- 1Institute for Land, Water and Society, Charles Sturt University, Albury, NSW, Australia
- 2Aquatic Systems Biology, Technical University of Munich, Freising, Germany
- 3Fisheries Research Station Baden-Württemberg, LAZBW, Langenargen, Germany
- 4Institute for Limnology, University of Constance, Konstanz, Germany
The fish community of Lake Constance, a large, deep, oligotrophic lake has undergone drastic changes in recent years, with the sudden rise to dominance of invasive three-spined stickleback (Gasterosteus aculeatus) in the pelagic zone, a rather atypical habitat for this species in Central Europe. The core objective of this study was to compare the feeding ecology of stickleback and young Eurasian perch (Perca fluviatilis) in this unique situation to identify reasons for this unexpected dominance, a possible food niche and feeding time overlap, and to discuss consequences for the reshaped pelagic fish community. The diel feeding patterns and prey compositions of pelagic sticklebacks and juvenile (0+) perch sampled in October 2018 and March 2019 were studied analyzing stomach contents. The diets of both species mostly comprised zooplankton, with copepods appearing in the greatest numbers. Benthic and airborne insects were consumed occasionally, mostly by sticklebacks. Both species exhibited peaks of feeding activity early in the morning, afternoon and dusk, and in both species, stomachs were fullest at dusk. Stickleback stomachs contained about 20% more prey at night than perch, and mean estimated nocturnal stomach fullness values were almost 50% greater. Night feeding in sticklebacks was confirmed by digestive states, pointing to a possible competitive advantage over perch. Dietary composition varied over a 24-h cycle and the pattern of consumption of different prey varied between the species. Perch consumed more comparatively small cladocerans (Bosmina spp.), while larger Daphnia appeared more often in stickleback stomachs. In both species, seasonal variation in diet mirrored food availability, indicating some degree of opportunism. A Morisita-Horn index value of 0.95 confirmed dietary niche overlap between species, suggesting the large population of sticklebacks may exert a competitive effect on juvenile perch when resources are limited. Both the longer feeding periods and greater intake of nutritive high quality prey like daphnids can contribute to the rapid success of stickleback in dominating the pelagic zone of Lake Constance.
Introduction
The three-spined stickleback (Gasterosteus aculeatus L. 1758) is a common fish species inhabiting both lotic and lentic freshwater ecosystems, as well as brackish and marine habitats throughout the northern hemisphere (Paepke, 1996; Dußling et al., 2018). The species is considered a highly adaptive and flexible feeder, with a diet typically comprising small invertebrates, zooplankton, amphibian larvae, fish eggs, and fry. Feeding strategy can shift between opportunist and specialist depending on habitat type, season, and other environmental factors (Gill and Hard, 1994; Sánchez-Gonzáles et al., 2001; Wootton, 2012; Demchuk et al., 2015). In Lake Constance, the three-spined stickleback is an invasive species, which first became established in the littoral zone between 1940 and 1950 (Roch, 2018). Since 2012/13 however, the species has appeared in very large numbers in the pelagic zone of Upper Lake Constance (Rösch et al., 2018; Eckmann and Engesser, 2019), and in 2014 it comprised more than 95% of pelagic fish abundance and made up about 28% of total fish biomass (Alexander et al., 2016). During recent years, highest abundances of sticklebacks were found in late summer, when densities exceeded 10,000 individuals per hectare (Gugele et al., 2020). To clarify the situation in Upper Lake Constance and the impact of the stickleback mass occurrence on co-occurring species and the pelagic food web, it is important to understand the species’ feeding ecology in relation to other species. The feeding ecology of the three-spined stickleback has been well-documented in a number of different habitats and localities, including small, and medium-sized lakes in Canada Manzer (1976) and Great Britain (Allen and Wootton, 1984; Wooton, 2012), small brackish or saltwater coastal lagoons Sánchez-Gonzáles et al. (2001) and the marine environment of the Baltic Sea (Peltonen et al., 2004; Bergström et al., 2015). However, almost no information has been published about sticklebacks feeding in the pelagic waters of large, deep oligotrophic lakes, which were not known as suitable habitats for the species so far.
The Eurasian perch (Perca fluviatilis L. 1758) is a common native and opportunistic species in the most parts of Europe and an ecologically significant component of many European freshwater food webs (Eckmann and Schleuter-Hofmann, 2013; Semeniuk et al., 2015). The species inhabits the pelagic zone of Lake Constance during early life (Wang and Eckmann, 1994; Wang and Appenzeller, 1998). Adult perch inhabit mostly the near-shore benthic habitat and are targeted by commercial fishermen and recreational anglers. Individuals switch from planktonic to benthic feeding behavior during their lifetime, and individual growth is strongly affected by ontogenetic dietary shift towards piscivory (Craig, 1978; Rask, 1986; Eckmann and Schleuter-Hofmann, 2013). Several authors have addressed the life history and feeding ecology of juvenile perch including studies of feeding rate in a laboratory (Nurminen et al., 2010), dietary composition in large lakes (Guma'a, 1978), and feeding activity and diel migration in small forest lakes (Rask, 1986). However, less is known about the feeding habits of young perch in the pelagic waters of large oligotrophic lakes, especially in the context of potential competition with invasive species.
Dietary analysis can help to quantify the risk to native fishes by predation or food competition by an introduced or invasive species (Fritts and Pearsons, 2004; Brandner et al., 2013; Manko, 2016). Diel patterns of feeding activity vary between fish species potentially affecting competition, with many species exhibiting peaks at certain times of day (López-Olmeda and Sánchez-Vázquez, 2010). In the absence of stickleback, Eurasian perch often exhibit highest feeding activity during the twilight periods of dusk and/or dawn, high feeding activity during midday and minimal feeding at night (Rask, 1986; Huusko et al., 1996; Jacobsen et al., 2002). Sticklebacks show clear feeding activity peaks at similar times in the absence of co-occurring perch (Manzer, 1976). Seasonal changes in feeding activity and behavior have been observed for both species (Craig, 1978; Allen and Wootton, 1984; Schleuter and Eckmann, 2008) in other waters.
Niche separation and the avoidance of direct competition between co-occuring species are fundamental for continued coexistence (Ward et al., 2006; Quirino et al., 2015). While previous studies have considered the feeding interaction and interspecific competition between juvenile perch and other fish species such as roach (Rutilus rutilus L. 1758) (Persson and Greenberg, 1990; Kahl and Radke 2006) and ruffe (Gymnocephalus cernua L. 1758) (Schleuter and Eckmann, 2006), interactions between stickleback and perch co-occurring in the pelagic zone of lakes have not yet been assessed.
The core objective of this study was to compare the feeding ecology and assess possible food niche and feeding time overlap between stickleback and perch from the pelagic zone of upper Lake Constance, based on a comparison of diel and seasonal feeding patterns and analysis of stomach contents. These aspects are relevant to identify reasons for the unexpected dominance of stickleback and to discuss consequences for the reshaped pelagic fish community. It was hypothesized that (i) peak feeding times for both species would coincide and that (ii) there would be large dietary overlap. Both of these aspects could set the basis for a possible competition for food between the two species.
Materials and Methods
Lake Constance is a large prealpine, monomictic, and oligotrophic lake in the Rhine drainage basin bordered by Austria, Germany and Switzerland (47°38′N; 9°22′E) (Werner et al., 2005; IGKB, 2013). It is the third-largest lake in Central Europe, after Lake Geneva and Lake Balaton, with a water surface of 536 km2. The lake consists of a 473 km2 upper lake and a 63 km2 lower lake connected by a narrow river, the “Seerhein” (Lang et al., 2010). Total lake volume is 48 km³, maximum depth is 254 m and shore length is 273 km (IGKB, 2013).
Fish were sampled in pelagic locations in Upper Lake Constance with known co-occurrence of native perch and invasive stickleback. Sampling was conducted with two different methods, namely trawling and gillnetting. The bulk of the samples was collected over a period of several days and nights in autumn 2018, with an additional night trawl and day of gillnet fishing conducted in spring in March 2019 to gain insight into seasonal feeding differences. The trawling was conducted over three consecutive nights from the 8th to the October 10, 2018 inclusive, and on the night of March 27—28, 2019 in 10–12 min bouts at approximately hourly intervals from 17:00 to 07:00 (Central European Time, CET), at an average speed of 3.5 km/h. The net frame was 3 × 2 m wide and the mesh size was 6 mm (knot to knot) in the front part and 4 mm in the codend. The net was fixed 112.1 m behind the vessel and set at depths of 3, 6, 9, or 12 m, depending on the location of fish signals visible on an echo sounder. Daytime sampling was performed with transparent gill nets on 15th, 16th, 17th, 25th October and November 5 and 6, 2018 (autumn) and on March 15, 2019 (spring). On each occasion sampling was conducted at two hourly intervals from 07:00 to 17:00, using gill nets with mesh sizes of 6, 8, 10, and 12 mm. The nets were 3 m deep and the total length was 120 m. They were set at depths of 9 and 12 m and fixed with an anchor and buoys. The depth of the lake at the sampling locations ranged from 100 to 186 m. In autumn, sunrise was around 07:30 and sunset around 19:50, varying about a few minutes depending on the sampling days. In spring, sunset of the sampled night was at 18:43 and sunrise at 06:09. The water temperature was 13–15°C in October and 5–7°C during March sampling. More than 94% of all captured perch, and more than 90% of all captured sticklebacks were sampled in co-occurrence with the other species in autumn. In all samples, 99.8% of the sampled fish were stickleback or perch, and only 0.2% were other species which were native whitefish (Coregonus spp. L. 1758), roach (Rutilus rultilus L. 1758), and bleak (Alburnus alburnus L. 1758). Captured fish were anaesthetized and killed immediately on site with an overdose of clove oil. In order to preserve the stomach content as quickly as possible, 70% ethanol was injected with a syringe via the mouth into the digestive tract and entire fish were placed into laboratory bottles filled with 70% ethanol where they were stored for at least 3 weeks until analysis. All caught perch were classified as 0 + perch according to total length, sampling place and gonadal development (see Wang and Eckmann, 1994). An additional age reading of the opercula from 20 randomly selected perch confirmed this outcome.
