- 1Freshwater Fisheries Research Center, Chinese Academy of Fishery Sciences, Scientific Observing and Experimental Station of Fishery Resources and Environmentin the Lower Reaches of the Changjiang River, Wuxi, China
- 2Wuxi Fishery College, Nanjing Agricultural University, Wuxi, China
Interaction effects of temperature, light, nutrients, and pH on growth and competition of Chlorella vulgaris and Anabaena sp. strain PCC were evaluated using an orthogonal design method to elucidate how these environment factors promote the growth of beneficial algae and limit the growth of harmful algae. The optimal conditions for the growth of C. vulgaris in the mono-culture system were as follows: temperature, 35°C; light, 660 lx; N concentration, 0.36 mg L−1; P concentration, 0.1 mg L−1; and pH, 9.0; and those for Anabaena were as follows: temperature, 30°C; light, 6,600 lx; N concentration, 0.18 mg L−1; P concentration, 0.1 mg L−1; and pH, 7.0. The optimal conditions for the growth of C. vulgaris in the co-culture system were as follows: temperature, 25°C; light, 4,400 lx; N concentration, 0.18 mg L−1; P concentration, 0.5 mg L−1; and pH, 6.0; and those for Anabaena were as follows: temperature, 35°C; light, 4,400 lx; N concentration, 0.36 mg L−1; P concentration, 0.5 mg L−1; and pH, 6.0. Both competition-inhibition parameters of Anabaena against C. vulgaris and those of C. vulgaris against Anabaena were the largest under the following conditions: temperature, 30°C; light intensity, 6,600 lx; N concentration, 0.36 mg L−1; P concentration, 0.025 mg L−1; and pH, 8.0. According to the Lotka–Volterra competition model, Anabaena won in the competition in the co-culture system with the following conditions: 1) temperature, 15°C; light, 660 lx; total N (TN), 0.18 mg L−1; total P (TP), 0.025 mg L−1; pH, 6; 2) temperature, 15°C; light, 2,200 lx; TN, 0.36 mg L−1; TP, 0.025 mg L−1; pH, 7; 3) temperature, 15°C; light, 6,600 lx; TN, 3.6 mg L−1; TP, 0.5 mg L−1; pH, 9; 4) temperature, 30°C; light, 4,400 lx; TN, 0.18 mg L−1; TP, 0.05 mg L−1; pH, 9; 5) temperature, 35°C; light, 660 lx; TN, 3.6 mg L−1; TP, 0.05 mg L−1; pH, 8; and 6) temperature, 35°C; light, 2,200 lx; TN, 0.72 mg L−1; TP, 0.025 mg L−1; pH, 9. However, C. vulgaris could not win in the competition in the co-culture system under all conditions tested.
Introduction
Harmful cyanobacterial blooms are becoming increasingly common in eutrophic water bodies, and they may result in a wide range of environmental, social, and economic consequences. For example, cyanobacterial blooms can increase oxygen demand, which may lead to localized incidents of hypoxic or anoxic conditions causing fish deaths (Anderson et al., 2002; Paerl, 2008). Surface blooms can also block light from reaching benthic primary producers, which may adversely affect food web dynamics depending on lake-bottom habitats (Bricelj and Lonsdale, 1997; Gallegos and Bergstrom, 2005). The social and economic effects of cyanobacterial blooms include negative effects on recreational opportunities due to the closure of affected areas, declining local fisheries, and increased water treatment costs (Hoagland et al., 2002; Paerl, 2008). In addition to their wider ecological effects, cyanobacteria are known to produce a suite of secondary metabolites that include hepatotoxins, neurotoxins, and dermatotoxic compounds. These toxins have been linked to reduced water quality and detrimental effects at higher trophic levels (Leonard and Paerl, 2005; Ferrão-Filho et al., 2009), small-animal illness and even mortality (Boyer, 2007; Jacoby and Kann, 2007), and adverse health risks in humans (Paerl, 2008).
Anabaena is a microscopic, bloom-forming, harmful cyanobacterium that has been reported to cause harmful algal blooms (Bouma-Gregson et al., 2017) and produce algal toxins in waters worldwide (Cai et al., 2006; Lawton and codd, 2010). It also causes ecological damage through high biomass and other related effects (Al-Mamoori et al., 2020). To improve water quality, protect aquatic animals, and ensure the safety and quality of aquatic products, scientists have carried out extensive research on how to control the growth of Anabaena in water bodies, especially in aquaculture ponds (Yue et al., 2006; Yu et al., 2016; Peng X. et al., 2020). Chlorella vulgaris is a typical microalga in water bodies. It can be easily digested by fish and other aquatic animals and is considered to be useful in water bodies (Meng et al., 2017). Therefore, domination by C. vulgaris in a water body is considered an indicator of good water quality (Yang and Lu, 2014; He et al., 2019).
Several environmental factors can influence the growth of algae (Zhao et al., 2014), including abiotic factors such as hydrodynamics (i.e., turbulence, wind-driven currents, and stratification) (Hotto et al., 2007; Fortin et al., 2010), nutrients (Downing et al., 2001; Heisler et al., 2008; Elliott, 2012), temperature (Cires et al., 2011), light availability (Cires et al., 2011; Renaud et al., 2011), and biotic interactions such as competition and grazing (Gobler et al., 2007; Ger et al., 2010). Temperature, light, nutrients, and pH are important ecological factors influencing algal growth (Mao et al., 2007), but there are only a few reports on their interactive effects on C. vulgaris and Anabaena. Changes in any of the factors may alter the phytoplankton community structure (Mei et al., 2003; Meng et al., 2013). It is important to understand how environmental factors can be manipulated to promote the growth of beneficial algae and limit the growth of harmful algae. Therefore, using an orthogonal design, in this study, we aimed to evaluate whether the interactions between temperature, light, nutrients, and pH influence the growth and competition of C. vulgaris and Anabaena, and to determine the optimal conditions for the dominance of C. vulgaris over Anabaena in water. The results might be valuable in guiding the control of environmental factors in pond and tank aquaculture.
Materials and Methods
Algae and Growth Conditions
Chlorella vulgaris and Anabaena sp. strain PCC 1042 were obtained from the Institute of Hydrobiology, Chinese Academy of Sciences. All algae were cultured in BG11 medium (Meng et al., 2015) in a plant growth chamber (PGX-150B; Wuxi Woxin Apparatus Co., Ltd., China). The culture conditions were as follows: temperature, 25°C; light intensity, 2.2 × 103 lx (using a fluorescent lamp as the light source); and photoperiod, 12-: 12- h light/dark.
The experiments were conducted in 250 ml Erlenmeyer flasks with 200 ml of BG11 medium (Meng et al., 2015). The cultures were shaken gently once every 2 h during the light period and allowed to stay still during the dark period. Before sampling, they were shaken again to ensure homogeneous cell distribution.
