- College of Agriculture, Guizhou University, Guiyang, China
Contamination of food with the heavy metal Cd is a significant global concern. In this study, a field survey was performed to investigate the characteristics of Cd transfer from soil to potato tubers (n = 105). The results showed that the bioaccumulation factor of the potato tuber ranged from about 0.1 to 1. The soil threshold of Cd derived from the cumulative probability distribution was 0.15 mg kg−1 in order to protect 95% of potatoes. Additionally, prediction models for Cd transfer were constructed based on soil properties and the concentration of CaCl2-extractable soil Cd. The results of the analysis showed that pH was the critical factor affecting Cd uptake by potatoes. Additionally, the R2 of different empirical models increased from 0.354 to 0.715 as the number of soil parameters was increased, and the predicted soil Cd concentration approached the measured values at values of about 0–15 mg kg−1. The results of this study suggest that the probability distribution method was stricter than the empirical prediction models for estimating the ecological risk of Cd contamination of potatoes in karst soils.
Introduction
With the development of industry and agriculture in China, contamination of food by the trace metal cadmium (Cd) has become a public concern due to its toxicity and persistence in soil. Soil Cd has negative effects on crop growth and production (e.g., wheat and rice) and can affect human health through the food chain (Diao, et al., 2005). Potatoes are an important food resource in China, which has the largest potato production in the world. In 2019, the Chinese potato planting area reached 4.7895 million hectares, and China’s potato production of 91.938 million tons accounted for 24.91% of the global total. The consumption of potatoes that have accumulated Cd from contaminated soil is a major issue because potatoes are widely planted around the world. For example, excessive Cd accumulated in potatoes (about 0.18 mg kg−1) was shown to be caused by Cd pollution (1.25 mg kg−1) in sierozem soil in Gansu Province, China (Liu, et al., 2019). Soil minerals in karst areas have high heavy metal content, and the metals in these minerals have been brought to the soil surface by geological and biological processes. Metal elements that have migrated to the surface have weak migration ability in the soil. Moreover, human activities in karst areas lead to a certain amount of heavy metal contamination in the local soil, further increasing the content of heavy metals and eventually leading to abnormal metal content (Tang, et al., 2021; Zhang, et al., 2021). Therefore, it is necessary to study the transfer of Cd from soil to crops in order to better control soil risks and ensure food safety.
In general, plant Cd concentrations are correlated with the soil total Cd. For example, soil total Cd accounted for 64% of the variation in the Cd concentration in potato tubers based on the results of stepwise multiple regression (n = 49) when irrigation was performed with river water contaminated by mining (Carla, et al., 2007). However, McLaughlin et al., (1997) found no significant correlation between the Cd content in soil and that in potato tubers in a study of 352 potato production sites in Australia. These contradictory results may be due to differences in study regions, experimental conditions, and plant varieties. Soil total Cd concentrations can indicate the pollution status of soil, but cannot accurately reflect the pollution risk of soil to plants. The content of bioavailable Cd in soil mainly refers to the Cd that can be absorbed by crops during the crop growth period. In fact, many researchers have found that plant Cd content is closely related to the available Cd content in soil that is extractable by chemical extractants like CaCl2 or CH3COONH4. The Tessier sequential extraction procedure can be used to extract five fractions of Cd with different reagents. For instance, Wei et al., 2020 used the five-step batch metal fractionation method to study the influence of spent mushroom substrate on Cd immobilization and soil amendment, and Mitchell et al., 2020 employed a similar method to explore the impact of low-temperature biochar on the Cd concentration of calcareous river sediments. While single extraction methods seem to be more convenient and practical, Cd and Zn extracted by 0.01 M CaCl2 were successfully used to predict Cd levels in brown rice planted in paddy fields across the western plains in Taiwan (Römkens, et al., 2009; Kara et al., 2004) assessed the soil Cd content in sowing regions of potato fields from a total of 45 different sampling stations in Turkey by using HCl + H2SO4. 0.01 M CaCl2 is a commonly used chemical extractant in various extraction methods used to research the bioavailability of Cd because 0.01 M CaCl2 solution matches the soil with respect to pH, concentration, and composition (Zhou, et al., 2019). Additionally, the physical and chemical properties of soil also have a great influence on the accumulation and absorption of Cd by crops. For example, soil pH and concentrations of Cl and Zn were found to be the main factors affecting the Cd concentration of potato tubers in Australia (McLaughlin et al., 1997). Furthermore, using a stepwise regression model, Rafiq et al., 2014 showed that the Cd phytoavailability of pak choi grown in Chinese soils was dependent on soil properties such as pH and organic matter content.