In preparation for analysis of stomach contents, fish were briefly air dried with tissue paper for 1 min. Each individual was then weighed to the nearest 0.0001 g on a micro-balance (Sartorius, Extend). Total length (TL) was measured to the nearest mm. The sex of sticklebacks was noted, but this was not possible for perch due to their early stage of gonadal development. Müller, 1776 Digestive tracts were removed and parasites (all Schistocephalus solidus) were counted, weighed and measured. The digestive tract was divided into oesophagus, stomach, and intestine, cleaned of other visceral material and remains, dried with tissue paper and weighed to the nearest 0.0001 g on a micro-balance. The stomach was then severed from the hindgut and opened with a small longitudinal slit. Fullness was categorized on a scale from 0–6, whereby 0 = empty; 1 = trace of food—25% full; 2 = 25–50% full; 3 = 50% or half full; 4 = 50–75% full; 5 = 75–100% full and 6 = distended/stuffed. The fullness scale and other methodological aspects of stomach content analysis were adapted from AFSC (2015); Brandner et al. (2013); Cunha et al. (2005); Garrido et al. (2008a) and Manko (2016). The fullness was first estimated in percent (0–100%) as described by Manko (2016) and then assigned to the appropriate fullness value. The contents of each stomach were carefully extracted using a blunt probe and washed into a Petri dish with a squirt bottle. The empty stomach and hindgut were dried and weighed to the nearest 0.0001 g to obtain the mass of gut contents. A microscopy coverslip was used to scrape out the hindgut contents, which were weighed separately. Stomach contents were not weighed, because the process would have affected the condition of prey and the assessment of digestive state. Values for the total mass of stomach content were instead calculated from the difference between the mass of the empty stomach and the mass of the intact stomach. Weight-based stomach fullness for each fish was calculated with the “Index of fullness” (IF) formula according to Hyslop (1980): (WS/WF) × 100, where WS is the stomach content weight and WF the total fish weight. In the case of parasite occurrence, the weight of the parasites was deducted from the total fish weight. Following the methodology of previous authors (e.g. Brandner et al., 2013; Manko, 2016), food items were determined and counted in a zooplankton counting chamber and assigned to the following categories: Bosmina, Copepoda, Daphnia/Diaphanosoma, Predatory zooplankton (Bythotrephes longimanus and Leptodora kindtii), Benthic organisms, Fish larvae/eggs, Airborne insects and Other (including all food items which could not be assigned to the selected groups).
Digestive state was used as a descriptor of stomach contents as a whole, based on the condition of the majority of material, assigned to one of six categories using the modified protocols of AFSC (2015) and Manko (2016), as follows: 1 = totally digested/stomach empty; 2 = traces of prey items in the stomach, with zooplankton not or only vaguely identifiable; 3 = < 50% of contents intact, 4 = 50–75% intact; 5 = 75–100% intact; 6 = no apparent digestion). In order to identify diel feeding pattern, stomach content samples were grouped into 2 h intervals over a 24 h period. In order to ensure an adequate sample size for each period, samples collected over multiple days were pooled into a single 24 h diel cycle, in close adherence to the recommendations of Benkwitt et al. (2009). For statistical comparison, data were additionally categorized by collection time as “dawn” (05:00–09:00), “day” (09:00–17:00), “dusk” (17:00–21:00) and “night” (21:00–05:00). Nautical twilights (dawn and dusk) thus contained two 2-h sampling periods each, since the precise times for actual twilight straddled both sampling periods. To quantify stomach fullness during the diel cycle, reduce size-dependent variation, and allow for comparison between fish of different size means and standard deviations for number of food items per TL (in cm) was calculated for each individual. Length was used instead of weight because the parasite Schistocephalus solidus has a significant effect on the weight of sticklebacks, but not on length (Barber and Svensson, 2003). All measuring and weighing was conducted after preservation. Due to the low impact of preservation method (Nordeide, 2020), the shrinking effect on length and weight of the fish was neglected. Data from fish sampled in autumn and spring were analyzed and evaluated separately, except for those used in the regression models, for which data were treated as one set. The analysis of diel stomach content patterns over 24 h was based on autumn data, excluding specimens with empty stomachs. The number of individuals of each food item category was counted for each stomach and combined composition of stomach contents was charted over a theoretical 24-h cycle. The relative proportions of food in each category were calculated for every stomach as IN = (Number of items in food category/Total number of food items) × 100 (Hyslop, 1980; Zacharia and Abdurahiman, 2004). Additional information about the relative relevance of a particular food type in the diet of both species was then determined as a percentage after Hyslop (1980), according to frequency of occurrence, whereby I0 = (Number of fish containing food type/Number of fish with food in stomachs) × 100. A simplified Morisita index of overlap, the Morisita-Horn Index (Horn 1966), was used to quantify dietary overlap between species. The formula for this index was:
where
For sticklebacks, a linear regression model was generated to quantify relationships between the Index of fullness If (Hyslop, 1980) and parasite infection, time of day, sex, length, season, length*sex, and length*parasite infection. A similar regression model was used for perch, but did not account for parasite infection, sex, or associated interactions. An additional logistic regression using a binominal distribution was conducted to model the representation of copepods, Daphnia/Diaphanosoma, Bosmina and predatory zooplankton in separate models which incorporated the explanatory variables length, sex, parasite infection, and time of day. The same model was generated for perch, without the variables parasite infection or sex, which the literature suggests may influence prey choice (Manzer, 1976; Worgan and FitzGerald, 1981; Rask 1986; Tierney, 1994; Sánchez-Gonzáles et al., 2001).
To compare mean stomach fullness and mean prey numbers between sticklebacks and perch at different times of day, t-tests were deployed where distribution was normal and Wilcoxon tests used where the data was not normal. The resulting values were presented as arithmetic mean ± standard deviation (SD). Tests were deemed significant at p < 0.05. For all boxplots, whiskers show the maximum and minimum values, with the exception of outliers (IQR ≥ 1.5). Statistical analyses and descriptive statistics were calculated and plotted using the software JMP Pro 14.64 bit (SAS Institute Inc.), and IBM SPSS Statistics 25.64 bit (IBM Corp.), and Microsoft Word Excel 2016.
Results
Altogether 235 perch and 280 sticklebacks were included in the investigation of both seasons (Table 1). Of the 203 sticklebacks caught in autumn, 45.3% were male and 54.7% female. In spring, of the 77 captured sticklebacks, 36.4% were male and 63.6% female. Female sticklebacks (N = 160) had a mean TL of 5.8 cm (± 1.05 cm SD) while males (N = 120) were significantly smaller at 5.3 cm (± 0.78 cm SD) (t-test, p= < 0.0001). Plerocercoids of the tapeworm Schistocephalus solidus were found in 28 sticklebacks sampled in autumn 2018 (13.8% prevalence) and in two sampled in spring 2019 (2.6% prevalence). The parasite accounted for a mean of 11% (± 8% SD) of the bodyweight of infected fish, up to a maximum of 28%. Mean TL of perch pooled over both seasons was 7.5 cm (± 0.33 cm SD).

TABLE 1. Number and morphometrics of sticklebacks (G. aculeatus) and perch (P. fluviatilis) used for the stomach content analysis per species and season. In total, 280 G. aculeatus and 235 P.fluviatilis from Lake Constance were analyzed for both seasons.
Of the fish captured in autumn 2018 (Table 1), 13 sticklebacks (6.4%), and 27 perch (12.3%) had no food items in their stomachs, while of 77 sticklebacks caught in spring only one had an empty stomach and all 15 spring-caught perch contained prey in their stomachs.
Analysis of stomach contents revealed that both species fed almost exclusively on planktonic crustaceans. In autumn-sampled sticklebacks, copepods accounted for half of all food items identified in the stomachs, and Daphnia/Diaphanosoma for around one third (32.5%) of 92,792 counted food items in total. Sixteen percent of food items were Bosmina and only <0.5% were assigned to the other categories of Predatory zooplankton, Benthic invertebrates (Chironomidae, pupae of Ephemeroptera, or Plecoptera), Airborne insects (e.g. Diptera or Formicidae) or Other food items. In March 2019, 91,532 food items were identified in stomachs of 76 sticklebacks. Copepods were overwhelmingly dominant, making up 99.6% of all food items. Daphnia and Diaphanosoma represented 0.3%, while Bosmina (n = 9), Predatory zooplankton (n = 4), Benthic invertebrates (n = 54), Airborne insects (n = 11), and Other food items (n = 2) occurred only marginally.
In the stomachs of 193 perch caught in autumn, 149,216 food items were identified. Copepods were the most common food items, accounting for 44% of the diet, followed by Bosmina at 32.7% and Daphnia/Diaphanosoma in third place, constituting 22.7% of the diet. Predatory zooplankton accounted only for 0.34% of observed food items. All other types of food were recorded only once each and therefore represented a marginal amount of perch diet. In March 2019, 13,809 food items were identified in the stomachs of 15 perch. As with sticklebacks at this time of year, copepods were overwhelmingly dominant, making up 99.2% of stomach content. Daphnia and Diaphanosoma were the other item occurring in perch stomachs in spring, but these were found rarely and amounted to only 0.8% of stomach contents. Additionally, one airborne insect was found.
