- 1Faculty of Biological and Environmental Sciences, Ecosystems and Environment Research Programme, University of Helsinki, Helsinki, Finland
- 2Municipality of Kaustinen, Kaustinen, Finland
Views on the entry of organic pollutants into the organic matter (OM) decaying process are divergent, and in part poorly understood. To clarify these interactions, pesticide dissipation was monitored in organic and mineral soils not adapted to contaminants for 241 days; in groundwater sediment slurries adapted to pesticides for 399 days; and in their sterilized counterparts with and without peat (5%) or compost-peat-sand (CPS, 15%) mixture addition. The results showed that simazine, atrazine and terbuthylazine (not sediment slurries) were chemically dissipated in the organic soil, and peat or CPS-amended soils and sediment slurries, but not in the mineral soil or sediment slurries. Hexazinone was chemically dissipated best in the peat amended mineral soil and sediment slurries. In contrast, dichlobenil chemically dissipated in the mineral soil and sediment slurries. The dissipation product 2,6-dichlorobenzamide (BAM) concentrations were lowest in the mineral soil, while dissipation was generally poor regardless of plant-derived OM, only algal agar enhanced its chemical dissipation. Based on sterilized counterparts, only terbutryn appeared to be microbially degraded in the organic soil, i.e., chemical dissipation of pesticides would appear to be utmost important, and could be the first response in the natural cleansing capacity of the environment, during which microbial degradation evolves. Consistent with compound-specific dissipation in the mineral or organic environments, long-term concentrations of pentachloroaniline and hexachlorobenzene were lowest in the mineral-rich soils, while concentrations of dichlorodiphenyltrichloroethane (DTT) and metabolites were lowest in the organic soils of old market gardens. OM amendments changed pesticide dissipation in the mineral soil towards that observed in the organic soil; that is OM accelerated, slowed down or stopped dissipation.
Introduction
Soil organic matter (OM) formation and litter decomposition have initially been thought to be separate processes in so called “humification”. Later on, the low molecular weight biodegradation products of plant and microbial biopolymers have been assumed to condense abiotically into humic polymers (Cotrufo et al., 2013). After the discovery that soil OM consists of mixtures of microbial and, to lesser extent, plant constituents and their derivatives at different stages of degradation, the super constructions they form were thought to be stabilized by e.g., hydrogen and hydrophobic bonds, occlusion, intercalation, and encapsulation (Sollins et al., 1996; Burdon, 2001; Lützow et al., 2006; Cotrufo et al., 2013). Then more sophisticated models were developed that emphasized the role of microbes in OM decomposition (Lehmann and Kleber, 2015; Kallenbach et al., 2016; Vermeire et al., 2018; Overy et al., 2021).
The selective preservation model states that microorganisms in soil first utilize readily metabolized compounds as nutrients, and then more recalcitrant OM is consumed as microbial carbon and energy sources, resulting in an increase in average recalcitrance (Sollins et al., 1996; Lützow et al., 2006). The subsequent progressive decomposition model emphases that soil OM consists of plant and microbial substances of all sizes at different decomposition stages (Cotrufo et al., 2013; Lehmann and Kleber, 2015). Abiotic dissipation pathways have been regarded as minor in selective preservation and progressive decomposition models (Burdon, 2001; Lützow et al., 2006), while OM as a nutrient in soil has been thought to increase microbial activity and thereby the microbial degradation of harmful persistent compounds (Burauel and Baßmann, 2005; Krutz et al., 2010; Fenner et al., 2013). Finally, the soil continuum model (SCM) states that OM is a continuum of progressively decomposing organic substances in biological, physical and chemical processes, which include biotic and abiotic degradation, adsorption, desorption, and exchange reactions between OM competing compounds (Lehmann and Kleber, 2015). Chemical and physical mechanisms are regarded to stabilize soil OM e.g., via organo-mineral bonds (Lehmann and Kleber, 2015; Totsche et al., 2018; Baveye and Wander, 2019; Lavallee et al., 2020).
Organic matter in soil adsorbs pollutants, and prevents their bioavailability and drainage to water bodies and groundwater, where they can be persistent (Lal, 2004; Lapworth et al., 2012; Fenner et al., 2013; James et al., 2019; Barchańska et al., 2020). The views of the nature of soil OM and pollutant transformations, and interactions with the mineral matrix are widely divergent (Lehmann and Kleber, 2015). Among the challenging issues in pollutant transformation is the importance of abiotic and biotic degradation processes. For example, triazines were initially thought to dissipate in soil by chemical hydrolysis. Since the discovery of degrader microbes, biodegradation has been considered as the major degradation pathway (Krutz et al., 2010). Experiments to reveal mechanisms for the entry of persistent organic pollutants into the OM decomposition processes should be designed so that mechanisms operating simultaneously do not interfere with each other (Sollins et al., 1996; Lützow et al., 2006). The potential contribution of organic matter in enhancing the chemical dissipation of pollutants has been almost completely ignored, while the focus has been on adsorption and enhancing microbial degradation (James et al., 2019; Barchańska et al., 2020).
To assess the interactions between OM and pollutants, low OM mineral and rich OM organic soils not adapted to pesticides, low OM groundwater sediment slurries adapted to pesticides, and similar autoclave-sterilized counterparts were amended peat or compost-peat-sand mixture (CPS) (Kerminen et al., 2018). Dissipation of the pesticides simazine, atrazine, terbutylazine, hexazinone, terbutryn, dichlobenil and its degradation product 2,6-dichlorobenzamide (BAM) was recorded after 241–399 days. The results were compared to the dissipation in old greenhouse soils, and to the ability of algae product, agar to enhance herbicide dissipation. The hypotheses of the work were that part of the pesticides are resistant to dissipation in the presence of OM, while others are dissipated, the null hypothesis being that OM does not affect xenobiotic dissipation.
Materials and Methods
Soils, Sediments and Chemical Analyses
Organic and mineral topsoils (depth 0–10 cm, three separate samples of the same size were pooled) were collected in a garden not adapted to pesticides (Tuusula, Finland; 60°40′49″ N/25°07′49″ E). Organic soil was fertilized with composted source-separated biowaste consisting mainly of food waste and to a lesser extent garden waste. The particle size of the sandy mineral soil was about 1 mm in maximum. The sediments from a depth of 11–15 m were transferred to sterilized plastic bags during drilling next to the railway station in Lahti (Finland; 60°97′66″ N/25°65′03″ E) in a groundwater area with atrazine (0.2 µg/15 g dry weight), simazine (0.6 µg/15 g), and BAM (1.1µg/15 g) in the water and sediments (Kerminen et al., 2018). Old market garden soils (depths 0–0.3 m) from demolished greenhouse areas in Kokkola, Alahärmä, and Kuortane (Finland) were collected in sealed plastic bags in July-August 2006 using a multi-purpose drill (GM-25, GM-75, Auger). The gardening activities were continued for 12–50 years until they ended in 1978–91 (Närhi, 2008).
To determine the dry weight, 3 g of soil or sediment was heated at 105°C for 16 h, followed by ignition at 550°C for 4 h to determine OM (Kerminen et al., 2018). The water holding capacity was weighed after stabilizing 10 g of wetted soil in a funnel for 24 h. To measure pH, 3 g of soil and water (1:5, weight vol−1) were shaken for 1 hour, followed by centrifugation (3,000 × g, 10 min, Biofuge primo R, Heraeus, Hanau, Germany), and the pH analysis (ION85 Ion Analyzer, Radiometer, Copenhagen, Danmark). To determine total carbon (total-C) and nitrogen (total-N), soils and sediments were analyzed using LECO Model 2000 CNS analyzer (St. Joseph, MI, United States) using helium as a carrier gas according to the manufacturer’s instructions (LECO Corporation, 2019). All analyses were done in triplicate.
Degradation Experiments
Peat (5%), or compost-peat-sand (CPS, 15%) mixture (Mustamulta, Biolan, Kauttua, Finland) was added to the soils (15.0 g dry weight) in a 100 ml flask with a hole in the cap covered with aluminum foil (Pukkila and Kontro, 2014; Kerminen et al., 2018). Soils and additives for sterilized counterparts were autoclaved for 1 h (121°C, 101 kPa, Instru, Santasalo-Sohlberg, Helsinki, Finland) on three successive days. Pesticides (simazine, atrazine, terbuthylazine, hexazinone, terbutryn, dichlobenil) and degradation product (2,6-dichlorobenzamide, BAM) were added (67 µg g−1 of soil in methanol: water, 3 : 1 vol vol−1; total methanol volume 2.3 mL; LGC Standards GmbH, Germany), and the flasks were shaken (120 rpm; Laboshake, Gerhardt, Königswinter, Germany) at 21 ± 2 °C in the dark for 241 days. Soil moisture was checked during six samplings, and kept at 70% of the water holding capacity by weighting the flasks regularly, and replacing the evaporated water.
Sediments (15 g dry weight) without or with peat (5%) or CPS (15%) in the above-presented flasks and similarly sterilized counterparts were amended with 50 mL of sterilized distilled water (Kerminen et al., 2018). The pesticides simazine (15 mg L−1; 50 µg g−1), atrazine (15 mg L−1; 50 µg g−1), hexazinone (25 mg L−1; 83 µg g−1), dichlobenil (20 mg L−1; 67 µg g−1), and BAM (20 mg L−1; 67 µg g−1) in methanol: water (3:1 vol vol−1; total methanol volume 1.7 mL) were added, and the flasks were shaken as above in the dark under aerobic and anaerobic (jar with Anaerocult® A, Millipore, Burlington, MA, United States) conditions for 399 days, during which time evaporated water was regularly replaced with sterilized distilled water.
To study agar effects on atrazine (28 mg L−1), hexazinone (30 mg L−1), terbutryn (29 mg L−1), and BAM (28 mg L−1) dissipation, 1.5% agar in 330 ml of water was autoclave-sterilized for 20 min at 121°C. After cooling to 50°C, pesticides were added in 3.8 ml of methanol: water (3 : 1 vol vol−1; 86 µL of methanol in 10 ml). After careful mixing, 10 ml of solution was divided to 20 ml test tubes, and the tubes were incubated at 21 ± 2°C for 33 days. All degradation experiments were done in triplicate.