Empirical models such as Freundlich-type models have been widely used to study the accumulation and transfer of heavy metals between soil and plants. The Cd concentrations of plants and soil, as well as bioaccumulation factors (BAFs), could be added to Freundlich-type models in order to predict the phytoavailability of metals for the evaluation of environmental quality. For example, the Freundlich relationship was applied to the empirical modeling of soil parameters including clay content, soil organic matter, cation exchange capacity, and soil Cd to predict the Cd content in wheat grain (François, et al., 2009). Additionally, multiple linear regression models have been used to predict the metal content of plants. For instance, the uptake and transfer of Cd in potatoes (n = 10) was predicted based on metal bioavailability (ethylenediamine tetraacetic acid extract) and soil properties in two contaminated regions of Kosovo (Zogaj and Düring, 2016). Moreover, Xu et al., 2019 used a polynomial model to derive Cd soil–plant relationships and soil criteria in order to provide suggestions for food safety and soil management. In recent years, the cumulative probability distribution method has been applied to study the soil threshold Cd concentrations in order to improve crop protection. For example, species sensitivity distribution (SSD) methodology is mainly used to determine the concentration of a toxicant that is protective of different ratios of species (50, 90, or 95%) in the environment. At present, the probability distribution functions used to fit the toxicological data of pollutants include log-normal, log-logistic, and Burr III type models. Liu et al., 2015 derived the soil threshold of Cd in a wheat-producing area of China (n = 18) based on probability estimation using the log-normal function. Moreover, Ding et al., 2016 used the SSD to obtain the soil thresholds of Pb for root vegetables with a BAF-based prediction model depending on the combination of soil pH and cation exchange capacity (CEC). Additionally, Ding et al., 2018 applied a similar cumulative probability distribution (SSD) to determine the soil threshold of Cd based on 12 root vegetable cultivars fitted by a Burr type III distribution with three different Cd treatments in various types of soil. These studies show that linear regression models can be used to describe the transport and enrichment of heavy metals, and the relationships between heavy metals in soil and plants. Therefore, probability statistics such as the SSD method can be used to perform risk assessment of pollutants at the level of the whole ecosystem and set soil thresholds.
Paired soil and plant samples (n = 105) were collected, and concentrations of Cd were measured. The present study aimed to 1) investigate the characteristics of Cd transfer from soil to potato tubers in a karst area of Guizhou Province, China, 2) obtain the soil Cd threshold based on the cumulative probability distribution using the log-normal function, and 3) explore an empirical prediction model based on plant and soil Cd concentrations and soil factors like pH and organic matter in order to improve soil environmental quality and protect food safety and human health.
Materials and Methods
Sample Collection
Field research was carried out in farmland in Guizhou Province, China. This province has a high background content of Cd due to the prevalent karst geochemical conditions, and the problem of soil Cd pollution is particularly prominent. For example, the relationships between soil properties and the accumulation of heavy metals in Brassica campestris L. in a karst area of Guizhou Province showed that the background levels of heavy metals in the soil of Guizhou Province are generally high due to the influence of topography and the presence of soil parent material (Zhang, et al., 2020). An analysis of the risk posed by eight typical heavy metals in soils in Guizhou Province using province-wide data available in the literature revealed that Cd showed a very high potential ecological risk (Yu, et al., 2021). We selected the counties of Weining, Hezhang, Ceheng, Changshun, and Leigong, each of which contains a large proportion of carbonate rocks, for sampling. Soil was collected from the upper layer of the soil (0–20 cm). The distance between the sampling point and the local trunk road was more than 100 m.
Sample Analysis
The collected soil samples were air-dried and then crushed with wooden sticks before being passed through a nylon sieve with a diameter of 0.149 mm. The collected potato samples were washed with tap water to remove surface soil particles and were then washed with distilled water, put into an oven at 105–110°C for 15 min, and dried to a constant weight at 70–90°C in the oven. Finally, the samples were smashed for the subsequent analysis. The soil physical and chemical properties were determined by routine methods (Liu, et al., 2015), soil pH was determined by a glass electrode at a soil:water ratio of 1:2.5 (g ml−1), organic matter content was measured based on an oil bath heating method (potassium dichromate oxidation), and the results are shown in Table 1.
The total Cd in soil was determined as follows: we accurately weighed 0.1 g (accurate to 0.0001 g) of the sieved soil sample and placed it in a polytetrafluoroethylene (PTFE) tank, added 3 ml HNO3 and 3 ml HCl, and placed the capped tank in a matched steel pipe, which was sealed. The steel pipe was placed in an incubator and heated at 185°C at a pressure of 790 kPa for 8 h. After cooling to room temperature, the digestion solution was removed from the pipe, placed on an electric heating plate, heated to 160°C, and steamed until it was viscous. The residual digestion solution was transferred to a 50 ml volumetric flask and diluted to 50 ml with ultrapure water.
