- 1MARE—Marine and Environmental Sciences Centre, NOVA School of Science and Technology, NOVA University, Lisbon, Portugal
- 2University of Coimbra, MARE–Marine and Environmental Sciences Centre, Department of Life Sciences, Coimbra, Portugal
Microplastics (MP) have been confirmed as emerging pollutants in the marine environment due to their ubiquity, bioavailability, persistence and potential toxicity. This study contributes with valuable data regarding the abundance and characteristics of the MP found in five species collected from Portugal. The mussel Mytilus galloprovincialis (n = 140) was collected from the Tagus estuary and Porto Covo coastal area, the peppery furrow shell Scrobicularia plana (n = 140) and the polychaete Marphysa sanguinea (n = 30) both from the Sado estuary, and Trachurus trachurus (n = 82) and Scomber colias (n = 82) fished off Figueira da Foz and Sesimbra. Soft tissues of all individuals were digested using a KOH (10%) solution, which allowed the extraction of MP. All studied species presented MP. In a total of 502 MP observed from all samples, 80% were fibers and 20% were fragments, with a size range of 73 μm–4,680 μm and blue was the most common color recorded (46%). The frequency of occurrence of MP was higher in T. trachurus (70%) and lowest in M. sanguinea (17%). MP abundance ranged from 0.30 ± 0.63 MP. ind−1 in S. plana, to 2.46 ± 4.12 MP. ind−1 in S. colias. No significant correlation was found between the individual biometric parameters and total MP, fibers and fragments ingested by each species. The FTIR analysis revealed that polyester and polyethylene were the most common polymers present. These results can be used as a reference for future studies regarding the use of indicator species for monitoring MP pollution in the coast of Portugal.
Highlights
• Microplastics were recorded in Mytilus galloprovincialis, Scrobicularia plana, Marphysa sanguinea, Trachurus trachurus and Scomber colias from Portugal;
• S. plana presented the lowest quantities of ingested MP;
• T. trachurus presented the highest percentage of individuals contaminated with MP;
• Fibers were the most common MP in mussels, peppery furrow shell and fish, accounting for approximately 80%;
• In the polychaete M. sanguinea, plastic fragments were dominant (83%);
• Blue microplastics were dominant over other detected colors.
Introduction
Plastic production and consumption have been increasing since the 1950s (GESAMP, 2016), which completely changed the profile of the waste produced (Sheavly, 2005) and leads to plastic accumulation in the environment. In 2015, the global plastic production was 322 million tons (GESAMP, 2016), including high levels of production of specific polymers such as polyethylene (PE) and polypropylene (PP) (PlasticsEurope, 2018), which coincides with the two most common polymers found in marine debris (Erni-Cassola et al., 2017). It is estimated that 80% of marine litter is composed by plastics and that about 5–13 million metric tons of plastic end up in the oceans each year (Jambeck et al., 2015). It is also estimated that there are more than five trillion plastic pieces floating in the oceans, weighing over 250,000 tons (Eriksen et al., 2014). Due to their properties, plastics can last up to hundreds of years in the environment (Thompson and Moore, 2009). Microplastics (MP) are defined as any plastic particle with less than 5 mm in size (Arthur et al., 2009) and can be classified as primary or secondary, according to their source. Primary MP can be found in cosmetic and personal healthcare products, such as exfoliants (Godoy et al., 2019) and tooth pastes (UNEP, 2016), house cleaning products (Napper et al., 2015) and in the form of virgin or recycled plastic pellets used as raw material for production (Browne et al., 2011). Secondary MP are a result of the fragmentation and degradation of larger plastic debris on land or sea (GESAMP and Kershaw, 2016), which can be induced by factors such as light (and ultraviolet light), higher temperatures, availability of oxygen and mechanical actions and also by biological interactions (Veiga et al., 2016). These MP include fibers from synthetic fabrics that can be released during laundering, in which a single piece of clothing can release up to 1900 fibers per wash (De Falco et al., 2019). More recently, Frias and Nash (2019) proposed a new definition for MP: “Microplastics are any synthetic solid particle or polymeric matrix, with regular or irregular shape and with size ranging from 1 μm to 5 mm, of either primary or secondary manufacturing origin, which are insoluble in water.”
MP have been found in several aquatic environments such as oceans (Pan et al., 2019), rivers (Jiang et al., 2019), estuaries (Hitchcock and Mitrovic, 2019; Rodrigues et al., 2019; Yan et al., 2019) and regions from the Arctic (Kanhai et al., 2019) and the Antarctic (Suaria et al., 2020). Being sampled from the water surface (Cincinelli et al., 2019; Tan et al., 2019), beaches (Retama et al., 2016; Piperagkas et al., 2019), marine sediment (Reed et al., 2018) and biota (Stock et al., 2019), they pose a challenge in terms of determining sources, pathways and potential effects (Veiga et al., 2016). In fact, MP enter the ocean through wide variety of land- and sea-based sources, rivers, wastewater and coastline run-offs, losses or discharges at sea and atmospheric transport, and at every level of plastic’s life cycle (Fahrenfeld et al., 2019), and there is a general trend toward finding more MP near densely populated coastal environments (Ivar Do Sul and Costa, 2014). Once in the oceans, MP have complex dynamics determined by factors such as currents, waves, wind and their polymer composition types (Triebskorn et al., 2019). Due to their physicochemical properties, MP have the ability to sorb persistent organic pollutants (POPs) (Fred-Ahmadu et al., 2020). There are also other low-molecular weight chemical species that raise concern in MP, such as the additives used in the manufacture of plastics (stabilizers or flame-retardants) (Sun et al., 2019). These chemicals may be released when in contact with organisms and be a potential chemical hazard (Wang et al., 2018; Prokić et al., 2019). MP have been found in a wide variety of different organisms with different feeding strategies and trophic levels (Gall and Thompson, 2015). These include zooplankton (Desforges et al., 2015), sponges, cnidaria and echinoderms (de Sá et al., 2018), annelids (Hurley et al., 2017), molluscs (Su et al., 2018), fish (Bessa et al., 2018) and also seabirds (Tanaka et al., 2013) and turtles (Hoarau et al., 2014). More than 220 different species have been reported to ingest MP debris in wildlife and in some species, ingestion levels are as high as 80% of the sampled individuals (Ory et al., 2017). MP can be taken up by organisms via direct ingestion (Lusher, 2015), indirect ingestion through ingested prey (Farrell and Nelson, 2013), ventilation (Watts et al., 2014), absorption (Long et al., 2015) or adherence to soft tissues (Kolandhasamy et al., 2018). Contamination with these particles can lead to negative health effects in the individuals exposed to them (Galgani et al., 2010; Besseling et al., 2013; Avio et al., 2015).
Monitoring MP and identifying the potential sources is essential for the assessment of the levels, composition and type of plastic polymers entering the marine environment, to provide knowledge about the behavior and impacts of MP and to create mitigation methods to reduce their inputs (GESAMP, 2019). There are several international and national actions under development, focused in protecting the marine environment and minimizing MP pollution impacts, namely the European Plastics Strategy (European Commission, 2018a) and Single-use Plastics Directive to reduce marine litter (European Commission, 2018b). In Europe, the Marine Strategy Framework Directive is establishing environmental targets for marine litter (and in particular MP) and associated indicators to achieve Good Environmental Status (GES) until the present year.
This study investigates the presence of MP in Mytilus galloprovincialis, Scrobicularia plana, Marphysa sanguinea, Trachurus trachurus and Scomber colias, from the Portuguese coast. The species were selected due to their different feeding strategies and habitats, as well as economic importance. The study also aims to contribute to the Marine Strategy Framework Directive (MSFD) 2008/56/EC with baseline data and knowledge.
M. galloprovincialis are benthic bivalves, with a filter feeding strategy and wide geographical distribution (Gosling, 1992) and can survive under polluted conditions and accumulate pollutants (Arienzo et al., 2019). All these characteristics make mussels a successful indicator of marine pollution (Li et al., 2019). Mussels also have a high economic interest due to their use in gastronomy in Portugal.
S. plana is an endobenthic bivalve with a deposit feeding strategy and can accumulate contaminants from both sediments and water (González-Domínguez et al., 2016). S. plana has commercial value as a human food resource in Portugal and its ecological importance, extensive distribution and sedentary lifestyle makes it a valuable biomonitor organism for contaminants (Langston et al., 2007) including MP (Ribeiro et al., 2017).
M. sanguinea is a large-sized omnivore annelid (Fauchald and Jumars, 1979) that lives in the sediment (Prevedelli et al., 2007). This polychaete has an ecological value due to its sediment turnover and an economical value due to its use as live bait for line fishing (Seo et al., 2016).
