- Department of Water, Atmosphere and Environment, Institute of Hydrobiology and Aquatic Ecosystem Management, University of Natural Resources and Life Sciences, Vienna, Austria
Land uses affect flood risks in various ways. The comparative analysis of the historical and current land cover/uses helps to better understand changing flood regimes. Based on historical cadastre maps from 1826 to 1859, the land cover/uses in the Austrian catchments of the rivers Rhine, Salzach and Drava were reconstructed to almost the level of exact plots of land. Catchment-wide analysis reveals a six-fold expansion of settlement areas, a decline of arable land by 69% and a shrinking of the formerly glaciated areas by 73% until 2016. In the Alpine fluvial corridors, i.e. flood-prone areas at the valley floors and valley sides at ca. 300-year floods, settlements even expanded 7.5-fold, severely increasing the potential for flood damages. At the same time, the overall channel area of running waters has been reduced by 40% and 95% the formerly large wetlands have been lost. Overall, the fluvial corridors were truncated by 203 km2 or 14%, thereby reducing flood retention capacity. The concentration of intensive forms of human land uses at lower altitudes, coupled with an upward shift of less intensively used, near-natural forms of land cover, has led to a both spatial and vertical separation of Alpine landscape features over the long term. Warmer temperatures due to climate change are expected to promote the demonstrated upward shifts of Alpine vegetation.
Introduction
The composition of land cover within a catchment is a basic factor for the surface runoff and, consequently, the flow, flood and sediment regimes of rivers (Overland and Kleeberg, 1991). Such relationships between terrestrial and fluvial systems, i.e., the consequences of deforestation and intensified land use on runoff and sediment supply, have been widely discussed since the late 18th century (Andréassian, 2004; Green and Alila, 2012). In the 1790s, French engineers concluded that deforestation and subsequent erosion were a major cause of a series of devastating floods in the French Pyrenees in the 1760s and 1770s (Pfister and Brändli, 1999). In respect of the Austrian Alps, such relationships were debated since the early 19th century, when the phenomenon became widely accepted (Wex, 1873). Beyond climate change, river channelization and the construction of flood protection facilities, increasing awareness is being devoted to the role of land cover in respect of amplified flood risks (Crooks and Davies, 2001; Bronstert et al., 2002; Brath et al., 2006; Haidvogl et al., 2018). Thus, the “UNESCO Division of Water Sciences” initiated a working group to analyze the relative role of climate change and land cover change on floods and low flows (Blöschl et al., 2007). Generally, the impact of land cover changes on flood regimes is difficult to estimate due to the spatial heterogeneity of larger catchments and the numerous associated–partially opposing–hydrological processes (Rogger et al., 2017; Blöschl, 2020). Hydrological models, i.e., spatial rainfall-runoff models, enable simulating the impacts of land cover changes on floods. Such models indicate that land-cover-related influences generally show larger effects at smaller floods than at extreme floods (Salazar et al., 2012; Blöschl et al., 2018). Moreover, in smaller catchments (up to 100 km2) the effects of land cover changes are more pronounced than in larger catchments. In smaller catchments, floods are mostly generated by highly intensive, short precipitation periods, so that soil infiltration surplus is the dominant process. In larger catchments, however, floods are mostly generated by longer lasting precipitation of lower intensities, so that the mechanism of saturation surplus dominates. Since land use changes usually influence the infiltration capacity (reflecting soil compaction), the effect on floods decreases with catchment area size (Hall et al., 2014; Rogger et al., 2017).
Beyond soil infiltration processes, land cover additionally plays an important role in flood conveyance areas adjacent to running waters. Here, the “hydraulic roughness” of the diverse types of land cover affects the potential for “flowing flood retention” (Teschke and Pasche, 2004). Dense alluvial forests generally show the highest hydraulic roughness, resulting in amplified “flowing retention”. Thus, alluvial forests slow down the flood wave propagation and reduce the flood peaks (Pasche and Ploeger, 2004; Horn and Richards, 2006).
Assessing the role of human land cover modifications for today’s Alpine flood risks requires large-scale analyses based on historical maps from the 19th century. Numerous comparative studies on the historical and current land cover/use have been published in recent decades from the perspective of agriculture, ecology, socio-economy and spatial planning (e.g., Krausmann, 2008; Tasser et al., 2009, 2011; Arnold et al., 2020). Many studies focus on shorter time frames of several decades up to 70 years depending on the availability of remote sensing data, which allow for automatized analysis (Feranec et al., 2000; Brath et al., 2006). Studies extending over longer periods (e.g., 200 years) have been conducted mostly for smaller sample areas due to the lengthy processing time and high costs of using historical cartographical sources (Zimmermann et al., 2010; Skalos et al., 2011). In Central Europe, such research is often based on the “Franziscean cadastre”, a spatially accurate land register that was compiled for the entire Habsburg Monarchy between 1817 and 1861 (Fuhrmann, 2007; Andric et al., 2010; Harvey et al., 2014). Studies on the historical land cover changes in larger regions often apply less accurate small-scale historical maps or conceptual/numerical models that rely on the changes of socio-economic variables such as population density or the progress of industrialization (Pongratz et al., 2008; Rutherford et al., 2008; Kaplan et al., 2009; Klein Goldewick et al., 2017).
To date, no detailed reconstruction of the historical land cover/use across larger Alpine areas that enables an in-depth comparative analysis of the current situation–both two and three-dimensionally–is available. The present study aims to partially fill this gap in knowledge as part of the interdisciplinary research project “Integrated Flood Risk Management in Mountain Areas: Assessing Sectoral Interdependencies, Conflicts and Options for Policy Coordination” (POCO-FLOOD). In that framework, the historical land cover changes in 23% of entire Austria were analyzed almost to the plot level using the “Franziscean cadastre”. The resulting data set will be used to model changes in flood risks due to human modifications of the Alpine land cover. In the present study, as a first step, we comparatively analyze the historical land cover/use between 1826–1859 and the changes until today, with a special focus on the Alpine fluvial corridors. In particular, the resulting GIS dataset helps answer the following research questions:
(1) How did the land cover change in the Austrian catchments of the Alpine rivers Rhine, Salzach and Drava?
As agrarian society declined, the agriculturally most productive areas became concentrated, whereas unfavorable sites were extensified or abandoned (Bätzing, 2003). New forms of use, such as settlement expansion and urbanization, along with increased leisure use and energy generation, began to establish in the second half of the 20th century. In the Austrian and Italian Alps the areas used for agriculture decreased considerably by 6–67% depending on the region (Tappeiner et al., 2006). Abandoned areas–many of them former upland Alpine pastures–have undergone natural regeneration of forests or have been afforested. In addition, arable land significantly decreased since the mid-20th century and was replaced by grassland and settlement areas. Similar developments have been reported by Rutherford et al. (2008) for the Swiss Alps or for other mountain regions in Europe (MacDonald et al., 2000; Zimmermann et al., 2010).
(2) Did the Alpine fluvial corridors follow a distinct, different development trajectory compared to the catchment-wide changes?
Historically, land use management and river engineering practices have varied regionally (Haidvogl et al., 2019). River corridors in the Alpine countries today therefore reflect different forms and intensities of human pressure. Generally, the most productive areas are located in the flat valley bottoms, which are characterized by an intensification of human uses (Schinegger et al., 2012; Muhar et al., 2019). This includes the urbanization of arable land or grassland. In the Alps, however, not all areas are equally affected by these dynamics. For example, in Carnia (NE. Italy) more than 50% of the former agricultural land in the valley bottoms have been taken out of use and are forested today (Tappeiner et al., 2006).
(3) How did the altitudinal distribution of the individual land cover/use types change over time?
The vertical distribution of certain forms of land cover primarily depends on the climatic conditions of the respective altitudinal zone (McVicar and Körner, 2013). While the foothills of the Alps and the Montane zone were largely wooded prior to human interventions, the Subalpine zone was characterized by the transition to Alpine shrubland. The Alpine zone was (or still is) dominated by grassland and wasteland. In the highest zone, the Nival or Glacial zone, wasteland and glaciers prevail (Kilian et al., 1994). Pre-industrial agriculture was based on principles of subsistence, and land use types were adapted to the varying environmental characteristics that are closely interrelated with the altitudinal zones. In contrast, industrialized agriculture, which developed in the Alps from the 1950s onwards in parallel to the construction of transport networks, is characterized by trading with agricultural products and by a concentration of land use in favorable areas along with abandonment in less productive land (Krausmann, 2016; Haidvogl et al., 2019). We assume that the retreat of agricultural uses in unfavorable locations and concentration in the valley bottoms is reflected by altered altitudinal distributions of settlement areas, arable land, grassland and forests. Climate change has also played a role in modified vertical land cover patterns. The historical reference (1826–1859) refers to the end of the “Little Ice Age”. Since then, increasing temperatures led to the encroachment of Alpine vegetation (grass, shrubs) into higher ranges (Dullinger et al., 2003, 2004; Walther et al., 2005; Fischer et al., 2019).
The present study provides a solid basis for further investigations such as land-cover-dependant effects on Alpine hydrology, flood risks and areal denudation processes. The large-area reconstruction of historical land cover/uses nearly to the exact plot of land facilitates new analyses at almost the same level of precision as current datasets. It also contributes to our current understanding of the role of human land use practices in Alpine catchments and fluvial corridors.
Study Area
The study includes the Austrian parts of the catchments of the Rhine River in the province Vorarlberg, the Salzach River (Salzburg) and the Drava River (Eastern Tyrol and Carinthia) within the area defined by the Alpine Convention (Figure 1). In total it stretches across an area of 19,307 km2 referring to 23% of entire Austria. Beyond parts of the main Alpine complex, the study area also includes some smaller areas of the Alpine foreland at its fringes. Limestone Alps and the Central Alps comprising crystalline and Palaeozic rocks cover the largest share of the study area. Sedimentary rocks, i.e., Flysch, form smaller areas of the northern foothills (based on the “International Geological Map of Europe”; Asch, 2003). In vertical dimension, the study area ranges between 338 and 3,798 m a. sl., covering vegetation zones between Submontane and Glacial levels (Kilian et al., 1994).
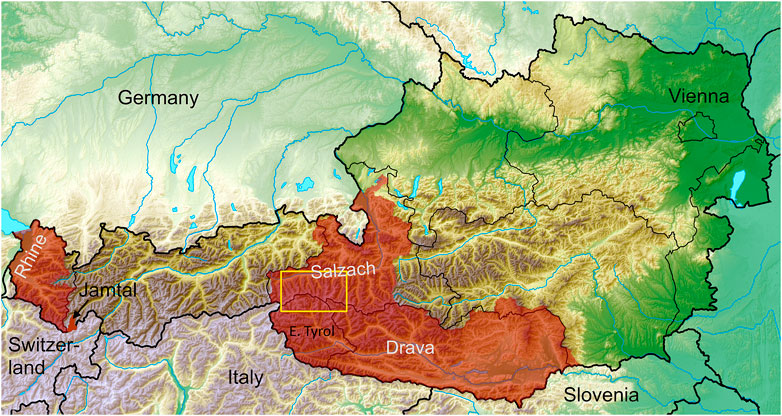
FIGURE 1. Study areas (red) in the Austrian Alps including the catchments of the rivers Rhine, Salzach and Drava. The “Jamtal” valley in the province Tyrol was analyzed in detail to validate the historical land cover reconstruction (yellow rectangle: see detail in Figure 3; basemap: Wikimedia Commons, Tschubby 2018).