Soil bioavailable Cd was determined by CaCl2 extraction. A soil sample (2.00 g) was passed through a 0.2 mm sieve and placed in a 50 ml centrifuge tube. Then, 0.01 mol L−1 CaCl2 solution was added and the tube was shaken for 2 h and centrifuged at 3,500 r·min−1 for 5 min. The level of Cd in the supernatant was detected. We accurately weighed 0.3 g (accurate to 0.0001 g) of each plant sample, placed it in a PTFE tank, and soaked it in 6 ml of HNO3 overnight. Then, 2 ml of H2O2 solution was mixed into the above solution and the tank was covered and placed in a supporting steel pipe and sealed. Plant Cd was determined as follows: the steel pipe was placed in an oven and heated at 100°C for 2 h. Next, the temperature was raised to 140°C for 2 h, after which it was raised to 160°C for 4 h. The method for solution transferal and detection of the plant Cd concentration was the same as that used for the soil samples. A total of 10 ml of each digested sample solution was analyzed by inductively coupled plasma mass spectrometry to determine its Cd concentration (ICP-MS; Thermo Fisher Scientific, Waltham, MA, United States, and x2). Reference material GSS-5 and GSB-1 was adopted to ensure the quality control and the detected Cd concentration in soil and plant deviated about 15% from the true value.
Modeling
Statistical analysis was performed using Microsoft Excel 2010 and SPSS 22.0 software. The log-normal function was applied to fit the cumulative probability distribution curve (SSD curve) using SSD Generator V1 software. The cumulative probability distribution was obtained from the toxicological data for pollutants based on the concept of BAFs (Csoil = 0.1/BAF, 0.1 mg kg−1 was the limit of the Chinese food safety standard (GB 2762-2017) for Cd). The soil threshold values corresponding to different levels of protection or risk were obtained from the curve.
The BAF is the ratio of the metal concentration in plant tissues to that in the soil, and is calculated as follows:
where Cplant is the Cd concentration in the tuber of the test plant and Csoil is the Cd concentration in the test soil.
The relationships among soil Cd, plant Cd concentration, and soil properties were expressed by an empirical model as follows:
where a, b, and c reflected the relationships among soil Cd, soil plant Cd, and soil properties, while k was the intercept.
Results and Discussion
Probability Statistics for Soil Cd Threshold
The total Cd concentration of the soil samples varied between 0.15 and 37.4 mg kg−1, with an average value of 4.98 mg kg−1, and the Cd concentration of the potato samples varied between 0.03 and 0.96 mg kg−1, with an average value of 0.29 mg kg−1 (Figure 1A). The soil and plant Cd concentrations generally exceeded the Chinese soil environmental quality risk control standard for soil contamination of agricultural land (GB 15618-2018; soil screening levels of 0.3 and 0.6 mg kg−1, respectively, for pH ≤ 7.5 and pH > 7.5) and food hygiene standards (GB 2762-2017, <0.1 mg kg−1). The soil available Cd concentration varied between 0.0003 and 0.67 mg kg−1, with an average value of 0.07 mg kg−1 (Figure 1B). The extractable Cd was far less than the soil total Cd; the average soil total Cd concentration was about 71 times that of the average extractable Cd concentration. Although the total Cd concentration in the soil was high, only a small proportion of this Cd could be absorbed by plants. The variation in soil total Cd and soil available Cd concentration are shown in Figures 1A,B. Previous studies found that the soil Cd significantly affected the Cd content of plants; for example, Liu et al., 2010 observed a significant difference (p < 0.05) in the Cd concentration of 40 Chinese cabbage shoots (from 0.88 to 7.76 mg kg−1) when the soil Cd concentration increased from 1.0 to 5.0 mg kg−1. However, Christopher et al., 2019 found that the Cd concentration in the flesh of potato was 0.2 mg kg−1 when the soil Cd concentration was lower than the detection limit. The results of experiments assessing the relationship between soil Cd content and plant Cd content have been inconsistent. Some studies have found that soil Cd levels were significantly related to plant Cd levels, while others have reported no relationship between these factors, perhaps due to the differences in plant species, climate, and other conditions.
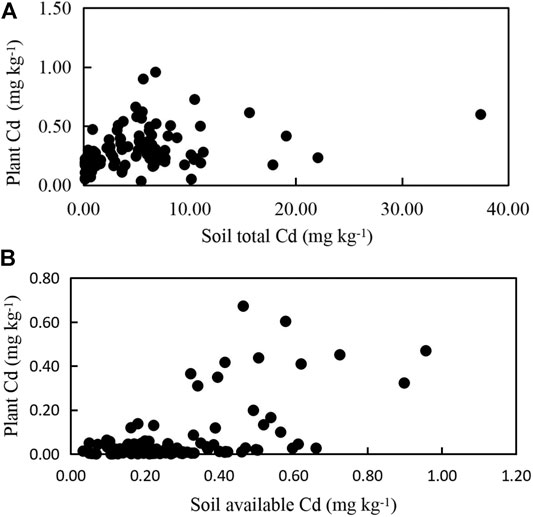
FIGURE 1. Relationship between the plant Cd concentration and soil total Cd (A), and the relationship between the plant Cd concentration and soil available Cd (B).