T. trachurus and S. colias are characterized for being pelagic oceanodromous fish, however, T. trachurus also displays benthopelagic behavior (FAO, 2005). Their geographic distribution and depth ranges are similar. The feeding behaviors of S. colias are based on zooplankton (fish larvae, small crustaceans and pteropods) and T. trachurus feeds on crustaceans (copepods), shrimps, small fish and squids. T. trachurus tend to be in demersal waters during the day and at night they rise to the surface for feeding, while S. colias are in the pelagic zone and occurs in schools close to surface waters, feeding on living organisms and other organic particles present in these areas (FAO, 2005).
Material and Methods
Study Area and Sampling
M. galloprovincialis were collected by hand directly on site at the Tagus estuary (Portinho da Costa beach, on the South bank) and Porto Covo (Figure 1). Clams and polychaetes were collected from the Sado estuary (near Carrasqueira, on the South bank). Fish were made available by Docapesca, S.A. at Figueira da Foz and Sesimbra fishing ports. To prevent the possible loss of MP via physiological activities, all individuals were frozen within 1 h after being collected. All species were analyzed for MP presence. The total number of individuals sampled was 474: 70 M. galloprovincialis from the Tagus estuary (T); 70 M. galloprovincialis from the Porto Covo coastal area (PC); 140 S. plana and 30 M. sanguinea from the Sado estuary (SE), 82 fish (41 T. trachurus and 41 S. colias) from Sesimbra (S); and 82 fish (41 T. trachurus and 41 S. colias) from Figueira da Foz (F). Sampling campaigns were held in May 2017 and all sites were chosen primarily due to the ease of access.
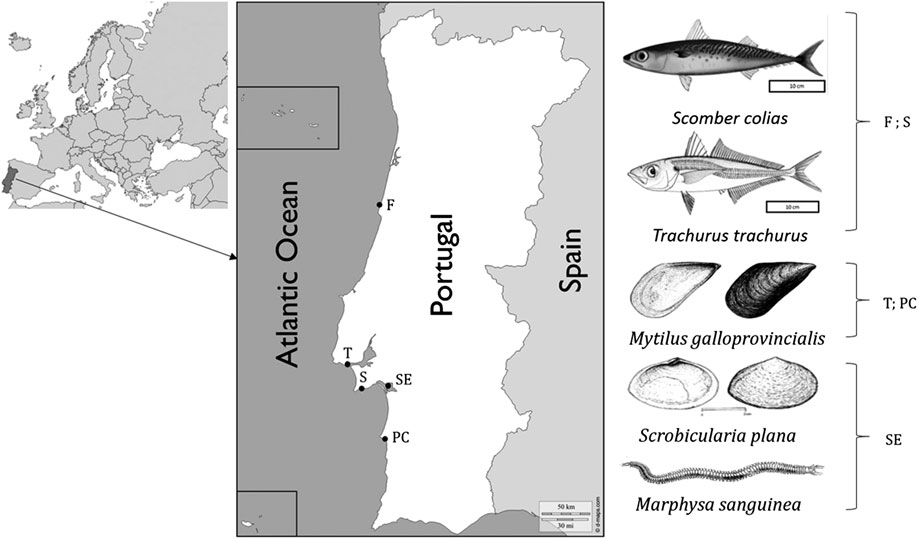
FIGURE 1. Sampling sites and the species collected along the Portuguese coast—F: Figueira da Foz; T: Tagus Estuary; S: Sesimbra; SE: Sado Estuary; PC: Porto Covo.
Laboratory Procedures
Samples were processed in the laboratory for MP detection and identification. The shells of mussels and clams were measured to determine their length and width and, after dissection, the wet weight of each individual was recorded, as well as for polychaetes. Fish were measured (standard length and total length) and weighed (total wet weight) and the wet weight of individual gastrointestinal (GI) tracts was obtained after dissection.
All individuals (clams, mussels and polychaetes) and GI tracts from fish were stored in glass flasks for alkaline digestion. All the equipment used in the dissection was pre-washed using distilled MilliQ water. The samples were chemically digested by a solution of potassium hydroxide at 10% (KOH). This method was chosen after reviewing the works of other authors that confirmed the efficiency of KOH in removing biogenic material while preserving the polymers (Foekema et al., 2013; Kühn et al., 2017), and that it has no significant impact in polymer mass or form, except for cellulose acetate, which makes it suitable for the digestion of molluscs and fish tissues and considered one of the best methods for extraction and identification of MP from biota (Dehaut et al., 2016; Karami et al., 2017; Bessa et al., 2019). The jars were covered with aluminum foil and stored at room temperature for 2 days. The jars were not stirred or shaken to prevent damaging of MP by other hard particles such as sand or other inorganic compounds. On average, after 48 h, a complete digestion of the biological material was observed.
Once digestion of the biological material was completed, the solution was filtered with a vacuum filtration system onto Fiorini and Whatman glass fiber filters (∼1 μm pore size). Filters were stored in covered Petri dishes, dried at room temperature and observed under a Leica® stereoscopic microscope equipped with a Leica Microsystems DFC480 digital camera. MP were classified into two different types: fibers and fragments and counted for each species. All MP were measured using ImageJ® software and their color was noted, except for fish, where a subsample of 183 MP was pooled for both species. To account for airborne contamination, the number of fibers in the controls was subtracted from the total of fibers in the samples. Visual identification of MP is open to bias and chemical confirmation of the polymers present must be performed. In this work, and as suggested by Hanke et al. (2013), a subsample of 10% of the total MP observed was randomly selected and analyzed by Fourier transformed infrared spectroscopy in attenuated total reflectance mode (FTIR). Spectra were acquired using an Agilent Handheld 4300 FTIR Spectrometer with a DTGS detector, with controlled temperature, and a diamond ATR sample interface; the analysis was performed at the sample surface. All spectra were obtained with a resolution of 4 cm−1 and 32 scans. Spectra are shown as acquired, without any further manipulation. The identification of the samples relied on the match over 80% between the sample and the library data (Agilent FTIR Spectral Libraries and Nicolet™ Condensed phase Sampler FTIR Spectral Library), and on best expert judgment from the presence of specific absorption bands for degraded polymers or copolymers.
Quality Control
Special caution was taken regarding contamination by airborne MP, with the use of cotton lab coats and controls. During the dissection and digestion procedures, one control was created for each 5 samples processed, by following the same steps described for biological samples to account for possible airborne MP contamination. The control filters were then examined for MP. New blanks consisting of wet filters were placed close to the stereoscopic microscope (2 controls for each group of 10 samples examined) to assess airborne fibers contamination during microscope observation.
Statistical Analysis
All data was tested for normality using Kolmogorov-Smirnov and tested for homoscedasticity using Levene’s test. Statistical analysis was made using α = 0.05. As data was not normally distributed (Kolmogorov-Smirnov: p < 0.05) and not homoscedastic (Levene’s test: p < 0.05), non-parametric tests were performed. The Mann-Whitney U test was used for pairwise comparisons between the total number of MP found in M. galloprovincialis collected in the Tagus estuary and Porto Covo coastal area. The Spearman correlation coefficient was used to assess correlations between the individual biometric parameters and total MP, fibers and fragments ingested by species. In fish, Kruskal-Wallis H test was used for comparisons between the number of fibers and fragments for each species and sampling site, followed by the post-hoc Dunn’s test for pairwise comparisons. Significance level established was 95% (α = 0.05) for all the analysis. All calculations were performed with Statistica® software.
Results
Biometric parameters for all species are shown in Table 1. Microplastics (fibers and fragments i.e., irregular shaped particles) were registered in all the species analyzed and showed variations in length, size and color. Figure 2 shows some examples of MP selected for polymer identification by FTIR.
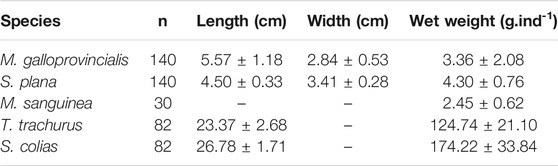
TABLE 1. Biometric parameters of the studied species (average ± standard deviation (SD), n—number of individuals).
