- 1Norwegian Geotechnical Institute (NGI), Oslo, Norway
- 2Department of Geosciences, University of Oslo, Oslo, Norway
- 3Norwegian Institute for Water Research (NIVA), Oslo, Norway
- 4Department of Environment and Health, VU University Amsterdam, Amsterdam, Netherlands
Urbanization has resulted in increased contaminant run-off in densely populated areas. Climate change is expected to result in a higher frequency of extreme weather events including torrential rainfall and storms. The contaminant levels of polycyclic aromatic hydrocarbons (PAH), chlorinated paraffins (CPF) and selected metals, in a small urban river were monitored during snow-melting and rainfall events to quantify the contribution to the contamination load of receiving waters of the inner Oslo fjord, Norway. Suspended particulate matter (SPM) was characterized with respect to levels of contaminants as well as toxic response using a battery of bioassays. The contaminant flux from the river to the fjord was quantified and assessed relative to sediment data. Historic data for near-shore sediment samples from the fjord were used to document urban input. The results show a clear episodic response in contaminant load emitted from the river to the fjord. The main historic input to the fjord was found to be PAH from pyrogenic sources like coal and wood burning as well as traffic. A significant reduction in the level of PAH was observed since the 1980s. The measured flux of CPF is consistent with on-going societal use despite a ban on the use of short chain CPF imposed in Norway from 2002.
Introduction
Expected climatic changes (CC) will lead to more extreme river discharges, extensive rainfall in the watershed and increased seawater levels (Hanssen-Bauer et al., 2017). Along with the obvious effect on water quantity, these developments will have consequences for the water quality in terms of concentrations of nutrients and contaminants that are flushed into rivers and subsequently potentially transported to coastal zones. In the Scandinavian environment, precipitation is accumulated as snow during the winter months which simultaneously traps atmospheric contaminants from e.g., traffic exhaust and woodstoves. In the urban environment, several non-atmospheric sources, such as tire wear, road dust and runoff from building facades, contribute to the contaminant load stored in the snow pack (Viklander, 1999). During a short snow melting period of a few weeks in spring (French and Binley, 2004), this contaminant load can be released to urban watersheds, which can result in peak concentrations.
Previous studies related to this topic are scarce. Meyer et al. (2011) reported that transport of polycyclic aromatic hydrocarbons and pesticides increased considerably during snowmelt in an urban watershed near Toronto. The contaminant release was related to the intensity of the melting process as well as the properties of the organic chemicals (Meyer and Wania, 2011). In Northern Sweden, eight times higher concentrations and five times higher loads of particles were observed during a snowmelt period compared to a rain period (Westerlund and Viklander, 2006). During melting, the total suspended solid concentration was strongly correlated with concentrations of Cd, Cu, Ni, Pb and Zn in the run-off.
In addition to changes in hydrology, climate change will influence the environmental distribution and toxicity of contaminants as reviewed by Noyes and co-workers (2009). Alterations in food webs, lipid dynamics, ice and snow melt, and organic carbon cycling could result in increased levels of persistent organic pollutants in water, soil, and biota. Increased precipitation can reduce air pollution but might enhance surface deposition and subsequently surface run-off of such pollutants (Noyes et al., 2009). Increase in the intensity and frequency of storm events that has been predicted as a consequence of climate change (Hanssen-Bauer et al., 2017) could lead to increased contamination of water courses.
The objective of the present study was to investigate the transport of freely dissolved contaminants and contaminants associated with suspended particulate matter during events of increased surface run-off caused by weather conditions such as snow melting and extensive rainfall periods. The hypothesis of the work was that these events would increase the run-off of chemicals sorbed to the suspended particulate matter from the urban environment into receiving waters. The Aker River in Oslo, is used as a model river in this study. This river is included in the Oslo Water Works continuous monitoring program of water quantity and quality (Oslo kommune, 2017). The contaminant transport was evaluated by considering contaminant levels observed in the receiving fjord. Polycyclic aromatic hydrocarbons (PAH), polychlorinated biphenyls (PCB), chlorinated pesticides (OCP), chlorinated paraffins (CPF) and selected metals were quantified. Given the complex mixture of contaminants present, bioassays were included to address the toxicity potential of the contaminants associated with SPM.
Materials and Methods
The Aker River
The Aker River is part of the Nordmarka water course with a length of 50 km and drains in the forested area North of Oslo. Aker River is the name given to the last 10 km stretch of the water course that starts at lake Maridalen (the drinking water supply of the city of Oslo) and passes through previously industrialized areas that were a serious source of contamination until the end of the 1970s. The Aker River passes through Oslo city center on the way to the harbor area of Oslo where it discharges in to the fjord near to the opera house (Figure 1).
In 2009 the Norwegian Environment Agency performed a monitoring program of the local rivers in Oslo (Ranneklev et al., 2009). Several emerging contaminants of that time were detected in sediments from the Aker River. This included chlorinated paraffins, mainly short chain chlorinated paraffins (SCCP, max 870 μg/kg d.w.) and in addition, legacy contaminants (PAH and PCB) were detected. Urban run-off was identified as the major source of contaminants.
Sampling Sites
The water quality in the Aker River was investigated by event-based sampling surveys under snow melting and low and high-water flow conditions following extensive rainfall. Three stations (AKR 1, 3, 7) in addition to a permanent monitoring station operated by Oslo Water Works (STA) were included. In 2011 lake Maridalen was included as well. The stations were distributed along the 10 km river stretch covering the upper region, with limited urban influence down stream of lake Maridalen to the confluence with the Oslo fjord in the inner city of Oslo, with high urban influence (Figure 1). The permanent station is equipped with infrastructure for continuous monitoring of water flow and turbidity as well as time-composite sampling of the river water used to collect weekly samples. Details on the exact position of the sampling stations is presented in the supplementary material, Supplementary Table S1.
Water Sampling
Grab water samples, capturing both freely dissolved and particle bound contaminants, were collected in the middle of the stream from a bridge (AKR 1, 3 and 7) or from the water inlet of the permanent monitoring station. Six campaigns covering two years (2010-2011) included snow melting, low flow and extreme rain events. For metals and nutrients, 250 ml pre-cleaned PE bottles were used, while 1-L amber glass bottles were used for the analysis of PAH, PCB and OCP. In addition, time composite samples were taken on a weekly basis at the permanent monitoring station that were analyzed for content of SPM, organic carbon (OC), nutrients and metals. An overview of the sampling dates and parameters is given in Supplementary Table S1.
