- 1Key Laboratory of State Forestry and Grassland Administration on Soil and Water Conservation, Beijing Forestry University, Beijing, China
- 2Engineering Research Center of Forestry Ecological Engineering, Ministry of Education, Beijing Forestry University, Beijing, China
- 3Yanchi Research Station, School of Soil and Water Conservation, Beijing Forestry University, Beijing, China
Revegetation is regarded as an effective means to improve the ecological environment in deserts and profoundly influences the potential ecological functions of the soil fungal community. Therefore, Illumina high-throughput sequencing was performed to characterize the soil fungal diversity and community composition at two soil depths (0–10 cm and 10–20 cm) with four revegetation durations (natural grassland, half-mature, nearly mature, and mature Pinus. sylvestris var. mongolica plantations) in the Mu Us Sandy Land, China. The effects of soil properties on soil fungal communities were also examined to reveal the connection between fungal function and soil environment. The results indicated that 1) soil nutrient content and enzyme activity showed significant differences through the restoration durations, 2) there was no significant effect of soil depth on soil fungal diversity, while the Shannon diversity index of all fungal communities was significantly different among different revegetation durations, 3) compared with grassland, ectomycorrhizal fungi (notably, Inocybe, Tuber, and Calostoma) were abundant in plantations. The endophyte fungus Mortierella was among the top 10 genera in all soil samples and arbuscular mycorrhizal fungus Diversispora was the indicator genus of the grassland, and 4) catalase and total nitrogen were the main factors affecting fungal community composition and were closely related to saprotrophs and pathotrophs, respectively. This new information indicates the variation of soil fungal communities along revegetation durations and highlights the interaction between fungal functions and desert ecosystems.
Introduction
Due to long-term and unreasonable land use, the native land has suffered serious ecological degradation and water and soil erosion, which has resulted in the loss of ecological function and services (Nearing et al., 2017; Deng et al., 2019). Revegetation is considered an effective way to impart ecological benefits (Nunez-Mir et al., 2015; Xiao et al., 2015), including improving the quality of soil and vegetation coverage (De Deyn and Van der Putten, 2005; Wang et al., 2012), rehabilitating degraded environments (Jing et al., 2014), and accelerating the recovery of degraded lands (Qin et al., 2019). Vegetation restoration enhances the ecological stability in restored lands along with the fosterage of soil microbial community succession (Zhang et al., 2017b; Guo et al., 2018).
Soil microbial communities play pivotal roles by maintaining ecosystem functioning and stability in response to changes in soil environment (Kubartova et al., 2012). It is logical that they are expected to be more important than physico-chemical characters and may potentially serve as primary signals for soil health and productivity (Cardinale et al., 2006; Shen et al., 2010). Fungi perform different ecological functions according to certain trophic strategies (Nguyen et al., 2016). Saprotrophs participate in the mineralization of organic matter, while pathotrophs may induce plant diseases (Bardgett and van der Putten, 2014). Symbiotrophs promote plants’ ability to absorb nutrients and increase resistance to drought, heavy metals, diseases, and insect pests (Liu et al., 2007; Peay et al., 2013). The diversity and distribution of the functional groups in soil fungi determine the ecological effects of soil fungal communities, which are of great significance for the revegetation process under stress (Jin et al., 2016; Detheridge et al., 2018; Wang et al., 2020).
The temporal variation of the soil fungal community along the revegetation duration is mainly caused by abiotic factors and vegetation characteristics. Soil conditions directly influence soil fungal diversity and community composition (Marín et al., 2017; Nie et al., 2018). For example, in a global context, soil pH is the primary driver of fungal community structure and function (Tedersoo et al., 2014) and water availability may increase soil fungal community diversity and composition (Brockett et al., 2012; Zheng et al., 2017). As key components involved in carbon and nutrient cycling, functional composition of soil fungi is closely related to soil nutrients (Peay et al., 2016; Schlatter et al., 2018). Previous studies have also confirmed that soil fungi are more sensitive to soil enzyme activity than other microbial populations (Stursova et al., 2016).
The vegetation characteristics mainly include aboveground vegetation species (Pickles et al., 2015; Toju et al., 2018), species richness and diversity of vegetation (Chen et al., 2017; Francioli et al., 2020), and stand age (Miao et al., 2019; Guo et al., 2020b). In the early stage, revegetation substantially improves the physiological activities of soil fungi and changes the fungal community structure (Liu et al., 2019a; Yang et al., 2020). With the maturation of vegetation, the compositions of mycorrhizal and saprotrophic fungi are altered due to resource availability that change with plant litter, exudates, and soil properties (Husband et al., 2002; Broeckling et al., 2008; Llado et al., 2018). Therefore, it is important to elucidate the connection between revegetation duration and fungal functional groups and find the role of soil environment in driving fungal community dynamics during revegetation.
The Mu Us Sandy Land is a classical semi-arid region in Northern China, characterized by poor soil, severe nutrient depletion, scarce resources, and limited water availability. It suffers from serious water and soil erosion, hence, ecological revegetation is urgently needed. Pinus. sylvestris var. mongolica (P. sylvestris) originating from the Hulunbuir Sandy Land was successfully introduced into the Mu Us Sandy Land and this contributed significantly to ecological restoration (Lyu et al., 2020). So far, the changes in the below-ground microbial community along revegetation durations, particularly in a desert ecosystem remain unclear, and should be elucidated to generate an understanding of the sustainable development associated with long-term vegetation restoration and natural restoration.
Therefore, to investigate the soil fungal diversity and functional composition responses to revegetation durations, Illumina high-throughput sequencing and FUNGuild platform were used to examine soil fungal taxonomic and functional compositions at different soil depths (0–10 cm and 10–20 cm) during revegetation durations (natural grassland, half-mature, nearly mature, and mature P. sylvestris plantations). We hypothesized that the variation in fungal diversity and community composition was largely induced by restoration stages rather than soil depths, and that soil properties may help explain these different responses. In addition, we predicted that the potential ecological functions of some keystone/indicator fungal species were different during the revegetation durations and they further helped to reveal soil conditions and quality. Based on these hypotheses, the objectives of this study were to examine how soil fungal functional groups respond to different revegetation durations and develop their potential functions and to further identify which factors drive the dynamics of the fungal community.
Materials and Methods
Study Site
The study site was located in the Hongshixia Sandy Botanical Garden, which is at the southern edge of the Mu Us Sandy Land (38°26′ N, 109°12′ E; 1080 m elevation), Shaanxi Province (Fig. S1). The region has a warm temperate continental monsoon climate with an average annual temperature of 9.1°C, average annual precipitation of 385.5 mm, and average annual evaporation of 2502 mm. The soil was classified as an azonal aeolian sandy soil with loose topsoil structure and poor water retention. The predominant vegetation types were P. sylvestris, Artemisia sacrorum, Tribulus terrestris, Bidens pilosa, and Agropyron cristatum.
Sample Collection
Sample collection was carried out in August 2017, with peak microbial activity in the growth season. Four 50 m × 50 m revegetation duration plots, one grassland plot, and three P. sylvestris plantation plots, that did not have significant interference from nurturing practices and human activities were chosen for the study. The grassland plot (natural grassland, MUg) was regarded as a control, where the understory herbs were most similar to plantations. Initially, the three plantation plots were replanted with 3-year-old P. sylvestris saplings. With revegetation durations of 23, 29, and 40 years, the stand ages were 26, 32, and 43 years, which represented half-mature (MUh), nearly mature (MUn), and mature (MUm) forests, respectively.
In grassland, three random 1 m × 1 m quadrats were selected for inventory. In plantations, three standard trees of P. sylvestris were randomly chosen after measuring individual tree height, diameter at breast height, and crown width. The sampling points were the center in the quadrats and standard trees, respectively. Each sampling point was at least 10 m from the others. Three composite samples (consisting of four replicate soil samples from four directions at a sampling point) were collected from each plot. The vegetation and litter from the ground were removed to collect soil samples at a depth of 0–10 cm (a) and 10–20 cm (b) (Table 1). Therefore, all 24 samples from the plots (four revegetation duration plots × two soil depths × three composite samples) were packaged and labeled. Each soil sample was divided into three parts and subjected to three different procedures: 1) Take back directly to determine soil water content (SWC), soil pH, soil organic matter (SOM), total nitrogen (TN), and total phosphorus (TP). 2) Keep in a portable thermostat at 4–6°C to analyze soil catalase, urease, and phosphatase enzyme activities. 3) Store at −80°C for gene sequencing.