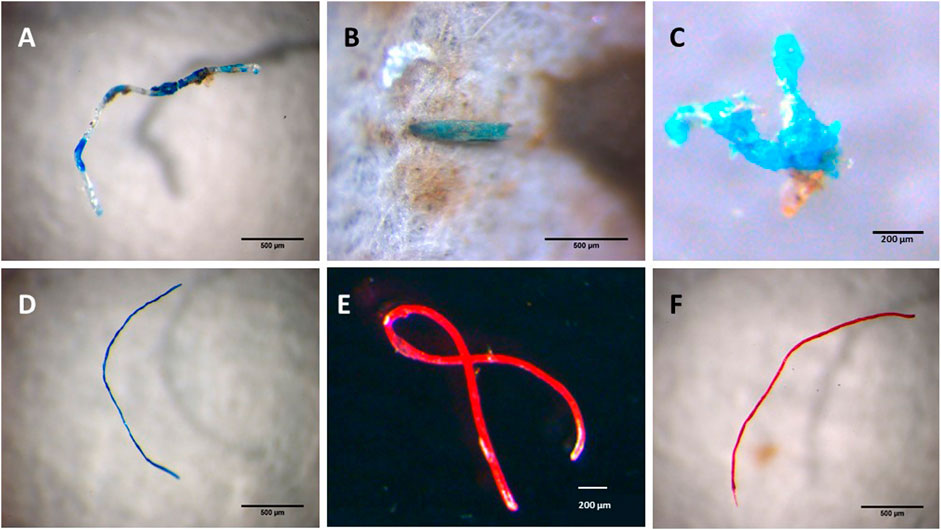
FIGURE 2. Different types of MP. (A) - PET fiber found in S. plana; (B) - PVC fragment found in S. plana; (C) - PE fragment found in S. colias; (D) - PET fiber found in M. galloprovincialis; (E) - PP fiber found in S. colias; (F) - PET fiber found in S. plana.
A total of 502 MP were registered, 80% being fibers and 20% fragments. Table 2 presents the number of MP per individual wet weight (MP.g−1) and per individual (MP.ind−1) for each species (average ± SD) as well as the percentage of individuals with MP.
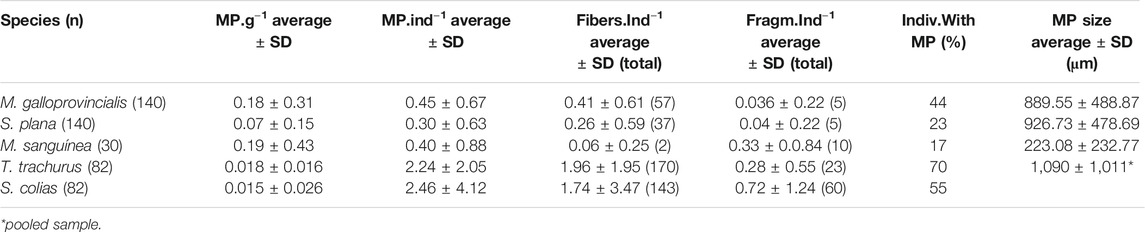
TABLE 2. Microplastics in the five species analyzed: MP per individual wet weight (MP.g−1) and per individual (MP.ind−1), fibers and fragments per individual (average ± SD and total number of each), percentage of individuals with ingested MP and size range of MP (μm), n—number of individuals.
From all the studied species, polychaetes showed the lowest percentage of individuals with MP (17%) while T. trachurus had the highest percentage (70%). S. colias had the highest average of MP per individual (2.46 ± 4.12 MP.ind−1) and the highest average of fragments per individual (0.72 ± 1.24 Fragm. ind−1). The number of MP found in a single individual ranged from one to three in mussels, one to two in polychaetes and clams and 1 to 20 in fish. Figure 3A presents the type of MP collected for each species, in percentage. As shown in Table 2, polychaetes showed the lowest average size of MP (223 ± 233 μm) and the highest average size was observed in fish (1,090 ± 1,011 μm). Average MP size for mussels was 890 ± 489 μm and for the clams was 927 ± 479 μm. MP sizes ranged from 90–2,574 μm in mussels, 90–1827 μm in S. plana, 73–822 μm in M. sanguinea and 87–4,680 μm in fish.
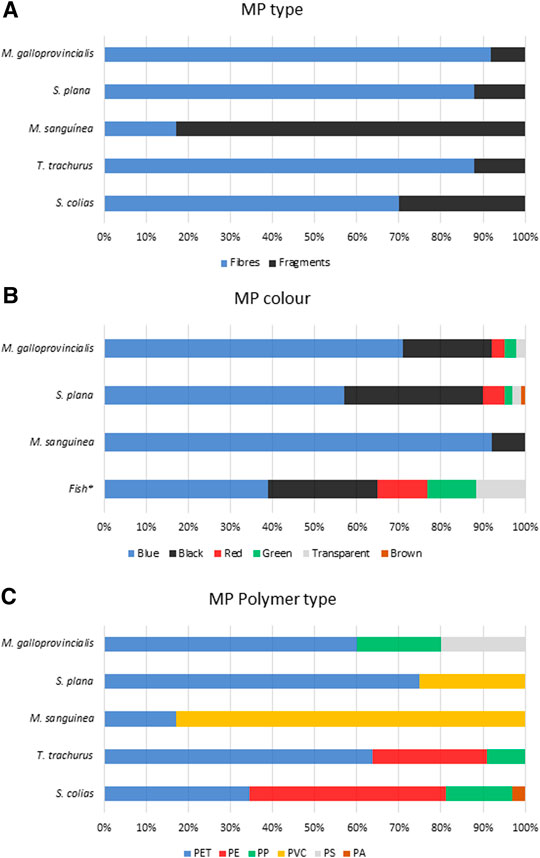
FIGURE 3. Microplastics (type (A), color (B), and polymer type (C)) found in fish, mussels, clams and polychaetes from the Portuguese coast (* pooled sample).
Fragment ingestion in mussels from Porto Covo was significantly higher when compared to mussels from Tagus (Mann-Whitney U, p < 0.05). No correlation was found between the individual biometric parameters and total MP, fibers and fragments ingested by each species (Spearman test, p > 0.05).
The presence of fibers in S. colias was significantly higher in Figueira da Foz, when compared with the same species from Sesimbra (Kruskal-Wallis H test, p < 0.05). At Sesimbra, fibers ingested by T. trachurus were significantly higher when compared to S. colias (Kruskal-Wallis H test, p < 0.05). At Figueira da Foz, the ingestion of fragments was significantly higher in S. colias when compared with T. trachurus (Kruskal-Wallis H test, p < 0.05).
Except for M. sanguinea, ingested fibers were dominant over fragments (Figure 3A). MP colors found were blue, black, red, green, brown and transparent (Figure 3B). Overall, blue was the most common color, representing 46% of all MP, followed by black with 26%. MP in polychaetes were only blue and black.
The FTIR spectral matches identified polyester (PET), polyethylene (PE), polypropylene (PP), polyvinyl chloride (PVC), polystyrene (PS) and nylon (PA). PET was the most common polymer found in MP extracted from M. galloprovincialis (60%), S. plana (65%) and T. trachurus (64%). In contrast, in polychaetes, PVC was the most common polymer (83%) and in S. colias the most common was PE (47%). Additionally, in mussels, 60% of the MP were identified as PET, 20% as PP and 20% as PS; in clams 75% as PET and 25% as PVC; in polychaetes 17% as PET and 83% as PVC; in the Horse mackerel 64% as PET, 27% as PE and 9% as PP; and in the Atlantic chub mackerel 34% as PET, 47 as PE, 16% as PP and 3% as PA. Selected spectra from the analyzed MP are shown in Figure 4.
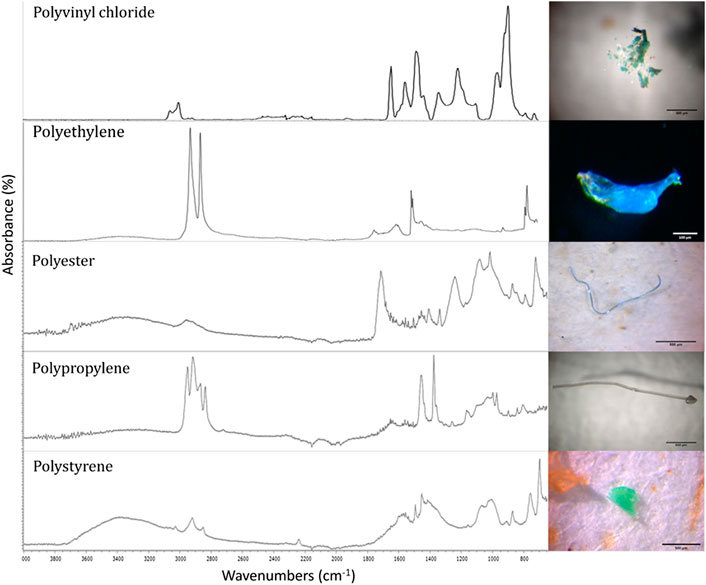
FIGURE 4. Selected infrared spectra of microplastic in the studied species: PVC fragment found in S. plana; PE fragment found in S. colias; PET fiber found in S. plana; PP fiber found in M. galloprovincialis; PS fragment found in M. galloprovincialis (scale bar: 500 μm).
