- 1College of Environmental Science and Tourism, Nanyang Normal University, Nanyang, China
- 2Henan Key Laboratory of Ecological Security for Water Source Region of Mid-Line of South-to-North Diversion Project, Nanyang, China
- 3College of Non-Major Foreign Language Teaching, Nanyang Normal University, Nanyang, China
- 4School of Earth and Environment, Anhui University of Science and Technology, Huainan, China
- 5Key Laboratory of Soil Environment and Pollution Remediation, Chinese Academy of Science, Institute of Soil Science, Nanjing, China
- 6School of Environment, Henan Normal University, Xinxiang, China
- 7The Key Laboratory of Tobacco Biology and Processing, Ministry of Agriculture and Rural Affairs, Tobacco Research Institute of Chinese Academy of Agricultural Sciences, Qingdao, China
The water-soluble heavy metal ions in contaminated soil may enter aquatic ecosystem through runoff, thus causing negative impact on the water environment. In this study, a two-year in situ experiment was carried out to explore an effective way to reduce the runoff erosion and water-soluble copper (Cu) and cadmium (Cd) in a contaminated soil (Cu: 1,148 mg kg−1, Cd: 1.31 mg kg−1) near a large Cu smelter. We evaluated the ability to influence soil properties by four Cu-tolerance plant species (Pennisetum sp., Elsholtzia splendens, Vetiveria zizanioides, Setaria pumila) grown in a contaminated acidic soil amended with lime. The results show that the addition of lime can significantly reduce the exchangeable fraction (EXC) of Cu and Cd in soil (81.1–85.6% and 46.3–55.9%, respectively). Plant species cannot change the fraction distributions of Cu and Cd in the lime-amended soils, but they can reduce the runoff generation by 8.39–77.0%. Although water-soluble Cu concentrations in the runoff were not significantly differed and water-soluble Cd cannot be detected among the four plant species, the combined remediation can significantly reduce 35.9–63.4% of Cu erosion to aquatic ecosystem, following the order: Pennisetum sp. > Elsholtzia splendens > Vetiveria zizanioides > Setaria pumila. The implication of this study would provide valuable insights for contaminated soil management and risk reduction in the Cu and Cd contaminated regions.
Introduction
Increasing anthropogenic activities such as smelting and irrigation using waste water and atmospheric deposition have caused severe heavy metal contamination in soil around the world (Xu X et al., 2018). Heavy metals entering the soil will harm human health through the food-chain (Ouyang et al., 2018a). Meanwhile, Soil erosion has been a worldwide land degradation process and a serious threat to the sustainability of agriculture (Borrelli et al., 2015; Wang R et al., 2016; Wang Y et al., 2016). In China, 28.3% of the total soil loss occurs on agricultural lands, which account for only 6.8% of the total area of soil loss (MWR, 2007) (Li et al., 2014). The researches show that heavy metals mainly accumulate in the surface layer of soil and heavy metals in the surface layer can enter the surface runoff with the process of soil erosion (Devi and Bhattacharyy, 2018). Moreover, the migration of heavy metals with surface runoff will cause the expansion of heavy metal pollution area (Ouyang et al., 2018b).
In order to reduce the mobility and bioavailability of heavy metals in agricultural soils, many cost-effective and environmentally friendly techniques have been developed during the past decades (He et al., 2019). Lime has been used for in situ remediation of metal contaminated soil because it is cheap and easy to get (Guo et al., 2018; Zhang et al., 2019). At the same time, phytoremediation has been widely used in remediation of heavy metal contaminated soil because it is considered as an environmentally friendly and economic method to treat the pollutant (Ashraf et al., 2019; Liu et al., 2020).
The previous studies have greatly contributed to the knowledge of runoff and soil loss in red soil region of China (Xu Y et al., 2018). Many scholars have also done research on remediation of soil heavy metal pollution in this area (Liu et al., 2019). Nevertheless, changes of soil runoff after pollution remediation have received relatively little attention. The existing research mainly focuses on the soil heavy metal pollution situation, pollution sources, hazards and remediation measures. Unfortunately, the relationships between these remediation measures and runoff such as runoff and concentration of heavy metals in runoff have seldom been discussed. Meanwhile, few studies have compared the different remediation measures for optimisation purposes.
This study explores the various effects of the combined remediation of lime and Pennisetum sp., Elsholtzia splendens, Vetiveria zizanioides on speciation and availability of heavy metals in soil and runoff and concentration of heavy metals in runoff. Setaria pumila, an indigenous plant didn’t need to be planted artificially and could grow normally after the lime was applied in our previous studies, but would not grow in the soil without lime (Xu et al., 2017). So our study included five treatments: untreated soil, lime and native Setaria lutescens (indigenous plant, didn’t need to be planted artificially), lime and Elsholtzia splendens, lime and Vetiveria zizanioides, lime and Pennisetum sp. We hypothesised that the combined remediation would reduce surface runoff and concentration of heavy metals in runoff. Specifically, the objectives are to define the relationship between plant species and runoff generation and to identify the best remediation activity for contaminated soil.