Soil Property Analysis
SWC was measured gravimetrically after drying the soil samples in an oven at 105°C for 12 h. Soil pH was determined from a 1:2.5 mixture of soil and water using a PHS-3E pH meter (INESA, Shanghai, China). SOM was obtained using the K2Cr2O7-H2SO4 oxidation technique (Walkley and Black, 1934). An automated chemical analyzer (Smartchem 200, Italy) was used to analyze TN and TP based on the indophenol-blue spectrophotometric method and Mo-Sh anti-colorimetric analysis method, respectively (John and Matt, 1970; Mason et al., 1999). All enzyme activities were tested through antitheses, without soil and substrate (Gianfreda et al., 2005). Catalase activity was measured by performing potassium permanganate titration and expressed in milliliters of potassium permanganate devoured per hour per gram of soil. Urease and phosphatase activity were determined using a programmed microplate reader (SpectraMax Paradigm, Molecular Devices, San Jose, CA, United States), and expressed as the sum of the enzyme required to convert 1 mM of substrate in 1 min (Tarafdar and Marschner, 1994).
Sequence Data Analysis
DNA was extracted from 0.4 g soil using the PowerSoil DNA isolation kit (Mo Bio Laboratories, Carlsbad, CA, United States) according to the manufacturer's instructions. DNA concentration was quantified using a Nanodrop spectrophotometer (Thermo Scientific, United States). The fungal ITS1 region was amplified using the primers ITS1F (5’-CTTGGTCATTTAGACGAAGTAA-3') and ITS2 (5’-GCTGCGTTCTTCATCGATGC-3’) (Op De Beeck et al., 2014). The AXYGEN Gel Extraction Kit (QIAGEN, Germany) and qPCR were used to detect and quantify the PCR amplification products. After gel imaging, the qualified PCR products were sent to Allwegene Technology Inc. for sequencing using the Illumina MiSeq sequencing system (Illumina, San Diego, CA, United States).
Trimmomatic and FLASH were used to filter the quality and merge the raw fastq files and sequences with quality scores >20 and <50 bp were used for analysis (Zhou et al., 2019; Guo et al., 2020a). Chimeric sequences were removed using the Usearch software package (Version 8.1.1861, http://www.drive5.com/usearch/). Sequences with 97% similarity were classified as operational taxonomic units (OTUs) (Edgar, 2013). To reduce the error caused by the different data sizes, all samples were normalized to 21,260 sequences. The Ribosomal Database Project (RDP) classifier was used to perform taxonomic assignment. Assigned taxa were verified using NCBI BLAST (https://www.ncbi.nlm.nih.gov/). All raw sequence information was deposited in GenBank (SRA accession: PRJNA663124).
Fungal functional guilds were identified using the FUNGuild platform (http://www.stbates.org/guilds/app.php) (Nguyen et al., 2016). Initially, the results with groups “highly probable” or “probable” were accepted and ambiguous groups were confirmed by previous literature findings (He et al., 2017). In this study, the fungal trophic modes were divided into symbiotroph, saprotroph, pathotroph, and combined trophic modes, which included 14 guilds. For combined trophic modes and combined guilds, they were incorporated into “Other fungi” and “Other symbiotroph/saprotroph/pathotroph fungi” groups respectively.
Data Analysis
One-way analysis of variance (ANOVA) with Duncan's multiple range test was used to determine if a significant difference existed among soil variables, while two-way ANOVA with post-hoc Tukey’s tests was used to compare soil properties among soil depths and revegetation durations. All analyses were performed using SPSS 20.0 at the 0.05 significance level. Pearson's correlation was used to determine the relationship of soil variables with R-3.6.1.
For the convenience of description, we classified soil fungi according to their relative abundance as follows: dominant (>10.00%), common (10.00%–1.00%), and rare genera (<1.00%). Alpha diversities (richness and Shannon indices) based on OTU data were calculated using the ‘vegan’ package in R-3.6.1. The soil fungal functional groups and diversity indices were visualized using Origin 2018. Indicator species showed an ecological preference for one or a few habitat types, which were used as a prediction index for environmental changes (Cáceres and Legendre, 2009). The indicator genus of soil fungi was identified to further elucidate the distribution of soil fungi at different soil depths and revegetation durations, which were implemented using the ‘indicspecies’ and ‘labdsv’ packages in R-3.6.1. The contribution of soil depth and revegetation duration on fungal functional communities were analyzed by using Permutational multivariate analysis of variance (PERMANOVA) with the ‘vegan’ package in R-3.6.1.
To explain the relationship between soil properties and soil fungal functional groups, all soil properties were converted to a matrix to perform Mantel test with fungal functional groups and a visual correlation was performed using the ‘corrplot’ package in R-3.6.1. Subsequently, canonical correlation analysis (CCA) was performed and significance of each environmental variable was analyzed using Canoco 4.5.
Results
Variations in Soil Properties due to Soil Depths and Revegetation Durations
The soil properties varied with soil depth and revegetation duration (Figure 1; Supplementary Table S1). In the P. sylvestris plantation, the SWC and pH in the 0–10 cm layer were higher than those in the 10–20 cm layer, while the opposite results were observed in grasslands (Figure 1). According to the two-way ANOVA, soil nutrients (SOM, TN, and TN) and enzyme activities (catalase, urease, and phosphatase) were significantly different among revegetation duration (p < 0.05; Supplementary Table S1). Between soil depths, significant differences were noted in the content of SOM, TN, and TP (except TP in grassland), with a higher nutrient content observed in upper soil (p < 0.05; Figure 1). In contrast, the effect of soil depth on catalase and urease activities was not significant (p > 0.05), and all enzyme activities had the highest values in mature forests (Supplementary Table S1). In addition, a strong positive correlation was observed between phosphatase and TN (p < 0.05), and a negative correlation was noted between catalase and SWC (p < 0.05; Supplementary Table S2).
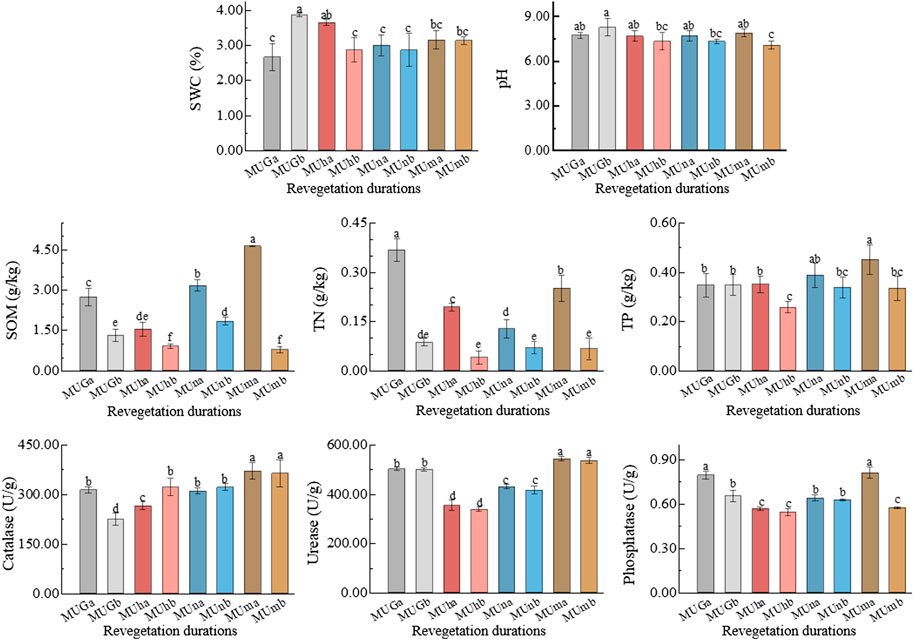
FIGURE 1. Soil properties in different soil depths trough revegetation durations. Different letters are significantly different (p < 0.05).