Pesticide Analyses
Soils (100 mg), the internal standard propazine (72 µg in 360 µL of methanol) and 1.5 ml of methanol: water (3:1 vol vol−1) were sonicated (40 kHz, 320 W; Branson 8,510 ultrasonic bath, W.A. Brown Industrial Sales Inc., Richmond, VA, United States) for 15 min at 20°C (Pukkila and Kontro, 2014), and then extracted by shaking (200 rpm) overnight at 21 ± 2°C. The extract was separated by centrifugation (1,250 × g, 10 min), and stored at -20°C. The extraction was repeated two more times and the extracts were combined. Groundwater sediment slurries (200 µL), the internal standard propazine (9.96 µg in 100 µL of methanol) and methanol: water (3:1, vol vol−1) were mixed (Kerminen et al., 2018). Standard concentrations in methanol: water (3:1 vol vol−1) were 0.1, 0.5, 5.0, 10.0, 15.0 and 30.0 µg mL−1, and 16.7 µg mL−1 of propazine was added as an internal standard. All samples were filtrated through a 45 μm GPH membrane (Acrodisc®, Gelman, Pall Corporation Ltd., Port Washington, NY, United States), and 20 µL was analysed by high performance liquid chromatography (HPLC) equipped with Shimadzu Prominence (Shimadzu, Kyoto, Japan) SIL-10A auto sampler; LC-20AT solvent delivery module; DGU-20A5 on-line degasser; SPD-20A UV/VIS detector (wavelength 215 nm for dichlobenil, BAM, and propazine; and 225 nm for simazine, atrazine, desethylatrazine (DEA), deisopropylatrazine (DIA), terbutylazine, hexazinone, terbutryn, and propazine); SunFire column (C18, 3.5 μm, 3.0 × 150 mm, Waters, MA, United States), and the flow rate of 0.4 mL min−1 was controlled by a Shimadzu LC Solution software. Acetonitrile and filtered ultrapure water mobile phase changed as follows: Acetonitrile concentration increased from 35 to 55% in 3.5 min; held at 55% for 7 min; increased to 100% in 7.5 min; held at 100% for 7 min, and finally declined back to 35% in 5 min. The limit of detection was about 0.05 µg L−1, and the limit of quantification was about 0.1 µg mL−1. The quantification accuracy, i.e., the mean of measured concentrations/actual value ×100%, ranged from 93 to 103%, and the quantification precision, i.e., the coefficient of variation ×100%, ranged from 1.5 to 13.0%, both were measured at concentrations of 5.0, 10.0, 15.0 and 30.0 mg L−1 (n = 6).
To extract pesticides from 10 mL of 1.5% agar, the agar was broken to small particles using spatula, and 20 µg of propazine in 40 µL of methanol was added as an internal standard. Pesticides were extracted with 40 mL of methanol: water (3:1, vol vol−1) overnight at 21 ± 2°C after sonication at 20°C for 15 min (43 kHz, RF-power 320 W; W.A. Brown Industrial Sales Inc., Richmond, VA, United States). The sample was centrifuged (2,500 × g, 10 min, Multifuge® Heraeus, DJB Labcare, Buckinghamshire, United Kingdom), and the supernatant was stored at -20°C. The extraction was repeated two more times. The pooled supernatants were evaporated under a nitrogen flow, and the residue was solubilized in acetonitrile for the analysis by gas chromatography-mass spectrometry (GC-MS) as presented (Kerminen et al., 2017, 2018). The limit of detection in the sample was about 2 ng, and the limit of quantification in the sample was about 0.01 µg, the quantification accuracy varied between 95 and 109%, and the quantification precision varied between 1.2 and 10.8%, (measured at concentrations of 5.0, 10.0 and 15.0 mg L−1).
Pesticides [1,1,1-trichloro-2,2-bis(4-chlorophenyl)ethane (4,4′-DDT); 1,1,1-trichloro-2-(2-chlorophenyl)-2-(4-chlorophenyl)- ethane (2,4′-DDT); 1,1-Dichloro-2,2-bis(4-chlorophenyl)ethane (4,4′-DDD); 1,1-Dichloro-2,2-bis(4-chlorophenyl)ethene (4,4′-DDE); pentachloroaniline (PCA), hexachlorobenzene (HCB)] from old market garden soils were analysed as purchased services at Eurofins Scientific Finland Ltd. (Finland). All other methods were performed according to the good laboratory practices of the University of Helsinki Environmental laboratory (Lahti, Finland).
Calculations
Pesticide and metabolite DEA, DIA and BAM concentrations were calculated as percentages of initial concentrations on a dry weight basis under stabilized concentrations, for soils as averages (±S.D.) from the results of days 178 and 241, and for sediments as averages (±S.D.) of the results on days 287, 360, and 399. In some cases, more sampling points from stabilized conditions were included to increase the number of replicates and ensure reliable results. Three-factor (additive-soil-sterilization for soils, and additive, oxygen, sterilization for sediments) Kruskal-Wallis test (KW) followed by Mann-Whitney’s test (MW) were used to elucidate statistically significant differences in pesticide and metabolite dissipations (SPSS Statistics for Windows, Version 22.0. IBM Corporation, Chicago, IL, United States).
Results and Discussion
To investigate the relationship between OM and organic pollutant dissipation, pesticides were used as model compounds in a three-factor (soil, organic additive, sterilization) experiment in low OM mineral soil (OM, 29 ± 4 mg g−1), rich OM organic soil (OM, 123 ± 3 mg g−1), and in similar autoclave-sterilized counterparts in the dark (Table 1). The soils were not adapted to pesticides. According to the statistical analysis, the effects of additives on pesticide concentrations differed in the mineral and organic soils (KW, interaction between soils and additives, p ≤ 0.050) (Figures 1, 2). The long-term relationship between OM and pesticide dissipation was further investigated in a three-factor (organic additive, oxygen, sterilization) experiment in low OM (4.3 ± 0.2 mg g−1) groundwater sediment slurries and their sterilized counterparts in aerobic and anaerobic conditions (Table 1; Kerminen et al., 2018). Sediments were adapted to pesticides. According to the statistical analysis, the additives affected pesticides concentrations, but not oxygen or sterilization (KW, p < 0.050) (Figure 3).
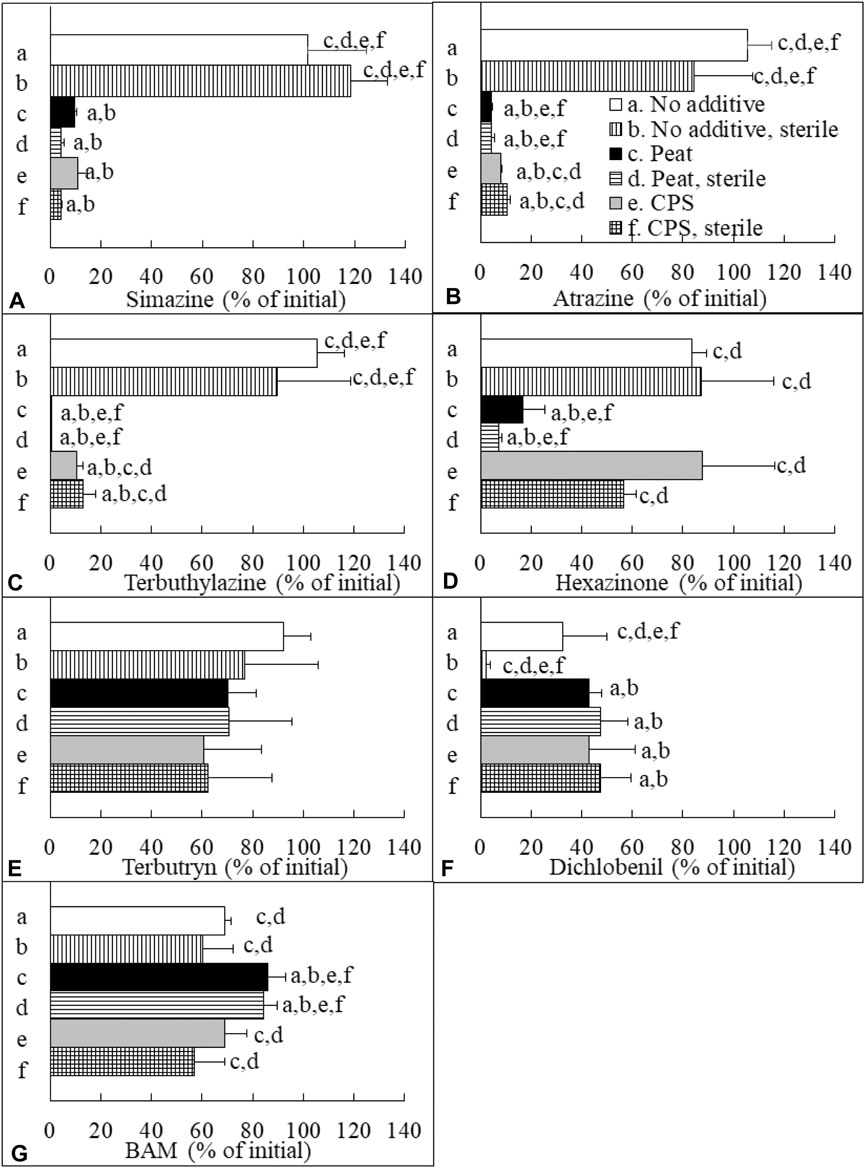
FIGURE 1. (A) Simazine (B) atrazine (C) terbuthylazine (D) hexazinone (E) terbutryn (F) dichlobenil, and (G) 2.6-dichlorobenzamide (BAM) concentrations as % of the initial in the mineral soil after 241 days incubation without or with peat or compost-peat-sand (CPS) addition, including sterilized counterparts. (a) Soil without additives, (b) sterilized soil without additives, (c) soil with peat, (d) sterilized soil and peat, (e) soil with CPS, and (f) sterilized soil and CPS. The error bars indicate standard deviations (n = 3), and the letters next to the bars show statistically significant differences (Kruskal-Wallis test followed by Mann-Whitney’s test).
