrocław was in operation from 1966 to 2000. The total capacity of the landfill is about 2 million m3, and the total area of its quarters is 11.7 ha. The second inactive landfill is located in Bielawa; the landfill was in operation from 2001 to 2011, its total capacity is 37.6 thousand m3 and the area of its quarters is 0.86 ha.
Two other sites (landfill in Legnica site: N 51° 14′ 21.317″ E 16° 11′ 0.251″ and landfill in Jawor: N 51° 3′ 56.112″ E 16° 12′ 38.927″) are still in operation. The landfill in Legnica was opened in 1977, its total capacity is about 2.3 million m3 and the total area of the quarters is 14.2 ha. The second exploited landfill in Jawor has been functioning since 1997, its total capacity is about 231 thousand m3 and the total area of the quarters is 3.37 ha. Figure 1 presents the location of the research facilities and marks the places where samples were taken for testing. At sites 1, 2, and 3 leachate was collected from tanks, while at site 4 leachate was collected from wells.
Landfill leachate quality studies were conducted in September and December 2019. The leachates were tested for pH, chemical oxygen demand (COD), total Kjeldahl nitrogen (TKN), ammonium nitrogen (AN), total dissolved solids (TDS), chlorides (Cl), iron (Fe), zinc (Zn), copper (Cu), chromium (Cr), lead (Pb), and nickel (Ni). Immediately after sampling, the samples were transported in refrigerated conditions to the Environmental Research Laboratory of the Institute of Environmental Engineering, Wroclaw University of Life Sciences. Determinations of parameters were performed according to the methodology presented in ISO standards. Laboratory analyses that did not require mineralization of the samples were performed within 24 h of their collection (Tomczyk, 2021). At the same time, mineralization was carried out, and then analyses requiring mineralization were also carried out.
Due to the fact that in this study, selected parameters, which are part of the LPI index, were analyzed, a modified equation, described by Kumar and Alappat (2005a), was used for cases when the full range of research results is not available:
where: LPI-leachate pollution index, wi-weight of the i-th parameter, pi-individual pollution index, read from the curve, made on the basis of experts’ evaluations, m-number of studied variables (for m < 18
The weight (wi) of the pollutant indicates the importance of each parameter in the overall level of leachate contamination. With the full range of tests, the sum of the weights of all 18 parameters is 1. An individual pollutant index of the sub-index value (pi), is read from the curves showing the relationships between the concentrations of the analyzed parameters and the values of the corresponding sub-indexes (Kumar and Alappat, 2005a).
The LPI has been divided into three sub-indices. The three partial indices are based on the leachate characteristics and indicate the dominant contamination present in the leachate. The eighteen variable pollutants in the leachates selected for the LPI are grouped into three components: organic compounds-LPIor (i.e.,: COD, BOD, Phenolic compounds, Total coliform bacteria), inorganic components of leachate-LPIin (i.e.,: pH, TKN, AN, TDS, Chloride), and heavy metals-LPIhm (Total Chromium, Lead, Mercury, Arsenic, Cyanide, Zinc, Nickel, Copper, Iron) (Kumar and Alappat, 2005b).
When calculating the overall LPI, the m values shown in Eq. 2 correspond to the number of pollutants included in the sub-LPI. The three sub-LPIs have their own distinct meanings which are particularly important in making decisions related to the treatment of leachate.
An individual pollutant index of the sub-index value (pi), is read from the curves showing the relationships between the concentrations of the analyzed parameters and the values of the corresponding sub-indexes (Kumar and Alappat, 2005a). The three sub-LPI values (LPIor, LPIhm, LPIin) were calculated using the new weighting factors provided by Kumar and Alappat (2005c) and according to a modified equation in the absence of the full range of test results Eq. 2.
The following equation was derived by Kumar and Alappat (2005c) S based on the new weighting factors for the eighteen parameters in the LPI standard and their contribution to each sub-LPI.
where: Overall LPI-leachate pollution index, LPIor-sub-leachate pollution index organic component value, LPIin-sub-leachate pollution index inorganic component value, LPIhm-sub-leachate pollution index heavy metal component value.
The analyzed leachate samples were subjected to 3-days acute toxicity tests using white mustard seed (Sinapis alba L.) based on PhytotoxkitTM methodology. White mustard was chosen for this study due to its sensitivity to a number of substances and its frequent use in toxicity testing (Zloch et al., 2018; Vaverková et al., 2020). The test was performed twice (in September and December 2019). The tests were conducted in containers lined with foam pads and paper filters, which were wetted with 20 ml of leachate at different concentrations. The final dilution range for each test sample was selected based on previously conducted analyses: 100, 50, 25, 12.5, 6.25% (except for leachate from the landfill in Wrocław where the concentration range in September 2019 was 100 and 50% and in December 2019 it was 100, 50, and 25%). Dechlorinated tap water was used for dilutions and also served as a control sample.
Dried white mustard seeds of equal size and weight were placed on previously leachate-soaked test plates. Ten seeds were placed on each plate. The prepared boxes were inactive and placed in a rack in an upright position. The experiment was conducted at room temperature (approximately 25°C), in the darkness, for a period of 3 days. After 3 days, the number of germinated seeds and root and shoot length increments were recorded. Calculation of the toxic effect of leachate, manifested by inhibition of root and shoot growth, was done according to the formula:
where: EtL-toxic effect [inhibition of root and shoot growth (%)], L(a)-mean root or shoot length in the control trial (mm), and L(b)-mean root or shoot length in the test trial (mm) (Based on PhytotoxkitTM methodology).