Passive Sampling
Passive samplers were used to quantify the freely dissolved concentration of PAH. Samplers were exposed at all four stations for a period varying from three to four weeks to attain an equilibrium with the free water column at the sampling station (Cornelissen et al., 2008). During the second-year passive samplers were also deployed in lake Maridalen at the inlet of the river. The passive samples consisted of approximately 50 cm strips of additive-free 55 µm thick polyoxymethylene (POM-55, Jonker and Koelmans, 2001). POM-55 was pre-cleaned by washing in hexane and methanol, both for 24 h each, followed by rinsing with deionized water. Samplers were stored in deionized water until use. Blank POM-55 samples that had not been exposed in the field had concentrations of almost all PAHs below the analytical limit of detection (1 ng/L), except for naphthalene and phenanthrene where blank concentrations were <1% of final concentrations after exposure. The principle behind equilibrium passive samplers is that freely dissolved water concentrations can be deduced from passive sampler concentrations, provided that equilibrium between the sampler and the surrounding water phase has been established and the concentration independent equilibrium partitioning constant (K-value) for the specific passive sampler and compound is known (Lydy et al., 2014). K-values for POM-55, KPOM-55 were established for PAHs in a previous study (Cornelissen et al., 2008) and used here.
Suspended Particulate Matter
A continuous flow centrifuge (CFC) was used to separate SPM from the water phase at the permanent monitoring station (STA). Water was fed into the centrifuge by a peristaltic pump at a rate of 1.5 l/min, using precleaned 1 cm-diameter silicone tubing. To collect sufficient material for subsequent analyses, the centrifuge was operated continuously for a week at each sampling event (Supplementary Table S1). The suspended solids deposited on the inner wall of the rotating stainless-steel drum. To increase recovery, remaining sediments were washed from the inside of the drum using Milli-Q water, which was subsequently filtered with a 0.45 μm cellulose nitrate filter and added to the sample. Kessler et al. (2020) studied different SPM sampling methods and indicated that CFC is able to separate the solid phase of a total sample almost completely from the water phase. It has previously been reported that more than 90% of the fraction <20 µm can be retained, indicating minimal colloidal losses (Pohlert et al., 2011).
Chemical Analyses
Standard water parameters including pH, electrical conductivity (EC), turbidity, suspended particle matter and total organic carbon were analyzed at the laboratory of Oslo Water and Sewerage Works, following internal procedures accredited according to NS-EN-ISO/IEC 17025 (2005). Nutrients (NH4+, PO43- as well as total N and total P) and metals (Cd, Cr, Cu, Pb, Hg, Ni and Zn) were analyzed at the same laboratory.
Organic contaminants in the grab water samples and passive samplers were analyzed according to the methods outlined by Hale et al. (2012). In short, 2 L water samples without any pretreatment to remove particles, were extracted with heptane to obtain the total water concentration. The heptane was cleaned up using a silica gel column that was eluted with heptane, and the eluate was concentrated under a nitrogen stream and analyzed on an Agilent 6850 Gas Chromatograph (DB-XLB column length 30 m, internal diameter 0.25 mm and 0.1 µm film thickness) coupled to an Agilent 5973 mass spectrometer. The POM passive samplers were extracted with 20 ml heptane by shaking for 48 h and were cleaned up and analyzed as above using 6 deuterated PAH internal standards. Suspended sediment samples from the CFC were freeze dried and analyzed for PAH16 and PCB7. In addition, the extracts were analyzed for chlorinated pesticides (OCP) and chlorinated paraffins (CPF) using a GC x GC method (Korytár et al., 2005). Short chain chlorinated paraffins (SCCP) with a chain length from C10-C13 and a varying degree of chlorination as well as medium chain chlorinated paraffins (MCCP) C14-C17 were quantified. Total carbon and nitrogen as well as their stable isotopes 13C and 15N were analyzed on a ThermoFisher Scientific Delta Plus XP isotope ratio mass spectrometer (ISMER, the University of Quebec at Rimouski). The fraction of black carbon was determined by the same elemental analysis method following thermal oxidation at 375°C (CTO 375, Cornelissen et al., 2005).
Toxicity Test
Sample Extraction
Separate SPM extractions were performed for the bioassays without the addition of internal standards used in the chemical analyses. A detailed description of the method is given in Hamers et al. (2015). In short, 5 g dry SPM samples were extracted by accelerated solvent extraction using a 3:1 dichloromethane:acetone solvent mixture at 90°C. Extracts were cleaned by Gel Permeation Chromatography (GPC). The eluate was split in two parts. A “total extract” was obtained by evaporating 90% of the eluate under N2, followed by a transfer to DMSO. “Stable extracts” were prepared by further clean-up of the remaining 10% of the GPC eluate on a sulfuric acid silica column eluted with 97:3 (v/v) hexane-diethylether, followed by evaporation under N2 and transfer to DMSO.
Bioassays
Total extracts of all the SPM samples were tested in a battery of in-vitro bioassays. The bioassays comprised decrease in microbial respiration measured in the Aliivibrio fischeri luminescence bioassay based on the Microtox bioassay adapted to a 96-well microtiter plate version by Hamers et al. (2001). Thyroid hormone-like activity was determined as the potency to compete with 125I-radiolabeled thyroid hormone thyroxine (T4) for binding to its plasma transport protein transthyretin (TTR) see Hamers et al. (2006) for details. Androgenic activity was determined as activation of the androgen receptor (AR) in the AR EcoScreen reporter gene assay (Araki et al., 2005), estrogenic activity as activation of the estrogen receptor (ER) in the VM7Luc4E2 reporter gene assay [ER-LUC; formerly known as BG1Luc4E2 (Rogers and Denison, 2000)], and activation of the aryl-hydrocarbon receptor (AhR) in the H4Ll.lc4 reporter gene assay (DR-LUC; Garrison et al., 1996). The latter bioassay was also applied on the stable extracts, to distinguish the AhR activating potency of non-persistent compounds (e.g., polycyclic aromatic hydrocarbons) from the true dioxin-like potency. Mutagenic activity was determined in the Ames II fluctuation test (Reifferscheid et al., 2012), with the frame-shift mutation indicative TA98 bacteria strain and with a metabolizing system consisting of Aroclor-1254 induced rat liver S9-mix (MP Biomedicals, Netherlands).