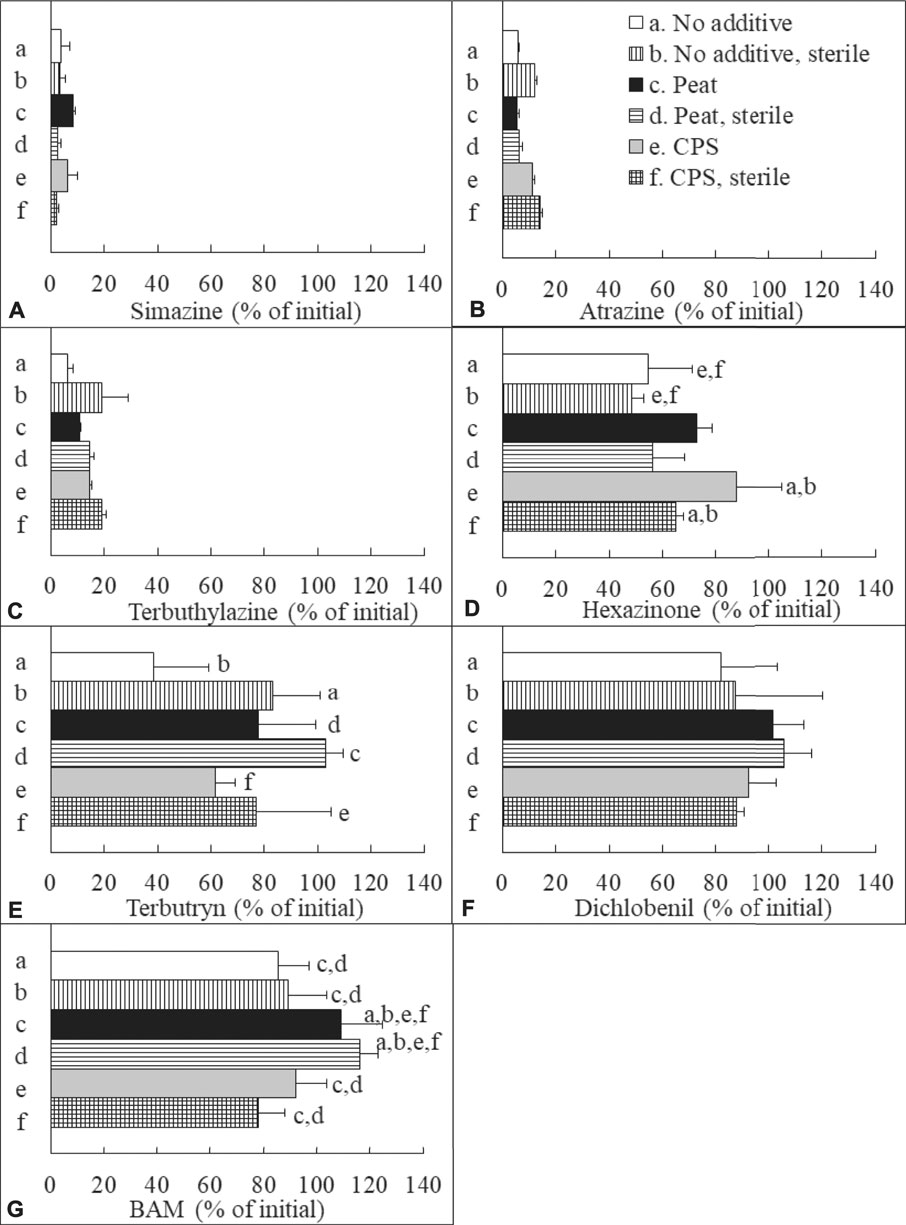
FIGURE 2. (A) Simazine, (B) atrazine, (C) terbuthylazine, (D) hexazinone, (E) terbutryn, (F) dichlobenil, and (G) 2.6-dichlorobenzamide (BAM) concentrations as % of the initial in the organic soil after 241 days incubation without or with peat or compost-peat-sand (CPS) addition, including sterilized counterparts. (a) Soil without additives, (b) sterilized soil without additives (c), soil with peat (d) sterilized soil and peat, (e) soil with CPS, (f) sterilized soil and CPS. The error bars indicate standard deviations (n = 3), and the letters next to the bars show statistically significant differences (Kruskal-Wallis test followed by Mann-Whitney’s test).
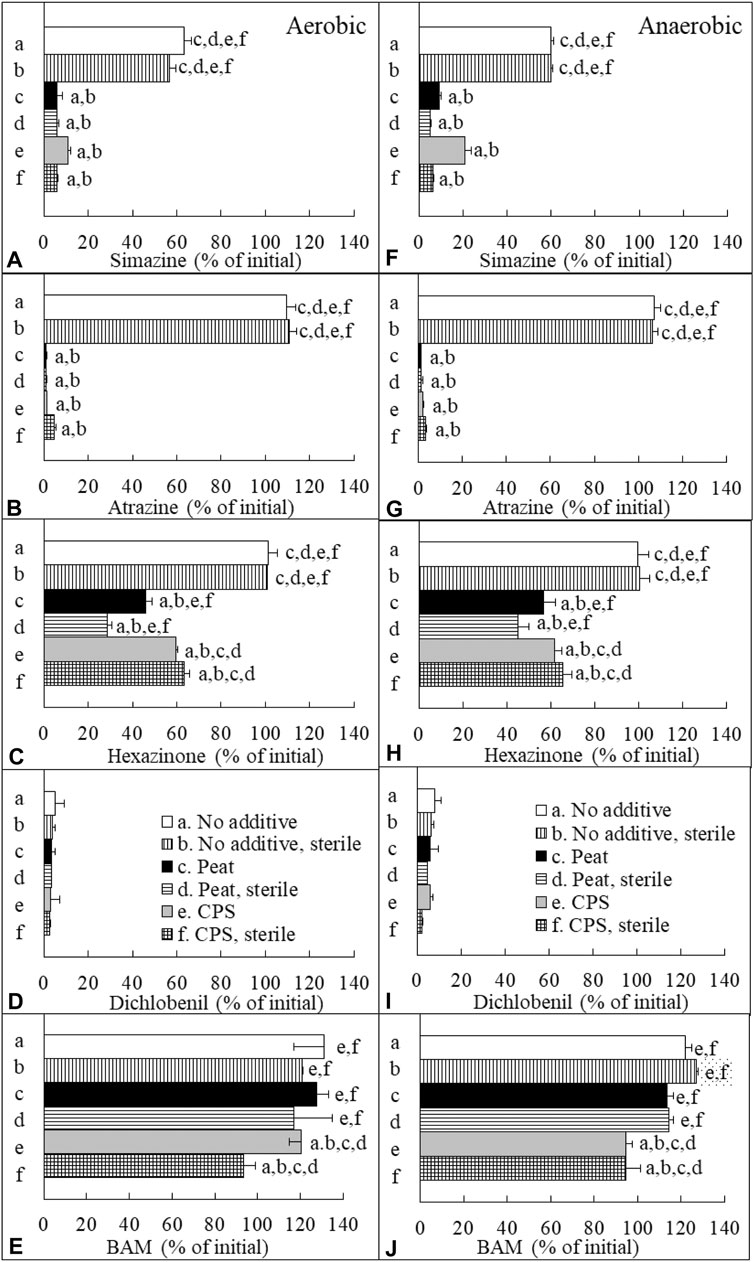
FIGURE 3. (A) Simazine (B) atrazine (C) hexazinone (D) dichlobenil and (E) 2,6-dichlorobenzamide (BAM) concentrations as % of the initial in the groundwater sediment slurries under aerobic conditions; and (F) simazine (G) atrazine (H) hexazinone (I) dichlobenil and (J) 2,6-dichlorobenzamide (BAM) concentrations as % of the initial in the groundwater sediment slurries under anaerobic conditions. (a) Slurries without additives, (b) sterilized slurries without additives (c), slurries with peat (d) sterilized slurries and peat, (e) slurries with CPS, (f) sterilized slurries and CPS. The error bars indicate standard deviations (n = 3), and the letters next to the bars show statistically significant differences (Kruskal-Wallis test followed by Mann-Whitney’s test).
The organic additives used in the organic and mineral soils, as well as in groundwater sediment slurries were 5% peat (OM, 967 ± 1 mg g−1) and 15% compost-peat-sand mixture (CPS OM, 508 ± 11 mg g−1) (Table 1). The total-C content was 526.9 ± 0.5 mg g−1 in peat and 262.4 ± 6.8 mg g−1 in CPS, and the total-N content was 12.4 ± 0.2 mg g−1 in peat, and 10.5 ± 0.2 mg g−1 in CPS. The total-C and total-N concentrations in the organic soil were 49.0 ± 2.9 mg g−1 and 3.4 ± 0.3 mg g−1, respectively, while their quantities in the mineral soil and groundwater sediments were below the quantification limit.
Peat and Pesticide Dissipation in Soil and Sediment Slurries
The long-term dissipation of simazine, atrazine, terbuthylazine, hexazinone and terbutryn, as well as dichlobenil and its degradation product BAM was compared in the mineral and organic soils and their sterilized counterparts without and with 5% peat (OM 96.7%) after 241 days of incubation (Table 1). The results showed that simazine, atrazine, and terbuthylazine dissipated down to 0–19% of the initial concentration in the organic soil, and in the peat-amended soils, regardless of sterilization (MW, p ≤ 0.004 in mineral soil; Figures 1, 2). Chlorinated dissipation products DEA, and DIA were detected but did not accumulate. In addition, peat in the mineral soil enhanced hexazinone dissipation down to 7–17% of the initial concentration compared to 83–87% of the initial in the mineral soil without peat (MW, p = 0.004). However, in the organic soil, peat had no significant effects on hexazinone concentrations ranging from 48 to 73% of the initial, all regardless of sterilization (MW, p = 0.078). Peat addition did not affect terbutryn concentrations in the mineral and organic soils (MW, p = 0.078), where the concentrations varied quite much between 39 and 100% of the initial. However, terbutryn concentrations in the organic soils were lower than in the sterilized counterparts, regardless of additives (MW, p < 0.001).