In the Austrian Alps, humans became a major geomorphological agent during the Middle Ages, when land reclamation, deforestation as well as salt and ore mining were significantly promoted also north of the Alpine crest (Haidvogl et al., 2019). Smaller streams were rerouted or water was abstracted from larger rivers to mills for energy extraction. Alpine pastures in high altitudes go back to the early Iron Age and for the most part to the Middle Ages, thereby wiping out woody vegetation and lowering the treeline (Ellenberg, 1996). Systematic channelization of Alpine rivers and associated transformation of valley floors started in the early to mid-19th century (Hohensinner et al., 2021). The main purposes for the ambitious hydraulic projects were flood protection, land reclamation and the improvement of running waters for log driving and rafting (Haidvogl et al., 2019; Hauer et al., 2019). Further river straightening programs and the construction of dams and reservoirs for hydropower production during the 20th century additionally transformed Austria’s Alpine landscape.
Materials and Methods
Data Sources
The “Franziscean cadastre” that was produced in the scales 1:2,880 or 1:5,760 (1: 1,440 in urban areas) for the entire Habsburg Monarchy between 1817 and 1861 is the main historical source used for the present study (k.k. Landesregierung im Erzherzogtum Österreich unter der Enns, 1824; Fuhrmann, 2007; compare www.mapire.eu/en/map/cadastral/). The cadastre maps were created by military surveyors to calculate land taxes and show the land cover/uses of each plot of land. For the headwater region of the Drava River in Eastern Tyrol, however, the original cadaster maps were not available for GIS reconstruction (Figure 1). Here we had to rely on the so-called “Kulturen-Skelett-Karte” from the Provincial Archive Tyrol. This series of maps from around 1875 is a reproduction of the original cadastre maps in the larger scale of 1:36,000. Thus, it lacks details on small parcels and individual buildings. Because the cadastre is less accurate in high Alpine ranges, i.e., wasteland and glaciers above approximately 3,000 m a. sl., we additionally used the “Second Military Survey” (“Franziscean land survey”) produced by the later “k.k. Militärgeographische Institut” between 1807 and 1836 with a scale of 1:28,800 (www.mapire.eu/en/browse/country/secondsurvey/). It was dedicated for military use and therefore primarily focuses on the geographical situation and landscape structures such as water courses and terrain topography. Both map series optimally complement each other. Smaller wasteland areas that are not depicted in the historical sources, such as rock faces or minor talus fans, were introduced from the current land cover dataset to the extent that they could be assumed to have already existed in the early 19th century.
Surprisingly, it was almost easier to compile the data sources for the historical land cover than for the current state. A Sentinel-2 based land cover map with a resolution of 10 m created in the framework of the “Land information System Austria” (LISA) provides the main source for the dataset we compiled for the current situation (GeoVille Information Systems, 2017). However, arable land and grassland cannot be clearly differentiated in the LISA dataset. The differentiation depends on whether a field was just ploughed or on the planted crop. We therefore combined it with the INVEKOS dataset (“Integriertes Verwaltung-und Kontrollsystem”) from the Federal Ministry of Agriculture, Regions and Tourism (BMLFUW and BAB, 2017). This dataset comprises almost all parcels in Austria that are used for various agricultural purposes. In particular, we added areas used as meadows and pastures (grassland), arable land, vineyards and fruit plantations to the LISA dataset. Current extents of glaciers were derived from Buckel and Otto (2018). The surface area of mid-sized rivers and that of smaller ponds was added from OpenStreetMap as GIS shapefiles (Ramm, 2019). Finally, smaller rivers and streams were integrated from a dataset of the project “Strategic Planning for Alpine River Ecosystems” (Muhar et al., 2018). The resulting dataset approximates the land cover in 2016.
Several flood hazard datasets were used to delineate the extents of the fluvial corridors. A GIS dataset comprising the flood-prone areas at extreme 300-year floods was provided by the above-mentioned federal ministry. It includes the flood danger zones along larger rivers (from Bundeswasserbauverwaltung), smaller torrents and gullies in valley sides (from Wildbach-u. Lawinenverbauung). Because these data were missing for individual rivers, we combined the GIS dataset with the “Austrian Flood Hazard Map” (HORA) for 200-year floods (BMLFUW, 2014). Artificial gaps between the different spatial datasets were manually adjusted. Finally, the 3D-analyses were conducted based on a digital elevation model (DEM) with a resolution of 10 m (data.gv.at–Open Data Austria). Most of the resulting dataset refers to (former) floodplains in broader valley floors, but also integrates very narrow fluvial corridors of steep torrents and narrow to wide flood-prone areas at the valley sides.
Land Cover/use Classification
In the study area, the “Franziscean cadastre” reveals approximately 30 types of land uses and land cover that are supplemented by approximately 70 cartographic symbols (k.k. Landesregierung im Erzherzogtum Österreich unter der Enns, 1824; Fuhrmann, 2007). The dataset compiled for the current situation distinguishes between 23 classes of land cover/uses. In agreement with the requirements for later rainfall-runoff modeling, the historical and current classes were aggregated to 11 classes (Table 1).
GIS and Data Analyses
The georeferenced historical cadastre maps were manually vectorized using ESRI ArcGIS 10.6. Almost all parcels were drawn as shapefile polygons with the respective land cover/use class added as attribute values (Berger, in prep; Bozzetta, in prep.; Höberth, in prep.; Kofler, 2021; Rapottnig, 2021; Sterle, in prep.). In order to keep the data preparation within reasonable time limits, parcels with mean widths <10 m between larger parcels referring to other classes were not separately delineated. Similarly, small streams with channel widths of less than 5 m (including sediment bars) were not vectorized. The resulting channel network includes all running waters with catchments >10 km2. In addition, streams in smaller catchments were also considered if their historical channel width exceeded 5 m. In order to enable a methodologically sound comparative analysis with the current state, the dataset for 2016 includes only those water courses that are shown by the historical dataset. To validate the accuracy of the historical dataset, the “Jamtal” valley in the province Tyrol served as the detail study site (Figure 1). In the framework of the research project “Changing debris cover on Eastern Alpine glaciers: quantification and hydrological impacts” (Hidden.Ice) the land cover changes in that valley were reconstructed using the “regressive-iterative” method described in Hohensinner et al. (2013). Here, starting from the state in 2015, those in 1970, 1921, 1870, and 1820 were reconstructed going back in time based on numerous historical sources (Atzler, in prep.)1. This method enables a significantly more accurate reconstruction of former landscape features compared to the analysis of the state at a single point in time.
The current extents of the flood-prone areas at ca. 300-year floods served as a basis for approximating the historical fluvial corridors, i.e. the valley floors, torrents and valley-side gullies that were inundated at extreme floods. For all running waters the elevations of the waterline along the current inundation areas were identified using a 10 m DEM (depending on the fluvial morphology with a longitudinal interval of 50–200 m along the water courses). In a next step, we determined whether the terrain adjacent to the waterline was artificially raised for road construction, railway lines or new settlement areas. This procedure also incorporated terrain changes in valley bottoms due to river straightening measures. The “Franziscean cadastre”, the DEM, current orthophotos and additionally Google Earth were used for this purpose. In cases where the data indicated a currently higher terrain than more than 170 years earlier, the potential historical waterline outside of the current inundation area was determined. In most cases the historical land uses shown by the cadastre maps clearly revealed the lateral extents of the former floodplains. At some larger rivers, intensive river straightening measures led to significant incisions of the channel bottoms and, consequently, lowering of the water tables during floods (Hohensinner et al., 2021). If the cadastre maps and the orthophotos clearly showed that the elevation of the former water table must have been higher and the floodplain wider, we also assumed historically larger inundation areas than today. In such cases we matched laterally differing forms of land use, such as the boundaries between pastures/grassland and arable land or narrow strips of pastures or wooded areas that indicate small terrain steps, in the cadastre with the DEM. The extents of the former flooded areas do not allow concrete statements about the associated flood recurrence interval, i.e., whether they corresponded to 100, 300, or 500-year floods under the climatic and hydrological conditions in the first half of the 19th century. One can, however, state that, historically, the reconstructed inundation areas were potentially water covered at floods with similar water levels as today. The applied method merely allows approximating the former extents of the flood-prone areas. It was not feasible to apply flood simulations for all running waters in the entire study area (this would also mean creating an adjusted DEM of the historical river landscapes).
In a last step, the 10 m DEM was intersected with the catchment-wide datasets. This yielded altitudinal distributions for each historical and current class of land cover/use.
Results
Catchment-wide Changes in Alpine Land Cover
Between 1826 and 1859, forests covered the largest area in the Austrian catchments of the rivers Rhine, Salzach and Drava (37%, Figure 2). While the diverse forms of grassland made up 22%, the proportion of arable land was only half of that. Sparsely wooded areas that in most cases were used for pasture feeding encompassed 16% of the entire study area. Accordingly, only one fifth of the entire land used for any kind of agricultural purposes was used for grain and vegetable cultivation. Apparently, many sites in steeper and higher locations were unsuitable as cropland. Except for wasteland, all other investigated types of land cover/use played only a minor role.
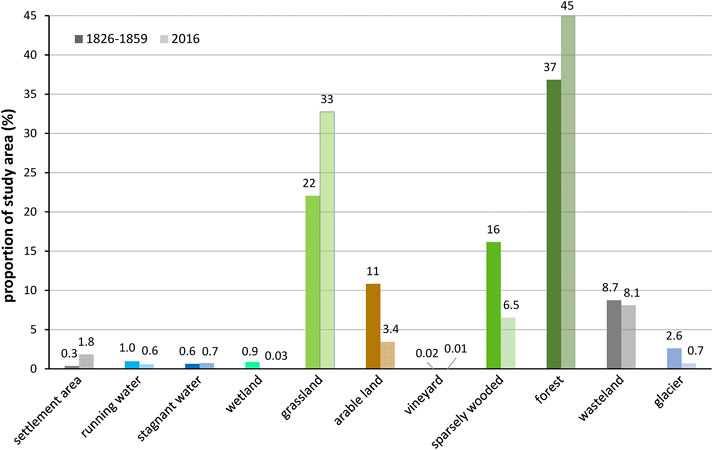
FIGURE 2. Proportions of the land cover/use types in the Austrian catchments of the rivers Rhine, Salzach and Drava (%) (left column: 1826–1859, right: 2016).