The observed bioassay response in the SPM extracts, were interpolated in a calibration curve of a bioassay-specific reference compound. The observed toxic potencies in the SPM material could in that way be expressed as an equivalent concentration of the reference compound. For details is referred to Hamers et al. (2015). For the A. fischeri luminescence bioassay this method cannot be applied since respiration is not a general response which cannot be referred to a specific mode of action and reference compound. Therefore, the concentration causing 50% inhibition was determined and the reciprocal calculated to express an effect concentration that increases with toxicity. Similarly, for the Ames II fluctuation bioassay, the lowest observed effect concentration (LOEC) was determined and the reciprocal value reported to express toxicity (Hamers et al., 2015).
Results
Suspended Solids
The concentration of SPM in the river water over the two-year monitoring period showed low baseline values below 1 mg/L during the winter months with elevated levels (maximum values 36 mg/L, April 2010) during snow melting and severe rainfall events. Figure 2 shows the six sampling campaign dates in relation to the measured SPM levels and weekly precipitation data. Total precipitation in Oslo was 739 mm in 2010, while it was 994 mm in 2011. A complete overview over the monitoring data including, river flow, pH, electrical conductivity (EC), TOC and levels of nutrients is presented in Supplementary Figure S1. EC was responsive to the snow melting periods that were observed around March/April 2010 and 2011.
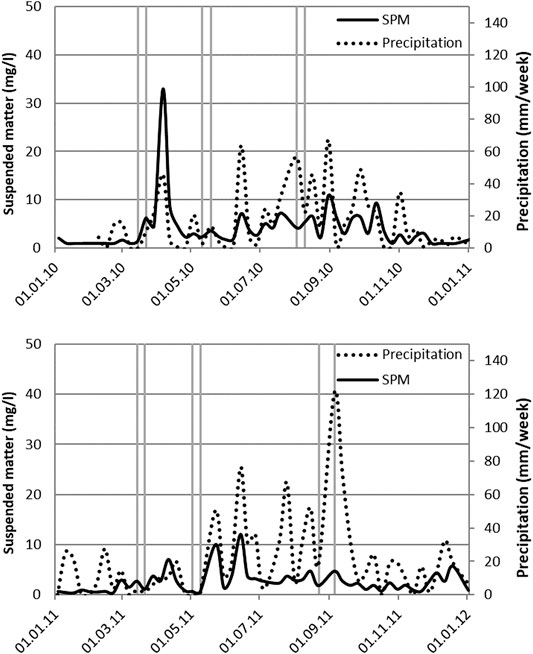
FIGURE 2. Concentrations of suspended particulate matter (weekly time composite samples) in the Aker River during the 2-year monitoring period of the study, weekly integrated precipitation data are indicated with dashed line (the intervals of the six sampling events are indicated with gray lines).
Metals
Metals were measured in the time composite weekly sample by Oslo Water Works during 2010. Aluminum, iron and copper showed strong co-variation, and their peak concentrations coincided with the peak in SPM (April 2010). Zinc levels were generally low and close to the analytical detection limit (0.01 mg/L). Cadmium and lead showed a similar top as observed for Al, Fe and Cu in April, but in contrast, elevated levels were also observed during the summer months (Supplementary Figure S2). To correct for the elemental contribution of clay minerals in the SPM, the concentrations were normalized to Al (Birch, 2020; Figure 3). Al normalized Pb concentrations increased almost 10-fold during the summer months, while Cd only showed 2 distinct peaks.
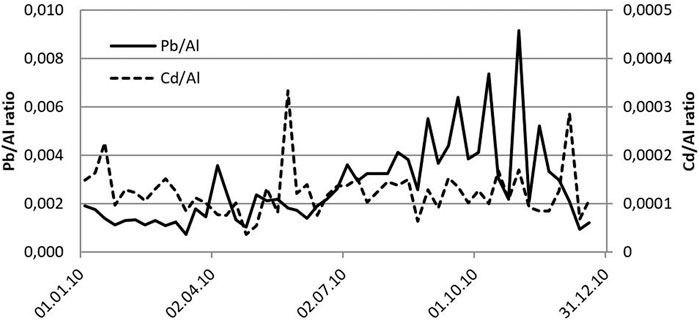
FIGURE 3. Aluminum normalized concentrations of Pb and Cd measured in the weekly time‐composite samples.
PAH
Both total water concentrations and freely dissolved water concentrations of PAH were determined along the complete length of the river. Concentrations of PAH16 in lake Maridalen (Supplementary Table S5) and the upper most water sampling station (AKR 1) were low (3-13 and 10-44 ng/L respectively). Downstream, total concentrations increased during the snow melting period (March/April), while there was no clear trend observed during the summer months despite severe rainfall events (Figure 4). Several PAH compounds (3-11) were below the limit of detection and the total PAH profile was dominated by phenanthrene (0.3- 12.3 ng/L), fluoranthene (0.3-7.0 ng/L) and pyrene (0.3-8.4 ng/L). The freely dissolved water concentrations, determined by passive sampling, showed less variation (7-30 ng/L PAH16) than total concentrations (3-79 ng/L PAH16) except for the station near the harbor (AKR 7) where high values of freely dissolved PAH were measured in both May and August 2011 (50 and 140 ng/L PAH16 respectively, Supplementary Table S4).
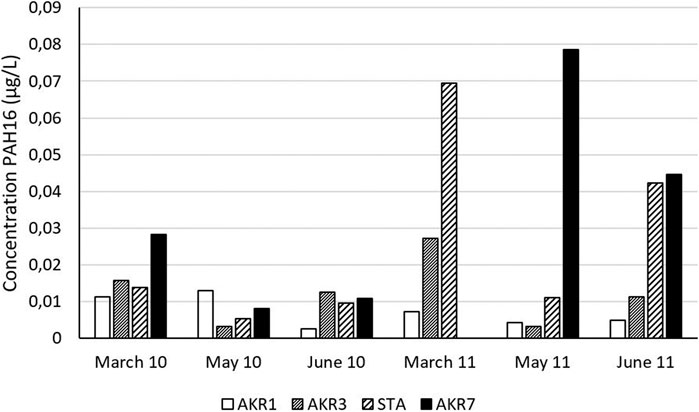
FIGURE 4. PAH16 concentrations in the grab samples during the 6 sampling events (sample AKR7 March 2011 was lost).
PAH concentrations in the SPM sampled at the permanent monitoring station (STA, 3.4-14.7 mg/kg PAH15, naphthalene was not quantified) had a significant contribution of the six heavy 5- and 6-ring PAH to the total PAH15 concentration (31-39%). A strong variation in the concentration of ΣPAH15 was observed during the three sampling events in 2010 (3.4-14.7 mg/kg d.w.), while concentrations were stable around 5 mg/kg d.w in 2011 (Supplementary Table S6). However, the relative contribution of the various PAHs was stable in all samples (Figure 5).