In spring, no other food category than copepods made a significant contribution to the gut content of either species, and the diets of stickleback and perch at this time of year were almost identical (Figure 1). Sticklebacks generally consumed a greater number of non-zooplankton food items than perch. All other food categories only made up a very small amount of the total diet in both seasons. Extraordinary findings in stickleback stomachs were a piece of a bird’s feather and a pappus of a floret from a composite-flowered plant (Asteraceae).
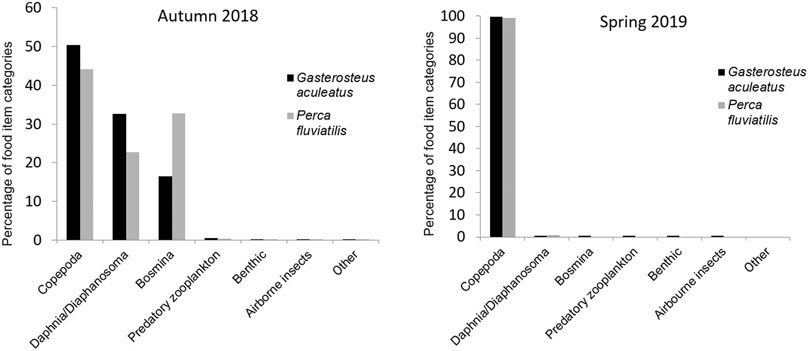
FIGURE 1. Prey composition based on food item numbers in stomachs of investigated stickleback and perch from Lake Constance in autumn 2018 and spring 2019.
The Morisita-Horn index value of 0.95 for autumn data demonstrates a high degree of niche overlap between stickleback and perch. Thereby, it not only exceeded the threshold 0.6 indicating an already relevant food niche overlap (Wallace, 1981; Krebs, 1989), but was even close to 1, demonstrating an essentially almost identical food choice of stickleback and juvenile perch, irrespective of species differences. In autumn, both species supplemented copepods with large numbers of Daphnia and Bosmina, though there was variation in the consumption of the latter two categories between species. Perch consumed more Bosmina, while Daphnia appeared more often in stickleback stomachs.
In perch stomachs sampled in autumn, Bosmina was the most prevalent food item, occurring in 96% of individuals. Copepods and Daphnia were also very common, occurring in 91%, and 92% of stomachs, respectively. Furthermore 52% of autumn-caught perch had also consumed predatory zooplankton, though their overall abundance was very low. Overall, the prevalence of zooplankton was similar in occurrence for both perch and stickleback, except that perch were slightly more likely to have consumed predatory zooplankton, in autumn (Table 2). Benthic, airborne and other foods were found relatively more often and in larger numbers in stomachs of sticklebacks in both seasons. In spring, copepods were present in every stomach that contained food in both species fish, and Daphnia/Diaphanosoma were present in relatively more perch stomachs, where they were the only exception to the otherwise dominant copepods.

TABLE 2. The frequency of occurrence (according to Hyslop, 1980) indicates the percentage of sampled sticklebacks and perch from Lake Constance consuming each type of prey.
A complete 24 h diel cycle analyzed for autumn data (Figure 2) revealed that between 07:00 and 17:00 the number of food items in stickleback stomachs increased continuously, with the exception of the midday 11:00–13:00 time slot, and abundance peaked between 17:00 and 21:00. Thereafter the number of food items in the stomachs decreased continuously, reaching a minimum just before sunrise (Figure 2). Feeding activity of perch showed the same pattern, with a clear peak during dusk and a minimum between 03:00 and 07:00, when most empty perch stomachs were observed. However, the perch data showed less variability than that of sticklebacks and the two species varied significantly in the total number of items consumed by day (Wilcoxon, p = 0.0035) and at night (p = 0.0066). In daylight hours, perch consumed a significantly higher number of food items than sticklebacks, with stomachs containing a mean of 133 and 90 food items, respectively. At night, the opposite was true, with perch stomachs containing a mean 67 food items, and sticklebacks a mean of 82. Overall, stickleback stomachs contained 22.4% more food items during night than perch (Wilcoxon, p < 0.05) in autumn. An examination of standard deviation values shows that number of food items consumed varied strongly between individual fish sampled by day, at dusk, and at night. However, standard deviations were consistently low around dawn, indicating a similar low feeding level across all fishes. There were significant differences between male and female sticklebacks concerning the fullness feeding pattern if considered independently for the different daytimes, during dusk (Wilcoxon, p = <0.01) and night (Wilcoxon, p = <0.05). Male sticklebacks showed higher fullness during both times. In interaction with other feeding affecting factors such as daytime or total length, sex showed no significant influence on fullness for all sticklebacks at all.
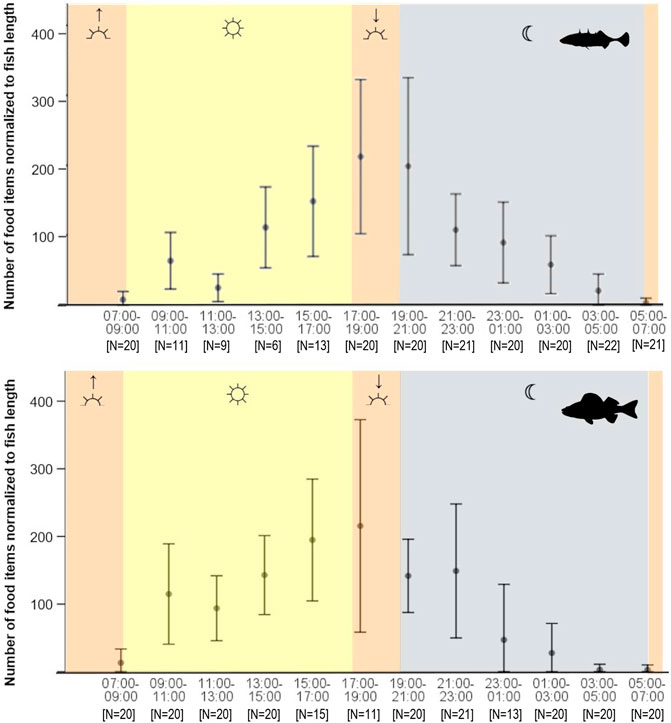
FIGURE 2. Food items consumed by daytime of stickleback (top) and perch (bottom) from Lake Constance in autumn (mean ± SD). The approximate times of twilights (dusk and dawn) are shown as orange areas, nighttime is marked grey and daylight hours yellow.
In autumn the composition of stomach contents varied during a diurnal cycle (Supplementary Material). By day, sticklebacks fed mainly on Daphnia, especially during the period from 09:00 to 11:00, when Daphnia/Diaphanosoma accounted for 95.7% of food items consumed. Around dawn and at night, however, sticklebacks mainly consumed copepods. The consumption of Bosmina by sticklebacks on the other hand is more uniformly distributed, though they were consumed less during late morning and midday. The consumption of predatory zooplankton by sticklebacks was highest around noon. The logistic regression model for individual prey types identified diel patterns in the consumption of copepods and Daphnia by sticklebacks, with time of day shown to be influential on the percentages of both food types in stomach contents. In both fish species stomachs, copepods were recorded significantly more often at night than at dawn or by day, whereas Daphnia were mostly consumed during the daytime until dusk.
Perch feeding also showed a seasonal change in the diel pattern. In autumn, perch took very little food at night but the majority of the small number of food items present in the stomach in the early hours between 03:00 and 05:00 were Bosmina, at 88%. Around dawn, especially from 07:00 to 9:00 (71.6%) and again in the early part of the night, the bulk of the diet comprised copepods. Around sunset and into dusk, the consumption of copepods increased, while cladocerans were consumed less, and all feeding rapidly declined after 23:00. At night, the mean number of Bosmina identified in stomachs was very similar for stickleback and perch (Wilcoxon, p = 0.0009) in autumn. Compared to the other food classes, predatory zooplankton was consumed more often by perch during dusk (Wilcoxon, p = 0.0006) and by stickleback during the night (Wilcoxon, p = 0.0159). The statistical model for perch showed no significant correlation between zooplankton types and the studied variables fish length and daytime.
The estimated degree of fullness data shows a similar pattern to that observed for number of food items (Figure 3). In autumn, both fish species exhibited increasingly full stomachs before noon then a decrease in fullness and prey number around noon. After noon, stomach fullness increased again and remained more or less constant until 23:00. Thereafter, the fullness of perch decreased continuously. There was an overnight decline in fullness for sticklebacks as well, but it was less pronounced and started later into the night. Overall, stickleback stomachs remained fuller than those of perch. There were significant differences in the mean of estimated fullness between sticklebacks (3.57 ± 1.60) and perch (2.40 ± 1.97) during night (Wilcoxon, p < 0.0001). All sticklebacks had less deviating fullness values during dusk and dawn, while during day and night the values were more different. Perch consistently had empty stomachs during the dawn period. The weight of stomach contents showed a very similar pattern between both species, indicating similar feeding activities. (Figure 4). However, after 23:00, the stomachs of perch emptied rapidly, while fullness in sticklebacks declined much more slowly. Index of fullness in spring was only available for stickleback sampled during the night hours, and the evening fullness wain maintained until about 01:00, suggesting that feeding was continuing well into the night. The mean nocturnal weight-based fullness for sticklebacks was significantly higher in autumn than in spring (t-test, p < 0.0001).