Materials and Methods
Study Site
The study site is located in Guixi City, Jiangxi Province, China (116°55′ E, 28°12′ N). The area has a subtropical monsoon climate, with an average annual precipitation of 1808 mm. Farmers had used water containing heavy metals to irrigate for a long time, which lead to heavy metal contamination in soil (mainly Cu and Cd) and Cd concentrations in rice exceeding the National food health standards (0.2 mg kg−1, GB 15201-94). The soil texture is sandy loam and the basic soil properties are listed in Table 1.
Lime and Plant Species
The Cu and Cd concentrations in the lime (particle size 0.25 mm, purchased from building materials market, Jiangxi, China) were 1.36 and 0.873 mg kg−1, respectively; the pH was 12.2. Four phytoextractors were selected, including a Cu-tolerant plant (Elsholtzia splendens), energy plant (Pennisetum sp. and Vetiveria zizanioides) and a native weed (Setaria pumila). All the plants used in the experiment were obtained by indoor cultivation.
Plot Design
Five treatments were set up in this study: untreated soil (CK), lime and native Setaria lutescens (LW), lime and Elsholtzia splendens (LE), lime and Vetiveria zizanioides (LV), lime and Pennisetum sp. (LP), and every treatment was design with three replicates. The field plots were designed as 200 cm (length) × 200 cm (width) and were separated by plastic plates. 0.2% lime (based on the 0–17 cm soil weight) was applied every plot on December 23, 2012, then the lime was mixed thoroughly with the soil by rotary tillage. Elsholtzia splendens (planting density 20 × 20 cm), Vetiveria zizanioides (planting density 30 × 30 cm), and Pennisetum sp. (planting density 50 × 50 cm) were planted on 26 April each year (2013, 2014). Weeds (mainly Setaria lutescens) were cleared from all plots before planting every year and no more weeding after planting. All field plots were managed in the same management. Runoff was collected at the bottom of the slope in a channel that was connected to a 50 L plastic bucket, the runoff after each rainfall was calculated by weighing. The average slope gradient for the plots was approximately 3°.
Sample Collection
All the aboveground parts (shoots) of the plants were harvested in the middle of December each year. Some plant samples were brought back to the laboratory, and washed with tap water and then with ultrapure water. Thereafter, the plant samples were weighted after drying to constant weight in an oven at 80°C, subsequently, plant samples were crushed by a grinder and used for heavy metal analysis.
After the plant samples were harvested, five soil samples were collected in each plot and fully mixed to form a composite soil sample (about 1 kg), then the samples were air-dried and ground for physicochemical analysis.
We mainly study the runoff from April to June, because this period is rainy season and plants grown vigorously, which can have a significant impact on the runoff process. The runoff after each heavy rainfall was calculated by weighed, after the water sample in the plastic bucket was mixed, and 1 L water sample was taken out and shipped to our laboratory. After the sample was stationary for 3 days, the supernatant was separated by siphon method and prepared for chemical analysis.
Sample Analysis
The soil pH was measured with a glass electrode at a water soil ratio of 2.5:1 (PHS-2CW-CN, Bante, Shanghai, China). Walkley–Black procedure was used to measure the soil organic carbon (SOC), total nitrogen (TN) and total phosphate (TP) (Walkley and Black, 1934). The soil total potassium (TN) was measured according to Olsen (1954).
The Cu and Cd in soil was measured by atomic absorption spectrophotometry (SpectrAA-220) after the samples were digested (Xu et al., 2016). In order to ensure the reliability of the experimental data, a standard soil sample (GBW07405, National Research Center for Certified Reference Materials, China) was used during the experiment. The fractions of Cu and Cd were determined by the modified Tessier sequential chemical extraction procedures which divided soil fractions into five grades (Xu et al., 2016). The Cu and Cd in the runoff were measured by atomic absorption spectrophotometry after filtered by 0.45 μm filter membrane.
Statistical Analysis
SPSS20.0 (IBM SPSS, Somers, NY, United States) was used for one-way ANOVA and correlation analysis and all the graphics were plotted by Sigmaplot 12.5.
Results and Discussion
Surface Soil Properties
The properties of surface soil in plots of the five treatments are summarized in Table 2. The soil pH were significantly improved after the lime was applied, the range of improvement was 0.46–0.98. The addition of lime increased soil pH, mainly because lime was an alkaline substance, and its CaCO3 content was high which contributed greatly to the improvement of soil pH (Li et al., 2019). There were difference during the different treatments of plants, but the effect of plants on soil pH was not significant in 2013 and 2014. At the same time, there was a reduction of the soil pH 1 year after the lime application, and the decreasing range was 0.16–0.52. This might be due to that the area was located in an acid deposition area, and a large amount of acid gas was emitted from the smelters and fertilizer plants. The soil organic carbon contents were similar among the five treatments in 2013 but variable in 2014. The highest soil organic carbon content in 2014 was found in the lime–Pennisetum sp (LP) treatment (19.1 g kg−1). This result might be related to three factors: first, vegetation restoration in soil erosion areas increased vegetation coverage and reduced soil erosion and nutrient loss (Tao et al., 2020); at the same time, the presence of plant residues, roots and root exudates also increased the input of organic matter to the soil (Lu et al., 2019); last, after the combined remediation, the changes of microbial community structure and function related to organic carbon turnover might affect the accumulation of organic carbon (Zhao et al., 2015). These changes in soil ultimately affect the concentration of soil organic carbon. Compared with the CK, the combined treatment of lime and plants did not significantly change the total Cu and Cd in soil.