Until 2016, a significant expansion of wooded areas (from 37 up to 45% of the study area) was recorded (Figure 2). Grassland also expanded substantially from 22 to 33%, whereas arable land showed a significant decline to merely 3.4% in 2016. A similar decline of sparsely wooded areas, pointing to human transformation to either grassland or forests, was evident. Unsurprisingly, settlement areas expanded tremendously since the first half of the 19th century. Today they show a six-fold extension compared to the historical state. Rivers and smaller streams have been reduced by 40% due to river straightening and channel narrowing. Wetlands, i.e., swamps, moors and reeds, have been almost wiped out. The situation is similar for glaciated areas: they have been severely reduced from 2.6 to 0.7% of the entire study area. The dramatic retreat of the glaciers would lead one to expect a significant increase in wastelands. In 2016, however, they even showed a slightly lower areal proportion than between 1826 and 1859. This means that large areas of former wastelands must have been colonized by vegetation until today.
Figure 3 illustrates the changes identified for the whole study area in detail. Since 1826–1859, the region around Austria’s highest peaks in the Central Alps has undergone a significant transformation. Most obvious is the comprehensive retreat of Austria’s largest glaciers such as the “Pasterze” at the “Großglockner”. In parallel, Alpine grassland and partially sparsely wooded areas (i.e., crooked wood) encroached into higher areas. The valley floor of the Salzach River north of the glaciers was originally characterized by extended swamps and reeds (Figure 3A). By 2016 they have been largely wiped out. This was accompanied by a tremendous expansion of settlement areas (Figure 3B). In addition, new stagnant waters, i.e., reservoirs constructed for energy production after World War II, are now dominant landscape features. Some of them replaced former Alpine wetlands. Moreover, former arable land has transformed to grassland in the Salzach valley.
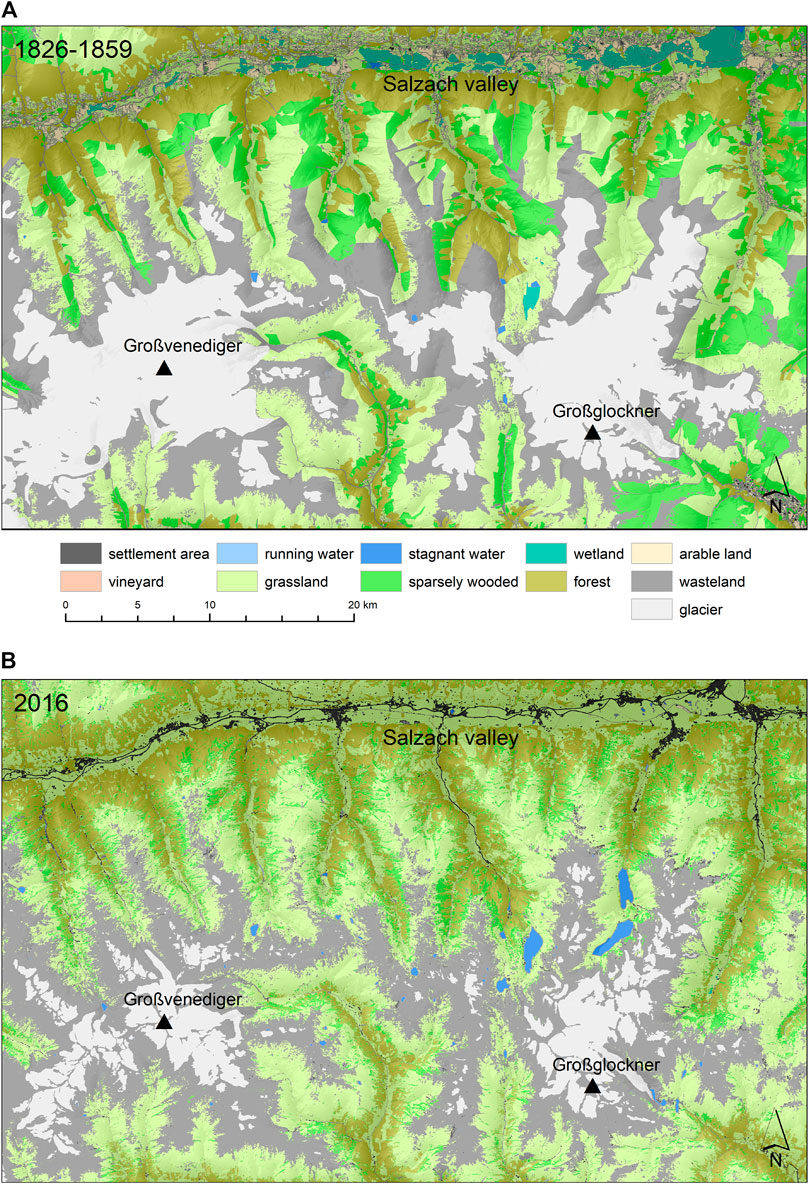
FIGURE 3. (A) Land cover between 1826 and 1859 in the region of Austria’s highest peaks south of the Salzach valley; (B) land cover in 2016 (see location of the detail in Figure 1).
What Figure 3 clearly reveals at the small scale can also be traced for the entire study area. The land cover transformation matrix in Table 2 shows that stagnant waters and forests are the most stable forms of land cover. Alpine wasteland was also comparably stable, but 16% of its former extension were replaced by grassland and by sparsely wooded areas (ca. 7% crooked wood). In contrast, only 27% of the former glaciers still exist today. Two thirds of them have transformed to wasteland and ca. 4% to grassland. Agrarian areas, i.e., grassland and arable land, showed a contrary development. While approximately two thirds of the former still existed in 2016, only 21% of the arable land has remained until today. Most of the former cropland is now used as grassland. On the other hand, almost one quarter of the past grassland is wooded today. From the nature conservation point of view the development of the Alpine wetlands and running waters is of special interest. Swamps, moors and reeds declined drastically: only 1.9% of their former extent still exists today (Table 2). Most of the wetland areas have been transformed to grassland, partially also to arable land or forests. Similarly, but less severely, the area of the historical running waters declined by two thirds. The areas gained by river straightening today host forests, grassland or settlement areas. The historical development of the latter is ambiguous. The analysis reveals that only 47% of the former settlements still exist and that 36% have transformed to grassland. Thus, the values related to settlements should be interpreted with caution because they rather reflect the methodological limits of the compiled datasets than the actual development (see Inaccuracies of the Basic Data and Potential Misinterpretations).
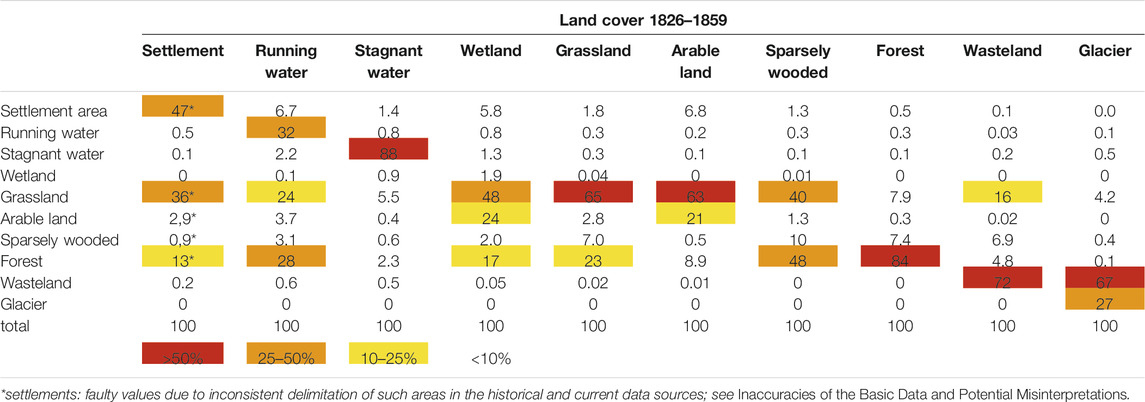
TABLE 2. Matrix of land cover transformation: percent shares of land cover classes 1826–1859 (upper row) that changed to other classes by 2016 (columns). Values along the diagonal from settlement/settlement to glacier/glacier represent unchanged shares. Color cells symbolize different intensities of land cover changes.
Land Cover Changes in Alpine Fluvial Corridors
The modifications of the land cover/use in the fluvial corridors, i.e. in areas that are flood-prone at approximately 300-year floods, reflect the catchment-wide development (Figure 4; Figure 5). Several types of land cover in such areas–of which the vast majority is located in broader valley floors–even showed a more pronounced transformation. Historically, here, as opposed to the entire study area, grassland made up the largest area proportion, followed by arable land (29 and 22%, respectively, Figure 6). Between 1826 and 1859 only 15% of the fluvial corridors were covered by (alluvial) forests. In comparison to stagnant waters, running waters clearly dominated the valley floors with 13%. No wonder, wetlands made up much larger area shares (7.3%) than in the entire study area. Settlements also claimed much higher area shares in the fluvial corridors.
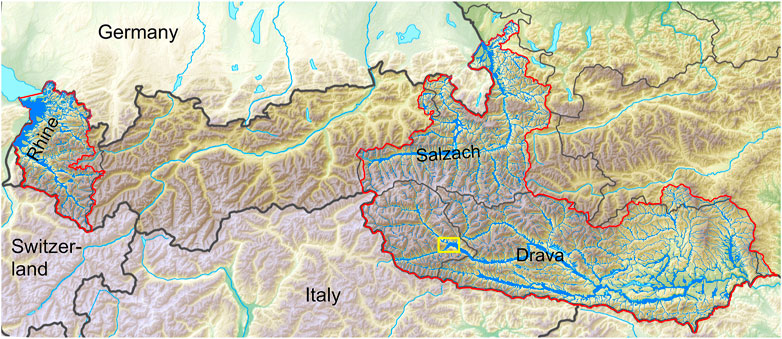
FIGURE 4. Spatial distribution of the fluvial corridors at approximately 300-year floods between 1826 and 1859 (blue) within the study area (red; yellow rectangle: see detail in Figure 5).