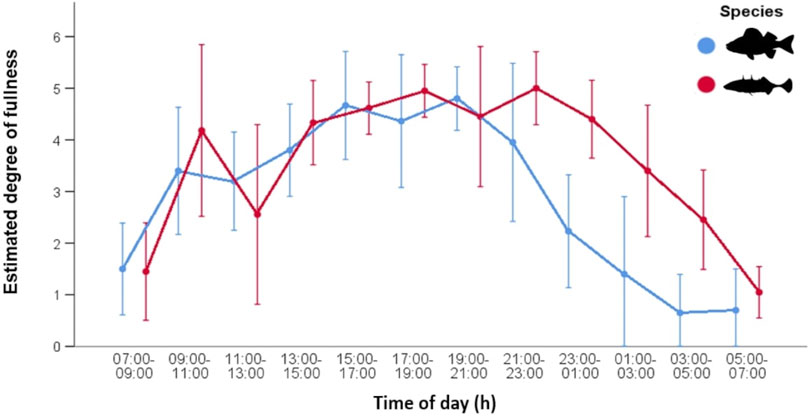
FIGURE 3. Stomach fullness (mean ± SD) of stickleback (N = 203) and perch (N = 220) from Lake Constance during the 24-h cycle in autumn. Values were estimated from 0 (empty) to 6 (distended/stuffed).
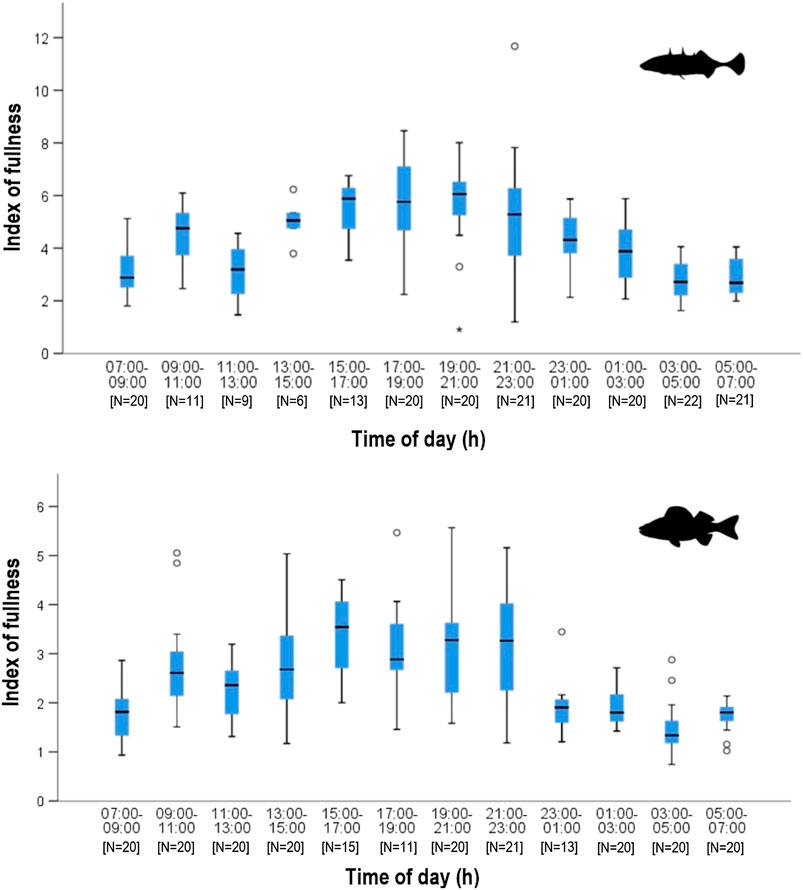
FIGURE 4. Weight-based stomach fullness (Hyslop 1980) during daytime in stickleback (top) and perch (bottom) from Lake Constance in autumn. Higher fullness index corresponds to fuller stomachs.
In sticklebacks, significant associations were recorded between index of fullness and season, total length and time of day, while none of the other tested variables appeared to exert any influence (Table 3). The model indicated that small fish exhibited a higher fullness than large ones. Fullness was significantly reduced at night and during the dawn period (p < 0.006), but rose during the day and at dusk. The model also shows a seasonal effect on index of fullness, whereby stickleback stomachs were fuller in autumn than in spring. In perch, the only significant influence on the index of fullness was time of day, with stomachs appearing more full during daylight hours and around dusk, and least full around dawn (Table 4). There was no apparent influence of season on fullness of perch stomachs.
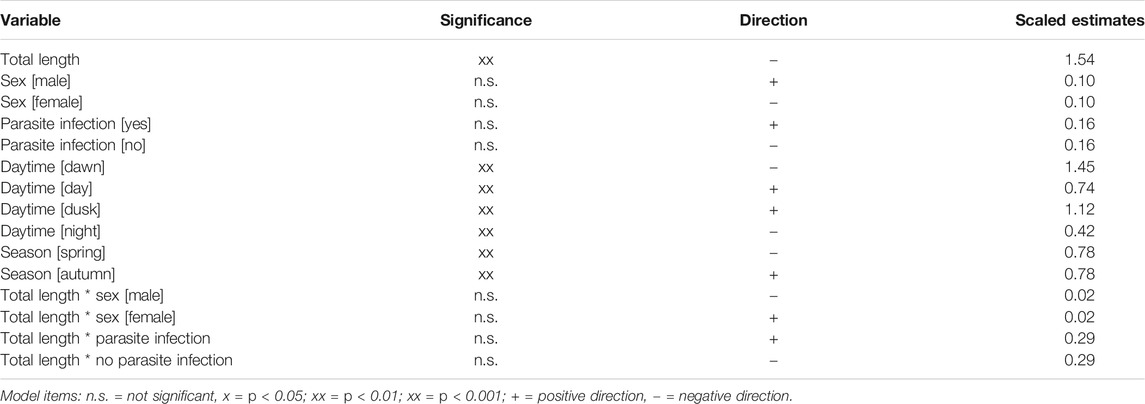
TABLE 3. The significance, scaled estimates and impact direction of parameters and factors on weight-based fullness (Hyslop, 1980) of Gasterosteus aculeatus from Lake Constance in autumn 2018 and spring 2019; N = 280 (GLM; r2adj.: 0.7332; p < 0.0001; f-value = 0.9455; DV = Weight-based fullness).
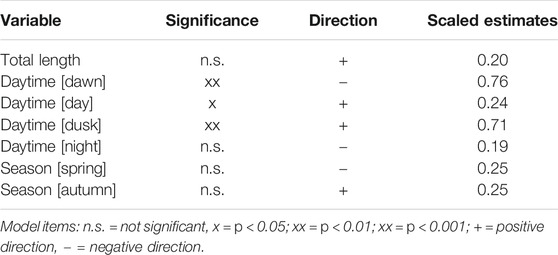
TABLE 4. The significance, scaled estimates and impact direction of parameters and factors on weight-based fullness (Hyslop, 1980) of Perca fluviatilis from Lake Constance in autumn 2018 and spring 2019; N = 235 (GLM; r2adj.: 0.2903; p < 0.0001; f-value = 0.6503; DV = Weight-based fullness).
None of the prey identified in stickleback stomachs in autumn were in perfect condition (i.e. digestive state category 6), but almost all other digestive state categories were recorded in the busy feeding period after sunset (Figure 5). From 09:00 to 11:00 in the morning a little over half of stickleback stomachs contained relatively fresh food (digestive state category 4 and 5). Thereafter food intake declined, with less fresh food apparent in the stomach until early afternoon. Greatest quantities of undigested stickleback food were observed in the afternoon, after which prey items became discontinuously more digested through the night. Between 05:00 and 07:00, stickleback stomachs contained mainly strongly digested food. In perch stomachs, the proportion of fresh food was greatest in the afternoon and around sunset in autumn. After 21:00, fresh-looking food decreased in stomach contents and was increasingly more digested until 03:00, by which time most material was completely digested.
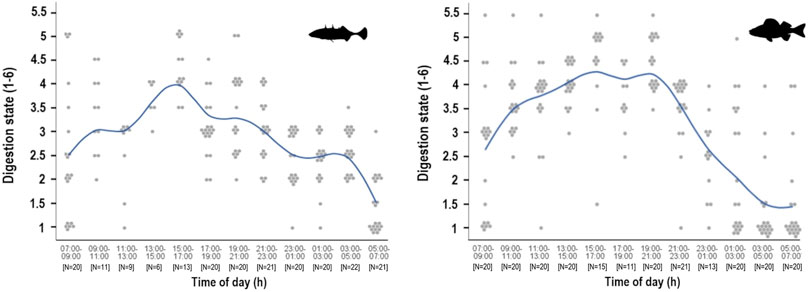
FIGURE 5. Digestion state during the 24-h cycle of stickleback (left) and perch (right) from Lake Constance in autumn 2018. Digestion state of the diet was estimated from 1 (totally digested/stomach empty) to 6 (no apparent digestion). The function line shows the course of the digestion state means, while the points are the actual total digestion classification value of each investigated stomach.
In comparison, the stomachs of sticklebacks continuously showed a higher amount of undigested food after sunset. The contents of stickleback stomachs were also more digested at night, but the decrease in fresh food was less rapid. Perch exhibited slightly greater quantities of less digested food than sticklebacks in daylight hours (Wilcoxon, p = 0.0203) and around dusk (Wilcoxon, p = 0.0096). In spring, the overall digestive state of the stickleback stomach contents indicates continued uptake of fresh food at night until around 01:00.
Discussion
Sticklebacks are not normally known to inhabit the pelagic waters of large deep lakes in Central Europe but are common in eutrophic waters or littoral areas. The data from Lake Constance suggest this species can be a successful forager under these conditions competing with perch for food in this habitat. As evident from the findings of this study, sticklebacks, and perch exhibit broadly similar diel feeding patterns, with peaks during daytime, but sticklebacks are also able to continue feeding well after sunset into the hours of darkness, increasing their feeding time and probably competitive advantage over perch. In addition, both fish consumed almost exactly the same prey, differing only in the relative proportions of different food types. This is underlined by the results of the Morisita-Horn index showing an extremely high degree of niche overlap between species. Diet varied by season, mirroring changes in prey availability (IGKB, 2020), thus indicating a generally high degree of opportunism in both species.