Soil Fungal Community Diversity
In total, 831,489 high-quality sequences were clustered into 1,355 OTUs from all soil samples. There was no significant difference in richness index of all fungal communities, saprotrophs, and pathotrophs at different soil depths or revegetation durations (Supplementary Table S3; Supplementary Table S4). Soil depth did not have a significant impact on Shannon diversity (p > 0.05; Supplementary Table S4), and values of Shannon indices first decreased and then increased during restoration durations (Figure 2). The Shannon index showed no significant difference between different soil depths (p > 0.05; Supplementary Table S4), however, it first decreased and then increased during restoration durations (Figure 2). The Shannon and richness indices of symbiotrophs significantly correlated with revegetation duration (p < 0.05; Supplementary Table S4). For the alpha diversity indices of the three trophic modes, the highest values were noted in saprotrophs, followed by symbiotrophs, and lowest in pathotrophs.
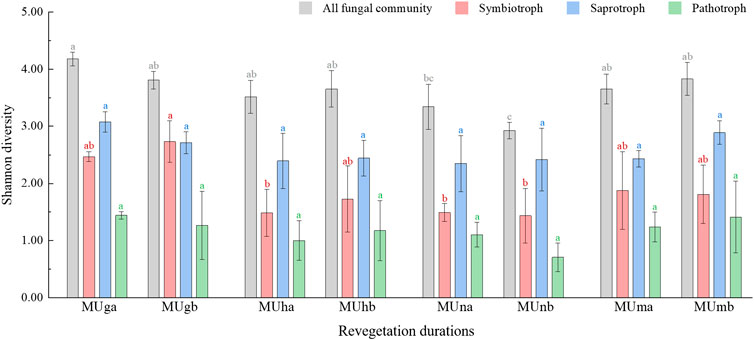
FIGURE 2. Shannon index of all fungi community and functional groups in different soil depths trough revegetation durations. Different letters are significantly different (p < 0.05).
The dissimilarities in soil fungal composition among different sampling plots mainly associated with revegetation duration rather than soil depth (Supplementary Table S5). Between soil depths, the structure showed no significant difference in all fungal communities or functional groups (p > 0.05), while revegetation duration significantly distinguished the fungal community composition (p < 0.05; Supplementary Table S5).
Soil Fungal Community Composition of Taxonomic and Functional Groups
All fungal OTUs were assigned to 12 phyla, 40 classes, 102 orders, 202 families, and 339 genera. Ascomycota was the dominant phylum with the highest relative abundance (60.32–79.81%), followed by Basidiomycetes (5.33–30.01%) and Mortierellomycota (1.44–10.03%; Figure 3A). The proportional distribution of phyla was relatively stable (Supplementary Table S6) and their relative abundance between soil depths showed no significant difference (p > 0.05); only Basidiomycota showed significant differences through restoration durations (p < 0.05; Supplementary Table S6).
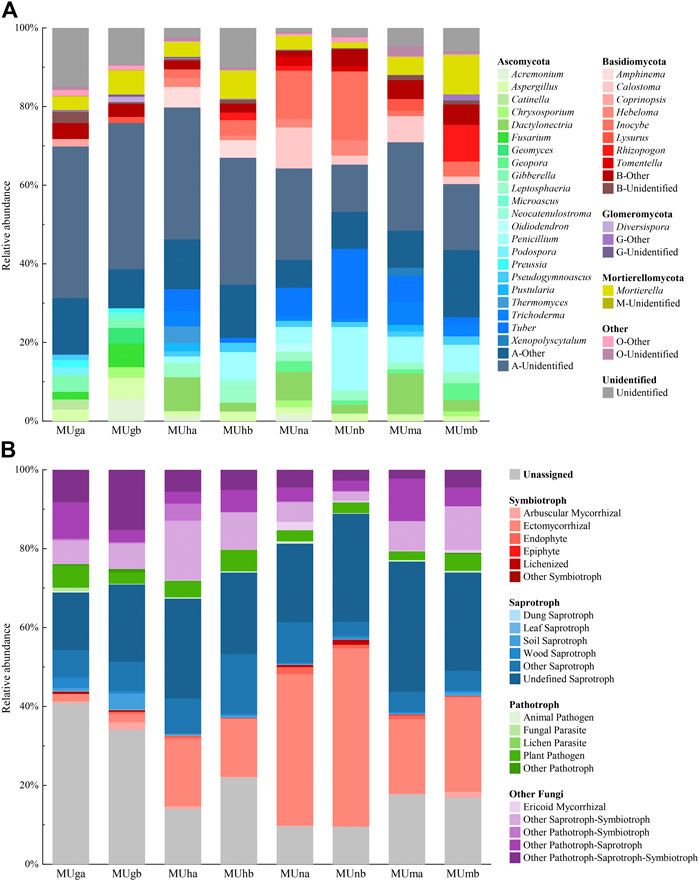
FIGURE 3. Distribution and relative abundance of soil fungal (A) taxonomic and (B) functional groups in different soil depths trough revegetation durations. Genera>1.00% were listed.
The composition of functional groups was similar in each soil depth and restoration duration, however, the corresponding relative abundance fluctuated significantly (Figure 3B). Soil depths barely affected the relative abundance of functional groups, however, an opposite trend appeared in restoration durations (Supplementary Table S6). Compared with plantations, grasslands contained more unassigned fungal functional groups and fewer symbiotrophs. In plantations, saprotrophs were the dominant fungal functional groups in half-mature and mature forests, while symbiotrophs were dominant in nearly mature forests. Ectomycorrhizal (EcM) fungi constituted the most predominant portion of symbiotrophs and a small amount of arbuscular mycorrhizal (AM) and ericoid mycorrhizal fungi were observed. Plant pathogens were the main pathotrophs (Figure 3B).
The dominant and common genera in grasslands were different from those observed in plantations (Supplementary Table S7). In grasslands, there were no dominant and the common genera mostly belonged to saprotrophs, while the majority of dominant and common genera in plantations possessed symbiotic function. The endophyte fungus Mortierella was among the top 10 genera in all soil samples. The plant pathogen Gibberella and combined trophic modes fungi Fusarium were the top 10 genera only in grasslands and both were identified as indicator genera. In plantations, the relative abundance of Inocybe and Tuber was greater than 1.00%. In the nearly mature forest, EcM fungi Inocybe (14.92%) was the dominant genus and Tomentella (1.80%) was a common genus, and both were indicator genera. Dactylonectria had a higher relative abundance in topsoil and was regarded as an indicator genus of topsoil.
Relationship Between Fungal Communities and Soil Environment Factors
At different depths, soil fungal community composition through revegetation durations was driven by soil properties (Figure 4; Figure 5). Soil properties explained 40.7% of the variation in soil fungal distribution (Figure 4). Of these, SOM (p = 0.025), TN (p = 0.043), and phosphatase (p = 0.003) had a stronger impact on fungal composition, which was mainly related to topsoil in grassland and subsoil in mature forests. Catalase (p = 0.012) played an important role in the composition of topsoil in plantations. Furthermore, saprotrophs were significantly affected by SWC (p < 0.05) and catalase (p < 0.05; Figure 5). Pathotrophs showed a significant positive correlation with TN (p < 0.05; Figure 5).
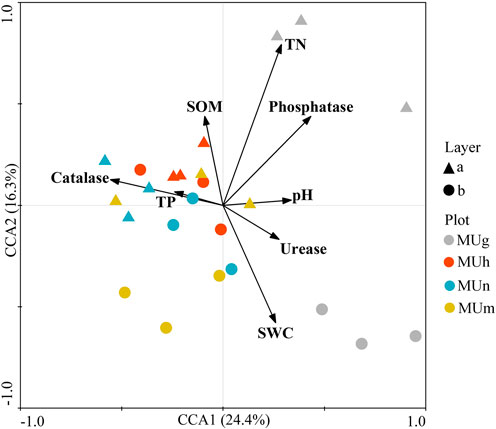
FIGURE 4. CCA of the soil fungi communities with soil properties in different soil depths through revegetation durations. Each arrow points in the expected direction of the steepest increase of values of environment variable. The angles between arrows indicate correlations between individual environment variable.