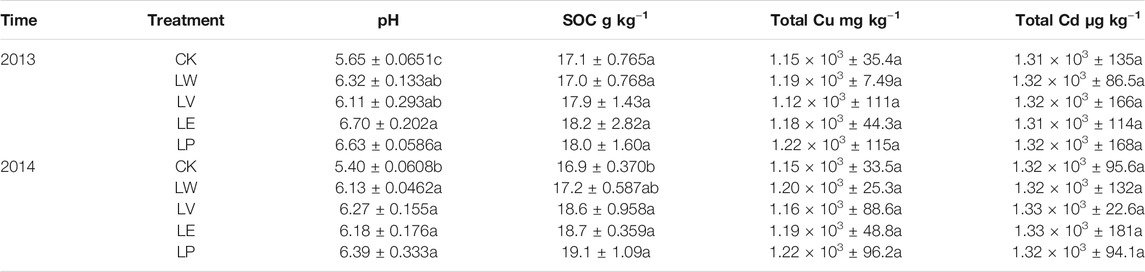
TABLE 2. Surface soil properties after treatments (SOC = soil organic carbon, CK = untreated soil, LW, lime + Setaria lutescens; LE, lime + Elsholtzia splendens; LV, lime + Vetiveria zizanioides; LP, lime + Pennisetum sp., Different lowercase letters indicate significant differences between treatments, n = 3, p < 0.05).
Chemical Fractions of Cd and Cu
Heavy metals in soil can be divided into solid and solution phases according to their existing forms, and they mainly exist in the solid phase (Xiao et al., 2017). Moreover, heavy metals in solid-phase can be divided into different fractions according to their solubility, mobility, bioavailability, and potential environmental toxicity, therefore, a single extraction step cannot fully evaluate the toxicity and migration of heavy metals in soil (Hou et al., 2017). In order to evaluate the migration and bioavailability of heavy metals in soil, a sequential extraction procedure was used in the present study. The fractions of Cu and Cd in soil were listed in Table 3. The total Cu concentration was 1.15 × 103 mg kg−1 in the soil of CK, The Fe-Mn fraction of Cu (409 mg kg−1, 35.6%) was most abundant, followed by the OM fraction (275 mg kg−1, 23.9%) and CA fraction (217 mg kg−1, 18.9%); the RES fraction (143 mg kg−1, 12.4%) and EXC fraction (77.3 mg kg−1, 6.72%) were the lowest. When the lime was added to the soil, the EXC fractions of all the other four treatments were reduced significantly in 2013 and 2014. Both of the most maximum reduction in 2013 and 2014 were found in LP, where EXC fraction with 85.6% reduction from 77.3 to 11.1 mg kg−1 and a 82.4% reduction from 82.6 to 16.6 mg kg−1, respectively. Conversely, the RES fractions were significantly improved in the combined remediation compared with CK. Just as the EXC fraction, the most maximum promotion of RES fractions in 2013 and 2014 were found in LP, and the RES fraction was increased by 51.0 and 40.9%, respectively. While there was no significant difference of the CA fraction, Fe-Mn fraction and OM fraction among the five treatments. It is worth noting that there was no significant difference in Cu fractions among the four different plant treatments (LW, LV, LE, LP), in other words, almost all the changes of Cu fractions were caused by the addition of lime but not the cultivation of plant.
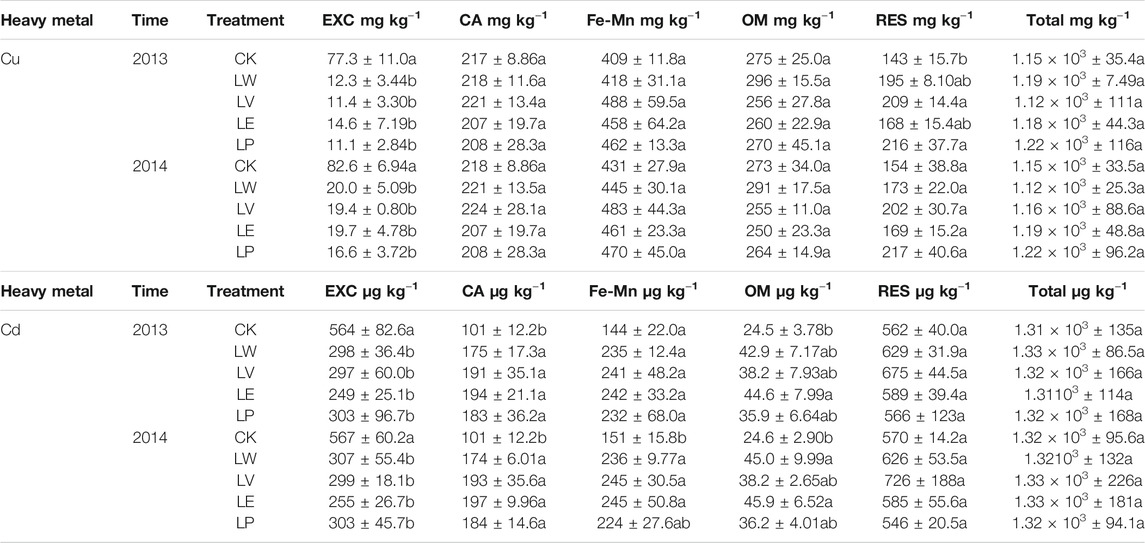
TABLE 3. Soil Cu and Cd fractions after the treatments (CK, untreated soil; LW, lime + Setaria lutescens; LE, lime + Elsholtzia splendens; LV, lime + Vetiveria zizanioides; LP, lime + Pennisetum sp.; EXC, exchangeable fraction; CA, carbonate-bound fraction, Fe–Mn, Fe–Mn oxides-bound fraction; OM, organic matter-bound fraction; RES, residual fraction. Different lowercase letters indicate significant differences between treatments, n = 3, p < 0.05).