Low densities of zooplankton found in oligotrophic lakes are known to lead to increased competition between 0 + fish as has already been shown for perch and burbot (Lota lota L. 1758) in Lake Constance (Probst and Eckmann, 2009). There is indirect evidence from growth and recruitment point data that strong competition exists between sticklebacks and native whitefish, Coregonus spp. (Roch et al., 2018; Rösch et al., 2018; Gugele et al., 2020). The clear food niche overlap identified in this study points at an additional competition between stickleback and perch, potentially contributing to the creation of a novel food web structure.
It is often mentioned that planktivorous fish prefer cladocerans like Bosmina spp, Daphnia spp., Diaphanosoma spp. or Leptodoridae rather than copepods (Zaret, 1980; Rajasilta and Vuorinen, 1983). Nevertheless, availability is an essential factor in prey choice (Wetterer, 1989) and since copepods were the most abundant prey type in Lake Constance in autumn (LUBW, 2020), both species fed mainly on them. Still, a clear difference is visible in the share of cladocerans in the prey. Possible food niche separation could explain why perch fed more on Bosmina, while sticklebacks preferred Daphnia and Diaphanosoma. The latter are larger, and therefore considered easier to catch while possessing a greater nutritional value (c.f.Bohl, 1982; Wetterer, 1989). In an environment with a high abundance of large prey items, fish show a tendency to size-selective predation, while in areas of lower abundance of large prey, this preference gradually vanishes (Rajasilta and Vuorinen, 1983). The influence of the high abundance of stickleback on the prey composition may drive perch to an increased consumption of smaller Bosmina, since it is known that sticklebacks are highly competitive foragers on Daphnia (Gibson, 1980; Visser, 1982). This dietary foraging competence, while small in magnitude, is therefore a possible explanation that perch are forced to switch to inferior food, as a way of reducing interspecific food competition. However, in-depth analysis of time series data covering several years of zooplankton density and consumption would be necessary to unravel the whole picture. In doing so, further detail could be obtained on the extent to which both species rely on available food resources and respond to scarcity. It should be kept in mind that while perch in other systems have been seen to vary their diet under the influence of newly arrived fish species with similar prey (e.g. Schulze et al., 2012; Semeniuk et al., 2015), relatively little is known about the detailed dietary composition of pelagic 0 + perch in Upper Lake Constance before the appearance of stickleback in the same waters. Nevertheless, given the high numbers of sticklebacks now present in pelagic waters (Eckmann and Enggeser, 2019; Gugele et al., 2020), effects on the performance of perch are to be expected.
Both stickleback and perch are considered opportunistic feeders, in which changes in diet as result of interspecific effects are not extraordinary (Gill and Hard, 1994; Eckmann and Schleuter-Hofmann, 2013; Semeniuk et al., 2015). Additional non-zooplankton food items such as insects were more often taken by sticklebacks and this broader food spectrum suggest enhanced foraging competence compared to the juvenile pelagic perch, which seem to be more strictly limited to zooplankton prey. The results of the Morisita-Horn index, the occurrences, proportions, and numbers of prey types in both species during both seasons confirm our hypothesis of a significant dietary overlap.
Previous studies have reported flexibility in stickleback foraging linked to seasonal changes in the availability or density of prey (Manzer, 1976; Wootton, 2012). This flexibility is in line with the findings of the current study, in which, for example, the diets of both fish varied seasonally according to the availability of different prey (compare LUBW, 2020). This fluctuation in zooplankton availability for pelagic fish in Lake Constance was also described by Probst and Eckmann (2009). In the current results, spring-caught sticklebacks exhibited reduced fullness at night, compared to sticklebacks during night in autumn, most likely as a reflection of the smaller food supply in spring. Contrarily, several other studies have shown mean stomach fullness to be greater in spring compared to autumn (Allen and Wootton, 1984; Worgan and FitzGerald, 1981; Manzer, 1976). In these earlier studies, the spring sampling was conducted later than in the current investigation, coinciding with the breeding season, when energy expenditure is very high (Wootton, 2012). Furthermore, these previous analyses involved sticklebacks caught mostly in shallow benthic habitats, and not in pelagic waters. The early sampling date and small spring sample size in the current study may thus be a factor in the differing result. Furthermore, the size range in spring samples is also narrower, missing sticklebacks under 45 mm and over 70 mm, what may also contribute to the differences. In March, it was only possible to carry out one nighttime sampling trawl and one daytime with gillnet sampling, which had to be aborted because of bad weather conditions. Further sampling was not possible because of time restrictions and the expectation that most sticklebacks would leave the pelagic waters in spring to spawn (Marques et al., 2016). Achieving a more meaningful picture of seasonal changes will require a much larger spring dataset and stomach analysis of further samples gathered over several days in winter and summer.
Stickleback and perch show clear photoperiodic peaks in consumption that are especially evident in autumn data between 9:00 and 11:00. This is consistent with reports in the literature that stickleback shows high feeding activity after sunrise (Manzer, 1976; Worgan and FitzGerald, 1981; Allen and Wootton, 1984). Most feeding occurred during the day and especially around dusk, in line with the findings of Allen and Wootton (1984) and Manzer (1976). In the case of perch, fullness data indicates that the main feeding periods are around dawn and during dusk, and that food intake decreases throughout the night and reaches its lowest level just before dawn, as previously described for this species by Jamet and Lair (1991) and Kratochvíl et al. (2008). Indications of reduced foraging rates were observed for both fish species around noon. This reduction may be related to the observation by Gill (2003) and Kislalioglu and Gibson (1975), that fish become more size-selective as they become more satiated, but further research is necessary if this hypothesis is to be validated.
Stickleback stomachs contained about 20% more food items at night than perch, but achieved almost 50% greater fullness. The difference probably results from the characteristics of Bosmina, which are smaller in size and volume than other food items. However, the data suggest that sticklebacks feed longer in the dusk and nighttime periods, while perch stop feeding more abruptly after dark. Manzer (1976) and Wootton (2012) reference feeding activity peaks in the dawn and dusk periods for sticklebacks, but Manzer (1976) also found relatively fresh food in stomachs from fish taken late at night. The ability of sticklebacks to hunt in total darkness (Mussen and Peeke, 2001) and in turbid conditions suggests they are far from the purely visual predators they are sometimes described as (Beukema 1968; Wootton, 2012). Olfactory and visual cues must play a role in their feeding behavior (Webster et al., 2007). In contrast, perch are considered a predominantly visually oriented fish (Eckmann and Schleuter-Hofmann, 2013) whose activity is limited to twilight and daylight hours (Jamet and Lair, 1991; Imbrock et al., 1996). Diehl (1988) observed low prey capture rates during darkness and Jamet and Lair (1991) observed empty stomachs in the early morning hours, indicating no food intake during the night. However, laboratory studies have reported perch feeding in darkness (Diehl, 1988; Janssen, 1997; Schleuter and Eckmann, 2006), a phenomenon which Schleuter and Eckmann (2006) attributed to artificial conditions that confined predators and prey in a small space. The current study found no evidence of perch feeding during the night. Both digestive state and stomach fullness changed steeply after 21:00. Thus it seems likely that nocturnal feeding could be a distinct advantage for sticklebacks over perch in pelagic waters of Lake Constance, similar to that previously identified for ruffe which also have some ability to feed in darkness (Schleuter and Eckmann, 2006). Allen and Wootton (1984) suggested that moonlight might have an influence on the ability of sticklebacks to feed during night. However, autumn samplings took place over three nights with new moons suggesting that sticklebacks still fed, despite the almost complete lack of moonlight.
For both species, clear diel patterns are apparent in the numbers of prey from different categories. Stickleback stomachs contained mostly large Daphnia in the morning and afternoon, and greatest numbers of copepods after 17:00. Perch fed mainly on Bosmina between 13:00 and 19:00 and switched to copepods around dusk. This may reflect changes in the accessibility of prey items over a 24-h period, e.g. due to vertical migration or patchy distribution (Allen and Wootton, 1984; Rinke et al., 2009). Unlike Worgan and FitzGerald (1981), who reported that female sticklebacks fed almost exclusively in the morning, this study observed no differences in feeding behavior between female and male sticklebacks. Still, significant differences in fullness were apparent on a diel basis between female and male sticklebacks sampled at night and dusk. No fish size-dependent feeding activity was detected. However, the linear regression model showed a negative correlation between fullness and fish length. This may stem from the fact that relative stomach mass tends to be lower for large sticklebacks than small ones (Garrido et al., 2008b). However, the larger surface-to-volume ratio of the stomachs of smaller fish also increases apparent fullness (Jobling, 1981). Another possible factor might be the higher feeding intensity of small fish and possible consequences for digestion rate (Garrido et al., 2008b). It may also be that the differences in fullness observed between male and female fish are a product of size, given that females are significantly larger than males.
The comparison between sticklebacks and perch shows interspecific differences in the total number of Bosmina consumed relative to other prey items. Some caution is necessary here, however, because of the relative durability of hard body parts of Bosmina in the stomachs of fish. Zooplankton groups differ in their digestibility (Gannon, 1976; Sutela and Huusko, 2000) and Sutela and Huusko (2000) identified a high proportion of Bosmina in every quarter of the digestive tract of analyzed fish (about 20% per gut quarter), whereas more soft-bodied food items were only found in the first two or three gut quarters. This might have a bearing on the amount of relatively intact material present during the night, when Bosmina is clearly the most common prey item but intake is low. While it is important to acknowledge this possible bias, the current study sought to minimize it by focusing on foregut (stomach) contents rather than the digestive tract as a whole and a comparison between sticklebacks and perch shows interspecific differences in the number of Bosmina relative to other prey items.