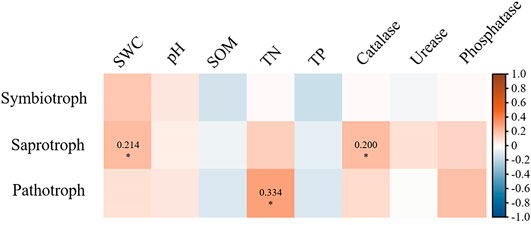
FIGURE 5. Correlation between soil fungal functional group and soil properties. The color represents Mantel’ r value. The * indicates significant correlation, and the Mantel’ r values of significant correlation were labeled.
Discussion
Revegetation Duration Shaped Fungal Diversity and Composition
Vegetation recovery and reconstruction regulate and control the interactions between microbial communities and forest development, which mainly manifest in the dynamics of microbial diversity and structure (Cavard et al., 2011; Chanthorn et al., 2017). In this study, soil fungal community diversity and structure response to revegetation duration were investigated; these have been confirmed in abandoned farmland (Wang et al., 2020), mining area (Prado et al., 2019), and desert areas (Yu et al., 2017). The variation of Shannon diversities initially decreased and then increased with temporal succession due to two reasons. First, the premier soil fungi existing perpetually find it hard to adapt to the sudden changes in the external conditions when the P. sylvestris saplings were replanted, because the dominant plant species and root exudates are different (Jing et al., 2015; Mcdaniel et al., 2016). Second, with the growth of P. sylvestris plantations, diversity indices became highest in mature forests. The increase in plant litter and debris provides more diverse habitats and substrates for soil fungal communities to promote fungal diversity (Ren et al., 2019).
In short, the revegetation of P. sylvestris in desert areas influenced the soil fungal alpha diversities, even though they decreased at the beginning due to sudden changes in habitats, and then rose gradually after the establishment of a stable interrelation between fungi and plant-soil. In the later stages of P. sylvestris plantation development, the increase in diversity confirmed that successional pathways may play a positive role in controlling the development of soil fungi in forest ecosystems (Dang et al., 2017; Shi et al., 2019).
Soil fungal diversity in different trophic modes also varied with vegetation restoration. First, saprotrophs were more diverse than symbiotrophs throughout the whole revegetation period and seemed to remain steady. The higher diversity of saprotrophs may be attributable to their greater adaptability to the external environment (Boddy and Hiscox, 2016) as they participate in the functional traits associated with essential circulation in nature, such as decomposition and nutrient cycling for leaf litter (Ceci et al., 2019; Francioli et al., 2020). Furthermore, the increase in symbiotroph diversity in grasslands may be related to AM fungi, which had the highest proportion in grasslands. Finally, the Shannon diversity of saprotrophs was significantly higher than that of symbiotrophs in grassland, but not in plantations. This confirmed that the AM fungi may not compete as strongly with saprotrophs as EcM fungi (Carteron et al., 2020).
The proportion of the soil fungal functional groups in P. sylvestris plantations was influenced by revegetation duration. Due to the poor adaptability to environmental change and the more unstable biological community structure in grassland ecosystems, the unassigned group in grassland had higher relative abundance than in plantation. Symbiotrophs were abundant in P. sylvestris plantations as P. sylvestris is a classical EcM fungi dependent tree species (Zhu et al., 2008). With the passage of revegetation duration in plantations, the dominant fungal functional group changed from saprotrophs to symbiotrophs and returned to saprotrophs. Following were the main reasons for these dynamic changes: 1) P. sylvestris plantations demanded more nutrients when they entered into the vigorous growth period and increase in symbiotrophs was conducive to promote the absorption of nutrients for the host (Kolarikova et al., 2017). With the growth slowing down and litter accumulating, symbiotrophs decreased and saprotrophs increased, which helped to decompose litter and produce available nutrients (Bardgett and van der Putten, 2014; Zhao et al., 2020). 2) The heterogeneity of soil fungal composition may be indirectly caused by root exudates, whose components are related to stand age. Moreover, specific fungal species, with preference for specific components, can be attracted to different root exudates (Llado et al., 2018).
Dynamics of Soil Fungal Ecological Functions in Different Soil Depths Based on Revegetation Durations
In the complicated succession process of soil fungi, some of them appear to flourish or decline as a reciprocation, while others are universal and exist for a long time. In this study, the community composition at the genus level was similar in all soil samples, and the dominant and common genera altered with revegetation duration. Mortierella actively participates in soil nutrient transformation and promotes the growth of plants, and its ubiquity may have a positive effect on vegetation restoration in desert areas (Zhang et al., 2011; Walter Osorio and Habte, 2014; Tamayo-Velez and Osorio, 2018). Gibberella and Fusarium were among the top 10 genera only in grassland. Gibberella and the sexual/teleomorphs states of Fusarium species are common pathogens causing wilting, root rot, and other diseases (Dumroese and James, 2005; Wollenberg et al., 2019). In contrast, Penicillium, the dominant or common genus only in plantations, solubilizes phosphates and excretes antifungal substances that potentially hinder the growth of plant pathogenic fungi (Efthymiou et al., 2018). The increase of Penicillium in P. sylvestris plantations may contribute to plant health and soil quality.
Diversispora, an AM fungus in Glomeromycota, is an indicator of grassland. This was closely related to vegetation (the herb species in grassland) and host specificity or preference was a key driver of soil fungal community composition (Horn et al., 2017). The top genera in plantations comprise a large proportion of EcM fungi (notably, Inocybe, Tuber, and Calostoma), which have also been identified as common EcM fungi in Pinaceae on a global scale (Hayward et al., 2015; Long et al., 2016; Guo et al., 2020a). EcM fungi facilitate the efficient uptake of nutrients (Rao et al., 2020). They can provide limiting nutrients, including nitrogen, phosphorus, copper, iron, and zinc, and resist disease and drought (van der Heijden et al., 2008). Inocybe and Tomentella are indicator genera of nearly mature forests. The sudden increase in their relative abundance is responsible for the highest proportion of symbiotrophs in nearly mature forests. In addition, previous studies have confirmed that AM habitats experience greater disturbance from pathotrophs compared with ericoid mycorrhiza (ErM) habitats (Bahram et al., 2020), which may explain the higher number of pathogens in grasslands. Therefore, we reasonably speculated that significant quantity of EcM fungi effectively solved the problem of low soil nutrient content in the desertification area where P. sylvestris planted.
It is worth mentioning that some soil fungi were provided with multiple functional groups, such as Mortierella, Fusarium, Microascu, and Podospora. The variable trophic modes mean that soil fungi can regulate ecological strategies and behavior to adapt to environmental change (Porras-Alfaro and Bayman, 2011). Some EcM fungi express saprotrophic capacity, even though they usually show a poor competence to decompose carbon compounds (Lindahl and Tunlid, 2015).
Effects of Soil Properties on Fungal Community Composition
In the process of vegetation restoration, soil fungal community was significantly affected by the changing soil properties, which in turn, were most likely affected by vegetation. Soil pH has a ubiquitous impact on soil fungal community composition (Rousk et al., 2011; Tedersoo et al., 2014; Barnes et al., 2018), however, it was not confirmed in this study. This may be because fungi possess a low sensitivity and a wide optimum range of soil environment (Rousk et al., 2010), or the fluctuation of soil pH along vegetation restorations was not significant. It has been demonstrated that vegetation restoration may not be an inevitable factor in changing soil pH in deserts (Zuo et al., 2009).
Moreover, as time of revegetation increased, the accumulation of litter increased the soil nutrients, especially the topsoil. This means that more soil nutrients are present in the topsoil than the subsoil for nutrient leaching, which creates a suitable environment to accommodate eutrophic fungal communities (Liu et al., 2019b; Ren et al., 2019). Another description of revegetation restoration is stand age. The influence of forest development on soil fungal communities has also been reported in evergreen broad-leaved species (Wu et al., 2013) and pine forests (Kyaschenko et al., 2017).