The total Cd concentration in soil was 1.31 × 103 μg kg−1, but different from the distribution of Cu, the Cd in the soil of CK was mainly present in the EXC fraction (564 × 103 μg kg−1, 43.1%), which indicated that the bioavailability of Cd was higher than that of Cu in this area. Additionally, the RES fraction (562 × 103 μg kg−1, 42.9%) was abundant and followed by the Fe-Mn and CA fraction which were 144 × 103 μg kg−1 (11.0%) and 101 × 103 μg kg-1 (7.71%), respectively. The OM fraction (24.5 × 103 μg kg−1, 1.87%) was the least in the soil of CK. With lime application, the total Cd concentration was almost unchanged, but the EXC fraction of Cd was significantly by 46.3–55.9% and 45.9–55.0% in 2013 and 2014, respectively. While the CA fraction was markedly increased, the decrease rates in 2013 and 2014 were 73.3–92.1% and 72.3–95.0%, respectively. However, the addition of lime had no significant effect on Fe-Mn, OM and RES fraction of Cd in soil. Similar to the fraction of Cu in soil, there was no significant difference in Cd fractions among the four different plant treatments (LW, LV, LE, LP).
The EXC fraction of heavy metals is considered as a phase which is much easier to migrate and has higher bioavailability (Markovic et al., 2019). The EXC fraction of Cd is often present at low concentrations (<10% of total Cd) in uncontaminated soil (Wong et al., 2002). However, in this study, the EXC fraction of Cd constituted 43.1% of the total Cd in the soil of CK, which far more than that in uncontaminated soil. We speculated that the high EXC fraction of Cd was not only related to irrigation, but also introduced from atmospheric deposition of the nearby copper smelter. Research shows that the Cd which enters the soil through atmospheric deposition has high activity and bioavailability (Zhou et al., 2019). Additionally, the EXC fraction can be used to evaluate the bioavailability and environmental toxicity of heavy metals (Mohamed et al., 2017). In this study, the EXC fraction of Cu and Cd were significantly decreased by the combine remediation, meanwhile the RES and CA fractions were increased after the application. At the same time, the percentages of EXC fraction of both Cd and Cu were significantly decreased after the combine remediation. This demonstrated that lime could be used as an effective amendment to decrease the bioavailability and mobility of Cd and Cu in the contaminated soil. Meanwhile, the reduction of EXC fraction Cu (85.6%) by application of lime was much greater than that of Cd (55.9%) in terms of the reduction range, which indicated that the ability of lime to reduce the bioavailability and mobility of Cu was better than that of Cd.
Runoff Generation Response
A total of seven rainfall events greater than 50 mm were record during 2013 and 2014. The runoff generation was shown Figure 1, the runoff was significantly different among the five treatments. There was no significant difference of the runoff generation among the different treatments in 2013-05-10, 2013-05-22 and 2014-05-03. In the other four rainfall events, the CK treatment showed the largest runoff generation in every rainfall event among all of the plots, while the minimum runoff generation was LP treatment. Compared with CK, LW treatment can reduce runoff generation by approximately 9.95–32.6%. The runoff reduction rates of LV, LE and LP were calculated as 16.8%–53.4%, 23.3%–60.1% and 38.4–77.0%, respectively. While we found that the larger the shoot biomass, the smaller the runoff generation (Figures 1, 2). The results showed that the combined remediation of lime and plants could effectively reduce the runoff generation in the process of rainfall. However, this effect only appears after the plant had grown for a period of time. The above ground part of hedgerow had the function of mechanical blocking to retain runoff and reduce runoff and sediment; the root system of underground part could fix soil and improve soil physical and chemical properties (Cao et al., 2015). After planting plants, the runoff generation time of the slope land could be lagged behind than control, and the more vigorous the aboveground part of the plants grow, the more obvious of the lag time of runoff generation was. The aboveground part of the plant had the mechanical blocking function to retain runoff and reduce runoff and sediment, the underground part of the root system could fix the soil and improve the soil physical and chemical properties (Wang et al., 2017; Wang et al., 2018).