The obtained results of leachate properties and phytotoxicity tests were subjected to statistical analysis using Statistica 13.1 software (StatSoft Poland, StatSoft, Inc., Tulsa, OK, United States). Two types of tests were used to evaluate the differences between the obtained phytotoxicity test results: the Student’s t test for dependent samples was used to detect statistically significant differences between leachate dilutions, while the Student’s t test for independent samples was used to detect statistically significant differences between the control sample (containing dechlorinated tap water) and samples containing leachate at different concentrations. The evaluation of the relationship between the calculated LPI values and the toxic effect obtained for the different leachate concentrations is presented in the form of correlation coefficient.
The quality of leachate depends on many factors, including the composition of waste, age of a landfill, extent of waste bed processes, location or local climate. Consequently, leachate composition is variable and site-specific. Table 1 presents the characteristics of the physicochemical composition of the analyzed leachates from four landfills.
Leachates generated during the initial period of landfill operation (up to 5 years) are characterized by pH values in the range of 3.7–6.5. The low pH value of leachates from young landfills is associated with the presence of carboxylic acids and bicarbonate ions. As landfills age, the pH of leachates changes from values corresponding to acidic to alkaline solutions. It is usually in the range of 8–8.5 pH, which is associated with the formation of alkaline compounds (Słomczyńska and Słomczyński, 2004). The pH values of the studied leachates, from active and inactive landfills were in the range of 7.4–8.7, which is typical of leachates from older and mature landfills (Jorstad et al., 2004).
The pH value of leachate affects seed germination process. It was found that it can negatively affect plant germination, both in very acidic (pH < 2) and strongly alkaline environment (pH > 13) provided that heavy metals are present in the leachate. The development and growth of roots and shoots is most favorably affected by pH above seven but not higher than 13, which is determined, among other things, by the ability to transport more solutes (Phoungthong et al., 2016; Naveen et al., 2017). In the case of the tested leachates, pH should not adversely affect germination and root and shoot growth.
TDS values in inactive landfills ranged from 2,225 mg/l (Wroclaw, September series) to 2,970 mg/l (Bielawa, September series). In active landfills the values varied more, from 3,975 mg/l (Jawor, September series) to 7,830 mg/l (Legnica, September series). Based on the study, it can be concluded that the TDS value was related to the age of the landfills, which could be determined by a decrease in the concentration of organic and inorganic dissolved substances (Tatsi and Zouboulis, 2002), confirmed by the conducted study. Higher TDS concentrations were found in leachate from active landfills.
The COD values of the leachates from the two landfills remained stable: the lowest values characterized the samples collected at the landfill in Wroclaw (inactive) and the highest values occurred in the case of leachates from the landfill in Legnica (active). These are the biggest of the investigated landfills and accumulation of large amounts of waste with stabilized decomposition processes (Wrocław) and fresh waste (Legnica) might have influenced the stable level of COD values. In case of the remaining landfills (inactive in Bielawa, active in Jawor), the December series of studies showed an increase of COD value in comparison with the September series. The studies showed similar level of COD values in leachates from these landfills. It should be noted that the landfills were put into operation at a similar time (1997–2001) and the results of the investigations may confirm a similar character of the decomposition processes taking place in the deposits of the deposited wastes.All COD values obtained in the conducted studies were within the ranges reported also by other authors (Kjeldsen et al., 2002; Tatsi and Zouboulis, 2002; Wdowczyk and Szymańska-Pulikowska, 2020), confirming that one of the main factors affecting their values is the age of the landfill site (or rather the age of the deposited waste), because with the passage of time the susceptibility of waste to biological and chemical degradation processes decreases.
Among the nitrogen forms that make TKN up, AN is the most abundant in landfill leachate. This relationship was confirmed by studies carried out in the landfills in Legnica, Jawor, and Bielawa. Only the results of the studies on leachates from the landfill in Wrocław did not confirm this relationship.
AN concentrations in leachates from active landfills usually range from 10 to 100 mg/l, but higher values have also been reported, reaching even above 10,000 mg/l (Tatsi and Zouboulis, 2002). In leachates from active landfills, AN concentrations were higher than in inactive facilities. The lowest AN values (< 1 mg/l) were recorded at the landfill in Wrocław. Much higher values were observed in the leachates from the second inactive landfill (in Bielawa), where this value exceeded 100 mg/l during two series of studies. However, in active landfills the values were even four times higher, exceeding 460 mg/l. Other authors in their works also emphasized that ammonium nitrogen belongs to the main pollutants occurring in landfill leachates (Kjeldsen et al., 2002; Tatsi and Zouboulis, 2002).
All samples collected in September 2019 were characterized by high chloride concentrations, and a clear variation in the results obtained was also evident: leachate samples from active landfills contained from 853 mg Cl/l (Jawor) to 2,670 mg Cl/l in Legnica. At the same time, the leachate samples from the inactive landfills contained from 110 mg Cl/l in Wrocław to 674 mg Cl/l in Bielawa. In leachate samples collected in December 2019, a marked decrease in chloride concentrations was observed, but differences between active and inactive landfills were still evident. Significantly higher concentrations of chloride in the leachate could have been caused by high precipitation in September 2019, exceeding the 1971–2000 norm for that month (115.4% of the norm). They could cause increased leaching of pollutant components from the waste (Meteorological Yearbook, 2019)
Leachates from active landfills contained from 11.5 mg Cl/l (Jawor) to 21.95 mg Cl/l (Legnica) and leachates from inactive landfills contained from 0.82 mg Cl/l (Wrocław) to 5.5 mg Cl/l (Bielawa).
According to the data presented in literature, usually in middle-aged landfills (i.e., more than 5 years old) chloride content in leachates decreases significantly, ranging from 100 to 400 mg Cl/l (Deng and Englehardt, 2007), which is confirmed by the results obtained during the second series of studies, both in active and inactive landfills. The results obtained in the first series of studies were clearly outside this range, however, such high concentrations were also observed by other authors, e.g., during studies of leachates from the landfill in Sydney carried out by Bowman et al. (2002) values reaching up to 8,000 mg Cl/l were observed.