Experimental Design
An orthogonal array L16 54) with five environmental factors (temperature (T), light (L), N concentration (TN), P concentration (TP), and pH) and four levels (Table 1) were set using software Minitab 15 (The water temperature that good for the growth of most fish species is higher than 15°C, and the highest water temperature is about 35°C in summer, so the four temperature of 15°C, 25°C, 30°C, 35°C were chosen. The phosphorus concentration of 0.02 mg L−1 is critical point for eutrophication happening (Shi et al., 2004), and that of 0.5 mg L−1 is the first level standard value for the waste water discharged from aquaculture pond (Ministry of Agriculture and Rural Affairs of the PRC, 2007), so the four phosphorus concentrations of 0.025 mg L−1, 0.05 mg L−1, 0.1 mg L−1, 0.5 mg L−1 were chosen. Our other test showed that the nitrogen and phosphorus ratio for the best growth of Chlorella vulgaris is 7.2:1 (Wang et al., 2015), so the four nitrogen concentrations of 0.18 mg L−1, 0.36 mg L−1, 0.72 mg L−1, 3.6 mg L−1 were chosen based on the four phosphorus concentrations. According to the “Environmental quality standards for surface water” (Ministry of Ecology and Environment of the PRC, 2002), the pH should be between 6 and 9, so the four pH levels of 6, 7, 8, 9 were chosen. Although the natural light intensity in summer is as high as 100,000 lx in summer, the best light intensity for the growth of most fish species is lower than 7,000 lx (Xu et al., 2014), so the four light intensity levels of 660, 2,200, 4,400, 6,600 were chosen.); there were 16 groups in total, as shown in Table 2. There were three types of algal culture in every group, namely, mono-culture of C. vulgaris (treatment C), mono-culture of Anabaena (treatment A), co-culture of C. vulgaris and Anabaena (treatment CA). Three replicates for each group were prepared.
The initial density of C. vulgaris and Anabaena in each group was 5 × 105 cell ml−1, and the inoculations were carried out according to the method of Meng et al. (2015).
General Analyses
During the algal culture period, the liquid medium in each group was sampled with a 0.45-μm filter needle to determine total N and total P concentrations. Subsequently, NaNO3, KH2PO4, and ammonia-free water were added to increase the N and P concentrations and liquid medium volume to the initial level according to the determination results.
The cells were counted every 24 h after the beginning of the test. The test was terminated when a negative growth of algae was found, and the density 1 day before the negative growth was used as the maximum density of each algal species. The algal density was determined according to the methods described in the Standard Methods for the Examination of Water and Wastewater (Chinese National Environmental Protection Agency, 2002).
Data Analysis Methods
Specific Growth Rate
Specific growth rate was calculated using the following formula (Meng et al., 2012):
where, μn is the growth rate on day n, N is cell density (cells ml−1), and t is the culture time (d). The average specific growth rate (μ) is the algal growth rates from day 1 of the test to the day when the maximum cell density appeared, and μ was used to compare the growth rate of algal cells in different treatments.
Algae Growth Curve Fitting
The growth of algae was fitted by the logarithmic form of a logistic equation, and the least squares method was used for regression analysis to obtain the intercept and slope rate of the equation as estimated values for a and r respectively:
where, N is the biomass of the algae (count the biomass of algae in each treatment every day, and this biomasses are N values), K is the maximum biomass of the algae (using the maximum biomass of the algae in each treatment as its K value), r is the intrinsic growth rate, t is culture time.
Calculation of Competition–Inhibition Parameter
The Lotka–Volterra competition model was adopted to calculate the competition–inhibition parameters of C. vulgaris and Anabaena (Meng et al., 2012):
where, Nc and Na are the biomass (× 104 cells ml−1) of C. vulgaris and Anabaena in the co-culture system at time t, respectively; rcand ra are the intrinsic growth rate of C. vulgaris and Anabaena in the mono-culture system, respectively; Kc and Ka are the maximum biomass of C. vulgaris and Anabaena in the mono-culture system, respectively; α is the competition–inhibition parameter of Anabaena against C. vulgaris in the co-culture system, and β is the competition–inhibition parameter of C. vulgaris against Anabaena in the co-culture system.
The restraint beginning point and inhibition parameter were calculated according to Chen et al. (1999).
Statistical Analysis
Statistical analyses were performed using SPSS 15.0. Significant differences in algae biomass caused by the environment factors combination of T, L, TN, TP and pH, that is to say between the different treatments, were analyzed using the one-way analysis of variance (ANOVA), and Tukey’s multiple comparisons were used for statistical comparisons. Data were tested for normality of distribution (Shapiroe–Wilk test) and homogeneity of variance (Levene’s test) before analysis. Data that did not meet the assumptions of normality and homoscedasticity were transformed (lg), and then analyzed using the one-way ANOVA. Student’s t-test was used for testing the significance of the coefficients in the regression equations. Results with p < 0.05 were considered significant.
Results
Growth of C. vulgaris and Anabaena
The growth curves of C. vulgaris and Anabaena are shown in Figure 1 and their growth rates in Table 3. The main effect analysis plots (Supplementary Figures S1, S2) showed that the optimal growth conditions for C. vulgaris in the mono-culture system were as follows: temperature, 35°C; light intensity, 660 lx; N concentration, 0.36 mg L−1; P concentration, 0.1 mg L−1; and pH, 9.0; and those for Anabaena were as follows: temperature, 30°C; light intensity, 6,600 lx; N concentration, 0.18 mg L−1; P concentration, 0.1 mg L−1; and pH, 7.0. As shown in Supplementary Figures S3, S4, the optimal growth condition for C. vulgaris in the co-culture system were as follows: temperature, 25°C; light intensity, 4,400 lx; N concentration, 0.18 mg L−1; P concentration, 0.5 mg L−1; and pH, 6.0; and those for Anabaena were as follows: temperature, 35°C; light intensity, 4,400 lx; N concentration, 0.36 mg L−1; P concentration, 0.5 mg L−1; and pH, 6.0.