It should be mentioned that two different sampling methods were necessary for securing catches during the 24 h cycle. Both methods may potentially result in differences when it comes to assessing feeding activity. It is known that fish in very clear water can react to an approaching trawl net at a distance of 40 m during daylight, whereas at night in the same water the reaction is only triggered when the net is within 1–2 m (Walsh, 1991). Additionally, it is plausible that perch and stickleback are more evenly distributed in the water at night and more aggregated during the day, and that this may affect relative catch efficiency (Jůza and Kubečka, 2007). Nevertheless, both species were caught during night by trawls and during day by gill nets. Therefore, the influence of both methods for catch and analysis for both species were the same.
Due to the analyses of gut content samples where digestion processes inevitably affect morphometric properties, a finer taxonomic resolution of the prey samples was not possible. However, the counting of food items gives a detailed quantitative result, and provides a proxy for feeding activity even if size differences are not considered. Therefore, due to the comparative approach of this study, a high influence of both taxonomic classification and size differences seems feasible especially when it comes to relative comparisons. The differing digestive anatomy of perch and sticklebacks has implications for weight-based fullness indices and so these values were only compared within species.
Our results are based on both ingestion (i.e. consumption) and digestion and cannot be directly transferred to a measure of actual, total diel food consumption rates. Nevertheless, the results indicate peaks in active feeding. Estimations of fullness are in a way subjective but informative. However, combination of several methods provides a detailed overall result. The combinations all show similar patterns and clarify different aspects of feeding (see Manko, 2016). Altogether, the comparison of the feeding patterns confirms the hypothesis that peak feeding times for both species coincide, regardless of the longer feeding periods of stickleback.
Sticklebacks appeared since 2013 in the pelagic habitat in high numbers. Before that, they almost exclusively lived in the near-shore benthic habitats (Rösch et al., 2018) and did not affect the pelagic-occurring 0 + perch. Even though the exact reasons for this sudden change remains unclear, the example of Lake Constance with the development of a novel pelagic food web within a short time illustrates the importance of understanding interactions of species early on, despite the fact that management of invasive or undesired species remains a challenge once they have become established. Species interactions may also change under changing environmental conditions, which makes a thorough documentation mandatory. In addition to the ecological impacts, sudden changes of food web structures are also linked to ecosystem services related to fisheries and other societal aspects that need to be equally considered. Within a large and complex ecosystem such as Lake Constance the ecological dynamics and interactions between different species and their environment are difficult to qualify. The arrival of an invasive species such as three-spined stickleback is expected to affect the dynamics of other species and many interactions including food web structure. While diet analysis only provides a limited “snapshot” of pelagic fish ecology, it can point to tendencies in species behavior, environmental conditions and the interactions and impacts of a species within an ecosystem. In the case of sticklebacks and juvenile perch in Lake Constance, the results describe a situation with two species targeting almost exactly the same prey, thereby for the potential of competition if resources are scarce. The non-native stickleback seems to be more flexible and competent in exploitation of alternatives to zooplankton and exhibits a longer period of daily feeding activity than 0 + perch. Both species exhibit overlapping peak feeding times around dawn and dusk, and in the afternoon. But sticklebacks apparently are additionally able to feed into the night. The implication is that the flexibility and feeding behavior of sticklebacks render them superior to 0 + perch in the Upper Lake Constance.
In conclusion, our study characterizes the feeding activity and dietary composition of native perch and invasive stickleback in the pelagic waters of Lake Constance, suggesting likely competition between both species as evident from similar prey preferences and food intake peaks, but an extended feeding period of stickleback into the night. The results point to sticklebacks as the more advantaged species, and provide a compelling explanation for their prolonged abundance and dominance in the pelagic zone of Lake Constance over the past 8 years, with significant consequences on the aquatic ecosystem.
Data Availability Statement
The raw data supporting the conclusion of this article will be made available by the authors, without undue reservation.
Ethics Statement
Ethical review and approval was not required for the animal study because According to the German Animal Welfare Act (TierSchG), approval for the present study by a review board institution or ethics committee was not necessary because only dead fish were investigated. All fish were caught by permission of the local fisheries administration (Fisheries administration RP Tübingen). The qualifications of participating personnel (fishing licenses) were checked regularly by the local fisheries administration, too. All perch and sticklebacks were euthanized with an overdose of clove oil (1 ml L−1) according to the German Animal Welfare Act (TierSchG) and the ordinance of slaughter and killing of animals (Tierschutzschlachtverordnung § 13).
Author Contributions
Ideas: AB, JB, JBB, JG, SG. Data Generation: JBB, SG. Data analysis: JBB, AB, JB. Manuscript Preparation: JBB, AB, JG, JB. Funding: AB, JG.
Funding
There was no external funding for this research, except for SG. SG was funded by the Fischereiabgabe of the federal State of Baden-Württemberg. The funder had no role in study design, data collection, and analysis, decision to publish, or preparation of the manuscript.
Conflict of Interest
The authors declare that the research was conducted in the absence of any commercial or financial relationships that could be construed as a potential conflict of interest.
Acknowledgments
The authors would like to thank A. Revermann, M. Bopp, C. Wenzel and M. Schumann for the help on conducting fisheries. We thank Amy-Jane Beer for the editing and text clarifications. Furthermore, we thank T. DeWeber who also supported the study with professional assistance. SG was supported by the Deutsche Forschungsgemeinschaft (DFG, German Research Foundation)- 298726046/GRK2272 and supported by the grant no. ABH060 “SeeWandel: Life in Lake Constance-the past, present and future” within the framework of the Interreg V programme “Alpenrhein- Bodensee-Hochrhein (Germany/Austria/Switzerland/Liechtenstein)” which funds are provided by the European Regional Development Fund as well as the Swiss Confederation and cantons. The funders had no role in study design, data collection and analysis, decision to publish, or preparation of the manuscript.
Supplementary Material
The Supplementary Material for this article can be found online at: https://www.frontiersin.org/articles/10.3389/fenvs.2021.670125/full#supplementary-material
References
AFSC (Alaska Fisheries Science Center) (2015). Resource Ecology and Ecosystem Modeling Stomach Content Analysis Procedures Manual. AFSC, Seattle. Available at: https://www.fisheries.noaa.gov/resource/document/resource-ecology-and-ecosystem-modeling-stomach-content-analysis-procedures (last accessed 20.03.2020).
Alexander, T. J., Vonlanthen, P., Périat, G., Raymond, J. C., Degiorgi, F., and Seehausen, O. (2016). Artenvielfalt und Zusammensetzung der Fischpopulation im Bodensee. Kastanienbaum: Projet Lac, Eawag. Available at: http://www.ibkf.org/wp-content/uploads/2018/03/ProjetLac_Bodensee_2014_fin_web.pdf (last accessed 03 18, 2020)
Allen, J. R. M., and Wootton, R. J. (1984). Temporal Patterns in Diet and Rate of Food Consumption of the Three-Spined Stickleback (Gasterosteus aculeatus L.) in Llyn Frongoch, an upland Welsh lake. Freshw. Biol 14 (4), 335–346.
Barber, I., and Svensson, P. A. (2003). Effects of Experimental Schistocephalus Solidus Infections on Growth, Morphology and Sexual Development of Female Three-Spined Sticklebacks, Gasterosteus aculeatus. Parasitology 126 (4), 359–367. doi:10.1017/s0031182002002925
Benkwitt, C. E., Brodeur, R. D., Hurst, T. P., and Daly, E. A. (2009). Diel Feeding Chronology, Gastric Evacuation, and Daily Food Consumption of Juvenile Chinook Salmon in Oregon Coastal Waters. Trans. Am. Fish. Soc. 138 (1), 111–120. doi:10.1577/t08-060.1
Bergström, U., Olsson, J., Casini, M., Eriksson, B. K., Fredriksson, R., Wennhage, H., et al. (2015). Stickleback Increase in the Baltic Sea - A Thorny Issue for Coastal Predatory Fish. Estuarine, Coastal Shelf Sci. 163, 134–142. doi:10.1016/j.ecss.2015.06.017
Beukema, J. J. (1968). Predation by the Three-Spined Stickleback (Gasterosteus aculeatus L.): the Influence of Hunger and Experience. Behav 31, 1–125. doi:10.1163/156853968x00018
Bohl, E. (1982). Food Supply and Prey Selection in Planktivorous Cyprinidae. Oecologia 53 (1), 134–138. doi:10.1007/bf00377148
Brandner, J., Auerswald, K., Cerwenka, A. F., Schliewen, U. K., and Geist, J. (2013). Comparative Feeding Ecology of Invasive Ponto-Caspian Gobies. Hydrobiologia 703 (1), 113–131. doi:10.1007/s10750-012-1349-9
Craig, J. F. (1978). A Study of the Food and Feeding of Perch, Perca fluviatilis L., in Windermere. Freshw. Biol 8 (1), 59–68. doi:10.1111/j.1365-2427.1978.tb01426.x
Cunha, M. E., Garrido, S., and Pissarra, J. (2005). The Use of Stomach Fullness and Colour Indices to Assess Sardina Pilchardus Feeding. J. Mar. Biol. Ass. 85 (2), 425–431. doi:10.1017/s0025315405011367h
Da Silva, J. C., Gubiani, É. A., Neves, M. P., and Delariva, R. L. (2017). Coexisting Small Fish Species in Lotic Neotropical Environments: Evidence of Trophic Niche Differentiation. Aquat. Ecol. 51 (2), 275–288. doi:10.1007/s10452-017-9616-5
Demchuk, A., Ivanov, M., Ivanova, T., Polyakova, N., Mas-Martí, E., and Lajus, D. (2015). Feeding Patterns in Seagrass Beds of Three-Spined Stickleback Gasterosteus aculeatus Juveniles at Different Growth Stages. J. Mar. Biol. Ass. 95, 1635–1643. doi:10.1017/s0025315415000569
Diehl, S. (1988). Foraging Efficiency of Three Freshwater Fishes: Effects of Structural Complexity and Light. Oikos 53, 207–214. doi:10.2307/3566064
Dußling, U., Baer, J., Gaye-Siessegger, J., Schumann, M., Blank, S., and Brinker, A. (2018). Das große Buch der Fische Baden Württembergs. Stuttgart: Ministerium für ländlichen Raum und Verbraucherschutz Baden-Württemberg, 292–293.