Fe content in leachates from inactive landfills ranged from 0.019 mg/l (Wroclaw 12.2019) to 8.66 mg/l (Bielawa 09.2019), while in active landfills the values were higher, ranging from 2.6 mg/l (Legnica 12.2019) to 38.73 mg/l (Jawor 12.2019).
In both series of studies, in leachates from active and inactive landfills, Cu, Ni, Pb, and Cr contents did not exceed the value of 1 mg/L. Low contents of heavy metals (< 1 mg/l) in leachates from active landfills may indicate that the landfilled waste is mainly municipal waste, not containing these components (Naveen et al., 2017). Also in other studies, the authors reported low concentrations of heavy metals in leachates, emphasizing that their presence is not a major concern at present (Öman and Junestedt, 2008; Bożym et al., 2020). The clearly higher concentrations of chromium in the leachate (except for the landfill in Jawor) could have been caused (as in the case of chromium) by high precipitation in September 2019.
The zinc content in leachates from inactive landfills ranged from 0.554 mg/l at the landfill in Wrocław to 1.572 mg/l at the landfill in Bielawa. However, in active landfills, the value reached 2,560 mg/l (Jawor 12.2019).
Metals, such as Zn, Fe, Cu, Cr, Ni, act as micronutrients, needed for plant development. Therefore, in certain concentrations they are necessary for their proper functioning, they can stimulate growth, but after exceeding threshold values they become toxic for them. Metals in too high concentrations can lead to germination stopping and inhibition of root or shoot growth. For some heavy metals, such as Cd or Pb, toxic effects to plants can occur even at low concentrations (Umar et al., 2010; Kranner and Colville, 2011).
The LPI is a tool that allows the obtained results to be standardized and compared with each other (Naveen et al., 2017). The characteristics of the selected leachate series for one of the four sites where the study was conducted, i.e., Jawor landfill (December 2019 series), and the method of calculating the LPI value along with the parameters used for its calculation, i.e.,: weight parameter (wi), contaminant concentration and sub-index value (pi) are presented in Table 2.
12 out of the 18 parameters selected for LPI calculation were determined in the leachate samples. The calculated LPI values for the four landfills along with the shares of each parameter are presented in Figure 2.
FIGURE 2. LPI values and shares of particular parameters in calculated values for the investigated active (L-Legnica, J-Jawor) and inactive (W-Wrocław, B-Bielawa) landfills.
LPI values in active landfills ranged from 11.9 (Jawor 09.2019) to 15.1 (Jawor 12.2019). In inactive landfills, the values were lower and ranged from 5.2 (09.2019) in Wrocław, to 10.9 in Bielawa (12.2019).
The age of the landfill and the phase of operation influence the composition of leachate and thus the value of LPI (Singh et al., 2016), which is confirmed by the analyses performed. In inactive landfills, the value of LPI was lower than in active landfills, which indicates the stabilization of decomposition processes and is consistent with the results obtained by other authors (Kumar and Alappat, 2005a; Ahmed and Lan, 2012).
The smallest variation in the LPI value was recorded at the landfill in Wrocław. Such low values may result from the fact that the landfill was inactive in 2000 and the waste decomposition processes are stabilized.
The calculated LPI values were influenced by, among other things, low concentration of heavy metals in the leachate, which is related to the age of the landfills. With the passage of time, the amount of heavy metals in leachates decreases due to the change of reaction from acidic to basic, which is a result of organic acids consumption by methane bacteria and formation of hardly soluble forms of metals (Šourková et al., 2020). In the analyzed landfills (except for the landfill in Wrocław), higher contaminant values were recorded for COD, AN, and TKN, which influenced higher LPI values.
Calculated LPI values indicate that leachates from inactive landfills are contaminated to a lesser extent than from active sites. In the study performed, the LPI values did not exceed 16, and according to literature, only LPI > 35 indicates a poor environmental condition (Kumar and Alappat, 2005a). Such high values (at 34.8) were recorded at a landfill site in Mexico, as well as in leachates from Chandigarh, India (LPI = 33.2) and Bhalswa, New Delhi, India (LPI = 31.5), and Panchkula, India (LPI = 21.9). LPI values recorded in other studies ranged from 11.5 in Pajam 2, Malaysia, to 18.5 in leachates from Toluca (TOL), Mexico. In our study, similar LPI values were obtained (ranging from 11.1 to 15.9), only at the landfill in Wrocław the LPI value was much lower than reported in the literature and was 7.4 (Table 3).
The LPI does not show the influence of the different groups of pollutants in the leachate. Therefore, Kumar and Alappat have formulated sub-indices of LPI: LPIin, LPIor and LPIhm.
The three sub-LPIs were calculated using the procedure explained in Eq. 2 and are shown in Table 4.
The overall LPI was calculated using the procedure explained in Eq. 3.
At the closed landfill in Wrocław, the overall LPI value ranged from 5.43 (series 09.2019) to 6.03 (series 12.2019). The dominant pollutants were heavy metals-LPIhm was 42.4(series 12.2019)–48.8 % (series 09.2019). A high content of organic substances was also noted, LPIor ranged from 25.6 (series 09.2019) to 34.6 % (series 12.2019). Inorganic substances had a smaller share, LPIin ranged from 23 to 25.5%.
In the remaining three landfills, organic substances were the dominant group of pollutants. The corresponding LPIor values at the closed landfill in Bielawa were from 74.1% (series 09.2019) to 76.4% (series 12.2019). At an active landfill in Legnica, the LPIor values were from 66.97 % (series 09.2019) to 65.5% (series 12.2019At the second active landfill in Jawor, the LPIor was in the range from 65.9% (series 12.2019) to 69.2% (series 09.2019).