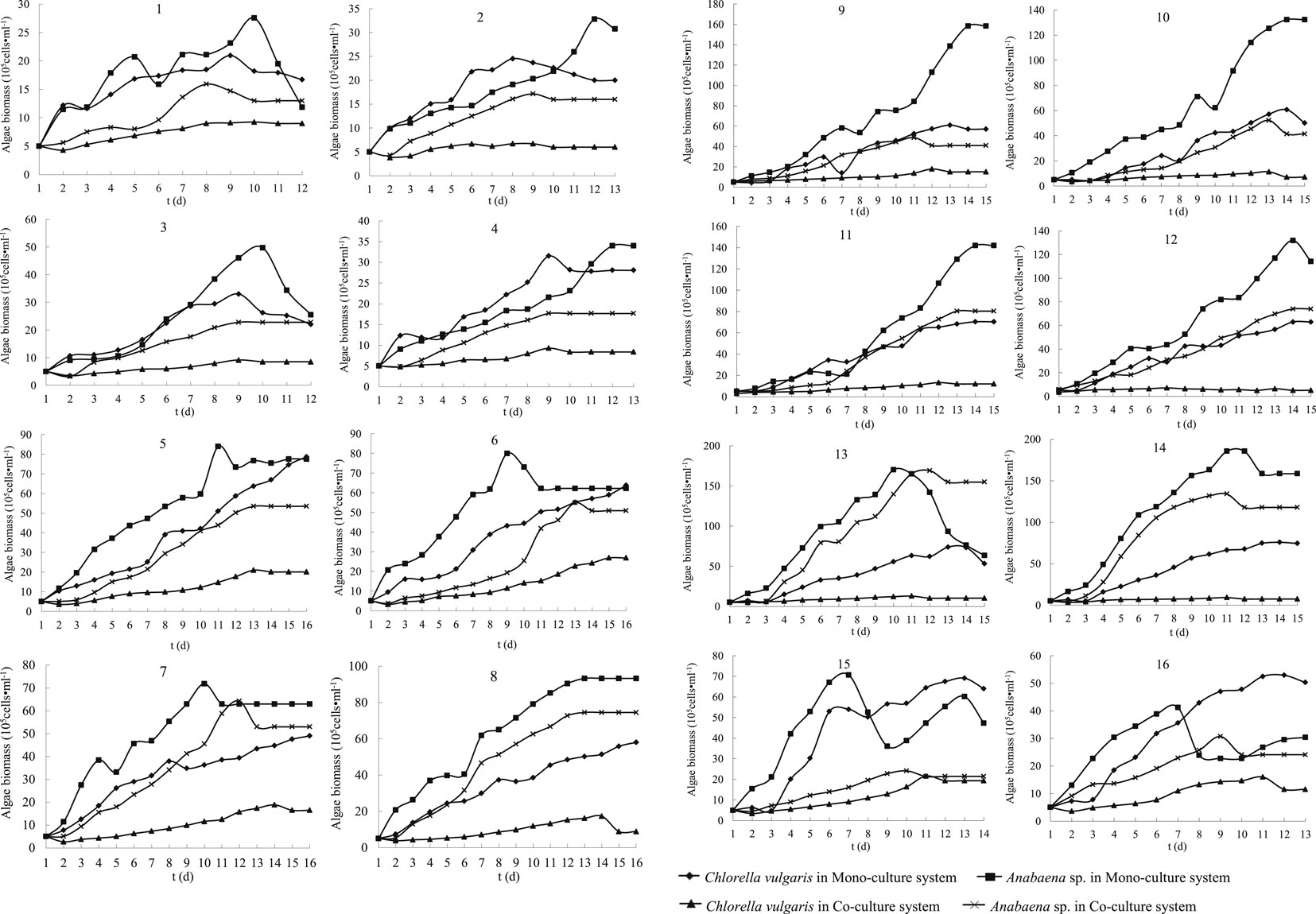
FIGURE 1. Growth curves of Chlorella vulgaris and Anabaena sp. strain PCC in mono-culture and co-culture systems at different conditions (from Treatment 1 to Treatment 16).
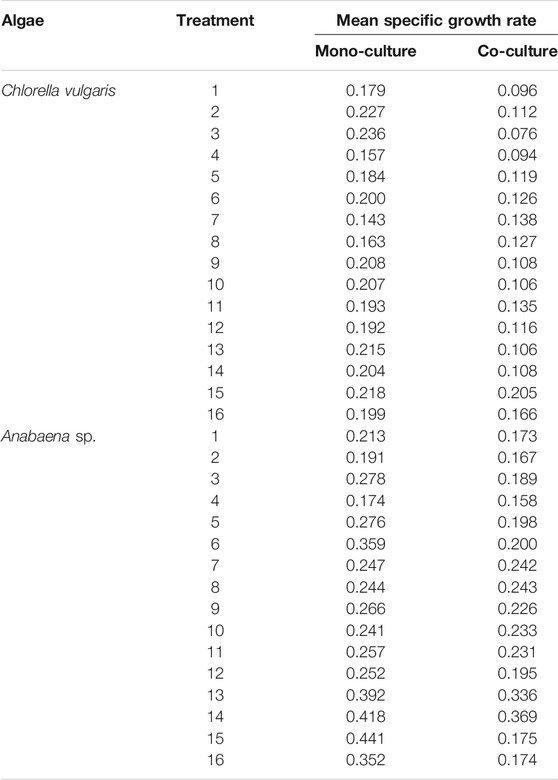
TABLE 3. Mean specific growth rate (μ) of Chlorella vulgaris and Anabaena sp. strain PCC at different conditions.
The maximum cell density of C. vulgaris in the mono-culture system was observed in treatment 5 (temperature, 25°C; light intensity, 660 lx; N, 0.36 mg L−1; P, 0.1 mg L−1; pH, 9.0); under these conditions, the maximum cell density was 787.0 × 104 cells L−1. The maximum cell density of Anabaena in the mono-culture system was found in treatment 14 (temperature, 35°C; light intensity, 2,200 lx; N, 0.72 mg L−1; P, 0.025 mg L−1; pH, 9.0); under these conditions, the maximum cell density was 1,854.9 × 104 cells L−1. The maximum cell density of C. vulgaris in the co-culture system was in treatment 6 (temperature, 25°C; light intensity, 2,200 lx; N, 0.18 mg L−1; P, 0.5 mg L−1; pH, 8.0); under these conditions, the maximum cell density was 268.5 × 104 cells·L−1. The maximum cell density of Anabaena in the co-culture system was in treatment 13 (temperature, 35°C; light intensity, 660 lx; N, 3.6 mg L−1; P, 0.05 mg L−1; pH, 8.0); under these conditions, the maximum cell density was 1 690.2 × 104 cells·L−1.
The growth curves of both C. vulgaris and Anabaena in the mono-culture system fitted an “S” shape (Figure 1), indicating that the growth curve of the two algae in the mono-culture system could be fitted with a logistic model, and the inflection point could be calculated using a logistic equation (Supplementary Materials). To calculate the inflection point time, the growth curves of C. vulgaris and Anabaena in the co-culture system were also fitted by a logistic model (Supplementary Materials).