Discussion
This study reports MP presence in five different species (Mytilus galloprovincialis, Scrobicularia plana, Marphysa sanguinea, Trachurus trachurus and Scomber colias), with different feeding strategies and habitats, collected in different locations from the coast of Portugal. The results showed that there was a constant and widespread presence of MP in these species, during the studied period. The plastic particles have been detected in several aquatic environments and in wild conditions in fish, mussels and other species of polychaetes, as already documented by several authors (Li et al., 2015; Van Cauwenberghe et al., 2015; Vandermeersch et al., 2015; Digka et al., 2018; Qu et al., 2018; Fernández and Albentosa, 2019; Li et al., 2019). MP in S. plana have also been recently reported (Piarulli et al., 2020).
A total of 502 MP was recorded in all the 474 individuals analyzed, and their size varied from 73 to 4,680 μm. Fibers were the most common MP recorded in mussels, clams and fish, accounting for approximately 80% of the total counted, which is consistent with other studies for aquatic species (Murphy et al., 2017; Li et al., 2018). The overall abundance of blue MP in all species (64%) might have occurred due to the attractiveness of this color shown by some marine organisms (Ory et al., 2017; Weis, 2020).
MP higher presence in bivalves comparatively to polychaetes probably occurred due to their feeding strategies. Mussels and clams are filter feeding organisms and filter high volumes of water, which can increase their exposure to MP ingestion (Filgueira et al., 2013).
Microplastics in M. galloprovincialis
Microplastic concentrations in mussels did not differ significantly between sites (Tagus and Porto Covo), although the contrary was expected as estuaries are generally more polluted (Vandermeersch et al., 2015), except for fragments which were higher in M. galloprovincialis from Porto Covo.
Mussels are sentinel organisms used for biomonitoring and are commercially important as seafood for human consumption. MP presence in wild mussels has been documented in field studies worldwide (De Witte et al., 2014; Mathalon and Hill, 2014; Li et al., 2015; Van Cauwenberghe et al., 2015; Vandermeersch et al., 2015; Qu et al., 2018). Our results showed a concentration of 0.18 ± 0.31 MP g−1 and 0.45 ± 0.67 MP.ind−1, with a frequency of occurrence of 44%. Fibers were the most common MP type and blue was the most observed color.
Our concentrations are lower than the ones reported in mussels from Belgium (0.51 fibers g−1 and 0.26 fibers g−1), the North Sea (0.36 ± 0.07 MP g−1), Tagus estuary (0.34 ± 0.33 MP g−1), China (1.52–5.36 MP g−1 and 0.77 to 8.22 MP ind−1) and the United Kingdom (between 0.7 and 2.9 MP g−1 and 1.1 to 6.4 MP ind−1) (De Witte et al., 2014; Vandermeersch et al., 2015; Li et al., 2018; Qu et al., 2018). Vandermeersch et al. (2015) reported 0.12 ± 0.04 MP g−1 in mussels collected in Europe and Van Cauwenerghe et al. (2015) 0.2 ± 0.3 fragments g−1 in mussels from the North Sea Coast. These concentrations are similar to the ones reported in this study. The prevalence of fibers as the most common MP is in accordance with other studies (Li et al., 2015; Li et al., 2018; Qu et al., 2018; Scott et al., 2019). The presence of MP in the mussels collected provides further evidence that mussels can be used as MP pollution bioindicator in coastal waters and estuaries (Li et al., 2019).
Microplastics in S. plana
The average concentrations of MP recorded in S. plana were the lowest recorded overall, probably due to the location they were collected, in a lower contaminated zone of Sado estuary (Carrasqueira) (Caeiro et al., 2005). Fibers were the most common MP recorded in clams, similarly to mussels and fish. Blue was the most common color registered in MP ingested by clams, probably due to the presence of intensive fish farms (Caeiro et al., 2005) using blue fishing nets.
Piarulli et al. (2020) studied the presence of MP in different salt marsh species, in which 10 S. plana were sampled from the Schelde estuary in the Netherlands. One MP was found in the S. plana sample: a polyacrylonitrile fiber. Due to the difference in the sample size, this result is not comparable to ours.
There were no significant correlations between the biometric parameters and total MP, fiber and fragment presence in S. plana (Spearman test, α = 0.05). This suggests that MP presence occurs regardless of the size, weight of the clams. In accordance with other authors (Ribeiro et al., 2017), it is suggested to use S. plana as a future biomonitor for MP environmental risks.
Microplastics in M. sanguinea
In polychaetes, fragments were the most common MP, representing 83% of the total MP observed. Accumulation of MP in lugworms has already been studied by some authors that also have detected impacts of exposure to chemicals (Wright et al., 2013; Besseling et al., 2017). Jang et al. (2018) reported 131 ± 131 particles.ind−1 and 24 ± 15 particles.g−1 in M. sanguinea. These results are much higher than the ones reported here and can be explained by the use of EPS buoys for M. sanguinea to live in.
No published work was found about MP presence in M. sanguinea in the field. In this study a total of 12MP was observed in 30 polychaetes, with average concentrations being 0.19 ± 0.40MP.g−1 and 0.40 ± 0.88MP.ind−1. Unlike the other species in this study, fragments were the most common MP in M. sanguinea (83%). The polychaetes also registered the lowest MP average size (223 ± 233 μm), which could be explained by fragments being, in general, smaller than the fibers and might suggest that polychaetes will ingest fragments more easily than fibers. The results obtained from S. plana (sampled from the same site and with a deposit feeding strategy) also seem to support this idea, since only 12% of MP in S. plana were fragments, when compared with 83% fragments found in M. sanguinea.
This study contributes with valuable data regarding the abundance and characteristics of MP found in wild M. sanguinea for the first time, suggesting it as a potential biomonitoring species for MP contamination in sediments.
Microplastics in T. trachurus and S. colias
T. trachurus and S. colias registered a frequency of occurrence of MP of 70% and 55% respectively. This result is higher than the ones observed in T. trachurus (30%) and Scomber spp. (27%) captured between Cape Cantin and Cape Boujdour, Central zone of the Atlantic (Maaghloud et al., 2020) and T. trachurus (42%) from the North East Atlantic Ocean (Barboza et al., 2020). Sparks and Immelman (2020) studied seven fish species including T. trachurus, from the Agulhas Bank, South Africa and reported a frequency of occurrence of 87%, and Herrera et al. (2019) conducted a study on S. colias from the Canary Islands with a 78,4% MP occurrence. Barboza et al. (2020) also studied S. colias and reported a frequency of 62% in the North East Atlantic Ocean. These results are more similar with the result reported by this study. Lopes el al (2020) studied T. trachurus and S. colias from the Western and Southern Iberia and reported a frequency of 100% and 64%, respectively. While the frequency in T. trachurus was higher, in S. colias the result obtained is similar to the one reported here.
Most of the MP found were fibers (79%). This finding is supported by previous studies where fibers were also the most common MP type for several fish species (Neves et al., 2015; Güven et al., 2017; Bessa et al., 2018; Compa et al., 2018; Herrera et al., 2019; Valente et al., 2019; Koongolla et al., 2020; Lopes et al., 2020; Sparks and Immelman, 2020). In more detail, Herrera et al. (2019) found that 74.23% of MP collected in S. colias were fibers, while Barboza et al. (2020) reported that T. trachurus and S. colias specimens from the North coast of Portugal had more fragments (76%) than fibers (22%) and pellets (2%) in the gastrointestinal tract, which is a different result than the one reported in this study. However, other studies conducted in fish from Portuguese waters show a prevalence of fibers as the most common MP even in different habitats and areas (Neves et al., 2015; Bessa et al., 2018; Lopes et al., 2020). Differences in results from different locations could be related to different sources of pollution and waste management strategies (Rochman et al., 2015), and should be monitored.
Blue and black were the most common colors in ingested MP. The predominance of these colors in microplastics has also been previously reported in T. trachurus and S. colias in different parts of the world (Herrera et al., 2019; Barboza et al., 2020) but also in Portuguese waters for the same species (Lopes et al., 2020) and other species of fish (Neves et al., 2015; Bessa et al., 2018; Lopes et al., 2020), which is a widely reported pattern.