The variation in BAFs calculated by Eq. 1 was relatively large (about three orders of magnitude), facilitating the study of Cd transfer from soil to plants (Figure 2A). This large variation may be due to the fact that the external environment, including soil properties, plant varieties, irrigation, and fertilizer management, differed among the various study fields (Liu, et al., 2015). Statistical methods have been used to describe the probability distribution characteristics of BAFs in order to analyze Cd accumulation in potatoes. In the present study, it was found that the major BAF of Cd for plant uptake was in the range of 0.005–1.40, which is close to the BAF range found in a field survey of potatoes in four regions of New Zealand (Gray, et al., 2019). The critical value of the Cd concentration in soil may be deduced in terms of the limit of the Cd concentration in plants according to the measured bioaccumulation value (BAF = Cplant/Csoil, Cplant is 0.1 mg kg−1, which is the maximum value of Cd in potato tubers according to Chinese national food safety standards, and the corresponding Csoil is the soil Cd criteria) (Diao, et al., 2005). The soil Cd critical value of sampling soil sites can be used to fit the cumulative distribution curve of the soil threshold in order to prevent Cd contamination of potatoes in karst areas. In the present study, the soil Cd concentration that protected potatoes in 95% of soil sites was taken as the soil threshold of Cd. The resulting probability cumulative distribution can be built in many ways. For example, probability cumulative distribution functions, such as log-normal, log-logistic, or Burr type III, have been applied to study the cumulative distribution of the soil or water threshold of Cd. In general, it is necessary to estimate the potential risk of pollutants more conservatively for the risk assessment and derivation of soil environmental thresholds. In a previous study, the soil threshold of As for eight Chinese wheat varieties was calculated for 18 soils using cumulative probability distribution methods, including the Burr type III function, based on the protection of 95% of wheat varieties (Dai et al., 2016). In addition, hazardous concentrations of nonylphenol in the soil environment were estimated using probabilistic approaches based on acute and chronic species sensitivity distributions (Kwak, et al., 2017).
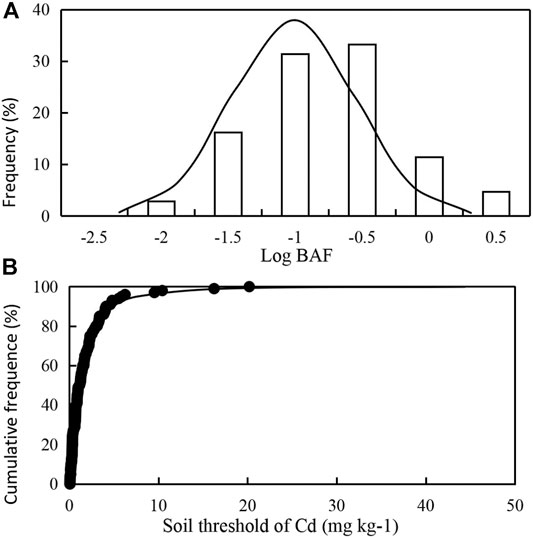
FIGURE 2. Frequency distribution of the bioaccumulation factor (BAF) (A) and the cumulative probability distribution of the soil Cd threshold (B).
The cumulative probability distribution curve shown in Figure 2B characterizes the ecological risk to ecosystems; the potential affected fraction of Cd for the environment was calculated according to a given soil threshold Cd concentration (such as 0.3 or 0.6 mg kg−1). Furthermore, the distribution curve was also used to determine the soil threshold Cd concentration based on a given protection probability. For example, when the cumulative frequency was 20, 80% (100–20%) of soil sites have a safe soil Cd concentration below the soil Cd threshold. In the present study, it was found that the soil Cd threshold for the study area was 0.15 mg kg−1 to protect the plant samples in 95% of the soil sites based on the cumulative probability distribution (cumulative frequency = 5%) (Figure 2B). The cumulative probability distribution can also be used to obtain other soil Cd thresholds for protecting different proportions of potatoes. The soil Cd threshold determined in this study as sufficient for protection of 95% of soil sites in karst areas was slightly lower than the Chinese soil environmental quality risk control standard for the contamination of agricultural soil (GB 15618-2018). These results indicate that potatoes grown in karst areas, in which tubers may more easily accumulate Cd from soil, may be more susceptible to soil Cd pollution in comparison with those grown in other areas.