Competition–Inhibition Parameters Between C. vulgaris and Anabaena
The competition–inhibition parameters of C. vulgaris against Anabaena and Anabaena against C. vulgaris in the co-culture system are shown in Table 4. The results revealed that the competition–inhibition parameters of Anabaena against C. vulgaris (α) were higher than those of C. vulgaris against Anabaena (β) in treatments 1, 2, 4, and 11. On the contrary, the competition–inhibition parameters of C. vulgaris against Anabaena (β) were greater than that of Anabaena against C. vulgaris (α) in other treatments. Both α and β reached the highest value in treatment 12. As shown in Table 4, the inflection point time of C. vulgaris was later than that of Anabaena in the mono-culture system in most treatments, but it was complicated, and there were no change trends for the inflection point time of C. vulgaris and Anabaena in the co-culture system.
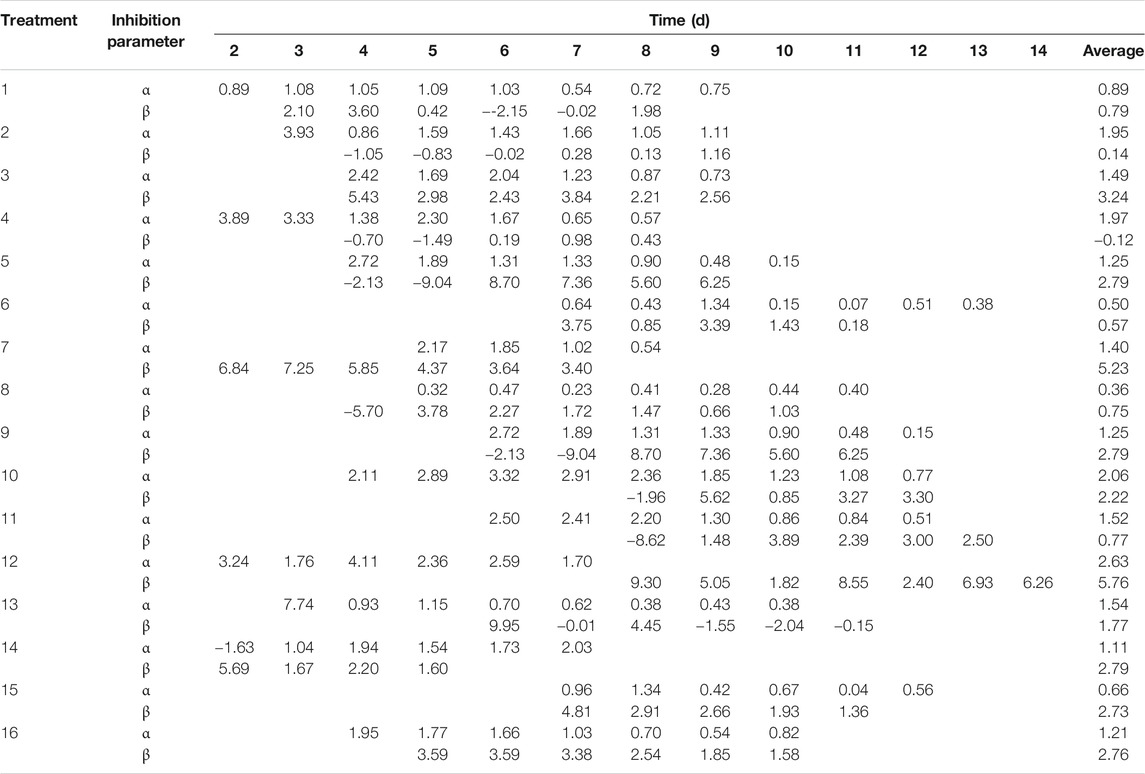
TABLE 4. Inhibition parameters of Chlorella vulgaris and Anabaena sp. strain PCC after inflection point at different conditions.
Discussion
Interaction Effects of Environmental Factors on the Growth of C. vulgaris and Anabaena in Mono-Culture System
The optimal growth conditions are different for different species of algae (Nakajima et al., 2020; Toth et al., 2020; Li et al., 2021). Long et al. (2011) reported that the optimal growth conditions for spring algal species are as follows: temperature, 20°C; light intensity, 4,700 lx; N concentration, 3.5 mg L−1; P concentration, 0.3 mg L−1; and water flow rate, 0.1 m s−1. Our analyses showed that the maximum cell density of C. vulgaris increased with temperature (Supplementary Figure S1) and reached the peak point at 35°C. The optimal growth conditions for C. vulgaris were as follows: temperature, 35°C; light intensity, 660 lx; N concentration, 0.36 mg L−1; P concentration, 0.1 mg L−1; and pH, 9.0; and those for Anabaena were as follows: temperature, 30°C; light intensity, 6,600 lx; N concentration, 0.18 mg L−1; P concentration, 0.1 mg L−1; and pH, 7.0. Song et al. (2013) observed an apparent exponential growth phase of Chlorella when the temperature increased to 20°C or 25°C, and its specific growth rate and maximum cell density also increased with the increase in temperature. In the present study, we found a similar relationship between maximum cell density and temperature.
As shown in Supplementary Figure S2, the maximum cell density of Anabaena at a light intensity of less than 2,200 lx was below the mean level, indicating that its growth was inhibited under such light conditions. A long period under light-limited conditions could increase the precipitation of Anabaena cells and result in cell loss (Wu et al., 2012), which might explain the phenomenon observed in our experiment.
Through regression analysis, Liang et al. (2010) found that water temperature and total P concentration are two key factors affecting the growth of Anabaena. Wang et al. (2005) reported that the effect of water temperature on algal biomass is closely related to the nutrient concentration in the water. They found that the algal biomass at a low temperature was greater than that at a high temperature in the water with low nutrient concentrations (inorganic N, 0.2–0.4 mg L−1; organic N, 0.2–0.4 mg L−1; and total P, 0.005–0.01 mg L−1), and the algal biomass at a low temperature was less than that at a high temperature in water with high nutrient concentrations (inorganic N, 0.3–0.65 mg L−1; organic N, 0.4–0.7 mg L−1; and total P 0.01–0.03 mg L−1). We obtained a similar result in our experiment, that is, the maximum cell density of C. vulgaris in treatments with a high temperature and low nutrient concentrations was lower than that in treatments with a low temperature and high nutrient concentrations. The activities of the algae increased with the increase in temperature, leading to a higher metabolic rate and greater nutrient consumption; thus, the available nutrients in the water depleted and finally limited the algal growth.
Interaction Effects of Environmental Factors on the Competitive Growth of C. vulgaris and Anabaena in the Co-culture System
Different microalgal species compete with one another for light, nutrients, and other resources (Cao et al., 2012); therefore, competition is a crucial factor promoting changes in the predominant algae in water bodies (Piazzi and Ceccherelli, 2002; Litchman, 2003; Burrows et al., 2021). Our experiment revealed that competition–inhibition parameters of C. vulgaris against Anabaena and those of Anabaena against C. vulgaris in the co-culture system differed under different conditions, indicating that the predominant positions of the two algae alternated with the environment. Chen et al. (2010) reported that competition–inhibition parameters between Oscillatoria and Microcystis changed at 20, 25, and 30°C. Moreover, Chen et al. (2009) showed that Microcystis is more competitive than Scenedesmus at light intensity of below 6,600 lx, and the vice versa at higher light intensity. In our analyses, the maximum cell densities of both C. vulgaris and Anabaena in the co-culture system were lower than in the mono-culture system; this indicated that the growth of both algae was inhibited in the co-culture system.