Polymer Types
Polymer analyses revealed the presence of polyester (PET), polyethylene (PE), polypropylene (PP), polyvinyl chloride (PVC), polystyrene (PS) and nylon (PA), which is in accordance with the polymers commonly found in the environment, namely PET, PE and PP (Browne et al., 2011), and reflect the recently reported polymer diversity globally described for MP in water and sediments (Gago et al., 2018), since these polymers are the three most abundant worldwide (White et al., 2018). Polyester fibers were found in the majority of the individuals and blue was the predominant color and have probably originated from their massive use in clothing worldwide. These fibers are leached into the environment (Browne et al., 2011) and contribute to ocean plastic pollution (Napper and Thompson, 2016), with sediments being known as sinks for microplastic fibers (Law et al., 2010; Morét-Ferguson et al., 2010; Cózar et al., 2014). Polyester has already been found in mussels (Li et al., 2018) and fish (Rochman et al., 2015; Lefebvre et al., 2019; Koongolla et al., 2020). Our results are also comparable to previous studies in fish collected in Portugal (Neves et al., 2015; Bessa et al., 2018; Barboza et al., 2020). It is worth noticing that in this study we report ingestion of PVC by two endobenthic species, S. plana and M. sanguinea, which can be related to PVC’s deposition on the sediment due to its higher density, once it was not detected in any of the other studied species.
MP ingestion can be a threat to aquatic organisms because, depending on the animal size, MP can be small enough to be expelled along with feces or, if larger, can be retained in the organism causing a false sense of satiety (Butterworth et al., 2012; Woods et al., 2018), while synthetic fibers can get tangled and create agglomerates, blocking organs and therefore hindering or preventing food ingestion (Derraik, 2002). Though there is no evidence of effects in wild aquatic species, laboratory studies reported inflammatory responses upon plastic ingestion in mussels (Von Moos et al., 2012) and neurotoxicity and oxidative damage in fish (Barboza et al., 2020), as well as in other aquatic species as reviewed by Barboza et al. (2018).
It is also important to refer that the comparison of results between different studies is difficult, due to the heterogeneity of the number of individuals analyzed, variability of laboratory procedures and MP extraction and identification methods, and the inconsistency of the reporting units used in results. There is a huge effort being made by the scientific community for standardization of protocols regarding MP studies, which will make future analysis and comparisons more efficient.
The presence of MP in the five species analyzed confirms the current and comprehensive contamination of the marine environment. Despite the knowledge regarding the levels of microplastics in the water and sediments from the coast of Portugal (Frias et al., 2014, 2016; Antunes et al., 2018; Rodrigues et al., 2020), there is still limited information regarding the distribution of microplastics in inland waters and sediments (such as estuarine areas) like those analyzed in the present study. This information would be important for assessing if the levels of microplastics found in the studied species reflect the concentrations found in the environment.
Microplastics entering the marine food webs may affect important seafood species. The results obtained should raise concern regarding bioaccumulation and possible human health risks associated with the consumption of MP contaminated fish and shellfish (Li et al., 2019). Selecting suitable species for monitoring microplastics pollution is an essential step toward achieving the good environmental status aimed by the Marine Strategy Framework Directive (MSFD). The suitable monitoring species listed for the Mediterranean Sea and the Northeast Atlantic, such as the sea turtle Caretta caretta and the sea bird Fulmarus glacialis, respectively, are very rare in Portuguese coastal waters, making it necessary to find and select suitable species for monitoring (IPMA, 2018). Despite the need for more research, this work provides baseline data from five species representing different habitats and feeding strategies with the potential to be used for monitoring microplastics. In addition, these species are ecologically and economically important and can be found in several locations along the Portuguese coast.
Data Availability Statement
The raw data supporting the conclusion of this article will be made available by the authors, without undue reservation.
Ethics Statement
Ethical review and approval was not required for this animal study because the vertebrates (fish samples) were fished by local fishermen for commercial purposes and were no longer alive when made available for this study. The remaining animal samples were bivalves and polychaetes—non-higher invertebrates.
Author Contributions
PS conceptualised the study; JP and VD collected the samples and performed the experiment; JA performed the micro-FTIR analysis; FB introduced and taught the laboratory procedures; JP and JA drafted the manuscript; JP, JA, FB, and PS contributed to improve the manuscript.
Conflict of Interest
The authors declare that the research was conducted in the absence of any commercial or financial relationships that could be construed as a potential conflict of interest.
Acknowledgments
The authors acknowledge FCT—Foundation for Science and Technology (Portugal) through the strategic project UIDB/04292/2020 granted to MARE. JA also acknowledges FCT—Foundation for Science and Technology (Portugal) for financial support through the JPI-Oceans BASEMAN project fellowship (Ref. JPIOCEANS/0001/2015). FB also acknowledges the University of Coimbra for her contract IT057-18-7252. The authors also acknowledge Docapesca, S.A. for providing the fish species.
References
Antunes, J., Frias, J., and Sobral, P. (2018). Microplastics on the Portuguese coast. Mar. Pollut. Bull. 131, 294–302. doi:10.1016/j.marpolbul.2018.04.025
Arienzo, M., Toscanesi, M., Trifuoggi, M., Ferrara, L., Stanislao, C., Donadio, C., et al. (2019). Contaminants bioaccumulation and pathological assessment in Mytilus galloprovincialis in coastal waters facing the brownfield site of Bagnoli, Italy. Mar. Pollut. Bull. 140 (January), 341–352. doi:10.1016/j.marpolbul.2019.01.064
Arthur, C., Baker, J., and Bamford, H. (2009). NOAA technical memorandum NOS-or and R-30. Proceedings of the International Research Workshop on the Occurrence, Effects and Fate of Microplastic Marine Debris, Tacoma, WA, United States, 9–11 September, 2008
Avio, C. G., Gorbi, S., Milan, M., Benedetti, M., Fattorini, D., d’Errico, G., et al. (2015). Pollutants bioavailability and toxicological risk from microplastics to marine mussels. Environ. Pollut. 198, 211–222. doi:10.1016/j.envpol.2014.12.021
Barboza, L. G. A., Dick Vethaak, A., Lavorante, B. R. B. O., Lundebye, A., and Guilhermino, L. (2018). Marine microplastic debris: an emerging issue for food security, food safety and human health. Mar. Pollut. Bull. 133, 336–348. doi:10.1016/j.marpolbul.2018.05.047
Barboza, L. G. A., Lopes, C., Oliveira, P., Bessa, F., Otero, V., Henriques, B., et al. (2020). Microplastics in wild fish from North East Atlantic Ocean and its potential for causing neurotoxic effects, lipid oxidative damage, and human health risks associated with ingestion exposure. Sci. Total Environ. 717, 134625. doi:10.1016/j.scitotenv.2019.134625
Bessa, F., Barría, P., Neto, J. M., Frias, J. P. G. L., Otero, V., Sobral, P., et al. (2018). Occurrence of microplastics in commercial fish from a natural estuarine environment. Mar. Pollut. Bull. 128 (January), 575–584. doi:10.1016/j.marpolbul.2018.01.044
Bessa, F., Frias, J., Kögel, T., Lusher, A., Andrade, J., Antunes, J., et al. (2019). Harmonized protocol for monitoring microplastics in biota, D4.3 BASEMAN Report. doi:10.13140/RG.2.2.28588.72321/1
Besseling, E., Quik, J. T., Sun, M., and Koelmans, A. A. (2017). Fate of nano- and microplastic in freshwater systems: a modeling study. Environ. Pollut. 220 (Part A), 540–548. doi:10.1016/j.envpol.2016.10.001
Besseling, E., Wegner, A., Foekema, E. M., Van Den Heuvel-Greve, M. J., and Koelmans, A. A. (2013). Effects of microplastic on fitness and PCB bioaccumulation by the lugworm Arenicola marina (L.). Environ. Sci. Technol. 47 (1), 593–600. doi:10.1021/es302763x
Browne, M. A., Crump, P., Niven, S. J., Teuten, E., Tonkin, A., Galloway, T., et al. (2011). Accumulation of microplastic on shorelines woldwide: sources and sinks. Environ. Sci. Technol. 45 (21), 9175–9179. doi:10.1021/es201811s
Butterworth, A., Clegg, I., and Bass, C. (2012). Untangled. Marine debris: A global picture of the impact on Animal welfare and of animal-focused solutions. London, United Kingdom: World Society for the Protection of Animals, 1–78