Prediction Model for Soil and Plant Cd Concentration
Since the biochemical behavior of Cd in soil is complex, and many factors affect Cd uptake by plants, it is important to study the toxicity of Cd in mature crops in order to protect human health. Empirical regression can be used to estimate the rate of accumulation or transfer of heavy metals from soil to crops based on soil properties. For example, Liang et al., 2013 log-transformed the Cd content of spinach and soil to normalize variance and match a Freundlich-type function, and they added soil properties such as pH to the prediction model to improve its fitting accuracy. Liu et al., 2015 used a similar empirical method to fit a soil Cd threshold model using 18 Chinese soil types to prevent contamination of wheat by Cd. In the present study, we found that log CdCaCl2 and log Cdtotal were both extremely significantly positively correlated with log Cdplant (p < 0.01). The Pearson correlation coefficient was 0.434 for both log CdCaCl2 and log Cdplant, where it was 0.499 for log Cdtotal and log Cdplant (Table 2). These results indicate that both the soil total Cd and the CaCl2-extracted Cd played an important role in Cd absorption by potato tubers. These findings are similar to those reported by Jun et al., 2018 from a study of the effects of alkaline amendment on Cd bioavailability in two Chinese cultivars of polished rice (Xiangwanxian 13 and Zhongyou 9,918) grown in paddy soil. A recent study using single extraction procedures, including methods utilizing EDTA, DTPA, CH3COOH, and HCl, revealed that the extractable Cd in soil was linearly correlated with the Cd concentration in wheat grains (Liu et al., 2019). These results are consistent with our findings, which showed a similar relationship between Cd in soil and plant samples.
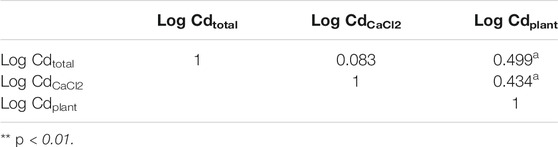
TABLE 2. Pearson correlations between soil total Cd (Cdtotal), soil CaCl2-extractable Cd (CdCaCl2), and plant Cd concentration (Cdplant).
A stepwise linear regression was performed to establish the relationship between log Cdsoil (soil total Cd), log CdCaCl2 (soil CaCl2-extracted Cd), and soil pH and organic matter (OM) (n = 105) based on Eq. 2. The results of the prediction models showed that Cdtotal was significantly correlated with the soil pH and OM, soil-extractable CdCaCl2, and Cdplant; the R2 value of the prediction model increased from 0.354 to 0.715 as the number of independent variables was increased (Table 3). Linear regression equations were obtained to describe the relationships between the total Cd concentration in soil and the Cd concentrations in wheat grains and roots (R2 = 0.62, 0.71 for grains and roots, respectively), as well as between the CaCl2-extracted Cd concentration in soil and the Cd concentrations in wheat grains and roots (R2 = 0.58, 0.49 for grains and roots, respectively). A recent study indicated that the soil total Cd and CaCl2-extracted Cd were useful predictors of the Cd transfer from soil to plants (Qu, et al., 2020), which was in agreement with the results described in the present study. Soil pH was an important predictive factor, as shown by its presence in every prediction model in Table 3, and, among the independent variables in equation (4), pH contributed approximately 47% of the variance in the dependent variables.
Prediction of soil threshold Cd values by linear equations is more convenient than prediction by the cumulative probability analysis method. The soil threshold calculated by the empirical prediction equation was greater than that calculated by the probability statistic method. For example, the soil thresholds of Cd were 0.35, 0.97, and 1.60 mg kg−1 when the soil pH was 5.5, 6.5, and 7, respectively, and the soilCaCl2, OM, and Cdplant were 0.1 mg kg−1, 10 g kg−1, and 0.1 mg kg−1, according to equation (4) in Table 3. Previous studies have shown that soil properties, especially pH, are crucial factors influencing the transport of Cd between soil and vegetables. pH mainly affects the equilibrium distribution of heavy metal ions at the two-phase interface of soil and water, as well as the formation and dissolution of soil carbonate. Soil organic matter has a large number of functional groups, and its ability to adsorb Cd is much higher than that of other mineral colloids. Humic acid formed by decomposition can chelate (complex) with heavy metals, inhibiting the absorption of metals by plants. Using a stepwise regression model, Rafiq et al., 2014 concluded that Cd phytoavailability to pak choi was significantly affected by soil pH, organic matter, and total Zn and Cd concentrations, and the R2 of the stepwise regression model in their report was 0.977. Furthermore, through stepwise forward multiple regressions, McLaughlin et al. (1997) found that soil parameters such as pH and Cl were important influences on the Cd concentration of potato tubers. Furthermore, the measured soil Cd concentrations in the present study were close to the values predicted using equation (4) (Table 3) for a certain soil Cd concentration range (about 0–15 mg kg−1). The predicted soil Cd concentrations were greater than the measured values for higher soil Cd concentrations (Figure 3). In contrast with our results, a recent study reported no significant relationships between soil properties and the Cd concentration in potatoes in three field sites across New Zealand (Gray, et al., 2019). A recent study in Hunan province reported a soil Cd threshold of 1.819–3.272 mg kg−1 based on the safety limit of Cd in cereals (0.2 mg kg−1), which was higher than that permitted by the national soil environmental standards of China (Chen, et al., 2019). Using a prediction model, the threshold of soil total Cd in rootstalk vegetable fields in Guangdong was calculated to be 0.94 mg kg−1, which was also higher than the national soil Cd threshold in China (Sun, et al., 2013). Although the threshold values of Cd from studies performed in non-karst area were higher than those from our research, these differences are likely due to differences in soil conditions, pollution conditions, plant species, and other factors. Further research is required to compare Cd uptake rates and soil threshold values for karst and non-karst areas.