Dichlobenil and BAM behaved differently. Dichlobenil and BAM concentrations in the mineral soil were always lower than in the organic soil (MW, p < 0.001) (Figures 1, 2). Dichlobenil and BAM dissipated in the mineral soil better than in the peat-amended mineral soil (MW, p ≤ 0.016), and they did not dissipate in the organic soil below the initial concentrations regardless of peat addition; all regardless of sterilization.
The long-term relationship between peat and pesticide dissipation was further evaluated in low-carbon groundwater sediment slurries and their sterilized counterparts under aerobic and anaerobic conditions without and with peat addition after 399 days (Kerminen et al., 2018). According to the results, the concentrations of simazine, atrazine, and hexazinone in the peat-amended sediment slurries decreased to 5.2–9.1, 1.0–1.3 and 28.8–56.8% of the initial compared to the levels without peat of 56.8–63.3, 97.0–107.3, and 99.4–101.4% of the initial, respectively, independent of oxygen and sterilization (MW, p < 0.001) (Figure 3). Dissipation products DEA, and DIA did not accumulate. Again, dichlobenil and BAM behaved differently. Dichlobenil dissipated down to 3.6–5.8% of the initial concentration in the peat-amended sediment slurries, and equally to 4.1–8.2% of the initial in the slurries without additives. BAM concentrations in the peat amended sediment slurries (113.6–127.7%) were almost the same as in the slurries without additives (121.1–131.2%). BAM concentrations above 100% of the initial may be due to dissipation of dichlobenil to BAM (Holtze et al., 2007).
The results showed that pesticide dissipation differed between the mineral and organic soils, and groundwater sediment slurries (Figures 1–3). Peat OM addition changed simazine, atrazine, terbuthylazine (not in sediment slurries), and hexazinone dissipation in the mineral soil and groundwater sediment slurries towards that found in the organic soil, i.e., peat addition improved the dissipation. Similarly, peat OM addition changed dichlobenil and BAM dissipation in the mineral soil towards that in the organic soil, but in this case, peat addition slowed dichlobenil and BAM dissipation. However, dichlobenil dissipated almost completely in the groundwater sediment slurries, regardless of peat, oxygen, or sterilization; that is the excess water removed the dichlobenil dissipation retarding effect of peat, observed in the mineral soil.
The general perception that OM increases soil microbial activity, leading to accelerated biotic xenobiotic dissipation (Burauel and Baßmann, 2005; Krutz et al., 2010; Fenner et al., 2013; Totsche et al., 2018) was not suitable for the soils and sediments of this study. Pesticide dissipation was chemical, and microbial degradation was hardly found in the soils not adapted to pesticides, and in the groundwater sediment slurries adapted to pesticides, based on comparison with the sterilized counterparts (KW, p > 0.050; Figures 1–3). Terbutryn concentrations in the organic soil were only slightly lower than in the sterilized counterparts regardless of peat (KW/MW, p < 0.001), suggesting possible microbial degradation, if autoclaving did not inactivate reactive sites in the sterilized organic soil.
To verify the reliability of the results, possible error sources were checked. Although 27% of sediment slurries showed microbial growth after 182 days, pesticide concentrations in the sterilized sediment slurries with and without microbial growth did not differ, while concentrations after 182 days had already stabilized at the same levels as measured after 399 days (Kerminen et al., 2018). Further, the greatest simazine and atrazine concentrations dissipated rapidly in all three parallel sterilized soil and sediment slurry flasks with OM in 1 month, i.e., during a time when the flasks had not been opened and could not all show microbial growth. The pesticides were added to all flasks last immediately prior to incubations in the same methanol volume, 2.3 ml for soils and 1.7 ml for slurries. Although methanol quantities were somewhat high, pesticides were not added first, followed by methanol evaporation, due to the high risk of contamination and uneven distribution. Despite of this, the OM-related changes in atrazine, terbutryn, dichlobenil, or BAM concentrations observed in the pesticide mixture experiments of this study were mostly very similar to those in previous experiments with low methanol quantities, indicating that methanol apparently did not significantly affect pesticide dissipation. For example, atrazine was added to the groundwater sediment slurries with 75–113 µL of methanol (Kerminen et al., 2018); atrazine and terbutryn were added to the groundwater deposit slurries with 250 µL of methanol (Talja et al., 2008); and dichlobenil and BAM were added to groundwater deposit slurries and soil with 110 µL of methanol (Pukkila and Kontro, 2014). In fact, commercial pesticides can also be stored in methanol due to good solubility and long-term stability. All these facts suggest that methanol was not a key factor in pesticide dissipation, although it has interacted with experimental materials, and may have activated microbes as an additional carbon source without causing microbial pesticide degradation.
To further assess the reliability, there may have been no problems with pesticide extraction. For example, simazine, atrazine, and terbuthylazine dissipated in three parallel organic soils down to 3.9–19.3% of the initial, while dichlobenil was quantitatively extracted from the same soils (Figure 2). Similarly, almost all triazines were extracted from three parallel mineral soils while dichlobenil dissipated (Figure 1). However, the low pH below 6.0 has caused abiotic triazine dissipation (Krutz et al., 2010), while microbial atrazine degradation has been retarded at low pH values below 5.5–5.7 (Goux et al., 2003; Mueller et al., 2010). Indeed, the pH dropped to 4.3 ± 0.1 in the mineral and organic soils after peat addition, and varied between 4.6–5.5 in the sediment slurries with peat. In addition, peat has activated microorganisms very little compared to, for example, compost (Mandelbaum and Hadar, 1990; Vepsäläinen et al., 2004; Jindo et al., 2016).
Compost-peat-sand Mixture and Pesticide Dissipation in Soil and Sediment Slurries
To further examine the impact of pH and OM on pesticide dissipation, the experiments in the mineral and organic soils, and in the groundwater sediment slurries were conducted using 15.0% compost-peat-sand (CPS) mixture (OM 50.8%) as an additive (Table 1). CPS has a better buffer capacity in soil than peat, and the pH was 6.5 ± 0.1 in the CPS-amended mineral and organic soils, and 6.2–7.7 in the CPS-amended groundwater sediment slurries.
In agreement with the results of peat-amended soils, regardless of sterilization simazine, atrazine, and terbuthylazine dissipated down to 2–19% of the initial concentration in the CPS-amended mineral and organic soils (MW, p = 0.004 in mineral soil, Figures 1, 2). DEA and DIA did not accumulate even though they were detected. CPS did not affect hexazinone dissipation in the mineral soil (MW, p = 0.423), though it slowed dissipation in the organic soil (MW, p = 0.025), all regardless of sterilization. CPS had only minor effects on terbutryn dissipation in the mineral and organic soils, but in the CPS-amended organic soil terbutryn dissipated more than in the sterilized counterpart (MW, p < 0.001). In contrast, dichlobenil dissipated in the mineral soil better than in the CPS-amended mineral soil (MW, p < 0.001), and it did not dissipate in the organic soil regardless of CPS addition, all regardless of sterilization (Figures 1, 2). CPS did not affect BAM dissipation in the mineral and the organic soils. Peat addition improved atrazine, terbuthylazine and hexazinone dissipation in the mineral soil more than CPS (MW, p ≤ 0.004), while peat addition slowed BAM dissipation in the mineral and organic soils more than CPS (MW, p ≤ 0.010).
The effects of CPS on pesticide dissipation in the groundwater sediment slurries were often similar to those of peat (Figure 3). Concentrations of simazine, atrazine, and hexazinone in the CPS-amended sediment slurries decreased to 6.1–20.8, 1.3–4.7 and 59.7–65.6% of the initial, respectively, independent of oxygen and sterilization (MW, p < 0.001). The dissipation products DEA, and DIA could be detected but did not accumulate. However, dichlobenil dissipated down to 2.4–6.1% of the initial in the CPS-amended sediment slurries regardless of oxygen and sterilization, i.e., the excess water reduced the peat and CPS-caused dichlobenil dissipation retarding effects observed in the mineral soil. BAM concentrations in the CPS amended sediment slurries varied between 93.4 and 120.3% of the initial, and were lower than in the sediment slurries without or with peat, regardless of oxygen and sterilization.
The results strongly suggest that OM may cause the chemical dissipation of chlorine containing triazines and hexazinone in soil and sediment slurries, and the dissipation was not due to low pH. In general, the molecules are protonated below pKa values, which are for simazine 1.6, atrazine 1.7, terbuthylazine 2.0, hexazinone 1.2, terbutryn 3.9, DEA 1.4, DIA 1.5, and pentachloroaniline 4.0. Adsorption is greatest at pH values close to pKa. Molecules become anions (weak bases) as the pH rises above pKa values, and they will bind to positively charged minerals or ions (Bouchard et al., 1988; Seybold and Mersie, 1996; Oliveira et al., 2001; Lide, 2002; Chefetz et al., 2004), which appeared to be appropriate for chemical dissipation in the soils and sediment slurries of this study. The high pKa value 13–14 of amides, such as BAM (Chiu and Lo, 2000), may be associated with the observed poor dissipation of BAM compared to other compounds.