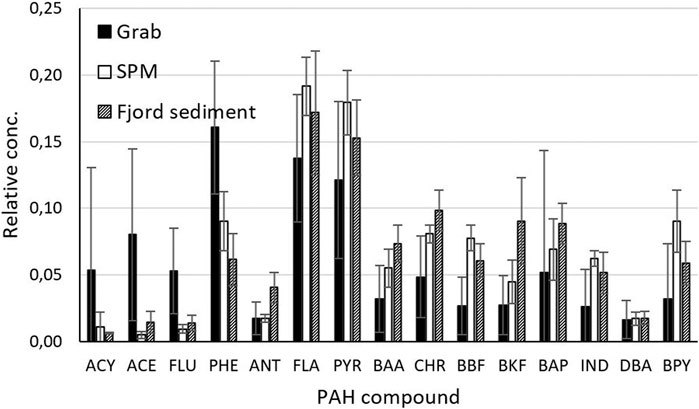
FIGURE 5. PAH profiles, expressed as the fraction of each compound as part of the total PAH15 concentration, in the grab water samples (n = 23), SPM samples (n = 6) compared to sediments in the Oslo fjord near the river mouth (n = 5) from Arp and Breedveld (2009).
PCB and OCP
The total water concentration of PCB was below the detection limit of 0.25 ng/L for all 7 congeners. The sum PCB7 concentrations in the SPM varied from 19.4 to 44.9 μg/kg d.w. (Supplementary Table S6) and showed a consistent distribution profile over the seven congeners (Figure 6). Twenty-one chlorinated pesticides (OCP) were quantified in the SPM samples and hexachlorobenzene (HCB) was found in the highest concentrations (4-140 μg/kg d.w.). Pentachlorobenzene, p,p’ DDT, DDE and DDD were also found in quantifiable concentrations (around 1 μg/kg d.w., Supplementary Table S7) in addition to dieldrin (<1 μg/kg d.w.).
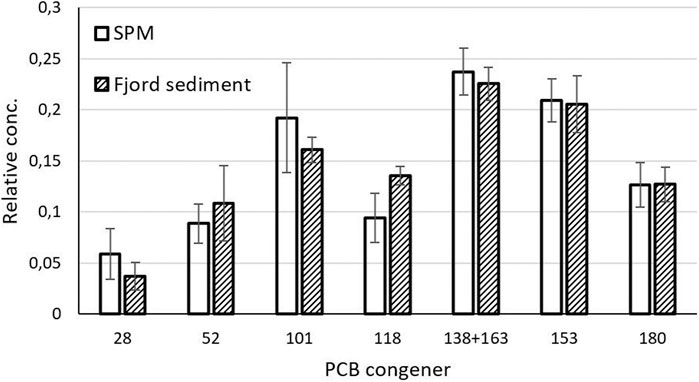
FIGURE 6. PCB profiles, expressed as the fraction of each compound as part of the total PCB7 concentration, in the SPM samples (n = 6) compared to sediment samples in the Oslo fjord near the river mouth (n = 5) from Arp and Breedveld (2009).
Chlorinated Paraffins
The concentrations of chlorinated paraffins in SPM was highly variable (300-23,000 μg/kg d.w.), with the highest level being observed during the wet period in August 2010 (Supplementary Table S7). All samples were dominated by medium chain length paraffins (MCCP, 68-92% of sum CPF). The SCCP and MCCP profiles were similar in all samples (Figure 7).
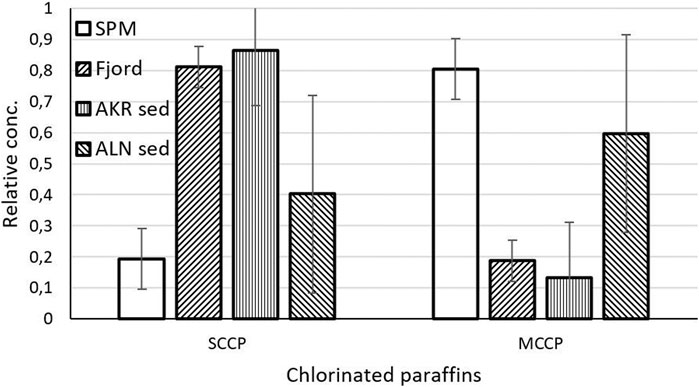
FIGURE 7. Profiles of chlorinated paraffins, expressed as the fraction of SCCP and MCCP as part of the total CPF, quantified in the SPM samples (2010/2011, n = 6) compared to sediment samples in the Oslo fjord (2004, n = 5; Arp and Breedveld, 2009), Aker River (Stations Ak, n = 5; Ranneklev et al., 2009) and Alna River (Stations Aln, n = 7; Ranneklev et al., 2009).
Toxicity
The results of the battery of seven bioassays show strong variation between the various assays. In general, the observed toxicity was higher in the 2010 SPM samples compared to 2011 (Supplementary Table S8). While the A. fischeri, TTR and AR response was highest for the May 2010 sample, was the ER response highest in March 2011. The AhR response showed the strongest variation, in the March 2010 sample both total and stable extracts was double the value for any other sample. Correcting for the amount of organic carbon in the SPM, under the assumption that this is the main sorbing phase for the contaminants responsible for the observed toxic response, did not change the overall picture. The SPM samples collected during snow melting in 2010 showed a clear stronger toxic response than the samples from the same period in 2011 (Supplementary Table S8).
Contaminant Load
The sediment transport in the river on a yearly basis was calculated by time integration of the weekly flow and SPM data. The geometric mean of the contaminant concentrations in the SPM across all sampling time points was calculated to determine the yearly contaminant flux as follows:
Where: Fi = contaminant flux i (g/yr); Ci,SPM = geometric mean of concentration i in SPM (µg/kg d.w.); FSPM = time integrated flux of SPM (kg/yr)
The calculated SPM transport in 2011 (803 ton/yr) was approximately twice the SPM transport in 2010, (434 ton/yr; Table 1). This difference was reflected in the estimated amount of PCB and CPF transported on a yearly basis (PCB7: 13 and 29 g/yr; CPF: 1.1 and 2.0 kg/yr for 2010 and 2011 respectively). However, in contrast to this, the amount of PAH15 transported by SPM only increased from 3.3 to just 4.0 kg/yr between 2010 and 2011. This reflects the lower PAH concentrations that were generally observed in the SPM in 2011 compared to 2010 (geometric mean 5.0 mg/kg d.w. vs. 7.5 mg/kg d.w. PAH15).