Soil fungi perform important functions in nutrient cycling, while soil nutrients shape soil fungal communities with different functional groups (Peay et al., 2016; Perez-Izquierdo et al., 2017; Li et al., 2018). However, the complex and equivocal interaction between soil nutrients and fungi makes it difficult to generalize the fungi-soil relationship. This explained that no significant correlation was observed between symbiotrophs/saprotrophs and soil nutrients (SOM, TN, and TP), while a variety of mycorrhizal/saprotrophic fungi participate in carbon, nitrogen, and phosphorus cycling (Fujimura and Egger, 2012). In contrast, TN had an important role in the soil fungal community, which was consistent with a previous large-scale research study (Cai et al., 2018; Schappe et al., 2020). In particular, pathotrophs are more susceptible to TN, which has been confirmed to be more inclined to the environment with enriched nutrients (Zhang et al., 2017a; Schappe et al., 2020). However, the type of pathogen determines the different effects of nitrogen, which means that a high nitrogen supply may increase infection by obligate parasites or decrease infection by facultative parasites (Dordas, 2008).
It is worth noting that soil depth did not have an obvious effect on soil fungal diversity and composition in this study, which is in contrast to the conclusions reported previously (Baldrian et al., 2012; Toju et al., 2016; Schlatter et al., 2018). We speculated that a small change in soil depth has a negligible effect on soil fungal diversity and structure. In other words, a small-scale spatial variation cannot be a preponderant trigger for the dynamics of soil fungal diversity and composition under drought and infertile conditions of the desert.
Conclusion
Soil nutrient content (SOM, TN, and TP) and enzyme activity (catalase, urease, and phosphatase) showed significant differences among restoration durations, while soil pH and SWC were not affected by soil depth or restoration duration. The alpha diversity of saprotrophs was higher than that of symbiotrophs and pathotrophs. The Shannon diversity index of all fungal communities was significantly different among different revegetation durations, but not soil depth. Heterogeneity was observed in the distribution of fungal taxonomic and functional groups through revegetation duration; grassland contained more unassigned fungal functional groups and fewer symbiotrophs than the plantation. EcM fungi, such as Inocybe, Tuber, Geopora, Tuber, Calostoma, Rhizopogon and Tomentella were the primary constituent of symbiotrophs. The endophyte fungus Mortierella was a dominant/common genus in all soil samples and AM fungus Diversispora was the indicator genus of grassland. The soil fungal community was mainly influenced by catalase and TN, which were significantly related to saprotrophs and pathotrophs, respectively.
Therefore, our study outcomes help to understand the conduciveness of soil fungal dynamics to the effect of revegetation and fungal function in deserts. We identified the major determinants affecting soil fungal community composition and discussed their potential functions in different restoration durations. It is important to appreciate the impact of soil fungi on soil quality and plant health. However, soil depth contributed little to soil fungi in the present study and selection of soil depth range should be expanded in future research.
Data Availability Statement
The datasets presented in this study can be found in online repositories. The names of the repository/repositories and accession numbers can be found below: https://www.ncbi.nlm.nih.gov/genbank/, SRA accession: PRJNA663124.
Author Contributions
YZ designed and performed the experiments, analyzed the data and wrote paper. HC, PZ, XW performed the investigation and experiments. GG acquired funding, projected administration and wrote paper. GD and MS supervised the whole progress and acquired funding.
Funding
This research was funded by the National Natural Science Foundation of China (no. 31700639 and no. 31600583), National Key Research and Development Program of China (no. 2016YFC0500802 and no. 2018YFC0507101) and Fundamental Research Funds for the Central Universities (no. 2017PT03 and no. 2015ZCQ-SB-02).
Conflict of Interest
The authors declare that the research was conducted in the absence of any commercial or financial relationships that could be construed as a potential conflict of interest.
Acknowledgments
The authors wish to acknowledge all members of research team for helpful discussions, key laboratory of state forestry and grass land administration on soil and water conservation for technical support and all funding for financial support.
Supplementary Material
The Supplementary Material for this article can be found online at: https://www.frontiersin.org/articles/10.3389/fenvs.2021.589068/full#supplementary-material.
References
Bahram, M., Netherway, T., Hildebrand, F., Pritsch, K., Drenkhan, R., Loit, K., et al. (2020). Plant nutrient-acquisition strategies drive topsoil microbiome structure and function. New Phytol. 227 (4), 1189–1199. doi:10.1111/nph.16598
Baldrian, P., Kolarik, M., Stursova, M., Kopecky, J., Valaskova, V., Vetrovsky, T., et al. (2012). Active and total microbial communities in forest soil are largely different and highly stratified during decomposition. ISME J. 6 (2), 248–258. doi:10.1038/ismej.2011.95
Bardgett, R. D., and van der Putten, W. H. (2014). Belowground biodiversity and ecosystem functioning. Nature 515 (7528), 505–511. doi:10.1038/nature13855
Barnes, C. J., van der Gast, C. J., McNamara, N. P., Rowe, R., and Bending, G. D. (2018). Extreme rainfall affects assembly of the root-associated fungal community. New Phytol. 220 (4), 1172–1184. doi:10.1111/nph.14990
Boddy, L., and Hiscox, J. (2016). Fungal ecology: principles and mechanisms of colonization and competition by saprotrophic fungi. Microbiol. Spectr. 4 (6), 19. doi:10.1128/microbiolspec
Brockett, B. F. T., Prescott, C. E., and Grayston, S. J. (2012). Soil moisture is the major factor influencing microbial community structure and enzyme activities across seven biogeoclimatic zones in western Canada. Soil Biol. Biochem. 44 (1), 9–20. doi:10.1016/j.soilbio.2011.09.003
Broeckling, C. D., Broz, A. K., Bergelson, J., Manter, D. K., and Vivanco, J. M. (2008). Root exudates regulate soil fungal community composition and diversty. Appl. Environ. Microbiol. 74 (3), 738–744. doi:10.1128/aem.02188-07
Cáceres, M. D., and Legendre, P. (2009). Associations between species and groups of sites: indices and statistical inference. Ecology 90, 3566–3574. 10.1890/08-1823.1
Cai, Z.-q., Zhang, Y.-h., Yang, C., and Wang, S. (2018). Land-use type strongly shapes community composition, but not always diversity of soil microbes in tropical China. Catena 165, 369–380. doi:10.1016/j.catena.2018.02.018
Cardinale, B. J., Srivastava, D. S., Emmett Duffy, J., Wright, J. P., Downing, A. L., Sankaran, M., et al. (2006). Effects of biodiversity on the functioning of trophic groups and ecosystems. Nature 443 (7114), 989–992. doi:10.1038/nature05202
Carteron, A., Beigas, M., Joly, S., Turner, B. L., and Laliberte, E. (2020). Temperate forests dominated by arbuscular or ectomycorrhizal fungi are characterized by strong shifts from saprotrophic to mycorrhizal fungi with increasing soil depth. Microb. Ecol. [Epub ahead of print]. doi:10.1007/s00248-020-01540-7