Although microbial activity was likely to increase especially in the CPS amended soils (Jindo et al., 2016) microbial pesticide degradation was hardly observed, apparently due to the absence of degrading microbes in the unexposed soils. Although groundwater sediment slurries were known to contain at least atrazine-degrading microorganisms, no microbial degradation was observed, as the complex formation ability of atrazine with sediment colloids may have inhibited bioavailability (Kerminen and Kontro, 2017). Microbial numbers in the sediments have varied between 3.2–3.7 × 107 cells g−1 based on phospholipid fatty acids (PLFAs), while cultured microbial numbers in the sediment slurries without additives have varied between 0.1–6.7 × 104 cfu mL−1 (Mattsson et al., 2015; Liu et al., 2020). The average copy numbers of bacterial, archaeal, and fungal genes in boreal arable soils have been 0.86–1.3 × 1010, 1.9–3.7 × 107, and 2.7–7.9 × 108 copies g−1 dry soil, while total-C and total-N concentrations have varied between 3.95–5.11 and 0.15–0.20 mg g−1 (Peltoniemi et al., 2021). Based on this background, the organic soil of this study may have had a very active microbial community, as it was fertilized with food-based compost, and total-C and total-N concentrations (Table 1) were higher than presented in Peltoniemi et al. (2021). However, microbial activity in the mineral soil and sediment slurries may have been low, as total-C and total-N were below the detection limits. As a result, OM did not appear to improve the bioavailability of atrazine-degrading microbes in the sediment slurries (Kerminen and Kontro, 2017), although it enhanced chemical dissipation. The results emphasize that the chemical dissipation of pesticides in the environment would appear to be utmost important. OM accelerates, slows down, or stops dissipation.
Long-Term Pesticide Dissipation in Soil and Agar Effects
To further assess the impact of OM and minerals on pesticide dissipation in soil, the long-term concentrations of PCA, HCB, DDT, and DDT metabolites were determined from soils collected from old market gardens, where gardening had continued 12–50 years until it ended in 1978–91 (Närhi, 2008). Interestingly, the lowest PCA and HCB concentrations were measured in the lowest OM soils (1.3–1.9%), while the lowest DDT and metabolite concentrations occurred in the rich-OM soils (≥7.3%) (Figure 4, correlation coefficient R ≥ 0.830). No previous reports of combined results on long-term pesticide concentrations and soil organic matter contents under stabilized conditions were found. Little is known about the aerobic biodegradation of chlorinated benzenes and DDT, and they are considered recalcitrant, as the focus has often been on reductive dechlorination under anoxic conditions (Brahushi et al., 2017; Kengara et al., 2019). However, HCB has dissipated in low dissolved carbon clay soils by destabilizing using anaerobic-aerobic cycles, with the addition of compost improving dissipation (Brahushi et al., 2017). The soils of this study were from the northern boreal zone, whose characteristic conditions, i.e., high soil OM storage accumulation (Smolander and Kitunen, 2021), may have contributed dissipation.
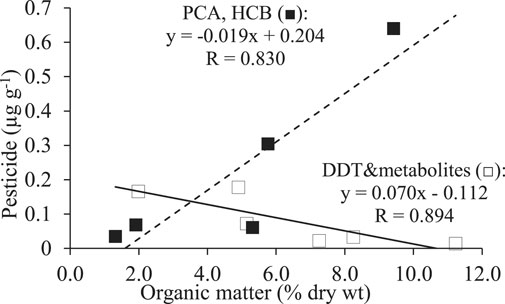
FIGURE 4. Organic matter correlations with pentachloroaniline (PCA) and hexachlorobenzene (HCB) concentrations (■); and with dichlorodiphenyltrichloroethane (DDT) and DDT metabolites concentrations (□) in old market garden soils, where gardening continued for 12–50 years until it ended in 1978–91 (n = 3).
Plant-derived OM appeared to enhance chlorinated triazine and hexazinone dissipation, and possibly also that of DDT and metabolites (Figures 1–4). To further investigate the importance of OM quality, pesticide dissipation was monitored on algal agar. As a result, atrazine dissipated slightly, and terbutryn, hexazinone and BAM dissipated more in agar-solidified water (Figure 5). The percentages of pesticides remaining increased as follows (averages of days 5–33): Terbutryn (31.7 ± 10.1%) < BAM (45.6 ± 16.9%) = hexazinone (51.4 ± 14.0%) < atrazine (78.1 ± 5.1%) (MW, p ≤ 0.010). The OM composition of algae agar improved terbutryn and BAM dissipation better than that of peat or CPS. However, 1.5% agar did not have enough reactive sites for complete dissipation of the four pesticides.
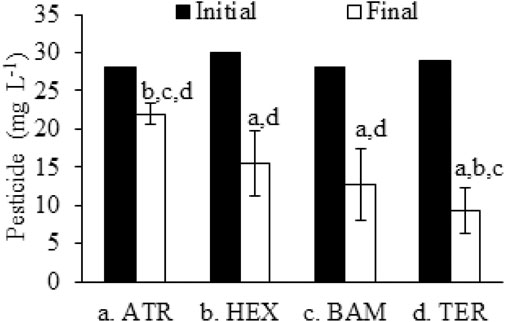
FIGURE 5. Atrazine (ATR), hexazinone (HEX), 2,6-dichlorobenzamide (BAM), and terbutryn (TER) concentrations in 1.5% agar-solidified water after stabilization of concentrations (days 5–33). The error bars indicate standard deviations (n = 15), and the letters next to the bars show statistically significant differences (Mann-Whitney’s test).
Organic Matter, Minerals, and Xenobiotic Dissipation
The results suggest that part of organic pollutants dissipate best in rich OM environments, and others in mineral-type environments without OM, such as chlorine containing triazines and DDT dissipated best in the organic soil, while dichlobenil, PCA, and HCB dissipated best in the mineral soil (Figures 1, 2, 4). Similarly, in the low-organic groundwater sediment slurries, OM enhanced triazine dissipation, whereas OM did not affect much dihlobenil dissipation (Figure 3). However, the quantity of OM-induced dissipation differed between the contaminants. Peat addition improved the dissipation of simazine, atrazine, terbutylazine and hexazinone in the mineral soil much more than that of terbutryn (Figure 1). CPS addition improved simazine, atrazine, and terbutylazine dissipation in the mineral soil, and at the same time had only minor effects on terbutryn, and hexazinone dissipation. In groundwater sediment slurries, both peat and CPS improved hexazinone dissipation.
Water solubility, vapor pressure, octanol/water partition coefficient (Kow), soil adsorption coefficient (Koc), and Henry’s constant could not be correlated with the dissipation of pesticides, although in some cases they can clearly explain dissipation (Kerminen et al., 2018). The differences in dissipation might be due to the different chemical structures of the compounds and the number of reactive sites in the OM. Chlorinated atrazine, simazine, and terbutylazine with rather similar structures dissipated almost equally, while hexazinone with a cyclohexyl ring and terbutryn with a methylthio group dissipated only slowly in the organic soil (Figure 2). Dichlobenil having benzene ring with a nitrile group surrounded by two chlorines, PCA having benzene ring with an amino group and five chlorines, and HCB having benzene ring with six chlorines dissipated best in the mineral soil (Figures 1, 4). However, BAM having benzene ring with an amide group surrounded by two chlorines dissipated poorly in soils and sediment slurries with plant-derived OM, only with agar (Figures 1–4). The OM quality also appeared to affect the dissipation of the compounds. For example, the addition of peat enhanced hexazinone dissipation in the mineral soil, but the addition of CPS did not (Figure 1). Furthermore, peat or CPS additions did not enhance terbutryn and BAM dissipation, but agar improved (Figures 1–4). Overall, the results suggest that chemical dissipation of pollutants in the environment may be much more important than previously has been assumed.
During peat formation, the concentrations of plant cellulose, hemicellulose, and lignin decrease, while the concentrations of humic acids, fulvic acids, and humin increase, and in addition, the peat contains waxes and resins (Virtanen et al., 2003). Atrazine and humic acids have been reported to form ionic and hydrogen bonds simultaneously with charge-transfer complexes and electron donor-acceptor processes involving free radical intermediates known to be related to molecule structure disruption. Hydrophobic HCB and DTT have been reported to become increasingly bound to soil lipid, lignin or polysaccharide-like substance fractions (Senesi et al., 1987; Xie et al., 1997; Gevao et al., 2000; Zhang et al., 2007; Barchańska et al., 2020; He et al., 2020). As a result of these ageing-related phenomena the toxicity of atrazine and DDT has decreased (Gevao et al., 2000). Based on the results of this study, the reduction in atrazine and DDT toxicity may have been in part due to chemical dissipation caused by OM, whereas HCB may have remained bound to OM without reacting (Figures 1–4).
The relative importance of abiotic and biotic contaminant dissipation has been much debated. Chemical atrazine dissipation is common in soil without a previous history of atrazine use (Blumhorst and Weber, 1994), and microbial degradation has hardly been observed (Lima et al., 2009), as could also be seen from the results of this study. Then, after repeated use of pesticides, both microbial and chemical dissipation have been measured (Winkelmann and Klaine, 1991) until finally, pesticide dissipation is based entirely on microbial degradation (Abdelhafid et al., 2000; Morgante et al., 2012). In the literature, at least in part, this development has led to the conclusion that chemical dissipation was thought to predominate before the 1990s, and since then it has been speculated that the microbial degradation is more important than abiotic dissipation (Krutz et al., 2010). However, abiotic dissipation and biotic degradation have rarely been separated in experiments properly using sterile controls.
The cleansing capacity of the environment has been discussed at least in the context of marine colloids and the atmosphere (Prinn, 2003; Santschi, 2018). The results of this study, together with previous literature (Winkelmann and Klaine, 1991; Blumhorst and Weber, 1994; Abdelhafid et al., 2000; Flores et al., 2009; Lima et al., 2009; Kerminen et al., 2018), strongly suggest that chemical dissipation could be the first natural purification method to remove persistent organic pollutants from the environment. When the reactive sites of organic and inorganic compounds in the environment are consumed, the simultaneously evolving microbial degradation of persistent organic pollutants remains (Abdelhafid et al., 2000; Morgante et al., 2012). In highly contaminated soils, microbial pollutant degradation can also be prevented due to contaminant toxicity (Fan and Song, 2014), thus depleting the cleansing capacity of the environment. Fortunately, the chemical cleansing capacity of the environment is renewed to some extent each year, for example, when tree leaves fall into the soil and provide more organic matter. In this way, pollutant concentrations could be sufficiently reduced through chemical dissipation and dilution, allowing microbial degradation to be again possible and even stimulated by the addition of OM.