The algae growing together compete for light, nutrients, and other resources and even secrete growth-inhibition substances to inhibit each other (Xu et al., 2004; Peng Y. et al., 2020; Stn and Bykiik, 2020). When the growth of one algal species is inhibited due to the deficiency of some nutrient elements, another species less dependent on these nutrient elements might grow quickly and become the dominant species (Wang et al., 2010; Xu et al., 2011). Hu et al. (2006) showed that Chlorella reduced the N and P concentration in the environment by absorbing them. Therefore, it could be inferred that the growth of C. vulgaris changes the N-to-P ratio in a co-culture system to a certain extent. This effect might explain why the competition–inhibition parameters of C. vulgaris against Anabaena were higher than those of Anabaena against C. vulgaris in the present study, even when the growth of C. vulgaris had been inhibited in treatments 1, 2, 4, 10, 11, 12, 13, and 16. Zhao H. T. et al. (2011) showed that, under non-steady-state conditions, Microcystis aeruginosa quickly adopted the dominant position when nutrients were added continuously, but Scenedesmus obliquus occupied the dominant position when the nutrient level was low. In our experiment, the nutrients in the medium were measured and restored to the initial level every 24 h. Under such conditions, the cell density of Anabaena was always higher than that of C. vulgaris, indicating that Anabaena had a greater affinity for nutrients. Zhao X. D. et al. (2011) revealed that under conditions of low N and P concentrations, M. aeruginosa became the dominant species. Zhao H. T. et al. (2011) found that Microcystis sp. could adapt to low-nutrient conditions by changing cell size. Therefore, it could be inferred that another species of cyanobacteria, namely Anabaena, might be able to absorb nutrients and inhibit the growth of C. vulgaris by changing cell size according to its cell density and the nutrient concentration in the environment. This would also explain why the competition–inhibition parameters of Anabaena against C. vulgaris were greater in treatments with lower N and P concentrations.
Competitive Growth of C. vulgaris and Anabaena in the Co-culture System
According to the algal competition ending described in the Lotka–Volterra competition model (Meng et al., 2012), the relationships between competition–inhibition parameters α, β, and environmental capacities Kc and Ka are shown in Table 5. As shown in Table 5, in treatments 1, 2, 4, 11, 13, and 14, 1/Ka <α/Kc and 1/Kc> β/Ka, indicating that Anabaena was dominant in the competitions; in treatments 3, 5, 9, 10, 12, 15, and 16, 1/Kc <β/Ka and 1/Ka <α/Kc, indicating that C. vulgaris and Anabaena could coexist, but not stably; in treatments 6, 7, and 8, 1/Kc> β/Ka and 1/Ka> α/Kc, indicating that C. vulgaris and Anabaena could coexist stably (Supplementary Figure S5). The phenomenon in our experiment showed that low phosphorus conditions are good for the victory of Anabaena in the competitions, and high phosphorus conditions are beneficial for C. vulgaris. Just as M. aeruginosa, the other harmful blue-green algae, could become the dominant species under conditions of low phosphorus concentration by the way of changing cell size to adapt to low-nutrient conditions. So maybe maintain a suitable condition with high phosphorus concentration (no less than 0.1 mg L−1) is useful to promote growth of beneficial algae such as C. vulgaris and inhibit growth of harmful algae such as Anabaena.
Conclusion
The optimal growth conditions for C. vulgaris in the mono-culture system were as follows: temperature, 35°C; light intensity, 660 lx; N concentration, 0.36 mg L−1; P concentration, 0.1 mg L−1; and pH, 9.0. The optimal growth conditions for Anabaena in the mono-culture system were as follows: temperature, 30°C; light intensity, 6,600 lx; N concentration, 0.18 mg L−1; P concentration, 0.1 mg L−1; and pH, 7.0. The optimal growth conditions for C. vulgaris in the co-culture system were as follows: temperature, 25°C; light intensity, 4,400 lx; N concentration, 0.18 mg L−1; P concentration, 0.5 mg L−1; and pH, 6.0. For Anabaena in the co-culture system, the optimal growth conditions were as follows: temperature, 35°C; light intensity, 4,400 lx; N concentration, 0.36 mg L−1; P concentration, 0.5 mg L−1; and pH, 6.0. Light was not the most critical factor affecting the growth of C. vulgaris, and pH was not the most critical factor affecting the growth of Anabaena.
Temperature, light, nutrients, and pH significantly influenced the competition–inhibition parameters of the two algal species. Both competition–inhibition parameters of Anabaena against C. vulgaris and C. vulgaris against Anabaena were the highest under the following conditions: temperature, 30°C; light intensity, 6,600 lx; N concentration, 0.36 mg L−1; P concentration, 0.025 mg L−1; and pH, 8.0. According to the Lotka–Volterra competition model, Anabaena won in the competition in co-culture system under the following conditions: 1) temperature, 15°C; light, 660 lx; TN, 0.18 mg L−1; TP, 0.025 mg L−1; pH, 6; 2) temperature, 15°C; light, 2,200 lx; TN, 0.36 mg L−1; TP, 0.025 mg L−1; pH, 7; 3) temperature, 15°C; light, 6,600 lx; TN, 3.6 mg L−1; TP, 0.5 mg L−1; pH, 9; 4) temperature, 30°C; light, 4,400 lx; TN, 0.18 mg L−1; TP, 0.05 mg L−1; pH, 9; 5) temperature, 35°C; light, 660 lx; TN, 3.6 mg L−1; TP, 0.05 mg L−1; pH, 8; and 6) temperature, 35°C; light, 2,200 lx; TN, 0.72 mg L−1; TP, 0.025 mg L−1; pH, 9.
Data Availability Statement
The original contributions presented in the study are included in the article/Supplementary Material, further inquiries can be directed to the corresponding authors.
Author Contributions
JC, PX, and SM designed the study. SM and XC planned the research activities. SM, XC, and JW performed the study, and collected and analyzed the data. SM wrote the manuscript. LF, LQ, and YZ revised the manuscript. All authors contributed to the article and approved the submitted version.
Funding
This work was supported by the National Key Research and Development Project (No. 2020YFD0900502); China Agriculture Research System of MOF and MARA (No. CARS-46); and Special Fund OF MARA (Monitoring and assessment of fishery ecological environment in lower reaches of Yangtze River).