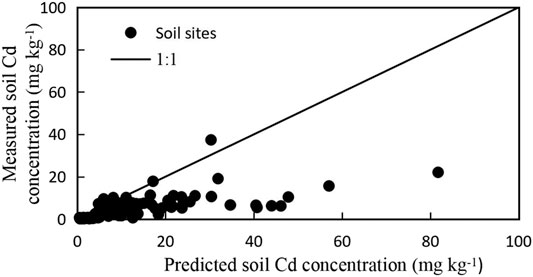
FIGURE 3. Relationship between the measured and predicted soil Cd concentrations for different soil sites.
Conclusion
In the frequency distribution of the BAF of potato tubers, BAF values from 0.1 to 0.3 occurred relatively frequently for soil Cd concentrations ranging from 0.15 to 37.4 mg kg−1. The transfer of Cd from soil with a high Cd concentration to potato tubers was found to be highly positively correlated with soil pH, OM, and CaCl2-extractable Cd (R2 = 0.715). The log-transformed plant Cd concentration was highly significantly correlated with the soil total Cd and soil CaCl2-extractable Cd concentrations, with Pearson correlations of 0.499 and 0.434, respectively. The log-normal function was used to establish the cumulative probability distribution of the soil Cd threshold. Our results show that the probability model and empirical model can each adequately describe the transfer of Cd from soil to crops.
Data Availability Statement
The original contributions presented in the study are included in the article/Supplementary Material, and further inquiries can be directed to the corresponding author.
Author Contributions
KL analyzed the data and wrote the manuscript, HL revised the manuscript, XZ collected the samples, and ZC and XW provided important suggestions for the manuscript.
Funding
This research was supported by the Joint Projects of the National Natural Science Foundation of China (42067028) and the Science and Technology Planning Project of Guizhou Province, China (Qiankehejichu no. (2019)1103, Qiankehehoubuzu no. (2020)3001).
Conflict of Interest
The authors declare that the research was conducted in the absence of any commercial or financial relationships that could be construed as a potential conflict of interest.
References
Carla, O., Carlo, V., and Erik, S. (2007). Elevated Cadmium Concentrations in Potato Tubers Due to Irrigation with River Water Contaminated by Mining in Potosí, Bolivia. J. Environ. Qual. 36, 1181–1186. doi:10.1016/j.ecoenv.2016.04.031
Chen, Q., Peng, P., Hou, H., Ding, X., Long, J., au, X., et al. (2019). Effects of Soil Properties on the Cd Threshold in Typical Paddy Soils Using BCR Sequential Extraction. Hum. Ecol. Risk Assess. Int. J. 25, 2160–2173. doi:10.1080/10807039.2018.1490998
Christopher, N., Shiv, P., Eman, E., Jaskaran, D., Ali, M., and Ramanbhai, P. (2019). Effect of Biochar on Heavy Metal Accumulation in Potatoes from Wastewater Irrigation. J. Environ. Manage. 232, 153–164. doi:10.1016/j.ecoenv.2016.04.031
Dai, Y., Lv, J., Liu, K., Zhao, X., and Cao, Y. (2016). Major Controlling Factors and Prediction Models for Arsenic Uptake from Soil to Wheat Plants. Ecotoxicology Environ. Saf. 130, 256–262. doi:10.1016/j.ecoenv.2016.04.031
Diao, W.-P., Ni, W.-Z., Ma, H.-Y., and Yang, X.-E. (2005). Cadmium Pollution in Paddy Soil as Affected by Different Rice (Oryza Sativa L.) Cultivars. Bull. Environ. Contam. Toxicol. 75, 731–738. doi:10.1007/s00128-005-0812-y
Ding, C., Ma, Y., Li, X., Zhang, T., and Wang, X. (2016). Derivation of Soil Thresholds for lead Applying Species Sensitivity Distribution: A Case Study for Root Vegetables. J. Hazard. Mater. 303, 21–27. doi:10.1016/j.jhazmat.2015.10.027
Ding, C., Ma, Y., Li, X., Zhang, T., and Wang, X. (2018). Determination and Validation of Soil Thresholds for Cadmium Based on Food Quality Standard and Health Risk Assessment. Sci. Total Environ. 619-620, 700–706. doi:10.1016/j.scitotenv.2017.11.137