Discussion
Contaminant Levels
Grab samples represent the total water concentrations and thus include particulate matter (Lydy et al., 2014). This is reflected by the metal concentrations where a strong co-variation is observed for the Al and Fe concentrations. Metals naturally present in or associated with clay minerals that are suspended in the water column can explain the variable Cu and Zn concentrations (Birch, 2020). For Cd and Pb it is probable that other sources such as the restauration of older buildings and subsequent removal of old paint (Jartun et al., 2008), have contributed to the observed concentrations that exceed the mineral associated fraction.
The PAH concentrations found in the grab water samples are dominated by 3 and 4 ring PAH as observed by Meyer et al. (2011) in a creek in the vicinity of Toronto. However, in that study the maximum concentration of PAH9 was 4.5 μg/L , more than an order of magnitude higher than observed here (0.14 μg/L) which might be a result of the lower concentrations of SPM in Aker River (mg/L vs. g/L in Meyer et al. (2011). The PAH profile of the grab samples differed from the SPM samples by a distribution pattern dominated by 5- and 6- ring PAH in the latter (Figures 4, 5) as expected based on their partitioning behavior. This observation is in agreement with Meyer et al. (2011) who observed an increase in the fraction of high molecular PAH with increasing SPM concentrations in the creek. In several of the Aker River samples, the freely dissolved water concentration is similar to the total concentration (Supplementary Table S4). This is unexpected since the colloidal fraction that is present in the grab samples is believed to contribute significantly to the total amount of PAH (Enell et al., 2016). However, given that there are generally low levels of SPM in the river, the similarities in concentration could be due to this. In tunnel-wash water, a high fraction of particle associated PAH was found with a limited accessible (which can be considered as similar to the freely dissolved) fraction for PAH with 4-rings or more (Allan et al., 2016). PAH found in tunnel-wash water have been associated with a combination of traffic exhaust as well as wear of tires and asphalt pavement (Meland et al., 2010). These are probably the main sources of PAH in the SPM samples in the current study.
The concentrations of chlorinated paraffins in the SPM were much higher than previously reported for bottom sediment in the same river (Ranneklev et al., 2009). In the study by Ranneklev, maximum concentrations of SCCP and MCCP of 870 and 39 μg/kg d.w. respectively upstream of the present sampling site (STA) were reported, as compared to 5,875 and 17,400 μg/kg d.w. respectively in this study. Since the profiles are similar in all 6 samples, it is likely that there is one source that is responsible for the contamination detected in the river. In an adjacent river draining through a more industrialized area of Oslo (Alna River, Ranneklev et al., 2009) lower levels of SCCP were found (max. 150 μg/kg d.w.), whereas considerably higher concentrations of MCCP were reported (max. 830 μg/kg d.w.). In the present study the CPF profile was dominated by MCCP, similar to the Alna River (Figure 6). The concentrations determined in this study are very high compared to what has previously been reported for various environmental media. de Boer (2010) and van Mourik et al. (2016) compiled concentrations of CPF in soils and sediments reported in the literature and found generally concentrations in the 10s-100s µg/kg d.w. The SPM sample from August 2010 taken after a heavy rainfall event had a concentration of sum SCCP and MCCP of 24 mg/kg d.w. which is in the range as reported for sediments near wastewater treatment plants in the United Kingdom (Nicholls et al., 2001). The exact source of CPF in the samples here and in the literature, is not clear. Svanberg and Linden (1979) pointed out that CPF were being used as PCB replacements in many applications such as secondary plasticizers, oil additives and flame retardants following restrictions on the use of PCB. More recently, used car tires have been identified as an important source of chlorinated paraffins to the environment (Brandsma et al., 2019). It seems plausible that both these sources have contributed to the CPF concentrations determined in these samples.
Effect of Snow Melting and Extreme Precipitation
The results from the sampling campaigns clearly showed that a higher load of contaminants was present in the samples collected following snow melting. This was especially evident for the load of SPM as well as the total concentrations of metals in the water samples (Supplementary Figure S2). Westerlund and Viklander (2006) found that the particle load increased by a factor of five during snow melting and that there was a clear correlation of particle load with levels of Cd, Cu, Ni, Pb and Zn. This was also evident in the samples taken in this work, with the exception of Pb. An increased concentration of metals was seen in the samples taken during the summer, when concentrations normalized to Al to correct for the contribution of clay minerals. PAH transport has been reported to be strongly dominated by snow melting in an urban creek in the Toronto area (Meyer et al., 2011). PAH concentrations in snow were correlated with distance to roads and traffic intensity in Finland (Kuoppamäki et al., 2014) where a maximum concentration of 4.5 μg/L ΣPAH13 was reported adjacent to the road. Urban snow is yearly removed from the inner city of Oslo and stored on a landfill. Meltwater from the Oslo snow disposal site contained an average of 7.4 μg/L ΣPAH16 in 2009 (Bækken and Pettersen, 2009). Melting urban snow as an important source of PAH is also observed in this study.
Contrary to the effect of snow melting, there was no clear positive correlation between levels of suspended particulate matter and contaminant concentrations during rainfall events. In our study rainfall events resulted in dilution, reduced contaminant concentrations and an improved water quality. However, the total contaminant load transported to the fjord and harbor area increased due to higher discharge of the river (Table 1).
Toxicity Response to Contaminant Load
The various bioassays in the test battery show different response in the samples collected during the 6 events. This makes interpretation in relation to contaminant concentrations and loads complicated. This was observed in samples taken at different episodic run-off events in the rivers Rhine and Meuse (Hamers et al., 2015). They analyzed 31 SPM samples covering several high and low flow events in the period 1993 to 2011. Compared to the bioassay responses for these samples the Aker River SPM samples from 2010 showed a strong response for AR-EcoScreen and especially DR-Luc. The mean value of the Aker River results exceeded the 75th percentile in the value observed in the river Rhine and Meuse samples (Table 2). Especially the results of the AhR receptor assay for the “total” extract were high with an average value close to the maximum value observed in the river Rhine (42.1 vs. 50.6 ng TCDD-EQ/g d.w.) and exceeding the observed maximum value in the river Meuse (30.4 ng TCDD-EQ/g d.w.). In the stable extracts this was still seen in the 2010 SPM samples with a AhR activation response of 0.3-0.6 ng TCDD-EQ/g d.w compared to 19-107 ng TCDD-EQ/g d.w. in the “total” extracts. In the 2011 SPM samples the stable extracts showed an AhR activation response of 0.02-0.05 ng TCDD-EQ/g d.w. as compared to 20-40 ng TCDD-EQ/g d.w. in the total extracts. While the response observed in the stable extracts is considered to be a true dioxin-like activity, unstable compounds like PAH contribute to the bioassay response in the “total” extracts. The strong AhR receptor response in the March 2010 sample, both stable and total extracts, coincides with high levels of PAH16 in the SPM. Relative potency factors for this bioassay have been determined for 10 of the 16 PAH analyzed in the SPM (Machala et al., 2001). Only 2-4% of the AhR activation response in the “total” extracts can be explained by PAH analyzed here (Supplementary Table S8). However, road run-off has been shown to contain many PAH compounds not comprised by standard target analysis of PAH16. Grung et al. (2017) found that of the 50 PAH identified in road tunnel particulate matter only 5 compounds were comprised by the PAH16 target analysis.