Conflict of Interest
The authors declare that the research was conducted in the absence of any commercial or financial relationships that could be construed as a potential conflict of interest.
Supplementary Material
The Supplementary Material for this article can be found online at: https://www.frontiersin.org/articles/10.3389/fenvs.2021.690191/full#supplementary-material
References
Al-Mamoori, A. M. J., Al-Shammari, R. H. H., Al-Amari, M. J. Y., and Al-Juboori, M. M. K. (2020). Removal of Anabaena Sp. Bloom and Microcystin-Lr by Coculturing with Mucor Rouxii Pellets. Aquat. Ecosyst. Health Manage. 23 (3), 267–273. doi:10.1080/14634988.2020.1816002
Anderson, D. M., Glibert, P. M., and Burkholder, J. M. (2002). Harmful Algal Blooms and Eutrophication: Nutrient Sources, Composition, and Consequences. Estuaries 25, 704–726. doi:10.1007/bf02804901
Bouma-Gregson, K., Power, M. E., and Bormans, M. (2017). Rise and Fall of Toxic Benthic Freshwater Cyanobacteria (Anabaena spp.) in the Eel River: Buoyancy and Dispersal. Harmful Algae 66, 79–87. doi:10.1016/j.hal.2017.05.007
Boyer, G. L. (2007). The Occurrence of Cyanobacterial Toxins in New York Lakes: Lessons from the MERHAB-Lower Great Lakes Program. Lake Reservoir Manage. 23, 153–160. doi:10.1080/07438140709353918
Bricelj, V. M., and Lonsdale, D. J. (1997). Aureococcus Anophagefferens: Causes and Ecological Consequences of Brown Tides in U.S. Mid-Atlantic Coastal Waters. Limnol. Oceanogr. 42, 1023–1038. doi:10.4319/lo.1997.42.5_part_2.1023
Burrows, R. M., Jonsson, M., Fältström, E., Andersson, J., and Sponseller, R. A. (2021). Interactive Effects of Light and Nutrients on Stream Algal Growth Modified by forest Management in Boreal Landscapes. For. Ecol. Manage. 492, 119212. doi:10.1016/j.foreco.2021.119212
Cai, J.,P., Cheng, L., Wu, B., and Song, Z. P. (2006). Toxins in Anatoxins and the Relevant Measurement and Removal Methods. Reservoir Fish. 26 (3), 3–6. doi:10.3969/j.issn.1003-1278.2006.03.002
Cao, Y. C., Wang, X. P., Li, Z. J., Wen, G. L., and Chen, S. W. (2012). Path Analysis on Competition of Nitrogen and Phosphorus Among Nannochloropsis Oculata, Cry Ptomonas Erosa and Oscillatoria Chlorine. Prog. Fish. Sci. 33 (4), 99–106. doi:10.1007/s11783-011-0280-z
Chen, D. H., Liu, Y. D., Yuan, J. F., Zhang, Z. S., Song, L. R., and Chen, J. (1999). Experiments of Mixed Culture and Calculation of Competitive Parameters between Microcystis (Cyanobacteria) and Scenedesmus (Green Algae). Acta Ecologica Sinica 19 (6), 908–913.
Chen, X. F., Pang, Y., and Yan, R. R. (2009). Effect of Light Intensity on Growth of Two Species of Algae under Competition Cultures. Environ. Sci. Technol. 32 (6), 6–11. doi:10.3969/j.issn.1003-6504.2009.06.002
Chen, J. Z., Meng, S. L., Hu, G. D., Qu, J. H., Wu, W., Fan, L. M., et al. (2010). Effect of Temperature on Interspecies Competition of Two Blue-green Algae. Chin. J. Ecol. 29 (3), 454–459. doi:10.1016/S1872-5813(11)60001-7
Chinese National Environmental Protection Agency (2002). Water and Wastewater Monitoring Analysis Method. 4th ed. Beijing: China Environmental Science Press.704
Cirés, S., Wörmer, L., Timón, J., Wiedner, C., and Quesada, A. (2011). Cylindrospermopsin Production and Release by the Potentially Invasive Cyanobacterium Aphanizomenon Ovalisporum under Temperature and Light Gradients. Harmful Algae 10, 668–675. doi:10.1016/j.hal.2011.05.002
Downing, J. A., Watson, S. B., and McCauley, E. (2001). Predicting Cyanobacteria Dominance in Lakes. Can. J. Fish. Aquat. Sci. 58, 1905–1908. doi:10.1139/f01-143
Elliott, J. A. (2012). Predicting the Impact of Changing Nutrient Load and Temperature on the Phytoplankton of England's Largest lake, Windermere. Freshw. Biol 57, 400–413. doi:10.1111/j.1365-2427.2011.02717.x
Ferrão-Filho, A. d. S., Soares, M. C. S., de Freitas Magalhães, V., and Azevedo, S. M. F. O. (2009). Biomonitoring of Cyanotoxins in Two Tropical Reservoirs by Cladoceran Toxicity Bioassays. Ecotoxicol. Environ. Saf. 72, 479–489. doi:10.1016/j.ecoenv.2008.02.002
Fortin, N., Aranda-Rodriguez, R., Jing, H., Pick, F., Bird, D., and Greer, C. W. (2010). Detection of Microcystin-Producing Cyanobacteria in Missisquoi Bay, Quebec, Canada, Using Quantitative PCR. Appl. Environ. Microbiol. 76, 5105–5112. doi:10.1128/aem.00183-10
Gallegos, C. L., and Bergstrom, P. W. (2005). Effects of a Prorocentrum Minimum Bloom on Light Availability for and Potential Impacts on Submersed Aquatic Vegetation in Upper Chesapeake Bay. Harmful Algae 4, 553–574. doi:10.1016/j.hal.2004.08.016
Ger, K. A., Teh, S. J., Baxa, D. V., Lesmeister, S., and Goldman, C. R. (2010). The Effects of Dietary Microcystis Aeruginosa and Microcystin on the Copepods of the Upper San Francisco Estuary. Freshw. Biol. 55, 1548–1559. doi:10.1111/j.1365-2427.2009.02367.x
Gobler, C. J., Davis, T. W., Coyne, K. J., and Boyer, G. L. (2007). Interactive Influences of Nutrient Loading, Zooplankton Grazing, and Microcystin Synthetase Gene Expression on Cyanobacterial Bloom Dynamics in a Eutrophic New York lake. Harmful Algae 6, 119–133. doi:10.1016/j.hal.2006.08.003
He, H. S., Yuan, J. L., Liu, M., Ni, M., and Gu, Z. M. (2019). Preliminary Study on Effects of Chlorella Vulgaris and Ipomoea Aquatica Regulation on the Water Quality and Growth of Penaeus Vannamei. Freshw. Fish. 49 (3), 102–107. doi:10.3969/j.issn.1000-6907.2019.03.017
Heisler, J., Glibert, P. M., Burkholder, J. M., Anderson, D. M., Cochlan, W., Dennison, W. C., et al. (2008). Eutrophication and Harmful Algal Blooms: a Scientific Consensus. Harmful Algae 8, 3–13. doi:10.1016/j.hal.2008.08.006