François, M., Grant, C., Lambert, R., and Sauvé, S. (2009). Prediction of Cadmium and Zinc Concentration in Wheat Grain from Soils Affected by the Application of Phosphate Fertilizers Varying in Cd Concentration. Nutr. Cycl Agroecosyst 83, 125–133. doi:10.1007/s10705-008-9204-0
Gray, C. W., Yi, Z., Lehto, N. J., Robinson, B. H., Munir, K., and Cavanagh, J.-A. E. (2019). Effect of Cultivar Type and Soil Properties on Cadmium Concentrations in Potatoes. New Zealand J. Crop Hortic. Sci. 47, 182–197. doi:10.1080/01140671.2019.1599028
Jun, M., Libin, Z., Lu, W., Xingmei, L., Caixian, T., Hongjin, C., et al. (2018). Contrasting Effects of Alkaline Amendments on the Bioavailability and Uptake of Cd in rice Plants in a Cd-Contaminated Acid Paddy Soil. Environ. Sci. Pollut. Res. Int. 25, 8827–8835. doi:10.1007/s11356-017-1148-y
Kara, E. E., Pirlak, U., and Özdılek, H. G. (2004). Evaluation of Heavy Metals' (Cd, Cu, Ni, Ph, and Zn) Distribution in Sowing Regions of Potato Fields in the Province of Niğde, Turkey. Water Air Soil Pollut. 153, 173–186. doi:10.1023/b:wate.0000019942.37633.31
Kwak, J. I., Moon, J., Kim, D., Cui, R., and An, Y.-J. (2017). Species Sensitivity Distributions for Nonylphenol to Estimate Soil Hazardous Concentration. Environ. Sci. Technol. 51, 13957–13966. doi:10.1021/acs.est.7b04433
Liang, Z., Ding, Q., Wei, D., Li, J., Chen, S., and Ma, Y. (2013). Major Controlling Factors and Predictions for Cadmium Transfer from the Soil into Spinach Plants. Ecotoxicology Environ. Saf. 93, 180–185. doi:10.1016/j.ecoenv.2013.04.003
Liu, K., Lv, J., He, W., Zhang, H., Cao, Y., and Dai, Y. (2015). Major Factors Influencing Cadmium Uptake from the Soil into Wheat Plants. Ecotoxicology Environ. Saf. 113, 207–213. doi:10.1016/j.ecoenv.2014.12.005
Liu, W., Zhou, Q., An, J., Sun, Y., and Liu, R. (2010). Variations in Cadmium Accumulation Among Chinese Cabbage Cultivars and Screening for Cd-Safe Cultivars. J. Hazard. Mater. 173, 737–743. doi:10.1016/j.jhazmat.2009.08.147
Liu, Z., Bai, Y., and Yang, T. (2019). Effect of Cd on the Uptake and Translocation of Pb, Cu, Zn, and Ni in Potato and Wheat Grown in Sierozem. Soil Sediment. Contam. 28, 650–669. doi:10.1080/15320383.2019.1643289
McLaughlin, M. J., Maier, N. A., Rayment, G. E., Sparrow, L. A., Berg, G., au, A., et al. (1997). Cadmium in Australian Potato Tubers and Soils. J. Environ. Qual. 26, 1644–1649. doi:10.2134/jeq1997.00472425002600060026x
Mitchell, K., Mendoza-González, C. V., Ramos-Gómez, M. S., Yamamoto-Flores, L., Guerrero-Barrera, A. L., Macias-Medrano, R., et al. (2020). The Effect of Low-Temperature Biochar and its Non-pyrolyzed Composted Biosolids Source on the Geochemical Fractionation of Pb and Cd in Calcareous River Sediments. Environ. Earth Sci. 79. doi:10.1007/s12665-020-08908-5
Qu, X., Xu, W., Ren, J., Zhao, X., Li, Y., and Gu, X. (2020). A Field Study to Predict Cd Bioaccumulation in a Soil-Wheat System: Application of a Geochemical Model. J. Hazard. Mater. 400, 123135. doi:10.1016/j.jhazmat.2020.123135
Rafiq, M. T., Aziz, R., Yang, X., Xiao, W., Stoffella, P. J., Saghir, A., et al. (2014). Phytoavailability of Cadmium (Cd) to Pak Choi (Brassica Chinensis L.) Grown in Chinese Soils: A Model to Evaluate the Impact of Soil Cd Pollution on Potential Dietary Toxicity. PLoS One 9, e111461. doi:10.1371/journal.pone.0111461