Soils are losing OM and facing an increase in xenobiotic emissions due to population growth and associated urbanization, intensive farming, chemicalization, and fossil fuel combustion along with climate change (Lal, 2004; Fenner et al., 2013). Even a small increase in microbial OM decomposition rate due to climate change-caused rising temperatures has been evaluated to become significant at longer timescales in increasing recalcitrant compounds in organic soils, and areas of mineral soil (Davidson and Janssens, 2006; Marschner et al., 2008). The results of this study suggest that as OM decreases and mineral soil areas increase, pollutants that naturally dissipate in contact with OM are less removed from the environment, leading to their increasing stabilization in the mineral soils. The depletion of reactive sites from OM also results in enrichment of pollutants in organic soils. Stabilized compounds are bioavailable to microorganisms only in relatively high concentrations (Ros et al., 2006; Talja et al., 2008; Kerminen and Kontro, 2017). Pollutants tend to move quite easily deeper into mineral soils, where they do not interact with biological organisms (Gevao et al., 2000; Sun et al., 2010). However, when draining with flowing water into water bodies and groundwater, pollutants can return to the habitat, and pose environmental risks to living organisms. Moreover, floods caused by climate change can modify pollutant behavior, such as dichlobenil dissipated in the groundwater sediment slurries regardless of peat or CPS in contrast to the soils, while BAM concentrations even increased in the sediment slurries compared to the mineral soil.
Pesticides and OM Decomposition Process
The original research question was how pollutants enter the OM decomposition process. The results of this study strongly suggest that the selective preservation and progressive decomposition models are too restrictive for pollutants, as they exclude abiotic dissipation (Sollins et al., 1996; Lützow et al., 2006; Cotrufo et al., 2013; Lehmann and Kleber, 2015). The emphasis of these two models on biotic degradation processes has been based on the observation that soil OM consists of mixtures of microbial and plant constituents at different stages of degradation (Sollins et al., 1996; Burdon, 2001; Lützow et al., 2006; Cotrufo et al., 2013; Kallenbach et al., 2016). However, the same metabolites can be formed as a result of both microbial and chemical reactions. For example, triazine degradation products DEA, and DIA are considered to be microbial metabolites (Wackett et al., 2002), but in this and a previous study (Kerminen et al., 2018) they were also found in small quantities in sterilized soils and sediment slurries, although they did not accumulate.
In the SCM, organo-mineral bonds have been regarded to stabilize soil OM. Mineral-associated organic matter has been evaluated to persist for longer time than particulate organic matter consisting of lightweight, relatively undecomposed organic components (Kögel-Knabner et al., 2008; Lützow et al., 2006; Mikutta and Kaiser, 2011; Barchańska et al., 2020; Lavallee et al., 2020; Lehmann and Kleber, 2015). Based on this study, both organo-mineral and organo-organo interactions could be involved in stabilizing pollutants in the soil for a long time. Stabilization by organo-mineral interactions could be related to the persistence of simazine, atrazine, terburylazine, hexazinone, terbutryn, BAM, and DDT, and metabolites in the mineral soil and groundwater sediment slurries (Meng and Carper, 2000; de Jonge et al., 2004; Kerminen and Kontro, 2017). Interestingly, this stabilization could be overcome by adding OM, especially in the case of atrazine, simazine, and terbuthylazine, which could then, however, lead to the stabilization of compounds like dichlobenil in the soils. Other compounds that appeared to become stabilized through organo-organo interactions were PCA and HCB, as their concentrations were highest in the organic market garden soils (Figure 4). The stabilization phenomenon has been associated with pollutant adsorption to OM, and inhibition of bioavailability (Gevao et al., 2000; Lal, 2004; Lapworth et al., 2012; Fenner et al., 2013; James et al., 2019; Barchańska et al., 2020). Depending on the compound, organo-mineral and organo-organo interactions appeared to lead either stabilization of dissipation of contaminants.
According to the SCM, OM consists of substances that progressively decompose in biological, physical, and chemical processes (Lehmann and Kleber, 2015). In this study, some pollutants were degraded in organic soils, following the SCM model, for example, levels of chlorinated triazines, DTT, and their metabolites were lowest in organic soils (Figures 2, 3). However, dichlobenil, BAM, PCA and HCB appeared to be very persistent in organic soils for very long periods of time, i.e. they become stabilized in plant-derived organic soils. If the reactive sites in the soil are consumed in chemical reactions and the compounds become stabilized, dissipation will also cease in a favorable environment, such as small amounts of DDT were observed in organic greenhouse soils after decades. As the pesticides were significantly preserved in the old market garden soils, the results suggest that factors like the photochemical mineralization of sunlight (Austin and Vivanco, 2006); annual organic load in the form of decaying plant and fauna (Lehmann and Kleber, 2015); and degrading microbes with prevented bioavailability (Krutz et al., 2010; Fenner et al., 2013; Kerminen and Kontro, 2017) do not change the observed trends in dissipation rates.
The long-term persistence is not included in the SCM, as the evolving microbial degradation is thought to remove contaminants (Lehmann and Kleber, 2015). However, in groundwater sediments, microbial degradation of atrazine was inhibited at high concentrations of about 57 mg L−1, apparently due to the formation of strong complexes with organic and inorganic colloids (Kerminen and Kontro, 2017). This phenomenon is well known to lead to the persistence of atrazine for up to decades in the groundwater environment (Talja et al., 2008; Kerminen and Kontro, 2017). Similarly, atrazine concentrations of 10 mg kg−1 in soil had little effects on microbial numbers and diversity compared to 100 mg kg−1, which could be due to poor bioavailability and low biological effects at low concentrations (Ros et al., 2006). If this long-term persistence will not be taken into account in the entry of pollutants into OM decaying process, it will lead to an underestimation of the persistence of pollutants, and increased environmental pollution.
Conclusion
Generally, OM addition changed contaminant dissipation towards that observed in the organic environment, i.e. OM accelerated, slowed down or stopped dissipation. Simazine, atrazine, terbuthylazine, hexazinone, DDT, and metabolites appeared to dissipate best in rich OM environments, while dichlobenil, BAM, PCA, and HCB dissipated best in mineral-rich environments. BAM generally dissipated poorly, and plant-derived OM reduced BAM dissipation, while algae agar improved terbutryn and BAM dissipation (Figures 1–5). The distribution of contaminants between those dissipating in OM-rich environments and those dissipating in mineral-rich environments has not been previously reported. Although contaminant concentrations have been surveyed in a wide variety of environments, no previous literature was found on the relationships between organic matter, physicochemical compositions of the environments, and long-term dissipation of contaminants.
Pesticide dissipation was mainly chemical, and microbial degradation could only be observed for terbutryn dissipation in the organic soil. The result was expected in the organic and mineral soils not exposed to pesticides. However, in the pesticide-exposed groundwater sediment slurries known to contain degrading microbes, the presence of only chemical dissipation was an unexpected result. The absence of microbial degradation was most likely due to the ability of pesticides to form colloids or adsorb to OM without reacting chemically, leading to inhibition of bioavailability (Kerminen and Kontro, 2017; James et al., 2019), while OM addition overcame chemical dissipation inhibition. Interestingly, water in sediment slurries slowed BAM and accelerated dichlobenil dissipation compared to soils. Water impact on pollutant dissipation in the environment can become significant in the context of climate change-related floods. Locally polluting contaminants that are poorly dissipated in soil and water can be spread over large areas in the event of flooding, such as pesticides in groundwater, and on the other hand, the remediation of water-dissipating contaminants could be improved by increasing water volume. The concept of natural cleansing capacity for pollutants in soil and groundwater was elucidated. Chemical dissipation of pollutants could be the primary response to environmental pollution during which microbial degradation develops. Once the chemically reactive sites for the pollutants have been consumed in the environment, microbial degradation remains, until the natural cleansing capacity of the very polluted environment is completely lost due to pollutant toxicity to degrading microbes.
Some of the pollutants dissipated rapidly in agreement with the SCM model (atrazine, simazine, terbuthylazine, less hexazinone, terbutryn, DDT), while some remained stable for very long periods of time (BAM, PCA, HCB). Underestimation of stabilization easily leads to increased environmental pollution. Even if the contaminants dissipated well, low concentrations can remain persistent for a long time. Organo-organo and organo-mineral interactions have been associated with OM stabilization, while organo-mineral interactions have been suggested to have a stronger stabilizing effect (Lavallee et al., 2020). However, in this study, the addition of OM in the form of peat or CPS mixture destabilized especially atrazine, simazine and terbuthylazine in the mineral soil and sediment slurries enabling chemical dissipation, suggesting that organo-mineral interactions could also be destabilized quite rapidly. Models related to the OM decaying emphasis the importance of microbes (Cotrufo et al., 2013; Lehmann and Kleber, 2015; Kallenbach et al., 2016). However, the results of this study indicated that microbial degradation products can also be formed chemically (DEA, DIA, BAM). In studies of contaminant dissipation and OM decaying process, sterilized controls are important to reveal the dissipation mechanisms prevailing in the environment.
Data Availability Statement
The original contributions presented in the study are included in the article, further inquiries can be directed to the corresponding author.
Author Contributions
AH, KK, IN, and MM data acquisition. AH and MK statistical analyses and manuscript writing.
Funding
This work was supported by Maj and Tor Nessling foundation, Kolli foundation, Soil Research and Rehabilitation Association (MUTKU), and Soil and Water Technology foundation (Maa-ja Vesitekniikan tuki ry).
Conflict of Interest
The authors declare that the research was conducted in the absence of any commercial or financial relationships that could be construed as a potential conflict of interest.
Publisher’s Note
All claims expressed in this article are solely those of the authors and do not necessarily represent those of their affiliated organizations, or those of the publisher, the editors and the reviewers. Any product that may be evaluated in this article, or claim that may be made by its manufacturer, is not guaranteed or endorsed by the publisher.