Caeiro, S., Costa, M. H., Ramos, T. B., Fernandes, F., Silveira, N., Coimbra, A., et al. (2005). Assessing heavy metal contamination in Sado Estuary sediment: an index analysis approach. Ecol. Indicat. 5 (2), 151–169. doi:10.1016/j.ecolind.2005.02.001
Cincinelli, A., Martellini, T., Guerranti, C., Scopetani, C., Chelazzi, D., and Giarrizzo, T. (2019). A potpourri of microplastics in the sea surface and water column of the Mediterranean Sea. Trac. Trends Anal. Chem. 110, 321–326. doi:10.1016/j.trac.2018.10.026
Compa, M., Ventero, A., Iglesias, M., and Deudero, S. (2018). Ingestion of microplastics and natural fibres in Sardina pilchardus (Walbaum, 1792) and Engraulis encrasicolus (Linnaeus, 1758) along the Spanish Mediterranean coast. Mar. Pollut. Bull. 128 (January), 89–96. doi:10.1016/j.marpolbul.2018.01.009
Cózar, A., Echevarría, F., González-Gordillo, J. I., Irigoien, X., Úbeda, B., Hernández-León, S., et al. (2014). Plastic debris in the open ocean. .Proc. Natl. Acad. Sci. United States 111 (28), 10239–10244. doi:10.1073/pnas.1314705111
De Falco, F., Di Pace, E., Cocca, M., and Avella, M. (2019). The contribution of washing processes of synthetic clothes to microplastic pollution. Sci. Rep. 9 (1), 6633. doi:10.1038/s41598-019-43023-x
de Sá, L. C., Oliveira, M., Ribeiro, F., Rocha, T. L., and Futter, M. N. (2018). Studies of the effects of microplastics on aquatic organisms: what do we know and where should we focus our efforts in the future?. Sci. Total Environ. 645, 1029–1039. doi:10.1016/j.scitotenv.2018.07.207
De Witte, B., Devriese, L., Bekaert, K., Hoffman, S., Vandermeersch, G., Cooreman, K., et al. (2014). Quality assessment of the blue mussel (Mytilus edulis): comparison between commercial and wild types. Mar. Pollut. Bull. 85 (1), 146–155. doi:10.1016/j.marpolbul.2014.06.006
Dehaut, A., Cassone, A. L., Frère, L., Hermabessiere, L., Himber, C., Rinnert, E., et al. (2016). Microplastics in seafood: benchmark protocol for their extraction and characterization. Environ. Pollut. 215, 223–233. doi:10.1016/j.envpol.2016.05.018
Derraik, J. G. B. (2002). The pollution of the marine environment by plastic debris: a review. Mar. Pollut. Bull. 44 (9), 842–852. doi:10.1016/s0025-326x(02)00220-5
Desforges, J. P., Galbraith, M., and Ross, P. S. (2015). Ingestion of microplastics by zooplankton in the Northeast pacific ocean. Arch. Environ. Contam. Toxicol. 69 (3), 320–330. doi:10.1007/s00244-015-0172-5
Digka, N., Tsangaris, C., Torre, M., Anastasopoulou, A., and Zeri, C. (2018). Microplastics in mussels and fish from the northern ionian sea. Mar. Pollut. Bull. 135 (June), 30–40. doi:10.1016/j.marpolbul.2018.06.063
Eriksen, M., Lebreton, L. C., Carson, H. S., Thiel, M., Moore, C. J., Borerro, J. C., et al. (2014). Plastic pollution in the world’s oceans: more than 5 trillion plastic pieces weighing over 250,000 tons afloat at sea. PLoS One 9 (12), e111913–15. doi:10.1371/journal.pone.0111913
Erni-Cassola, G., Gibson, M. I., Thompson, R. C., and Christie-Oleza, J. A. (2017). Lost, but found with nile red: a novel method for detecting and quantifying small microplastics (1 mm to 20 μm) in environmental samples. Environ. Sci. Technol. 51 (23), 13641–13648. doi:10.1021/acs.est.7b04512
European Commission (2018a). A European strategy to protect the planet, defend our citizens. Plastic Waste: http://europa.eu/rapid/press-release_IP-18-5_en.htm
European Commission (2018b). New EU rules to reduce marine litter. Single-use plastics: http://europa.eu/rapid/press-release_IP-18-3927_en.htm
Fahrenfeld, N. L., Arbuckle-Keil, G., Naderi Beni, N., and Bartelt-Hunt, S. L. (2019). Source tracking microplastics in the freshwater environment. Trac. Trends Anal. Chem. 112, 248–254. doi:10.1016/j.trac.2018.11.030
FAO (2005). A world overview of species of interest to fisheries. FIGIS Species Fact Sheets. Species identification and data programme—SIDP, FAO-FIGIS: www.fao.org/figis/servlet/species?fid=2473.
Farrell, P., and Nelson, K. (2013). Trophic level transfer of microplastic: Mytilus edulis (L.) to Carcinus maenas (L.). Environ. Pollut. 177, 1–3. doi:10.1016/j.envpol.2013.01.046
Fauchald, K., and Jumars, P. A. (1979). The diet of worms: a study of polychaete feeding guilds. Oceanography and Marine Biology. Annu. Rev. 17, 193–284
Fernández, B., and Albentosa, M. (2019). Insights into the uptake, elimination and accumulation of microplastics in mussel. Environ. Pollut. 249, 321–329. doi:10.1016/j.envpol.2019.03.037
Filgueira, R., Comeau, L. A., Landry, T., Grant, J., Guyondet, T., and Mallet, A. (2013). Bivalve condition index as an indicator of aquaculture intensity: a meta-analysis. Ecol. Indicat. 25, 215–229. doi:10.1016/j.ecolind.2012.10.001
Foekema, E. M., De Gruijter, C., Mergia, M. T., van Franeker, J. A., Koelmans, A. A., et al. (2013). Plastic in North Sea fish. Environ. Sci. Technol. 47 (15), 8818–8824. doi:10.1021/es400931b
Fred-Ahmadu, O. H., Bhagwat, G., Oluyoye, I., Benson, N. U., Ayejuyo, O. O., and Palanisami, T. (2020). Interaction of chemical contaminants with microplastics: principles and perspectives. Sci. Total Environ. 706, 135978. doi:10.1016/j.scitotenv.2019.135978
Frias, J. P., Gago, J., Otero, V., and Sobral, P. (2016). Microplastics in coastal sediments from Southern Portuguese shelf waters. Mar. Environ. Res. 114, 24–30. doi:10.1016/j.marenvres.2015.12.006
Frias, J. P., Otero, V., and Sobral, P. (2014). Evidence of microplastics in samples of zooplankton from Portuguese coastal waters. Mar. Environ. Res. 95, 89–95. doi:10.1016/j.marenvres.2014.01.001
Frias, J. P. G. L., and Nash, R. (2019). Microplastics: finding a consensus on the definition. Mar. Pollut. Bull. 138 (November 2018), 145–147
Gago, J., Carretero, O., Filgueiras, A. V., and Viñas, L. (2018). Synthetic microfibers in the marine environment: a review on their occurrence in seawater and sediments. Mar. Pollut. Bull. 127 (July 2017), 365–376. doi:10.1016/j.marpolbul.2017.11.070
Galgani, F., Oosterbaan, L., Poitou, I., Hanke, G., Thompson, R., Amato, E., et al. (2010). Marine strategy Framework directive. Task Group 10 Report Marine Litter. In Group (Issue 31210) Katsanevakis. doi:10.2788/86941
Gall, S. C., and Thompson, R. C. (2015). The impact of debris on marine life. Mar. Pollut. Bull. 92 (1–2), 170–179. doi:10.1016/j.marpolbul.2014.12.041
Godoy, V., Martín-Lara, M. A., Calero, M., and Blázquez, G. (2019). Physical-chemical characterization of microplastics present in some exfoliating products from Spain. Mar. Pollut. Bull. 139 (October 2018), 91–99. doi:10.1016/j.marpolbul.2018.12.026
González-Domínguez, R., Santos, H. M., Bebianno, M. J., García-Barrera, T., Gómez-Ariza, J. L., and Capelo, J. L. (2016). Combined proteomic and metallomic analyses in Scrobicularia plana clams to assess environmental pollution of estuarine ecosystems. Mar. Pollut. Bull. 113 (1–2), 117–124. doi:10.1016/j.marpolbul.2016.08.075
Gosling, E. M. (1992). The mussel Mytilus: ecology, physiology, genetics and culture. Amsterdam, Netherlands: Elsevier Science Publishers Amsterdam
Güven, O., Gökdağ, K., Jovanović, B., and Kıdeyş, A. E. (2017). Microplastic litter composition of the Turkish territorial waters of the Mediterranean Sea, and its occurrence in the gastrointestinal tract of fish. Environ. Pollut. 223, 286–294. doi:10.1016/j.envpol.2017.01.025
Hanke, G., Galgani, F., Werner, S., Oosterbaan, L., Nilsson, P., Fleet, D., et al. (2013). MSFD GES technical subgroup on marine litter. Guidance on monitoring of marine litter in European seas. Publications office of the European union. Luxembourg, United Kingdom: Joint Research Centre–Institute for Environment and Sustainability. doi:10.2788/99816
Herrera, A., Ŝtindlová, A., Martínez, I., Rapp, J., Romero-Kutzner, V., Samper, M. D., et al. (2019). Microplastic ingestion by Atlantic chub mackerel (Scomber colias) in the Canary Islands coast. Mar. Pollut. Bull. 139 (January), 127–135. doi:10.1016/j.marpolbul.2018.12.022
Hitchcock, J. N., and Mitrovic, S. M. (2019). Microplastic pollution in estuaries across a gradient of human impact. Environ. Pollut. 247 (January), 457–466. doi:10.1016/j.envpol.2019.01.069
Hoarau, L., Ainley, L., Jean, C., and Ciccione, S. (2014). Ingestion and defecation of marine debris by loggerhead sea turtles, Caretta caretta, from by-catches in the South-West Indian Ocean. Mar. Pollut. Bull. 84 (1–2), 90–96. doi:10.1016/j.marpolbul.2014.05.031
Hurley, R. R., Woodward, J. C., and Rothwell, J. J. (2017). Ingestion of microplastics by freshwater tubifex worms. Environ. Sci. Technol. 51 (21), 12844–12851. doi:10.1021/acs.est.7b03567
IPMA. (2018). DIRETIVA-QUADRO “estratégia MARINHA” descritor 10-lixo marinho. Relatório do segundo ciclo de avaliação do bom estado ambiental das águas marinhas nas subdivisões do Continente e da Plataforma Continental Estendida, Nijkerk, Netherlands: IPMA, 1–26.