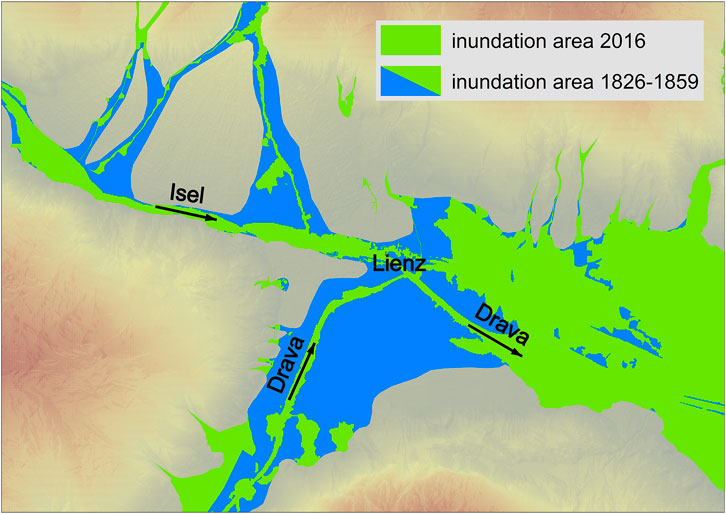
FIGURE 5. Fluvial corridors close to the town Lienz in Eastern Tyrol, where the tributary Isel joins the Drava River. Current inundation areas at approximately 300-year floods (green) are overlaid over historical ones (blue). Torrent regulation significantly reduced flood-prone areas along the tributaries of the Isel River. River regulation and flood protection measures substantially truncated former inundation areas upstream of Lienz (see location of the detail in Figure 4).
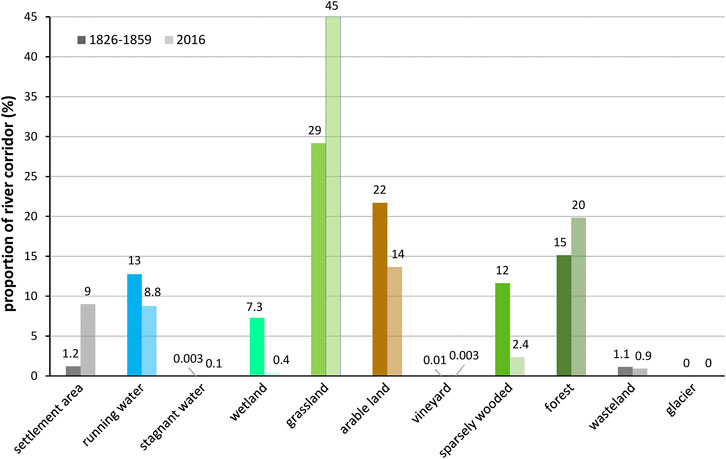
FIGURE 6. Proportions of the land cover/use types in Alpine fluvial corridors (%) (left column: 1826–1859, right: 2016; note that the percent values refer to different large corridor areas in 1826–1859 and 2016).
As illustrated in Figure 3 the development of settlements was closely tied to fluvial corridors. In 2016, they covered 7.5 times larger areas than between 1826 and 1859 (Figure 6). The decline in running waters was less than might be expected given the systematic river regulation programs (Hohensinner et al., 2021). In this respect, the construction of large impoundments at the Drava River in the province Carinthia was mostly responsible for partly compensating the huge areal losses due to river channelization. In contrast, wetlands were severely truncated by 95% of their former extent. Closely related with that major wetland shrinkage, grasslands increased from 29 to 45% of the fluvial corridors. The newly reclaimed areas, however, were partially also used for the cultivation of field crops (compare Table 2). Nevertheless, arable land was also reduced along rivers by one third. Abandonment of agrarian uses in former sparsely wooded areas (fruit tree meadows and partially wooded pastures) as well as in grassland is reflected by the increase in forests from formerly 15 to 20% in 2016.
Beyond the altered composition of the land cover/use types in the fluvial corridors since the 19th century, the overall extents of the potential inundation areas were also affected by human interventions. In 2016, the areas inundated at approximately 300-year floods amounted to 1,247 km2 in the entire study area. This value must be seen as a minimum because no data are available on the associated flood-prone areas for numerous smaller streams (compare GIS and Data Analyses). At flood levels comparable with today’s 300-year flood, the inundation zones were significantly larger in the first half of the 19th century (1,450 km2). Since then they have been reduced by 203 km2 or 14% for land reclamation (i.e., landfills) and flood protection (Figure 5).
Altitudinal Distribution of Land Cover/use Types
The three-dimensional analysis of the individual land cover types reveals additional insights into their spatial organization in the historical vs. current state. Historically, the gradient of increasing altitudes extends from settlement areas as the lowest to glaciers as the highest (Figure 7). Between 1826 and 1859 the median altitude of settlement areas was 599 m a. sl. By 2016 this value significantly decreased to 498 m a. sl. because the inhabited areas primarily expanded in low-lying valley floors (compare Salzach valley in Figure 3B). At the same time, smaller settlements and larger roads advanced into higher Alpine regions. Similarly, arable land was also primarily located in low-lying valley floors. Since the 19th century, the median elevation declined from formerly 698 to 513 m a. sl. in 2016. The boxplot in Figure 7B reveals that arable land is largely concentrated at around 500 m a. sl. today. The next higher class of land cover refers to forests, whose median values increased only slightly from 1,149 to 1,167 m a. sl. Nevertheless, in some locations forests reach higher altitudes today (Figure 7C).
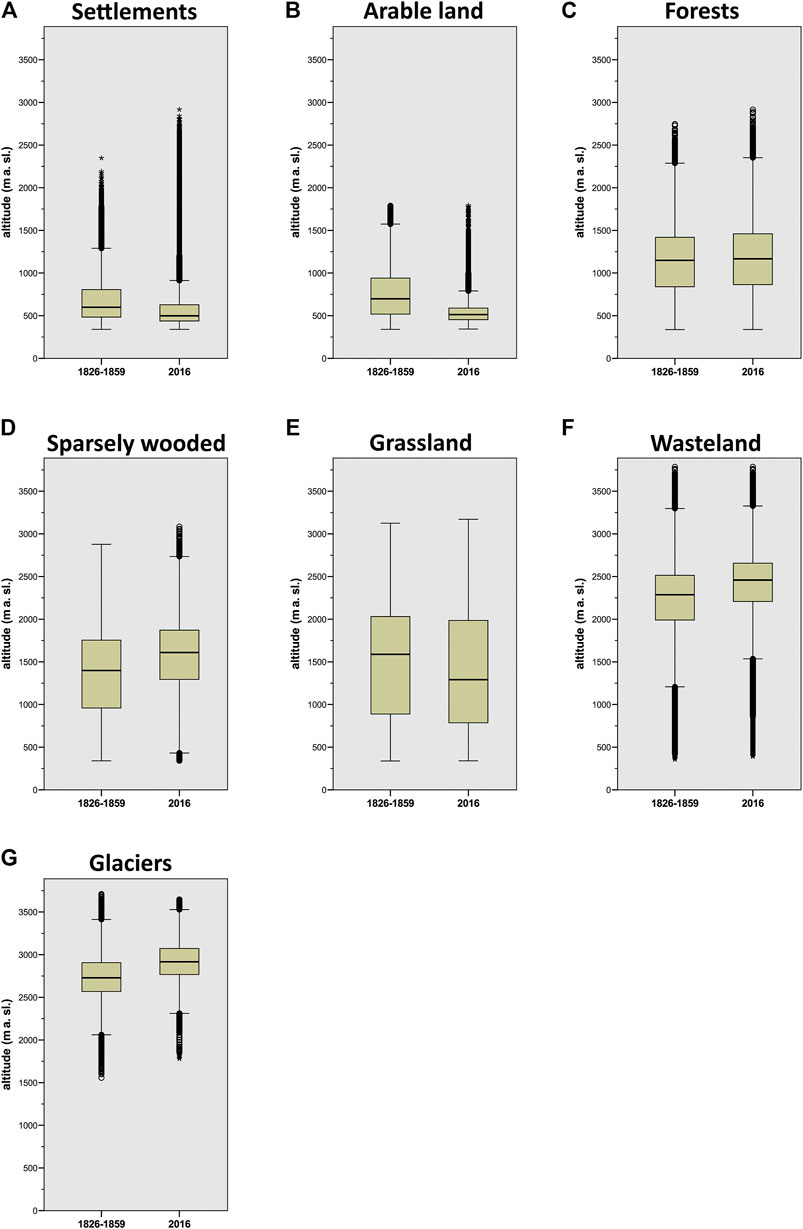
FIGURE 7. Altitudinal distribution of (A) settlement areas, (B) arable land, (C) forests, (D) sparsely wooded areas, (E) grassland, (F) wasteland and (G) glaciers.
Sparsely wooded areas as the next higher type of land cover historically showed a median elevation of 1,399 m a. sl. Today, that value is much higher (1,609 m a. sl.; Figure 7D). Grassland merits a closer look because it showed a contrary development in the past 170 years. Due to numerous high Alpine meadows, grassland originally showed a median altitude of 1,588 m a. sl. (Figure 7E). The comparable current value, 1,291 m a. sl., is significantly lower. These values reflect the abandonment of Alpine meadows in high altitudes and the transformation of arable land to pastures in low-lying valley floors.
Alpine wasteland has undergone a significant vertical shift by 173 m from formerly 2,286 m a. sl. to 2,459 m a. sl. in the early 21st century (Figure 7F). Similarly, glaciers substantially retreated since around 1860. Their median altitudes increased by 187 m from formerly 2,729 to 2,916 m a. sl. (Figure 7G).
Discussion
Inaccuracies of the Basic Data and Potential Misinterpretations
Using historical data sources entails several potential pitfalls which differ from those associated with present data. The map series of the “Franciscean cadastre” was produced by military cartographers working with very elaborate survey instructions (Fuhrmann, 2007). The creation of hundreds or even thousands of sheets of maps necessitated numerous actors who–despite detailed instructions–no doubt deployed different levels of accuracy in their field work. While more intensively used areas in the valleys were mapped very accurately, those in higher altitudes at the transition to the wasteland lack such accuracy. According to Ulbrich (1961), in the cadastre the mapped distances of 100 m showed average errors in length of 1.3 and 4.9 m at distances around 2,000 m. The purpose for the cadastre maps was land taxation. Accordingly, every plot of land was of interest that could be somehow used for agricultural or other economic purposes. Despite the harsh conditions in the transition zone from Alpine meadows to wasteland, even that area was of economic interest, e.g., for keeping goats. In nature, Alpine grassland or shrubs fade out as the terrain gets higher (Fischer et al., 2019). This is reflected by the more “patchy” dataset for the current state, but here the cadastre maps show sharp limits between grassland or sparsely wooded areas at the one side and wasteland at the other. Another difference between the historical cadastre and the current data sources is that the former show only one type of land cover/use per parcel. In contrast, the current data also show smaller patches of deviating types within larger parcels. Consequently, the median size of the GIS polygons referring to the current land cover/use (100 m2) is much smaller than for the historical state (1,100 m2).