Cavard, X., Bergeron, Y., Chen, H. Y. H., Paré, D., Laganière, J., and Brassard, B. (2011). Competition and facilitation between tree species change with stand development. Oikos 120 (11), 1683–1695. doi:10.1111/j.1600-0706.2011.19294.x
Ceci, A., Pinzari, F., Russo, F., Persiani, A. M., and Gadd, G. M. (2019). Roles of saprotrophic fungi in biodegradation or transformation of organic and inorganic pollutants in co-contaminated sites. Appl. Microbiol. Biotechnol. 103 (1), 53–68. doi:10.1007/s00253-018-9451-1
Chanthorn, W., Hartig, F., and Brockelman, W. Y. (2017). Structure and community composition in a tropical forest suggest a change of ecological processes during stand development. For. Ecol. Manag. 404, 100–107. doi:10.1016/j.foreco.2017.08.001
Chen, Y.-L., Xu, T.-L., Veresoglou, S. D., Hu, H.-W., Hao, Z.-P., Hu, Y.-J., et al. (2017). Plant diversity represents the prevalent determinant of soil fungal community structure across temperate grasslands in northern China. Soil Biol. Biochem. 110, 12–21. doi:10.1016/j.soilbio.2017.02.015
Dang, P., Yu, X., Le, H., Liu, J., Shen, Z., and Zhao, Z. (2017). Effects of stand age and soil properties on soil bacterial and fungal community composition in Chinese pine plantations on the Loess Plateau. PLoS One 12 (10), e0186501. doi:10.1371/journal.pone.0186501
De Deyn, G. B., and Van der Putten, W. H. (2005). Linking aboveground and belowground diversity. Trends Ecol. Evol. 20 (11), 625–633. doi:10.1016/j.tree.2005.08.009
Deng, J., Yin, Y., Luo, J., Zhu, W., and Zhou, Y. (2019). Different revegetation types alter soil physical-chemical characteristics and fungal community in the Baishilazi Nature Reserve. PeerJ 6, e6251. doi:10.7717/peerj.6251
Detheridge, A. P., Comont, D., Callaghan, T. M., Bussell, J., Brand, G., Gwynn-Jones, D., et al. (2018). Vegetation and edaphic factors influence rapid establishment of distinct fungal communities on former coal-spoil sites. Fungal Ecology 33, 92–103. doi:10.1016/j.funeco.2018.02.002
Dordas, C. (2008). Role of nutrients in controlling plant diseases in sustainable agriculture. A review. Agron. Sustain. Dev. 28 (1), 33–46. doi:10.1051/agro:2007051
Dumroese, R. K., and James, R. L. (2005). Root diseases in bareroot and container nurseries of the Pacific Northwest: epidemiology, management, and effects on outplanting performance. N. For. 30 (2–3), 185–202. doi:10.1007/s11056-005-4422-7
Edgar, R. C. (2013). UPARSE: highly accurate OTU sequences from microbial amplicon reads. Nat. Methods 10 (10), 996–998. doi:10.1038/nmeth.2604
Efthymiou, A., Jensen, B., and Jakobsen, I. (2018). The roles of mycorrhiza and Penicillium inoculants in phosphorus uptake by biochar-amended wheat. Soil Biol. Biochem. 127, 168–177. doi:10.1016/j.soilbio.2018.09.027
Francioli, D., van Rijssel, S. Q., van Ruijven, J., Termorshuizen, A. J., Cotton, T. E. A., Dumbrell, A. J., et al. (2020). Plant functional group drives the community structure of saprophytic fungi in a grassland biodiversity experiment. Plant Soil. [Epub ahead of print]. doi:10.1007/s11104-020-04454-y
Fujimura, K. E., and Egger, K. N. (2012). Host plant and environment influence community assembly of High Arctic root-associated fungal communities. Fungal Ecology 5 (4), 409–418. doi:10.1016/j.funeco.2011.12.010
Gianfreda, L., Rao, M. A., Piotrowska, A., Palumbo, G., and Colombo, C. (2005). Soil enzyme activities as affected by anthropogenic alterations: intensive agricultural practices and organic pollution. Sci. Total Environ. 341 (1–3), 265–279. doi:10.1016/j.scitotenv.2004.10.005
Guo, M.-s., Ding, G.-d., Gao, G.-l., Zhang, Y., Cao, H.-y., and Ren, Y. (2020a). Community composition of ectomycorrhizal fungi associated with Pinus sylvestris var. mongolica plantations of various ages in the Horqin Sandy Land. Ecol. Indicat. 110, 105860. doi:10.1016/j.ecolind.2019.105860
Guo, M., Gao, G., Ding, G., and Zhang, Y. (2020b). Drivers of ectomycorrhizal fungal community structure associated with Pinus sylvestris var. Mongolica differ at regional vs. local spatial scales in northern China. Forests 11 (3), 323. doi:10.3390/f11030323
Guo, Y., Chen, X., Wu, Y., Zhang, L., Cheng, J., Wei, G., et al. (2018). Natural revegetation of a semiarid habitat alters taxonomic and functional diversity of soil microbial communities. Sci. Total Environ. 635, 598–606. doi:10.1016/j.scitotenv.2018.04.171
Hayward, J., Horton, T. R., and Nunez, M. A. (2015). Ectomycorrhizal fungal communities coinvading with Pinaceae host plants in Argentina: Gringos bajo el bosque. New Phytol. 208 (2), 497–506. doi:10.1111/nph.13453
He, J., Tedersoo, L., Hu, A., Han, C., He, D., Wei, H., et al. (2017). Greater diversity of soil fungal communities and distinguishable seasonal variation in temperate deciduous forests compared with subtropical evergreen forests of eastern China. FEMS (Fed. Eur. Microbiol. Soc.) Microbiol. Ecol. 93 (7), fix069. doi:10.1093/femsec/fix069
Horn, S., Hempel, S., Verbruggen, E., Rillig, M. C., and Caruso, T. (2017). Linking the community structure of arbuscular mycorrhizal fungi and plants: a story of interdependence? ISME J. 11 (6), 1400–1411. doi:10.1038/ismej.2017.5
Husband, R., Herre, E. A., Turner, S. L., Gallery, R., and Young, J. P. W. (2002). Molecular diversity of arbuscular mycorrhizal fungi and patterns of host association over time and space in a tropical forest. Mol. Ecol. 11 (12), 2669–2678. doi:10.1046/j.1365-294X.2002.01647.x
Jin, Z., Li, X., Wang, Y., Wang, Y., Wang, K., and Cui, B. (2016). Comparing watershed black locust afforestation and natural revegetation impacts on soil nitrogen on the Loess Plateau of China. Sci. Rep. 6, 25048. doi:10.1038/srep25048
Jing, X., Sanders, N. J., Shi, Y. U., Chu, H., Classen, A. T., Zhao, K. E., et al. (2015). The links between ecosystem multifunctionality and above-and belowground biodiversity are mediated by climate. Nat. Commun. 6, 8159. doi:10.1038/ncomms9159
Jing, Z., Cheng, J., Jin, J., Su, J., and Bai, Y. (2014). Revegetation as an efficient means of improving the diversity and abundance of soil eukaryotes in the Loess Plateau of China. Ecol. Eng. 70, 169–174. doi:10.1016/j.ecoleng.2014.05.011
John, , and Matt, K. J. S. S. (1970). Colorimetric determination of phosphorus in soil and plant materials with ascorbic acid. Soil Sci. 109 (4), 214–220. doi:10.1097/00010694-197004000-00002
Kolarikova, Z., Kohout, P., Kruger, C., Janouskova, M., Mrnka, L., and Rydlova, J. (2017). Root-associated fungal communities along a primary succession on a mine spoil: distinct ecological guilds assemble differently. Soil Biol. Biochem. 113, 143–152. doi:10.1016/j.soilbio.2017.06.004
Kubartova, A., Ottosson, E., Dahlberg, A., and Stenlid, J. (2012). Patterns of fungal communities among and within decaying logs, revealed by 454 sequencing. Mol. Ecol. 21 (18), 4514–4532. doi:10.1111/j.1365-294X.2012.05723.x
Kyaschenko, J., Clemmensen, K. E., Hagenbo, A., Karltun, E., and Lindahl, B. D. (2017). Shift in fungal communities and associated enzyme activities along an age gradient of managed Pinus sylvestris stands. ISME J. 11 (4), 863–874. doi:10.1038/ismej.2016.184