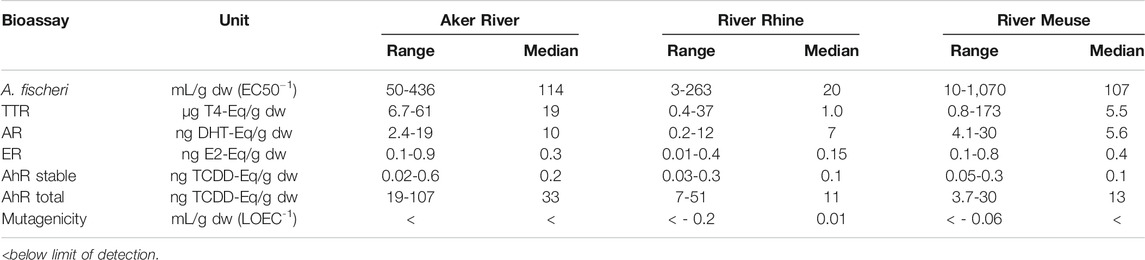
TABLE 2. Toxicity response in the SPM samples from Aker River (n = 6) compared with values from Hamers et al. (2015), river Rhine (n = 19), river Meuse (n = 12).
Influence of Riverine Run-off on the Sediment Quality in the Oslo Fjord
The hypothesis of this work was that increased surface run-off caused by weather conditions such as snow melting, and extensive rainfall events would increase the run-off of chemicals sorbed to the suspended particulate matter from the urban environment into receiving waters. Concentrations of selected contaminants found in the top 5 cm of sediments in the Oslo fjord near the outlet of the Aker River (measured in 2004) show PAH and PCB profiles identical to those observed in the SPM sampled in 2010 and 2011. The upper 5 cm of sediments sampled in 2004 represent sediments deposited during the period of 1999-2004 (Arp and Breedveld, 2009). This indicates that the main sources of the contaminants released from the urban environment may have been the same over this time span. However, median concentrations in the fjord sediments were 20 mg/kg d.w. PAH15 and 105 μg/kg d.w. PCB7, while concentrations in SPM were 5 mg/kg d.w. and 35 μg/kg d.w. respectively. This difference indicates a reduction in the contaminant load over the approximately 10-year period covered by these sampling campaigns and could be due to stricter regulation of these chemicals which has taken place over the same time span, like diesel engine exhaust (EU, 2002) and wood-burning stoves (NS-EN 13240, 2001).
Chlorinated paraffin profiles in the fjord sediments were dominated by SCCP (81%), while the SPM was dominated by MCCP (81%). This is similar to the finding of Ranneklev et al. (2009) who report that bottom sediments in the Aker River were dominated by SCCP (87%), while sediments in an adjacent river, Alna, were dominated by MCCP (60%). This supports the fact that the use of SCCP in Norway has significantly reduced owing to the ban put in to place in 2002 (Norwegian Ministry of Environment, 2001). Median concentration of the sum of chlorinated paraffins were 2.5 mg/kg d.w. in fjord sediments (Arp and Breedveld, 2009), identical to what was observed in SPM samples in Aker River (2.6 mg/kg d.w.). In the river bed samples, much lower concentrations were found (median 181 and 266 μg/kg d.w. for rivers Aker and Alna respectively, Ranneklev et al., 2009), indicating that SPM is the dominating transport vector for these compounds. This might be caused by the fact that the organic carbon content in the river bed samples (median 0.75%, n = 9) generally is much lower than in the SPM (median 13.1%, n = 6).
Conclusion
This study has shown that strong episodic changes in the concentration of contaminants in SPM and their toxic response are possible in river run-off to a fjord. Despite these episodic changes, major changes in the average contaminant load to the fjord are not always evident, as long as the release rates from the principle sources of these contaminants are constant. Monitoring trends in sediment quality in the coastal environment can be a feasible way to quantify the long-term contribution from urban contaminant sources to the coastal environment. It remains a challenge to ensure that every peak event is sampled in a dynamic river system. More “event-based” water quality data are needed to improve insights into these processes and their toxicological significance for the aquatic ecosystem.
Data Availability Statement
The original contributions presented in the study are included in the article/Supplementary Material, further inquiries can be directed to the corresponding author.
Author Contributions
GB: Projectleader and principal author of manuscript MH: Responsible for planning and performing fieldwork as well as data presentation in manuscript SH: Sample analysis and contribution to data interpretation in manuscript IA: SPM sampling and preparation as well as input to manuscript discussion TH: Toxicity testing as well as interpretation and discussion in manuscript.
Funding
This research has been supported by the Interreg IVB North Sea Region Program (2007–2013) through project: Impact of Climate Change on the Quality of Urban and Coastal Waters –Diffuse Pollution (DiPol) as well as Norwegian research council project 300560 NATWIP, part of the JPI project “Nature based solutions for water management in the peri urban; linking ecological, social and economic dimensions.”
Conflict of Interest
The authors declare that the research was conducted in the absence of any commercial or financial relationships that could be construed as a potential conflict of interest.
Acknowledgments
The authors acknowledge Mike Denison (University of California, Davis) for providing us with the VM7Luc4E2 and H4L1.1c4 reporter gene cell lines, Pim Leonards for his valuable help in the analyses of contaminants in SPM, Peter Cenijn, Jorke Kamstra and Eke Rijkmans for performing part of the bioassays (VU, Amsterdam). Richard St. Louis contributed with carbon and nitrogen elemental analyses of the SPM samples (ISMER, University of Quebec at Rimouski).
Supplementary Material
The Supplementary Material for this article can be found online at: https://www.frontiersin.org/articles/10.3389/fenvs.2021.601300/full#supplementary-material.