These differences in the accuracy of the data sources may lead to incorrect results at the local scale. However, the larger the study area is, the more they level off. Generalizing the more detailed current dataset to make it comparable to the historical one did not solve this issue properly because most types of land cover showed areal gains or losses. Moreover, generalizing the data would mean introducing new methodological uncertainties. In order to estimate the potential error of the reconstructed historical land cover/use types, a separate detail analysis was conducted for the 54.3 km2 large “Jamtal” valley in the province Tyrol (see location in Figure 1; based on Hohensinner et al., submitted). For that valley the historical land cover was reconstructed using the “regressive-iterative” GIS method as described by Hohensinner et al. (2013). Starting from the state in 2015, those in 1970, 1921, 1870, and 1820 were reconstructed going back in time based on numerous historical, topographical and geological sources (Atzler, in prep.). This chronological approach enables a more robust reconstruction compared to such that solely focus on one point in time. Comparing the large-scale dataset for 1826–1959 with the detail analysis of the “Jamtal” in 1820 reveals potential error ranges in the former dataset. Accordingly, settlement areas and arable land are underestimated by 15 and 10.4%, respectively, in the large-scale dataset (percentage deviation in relation to the detailed GIS analysis). The situation is similar for running waters, whose surface area was too low by 10.5%. Grassland was also underestimated by 6.5% and wasteland by 1.9%. The error range of the latter seems surprisingly low, but the historical reconstruction also contains those wasteland areas from the current dataset that probably already existed in the historical state (see Data Sources). In contrast, forests are overestimated by approximately 4%.
Glaciers show no deviation because they were delimited by the same historical source (“Franziscean land survey”). Moreover, they were reconciled with morains from the last glacial expansion in the 19th century, which in most cases are still visible in current orthophotos. The reconstructed sparsely wooded areas proved to be the most problematic type of land cover. In the large-scale dataset of the entire study area, they are overestimated by 48%. Sparsely wooded areas capture an intermediate state between grassland devoid of wood and dense forests. Thus, a clear separation between these three types of land cover is only partially possible. In the case of the “Franziscean cadastre” this separation was already conducted by the surveyors of that time. This is different in the dataset for 2016: here, the distinction between grassland, sparsely vegetated areas and forests is based on remote sensing analysis. Though the satellite data were calibrated by ground truthing, this method can also yield different results (GeoVille Information Systems, 2017). The information derived from the detail analysis in the “Jamtal” suggests that the percentage values shown for historically sparsely wooded areas and partially also forests in Figure 2; Figure 6 are too high. Consequently, the estimated decrease of sparsely wooded areas until 2016 would have been smaller than shown by the figures and the increase of forests would have been greater. In contrast, the values for grassland are too low in the mentioned Figures. Accordingly, the gap between the historical and current grassland extents would be smaller.
The dataset of the land cover types in 2016 also entails potential pitfalls. In settlement areas, it primarily shows buildings but not the smaller gardens and parks that are included in the historical dataset. Moreover, smaller homesteads of up to approximately six buildings are missing in many regions. Accordingly, both the historical and current area values for settlements are too low. These inaccuracies are reflected by the land cover transformation matrix (Table 2). Other than suggested by the settlement area values, it can be assumed that almost 100% of those areas in 1826–1859 have remained until 2016 and that the historical settlement areas have not transformed to grassland or forests to such high extents.
Concentration and Abandonment of Human Uses
The results of the present study show that the Alpine landscape in the last phase of the pre-industrial era, i.e., between 1826 and 1859, can by no means be attributed as “pristine”. Human interventions had already affected most areas in the Alpine sphere (McGrath et al., 2015; Bürgi et al., 2017; Haidvogl et al., 2019). At that time, only wasteland and glaciers were more or less untouched by humans. First river sections had already been straightened and smaller streams were diverted to operate numerous mills. Moreover, some smaller areas in the valley bottoms had been already drained and transformed to meadows, pastures or arable land.
Nevertheless, the era of industrialization in many of the Austrian Alpine valleys started late and was primarily concentrated in certain sections of the larger valleys (Egarter Vigl et al., 2016; Lavorel et al., 2017). The decline of the agrarian society in the 20th century went hand in hand with a comprehensive concentration process of land uses (Bätzing, 2003). This process is reflected by the new data in several dimensions–spatially and altitudinally. Tappeiner et al. (2006) reported that, depending on the region, between 6 and 67% of the areas formerly used for agriculture have been put out of operation since around 1850. Our data reveal that 49% of the study area were used as grassland, cropland, vineyards and sparsely wooded areas (Alpine meadows etc.). By 2016, such land uses were reduced to approximately 43%, equivalent to a decrease by ca. 12%. Taking into account that large parts of the current sparsely wooded areas are in fact crooked wood zones in high altitudes (not or less used for grazing), only approximately 36% of the entire study area are agriculturally used. This represents a reduction by ca. 27%.
In particular, the severe reduction of arable land by 69% of its former extent reflects the all-embracing retreat of intensive forms of land uses to the most favorable zones in the valley floors. In this respect, “favorable” is not only associated with suitable climate and soil conditions, it also includes the topographical aspects for an optimized usage of agricultural machines (Tappeiner et al., 2006). Abandoned grain or vegetable fields have primarily been transformed to grassland (meadows and pastures) for milk production. Some areas have been afforested or used as building land (compare Table 2). The specialization of Alpine agriculture in grassland farming and dairy production was a consequence of (agricultural) industrialization. In pre-industrial subsistence farming, energy and nutrient flows had to be maintained locally, especially to preserve soil fertility. Thereafter, the possibility to bring in energy and nutrients externally, along with the cheap transport of agricultural products, enabled specialization of individual farms (Gingrich et al., 2015; Krausmann, 2016).
The concentration of human land uses is also well reflected by the altitudinal distribution of arable land (Figure 7B). Between 1826 and 1859, 50% of the areas used for the cultivation of field crops were located between 519 and 941 m a. sl., i.e., a vertical range over 422 m. In 2016, 50% of these were located between 454 and 589 m a. sl., a range of only 135 m. This means that, today, arable land largely exists only in the floors of the large valleys. Though the cultivation of wine was of less economic interest in the Austrian Alpine sphere, it is worth mentioning that vineyards have been reduced by two thirds. It is remarkable that wine cultivation was much more frequent just before the end of the “Little Ice Age” than it is today.
From the hydrological point of view, the increasingly forested areas and the expansion of grassland to the detriment of arable land with open soils suggests that the large-scale land cover transformation in the Alpine catchments led to reduced surface runoff and decreasing denudation processes (McCarney-Castle et al., 2011). Reforestation to reduce denudation was part of late 19th century flood protection practices (Hauer et al., 2019). These changes would mitigate flood risks along Alpine rivers and reduce suspended sediments loads (Brath et al., 2006; Blöschl et al., 2018). On the other side, due to major glacier retreat, less precipitation is temporarily retained in form of ice, which would seasonally amplify the floods. Moreover, soil compaction due to the operation of heavy machines on agriculturally used areas potentially increases surface runoff (Birkas, 2008). Although not directly addressed in the current study, it can be assumed that such areas are more compacted today than in the 19th century (Alaoui et al., 2018).
Transformation of Fluvial Corridors
Compared to catchment-wide land cover changes, modifications in the fluvial corridors show more direct effects on the flood regime of rivers. Beyond altered soil infiltration/saturation as a consequence of land use changes, the “hydraulic roughness”, which affects “flowing flood retention” capacity, also plays a role (see Introduction; Teschke and Pasche, 2004). Accordingly, the increasingly forested valley floors slow down the propagation of flood waves and potentially reduce flood peaks. These effects are counteracted by the tremendous expansion of settlement areas close to rivers (Figure 6). While most streets and squares in villages and smaller towns were unpaved in the first half of the 19th century, all are sealed and hinder soil infiltration today. Moreover, elaborate flood protection facilities and land fillings prevent settlements, railways and roads from being inundated. This significantly reduces the “standing flood retention” capacity (storage space for flood water) in river corridors, leading to higher flood levels in not yet flood-protected areas (Haidvogl et al., 2018; Wesemann et al., 2020). In this respect, since 1826–1859 approximately 203 km2 or 14% of the former space originally available for “standing retention” at 300-year floods has been lost (compare Figure 5). This value might appear minimal. However, at such extreme floods most of the flood protection dikes that are commonly constructed for 100-year floods are overflowed. In fact, the inundation areas for 100-year floods have probably undergone a by far more severe truncation.
The geometry of river channels is crucial for the conveyance of floods. In Austria, starting in the early 19th century almost all Alpine rivers have been systematically channelized, whereas before that time, measures were limited to local scales (Hohensinner et al., 2021). These systematic efforts include straightening river courses and significantly narrowing channels. Combined, both measures result in higher velocities of the flood waves and higher flood levels (Wyzga, 1996). Figure 6 shows that the surface area of running waters related to the area of the fluvial corridors has declined by one third. In fact, the areal reduction of rivers and streams was even more severe. This, however, was somewhat counteracted by the later construction of impoundments (mostly along the Drava River, but also along the Salzach River), which laterally extended the formerly channelized rivers. Nonetheless, the flood conveyance area within river channels today is severely reduced compared to the historical state.
Parallel to river channelization, almost all wetlands were drained and converted to grassland, arable land or forests (Figure 6; Table 2). The consequences for floods are twofold because wetlands can either mitigate or amplify floods (Acreman and Holden, 2013). This depends on the type of wetland, the soil saturation status when the flood wave reaches the respective wetland, and wetland location within the river system. This complicates providing a definitive answer to the consequences of draining wetlands on the flood characteristics.
Due to the partly counteracting hydrological processes associated with diverse forms of land use changes, the consequences of such land transformations on the flood regime in Alpine valleys cannot be clearly answered at this stage. In general, such changes primarily affect the flood regime in smaller catchments (up to ca. 100 km2) and at smaller flood magnitudes (Salazar et al., 2012; Viglione et al., 2016). In larger catchments and at higher flood magnitudes, climate change and hydraulic constructions (flood protection, channelization etc.) play a major role. In the study area, 71% of the total length of the running waters are located in catchments up to 100 km2. In contrast, the vast majority of settlement areas is located in larger valleys, i.e., in catchments >100 km2 (compare Figure 3B; Figure 7A). In those valley sections, river straightening and human reclamation of former channel areas were significantly more rigorous and probably affected flooding processes more intensively than upstream land cover changes (Hohensinner et al., 2021). More profound insights will require applying a spatially distributed rainfall-runoff model. The here presented data will provide the basis for that effort.
Vertical Separation of Land Cover/uses
The analysis of the altitudinal ranges related to certain types of land cover/use shows two forms of alterations. Some types–settlements, arable land and grassland–experienced a more or less significant reduction in altitude (see Figure 7). As noted above, this reflects the concentration of certain land uses in larger and lower-lying valley floors. In respect of Alpine flood risk, the location of settlement areas within the river systems plays an important role. Figure 8 illustrates that populated places have not only markedly expanded since 1826–1859, they are now also concentrated at lower altitudes. This evidence supplements the two-dimensional analysis discussed in Transformation of Fluvial Corridors. Despite that, however, smaller settlements and larger roads reach higher altitudes today than in the 19th century (Figure 7A). Human infrastructure in high Alpine ranges is generally prone to multiple natural hazards (Glade et al., 2020).