LPIhm values, reflecting the content of heavy metals in the leachate from the closed landfill in Bielawa, were from 14 (series 12.2019) to 16% (series 09.2019). For an active landfill in Legnica, LPIhm was from 12.2% (series 12.2019) to 21.6 (series 09.2019), for the second active landfill in Jawor LPIhm was in the range 12.3 (series 12.2019) to 15.5 % (series 09.2019)
Values LPIin, representing the content of inorganic substances in the Bielawa leachate, ranged from 9.6 (series 12.2019) to 9.9% (series 09.2019). For an active landfill in Legnica, these values were higher, the LPIin was 11.45 (series 09.2019) to 22.31% (series 12.2019). At the second active landfill in Jawor, LPIin was in the range 15.3 (series 09.2019) to 21.74% (series 12.2019).
The LPI sub-index calculations showed a low content of heavy metals in the analyzed leachates, therefore they should not adversely affect the biological treatment process. The exception is the oldest, closed landfill in Wrocław, where heavy metals were the dominant group of pollutants, but they also remained at a low level.
The obtained results show that the presence of heavy metals in the leachate should not disturb their biological treatment. Additionally, the leachate has a high content of organic substances (LPIor), which means that biological treatment will be a good method of their purification.
The percentage of germinated seeds of mustard Sinapis alba L. at different concentrations of leachate from four landfills is shown in Table 5.
TABLE 5. Effect of increasing landfill leachate concentrations on seed germination of Sinapis alba L.
A high percentage of germinated seeds was found in the inactive landfills. The lowest values among inactive landfills were recorded in Bielawa, where the percentage of germinated seeds ranged from 90% (in leachate concentration: 6.25, 50, 100%) to 100% (in leachate concentration: 12.5%, 25%). For the landfill in Wroclaw, only in September 2019 at 50% leachate concentration 90% of the seeds germinated. The other trials showed no effect on the germination of Sinapis alba L.
For active landfills, a greater effect of leachate concentration on seed germination can be observed. Especially for the landfill in Jawor, where at 100% leachate concentration the proportion of germinated seeds was 0% (December 2019 series) and 10% (September 2019 series). The significant inhibition of germination may have been due to a damage to the plant defense system (Gupta and Rajamani, 2015). For the second active landfill (in Legnica), a higher percentage of germinated seeds was observed: in the raw leachate it was 20% (December 2019 series) and 50% (September 2019 series).
Studies conducted for four landfills show that a clear relationship between seed germination and leachate composition cannot always be observed. Only in the case of the landfill in Jawor the December series of tests showed unequivocally the influence of increasing leachate concentration on the decrease of germinating seeds. In the remaining cases there were deviations from this trend, despite the fact that single dilutions (e.g., 12.5 and 100% in Legnica in December, 25 and 100% in Jawor in September) caused a significant decrease in the germination capacity of the seeds of Sinapis alba L. Other researchers came to similar conclusions. During leachate toxicity tests on three species i.e., lettuce (Lactuca sativa L.), arugula (Eruca sativa Mill.) and onion (Allium cepa L.), seed germination test was found to be insensitive to leachate toxicity (Klauck et al., 2015). The same was found during the study conducted by Vaisi and Žaltauskaitė (2010) on lettuce (Lactuca sativa L.), which showed no clear relationship between seed germination and leachate concentration. Similar conclusions were also reached by Guerrero-Rodríguez et al. (2014) conducting a study on common bean, during which a negligible toxic effect on the seed stage was found.
Figures 3–6 show the results of tests of differences between samples (Student’s t tests), performed for the control sample (0), and leachate dilutions. The graphs show the basic characteristics (mean, standard deviation, minimum, and maximum) of the empirical root (Figures 3, 4) and shoot (Figures 5, 6) length distributions for the four landfills where the study was conducted. Letters “a” and “b” indicate test results for independent groups, i.e., dilutions (samples) that significantly differed from the control sample in root or shoot length: “a” indicates root or shoot lengths significantly greater than those obtained for the control sample, “b” are lengths significantly less than those obtained for the control sample. Additionally, symbol “x” denotes the dilutions (trials) that significantly differed from the other dilutions (without the control trial), which was confirmed by the results of the test conducted for dependent trials. The marked differences are significant with p < 0.05.
FIGURE 3. Characteristic values (mean, standard deviation, minimum, maximum) for root length measurement results-series 09.2019.
FIGURE 4. Characteristic values (mean, standard deviation, minimum, maximum) for root length measurement results-series 12.2019.
FIGURE 5. Characteristic values (mean, standard deviation, minimum, maximum) for shoot length measurements results-series 09.2019.
FIGURE 6. Characteristic values (mean, standard deviation, minimum, maximum) for the results of shoot length measurements-series 12.2019.
Many studies have pointed out that the root length test performs better than the seed germination test in phytotoxicity assessment, which is related, among other things, to its greater sensitivity to contaminants (Galbraith et al., 1995; Fuentes et al., 2004; Kummerová and Kmentová, 2004; Vaisi and Žaltauskaitė, 2010; Kranner and Colville, 2011). The analyses performed confirmed that seed germination is not as sensitive to increasing leachate concentrations as root growth. This is most likely due to the increased sensitivity of the root creative tissue, which directly affects permeability, growth hormone production, and cell differentiation (Arunbabu et al., 2017). Figures 3, 4 show the effect of leachate concentrations on root growth of Sinapis alba L.