References
Allan, I. J., O'Connell, S. G., Meland, S., Bæk, K., Grung, M., Anderson, K. A., et al. (2016). PAH accessibility in particulate matter from road-impacted environments. Environ. Sci. Technol. 50 (15), 7964–7972. doi:10.1021/acs.est.6b00504
Araki, N., Ohno, K., Takeyoshi, M., and Iida, M. (2005). Evaluation of a rapid in vitro androgen receptor transcriptional activation assay using AR-EcoScreen cells. Toxicol. In Vitro 19 (3), 335–352. doi:10.1016/j.tiv.2004.10.008
Arp, H. P., and Breedveld, G. D. (2009). Environment Agency Report TA-2479. Nye miljøgifter i sedimentprøver indre Oslofjord - kjemiske profiler i Bispevika/Bjørvika. Available at: https://www.miljodirektoratet.no/publikasjoner/publikasjoner-fra-klif/2008/desember/nye-miljogifter-i-sedimentprover-indre-oslofjord.--kjemiske-profiler-i-bispevikabjorvika/. accessed 06 July 2020
Bækken, T., and Pettersen, E. (2009). Avrenning fra snødeponiet ved Åsland Overvåkning av forurensninger tilført renseanlegg, bekker og drikkevannsbrønner i 2009. Available at: https://niva.brage.unit.no/niva-xmlui/handle/11250/214787. accessed 06 July 2020
Birch, G. F. (2020). An assessment of aluminum and iron in normalisation and enrichment procedures for environmental assessment of marine sediment. Sci. Total Environ. 727, 138123. doi:10.1016/j.scitotenv.2020.138123
Brandsma, S. H., Brits, M., Groenewoud, Q. R., Van Velzen, M. J., Leonards, P. E., and De Boer, J. (2019). Chlorinated paraffins in car tires recycled to rubber granulates and playground tiles. Environ. Sci. Technol. 53 (13), 7595–7603. doi:10.1021/acs.est.9b01835
Cornelissen, G., Gustafsson, Ö., Bucheli, T. D., Jonker, M. T., Koelmans, A. A., and van Noort, P. C. (2005). Extensive sorption of organic compounds to black carbon, coal, and kerogen in sediments and soils: mechanisms and consequences for distribution, bioaccumulation, and biodegradation. Environ. Sci. Technol. 39 (18), 6881–6895. doi:10.1021/es050191b
Cornelissen, G., Pettersen, A., Broman, D., Mayer, P., and Breedveld, G. D. (2008). Field testing of equilibrium passive samplers to determine freely dissolved native polycyclic aromatic hydrocarbon concentrations. Environ. Toxicol. Chem. 27, 499–508. doi:10.1897/07-253.1
Enell, A., Lundstedt, S., Arp, H. P., Josefsson, S., Cornelissen, G., Wik, O., et al. (2016). Combining leaching and passive sampling to measure the mobility and distribution between porewater, DOC, and colloids of native oxy-PAHs, N-PACs, and PAHs in historically contaminated soil. Environ. Sci. Technol. 50 (21), 11797–11805. doi:10.1021/acs.est.6b02774
EU (2002). Commission Directive 2002/80/EC of 3 October 2002 adapting to technical progress Council Directive 70/220/EEC relating to measures to be taken against air pollution by emissions from motor vehicles, Technical Paper 32002L0080. The Council of the European Communities
French, H., and Binley, A. (2004). Snowmelt infiltration: monitoring temporal and spatial variability using time-lapse electrical resistivity. J. Hydrol. 297 (1-4), 174–186. doi:10.1016/j.jhydrol.2004.04.005
Garrison, P. M., Tullis, K., Aarts, J. M., Brouwer, A., Giesy, J. P., and Denison, M. S. (1996). Species-specific recombinant cell lines as bioassay systems for the detection of 2,3,7,8-tetrachlorodibenzo-p-dioxin-like chemicals. Fundam. Appl. Toxicol. 30 (2), 194–203. doi:10.1093/toxsci/30.2.194
Grung, M., Kringstad, A., Bæk, K., Allan, I. J., Thomas, K. V., Meland, S., et al. (2017). Identification of non-regulated polycyclic aromatic compounds and other markers of urban pollution in road tunnel particulate matter. J. Hazard. Mater. 323, 36–44. doi:10.1016/j.jhazmat.2016.05.036
Hale, S. E., Elmquist, M., Brändli, R., Hartnik, T., Jakob, L., Henriksen, T., et al. (2012). Activated carbon amendment to sequester PAHs in contaminated soil: a lysimeter field trial. Chemosphere 87 (2), 177–184. doi:10.1016/j.chemosphere.2011.12.015
Hamers, T., Kamstra, J. H., Sonneveld, E., Murk, A. J., Kester, M. H., Andersson, P. L., et al. (2006). In vitro profiling of the endocrine-disrupting potency of brominated flame retardants. Toxicol. Sci. 92 (1), 157–173. doi:10.1093/toxsci/kfj187
Hamers, T., Kamstra, J. H., van Gils, J., Kotte, M. C., and van Hattum, A. G. M. (2015). The influence of extreme river discharge conditions on the quality of suspended particulate matter in Rivers Meuse and Rhine (Netherlands). Environ. Res. 143, 241–255. doi:10.1016/j.envres.2015.10.019
Hamers, T., Smit, M. G., Murk, A. J., and Koeman, J. H. (2001). Biological and chemical analysis of the toxic potency of pesticides in rainwater. Chemosphere 45 (4-5), 609–624. doi:10.1016/S0045-6535(01)00017-0
Hanssen-Bauer, I., Førland, E. J., Haddeland, I., Hisdal, H., Mayer, S., Nesje, A., et al. (2017). NCCS Report No.: 1/2017. Climate in Norway 2100–a knowledge base for climate adaptation, 204.