References
Abdelhafid, R., Houot, S., and Barriuso, E. (2000). Dependence of Atrazine Degradation on C and N Availability in Adapted and Non-adapted Soils. Soil Biol. Biochem. 32, 389–401. doi:10.1016/S0038-0717(99)00167-4
Austin, A. T., and Vivanco, L. (2006). Plant Litter Decomposition in a Semi-arid Ecosystem Controlled by Photodegradation. Nature 442, 555–558. doi:10.1038/nature05038
Barchańska, H., Czaplicka, M., and Kyzioł-Komosińska, J. (2020). Interaction of Selected Pesticides with mineral and Organic Soil Components. Arch. Environ. Prot. 46, 80–91. doi:10.24425/aep.2020.134538
Baveye, P. C., and Wander, M. (2019). The (Bio)Chemistry of Soil Humus and Humic Substances: Why Is the "New View" Still Considered Novel after More Than 80 years? Front. Environ. Sci. 7, 27. doi:10.3389/fenvs.2019.00027
Blumhorst, M. R., and Weber, J. B. (1994). Chemical versus Microbial Degradation of Cyanazine and Atrazine in Soils. Pestic. Sci. 42, 79–84. doi:10.1002/ps.2780420203
Bouchard, D. C., Wood, A. L., Campbell, M. L., Nkedi-Kizza, P., and Rao, P. S. C. (1988). Sorption Nonequilibrium during Solute Transport. J. Contaminant Hydrol. 2, 209–223. doi:10.1016/0169-7722(88)90022-8
Brahushi, F., Kengara, F. O., Song, Y., Jiang, X., Munch, J. C., and Wang, F. (2017). Fate Processes of Chlorobenzenes in Soil and Potential Remediation Strategies: A Review. Pedosphere 27, 407–420. doi:10.1016/S1002-0160(17)60338-2
Burauel, P., and Baßmann, F. (2005). Soils as Filter and Buffer for Pesticides-Experimental Concepts to Understand Soil Functions. Environ. Pollut. 133, 11–16. doi:10.1016/j.envpol.2004.04.011
Burdon, J. (2001). Are the Traditional Concepts of the Structures of Humic Substances Realistic? Soil Sci. 166, 752–769. doi:10.1097/00010694-200111000-00004
Chefetz, B., Bilkis, Y. I., and Polubesova, T. (2004). Sorption-desorption Behavior of Triazine and Phenylurea Herbicides in Kishon River Sediments. Water Res. 38, 4383–4394. doi:10.1016/j.watres.2004.08.023
Chiu, F. C. K., and Lo, C. M. Y. (2000). Observation of Amide Anions in Solution by Electrospray Ionization Mass Spectrometry. J. Am. Soc. Mass. Spectrom. 11, 1061–1064. doi:10.1016/S1044-0305(00)00183-5
Cotrufo, M. F., Wallenstein, M. D., Boot, C. M., Denef, K., and Paul, E. (2013). The Microbial Efficiency-Matrix Stabilization (MEMS) Framework Integrates Plant Litter Decomposition with Soil Organic Matter Stabilization: Do Labile Plant Inputs Form Stable Soil Organic Matter? Glob. Change Biol. 19, 988–995. doi:10.1111/gcb.12113
Davidson, E. A., and Janssens, I. A. (2006). Temperature Sensitivity of Soil Carbon Decomposition and Feedbacks to Climate Change. Nature 440, 165–173. doi:10.1038/nature04514
de Jonge, L. W., Kjaergaard, C., and Moldrup, P. (2004). Colloids and Colloid-Facilitated Transport of Contaminants in Soils: An Introduction. Vadose Zone J. 3, 321–325. doi:10.2136/vzj2004.032110.2113/3.2.321
Fan, X., and Song, F. (2014). Bioremediation of Atrazine: Recent Advances and Promises. J. Soils Sediments 14, 1727–1737. doi:10.1007/s11368-014-0921-5
Fenner, K., Canonica, S., Wackett, L. P., and Elsner, M. (2013). Evaluating Pesticide Degradation in the Environment: Blind Spots and Emerging Opportunities. Science 341, 752–758. doi:10.1126/science.1236281
Flores, C., Morgante, V., González, M., Navia, R., and Seeger, M. (2009). Adsorption Studies of the Herbicide Simazine in Agricultural Soils of the Aconcagua Valley, central Chile. Chemosphere 74, 1544–1549. doi:10.1016/j.chemosphere.2008.10.060
Gevao, B., Semple, K. T., and Jones, K. C. (2000). Bound Pesticide Residues in Soils: A Review. Environ. Pollut. 108, 3–14. doi:10.1016/S0269-7491(99)00197-9
Goux, S., Shapir, N., El Fantroussi, S., Lelong, S., Agathos, S. N., and Pussemier, L. (2003). Long-term Maintenance of Rapid Atrazine Degradation in Soils Incoulated with Atrazine Degraders. Water Air Soil Poll 3, 131–142. doi:10.1023/A:1023998222016
He, X.-S., Zhang, Y.-L., Liu, Z.-H., Wei, D., Liang, G., Liu, H.-T., et al. (2020). Interaction and Coexistence Characteristics of Dissolved Organic Matter with Toxic Metals and Pesticides in Shallow Groundwater. Environ. Pollut. 258, 113736. doi:10.1016/j.envpol.2019.113736
Holtze, M. S., Hansen, H. C. B., Juhler, R. K., Sørensen, J., and Aamand, J. (2007). Microbial Degradation Pathways of the Herbicide Dichlobenil in Soils with Different History of Dichlobenil-Exposure. Environ. Pollut. 148, 343–351. doi:10.1016/j.envpol.2006.10.028
James, T. K., Ghanizadeh, H., Harrington, K. C., and Bolan, N. S. (2019). Effect on Herbicide Adsorption of Organic Forestry Waste Products Used for Soil Remediation. J. Environ. Sci. Health B 54, 407–415. doi:10.1080/03601234.2019.1574170
Jindo, K., Chocano, C., Melgares de Aguilar, J., González, D., Hernandez, T., and García, C. (2016). Impact of Compost Application during 5 Years on Crop Production, Soil Microbial Activity, Carbon Fraction, and Humification Process. Commun. Soil Sci. Plant Anal. 47, 1907–1919. doi:10.1080/00103624.2016.1206922
Kallenbach, C. M., Frey, S. D., and Grandy, A. S. (2016). Direct Evidence for Microbial-Derived Soil Organic Matter Formation and its Ecophysiological Controls. Nat. Commun. 7, 13630. doi:10.1038/ncomms13630
Kengara, F. O., Doerfler, U., Welzl, G., Munch, J. C., and Schroll, R. (2019). Evidence of Non-DDD Pathway in the Anaerobic Degradation of DDT in Tropical Soil. Environ. Sci. Pollut. Res. 26, 8779–8788. doi:10.1007/s11356-019-04331-x
Kerminen, K., and Kontro, M. (2017). Sonication Effects on Atrazine Dissipation in Vadose Zone Sediment Slurries. Environments 4, 18. doi:10.3390/environments4010018
Kerminen, K., Le Moël, R., Harju, V., and Kontro, M. H. (2018). Influence of Organic Matter, Nutrients, and Cyclodextrin on Microbial and Chemical Herbicide and Degradate Dissipation in Subsurface Sediment Slurries. Sci. Total Environ. 618, 1449–1458. doi:10.1016/j.scitotenv.2017.09.302
Kerminen, K., Salovaara, V., and Kontro, M. H. (2017). A Zero-Valent Iron and Organic Matter Mixture Enhances Herbicide and Herbicide Degradation Product Removal in Subsurface Waters. J. Environ. Sci. 57, 411–417. doi:10.1016/j.jes.2016.12.013
Kögel-Knabner, I., Guggenberger, G., Kleber, M., Kandeler, E., Kalbitz, K., Scheu, S., et al. (2008). Organo-mineral Associations in Temperate Soils: Integrating Biology, Mineralogy, and Organic Matter Chemistry. J. Plant Nutr. Soil Sci. 171, 61–82. doi:10.1002/jpln.200700048
Krutz, J. L., Shaner, D. L., Weaver, M. A., Webb, R. M., Zablotowicz, R. M., Reddy, K. N., et al. (2010). Agronomic and Environmental Implications of Enhanced S -triazine Degradation. Pest Manag. Sci. 66, 461–481. doi:10.1002/ps.1909
Lal, R. (2004). Soil Carbon Sequestration Impacts on Global Climate Change and Food Security. Science 304, 1623–1627. doi:10.1126/science.1097396
Lapworth, D. J., Baran, N., Stuart, M. E., and Ward, R. S. (2012). Emerging Organic Contaminants in Groundwater: A Review of Sources, Fate and Occurrence. Environ. Pollut. 163, 287–303. doi:10.1016/j.envpol.2011.12.034
Lavallee, J. M., Soong, J. L., and Cotrufo, M. F. (2020). Conceptualizing Soil Organic Matter into Particulate and mineral‐associated Forms to Address Global Change in the 21st century. Glob. Change Biol. 26, 261–273. doi:10.1111/gcb.14859
LECO Corporation (2019). Determination of Carbon, Nitrogen and Sulfur in Soil. Application Note 203-821-600. St. Joseph, MI, USA: LECO Corporation.
Lehmann, J., and Kleber, M. (2015). The Contentious Nature of Soil Organic Matter. Nature 528, 60–68. doi:10.1038/nature16069
Lide, D. R. (2002). Handbook of Chemistry and Physics. Boca Raton, London, New York, Washington, D.D.: CRC Press.