In the first series of tests conducted in September 2019, it was observed that at a leachate concentration of 6.25%, root length was greater than for the control sample (Figure 3). Further increase in leachate concentrations resulted in inhibition of root development. In the active landfills (Legnica, Jawor) it can be observed that at the highest concentration (100%), the root length increments were significantly smaller than for the control sample.
In each of the analyzed cases better root growth was observed at low concentrations (compared to the control sample). The results presented here indicate that diluted leachate can have a positive effect on plant growth. This may be due to the high concentration of nitrogen and the presence of other nutrients necessary for growth (Calabrese and Blain, 2005; Jones et al., 2006; Vaisi and Žaltauskaitė, 2010; Guerrero-Rodríguez et al., 2014). Other authors, on the other hand, explain the increase in root or shoot length at low leachate concentrations by hormonal action and stimulation of defense reactions in plants, which lead to a general activation of metabolism (Cargnelutti et al., 2006; Klauck et al., 2015)
During the second series of tests, conducted in December 2019, in most cases it was observed (with the exception of the landfill in Wrocław) that at 100% leachate concentrations root lengths were significantly smaller than those obtained for the control sample (Figure 4). In the active landfills (Legnica, Jawor) it was observed that even at lower concentrations leachate had an inhibitory effect on root growth and their lengths were smaller than for the control sample. Similar conclusions were reached by Vaverková et al. (2020) who observed during a study conducted on white mustard Sinapis alba L. that regardless of the concentration, leachates caused root growth inhibition.
The analyzed leachate samples in both series showed effects on shoot growth (Figures 5, 6). The effects vary between series and depend on the dose selected. In both series, significant differences in shoot length increments can be observed depending on the origin of the leachate (from active or inactive landfills). In the case of active landfills (Jawor, Legnica), at high leachate doses (above 50%), shoot length increments were smaller.
During the series performed in September (Figure 5) in the active landfills, at high leachate concentrations (50 and 100%) a significant decrease in shoot length increments was observed in comparison with the control sample. However, at lower concentrations shoot lengths remained at a similar level or were slightly larger than for the control sample (Legnica and Jawor). At the inactive landfills (Bielawa, Wrocław) such a pronounced decrease in shoot length increment was not observed with increasing doses of leachate in comparison with the control sample, and larger differences occurred only at 100% concentration.
The results of the second series of shoot growth tests (Figure 6), conducted in December, show much greater differences between active and inactive landfills, compared to the September series. Raw leachate in all cases showed an inhibitory effect on shoot growth, compared to the control sample. In active landfills, shoot growth in raw leachate was completely stopped. At low doses, leachates showed little stimulating effect on shoot growth-except at the landfill in Legnica, where even low leachate concentration (6.25%) resulted in less shoot growth compared to the control sample. Also Gupta and Rajamani in their study on Vicia faba found that high leachate concentrations significantly reduced shoot growth (in relation to the control sample), which may be due to the organism’s ability to defend itself against stress. The plant could show stress resistance with low leachate concentrations but not with high concentrations (Klauck et al., 2015).
Table 6 shows the magnitudes of the toxic effect (calculated from the inhibition of root and shoot growth) and the correlation coefficient between the toxic effect and LPI values. The toxic effect and the correlation coefficient were calculated for leachates from the landfills in Legnica, Jawor, and Bielawa. The results of the studies on leachate from the landfill in Wrocław were not taken into account because due to the low level of contamination less dilutions were performed for them, which resulted in rejection of the series differing in numbers during the calculations.
TABLE 6. Toxic effect values (calculated from inhibition of root and shoot growth) and correlations between toxic effect values and LPI for analyzed leachate dilutions (marked values are significant for p < 0.05, N = 12).
Statistically significant correlations, ranging from 0.592 to 0.785, occurred for dilutions from 25 to 100%. Testing of leachate samples from three landfills showed that the toxic effect at higher concentrations results in inhibition of root and shoot growth, which correlates with high LPI contaminant index values. From the above results, it can be concluded that leachate toxicity is related to contaminant concentration. It should be remembered that it is also influenced by other factors such as persistence and bioavailability in the environment. The results obtained confirmed the existence of a relationship between plant toxic effect and LPI values.
1. The use of LPI as a tool for assessing the quality of leachate water from different landfills significantly facilitates their rapid comparison with each other, even with a different range of analyses performed. Thus, it is possible to take quicker actions to reduce the negative impact on the environment. The application of LPI can also be helpful in the selection of leachate treatment technologies.
2. The division into LPI index subgroups allowed for a better definition and grouping of pollutants present in the leachate and turned out to be more useful in selecting the method of their treatment.
3. In the case of the three analyzed landfills, the sub-LPI indexes allowed to classify the leachate as rich in organic substances and characterized by a low content of heavy metals. This classification indicates that the biological treatment method may be the best possible treatment option for the analyzed effluents. Only in the case of the closed landfill in Wrocław, it was observed that heavy metals constitute the dominant group of pollutants, but the content of organic substances remained only at a slightly lower level.
4. Calculated values of the leachate pollution index showed that leachates from selected Polish landfills are characterized by low and medium levels of LPI values. The variation in index values obtained was particularly evident between active and inactive landfills and was related to the physicochemical composition of leachates.
5. Phytotoxicity tests showed that leachate at low concentrations can promote plant growth. At higher concentrations (50 and 100%), leachates caused inhibition of root and shoot growth, which correlated with high LPI values. The results confirmed the relationship between the toxic effect on plants and the LPI values, so it can be considered as a reliable indicator of leachate toxicity.
The original contributions presented in the study are included in the article/Supplementary Material, further inquiries can be directed to the corresponding author.