Li, S., Shakoor, A., Wubet, T., Zhang, N., Liang, Y., and Ma, K. (2018). Fine-scale variations of fungal community in a heterogeneous grassland in Inner Mongolia: effects of the plant community and edaphic parameters. Soil Biol. Biochem. 122, 104–110. doi:10.1016/j.soilbio.2018.04.007
Lindahl, B. D., and Tunlid, A. (2015). Ectomycorrhizal fungi - potential organic matter decomposers, yet not saprotrophs. New Phytol. 205 (4), 1443–1447. doi:10.1111/nph.13201
Liu, G. y., Chen, L. l., Shi, X. r., Yuan, Z. y., Yuan, L. Y., Lock, T. R., et al. (2019a). Changes in rhizosphere bacterial and fungal community composition with vegetation restoration in planted forests. Land Degrad. Dev. 30 (10), 1147–1157. doi:10.1002/ldr.3275
Liu, J., Maldonado-Mendoza, I., Lopez-Meyer, M., Cheung, F., Town, C. D., and Harrison, M. J. (2007). Arbuscular mycorrhizal symbiosis is accompanied by local and systemic alterations in gene expression and an increase in disease resistance in the shoots. Plant J. 50 (3), 529–544. doi:10.1111/j.1365-313X.2007.03069.x
Liu, Y., Chen, X., Liu, J., Liu, T., Cheng, J., Wei, G., et al. (2019b). Temporal and spatial succession and dynamics of soil fungal communities in restored grassland on the Loess Plateau in China. Land Degrad. Dev. 30 (11), 1273–1287. doi:10.1002/ldr.3289
Llado, S., Lopez-Mondejar, R., and Baldrian, P. (2018). Drivers of microbial community structure in forest soils. Appl. Microbiol. Biotechnol. 102 (10), 4331–4338. doi:10.1007/s00253-018-8950-4
Long, D., Liu, J., Han, Q., Wang, X., and Huang, J. (2016). Ectomycorrhizal fungal communities associated with Populus simonii and Pinus tabuliformis in the hilly-gully region of the Loess Plateau, China. Sci. Rep. 6, 24336. doi:10.1038/srep24336
Lyu, Y. L., Shi, P. J., Han, G. Y., Liu, L. Y., Guo, L. L., Hu, X., et al. (2020). Desertification control practices in China. Sustainability 12 (8), 15. doi:10.3390/su12083258
Marín, C., Godoy, R., Valenzuela, E., Schloter, M., and Gschwendtner, S. (2017). Functional land-use change effects on soil fungal communities in Chilean temperate rainforests. J. Soil Sci. Plant Nutr. 17 (4), 985–1002. doi:10.4067/S0718-95162017000400011
Mason, C. J., Edwards, M., Riby, P. G., and Coe, G. (1999). The use of microwaves in the acceleration of digestion and colour development in the determination of total Kjeldahl nitrogen in soil. The Analyst 124 (11), 1719–1726. doi:10.1039/a903623g
Mcdaniel, M. D., Tiemann, L. K., and Grandy, A. S. (2016). Does agricultural crop diversity enhance soil microbial biomass and organic matter dynamics? A meta-analysis. Ecol. Appl. 24, 560–570. doi:10.1890/13-0616.1
Miao, Q., Yu, W., Kang, H., and Wang, J. (2019). Prolonging rotation of Chinese fir to over 25 years could maintain a better soil status in subtropical China. Forests 10(8), 80629. doi:10.3390/f10080629
Nearing, M. A., Xie, Y., Liu, B., and Ye, Y. (2017). Natural and anthropogenic rates of soil erosion. International Soil and Water Conservation Research 5 (2), 77–84. doi:10.1016/j.iswcr.2017.04.001
Nguyen, N. H., Song, Z., Bates, S. T., Branco, S., Tedersoo, L., Menke, J., et al. (2016). FUNGuild: an open annotation tool for parsing fungal community datasets by ecological guild. Fungal Ecology 20, 241–248. doi:10.1016/j.funeco.2015.06.006
Nie, S. a., Lei, X., Zhao, L., Brookes, P. C., Wang, F., Chen, C., et al. (2018). Fungal communities and functions response to long-term fertilization in paddy soils. Appl. Soil Ecol. 130, 251–258. doi:10.1016/j.apsoil.2018.06.008
Nunez-Mir, G. C., Iannone, B. V., Curtis, K., and Fei, S. (2015). Evaluating the evolution of forest restoration research in a changing world: a “big literature” review. N. For. 46 (5–6), 669–682. doi:10.1007/s11056-015-9503-7
Op De Beeck, M., Lievens, B., Busschaert, P., Declerck, S., Vangronsveld, J., and Colpaert, J. V. (2014). Comparison and validation of some ITS primer pairs useful for fungal metabarcoding studies. PLoS One 9 (6), e97629. doi:10.1371/journal.pone.0097629
Peay, K. G., Baraloto, C., and Fine, P. V. A. (2013). Strong coupling of plant and fungal community structure across western Amazonian rainforests. ISME J. 7 (9), 1852–1861. doi:10.1038/ismej.2013.66
Peay, K. G., Kennedy, P. G., and Talbot, J. M. (2016). Dimensions of biodiversity in the Earth mycobiome. Nat. Rev. Microbiol. 14 (7), 434–447. doi:10.1038/nrmicro.2016.59
Perez-Izquierdo, L., Zabal-Aguirre, M., Flores-Renteria, D., Gonzalez-Martinez, S. C., Buee, M., and Rincon, A. (2017). Functional outcomes of fungal community shifts driven by tree genotype and spatial-temporal factors in Mediterranean pine forests. Environ. Microbiol. 19 (4), 1639–1652. doi:10.1111/1462-2920.13690
Pickles, B. J., Gorzelak, M. A., Green, D. S., Egger, K. N., and Massicotte, H. B. (2015). Host and habitat filtering in seedling root-associated fungal communities: taxonomic and functional diversity are altered in 'novel' soils. Mycorrhiza 25 (7), 517–531. doi:10.1007/s00572-015-0630-y
Porras-Alfaro, A., and Bayman, P. (2011). “Hidden fungi, Emergent properties: Endophytes and Microbiomes,” in Annual review of phytopathology, Annual Reviews Editors N. K. VanAlfen, G. Bruening, and J. E. Leach, 291–315.
Prado, I. G. d. O., da Silva, M. d. C. S., Prado, D. G. d. O., Kemmelmeier, K., Pedrosa, B. G., Silva, C. C. d., et al. (2019). Revegetation process increases the diversity of total and arbuscular mycorrhizal fungi in areas affected by the Fundão dam failure in Mariana, Brazil. Appl. Soil Ecol. 141, 84–95. doi:10.1016/j.apsoil.2019.05.008
Qin, M., Shi, G., Zhang, Q., Meng, Y., Liu, Y., Pan, J., et al. (2019). Arbuscular mycorrhizal fungi serve as keystone taxa for revegetation on the Tibetan Plateau. J. Basic Microbiol. 59 (6), 609–620. doi:10.1002/jobm.201900060
Rao, M. V., Rice, R. A., Fleischer, R. C., and Muletz-Wolz, C. R. (2020). Soil fungal communities differ between shaded and sun-intensive coffee plantations in El Salvador. PLoS One 15 (4), e0231875. doi:10.1371/journal.pone.0231875
Ren, C., Liu, W., Zhao, F., Zhong, Z., Deng, J., Han, X., et al. (2019). Soil bacterial and fungal diversity and compositions respond differently to forest development. Catena 181, 104071. doi:10.1016/j.catena.2019.104071
Rousk, J., Bååth, E., Brookes, P. C., Lauber, C. L., Lozupone, C., Caporaso, J. G., et al. (2010). Soil bacterial and fungal communities across a pH gradient in an arable soil. ISME J. 4 (10), 1340–1351. doi:10.1038/ismej.2010.58
Rousk, J., Brookes, P. C., and Bååth, E. (2011). Fungal and bacterial growth responses to N fertilization and pH in the 150-year ‘Park Grass’ UK grassland experiment. FEMS (Fed. Eur. Microbiol. Soc.) Microbiol. Ecol. 76 (1), 89–99. doi:10.1111/j.1574-6941.2010.01032.x
Schappe, T., Albornoz, F. E., Turner, B. L., and Jones, F. A. (2020). Co-occurring fungal functional groups respond differently to tree neighborhoods and soil properties across three tropical rainforests in Panama. Microb. Ecol. 79 (3), 675–685. doi:10.1007/s00248-019-01446-z
Schlatter, D. C., Kahl, K., Carlson, B., Huggins, D. R., and Paulitz, T. (2018). Fungal community composition and diversity vary with soil depth and landscape position in a no-till wheat-based cropping system. FEMS (Fed. Eur. Microbiol. Soc.) Microbiol. Ecol. 94 (7), fiy098. doi:10.1093/femsec/fiy098