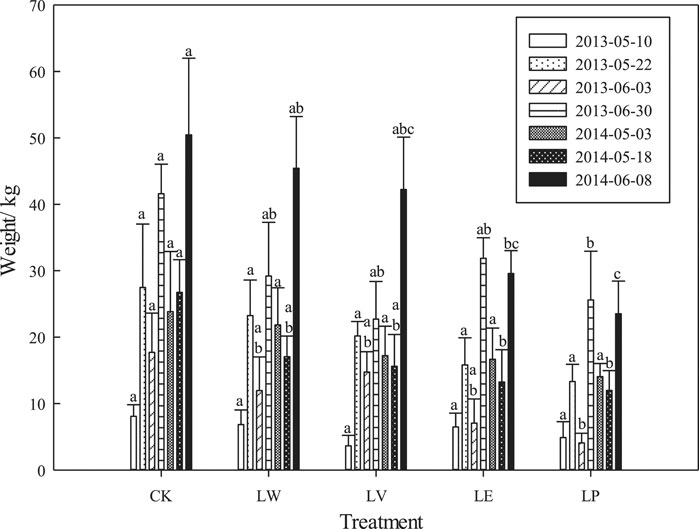
FIGURE 1. Cumulative runoff of single rainfall for different treatments in 2013 and 2014(CK, untreated soil; LW, lime + Setaria lutescens; LE, lime + Elsholtzia splendens; LV, lime + Vetiveria zizanioides; LP, lime + Pennisetum sp., Different lowercase letters indicate significant differences between treatments, n = 3, p < 0.05).
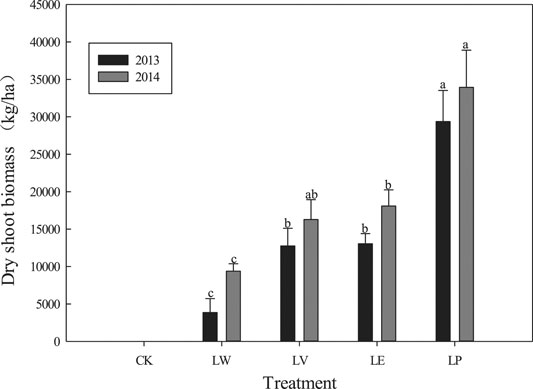
FIGURE 2. Dry shoot biomass of each treatment (CK = untreated soil, LW = lime + Setaria lutescens, LE, lime + Elsholtzia splendens; LV, lime + Vetiveria zizanioides; LP, lime + Pennisetum sp., Different lowercase letters indicate significant differences between treatments in the same year, n = 3, p < 0.05).
Water-soluble metal concentration and pH in runoff are listed in the Table 4. Based on the analysis, we found that the runoff pH was significantly increased by the combined remediation of lime and plant in runoff events of 2013-06-03, 2014-05-03, 2014-05-18 and 2014-06-08. However, the effect of plant on the pH of runoff was not significant after lime was applied. Similar to the pH of runoff, the addition of lime in soil could significantly reduce the water-soluble Cu concentration in runoff, but the plant had no significant effect on the water-soluble Cu concentration. The reduction rates of water-soluble Cu concentration in each rainfall was quite different, and the maximum reduction rate occurred in LE in 2013-06-03 (40.9%), the minimum reduction rate occurred in LW and LV in 2014-06-08 (13.6%). Combined with the data of runoff and Cu concentration in runoff, we found that the combine remediation could significantly reduce 35.9–63.4% of Cu erosion to aquatic ecosystem, following the order: Pennisetum sp. > Elsholtzia splendens > Vetiveria zizanioides > Setaria pumila. In this study, Cd was not detected in the runoff which might be due to the low concentration of Cd in soil compared with Cu, thus failed to reach the limit of instrument detection.

TABLE 4. Water-soluble metal concentration and pH in runoff of different remediation measures (CK, untreated soil; LW, lime + Setaria lutescens; LE, lime + Elsholtzia splendens; LV, lime + Vetiveria zizanioides; LP, lime + Pennisetum sp., Different lowercase letters indicate significant differences between treatments, n = 3, p < 0.05).
Numerous studies have shown that the activity and mobility of heavy metal can be significantly affected by soil pH (Zhai et al., 2018). The bioavailability and toxicity of heavy metals in soils are mainly depending on the activity of free ions rather than total amount. Soil pH is the most important factor affecting Cu and Cd availability among all parameters. The lower the environmental pH value is, the higher the mobility and activity of Cu and Cd is (Fei et al., 2018). Therefore, it is an effective measure to reduce the mobility and activity of Cu and Cd by adding lime to increase soil pH in acidic areas polluted by Cu and Cd (Rees et al., 2014). The EXC fraction of Cu in soil was reduced, and the water-soluble Cu concentration in runoff was reduced by increasing soil pH (Table 3, Table 4). The result is similar to Liu who found that the mass concentration of water-soluble Cd in runoff samples was significantly lower than that of other treatments after increasing soil pH by adding biochar in the same rainfall event (Liu et al., 2016). At the same time, previous study had shown that, the available Cu was converted to the stable component due to the decrease of redox potential (Eh) and the increase of pH under flooding condition, thus reducing the Cu activity (Cui et al., 2018). The results of correlation analysis showed that there was a significant correlation between Cu concentration in runoff and EXC fraction of Cu in soil (p = 0.621). This indicated that reducing the mobility and mobility of heavy metals in soil was an effective way to reduce the concentration of heavy metals in runoff. The addition of lime could significantly reduce the EXC fraction of Cu in soil, which might be the direct reason for the decrease of Cu concentration in runoff. At the same time, plant planting reduced the runoff generation, the combined effect of reduction of copper concentration in runoff and runoff generation decreased Cu erosion to aquatic ecosystem.