Conceptualization AW, AS-P. Methodology AW, AS-P. Validation AS-P Formal analysis AW, AS-P. Investigation AW. Resources AW, AS-P. Data Curation AW, AS-P. Writing-AW. Writing-Review and Editing AS-P. Visualization AW and AS-P. Supervision AS-P. Project administration AW.
B010/0004/20 UTRZYMANIE I ROZWÓJ POTENCJAŁU BADAWCZEGO KATEDR I INSTYTUTÓW-SUBWENCJA 2020-IKŚIG-INSTYTUT INŻYNIERII ŚRODOWISKA.
The authors declare that the research was conducted in the absence of any commercial or financial relationships that could be construed as a potential conflict of interest.
The Supplementary Material for this article can be found online at: https://www.frontiersin.org/articles/10.3389/fenvs.2021.693112/full#supplementary-material
Ahmed, F. N., and Lan, C. Q. (2012). Treatment of Landfill Leachate Using Membrane Bioreactors: A Review. Desalination 287, 41–54. doi:10.1016/j.desal.2011.12.012
Akinbile, C. O., Yusoff, M. S., and Shian, L. M. (2012). Leachate Characterization and Phytoremediation Using Water Hyacinth (Eichorrnia Crassipes) in Pulau Burung, Malaysia. Bioremediation J. 16, 9–18. doi:10.1080/10889868.2011.628350
Arunbabu, V., Indu, K. S., and Ramasamy, E. V. (2017). Leachate Pollution index as an Effective Tool in Determining the Phytotoxicity of Municipal Solid Waste Leachate. Waste Manag. 68, 329–336. doi:10.1016/j.wasman.2017.07.012
Aziz, H. A., Umar, M., and Yusoff, M. S. (2010). Variability of Parameters Involved in Leachate Pollution index and Determination of LPI from Four Landfills in Malaysia. Int. J. Chem. Eng. 2010, 3. doi:10.1155/2010/747953
Bove, D., Merello, S., Frumento, D., Arni, S. A., Aliakbarian, B., and Converti, A. (2015). A Critical Review of Biological Processes and Technologies for Landfill Leachate Treatment. Chem. Eng. Technol. 38, 2115–2126. doi:10.1002/ceat.201500257
Bowman, M. S., Clune, T. S., and Sutton, B. G. (2002). Sustainable Management of Landfill Leachate by Irrigation. Water. Air Soil Pollut. 134, 81–96. doi:10.1023/A:1014114500269
Bożym, M., Król, A., and Mizerna, K. (2020). Leachate and Contact Test with Lepidium Sativum L. To Assess the Phytotoxicity of Waste. International journal of Environmental Science and Technology. doi:10.1007/s13762-020-02980-x
Calabrese, E., and Blain, R. (2005). The Occurrence of Hormetic Dose Responses in the Toxicological Literature, the Hormesis Database: An Overview. Toxicol. Appl. Pharmacol. 202, 289–301. doi:10.1016/j.taap.2004.06.023
Cargnelutti, D., Tabaldi, L. A., Spanevello, R. M., de Oliveira Jucoski, G., Battisti, V., Redin, M., et al. (2006). Mercury Toxicity Induces Oxidative Stress in Growing Cucumber Seedlings. Chemosphere 65, 999–1006. doi:10.1016/j.chemosphere.2006.03.037
Christensen, T. H., Kjeldsen, P., Bjerg, P. L., Jensen, D. L., Christensen, J. B., Baun, A., et al. (2001). Biogeochemistry of Landfill Leachate Plumes. Appl. Geochem. 16, 659–718. doi:10.1016/S0883-2927(00)00082-2
Deng, Y., and Englehardt, J. D. (2007). Electrochemical Oxidation for Landfill Leachate Treatment. Waste Manag. 27, 380–388. doi:10.1016/j.wasman.2006.02.004
Eurostat (2014). Waste Generation and Treatment 2020-2021. Available online: https://ec.europa.eu/eurostat/data/databas (Accessed September 12, 2020).
Fuentes, A., Lloréns, M., Sáez, J., Aguilar, M. I., Ortuño, J. F., and Meseguer, V. F. (2004). Phytotoxicity and Heavy Metals Speciation of Stabilised Sewage Sludges. J. Hazard. Mater. 108, 161–169. doi:10.1016/j.jhazmat.2004.02.014
Galbraith, H., Lejeune, K., and Lipton, J. (1995). Metal and Arsenic Impacts to Soils, Vegetation Communities and Wildlife Habitat in Southwest Montana Uplands Contaminated by Smelter Emissions: I. Field Evaluation. Environ. Toxicol. Chem. 14, 1895–1903. doi:10.1002/etc.5620141111
Gao, J., Oloibiri, V., Chys, M., Audenaert, W., Decostere, B., He, Y., et al. (2014). The Present Status of Landfill Leachate Treatment and its Development Trend from a Technological point of View. Rev. Environ. Sci. Biotechnol. 14, 93–122. doi:10.1007/s11157-014-9349-z
Guerrero-Rodríguez, D., Sánchez-Yáñez, J. M., Buenrostro-Delgado, O., and Márquez-Benavides, L. (2014). Phytotoxic Effect of Landfill Leachate with Different Pollution Indexes on Common Bean. Water Air Soil Pollut. 225. doi:10.1007/s11270-014-2002-1
Gupta, A., and Rajamani, P. (2015). Toxicity Assessment of Municipal Solid Waste Landfill Leachate Collected in Different Seasons from Okhala Landfill Site of Delhi. JBiSE 08, 357–369. doi:10.4236/jbise.2015.86034
Hussein, M., Yoneda, K., Zaki, Z. M., Othman, N. A., and Amir, A. (2019). Leachate Characterizations and Pollution Indices of Active and Closed Unlined Landfills in Malaysia. Environ. Nanotechnology, Monit. Manag. 12, 100232. doi:10.1016/j.enmm.2019.100232
Jones, D. L., Williamson, K. L., and Owen, A. G. (2006). Phytoremediation of Landfill Leachate. Waste Manag. 26, 825–837. doi:10.1016/j.wasman.2005.06.014
Jorstad, L. B., Jankowski, J., and Acworth, R. I. (2004). Analysis of the Distribution of Inorganic Constituents in a Landfill Leachate-Contaminated Aquifer: Astrolabe Park, Sydney, Australia. Env Geol. 46, 263–272. doi:10.1007/s00254-004-0978-3
Kalčíková, G., Zagorc-Končan, J., Zupančič, M., and Gotvajn, A. Ž. (2012). Variation of Landfill Leachate Phytotoxicity Due to Landfill Ageing. J. Chem. Technol. Biotechnol. 87, 1349–1353. doi:10.1002/jctb.3776