Hoagland, P., Anderson, D. M., Kaoru, Y., and White, A. W. (2002). The Economic Effects of Harmful Algal Blooms in the United States: Estimates, Assessment Issues, and Information Needs. Estuaries 25, 819–837. doi:10.1007/bf02804908
Hotto, A. M., Satchwell, M. F., and Boyer, G. L. (2007). Molecular Characterization of Potential Microcystin-Producing Cyanobacteria in Lake Ontario Embayments and Nearshore Waters. Appl. Environ. Microbiol. 73, 4570–4578. doi:10.1128/aem.00318-07
Hu, K. H., Zhu, X., Wang, S. H., Liu, Y., and Lin, X. (2006). The Removal Effect of Chlorella Vulgaris Growth on Nitrogen and Phosphorus. J. Fujian Agric. For. Univ. (Natural Sci. Edition) 35 (6), 648–651. doi:10.3969/j.issn.1671-5470.2006.06.020
Jacoby, J. M., and Kann, J. (2007). The Occurrence and Response to Toxic Cyanobacteria in the Pacific Northwest, North America. Lake Reservoir Manage. 23, 123–143. doi:10.1080/07438140709353916
Lawton, L. A., and Codd, G. A. (2010). Cyanobacterial (Blue-green Algal) Toxins and Their Significance in uk and European Waters. Water Environ. J. 5 (4), 460–465. doi:10.1111/j.1747-6593.1991.tb00643.x
Leonard, J. A., and Paerl, H. W. (2005). Zooplankton Community Structure, Micro-zooplankton Grazing Impact, and Seston Energy Content in the St. Johns River System, Florida as Influenced by the Toxic Cyanobacterium Cylindrospermopsis Raciborskii. Hydrobiologia 537, 89–97. doi:10.1007/s10750-004-2483-9
Li, X., Manuel, J., Slavens, S., Crunkleton, D. W., and Johannes, T. W. (2021). Interactive Effects of Light Quality and Culturing Temperature on Algal Cell Size, Biomass Doubling Time, Protein Content, and Carbohydrate Content. Appl. Microbiol. Biotechnol. 105 (2), 587–597. doi:10.1007/s00253-020-11068-y
Liang, H., Chen, Z. L., Qu, F. S., Tian, J. Y., and Li, G. B. (2010). Regression Equations between Algae Propagation and Physic-Chemical Factors under Microcosm Environment. J. Harbin Inst. Technol. 42 (6), 841–844. doi:10.1080/1528008X.2014.921777
Litchman, E. (2003). Competition and Coexistence of Phytoplankton under Fluctuating Light: Experiments with Two Cyanobacteria. Aquat. Microb. Ecol. 31 (3), 241–248. doi:10.3354/ame031241
Long, T. Y., Zhou, P. R., and Wu, L. (2011). The Simulating experiment for the Impacts of Environmental Factors on Spring Algae Growth in Xiangxi River. China Environ. Sci. 31 (2), 327–331.
Mao, H., Xu, H., and Liu, Z. P. (2007). Effects of Water Temperature, Illumination, Light, Salinity and pH on the Growth of Chaetoceros Curvisetus. Ecol. Sci. 26 (5), 432–436.
Mei, H., Zhao, X. F., Guo, B., Liu, G. X., and Hu, Z. Y. (2003). Advances in Freshwater Algal Biodiversity in China. Ecol. Sci. 22 (4), 356–359.
Meng, S. L., Qiu, L. P., Hu, G. D., Qu, J. H., Fan, L. M., Song, C., et al. (2012). Effect of Nitrogen and Phosphorus Ratios on Growth and Competition of Two Blue-green Algae. J. Agro-Environmnet Sci. 31 (7), 1438–1444.
Meng, S. L., Qu, J. H., Qiu, L. P., Hu, G. D., Fan, L. M., Song, C., et al. (2013). Effect of Decreasing Phosphorus in Eutrophic Water on the Community Structure of Phytoplankton. Ecol. Environ. Sci. 22 (9), 1578–1582.
Meng, S. L., Wang, J., Qiu, L. P., Hu, G. D., Qu, J. H., Fan, L. M., et al. (2015). Effect of Nitrogen and Phosphorus Mass Concentrations on the Growth and Competition of Chlorella Vulgaris and Anabaenasp. Strain PCC. Ecol. Environ. Sci. 24 (4), 658–664.
Meng, S. L., Li, D. D., Qiu, L. P., Hu, G. D., Fan, L. M., Song, C., et al. (2017). Effect of Organic Fertilizer and Algae Addition to tilapia Aquaculture Water on the Community Structure of Phytoplankton. J. Agro-Environment Sci. 36 (10), 2099–2105.
Ministry of Agriculture and Rural Affairs of the PRC (2007). Requirement for Water Discharge from Freshwater Aquaculture Pond SC/T9101. Beijing, China: China Agriculture Press.
Ministry of Ecology and Environment of the PRC (2002). Environmental Quality Standards for Surface Water GB 3838. Beijing, China: Environmental Press.
Nakajima, M., Hokoi, S., Ogura, D., and Iba, C. (2020). Field Survey of the Relationship between Environmental Conditions and Algal Growth on Exterior walls. Building Environ. 169, 106575. doi:10.1016/j.buildenv.2019.106575
Paerl, H. (2008). Nutrient and Other Environmental Controls of Harmful Cyanobacterial Blooms along the Freshwater-marine Continuum. Cyanobacterial Harmful Algal Blooms. State. Sci. Res. Needs. 619, 217–237. doi:10.1007/978-0-387-75865-7_10
Peng, X., Meng, F., Wang, Y., Yi, X., and Cui, H. (2020). Effect of pH, Temperature, and CO2 Concentration on Growth and Lipid Accumulation of Nannochloropsis Sp. MASCC 11. J. Ocean Univ. China 19 (5), 1183–1192. doi:10.1007/s11802-020-4302-y
Peng, Y., Zhang, Z., Wang, M., Shi, X., Zhou, Y., Zhou, Y., et al. (2020). Inactivation of Harmful Anabaena Flos-Aquae by Ultrasound Irradiation: Cell Disruption Mechanism and Enhanced Coagulation. Ultrason. Sonochem. 69, 105254. doi:10.1016/j.ultsonch.2020.105254
Piazzi, L., and Ceccherelli, G. (2002). Effects of Competition between Two Introduced Caulerpa. Mar. Ecol. Prog. Ser. 225, 189–195. doi:10.3354/meps225189
Renaud, S. L. B., Pick, F. R., and Fortin, N. (2011). Effect of Light Intensity on the Relative Dominance of Toxigenic and Nontoxigenic Strains of Microcystis Aeruginosa. Appl. Environ. Microbiol. 77, 7016–7022. doi:10.1128/AEM.05246-11
Shi, X. F., Wei, S. Q., Xie, D. T., and He, J. P. (2004). Characteristics of Phosphorus Adsorption of the Soils in the Drawdown Area of the Three - Gorges Reservoir. J. Southwest Agric. Univ. (natural science) 26 (3), 331–335. doi:10.1300/J064v24n01_09
Song, Z. G., Gao, L., Fan, J., Zhang, H. T., Wu, C. X., and Wang, Y. S. (2013). Effects of Environmental Factors on Algal Growth in Fenhe Water Park. Chin. J. Environ. Eng. 7 (10), 3997–4003.