Eckmann, R., and Engesser, B. (2019). Reconstructing the Build-Up of a Pelagic Stickleback (Gasterosteus aculeatus) Population Using Hydroacoustics. Fish. Res. 210, 189–192. doi:10.1016/j.fishres.2018.08.002
Eckmann, R., and Rösch, R. (1998). Lake Constance Fisheries and Fish Ecology. Adv. Limnology 53, 285–301.
Eckmann, R., and Schleuter-Hofmann, D. (2013). Der Flussbarsch. Perca fluviatilis. Wolf, VerlagsKG, p. 13, p. 75-80; 92-98.
Fritts, A. L., and Pearsons, T. N. (2004). Smallmouth Bass Predation on Hatchery and Wild Salmonids in the Yakima River, Washington. Trans. Am. Fish. Soc. 133 (4), 880–895. doi:10.1577/t03-003.1
Gannon, J. E. (1976). The Effects of Differential Digestion Rates of Zooplankton by Alewife, Alosa pseudoharengus, on Determinations of Selective Feeding. Trans. Am. Fish. Soc. 105 (1), 89–95. doi:10.1577/1548-8659(1976)105<89:teoddr>2.0.co;2
Garrido, S., Ben-Hamadou, R., Oliveira, P., Cunha, M., Chícharo, M., and van der Lingen, C. (2008a). Diet and Feeding Intensity of Sardine Sardina Pilchardus: Correlation with Satellite-Derived Chlorophyll Data. Mar. Ecol. Prog. Ser. 354, 245–256. doi:10.3354/meps07201
Garrido, S., Murta, A. G., Moreira, A., Ferreira, M. J., and Angélico, M. M. (2008b). Horse Mackerel (Trachurus trachurus) Stomach Fullness off Portugal: index Calibration and Spatio-Temporal Variations in Feeding Intensity. ICES J. Mar. Sci. 65 (9), 1662–1669. doi:10.1093/icesjms/fsn169
Gibson, R. M. (1980). Optimal Prey-Size Selection by Three-Spined Sticklebacks (Gasterostus Aculeateus). A Test of the Apparent Size Hypothesis. Z. für Tierpsychologie 52, 291–307.
Gill, A. B., and Hart, P. J. B. (1994). Feeding Behaviour and Prey Choice of the Threespine Stickleback: the Interacting Effects of Prey Size, Fish Size and Stomach Fullness. Anim. Behav. 47 (4), 921–932. doi:10.1006/anbe.1994.1124
Gill, A. B. (2003). The Dynamics of Prey Choice in Fish: the Importance of Prey Size and Satiation. J. Fish Biol. 63 (s1), 105–116. doi:10.1111/j.1095-8649.2003.00214.x
Gugele, S. M., Baer, J., and Brinker, A. (2020). The Spatiotemporal Dynamics of Invasive Three-Spined Sticklebacks in a Large, Deep lake and Possible Options for Stock Reduction. Fish. Res. 232, 105746. doi:10.1016/j.fishres.2020.105746
Guma'a, S. A. (1978). The Food and Feeding Habits of Young Perch, Perca fluviatilis, in Windermere. Freshw. Biol 8 (2), 177–187. doi:10.1111/j.1365-2427.1978.tb01439.x
Horn, H. S. (1966). Measurement of "Overlap" in Comparative Ecological Studies. The Am. Naturalist 100 (914), 419–424. doi:10.1086/282436
Huusko, A., Vuorimies, O., and Sutela, T. (1996). Temperature- and Light-Mediated Predation by Perch on Vendace Larvae. J. Fish Biol. 49, 441–457. doi:10.1111/j.1095-8649.1996.tb00040.x
Hyslop, E. J. (1980). Stomach Contents Analysis-A Review of Methods and Their Application. J. Fish. Biol. 17 (4), 411–429. doi:10.1111/j.1095-8649.1980.tb02775.x
IGKB (2020): Jahresbericht der Internationalen Gewässerschutzkommission für den Bodensee: Limnologischer Zustand des Bodensees, Nr. 43 (2018-2019), Available online https://www.igkb.org/fileadmin/user_upload/dokumente/publikationen/gruene_berichte/43_gb43gesamtbericht.pdf (in German).
IGKB (2013): Bodensee-Daten. Edited by IGKB. Available online http://www.igkb.org/der-bodensee/seedaten/, www.igkb.org/massnahmen-und-erfolge/updated on 2016, checked on 10.06.2019.
Imbrock, F., Appenzeller, A., and Eckmann, R. (1996). Diel and Seasonal Distribution of Perch in Lake Constance: a Hydroacoustic Study and In Situ Observations. J. Fish Biol. 49 (1), 1–13. doi:10.1111/j.1095-8649.1996.tb00001.x
Jacobsen, L., Berg, S., Broberg, M., Jepsen, N., and Skov, C. (2002). Activity and Food Choice of Piscivorous Perch (Perca fluviatilis ) in a Eutrophic Shallow lake: a Radio-Telemetry Study. Freshw. Biol. 47 (12), 2370–2379. doi:10.1046/j.1365-2427.2002.01005.x
Jamet, J. L., and Lair, N. (1991). An Example of Diel Feeding Cycle of Two Percids, Perch (Perca fluviatilis) and Ruffe (Gymnocephalus Cernuus) in Eutrophic Lake Aydat (France). In Ann. des Sci. Nat. Zoologie biologie animale (Vol. 12, No. 3, pp. 99–105). Elsevier.
Janssen, J. (1997). Comparison of Response Distance to Prey via the Lateral Line in the Ruffe and Yellow Perch. J. Fish Biol. 51 (5), 921–930. doi:10.1111/j.1095-8649.1997.tb01531.x
Jobling, M. (1981). Mathematical Models of Gastric Emptying and the Estimation of Daily Rates of Food Consumption for Fish. J. Fish. Biol. 19 (3), 245–257. doi:10.1111/j.1095-8649.1981.tb05829.x
Jůza, T., and Kubečka, J. (2007). The Efficiency of Three Fry Trawls for Sampling the Freshwater Pelagic Fry Community. Fish. Res. 85 (3), 285–290.
Kahl, U., and Radke, R. J. (2006). Habitat and Food Resource Use of Perch and Roach in a Deep Mesotrophic Reservoir: Enough Space to Avoid Competition? Ecol. Freshw. Fish 15 (1), 48–56. doi:10.1111/j.1600-0633.2005.00120.x
Kislalioglu, M., and Gibson, R. N. (1975). Field and Laboratory Observations on Prey-Size Selection in Spinachia Spinachia (L.) In Proc 9th Europ Mar Biol Symp H Barnes. Aberdeen University Press, 29–41.
Kratochvíl, M., Peterka, J., Kubecka, J., Matena, J., Vasek, M., Vanícková, I., and Seda, J. (2008). Diet of Larvae and Juvenile Perch, Perca fluviatilis Performing Diel Vertical Migrations in a Deep Reservoir. Folia Zoologica 57 (3), 313.
Krebs, C. J. (1989). Ecological Methodology (No. QH541. 15. S72. K74 1999.). New York: Harper & Row, 466–472.
Lang, U., Schick, R., and Schröder, G. (2010). The Decision Support System Bodensee Online for Hydrodynamics and Water Quality in lake Constance. Decis. support Syst. Adv., 81–98.
López-Olmeda, J. F., and Sánchez-Vázquez, F. J. (2010). “Feeding Rhythms in Fish: from Behavioral to Molecular Approach.” in Biological Clock in Fish. Enfield: Science Publishers, 155–184.
LUBW (Landesanstalt für Umwelt Baden-Württemberg), (2020): ISF Arbeitsbericht 2018; Landesanstalt für Umwelt Baden-Württemberg 76231 Karlsruhe, Postfach 100163; pp.35.
Manko, P. (2016). Stomach Content Analysis in Freshwater Fish Feeding Ecology, 116. Prešov, Slovakia: University of Prešov.
Manzer, J. I. (1976). Distribution, Food, and Feeding of the Threespine Stickleback, Gasterosteus aculeatus, in Great Central Lake, Vancouver Island, with Comments on Competition for Food with Juvenile Sockeye salmon, Oncorhynchus nerka. Fishery Bulletin, U.S. Natl. Mar. Fish. Serv. 74, 647–668.
Marques, D. A., Lucek, K., Meier, J. I., Mwaiko, S., Wagner, C. E., Excoffier, L., et al. (2016). Genomics of Rapid Incipient Speciation in Sympatric Threespine Stickleback. PLoS Genet. 12 (2), e1005887. doi:10.1371/journal.pgen.1005887
Mussen, T., and Peeke, H. (2001). Nocturnal Feeding in the marine Threespine Stickleback (Gasterosteus aculeatus L.): Modulation by Chemical Stimulation. Behav 138 (7), 857–871. doi:10.1163/156853901753172683
Nordeide, J. T. (2020). Accuracy of Body Mass Estimates of Formalin‐preserved Fish - a Review. J. Fish. Biol. 96 (2), 288–296. doi:10.1111/jfb.14146
Nurminen, L., Pekcan-Hekim, Z., and Horppila, J. (2010). Feeding Efficiency of Planktivorous Perch Perca fluviatilis and Roach Rutilus rutilus in Varying Turbidity: an Individual-Based Approach. J. Fish Biol. 76 (7), 1848–1855. doi:10.1111/j.1095-8649.2010.02600.x
Paepke, H.-J. (1996). Die Stichlinge: Gasterosteidae (Die Neue Brehm-Bücherei) (2., Überarb. Aufl.). Westarp Wissenschaften: Magdeburg, 207.