Kjeldsen, P., Barlaz, M. A., Rooker, A. P., Baun, A., Ledin, A., and Christensen, T. H. (2002). Present and Long-Term Composition of MSW Landfill Leachate: A Review. Crit. Rev. Environ. Sci. Tech. 32, 297–336. doi:10.1080/10643380290813462
Klauck, C., Rodrigues, M., and Silva, L. (2015). Evaluation of Phytotoxicity of Municipal Landfill Leachate before and after Biological Treatment. Braz. J. Biol. 75, 57–62. doi:10.1590/1519-6984.1813
Kranner, I., and Colville, L. (2011). Metals and Seeds: Biochemical and Molecular Implications and Their Significance for Seed Germination. Environ. Exp. Bot. 72, 93–105. doi:10.1016/j.envexpbot.2010.05.005
Kumar, D., and Alappat, B. J. (2005b). Analysis of Leachate Pollution index and Formulation of Sub-leachate Pollution Indices. Waste Manag. Res. 23, 230–239. doi:10.1177/0734242X05054875
Kumar, D., and Alappat, B. J. (2005c). Analysis of Leachate Pollution index and Formulation of Sub-leachate Pollution Indices. Waste Manag. Res. 23, 230–239. doi:10.1177/0734242X05054875
Kumar, D., and Alappat, B. J. (2005a). Evaluating Leachate Contamination Potential of Landfill Sites Using Leachate Pollution index. Clean. Techn Environ. Pol. 7, 190–197. doi:10.1007/s10098-004-0269-4
Kumar, D., and Alappat, B. J. (2004). Selection of the Appropriate Aggregation Function for Calculating Leachate Pollution Index. Pract. Periodical Hazard. Tox. Radioactive Waste Manag. 8, 253–264. doi:10.1061/(asce)1090-025x(2004)8:4(253)
Kummerová, M., and Kmentová, E. (2004). Photoinduced Toxicity of Fluoranthene on Germination and Early Development of Plant Seedling. Chemosphere 56, 387–393. doi:10.1016/j.chemosphere.2004.01.007
Liao, S. W., and Chang, W. L. (2004). Heavy Metal Phytoremediation by Water Hyacinth at Constructed Wetlands in Taiwan. J. Aquat. Plant Manag. 42, 60–68.
Maiorana, S., Teoldi, F., Silvani, S., Mancini, A., Sanguineti, A., Mariani, F., et al. (2019). Phytotoxicity of Wear Debris from Traditional and Innovative Brake Pads. Environ. Int. 123, 156–163. doi:10.1016/j.envint.2018.11.057
McCutcheon, S. C., Susarla, S., and Medina, V. F. (2002). Phytoremediation: An Ecological Solution to Organic Chemical Contamination. Ecol. Eng. 18, 647–658. doi:10.1016/S0925-8574(02)00026-5
Meteorological Yearbook (2019). Institute of Meteorology and Water Management -National Research Institute. Warsaw: Instituts für Meteorologie und Wasserwirtschaft, 358. (in Polish).
Mohammad-pajooh, E., Weichgrebe, D., and Cuff, G. (2017). Municipal Landfill Leachate Characteristics and Feasibility of Retrofitting Existing Treatment Systems with Deammonification - A Full Scale Survey. J. Environ. Manage. 187, 354–364. doi:10.1016/j.jenvman.2016.10.061
Mor, S., Negi, P., and Khaiwal, R. (2018). Assessment of Groundwater Pollution by Landfills in India Using Leachate Pollution index and Estimation of Error. Environ. Nanotechnology, Monit. Manag. 10, 467–476. doi:10.1016/j.enmm.2018.09.002
Nagarajan, R., Thirumalaisamy, S., and Lakshumanan, E. (2012). Impact of Leachate on Groundwater Pollution Due to Non-engineered Municipal Solid Waste Landfill Sites of Erode City, Tamil Nadu, India. J. Environ. Health Sci. Engineer 9. doi:10.1186/1735-2746-9-35
Naveen, B. P., Mahapatra, D. M., Sitharam, T. G., Sivapullaiah, P. V., and Ramachandra, T. V. (2017). Physico-chemical and Biological Characterization of Urban Municipal Landfill Leachate. Environ. Pollut. 220, 1–12. doi:10.1016/j.envpol.2016.09.002
Öman, C. B., and Junestedt, C. (2008). Chemical Characterization of Landfill Leachates - 400 Parameters and Compounds. Waste Manag. 28, 1876–1891. doi:10.1016/j.wasman.2007.06.018
Phoungthong, K., Zhang, H., Shao, L.-M., and He, P.-J. (2016). Variation of the Phytotoxicity of Municipal Solid Waste Incinerator Bottom Ash on Wheat (Triticum aestivum L.) Seed Germination with Leaching Conditions. Chemosphere 146, 547–554. doi:10.1016/j.chemosphere.2015.12.063
Rana, R., Ganguly, R., and Gupta, A. K. (2018). Indexing Method for Assessment of Pollution Potential of Leachate from Non-engineered Landfill Sites and its Effect on Ground Water Quality. Environ. Monit. Assess. 190. doi:10.1007/s10661-017-6417-1
Singer, A. C., Bell, T., Heywood, C. A., Smith, J. A. C., and Thompson, I. P. (2007). Phytoremediation of Mixed-Contaminated Soil Using the Hyperaccumulator Plant Alyssum Lesbiacum: Evidence of Histidine as a Measure of Phytoextractable Nickel. Environ. Pollut. 147, 74–82. doi:10.1016/j.envpol.2006.08.029
Singh, S., Raju, N. J., Gossel, W., and Wycisk, P. (2016). Assessment of Pollution Potential of Leachate from the Municipal Solid Waste Disposal Site and its Impact on Groundwater Quality, Varanasi Environs, India. Arab. J. Geosci. 9, 1–12. doi:10.1007/s12517-015-2131-x
Šourková, M., Adamcová, D., Zloch, J., Skutnik, Z., and Vaverková, M. D. (2020). Evaluation of the Phytotoxicity of Leachate from a Municipal Solid Waste Landfill: The Case Study of Bukov Landfill. Environ. - MDPI 7, 1–14. doi:10.3390/environments7120111
Słomczyńska, B., and Słomczyński, T. (2004). Physico-chemical and Toxicological Characteristics of Leachates from MSW Landfills. Polish J. Environ. Stud. 13, 627–637.