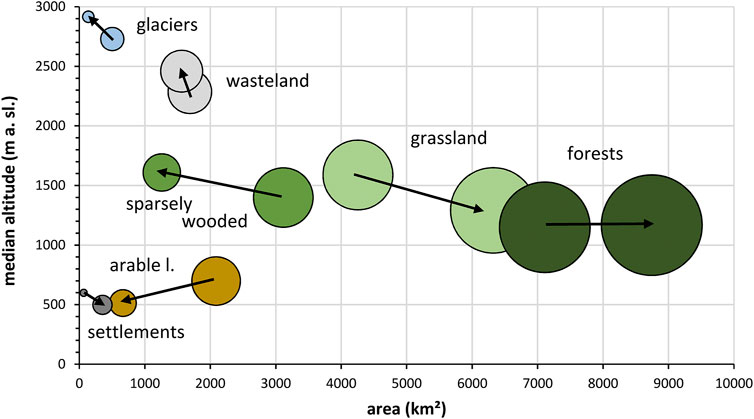
FIGURE 8. Altitudinal shifts (Y-axis) and changes in areal extents (X-axis) of selected types of land cover/use between 1826–1859 and 2016. Altitudes refer to median values; sphere size reflects relative changes in areal extents (arrows: development from 1826–1859 to 2016).
Sparsely wooded areas, wasteland and glaciers, in contrast, clearly show increasing altitudes (Figure 8). Because large surfaces of the sparsely wooded areas are formed by crooked wood at the transition between vegetated zones and the wasteland in high Alpine ranges, the significant altitudinal increase suggests a relationship to higher temperature due to global warming (Theurillat and Guisan, 2001). According to Kilian et al. (1994), the forest line, i.e., the transition from closed canopy forest to the crooked wood zone is located approximately at an altitude of 1,800 m a. sl. in the study area. Thus, a separate 3D GIS analysis of the sparsely wooded areas in that zone should reveal a potential shift toward higher altitudes. This, however, is not the case (median uplift = 2 m). Although locally some crooked wood has emerged in higher ranges, the significant increase of the median value shown in Figure 7D is primarily caused by the transformation of formerly sparsely wooded areas in grassland or forests in lower-lying areas (compare Table 2). Hence, this statistical effect is mainly attributed to abandonment and afforestation of former partially wooded pastures or to clearings and transformation to pure grassland.
Similar conclusions that land cover changes, i.e., abandonment of pastures, were the main driver for upward shifts of the forest or tree line are reported by Goudie and Viles, 2016 for the Swiss Alps. According to Ellenberg (1996) the forest and tree lines are anthropogenically lowered due to century or even millennia-long usage as Alpine pastures. In areas where such human practices were abandoned, the forest expanded upwards. This process occurs independent of global warming. Nevertheless, an upward shift of woody and herbaceous vegetation as well as grasses can be generally hypothesized as a consequence of climate change (Dullinger et al., 2004).
Separate 3D analyses for grassland, sparsely wooded areas and forests above 1,800 or 2,100 (ca. tree line) show ambiguous developments. Either they reflect land cover changes within the analyzed altitudinal belts, or they show a bias due to the differently structured datasets. While the dataset for 1826–1859 shows larger homogeneous parcels with the same type of land cover, that for 2016 is significantly more patchy (see Inaccuracies of the Basic Data and Potential Misinterpretations; compare Figures 3A,B). In order to eliminate the effects of patchiness, we merged forests, sparsely wooded areas and grassland into larger zones of vegetation of all kinds. Filtering out the upper vegetation boundary of that zones yields more robust results. Accordingly, this boundary has shifted upwards by a median 41 m in altitude between 1826–1859 and 2016. A separate analysis within a spatial buffer of 1,500 m around the historical glacier extents reveals a 56 m median upward shift of the high Alpine grassland. These values are rather low compared to other studies. Leonelli et al. (2011) reported a tree line shift in the Italian Alps by 115 m between 1900 and 2000. According to Walther et al. (2005), within one century, several species of vascular plants shifted upwards on average by 278 m in the Swiss Alps. In comparison, the more accurately (temporally stepwise) reconstructed land cover in the detail study site “Jamtal” yields an upper vegetation limit shift between 243 m (average related to the entire valley) and 460 m (in formerly glaciated sites) since the last glaciation maximum in 1864/70 (Hohensinner et al., submitted). We assume that the basic data we used for our large-scale study are too inaccurate in the high Alpine regions in order to provide a robust value in this respect. Nevertheless, our findings on these shifts are also reflected by our areal analysis showing that 16% of the former wasteland now hosts grassland. Moreover, 4.2% of formerly glaciated areas also feature grass cover today (Table 2).
Conclusion
The reconstruction of historical land cover/uses to a level nearly representing exact plots of land in large areas of the Austrian Alps enables more accurate, two- and three-dimensional analyses of long-term changes. Human modifications in the Alpine fluvial corridors differed from the catchment-wide changes. Altitudinal shifts of land cover/uses between 1826–1859 and 2016 revealed new insights into the three-dimensional organization of certain forms of land uses. Based on the compiled datasets, the main findings of the study, with a special focus on Alpine flood risks, are:
(1) Since the onset of industrialization, the catchments of the rivers Rhine, Salzach and Drava in Austria have undergone a comprehensive transformation. In 2016, settlements made up six times larger areas than 170 years ago. Land used for various agricultural purposes declined by approximately 27%, whereby arable land was reduced by even 69%. Abandonment of agrarian land in unfavorable sites is reflected by an increase of forests from 37 to 45% over the entire study area. Glaciers lost approximately 73% of their former extents.
(2) The Alpine fluvial corridors, i.e., flood-prone areas at ca. 300-year floods, more directly reflect the concentration of human land uses compared to the catchment-wide perspective. Here, settlements expanded 7.5-fold, severely increasing the potential for flood damage. In contrast, the overall channel area of running waters has been reduced by 40% and 95% the formerly large wetlands have been transformed to agricultural land, forests or settlement areas. By 2016, approximately 203 km2 or 14% of the former fluvial corridors had been lost, thereby reducing flood retention capacity. Retention areas inundated at 100-year floods probably show significantly greater areal losses.
(3) The concentration of intensive forms of human land uses at lower altitudes on the one hand, and upwards shifting of less intensively used, near-natural forms of land cover on the other hand led to both a spatial and vertical separation of Alpine landscape features over the long term. In particular, settlement areas and arable land are now concentrated in lower altitudes than in the 19th century. Warmer temperatures due to climate change may foster the already ascertained upward shifts of Alpine vegetation.
The results of this study highlight the generally increasing flood risks in Alpine valley floors. Building land located in “residual risk zones” that are inundated between 100-year floods (for which in most cases flood protection facilities already exist) and 300-year floods are particularly vulnerable. In case protection facilities fail, the increasing concentration of settlements and infrastructure in such zones significantly amplifies the damage potential (so-called “levee effect”; Di Baldassarre et al., 2013; Cutteret al., 2018). This calls for advanced flood risk management and adapted policies in spatial planning (Löschner et al., 2017).
Human interventions in the Alpine sphere are reflected by land cover changes that either amplify flood risks (expansion of settlements, reclamation of former channel areas, etc.) or mitigate flood risks (abandonment of agrarian sites, afforestation, etc.). Moreover, the influence of land cover changes on the flood regime is generally greater in smaller, upstream catchments than in larger, lower-lying valleys where most larger settlements are located. Here, climate change, flood protection facilities and river straightening more intensively affect the flood risk (Viglione et al., 2016; Blöschl, 2020). In order to more definitively determine the role of land cover changes for Alpine flood risks, the next step will involve using the presented data for further analysis in spatial rainfall-runoff models.
Data Availability Statement
The datasets presented in this article are not readily available because data are partly subject to third party restrictions. Selected data are available on request. Requests to access the datasets should be directed to severin.hohensinner@boku.ac.at.
Author Contributions
SH: conceptualization, methodology, investigation, validation, writing, project administration; UA: investigation; MB: investigation; TB: investigation; CH: investigation; MK: investigation; LR: investigation; YS: investigation; GH: writing, review.
Funding
This work was funded by the Austrian Academy of Sciences (ÖAW) within the Earth System Sciences (ESS) research program, i.e., the projects “Integrated Flood Risk Management in Mountain Areas: Assessing Sectoral Interdependencies, Conflicts and Options for Policy Coordination” (POCO-FLOOD) and “Changing debris cover on Eastern Alpine glaciers: quantification and hydrological impacts” (Hidden.Ice). Open access funding was provided by the University of Natural Resources and Life Sciences Vienna (BOKU).
Conflict of Interest
The authors declare that the research was conducted in the absence of any commercial or financial relationships that could be construed as a potential conflict of interest.
Footnotes
1Hohensinner, S., Atzler, U., Fischer, A., Schwaizer, G., and Helfricht, K. (submitted). Tracing the Long-Term Evolution of Land Cover along Glacier Streams: The Austrian Jamtal valley (1820–2015). Frontiers in Environmental Science.
References
Acreman, M., and Holden, J. (2013). How Wetlands Affect Floods. Wetlands 33, 773–786. doi:10.1007/s13157-013-0473-2
Alaoui, A., Rogger, M., Peth, S., and Blöschl, G. (2018). Does Soil Compaction Increase Floods? A Review. J. Hydrol. 557, 631–642. doi:10.1016/j.jhydrol.2017.12.052
Andréassian, V. (2004). Waters and Forests: from Historical Controversy to Scientific Debate. J. Hydrol. 291, 1–27. doi:10.1016/j.jhydrol.2003.12.015
Andric, M., Martincic, A., Stular, B., Petek, F., and Goslar, T. (2010). Land-use Changes in the Alps (Slovenia) in the Fifteenth, Nineteenth and Twentieth Centuries AD: A Comparative Study of the Pollen Record and Historical Data. The Holocene 20 (7), 1023–1037. doi:10.1177/0959683610369506
Arnold, C., Wilson, E., Hurd, J., and Civco, D. (2020). 30 Years of Land Cover Change in Connecticut, USA: A Case Study of Long-Term Research, Dissemination of Results, and Their Use in Land Use Planning and Natural Resource Conservation. Land 9 (8), 255. doi:10.3390/land9080255
Asch, K. (2003). “The 1: 5 Million International Geological Map of Europe and Adjacent Areas: Development and Implementation of a GIS-Enabled Concept,” in Geologisches Jahrbuch, SA (Stuttgart: E. Schweizerbart´sche Verlagsbuchhandlung).
Atzler, U. (in prep.). Historical Changes in Land Cover and Fluvial Morphology in the Jamtal (Tyrol). Master Thesis. Vienna: University of Natural Resources and Life Sciences.
Bätzing, W. (2003). Die Alpen. Geschichte und Zukunft einer europäischen Kulturlandschaft. Munich: Ch. Beck.