Römkens, P. F. A. M., Guo, H. Y., Chu, C. L., Liu, T. S., Chiang, C. F., and Koopmans, G. F. (2009). Prediction of Cadmium Uptake by Brown rice and Derivation of Soil-Plant Transfer Models to Improve Soil protection Guidelines. Environ. Pollut. 157, 2435–2444. doi:10.1016/j.envpol.2009.03.009
Sun, F. F., Wang, F. H., Wang, X., He, W., Wen, D., Wang, Q. F., et al. (2013). Soil Threshold Values of Total and Available Cadmium for Vegetable Growing Based on Field Data in Guangdong Province, South China. J. Sci. Food Agric. 93, 1967–1973. doi:10.1002/jsfa.6000
Tang, M., Lu, G., Fan, B., Xiang, W., and Bao, Z. (2021). Bioaccumulation and Risk Assessment of Heavy Metals in Soil-Crop Systems in Liujiang Karst Area, Southwestern China. Environ. Sci. Pollut. Res. 28, 9657–9669. doi:10.1007/s11356-020-11448-x
Wei, Y., Jin, Z., Zhang, M., Li, Y., Huang, S., au, X., et al. (2020). Impact of Spent Mushroom Substrate on Cd Immobilization and Soil Property. Environ. Sci. Pollut. Res. 27, 3007–3022. doi:10.1007/s11356-019-07138-y
Xu, G., Zhang, S., Song, J., Brewer, R., and Gao, H. (2019). Cadmium Uptake in Radish (Raphanus Sativus L.) and Surficial Contamination: Implications for Food Safety and Local Soil Management. J. Soils Sediments 19, 3585–3596. doi:10.1007/s11368-019-02290-x
Yu, G., Chen, F., Zhang, H., and Wang, Z. (2021). Pollution and Health Risk Assessment of Heavy Metals in Soils of Guizhou, China. Ecosystem Health and Sustainability 7. doi:10.1080/20964129.2020.1859948
Zhang, J., Mu, G., Zhang, Z., Huang, X., and Fang, H. (2021). Speciation Variation and Bio-Activation of Soil Heavy Metals (Cd and Cr) in Rice-Rape Rotation Lands in Karst Regions. Int. J. Env. Res. Pub. He. 18. doi:10.3390/ijerph18031364
Zhang, Z., Wu, X., Tu, C., Huang, X., Zhang, J., Fang, H., et al. (2020). Relationships between Soil Properties and the Accumulation of Heavy Metals in Different Brassica Campestris L. Growth Stages in a Karst Mountainous Area. Ecotox. Environ. Safe. 206. doi:10.1016/j.ecoenv.2020.111150
Zhou, W., Zhang, J., Zou, M., Liu, X., Di, X., Wang, Q., et al. (2019). Feasibility of Using rice Leaves Hyperspectral Data to Estimate CaCl2-Extractable Concentrations of Heavy Metals in Agricultural Soil. Sci. Rep.-UK 9. doi:10.1038/s41598-019-52503-z
Keywords: cadmium, potato, soil threshold, prediction model, probability statistics
Citation: Liu K, Liu H, Zhou X, Chen Z and Wang X (2021) Prediction of Cadmium Transfer From Soil to Potato in Karst Soils, China. Front. Environ. Sci. 9:684887. doi: 10.3389/fenvs.2021.684887
Received: 24 March 2021; Accepted: 18 May 2021;
Published: 15 June 2021.
Edited by:
Jun Zhou, University of Massachusetts Lowell, United StatesReviewed by:
Jiakai Gao, Henan University of Science and Technology, ChinaHongbiao Cui, Anhui University of Science and Technology, China
Copyright © 2021 Liu, Liu, Zhou, Chen and Wang. This is an open-access article distributed under the terms of the Creative Commons Attribution License (CC BY). The use, distribution or reproduction in other forums is permitted, provided the original author(s) and the copyright owner(s) are credited and that the original publication in this journal is cited, in accordance with accepted academic practice. No use, distribution or reproduction is permitted which does not comply with these terms.
*Correspondence: Hongyan Liu, aHlsaXVAZ3p1LmVkdS5jbg==