Tatsi, A. A., and Zouboulis, A. I. U. (2002). A FIeld Investigation of the Quantity and Quality of Leachate from a Municipal Solid Waste Landfill in a Mediterranean Climate (Thessaloniki, Greece). Adv. Environ. Res. 6, 207–219. doi:10.1016/S1093-0191(01)00052-1
Tałałaj, I. A., Biedka, P., Walery, M. J., and Leszczyński, J. (2016). Monitoring of Leachate Quality at a Selected Municipal Landfill Site in Podlasie, Poland. J. Ecol. Eng. 17, 175–184. doi:10.12911/22998993/63477
Tomczyk, P. (2021). The Effects of Hydropower Plants on the Physicochemical Parameters of the Bystrzyca River in Poland. Energies 14, 2075. doi:10.1007/s10639-021-10539-7
Tränkler, J., Visvanathan, C., Kuruparan, P., and Tubtimthai, O. (2005). Influence of Tropical Seasonal Variations on Landfill Leachate Characteristics-Results from Lysimeter Studies. Waste Manag. 25, 1013–1020. doi:10.1016/j.wasman.2005.05.004
Umar, M., Aziz, H. A., and Yusoff, M. S. (2010). Trends in the Use of Fenton, Electro-Fenton and Photo-Fenton for the Treatment of Landfill Leachate. Waste Manag. 30, 2113–2121. doi:10.1016/j.wasman.2010.07.003
United Nations (2019). 2019 Human Development Index Ranking | Human Development Reports. United Nations Dev. Program.
Vaisi, R., and Žaltauskaitė, J. (2010). Evaluation of Municipal Effluent Toxicity Using Higher Plants and Invertebrates. Environ. Res. Eng. Manag. 3, 17–23.
Vaverková, M. D., Elbl, J., Koda, E., Adamcová, D., Bilgin, A., Lukas, V., et al. (2020). Chemical Composition and Hazardous Effects of Leachate from the Active Municipal Solid Waste Landfill Surrounded by Farmlands. Sustainability 12, 4531. doi:10.3390/su12114531
Wdowczyk, A., and Szymańska-Pulikowska, A. (2020). Differences in the Composition of Leachate from Active and Non-operational Municipal Waste Landfills in Poland. Water 12, 3129. doi:10.3390/w12113129
Zalesny, J. A., Zalesny, R. S., Wiese, A. H., Sexton, B., and Hall, R. B. (2008). Sodium and Chloride Accumulation in Leaf, Woody, and Root Tissue of Populus after Irrigation with Landfill Leachate. Environ. Pollut. 155, 72–80. doi:10.1016/j.envpol.2007.10.032
Keywords: landfill, leachate, properties, leachate pollution index, phytotoxicity
Citation: Wdowczyk A and Szymańska-Pulikowska A (2021) Comparison of Landfill Leachate Properties by LPI and Phytotoxicity-A Case Study. Front. Environ. Sci. 9:693112. doi: 10.3389/fenvs.2021.693112
Received: 09 April 2021; Accepted: 18 May 2021;
Published: 23 June 2021.
Edited by:
Jinquan Wan, South China University of Technology, ChinaReviewed by:
Tumpa Hazra, Jadavpur University, IndiaCopyright © 2021 Wdowczyk and Szymańska-Pulikowska. This is an open-access article distributed under the terms of the Creative Commons Attribution License (CC BY). The use, distribution or reproduction in other forums is permitted, provided the original author(s) and the copyright owner(s) are credited and that the original publication in this journal is cited, in accordance with accepted academic practice. No use, distribution or reproduction is permitted which does not comply with these terms.
*Correspondence: Aleksandra Wdowczyk, YWxla3NhbmRyYS53ZG93Y3p5a0B1cHdyLmVkdS5wbA==
Disclaimer: All claims expressed in this article are solely those of the authors and do not necessarily represent those of their affiliated organizations, or those of the publisher, the editors and the reviewers. Any product that may be evaluated in this article or claim that may be made by its manufacturer is not guaranteed or endorsed by the publisher.
Research integrity at Frontiers
Learn more about the work of our research integrity team to safeguard the quality of each article we publish.