Conclusion
Five treatments were compared in terms of soil characteristics, runoff generation, water-soluble metal concentration and pH in runoff in this study. The results supported our hypothesis that four combine remediation (LW, LV, LE, and LP) could enhance the soil pH, SOC and reduce the EXC fraction of Cu and Cd in soil, while the water-soluble Cu concentration could be reduced and pH in runoff could be increased by this four remediation. Three combine remediation (LV, LE, LP) could reduce the surface runoff generation, which was vital to preventing sediment and heavy metal loss during water flow. However, the runoff generation cannot be significantly reduced by only lime application. Meanwhile, the LP treatment had the best performance in reducing runoff generation among the four treatments. As a result, the water-soluble Cu which into the river with the runoff is greatly reduced. Thus, the combine remediation can improve soil quality, decrease heavy metal availability and mobility in the soil and can be effective in reducing heavy metal loss. However, because the main purpose of our study was to explore the effect of combined phytoremediation and lime on the exchangeable fractions and migration of heavy metals with water, we mainly considered the possible effect when designed the experiment, so the research on the mechanism was lacking. We will add indoor simulation experiments and pay more attention to the mechanism in the future field trials.
Data Availability Statement
The original contributions presented in the study are included in the article/supplementary material, further inquiries can be directed to the corresponding authors.
Author Contributions
LX is responsible for the writing of the paper and the conduct of the experiment, XX has made contributions to the revision of the language of the paper, HC, JGZ, JNZ, JP, JB, XZ, and MJ share the ideas and logic modification of the paper.
Funding
The research was funded by the PhD Special Project of Nanyang Normal University (2018ZX018), Scientific and Technological Research Projects in Henan Province (212102310844), the National Natural Science Foundation of China (31901195, 41601340) and scientific research and service platform fund of Henan province (2016151).
Conflict of Interest
The authors declare that the research was conducted in the absence of any commercial or financial relationships that could be construed as a potential conflict of interest.
References
Ashraf, S., Ali, Q., Zahir, Z. A., Ashraf, S., and Asghar, H. N. (2019). Phytoremediation: Environmentally Sustainable Way for Reclamation of Heavy Metal Polluted Soils. Ecotoxicology Environ. Saf. 174, 714–727. doi:10.1016/j.ecoenv.2019.02.068
Borrelli, P., Märker, M., and Schütt, B. (2015). Modelling Post-Tree-Harvesting Soil Erosion and Sediment Deposition Potential in the Turano River Basin (Italian Central Apennine. Land Degrad. Develop. 26, 356–366. doi:10.1002/ldr.2214
Cao, L., Zhang, Y., Lu, H., Yuan, J., Zhu, Y., and Liang, Y. (2015). Grass Hedge Effects on Controlling Soil Loss from Concentrated Flow: A Case Study in the Red Soil Region of China. Soil Tillage Res. 148, 97–105. doi:10.1016/j.still.2014.12.009
Cui, H., Zhang, W., Zhou, J., Xu, L., Zhang, X., Zhang, S., et al. (2018). Availability and Vertical Distribution of Cu, Cd, Ca, and P in Soil as Influenced by Lime and Apatite with Different Dosages: a 7-year Field Study. Environ. Sci. Pollut. Res. 25, 35143–35153. doi:10.1007/s11356-018-3421-0
Devi, U., and Bhattacharyy, K. G. (2018). Mobility and Bioavailability of Cd, Co, Cr, Cu, Mn and Zn in Surface Runoff Sediments in the Urban Catchment Area of Guwahati, India. Appl. Water Sci. 8, 18–32. doi:10.1007/s13201-018-0651-8
Fei, Y., Yan, X. L., and Li, Y. H. (2018). Stabilization Effects of Fe-Mn Binary Oxide on Arsenic and Heavy Metal Co-contaminated Soils under Different pH Conditions. Environ. Sci. 3, 1430–1437. doi:10.13227/j.hjkx.201707101
Guo, F., Ding, C., Zhou, Z., Huang, G., and Wang, X. (2018). Stability of Immobilization Remediation of Several Amendments on Cadmium Contaminated Soils as Affected by Simulated Soil Acidification. Ecotoxicology Environ. Saf. 161, 164–172. doi:10.1016/j.ecoenv.2018.05.088