Stn, M. B., and Bykiik, H. B. (2020). Effects of Light, Temperature, Nutrients and Salinity on the Growth of Cylindrotheca Closterium (ehrenberg) Reimann & J. Lewin, 1964 Species Isolated from Izmir bay. Ege J. Fish. Aquat. Sci. 37 (2), 157–166. doi:10.12714/egejfas.37.2.06
Toth, G. B., Harrysson, H., Wahlström, N., Olsson, J., Oerbekke, A., Steinhagen, S., et al. (2020). Effects of Irradiance, Temperature, Nutrients, and pCO2 on the Growth and Biochemical Composition of Cultivated Ulva Fenestrata. J. Appl. Phycol 32 (5), 3243–3254. doi:10.1007/s10811-020-02155-8
Wang, Z. H., Chui, F. Y., and An, Q. (2005). Influence of Water Temperature and Trophic Value on Algae Blooming in Reservoirs. Ecol. Environ. 14 (1), 10–15. doi:10.1007/s10971-005-6694-y
Wang, C., Wang, H. R., Xu, X. H., Wu, Z. Y., Kong, H. N., and He, S. B. (2010). Interactive Effects of Irradiance and Phosphorus on Microcystis Aeruginosa. Environ. Sci. Technol. 33 (4), 35–38. doi:10.1631/jzus.A1000244
Wang, J., Qiu, L. P., Meng, S. L., Fan, L. M., Song, C., and Chen, J. Z. (2015). Influences of Nitrogen-Phosphorus Ratio on the Growth and Competition of Chlorella Vulga and Anabaena sp.Strain PCC. Agric. Sci. Technol. 16 (8), 1757–1762. doi:10.3969/j.issn.1009-4229.2015.08.042
Wu, J., Chen, X. C., Kong, H. N., An, Y., Wu, C., and He, S. B. (2012). The Effect of Light Intensity on the Cell Density and Chain Length of Anabaena Flos-Aquae. China Environ. Sci. 32 (5), 875–879. doi:10.3969/j.issn.1000-6923.2012.05.016
Xu, Q. J., Gao, G., and Chen, W. M. (2004). Studies on Microcystin from Population Competition Aspect. J. Biol. 21 (1), 17–19. doi:10.3969/j.issn.2095-1736.2004.01.007
Xu, H., Zhu, G. W., Qin, B. Q., and Gao, G. (2011). Influence of Nitrogen-Phosphorus Ratio on Dominance of Bloom-Forming Cyanobacteria (Microcystis Aeruginosa). China Environ. Sci. 31 (10), 1676–1683. doi:10.1080/00405000.2010.522047
Xu, G. C., Duan, D. X., Zhang, J. L., and Du, X. H. (2014). Effects of Environmental Factors on the Growth and Development in Fish. J. Shandong Normal Univ. (Natural Science) 29 (1), 147–150. doi:10.3969/j.issn.1001-4748.2014.01.034
Yang, K., and Lu, W. X. (2014). Study on Water Quality Purification of Chlorella Vulgaris in Aquaculture Water. Inner Mongolia Environ. Sci. 7, 62–64. doi:10.3969/j.issn.1007-0370.2014.07.019
Yu, J., Zhu, Y. G., Hu, J. C., and Mao, Z. K. (2016). Control Method for Cyanobacteria in Aquaculture Pond. Scientific Fish Farming 6, 60–61. doi:10.14184/j.cnki.issn1004-843x.2016.06.037
Yue, X. L., Zhang, X. P., Hu, X. W., and Dong, Y. Y. (2006). Inhibitory Effects on Anabaena Flos-Aquae Growth by Metsulfuron-Methyl and Bensulfuron-Methyl. Trans. Chin. Soc. Agric. Eng. 22 (8), 175–178. doi:10.1111/j.1744-7917.2006.00098.x
Zhao, L. F., Ding, X. Y., Lu, L., and Li, M. (2014). Effect of Nutrition and Ca2+ on Growth and Competition of M. Aeruginosa and S. Quadricauda. Environ. Sci. Technol. 37 (1), 13–17. doi:10.3969/j.issn.1003-6504.2014.01.003
Zhao, H. T., Yang, S. W., Chao, D. J., and Wang, S. R. (2011). Research on the Growth and Competition of Algae in Different Water. Anhui Agicultural Sci. Bull. 17 (14), 108–109. doi:10.3969/j.issn.1007-7731.2011.14.052
Keywords: Chlorella vulgaris, Anabaena sp. strain PCC, environment factors, interspecies competition, temperature, light, nutrients, pH
Citation: Meng SL, Chen X, Wang J, Fan LM, Qiu LP, Zheng Y, Chen JZ and Xu P (2021) Interaction Effects of Temperature, Light, Nutrients, and pH on Growth and Competition of Chlorella vulgaris and Anabaena sp. Strain PCC. Front. Environ. Sci. 9:690191. doi: 10.3389/fenvs.2021.690191
Received: 02 April 2021; Accepted: 02 July 2021;
Published: 16 July 2021.
Edited by:
Wei Wu, University of Southern Mississippi, United StatesReviewed by:
Min Chao, Chinese Academy of Fishery Sciences, ChinaBin Xia, Chinese Academy of Fishery Sciences (CAFS), China
Copyright © 2021 Meng, Chen, Wang, Fan, Qiu, Zheng, Chen and Xu. This is an open-access article distributed under the terms of the Creative Commons Attribution License (CC BY). The use, distribution or reproduction in other forums is permitted, provided the original author(s) and the copyright owner(s) are credited and that the original publication in this journal is cited, in accordance with accepted academic practice. No use, distribution or reproduction is permitted which does not comply with these terms.
*Correspondence: Jia Zhang Chen, Y2hlbmp6QGZmcmMuY24=; Pao Xu, eHVwQGZmcmMuY24=
†These authors have contributed equally to this work