Shen, J.-P., Zhang, L.-M., Guo, J.-F., Ray, J. L., and He, J.-Z. (2010). Impact of long-term fertilization practices on the abundance and composition of soil bacterial communities in Northeast China. Appl. Soil Ecol. 46 (1), 119–124. doi:10.1016/j.apsoil.2010.06.015
Shi, L., Dossa, G. G. O., Paudel, E., Zang, H., Xu, J., and Harrison, R. D. (2019). Changes in fungal communities across a forest disturbance gradient. Appl. Environ. Microbiol. 85 (12), e00080-19. doi:10.1128/AEM.00080-19
Stursova, M., Barta, J., Santruckova, H., and Baldrian, P. (2016). Small‐scale spatial heterogeneity of ecosystem properties, microbial community composition and microbial activities in a temperate mountain forest soil. FEMS Microbiol. Ecol. 92, fiw185. doi:10.1093/femsec/fiw185
Tamayo-Velez, A., and Osorio, N. W. (2018). Soil fertility improvement by litter decomposition and inoculation with the fungus Mortierella sp in avocado plantations of Colombia. Commun. Soil Sci. Plant Anal. 49 (2), 139–147. doi:10.1080/00103624.2017.1417420
Tarafdar, J. C., and Marschner, H. (1994). Phosphatase activity in the rhizosphere and hyphosphere of VA mycorrhizal wheat supplied with inorganic and organic phosphorus. Soil Biol. Biochem. 26 (3), 387–395. doi:10.1016/0038-0717(94)90288-7
Tedersoo, L., Bahram, M., Polme, S., Koljalg, U., Yorou, N. S., Wijesundera, R., et al. (2014). Global diversity and geography of soil fungi. Science 346, 1256688. doi:10.1126/science.1256688
Toju, H., Kishida, O., Katayama, N., and Takagi, K. (2016). Networks depicting the fine-scale co-occurrences of fungi in soil horizons. PLoS One 11 (11), e0165987. doi:10.1371/journal.pone.0165987
Toju, H., Tanabe, A. S., and Sato, H. (2018). Network hubs in root-associated fungal metacommunities. Microbiome 6, 116. doi:10.1186/s40168-018-0497-1
van der Heijden, M. G., Bardgett, R. D., and van Straalen, N. M. (2008). The unseen majority: soil microbes as drivers of plant diversity and productivity in terrestrial ecosystems. Ecol. Lett. 11 (3), 296–310. doi:10.1111/j.1461-0248.2007.01139.x
Walkley, A., and Black, I. A. (1934). An examination of the Degtjareff method for determining soil organic matter,and a proposed modification of the chromic acid titration method. Soil Sci. 37 (1), 29–38. doi:10.1097/00010694-193401000-00003
Walter Osorio, N., and Habte, M. (2014). Soil phosphate Desorption induced by a phosphate-solubilizing fungus. Commun. Soil Sci. Plant Anal. 45 (4), 451–460. doi:10.1080/00103624.2013.870190
Wang, B., Xue, S., Liu, G. B., Zhang, G. H., Li, G., and Ren, Z. P. (2012). Changes in soil nutrient and enzyme activities under different vegetations in the Loess Plateau area, Northwest China. Catena 92, 186–195. doi:10.1016/j.catena.2011.12.004
Wang, C., Zhang, W., Zhao, C., Shi, R., Xue, R., and Li, X. (2020). Revegetation by sowing reduces soil bacterial and fungal diversity. Ecology and Evolution 10 (1), 431–440. doi:10.1002/ece3.5906
Wollenberg, R. D., Sondergaard, T. E., Nielsen, M. R., Knutsson, S., Pedersen, T. B., Westphal, K. R., et al. (2019). There it is! Fusarium pseudograminearum did not lose the fusaristatin gene cluster after all. Fungal Biology 123 (1), 10–17. doi:10.1016/j.funbio.2018.10.004
Wu, Y. T., Wubet, T., Trogisch, S., Both, S., Scholten, T., Bruelheide, H., et al. (2013). Forest age and plant species composition determine the soil fungal community composition in a Chinese subtropical forest. PLoS One 8(6), 66829. doi:10.1371/journal.pone.0066829
Xiao, X., Wei, X., Liu, Y., Ouyang, X., Li, Q., and Ning, J. (2015). Aerial seeding: an effective forest restoration method in highly degraded forest landscapes of sub-tropic regions. Forests 6 (6), 1748–1762. doi:10.3390/f6061748
Yang, Y., Cheng, H., Liu, L., Dou, Y., and An, S. (2020). Comparison of soil microbial community between planted woodland and natural grass vegetation on the Loess Plateau. For. Ecol. Manag. 460, 117817. doi:10.1016/j.foreco.2019.117817
Yu, J., Xue, Z., He, X., Liu, C., and Steinberger, Y. (2017). Shifts in composition and diversity of arbuscular mycorrhizal fungi and glomalin contents during revegetation of desertified semiarid grassland. Appl. Soil Ecol. 115, 60–67. doi:10.1016/j.apsoil.2017.03.015
Zhang, H., Wu, X., Li, G., and Qin, P. (2011). Interactions between arbuscular mycorrhizal fungi and phosphate-solubilizing fungus (Mortierella sp.) and their effects on Kostelelzkya virginica growth and enzyme activities of rhizosphere and bulk soils at different salinities. Biol. Fertil. Soils 47 (5), 543–554. doi:10.1007/s00374-011-0563-3
Zhang, K., Adams, J. M., Shi, Y., Yang, T., Sun, R., He, D., et al. (2017a). Environment and geographic distance differ in relative importance for determining fungal community of rhizosphere and bulk soil. Environ. Microbiol. 19 (9), 3649–3659. doi:10.1111/1462-2920.13865
Zhang, Y., Dong, S., Gao, Q., Liu, S., Ganjurjav, H., Wang, X., et al. (2017b). Soil bacterial and fungal diversity differently correlated with soil biochemistry in alpine grassland ecosystems in response to environmental changes. Sci. Rep. 7, 1–10. doi:10.1038/srep43077
Zhao, P. S., Guo, M. S., Gao, G. L., Zhang, Y., Ding, G. D., Ren, Y., et al. (2020). Community structure and functional group of root-associated fungi of Pinus sylvestris var. mongolica across stand ages in the Mu Us Desert. Ecology and Evolution 10 (6), 3032–3042. doi:10.1002/ece3.6119
Zheng, Y., Hu, H.-W., Guo, L.-D., Anderson, I. C., and Powell, J. R. (2017). Dryland forest management alters fungal community composition and decouples assembly of root- and soil-associated fungal communities. Soil Biol. Biochem. 109, 14–22. doi:10.1016/j.soilbio.2017.01.024
Zhou, H., Zhang, D., Jiang, Z., Sun, P., Xiao, H., Wu, Y., et al. (2019). Changes in the soil microbial communities of alpine steppe at Qinghai-Tibetan Plateau under different degradation levels. Sci. Total Environ. 651, 2281–2291. doi:10.1016/j.scitotenv.2018.09.336
Zhu, J.-j., Li, F.-q., Xu, M.-l., Kang, H.-z., and Wu, X.-y. (2008). The role of ectomycorrhizal fungi in alleviating pine decline in semiarid sandy soil of northern China: an experimental approach. Ann. For. Sci. 65 (3), 304. doi:10.1051/forest:2008007
Keywords: revegetation duration, soil fungi, temporal variation, soil properties, symbiotrophs
Citation: Zhang Y, Cao H, Zhao P, Wei X, Ding G, Gao G and Shi M (2021) Vegetation Restoration Alters Fungal Community Composition and Functional Groups in a Desert Ecosystem. Front. Environ. Sci. 9:589068. doi: 10.3389/fenvs.2021.589068
Received: 31 July 2020; Accepted: 06 January 2021;
Published: 01 February 2021.
Edited by:
Xian Xue, Northwest Institute of Eco-Environment and Resources (CAS), ChinaReviewed by:
Na Li, Chinese Academy of Science, ChinaYonglong Wang, Baotou Teachers' College, China
Peter Edward Mortimer, Chinese Academy of Sciences, China
Copyright © 2021 Zhang, Cao, Zhao, Wei, Ding, Gao and Shi. This is an open-access article distributed under the terms of the Creative Commons Attribution License (CC BY). The use, distribution or reproduction in other forums is permitted, provided the original author(s) and the copyright owner(s) are credited and that the original publication in this journal is cited, in accordance with accepted academic practice. No use, distribution or reproduction is permitted which does not comply with these terms.
*Correspondence: Guanglei Gao, Z2FvZ3VhbmdsZWlAYmpmdS5lZHUuY24=; Mingchang Shi, c2hpbWNAZHRnaXMuY29t