He, L., Zhong, H., Liu, G., Dai, Z., Brookes, P. C., Xu, J., et al. (2019). Remediation of Heavy Metal Contaminated Soils by Biochar: Mechanisms, Potential Risks and Applications in China. Environ. Pollut. 252, 846–855. doi:10.1016/j.envpol.2019.05.151
Hou, D., O'Connor, D., Nathanail, P., Tian, L., and Ma, Y. (2017). Integrated GIS and Multivariate Statistical Analysis for Regional Scale Assessment of Heavy Metal Soil Contamination: A Critical Review. Environ. Pollut. 231, 1188–1200. doi:10.1016/j.envpol.2017.07.021
Li, Q. Y., Fang, H. Y., Sun, L. Y., and Cai, Q. G. (2014). USING THE 137 Cs TECHNIQUE TO STUDY THE EFFECT OF SOIL REDISTRIBUTION ON SOIL ORGANIC CARBON AND TOTAL NITROGEN STOCKS IN AN AGRICULTURAL CATCHMENT OF NORTHEAST CHINA. Land Degrad. Develop. 25, 350–359. doi:10.1002/ldr.2144
Li, Y., Cui, S., Chang, S. X., and Zhang, Q. (2019). Liming Effects on Soil pH and Crop Yield Depend on Lime Material Type, Application Method and Rate, and Crop Species: a Global Meta-Analysis. J. Soils Sediments 19, 1393–1406. doi:10.1007/s11368-018-2120-2
Liu, B., Wu, C., Pan, P., Fu, Y., He, Z., Wu, L., et al. (2019). Remediation Effectiveness of Vermicompost for a Potentially Toxic Metal-Contaminated Tropical Acidic Soil in China. Ecotoxicology Environ. Saf. 182, 109394–109402. doi:10.1016/j.ecoenv.2019.109394
Liu, H. L., Zhou, J., Li, M., Hu, Y. M., Liu, X., and Zhou, J. (2019). Study of the Bioavailability of Heavy Metals from Atmospheric Deposition on the Soil-Pakchoi (Brassica Chinensis L.) System. J. Hazard. Mater. 362, 9–16. doi:10.1016/j.jhazmat.2018.09.032
Liu, S., Yang, B., Liang, Y., Xiao, Y., and Fang, J. (2020). Prospect of Phytoremediation Combined with Other Approaches for Remediation of Heavy Metal-Polluted Soils. Environ. Sci. Pollut. Res. 27, 16069–16085. doi:10.1007/s11356-020-08282-6
Liu, X. L., Zeng, Z. X., Tie, B. Q., Chen, Q. W., and Wei, X. D. (2016). Cd Runoff Load and Soil Profile Movement after Implementation of Some Typical Contaminated Agricultural Soil Remediation Strategies. Environ. Sci. 37, 734–739. doi:10.13227/j.hjkx.2016.02.044
Lu, J., Dijkstra, F. A., Wang, P., and Cheng, W. (2019). Roots of Non-woody Perennials Accelerated Long-Term Soil Organic Matter Decomposition through Biological and Physical Mechanisms. Soil Biol. Biochem. 134, 42–53. doi:10.1016/j.soilbio.2019.03.015
Markovic, J., Jovic, M., Smiciklas, I., Sljivic-Ivanovic, M., Onjia, A., Trivunac, K., et al. (2019). Cadmium Retention and Distribution in Contaminated Soil: Effects and Interactions of Soil Properties, Contamination Level, Aging Time and In Situ Immobilization Agents. Ecotoxicology Environ. Saf. 174, 305–314. doi:10.1016/j.ecoenv.2019.03.001
Mohamed, B. A., Ellis, N., Kim, C. S., and Bi, X. (2017). The Role of Tailored Biochar in Increasing Plant Growth, and Reducing Bioavailability, Phytotoxicity, and Uptake of Heavy Metals in Contaminated Soil. Environ. Pollut. 230, 329–338. doi:10.1016/j.envpol.2017.06.075
Olsen, S. R. (1954). Estimation of Available Phosphorus in Soils by Extraction with Sodium Bicarbonate. Washington: Us Department of Agriculture Circ.
Ouyang, W., Wang, Y., Lin, C., He, M., Hao, F., Liu, H., et al. (2018a). Heavy Metal Loss from Agricultural Watershed to Aquatic System: A Scientometrics Review. Sci. Total Environ. 637-638, 208–220. doi:10.1016/j.scitotenv.2018.04.434
Ouyang, W., Yang, W., Tysklind, M., Xu, Y., Lin, C., Gao, X., et al. (2018b). Using River Sediments to Analyze the Driving Force Difference for Non-point Source Pollution Dynamics between Two Scales of Watersheds. Water Res. 139, 311–320. doi:10.1016/j.watres.2018.04.020
Rees, F., Simonnot, M. O., and Morel, J. L. (2014). Short-term Effects of Biochar on Soil Heavy Metal Mobility Are Controlled by Intra-particle Diffusion and Soil pH Increase. Eur. J. Soil Sci. 65, 149–161. doi:10.1111/ejss.12107
Tao, W., Wang, Q., Guo, L., and Lin, H. (2020). A New Analytical Model for Predicting Soil Erosion and Nutrient Loss during Crop Growth on the Chinese Loess Plateau. Soil tillage Res. 199, 104585–1045943. doi:10.1016/j.still.2020.104585
Walkley, A., and Black, I. A. (1934). An Examination of the Degtjareff Method for Determining Soil Organic Matter, and a Proposed Modification of the Chromic Acid Titration Method. Soil Sci. 37, 29–38. doi:10.1097/00010694-193401000-00003