Lima, D., Viana, P., André, S., Chelinho, S., Costa, C., Ribeiro, R., et al. (2009). Evaluating a Bioremediation Tool for Atrazine Contaminated Soils in Open Soil Microcosms: the Effectiveness of Bioaugmentation and Biostimulation Approaches. Chemosphere 74, 187–192. doi:10.1016/j.chemosphere.2008.09.083
Liu, X., Hui, N., Kontro, M. H., and Chelinho, S. (2020). Improved Short-Term Microbial Degradation in Circulating Water Reducing High Stagnant Atrazine Concentrations in Subsurface Sediments. Water 12, 2507. doi:10.3390/w12092507
Lützow, M. V., Kögel-Knabner, I., Ekschmitt, K., Matzner, E., Guggenberger, G., Marschner, B., et al. (2006). Stabilization of Organic Matter in Temperate Soils: Mechanisms and Their Relevance under Different Soil Conditions - A Review. Eur. J. Soil Sci. 57, 426–445. doi:10.1111/j.1365-2389.2006.00809.x
Mandelbaum, R., and Hadar, Y. (1990). Effects of Available Carbon Source on Microbial Activity and Suppression of Pythium aphanidermatum in Compost and Peat Container Media. Phytopathology 80, 794–804. doi:10.1094/phyto-80-794
Marschner, B., Brodowski, S., Dreves, A., Gleixner, G., Gude, A., Grootes, P. M., et al. (2008). How Relevant Is Recalcitrance for the Stabilization of Organic Matter in Soils? J. Plant Nutr. Soil Sci. 171, 91–110. doi:10.1002/jpln.200700049
Mattsson, M. K., Liu, X., Yu, D., and Kontro, M. H. (2015). Depth, Soil Type, Water Table, and Site Effects on Microbial Community Composition in Sediments of Pesticide-Contaminated Aquifer. Environ. Sci. Pollut. Res. 22, 10263–10279. doi:10.1007/s11356-015-4224-1
Meng, Z., and Carper, W. (2000). Effects of Hydration on the Molecular Structure of Metal Ion-Atrazine Dimer Complexes: a MOPAC (PM3) Study. J. Mol. Struct. THEOCHEM 531, 89–98. doi:10.1016/S0166-1280(00)00428-0
Mikutta, R., and Kaiser, K. (2011). Organic Matter Bound to mineral Surfaces: Resistance to Chemical and Biological Oxidation. Soil Biol. Biochem. 43, 1738–1741. doi:10.1016/j.soilbio.2011.04.012
Morgante, V., Flores, C., Fadic, X., González, M., Hernández, M., Cereceda-Balic, F., et al. (2012). Influence of Microorganisms and Leaching on Simazine Attenuation in an Agricultural Soil. J. Environ. Manage. 95, S300–S305. doi:10.1016/j.jenvman.2011.06.045
Mueller, T. C., Steckel, L. E., and Radosevich, M. (2010). Effect of Soil pH and Previous Atrazine Use History on Atrazine Degradation in a Tennessee Field Soil. Weed Sci. 58, 478–483. doi:10.1614/ws-d-09-00041.1
Närhi, I. (2008). Torjunta-aineiden Esiintyminen Maaperässä Ja Pohjavedessä Lopettaneilla Taimi- Ja Kauppapuutarhoilla. Vaasa: Länsi-Suomen ympäristökeskus, 7. 978-952-11-3291-9. http://urn.fi/URN.
Oliveira, R. S., Koskinen, W. C., and Ferreira, F. A. (2001). Sorption and Leaching Potential of Herbicides on Brazilian Soils. Weed Res. 41, 97–110. doi:10.1046/j.1365-3180.2001.00219.x
Overy, D. P., Bell, M. A., Habtewold, J., Helgason, B. L., and Gregorich, E. G. (2021). "Omics" Technologies for the Study of Soil Carbon Stabilization: A Review. Front. Environ. Sci. 9, 617952. doi:10.3389/fenvs.2021.617952
Peltoniemi, K., Velmala, S., Fritze, H., Lemola, R., and Pennanen, T. (2021). Long-term Impacts of Organic and Conventional Farming on the Soil Microbiome in Boreal Arable Soil. Eur. J. Soil Biol. 104, 103314. doi:10.1016/j.ejsobi.2021.103314
Prinn, R. G. (2003). The Cleansing Capacity of the Atmosphere. Annu. Rev. Environ. Resour. 28, 29–57. doi:10.1146/annurev.energy.28.011503.163425
Pukkila, V., and Kontro, M. H. (2014). Dichlobenil and 2,6-dichlorobenzamide (BAM) Dissipation in Topsoil and Deposits from Groundwater Environment within the Boreal Region in Southern Finland. Environ. Sci. Pollut. Res. 21, 2289–2297. doi:10.1007/s11356-013-2164-1
Ros, M., Goberna, M., Moreno, J. L., Hernandez, T., García, C., Insam, H., et al. (2006). Molecular and Physiological Bacterial Diversity of a Semi-arid Soil Contaminated with Different Levels of Formulated Atrazine. Appl. Soil Ecol. 34, 93–102. doi:10.1016/j.apsoil.2006.03.010
Santschi, P. H. (2018). Marine Colloids, Agents of the Self-Cleansing Capacity of Aquatic Systems: Historical Perspective and New Discoveries. Mar. Chem. 207, 124–135. doi:10.1016/j.marchem.2018.11.003
Senesi, N., Testini, C., and Miano, T. M. (1987). Interaction Mechanisms between Humic Acids of Different Origin and Nature and Electron Donor Herbicides: A Comparative IR and ESR Study. Org. Geochem. 11, 25–30. doi:10.1016/0146-6380(87)90048-9
Seybold, C. A., and Mersie, W. (1996). Adsorption and Desorption of Atrazine, Deethylatrazine, Deisopropylatrazine, Hydroxyatrazine, and Metolachlor in Two Soils from Virginia. J. Environ. Qual. 25, 1179–1185. doi:10.2134/jeq1996.00472425002500060002x
Smolander, A., and Kitunen, V. (2021). Soil Organic Matter Properties and C and N Cycling Processes: Interactions in Mixed-Species Stands of Silver Birch and Conifers. Appl. Soil Ecol. 160, 103841. doi:10.1016/j.apsoil.2020.103841
Sollins, P., Homann, P., and Caldwell, B. A. (1996). Stabilization and Destabilization of Soil Organic Matter: Mechanisms and Controls. Geoderma 74, 65–105. doi:10.1016/s0016-7061(96)00036-5
Sun, K., Gao, B., Zhang, Z., Zhang, G., Zhao, Y., and Xing, B. (2010). Sorption of Atrazine and Phenanthrene by Organic Matter Fractions in Soil and Sediment. Environ. Pollut. 158, 3520–3526. doi:10.1016/j.envpol.2010.08.022
Talja, K. M., Kaukonen, S., Kilpi-Koski, J., Malin, I., Kairesalo, T., Romantschuk, M., et al. (2008). Atrazine and Terbutryn Degradation in Deposits from Groundwater Environment within the Boreal Region in Lahti, Finland. J. Agric. Food Chem. 56, 11962–11968. doi:10.1021/jf802528a
Totsche, K. U., Amelung, W., Gerzabek, M. H., Guggenberger, G., Klumpp, E., Knief, C., et al. (2018). Microaggregates in Soils. J. Plant Nutr. Soil Sci. 181, 104–136. doi:10.1002/jpln.201600451
Vepsäläinen, M., Erkomaa, K., Kukkonen, S., Vestberg, M., Wallenius, K., and Niemi, R. M. (2004). The Impact of Crop Plant Cultivation and Peat Amendment on Soil Microbial Activity and Structure. Plant and Soil 264, 273–286. doi:10.1023/B:PLSO.0000047763.46795.cb
Vermeire, M.-L., Cornélis, J.-T., Van Ranst, E., Bonneville, S., Doetterl, S., and Delvaux, B. (2018). Soil Microbial Populations Shift as Processes Protecting Organic Matter Change during Podzolization. Front. Environ. Sci. 6, 70. doi:10.3389/fenvs.2018.00070
Virtanen, K., Hänninen, P., Kallinen, R.-L., Variainen, S., Herranen, T., and Jokisaari, R. (2003). The Peat Reserves of Finland in 2000. Kuopio: Geological Survey of Finland, Report of Investigation, 156.
Wackett, L. P., Sadowsky, M. J., Martinez, B., and Shapir, N. (2002). Biodegradation of Atrazine and Related s-Triazine Compounds: from Enzymes to Field Studies. Appl. Microbiol. Biotechnol. 58, 39–45.
Winkelmann, D. A., and Klaine, S. J. (1991). Degradation and Bound Residue Formation of Atrazine in a Western Tennessee Soil. Environ. Toxicol. Chem. 10, 335–345. doi:10.1002/etc.5620100306
Xie, H., Guetzloff, T. F., and Rice, J. A. (1997). Fractionation of Pesticide Residues Bound to Humin. Soil Sci. 162, 421–429. doi:10.1097/00010694-199706000-00004
Keywords: pesticides, long-term dissipation, organic soil, mineral soil, sediments, organic matter, peat, compost-peat-sand mixture
Citation: Harju AV, Närhi I, Mattsson M, Kerminen K and Kontro MH (2021) Organic Matter Causes Chemical Pollutant Dissipation Along With Adsorption and Microbial Degradation. Front. Environ. Sci. 9:666222. doi: 10.3389/fenvs.2021.666222
Received: 09 February 2021; Accepted: 05 August 2021;
Published: 20 September 2021.
Edited by:
Srđan Rončević, University of Novi Sad, SerbiaReviewed by:
Marijana Kragulj Isakovski, University of Novi Sad, SerbiaWojciech Smułek, Poznań University of Technology, Poland
Copyright © 2021 Harju, Närhi, Mattsson, Kerminen and Kontro. This is an open-access article distributed under the terms of the Creative Commons Attribution License (CC BY). The use, distribution or reproduction in other forums is permitted, provided the original author(s) and the copyright owner(s) are credited and that the original publication in this journal is cited, in accordance with accepted academic practice. No use, distribution or reproduction is permitted which does not comply with these terms.
*Correspondence: Merja H. Kontro, bWVyamEua29udHJvQGhlbHNpbmtpLmZp