Peltonen, H., Vinni, M., Lappalainen, A., and Pönni, J. (2004). Spatial Feeding Patterns of Herring (Clupea Harengus L.), Sprat (Sprattus Sprattus L.), and the Three-Spined Stickleback (Gasterosteus aculeatus L.) in the Gulf of Finland, Baltic Sea. ICES J. Mar. Sci. 61 (6), 966–971. doi:10.1016/j.icesjms.2004.06.008
Persson, L., and Greenberg, L. A. (1990). Juvenile Competitive Bottlenecks: The Perch (Perca Fluviatilis)-Roach (Rutilus Rutilus) Interaction. Ecology 71 (1), 44–56. doi:10.2307/1940246
Probst, W. N., and Eckmann, R. (2009). Diet Overlap between Young-Of-The-Year Perch,Perca fluviatilisL., and burbot,Lota lota(L.), during Early Life-History Stages. Ecol. Freshw. Fish 18 (4), 527–536. doi:10.1111/j.1600-0633.2009.00367.x
Quirino, B. A., Carniatto, N., Gaiotto, J. V., and Fugi, R. (2015). Seasonal Variation in the Use of Food Resources by Small Fishes Inhabiting the Littoral Zone in a Neotropical Floodplain lake. Aquat. Ecol. 49, 431–440. doi:10.1007/s10452-015-9535-2
Rajasilta, M., and Vuorinen, I. (1983). A Field Study of Prey Selection in Planktivorous Fish Larvae. Oecologia 59 (1), 65–68. doi:10.1007/bf00388074
Rask, M. (1986). “The Diet and Diel Feeding Activity of Perch, Perca fluviatilis L., in a Small lake in Southern Finland,” in Annales Zoologici FenniciFinnish Academy of Sciences, Societas Scientiarum Fennica, Societas pro Fauna et Flora Fennica and Societas Biologica Fennica Vanamo, 49–56.
Rinke, K., Huber, A. M. R., Kempke, S., Eder, M., Wolf, T., Probst, W. N., et al. (2009). Lake-wide Distributions of Temperature, Phytoplankton, Zooplankton, and Fish in the Pelagic Zone of a Large lake. Limnol. Oceanogr. 54 (4), 1306–1322. doi:10.4319/lo.2009.54.4.1306
Roch, S., von Ammon, L., Geist, J., and Brinker, A. (2018). Foraging Habits of Invasive Three-Spined Sticklebacks (Gasterosteus aculeatus) - Impacts on Fisheries Yield in Upper Lake Constance. Fish. Res. 204, 172–180. doi:10.1016/j.fishres.2018.02.014
Rösch, R., Baer, J., and Brinker, A. (2018). Impact of the Invasive Three-Spined Stickleback (Gasterosteus aculeatus) on Relative Abundance and Growth of Native Pelagic whitefish (Coregonus Wartmanni) in Upper Lake Constance. Hydrobiologia 824 (1), 243–254. doi:10.1007/s10750-017-3479-6
Sánchez‐Gonzáles, S., Ruiz‐Campos, G., and Contreras‐Balderas, S. (2001). Feeding Ecology and Habitat of the Threespine Stickleback, Gasterosteus aculeatus Microcephalus, in a Remnant Population of Northwestern Baja California, Mexico. Ecol. Freshw. Fish 10 (4), 191–197.
Schleuter, D., and Eckmann, R. (2006). Competition between Perch (Perca fluviatilis) and Ruffe (Gymnocephalus Cernuus): the Advantage of Turning Night into Day. Freshw. Biol 51 (2), 287–297. doi:10.1111/j.1365-2427.2005.01495.x
Schleuter, D., and Eckmann, R. (2008). Generalist versus Specialist: the Performances of Perch and Ruffe in a lake of Low Productivity. Ecol. Freshw. Fish 17 (1), 86–99. doi:10.1111/j.1600-0633.2007.00262.x
Schulze, T., Dörner, H., Baade, U., and Hölker, F. (2012). Dietary Niche Partitioning in a Piscivorous Fish Guild in Response to Stocking of an Additional Competitor - the Role of Diet Specialisation. Limnologica 42 (1), 56–64. doi:10.1016/j.limno.2011.08.001
Semeniuk, C. A. D., Magnhagen, C., and Pyle, G. (2015): Behaviour of Perch; in P. Couture, and G. Pyle (Eds.): Biology of Perch, Capture 9. Taylor and Francis Group. p.230–270.
Sutela, T., and Huusko, A. (2000). Varying Resistance of Zooplankton Prey to Digestion: Implications for Quantifying Larval Fish Diets. Trans. Am. Fish. Soc. 129 (2), 545–551. doi:10.1577/1548-8659(2000)129<0545:vrozpt>2.0.co;2
Tierney, J. F. (1994). Effects of Schistocephalus Solidus (Cestoda) on the Food Intake and Diet of the Three-Spined Stickleback, Gasterosteus aculeatus. J. Fish Biol. 44, 731–735. doi:10.1111/j.1095-8649.1994.tb01249.x
Visser, M. (1982). Prey Selection by the Three-Spined Stickleback (Gasterosteus aculeatus L.). Oecologia 55 (3), 395–402. doi:10.1007/bf00376928
Wallace, R. K. (1981). An Assessment of Diet-Overlap Indexes. Trans. Am. Fish. Soc. 110 (1), 72–76. doi:10.1577/1548-8659(1981)110<72:aaodi>2.0.co;2
Walsh, S. J. (1991). Diel Variation in Availability and Vulnerability of Fish to a Survey Trawl. J. Appl. Ichthyol. 7, 147–159. doi:10.1111/j.1439-0426.1991.tb00521.x
Wang, N., and Appenzeller, A. (1998). Abundance, Depth Distribution, Diet Composition and Growth of Perch (Perca fluviatilis) and Burbot (Lota Lota) Larvae and Juveniles in the Pelagic Zone of Lake Constance. Ecol. Freshw. Fish 7 (4), 176–183. doi:10.1111/j.1600-0633.1998.tb00184.x
Wang, N., and Eckmann, R. (1994). Distribution of Perch (Perca fluviatilis L.) during Their First Year of Life in Lake Constance. Hydrobiologia 277 (3), 135–143. doi:10.1007/bf00007295
Ward, A. J. W., Webster, M. M., and Hart, P. J. B. (2006). Intraspecific Food Competition in Fishes. Fish Fish. 7, 231–261. doi:10.1111/j.1467-2979.2006.00224.x
Webster, M. M., Atton, N., Ward, A. J. W., and Hart, P. J. B. (2007). Turbidity and Foraging Rate in Threespine Sticklebacks: the Importance of Visual and Chemical Prey Cues. Behaviour 144 (11), 1347–1360.
Werner, S., Mortl, M., Bauer, H.-G., and Rothhaupt, K.-O. (2005). Strong Impact of Wintering Waterbirds on Zebra Mussel (Dreissena polymorpha) Populations at Lake Constance, Germany. Freshw. Biol 50 (8), 1412–1426. doi:10.1111/j.1365-2427.2005.01411.x
Wetterer, J. K. (1989). Mechanisms of Prey Choice by Planktivorous Fish: Perceptual Constraints and Rules of Thumb. Anim. Behav. 37, 955–967. doi:10.1016/0003-3472(89)90140-1
Wootton, R. J. (2012). A Functional Biology of Sticklebacks. Springer Science & Business Media, 32–62.
Worgan, J. P., and FitzGerald, G. J. (1981). Diel Activity and Diet of Three Sympatric Sticklebacks in Tidal Salt Marsh Pools. Can. J. Zool. 59 (12), 2375–2379. doi:10.1139/z81-317
Zacharia, P. U., and Abdurahiman, K. P. (2004). Methods of Stomach Content Analysis of Fishes. Winter Sch. Towards Ecosystem Based Manage. Mar. Fisheries—Building Mass Balance Trophic Simulation Models 1, 148–158.
Keywords: diel feeding pattern, three-spined stickleback, Eurasian perch, Lake Constance, prey composition, fish community change, feeding ecology
Citation: Bretzel JB, Geist J, Gugele SM, Baer J and Brinker A (2021) Feeding Ecology of Invasive Three-Spined Stickleback (Gasterosteus aculeatus) in Relation to Native Juvenile Eurasian Perch (Perca fluviatilis) in the Pelagic Zone of Upper Lake Constance. Front. Environ. Sci. 9:670125. doi: 10.3389/fenvs.2021.670125
Received: 20 February 2021; Accepted: 23 June 2021;
Published: 06 July 2021.
Edited by:
Ross Thompson, University of Canberra, AustraliaReviewed by:
Norman Mercado-Silva, Universidad Autónoma del Estado de Morelos, MexicoJeyaraj Antony Johnson, Wildlife Institute of India, India
Ian Duggan, The University of Waikato, New Zealand
Copyright © 2021 Bretzel, Geist, Gugele, Baer and Brinker. This is an open-access article distributed under the terms of the Creative Commons Attribution License (CC BY). The use, distribution or reproduction in other forums is permitted, provided the original author(s) and the copyright owner(s) are credited and that the original publication in this journal is cited, in accordance with accepted academic practice. No use, distribution or reproduction is permitted which does not comply with these terms.
*Correspondence: Joachim Benedikt Bretzel, amJyZXR6ZWxAY3N1LmVkdS5hdQ==