Wang, R., Guo, J., Xu, Y., Ding, Y., Shen, Y., Zheng, X., et al. (2016). Evaluation of Silkworm Excrement and Mushroom Dreg for the Remediation of Multiple Heavy Metal/metalloid Contaminated Soil Using Pakchoi. Ecotoxicology Environ. Saf. 124, 239–247. doi:10.1016/j.ecoenv.2015.10.014
Wang, Y., Cao, L., Fan, J., Lu, H., Zhu, Y., Gu, Y., et al. (2017). Modelling Soil Detachment of Different Management Practices in the Red Soil Region of China. Land Degrad. Develop. 28, 1496–1505. doi:10.1002/ldr.2658
Wang, Y., Fan, J., Cao, L., and Liang, Y. (2016). Infiltration and Runoff Generation under Various Cropping Patterns in the Red Soil Region of China. Land Degrad. Develop. 27, 83–91. doi:10.1002/ldr.2460
Wang, Y., Fan, J., Cao, L., Zheng, X., Ren, P., and Zhao, S. (2018). The Influence of Tillage Practices on Soil Detachment in the Red Soil Region of China. Catena 165, 272–278. doi:10.1016/j.catena.2018.02.011
Wong, S. C., Li, X. D., Zhang, G., Qi, S. H., and Min, Y. S. (2002). Heavy Metals in Agricultural Soils of the Pearl River Delta, South China. Environ. Pollut. 119, 33–44. doi:10.1016/s0269-7491(01)00325-6
Xiao, R., Wang, S., Li, R., Wang, J. J., and Zhang, Z. (2017). Soil Heavy Metal Contamination and Health Risks Associated with Artisanal Gold Mining in Tongguan, Shaanxi, China. Ecotoxicology Environ. Saf. 141, 17–24. doi:10.1016/j.ecoenv.2017.03.002
Xu, L., Cui, H., Zheng, X., Zhou, J., Zhang, W., Liang, J., et al. (2017). Changes in the Heavy Metal Distributions in Whole Soil and Aggregates Affected by the Application of Alkaline Materials and Phytoremediation. RSC Adv. 7, 41033–41042. doi:10.1039/c7ra05670b
Xu, L., Cui, H., Zheng, X., Zhu, Z., Liang, J., and Zhou, J. (2016). Immobilization of Copper and Cadmium by Hydroxyapatite Combined with Phytoextraction and Changes in Microbial Community Structure in a Smelter-Impacted Soil. RSC Adv. 6, 103955–103964. doi:10.1039/c6ra23487a
Xu, X., Zheng, F., Wilson, G. V., He, C., Lu, J., and Bian, F. (2018). Comparison of Runoff and Soil Loss in Different Tillage Systems in the Mollisol Region of Northeast China. Soil Tillage Res. 177, 1–11. doi:10.1016/j.still.2017.10.005
Xu, Y., Seshadri, B., Sarkar, B., Wang, H., Rumpel, C., Sparks, D., et al. (2018). Biochar Modulates Heavy Metal Toxicity and Improves Microbial Carbon Use Efficiency in Soil. Sci. Total Environ. 621, 148–159. doi:10.1016/j.scitotenv.2017.11.214
Zhai, X., Li, Z., Huang, B., Luo, N., Huang, M., Zhang, Q., et al. (2018). Remediation of Multiple Heavy Metal-Contaminated Soil through the Combination of Soil Washing and In Situ Immobilization. Sci. Total Environ. 635, 92–99. doi:10.1016/j.scitotenv.2018.04.119
Zhang, W.-h., Sun, R.-b., Xu, L., Liang, J.-n., and Zhou, J. (2019). Assessment of Bacterial Communities in Cu-Contaminated Soil Immobilized by a One-Time Application of Micro-/nano-hydroxyapatite and Phytoremediation for 3 Years. Chemosphere 223, 240–249. doi:10.1016/j.chemosphere.2019.02.049
Keywords: soil contamination, lime, phytoremediation, soil erosion, runoff
Citation: Xu L, Xing X, Cui H, Zhou J, Zhou J, Peng J, Bai J, Zheng X and Ji M (2021) The Combination of Lime and Plant Species Effects on Trace Metals (Copper and Cadmium) in Soil Exchangeable Fractions and Runoff in the Red Soil Region of China. Front. Environ. Sci. 9:638324. doi: 10.3389/fenvs.2021.638324
Received: 06 December 2020; Accepted: 28 June 2021;
Published: 07 July 2021.
Edited by:
Ravi Naidu, University of Newcastle, AustraliaReviewed by:
Bhabananda Biswas, University of South Australia, AustraliaVarenyam Achal, Guangdong Technion-Israel Institute of Technology (GTIIT), China
Copyright © 2021 Xu, Xing, Cui, Zhou, Zhou, Peng, Bai, Zheng and Ji. This is an open-access article distributed under the terms of the Creative Commons Attribution License (CC BY). The use, distribution or reproduction in other forums is permitted, provided the original author(s) and the copyright owner(s) are credited and that the original publication in this journal is cited, in accordance with accepted academic practice. No use, distribution or reproduction is permitted which does not comply with these terms.
*Correspondence: Xuebo Zheng, emhlbmd4dWVib0BjYWFzLmNu; Mingfei Ji, amltZmR5QGdtYWlsLmNvbQ==