Ivar Do Sul, J. A., and Costa, M. F. (2014). The present and future of microplastic pollution in the marine environment. Environ. Pollut. 185, 352–364. doi:10.1016/j.envpol.2013.10.036
Jambeck, J. R., Geyer, R., Wilcox, C., Siegler, T. R., Perryman, M., Andrady, A., et al. (2015). Marine pollution. Plastic waste inputs from land into the ocean. Science 347, 768. doi:10.1126/science.1260352
Jang, M., Shim, W. J., Han, G. M., Song, Y. K., and Hong, S. H. (2018). Formation of microplastics by polychaetes (Marphysa sanguinea) inhabiting expanded polystyrene marine debris. Mar. Pollut. Bull. 131 (January), 365–369. doi:10.1016/j.marpolbul.2018.04.017
Jiang, C., Yin, L., Li, Z., Wen, X., Luo, X., Hu, S., et al. (2019). Microplastic pollution in the rivers of the tibet plateau. Environ. Pollut. 249, 91–98. doi:10.1016/j.envpol.2019.03.022
Kanhai, L. D. K., Johansson, C., Frias, J. P. G. L., Gardfeldt, K., Thompson, R. C., and O’Connor, I. (2019). Deep sea sediments of the Arctic Central Basin: a potential sink for microplastics. deep-sea research part I:. Oceanographic Research Papers 145 (June 2018), 137–142. doi:10.1016/j.dsr.2019.03.003
Karami, A., Golieskardi, A., Choo, C. K., Romano, N., Ho, Y., Salamatinia, B., et al. (2017). A high-performance protocol for extraction of microplastics in fish. Sci. Total Environ. 578, 485–494. doi:10.1016/j.scitotenv.2016.10.213
Kolandhasamy, P., Su, L., Li, J., Qu, X., Jabeen, K., and Shi, H. (2018). Adherence of microplastics to soft tissue of mussels: a novel way to uptake microplastics beyond ingestion. Sci. Total Environ. 610-611, 635–640. doi:10.1016/j.scitotenv.2017.08.053
Koongolla, J. B., Lin, L., Pan, Y. F., Yang, C. P., Sun, D. R., Liu, S., et al. (2020). Occurrence of microplastics in gastrointestinal tracts and gills of fish from Beibu Gulf, South China Sea. Environ. Pollut. 258, 113734. doi:10.1016/j.envpol.2019.113734
Kühn, S., van Werven, B., van Oyen, A., Meijboom, A., Bravo Rebolledo, E. L., and van Franeker, J. A. (2017). The use of potassium hydroxide (KOH) solution as a suitable approach to isolate plastics ingested by marine organisms. Mar. Pollut. Bull. 115, 86–90. doi:10.1016/j.marpolbul.2016.11.034
Langston, W. J., Burt, G. R., and Chesman, B. S. (2007). Feminisation of male clams Scrobicularia plana from estuaries in Southwest UK and its induction by endocrine-disrupting chemicals. Mar. Ecol.: Prog. Ser. 333, 173–184. doi:10.3354/meps333173
Law, K. L., Morét-Ferguson, S., Maximenko, N. A., Proskurowski, G., Peacock, E. E., Hafner, J., et al. (2010). Plastic accumulation in the North Atlantic subtropical gyre. Science 329, 1185. doi:10.1126/science.1192321
Lefebvre, C., Saraux, C., Heitz, O., Nowaczyk, A., and Bonnet, D. (2019). Microplastics FTIR characterisation and distribution in the water column and digestive tracts of small pelagic fish in the gulf of lions. Mar. Pollut. Bull. 142 (April), 510–519. doi:10.1016/j.marpolbul.2019.03.025
Li, J., Green, C., Reynolds, A., Shi, H., and Rotchell, J. M. (2018). Microplastics in mussels sampled from coastal waters and supermarkets in the United Kingdom. Environ. Pollut. 241, 35–44. doi:10.1016/j.envpol.2018.05.038
Li, J., Lusher, A. L., Rotchell, J. M., Deudero, S., Turra, A., Bråte, I. L. N., et al. (2019). Using mussel as a global bioindicator of coastal microplastic pollution. Environ. Pollut. 244, 522–533. doi:10.1016/j.envpol.2018.10.032
Li, J., Yang, D., Li, L., Jabeen, K., and Shi, H. (2015). Microplastics in commercial bivalves from China. Environ. Pollut. 207, 190–195. doi:10.1016/j.envpol.2015.09.018
Long, M., Moriceau, B., Gallinari, M., Lambert, C., Huvet, A., Raffray, J., et al. (2015). Interactions between microplastics and phytoplankton aggregates: impact on their respective fates. Mar. Chem. 175, 39–46. doi:10.1016/j.marchem.2015.04.003
Lopes, C., Raimundo, J., Caetano, M., and Garrido, S. (2020). Microplastic ingestion and diet composition of planktivorous fish. Limnology and Oceanography Letters 5 (1), 103–112. doi:10.1002/lol2.10144
Lusher, A. (2015). Microplastics in the marine environment distribution, interactions and effects. in Marine anthropogenic litter. Editors M. Bergmann, L. Gutow, and M Klages (Cham, Switzerland: Springer) doi:10.1007/978-3-319-16510-3_10
Maaghloud, H., Houssa, R., Ouansafi, S., Bellali, F., El Bouqdaoui, K., Charouki, N., et al. (2020). Ingestion of microplastics by pelagic fish from the Moroccan Central Atlantic coast. Environ. Pollut. 261, 114194. doi:10.1016/j.envpol.2020.114194
Mathalon, A., and Hill, P. (2014). Microplastic fibers in the intertidal ecosystem surrounding halifax harbor, nova scotia. Mar. Pollut. Bull. 81 (1), 69–79. doi:10.1016/j.marpolbul.2014.02.018
Morét-Ferguson, S., Lavender, K., Proskurowski, G., Murphy, E. K., Peacock, E. E., and Reddy, C. M. (2010). The size, mass, and composition of plastic debris in the western North Atlantic Ocean. Mar. Pollut. Bull. 60, 1873–1878. doi:10.1016/j.marpolbul.2010.07.020
Murphy, F., Russell, M., Ewins, C., and Quinn, B. (2017). The uptake of macroplastic and microplastic by demersal and pelagic fish in the Northeast Atlantic around Scotland. Mar. Pollut. Bull. 122 (1–2), 353–359. doi:10.1016/j.marpolbul.2017.06.073
Napper, I. E., Bakir, A., Rowland, S. J., and Thompson, R. C. (2015). Characterisation, quantity and sorptive properties of microplastics extracted from cosmetics. Mar. Pollut. Bull. 99 (1–2), 178–185. doi:10.1016/j.marpolbul.2015.07.029
Napper, I. E., and Thompson, R. C. (2016). Release of synthetic microplastic plastic fibres from domestic washing machines: effects of fabric type and washing conditions. Mar. Pollut. Bull. 112 (1–2), 39–45. doi:10.1016/j.marpolbul.2016.09.025
Neves, D., Sobral, P., Ferreira, J. L., and Pereira, T. (2015). Ingestion of microplastics by commercial fish off the Portuguese coast. Mar. Pollut. Bull. 101 (1), 119–126. doi:10.1016/j.marpolbul.2015.11.008
Ory, N. C., Sobral, P., Ferreira, J. L., and Thiel, M. (2017). Amberstripe scad Decapterus muroadsi (Carangidae) fish ingest blue microplastics resembling their copepod prey along the coast of Rapa Nui (Easter Island) in the South Pacific subtropical gyre. Sci. Total Environ. 586, 430–437. doi:10.1016/j.scitotenv.2017.01.175
Pan, Z., Sun, X., Guo, H., Cai, S., Chen, H., Wang, S., et al. (2019). Prevalence of microplastic pollution in the northwestern pacific ocean. Chemosphere 225 (March), 735–744. doi:10.1016/j.chemosphere.2019.03.076
Piarulli, S., Vanhove, B., Comandini, P., Scapinello, S., Moens, T., Vrielinck, H., et al. (2020). Do different habits affect microplastics contents in organisms? A trait-based analysis on salt marsh species. Mar. Pollut. Bull. 153, 110983. doi:10.1016/j.marpolbul.2020.110983
Piperagkas, O., Papageorgiou, N., and Karakassis, I. (2019). Qualitative and quantitative assessment of microplastics in three sandy Mediterranean beaches, including different methodological approaches. estuarine. Coastal and Shelf Science 219 (August 2018), 169–175. doi:10.1016/j.ecss.2019.02.016
GESAMP (2019). “Guidelines for the monitoring and assessment of plastic litter in the ocean (, and editors),” Rep. Stud. GESAMP, in IMO/FAO/UNESCO-IOC/UNIDO/WMO/IAEA/UN/UNEP/UNDP/ISA joint group of experts on the scientific aspects of marine environmental prote. Editors P.J. Kershaw, A. Turra, and F. Galgani, 99, 130.