Berger, M. (in prep). Land Cover in the Catchment of the Lower Drava River (Carinthia). Master Thesis. Vienna, Austria: University of Natural Resources and Life Sciences Vienna.
Blöschl, G., Ardoin-Bardin, S., Bonell, M., Dorninger, M., Goodrich, D., Gutknecht, D., et al. (2007). At what Scales Do Climate Variability and Land Cover Change Impact on Flooding and Low Flows? Hydrol. Process. 21, 1241–1247. doi:10.1002/hyp.6669
Blöschl, G. (2020). “Hochwässer,” in ExtremA 2019. Aktueller Wissenstand zu Extremereignissen alpiner Naturgefahren in Österreich. Editors T. Glade, M. Mergili, and K. Sattler (Vienna: Vienna University Press), 229–246.
Blöschl, G., Komma, J., Nester, T., Rogger, M., Salinas, J., and Viglione, A. (2018). Die Wirkung des Waldes auf Hochwässer. Wildbach- und Lawinenverbau 88 (181), 288–296.
BMLFUW and BAB (2017). INVEKOS-Datenpool 2017 des BMLFUW. Vienna. https://www.bmlrt.gv.at/land/direktzahlungen/Invekos.html (Accessed Nov 11, 2020).
BMLFUW (2014). Hochwasserrisikozonierung Austria – HORA. Report. Vienna: BMLFUW. https://hora.gv.at/(Accessed Nov 11, 2020).
Bozzetta, T. (in prep.). Land Cover Change in the Catchment of the Rhine River (Vorarlberg). Master Thesis. Vienna, Austria: University of Natural Resources and Life Sciences Vienna.
Brath, A., Montanari, A., and Moretti, G. (2006). Assessing the Effect on Flood Frequency of Land Use Change via Hydrological Simulation (With Uncertainty). J. Hydrol. 324, 141–153. doi:10.1016/j.jhydrol.2005.10.001
Bronstert, A., Niehoff, D., and Bürger, G. (2002). Effects of Climate and Land-Use Change on Storm Runoff Generation: Present Knowledge and Modelling Capabilities. Hydrol. Process. 16, 509–529. doi:10.1002/hyp.326
Buckel, J., and Otto, J.-C. (2018). “The Austrian Glacier Inventory Gl 4 (2015),” in ArcGIS (Shapefile) Format (Bremen-Bremerhaven, Germany: PANGAEA). doi:10.1594/PANGAEA.887415
Bürgi, M., Östlund, L., and Mladenoff, D. J. (2017). Legacy Effects of Human Land Use: Ecosystems as Time-Lagged Systems. Ecosystems 20, 94–103. doi:10.1007/s10021-016-0051-6
Crooks, S., and Davies, H. (2001). Assessment of Land Use Change in the Thames Catchment and its Effect on the Flood Regime of the River. Phys. Chem. Earth, B 26 (7-8), 583–591. doi:10.1016/s1464-1909(01)00053-3
Cutter, S. L., Emrich, C. T., Gall, M., and Reeves, R. (2018). Flash Flood Risk and the Paradox of Urban Development. Nat. Hazards Rev. 19 (1), 05017005. doi:10.1061/(ASCE)NH.1527-6996.0000268
Di Baldassarre, G., Kooy, M., Kemerink, J. S., and Brandimarte, L. (2013). Towards Understanding the Dynamic Behaviour of Floodplains as Human-Water Systems. Hydrol. Earth Syst. Sci. 17, 3235–3244. doi:10.5194/hess-17-3235-2013
Dullinger, S., Dirnböck, T., and Grabherr, G. (2004). Modelling Climate Change-Driven Treeline Shifts: Relative Effects of Temperature Increase, Dispersal and Invasibility. J. Ecol. 92, 241–252. doi:10.1111/j.0022-0477.2004.00872.x
Dullinger, S., Dirnböck, T., and Grabherr, G. (2003). Patterns of Shrub Invasion into High Mountain Grasslands of the Northern Calcareous Alps, Austria. Arctic, Antarctic, Alpine Res. 35, 434–441. doi:10.1657/1523-0430(2003)035[0434:posiih]2.0.co;2
Egarter Vigl, L., Schirpke, U., Tasser, E., and Tappeiner, U. (2016). Linking Long-Term Landscape Dynamics to the Multiple Interactions Among Ecosystem Services in the European Alps. Landscape Ecol. 31, 1903–1918. doi:10.1007/s10980-016-0389-3
Ellenberg, H. (1996). Vegetation Mitteleuropas mit den Alpen in ökologischer, dynamischer und historischer Sicht. 5th edition. Ulmer, Stuttgart.
Feranec, J., Šúri, M., Ot'ahel', J., Cebecauer, T., KolárWaszmuth, J., Kolář, T., et al. (2000). Inventory of Major Landscape Changes in the Czech Republic, Hungary, Romania and Slovak Republic 1970s - 1990s. Int. J. Appl. Earth Observation Geoinformation 2 (2), 129–139. doi:10.1016/s0303-2434(00)85006-0
Fischer, A., Fickert, T., Schwaizer, G., Patzelt, G., and Groß, G. (2019). Vegetation Dynamics in Alpine Glacier Forelands Tackled from Space. Sci. Rep. 9 (1), 13918. doi:10.1038/s41598-019-50273-2
Fuhrmann, S. (2007). Digitale historische Geobasisdaten im Bundesamt für Eich- und Vermessungswesen (BEV) – Die Urmappe des Franziszeischen Katasters. Österreichische Z. für Vermessung Geoinformation 1, 24–35.
GeoVille Information Systems (2017). Cadasterenv – Final Report (TD-9) – Public Version I 1.0. https://www.landinformationsystem.at/#/lisa/overview (Accessed Nov 11, 2020).
Gingrich, S., Haidvogl, G., Krausmann, F., Preis, S., and Garcia-Ruiz, R. (2015). Providing Food while Sustaining Soil Fertility in Two Pre-industrial Alpine Agroecosystems. Hum. Ecol. 43, 395–410. doi:10.1007/s10745-015-9754-0
Glade, T., Mergili, M., and Sattler, K. (2020). ExtremA 2019. Aktueller Wissenstand zu Extremereignissen alpiner Naturgefahren in Österreich. Vienna: Vienna University Press.
Goudie, A. S., and Viles, H. A. (2016). “Holocene Floodplain Sedimentation Related to Accelerated Erosion,” in Geomorphology in the Anthropocene. Editors A. S. Goudie, and H. A. Viles (Cambridge: Cambridge University Press), 155–161.
Green, K., and Alila, Y. (2012). A Paradigm Shift in Understanding and Quantifying the Effects of forest Harvesting on Floods in Snow Environments. No: AGU Release, 12–43.
Haidvogl, G., Eberstaller, J., Eberstaller-Fleischanderl, D., Fraiß, B., Gabriel, H., and Hohensinner, S. (2018). Historic Land Use and Settlement Development in River Floodplains and Flood protection: the Example of Traisen and St. Pölten 1870-2000. Österr Wasser- und Abfallw 70, 305–315. doi:10.1007/s00506-018-0473-9
Haidvogl, G., Pont, D., and Zwitter, Z. (2019). “The History of Human Use and Interference – Alpine Rivers as Resource and Risk Factor,” in Rivers of the Alps – Diversity in Nature and Culture. Editors S. Muhar, A. Muhar, G. Egger, and D. Sigrist (Bern: Haupt Verlag), 36–45.
Hall, J., Arheimer, B., Borga, M., Brázdil, R., Claps, P., Kiss, A., et al. (2014). Understanding Flood Regime Changes in Europe: a State-Of-The-Art Assessment. Hydrol. Earth Syst. Sci. 18, 2735–2772. doi:10.5194/hess-18-2735-2014
Harvey, F., Kaim, D., and Gajda, A. (2014). Analysis of Historical Change Using Cadastral Materials in the Carpathian Foothills. Eur. J. Geogr. 5 (3), 6–21.
Hauer, C., Wagner, B., Schober, B., Haun, S., Noack, M., Haidvogl, G., et al. (2019). “Floods and Flood Protection. Past Events and Future Strategies,” in Rivers of the Alps – Diversity in Nature and Culture. Editors S. Muhar, A. Muhar, G. Egger, and D. Sigrist (Bern: Haupt Verlag), 238–247.
Höberth, C. (in prep.). Land Cover Change in the Catchment of the Upper Drava River (Carinthia). Master Thesis. Vienna, Austria: University of Natural Resources and Life Sciences Vienna.
Hohensinner, S., Egger, G., Muhar, S., Vaudor, L., and Piégay, H. (2021). What Remains Today of Pre‐industrial Alpine Rivers? Census of Historical and Current Channel Patterns in the Alps. River Res. Applic 37, 128–149. doi:10.1002/rra.3751
Hohensinner, S., Sonnlechner, C., Schmid, M., and Winiwarter, V. (2013). Two Steps Back, One Step Forward: Reconstructing the Dynamic Danube Riverscape under Human Influence in Vienna. Water Hist. 5 (2), 121–143. doi:10.1007/s12685-013-0076-0
Horn, R. P., and Richards, K. S. (2006). “Modelling Hydraulics in Restored Floodplain Environments,” in Hydroecology and Ecohydrology: Past, Present and Future. Editors P. Wood, D. M. Hannah, and J. P. Sadler (Chichester: Wiley).
Kaplan, J. O., Krumhardt, K. M., and Zimmermann, N. (2009). The Prehistoric and Preindustrial Deforestation of Europe. Quat. Sci. Rev. 28, 3016–3034. doi:10.1016/j.quascirev.2009.09.028
Kilian, W., Müller, F., and Starlinger, F. (1994). Die forstlichen Wuchsgebiete Österreichs. Eine Naturraumgliederung nach waldökologischen Gesichtspunkten. Vienna: Forstliche Bundesversuchsanstalt.
k.k. Landesregierung im Erzherzogtum Österreich unter der Enns (1824). Instruction zur Ausführung der zum Behufe des allgemeinen Catasters in Folge des 8ten und 9ten Paragraphes des Allerhöchsten Patents vom 23. December 1817 angeordneten Landes=Vermessung. Vienna: k.k. Hof- und Staats-Aerarial-Druckerey.
Klein Goldewijk, K., Beusen, A., Doelman, J., and Stehfest, E. (2017). Anthropogenic Land Use Estimates for the Holocene - HYDE 3.2. Earth Syst. Sci. Data 9, 927–953. doi:10.5194/essd-9-927-2017
Kofler, M. (2021). Land Cover Change in the Catchment of the Drava River (Eastern Tyrol). Vienna, Austria: University of Natural Resources and Life Sciences Vienna. Master thesis.