Jartun, M., Ottesen, R. T., Steinnes, E., and Volden, T. (2008). Runoff of particle bound pollutants from urban impervious surfaces studied by analysis of sediments from stormwater traps. Sci. Total Environ. 396 (2-3), 147–163. doi:10.1016/j.scitotenv.2008.02.002
Jonker, M. T., and Koelmans, A. A. (2001). Polyoxymethylene solid phase extraction as a partitioning method for hydrophobic organic chemicals in sediment and soot. Environ. Sci. Technol. 35 (18), 3742–3748. doi:10.1021/es0100470
Kessler, S., Pohlert, T., Breitung, V., Wilcsek, K., and Bierl, R. (2020). Comparative evaluation of four suspended particulate matter (SPM) sampling devices and their use for monitoring SPM quality. Environ. Sci. Pollut. Res. Int. 27 (6), 5993–6008. doi:10.1007/s11356-019-07314-0
Korytár, P., Parera, J., Leonards, P. E. G., Santos, F. J., De Boer, J., and Brinkman, U. A. T. (2005). Characterization of polychlorinated n-alkanes using comprehensive two-dimensional gas chromatography--electron-capture negative ionisation time-of-flight mass spectrometry. J. Chromatogr. A. 1086 (1-2), 71–82. doi:10.1016/j.chroma.2005.05.003
Kuoppamäki, Kirsi., Setälä, Heikki., Rantalainen, Anna-Lea., and Kotze, D. Johan. (2014). Urban snow indicates pollution originating from road traffic. Environ. Pollut. 195, 56–63. doi:10.1016/j.envpol.2014.08.019
Lydy, M. J., Landrum, P. F., Oen, A. M., Allinson, M., Smedes, F., Harwood, A. D., et al. (2014). Passive sampling methods for contaminated sediments: state of the science for organic contaminants. Integr. Environ. Assess. Manag. 10, 167–178. doi:10.1002/ieam.1503
Machala, M., Vondrácek, J., Bláha, L., Ciganek, M., and Neca, J. V. (2001). Aryl hydrocarbon receptor-mediated activity of mutagenic polycyclic aromatic hydrocarbons determined using in vitro reporter gene assay. Mutat. Res. 497 (1-2), 49–62.doi:10.1016/S1383-5718(01)00240-6
Meland, S., Borgstrøm, R., Heier, L. S., Rosseland, B. O., Lindholm, O., and Salbu, B. (2010). Chemical and ecological effects of contaminated tunnel wash water runoff to a small Norwegian stream. Sci. Total Environ. 408, 4107–4117. doi:10.1016/j.scitotenv.2010.05.034
Meyer, T., Lei, Y. D., and Wania, F. (2011). Transport of polycyclic aromatic hydrocarbons and pesticides during snowmelt within an urban watershed. Water Res. 45, 1147–1156. doi:10.1016/j.watres.2010.11.004
Meyer, T., and Wania, F. (2011). Modeling the elution of organic chemicals from a melting homogeneous snow pack. Water Res. 45, 3627–3637. doi:10.1016/j.watres.2011.04.011
Nicholls, C. R., Allchin, C. R., and Law, R. J. (2001). Levels of short and medium chain length polychlorinated n-alkanes in environmental samples from selected industrial areas in England and Wales. Environ. Pollut. 114 (3), 415–430. doi:10.1016/S0269-7491(00)00230-X
Norwegian Ministry of Environment (2001). Forskrift om kortkjedete klorparaffiner. Available at: https://lovdata.no/dokument/LTI/forskrift/2000-12-13-1544. accessed 06 July 2020
Noyes, P. D., McElwee, M. K., Miller, H. D., Clark, B. W., Van Tiem, L. A., Walcott, K. C., et al. (2009). The toxicology of climate change: environmental contaminants in a warming world. Environ. Int. 35 (6), 971–986. doi:10.1016/j.envint.2009.02.006
NS-EN 13240 (2001). Roomheaters fired by solid fuel — requirements and test methods. Brussels, Belgium: European Committee for Standardization (CEN).
NS-EN-ISO/IEC 17025 (2005). General requirements for the competence of testing and calibration laboratories. Geneva, Switzerland: International Organization for Standardization.
Oslo Kommune (2017). Faktaark oslos byvassdrag. Available at: http://www.osloelveforum.org/wp-content/uploads/2015/10/Faktaark-Oslos-byvassdrag-2017.pdf. accessed 06 July 2020
Pohlert, T., Hillebrand, G., and Breitung, V. (2011). Effects of sampling techniques on physical parameters and concentrations of selected persistent organic pollutants in suspended matter. J. Environ. Monit. 13 (6), 1579. doi:10.1039/C1EM10045A
Ranneklev, S., Allan, I., and Enge, E. K. (2009). Environment Agency Report TA2495. Kartlegging av miljøgifter i Alna og Akerselva. Available at: https://niva.brage.unit.no/niva-xmlui/handle/11250/214480. accessed 06 July 2020
Reifferscheid, G., Maes, H. M., Allner, B., Badurova, J., Belkin, S., Bluhm, K., et al. (2012). International round-robin study on the Ames fluctuation test. Environ. Mol. Mutagen 53 (3), 185–197. doi:10.1002/em.21677
Rogers, J. M., and Denison, M. S. (2000). Recombinant cell bioassays for endocrine disruptors: development of a stably transfected human ovarian cell line for the detection of estrogenic and anti-estrogenic chemicals. In Vitro Mol. Toxicol. 13 (1), 67–82.
Svanberg, O., and Linden, E. (1979). Chlorinated paraffins: an environmental hazard?. Ambio 8 (5), 206–209.
van Mourik, L. M., Gaus, C., Leonards, P. E. G., and de Boer, J. (2016). Chlorinated paraffins in the environment: a review on their production, fate, levels and trends between 2010 and 2015. Chemosphere 155, 415–428. doi:10.1016/j.chemosphere.2016.04.037
Viklander, M. (1999). Substances in urban snow. a comparison of the contamination of snow in different parts of the city of Luleå, Sweden. Water Air Soil Pollut. 114 (3-4), 377–394. doi:10.1023/A:1005121116829
Keywords: particulate matter, PAH, chlorinated paraffins, snow-melting, rain, climate change, sediments, bioassays
Citation: Breedveld GD, Hansen MC, Hale SE, Allan IJ and Hamers T (2021) Effect of Extreme Weather Events on Contaminant Transport From Urban Run-Off to a Fjord System. Front. Environ. Sci. 9:601300. doi: 10.3389/fenvs.2021.601300
Received: 31 August 2020; Accepted: 26 January 2021;
Published: 04 March 2021.
Edited by:
Peter S Hooda, Kingston University, United KingdomReviewed by:
Clare Alexandra Wilson, University of Stirling, United KingdomØyvind Mikkelsen, Norwegian University of Science and Technology, Norway
Copyright © 2021 Breedveld, Hansen, Hale, Allan and Hamers. This is an open-access article distributed under the terms of the Creative Commons Attribution License (CC BY). The use, distribution or reproduction in other forums is permitted, provided the original author(s) and the copyright owner(s) are credited and that the original publication in this journal is cited, in accordance with accepted academic practice. No use, distribution or reproduction is permitted which does not comply with these terms.
*Correspondence: Gijs D. Breedveld, Z2JyQG5naS5ubw==