Krausmann, F. (2016). “From Energy Source to Sink: Transformations of Austrian Agriculture,” in Social Ecology. Society-Nature Relations across Time and Space. Human-Environment Interactions 5. Editors H. Haberl, M. Fischer-Kowalski, F. Krausmann, and V. Winiwarter (Cham: Springer International Publishing), 433–445. doi:10.1007/978-3-319-33326-7_21
Krausmann, F. (2008). Land Use and Socio-Economic Metabolism in Pre-industrial Agricultural Systems: Four Nineteenth-century Austrian Villages in Comparison. Vienna, Austria: Social Ecology Working Paper, 72.
Lavorel, S., Grigulis, K., Leitinger, G., Kohler, M., Schirpke, U., and Tappeiner, U. (2017). Historical Trajectories in Land Use Pattern and Grassland Ecosystem Services in Two European alpine Landscapes. Reg. Environ. Change 17, 1–14. doi:10.1007/s10113-017-1207-4
Leonelli, G., Pelfini, M., Morra di Cella, U., and Garavaglia, V. (2011). Climate Warming and the Recent Treeline Shift in the European Alps: The Role of Geomorphological Factors in High-Altitude Sites. Ambio 40, 264–273. doi:10.1007/s13280-010-0096-2
Löschner, L., Herrnegger, M., Apperl, B., Senoner, T., Seher, W., and Nachtnebel, H. P. (2017). Flood Risk, Climate Change and Settlement Development: a Micro-scale Assessment of Austrian Municipalities. Reg. Environ. Change 17, 311–322. doi:10.1007/s10113-016-1009-0
MacDonald, D., Crabtree, J. R., Wiesinger, G., Dax, T., Stamou, N., Fleury, P., et al. (2000). Agricultural Abandonment in Mountain Areas of Europe: Environmental Consequences and Policy Response. J. Environ. Manage. 59, 47–69. doi:10.1006/jema.1999.0335
McCarney-Castle, K., Voulgaris, G., Kettner, A. J., and Giosan, L. (2011). Simulating Fluvial Fluxes in the Danube Watershed: The 'Little Ice Age' versus Modern Day. The Holocene 22 (1), 91–105. doi:10.1177/0959683611409778
McGrath, M. J., Luyssaert, S., Meyfroidt, P., Kaplan, J. O., Bürgi, M., Chen, Y., et al. (2015). Reconstructing European forest Management from 1600 to 2010. Biogeosciences 12 (14), 4291–4316. doi:10.5194/bg-12-4291-2015
McVicar, T. R., and Körner, C. (2013). On the Use of Elevation, Altitude, and Height in the Ecological and Climatological Literature. Oecologia 171 (2), 335–337. doi:10.1007/s00442-012-2416-7
Muhar, S., Grüner, B., Böck, K., Scheikl, S., and Becsi, R. (2018). “SPARE Synthesis Report: "Balancing River protection Needs, Ecosystem Functions and Human Uses,” in European Regional Development Fund (ERDF) – Interreg Alpine Space (Vienna: University of Natural Resources and Life Sciences).
Muhar, S., Seliger, C., Schinegger, R., Scheikl, S., Brändle, J., Hayes, D. S., et al. (2019). “Status and Protection of Rivers: A Pan-Alpine Review,” in Rivers of the Alps – Diversity in Nature and Culture. Editors S. Muhar, A. Muhar, G. Egger, and D. Sigrist (Bern: Haupt Verlag), 302–319.
Overland, H., and Kleeberg, H.-B. (1991). Influence of Land Use Change on Discharge and Sediment Transport of Floods. Oxfordshire: IAHS Publication, 203.
Pasche, E., and Ploeger, W. (2004). “Retention Effect by Natural Rivers with Riparian forest,” in Hydroinformatics. Editors S.-Y. Liong, K.-K. Phoon, and V. Babovic (Singapore: World Scientific Publishing), 399–406. doi:10.1142/9789812702838_0049
Pfister, C., and Brändli, D. (1999). “Rodungen im Gebirge – Überschwemmungen im Vorland: Ein Deutungsmuster macht Karriere,” in Naturbilder. Wahrnehmungen von Natur und Umwelt in der Geschichte. Editors R. P. Sieferle, and H. Breuninger (Frankfurt/Main, New York: Campus Verlag), 297–323.
Pongratz, J., Reick, C., Raddatz, T., and Claussen, M. (2008). A Reconstruction of Global Agricultural Areas and Land Cover for the Last Millennium. Glob. Biogeochem. Cycles 22, a–n. doi:10.1029/2007gb003153
Ramm, F. (2019). OpenStreetMap Data in Layered GIS Format. Technical report. doi:10.4324/9780429284588https://download.geofabrik.de/europe/austria.html (Accessed Nov 11, 2020).
Rapottnig, L. (2021). Land Cover Change in the Catchment of the Middle Salzach River (Salzburg). Master Thesis. Vienna, Austria: University of Natural Resources and Life Sciences Vienna.
Rogger, M., Agnoletti, M., Alaoui, A., Bathurst, J. C., Bodner, G., Borga, M., et al. (2017). Land Use Change Impacts on Floods at the Catchment Scale: Challenges and Opportunities for Future Research. Water Resour. Res. 53, 5209–5219. doi:10.1002/2017wr020723
Rutherford, G. N., Bebi, P., Edwards, P. J., and Zimmermann, N. E. (2008). Assessing Land-Use Statistics to Model Land Cover Change in a Mountainous Landscape in the European Alps. Ecol. Model. 212, 460–471. doi:10.1016/j.ecolmodel.2007.10.050
Salazar, S., Francés, F., Komma, J., Blume, T., Francke, T., Bronstert, A., et al. (2012). A Comparative Analysis of the Effectiveness of Flood Management Measures Based on the Concept of "retaining Water in the Landscape" in Different European Hydro-Climatic Regions. Nat. Hazards Earth Syst. Sci. 12, 3287–3306. doi:10.5194/nhess-12-3287-2012
Schinegger, R., Trautwein, C., Melcher, A., and Schmutz, S. (2012). Multiple Human Pressures and Their Spatial Patterns in E Uropean Running Waters. Water Environ. J. 26, 261–273. doi:10.1111/j.1747-6593.2011.00285.x
Skalos, J., Weber, M., Lipský, Z., Trpáková, I., Santrucková, M., Uhlírová, L., et al. (2011). Using old military survey maps and orthophotograph maps to analyse long-term land cover changes-Case study (Czech Republic). Appl. Geogr. 31, 426–438. doi:10.1016/j.apgeog.2010.10.004
Sterle, Y. (in prep.). Changes in Land Cover and Land Use in the Upper Salzach Catchment (Salzburg/Tyrol) between 1830 and 2016. Vienna, Austria: University of Natural Resources and Life Sciences Vienna. Master thesis.
Tappeiner, U., Tasser, E., Leitinger, E., and Tappeiner, G. (2006). “Landnutzung in den Alpen: historische Entwicklung und zukünftige Szenarien,” in Alpine space – man & environment: Die Alpen im Jahr 2020 (Innsbruck: Innsbruck University Press), 23–39.
Tasser, E. (2011). “Die Zukunft des Alpenraums: Landnutzung, Raumentwicklung, Demographie,” in Proceedings of the 17 (Raumberg-Gumpenstein, Austria: Österreichische Jägertagung 2011), 1–8.
Tasser, E., Ruffini, F. V., and Tappeiner, U. (2009). An Integrative Approach for Analysing Landscape Dynamics in Diverse Cultivated and Natural Mountain Areas. Landscape Ecol. 24, 611–628. doi:10.1007/s10980-009-9337-9
Teschke, U., and Pasche, E. (2004). “A New Approach for One-Dimensional Unsteady Flow Simulation in Natural Rivers with Flood plains and Vegetation,” in Hydroinformatics. Editors S.-Y. Liong, K.-K. Phoon, and V. Babovic (Singapore: World Scientific Publishing), 332–339. doi:10.1142/9789812702838_0041
Theurillat, J.-P., and Guisan, A. (2001). Potential Impact of Climate Change on Vegetation in the European Alps: a Review. Clim. Change 50, 77–109. doi:10.1023/a:1010632015572
Ulbrich, K. (1961). Genauigkeit der ersten Meßtisch-Katastralvermessung in Österreich. Österreichische Z. für Vermessungswesen 49 (2), 44–53.
Viglione, A., Merz, B., Viet Dung, N., Parajka, J., Nester, T., and Blöschl, G. (2016). Attribution of Regional Flood Changes Based on Scaling Fingerprints. Water Resour. Res. 52, 5322–5340. doi:10.1002/2016wr019036
Walther, G. R., Beißner, S., and Burga, C. A. (2005). Trends in the Upward Shift of alpine Plants. J. Vegetation Sci. 16, 541–548. doi:10.1111/j.1654-1103.2005.tb02394.x
Wesemann, J., Eder, M., Habersack, H., Hogl, K., Löschner, L., Nordbeck, R., et al. (2020). Regional Development of Flood Risk under Consideration of Future Development. Hydrologie und Wasserbewirtschaftung 64 (3), 110–126. doi:10.5675/HyWa_2020.3_1
Wex, G. (1873). Über die Wasserabnahme in den Quellen, Flüssen und Strömen, bei gleichzeitiger Steigerung der Hochwässer in den Cultur-Ländern. Vienna, Jg: Zeitschrift des österreichischen Ingenieur- und Architekten-Vereines, 101–119.
Wyzga, B. (1996). Changes in the Magnitude and Transformation of Flood Waves Subsequent to the Channelization of the Raba River, Polish Carpathians. Earth Surf. Process. Landforms 21 (8), 749–763.
Keywords: land cover change, land use, historical GIS, flood risk, Alpine rivers, altitudinal land use shift
Citation: Hohensinner S, Atzler U, Berger M, Bozzetta T, Höberth C, Kofler M, Rapottnig L, Sterle Y and Haidvogl G (2021) Land Use and Cover Change in the Industrial Era: A Spatial Analysis of Alpine River Catchments and Fluvial Corridors. Front. Environ. Sci. 9:647247. doi: 10.3389/fenvs.2021.647247
Received: 29 December 2020; Accepted: 03 May 2021;
Published: 02 June 2021.
Edited by:
Ioan Cristian Ioja, University of Bucharest, RomaniaReviewed by:
Hana Skokanova, Silva Tarouca Research Institute for Landscape and Ornamental Gardening, CzechiaGavrilidis Athanasios-Alexandru, University of Bucharest, Romania
Copyright © 2021 Hohensinner, Atzler, Berger, Bozzetta, Höberth, Kofler, Rapottnig, Sterle and Haidvogl. This is an open-access article distributed under the terms of the Creative Commons Attribution License (CC BY). The use, distribution or reproduction in other forums is permitted, provided the original author(s) and the copyright owner(s) are credited and that the original publication in this journal is cited, in accordance with accepted academic practice. No use, distribution or reproduction is permitted which does not comply with these terms.
*Correspondence: Severin Hohensinner, severin.hohensinner@boku.ac.at