GESAMP (2016). Sources, fate and effects of microplastics in the marine environment, (IMO, FAO/UNESCO-IOC/UNIDO/WMO/IAEA/UN/UNEP/UNDP). In: (Ed.), Rep. Stud. GESAMP No. 90–96 pp). Joint group of experts on the scientific aspects of marine environmental protection. (2016). in Part 2 of a global assessment 93. Editor P.J. Kershaw
PlasticsEurope (2018). Plastics–the Facts 2018: an analysis of European plastics production, demand and waste data. Plastics Europe, association of plastic manufacturers, 38
Prevedelli, D., Massamba N’siala, G., Ansaloni, I., and Simonini, R. (2007). Life cycle of Marphysa sanguinea (polychaeta: eunicidae) in the Venice Lagoon (Italy). Mar. Ecol. 28 (3), 384–393. doi:10.1111/j.1439-0485.2007.00160.x
Prokić, M. D., Radovanović, T. B., Gavrić, J. P., and Faggio, C. (2019). Ecotoxicological effects of microplastics: examination of biomarkers, current state and future perspectives. Trac. Trends Anal. Chem. 111, 37–46. doi:10.1016/j.trac.2018.12.001
Qu, X., Su, L., Li, H., Liang, M., and Shi, H. (2018). Assessing the relationship between the abundance and properties of microplastics in water and in mussels. Sci. Total Environ. 621, 679–686. doi:10.1016/j.scitotenv.2017.11.284
Reed, S., Clark, M., Thompson, R., and Hughes, K. A. (2018). Microplastics in marine sediments near Rothera research station, Antarctica. Mar. Pollut. Bull. 133 (June), 460–463. doi:10.1016/j.marpolbul.2018.05.068
Retama, I., Jonathan, M. P., Shruti, V. C., Velumani, S., Sarkar, S. K., Roy, P. D., et al. (2016). Microplastics in tourist beaches of Huatulco Bay, Pacific coast of Southern Mexico. Mar. Pollut. Bull. 113 (1–2), 530–535. doi:10.1016/j.marpolbul.2016.08.053
Ribeiro, F., Garcia, A. R., Pereira, B. P., Fonseca, M., Mestre, N. C., Fonseca, T. G., et al. (2017). Microplastics effects in Scrobicularia plana. Mar. Pollut. Bull. 122 (1–2), 379–391. doi:10.1016/j.marpolbul.2017.06.078
Rochman, C. M., Tahir, A., Williams, S. L., Baxa, D. V., Lam, R., Miller, J. T., et al. (2015). Anthropogenic debris in seafood: plastic debris and fibers from textiles in fish and bivalves sold for human consumption. Sci. Rep. 5 (April), 14340. doi:10.1038/srep14340
Rodrigues, S. M., Almeida, C. M. R., Silva, D., Cunha, J., Antunes, C., Freitas, V., et al. (2019). Microplastic contamination in an urban estuary: abundance and distribution of microplastics and fish larvae in the Douro estuary. Sci. Total Environ. 659, 1071–1081. doi:10.1016/j.scitotenv.2018.12.273
Rodrigues, D., Antunes, J., Otero, V., Sobral, P., and Costa, M. H. (2020). Distribution patterns of microplastics in seawater surface at a Portuguese estuary and marine park. Front. Environ. Sci. 8, 582217. doi:10.3389/fenvs.2020.582217
Scott, N., Porter, A., Santillo, D., Simpson, H., Lloyd-Williams, S., and Lewis, C. (2019). Particle characteristics of microplastics contaminating the mussel Mytilus edulis and their surrounding environments. Mar. Pollut. Bull. 146 (March), 125–133. doi:10.1016/j.marpolbul.2019.05.041
Seo, J. K., Nam, B. H., Go, H. J., Jeong, M., Lee, K. Y., Cho, S. M., et al. (2016). Hemerythrin-related antimicrobial peptide, msHemerycin, purified from the body of the Lugworm, Marphysa sanguinea. Fish Shellfish Immunol. 57, 49–59. doi:10.1016/j.fsi.2016.08.018
Sheavly, S. B. (2005). Marine debris—an overview of an critical issue for the oceans. In sixth meeting of the UN open-ended informal consultative process on oceans and the law of the sea. 7 Available at: https://www.un.org/Depts/los/consultative_process/documents/6_sheavly.pdf
Sparks, C., and Immelman, S. (2020). Microplastics in offshore fish from the Agulhas Bank, South Africa. Mar. Pollut. Bull. 156, 111216. doi:10.1016/j.marpolbul.2020.111216
Stock, F., Kochleus, C., Bänsch-Baltruschat, B., Brennholt, N., and Reifferscheid, G. (2019). Sampling techniques and preparation methods for microplastic analyses in the aquatic environment–a review. Trac. Trends Anal. Chem. 113, 84–92. doi:10.1016/j.trac.2019.01.014
Su, L., Cai, H., Kolandhasamy, P., Wu, C., Rochman, C. M., and Shi, H. (2018). Using the Asian clam as an indicator of microplastic pollution in freshwater ecosystems. Environ. Pollut. 234, 347–355. doi:10.1016/j.envpol.2017.11.075
Suaria, G., Perold, V., Lee, J. R., Lebouard, F., Aliani, S., and Ryan, P. G. (2020). Floating macro- and microplastics around the southern ocean: results from the antarctic circumnavigation expedition. Environ. Int. 136, 105494. doi:10.1016/j.envint.2020.105494
Sun, J., Dai, X., Wang, Q., van Loosdrecht, M. C. M., and Ni, B. J. (2019). Microplastics in wastewater treatment plants: detection, occurrence and removal. Water Res. 152, 21–37. doi:10.1016/j.watres.2018.12.050
Tan, X., Yu, X., Cai, L., Wang, J., and Peng, J. (2019). Microplastics and associated PAHs in surface water from the feilaixia Reservoir in the beijiang river, China. Chemosphere 221, 834–840. doi:10.1016/j.chemosphere.2019.01.022
Tanaka, K., Takada, H., Yamashita, R., Mizukawa, K., and Fukuwaka, M. (2013). aki, and Watanuki, YAccumulation of plastic-derived chemicals in tissues of seabirds ingesting marine plastics. Mar. Pollut. Bull. 69 (1–2), 219–222. doi:10.1016/j.marpolbul.2012.12.010
Thompson, R. C., Moore, C. J., vom Saal, F. S., and Swan, S. H. (2009). Plastics, the environment and human health: current consensus and future trends. Philos. Trans. R. Soc. Lond. B Biol. Sci. 364 (1526), 2153–2166. doi:10.1098/rstb.2009.0053
Triebskorn, R., Braunbeck, T., Grummt, T., Hanslik, L., Huppertsberg, S., Jekel, M., et al. (2019). Relevance of nano- and microplastics for freshwater ecosystems: a critical review. Trac. Trends Anal. Chem. 110, 375–392 doi:10.1016/j.trac.2018.11.023
UNEP (2016). Marine plastic debris and microplastics – global lessons and research to inspire action and guide policy change. Nairobi, Kenya: United Nations Environment Programme.
Valente, T., Sbrana, A., Scacco, U., Jacomini, C., Bianchi, J., Palazzo, L., et al. (2019). Exploring microplastic ingestion by three deep-water elasmobranch species: a case study from the Tyrrhenian sea. Environ. Pollut. 253, 342–350. doi:10.1016/j.envpol.2019.07.001
Van Cauwenberghe, L., Claessens, M., Vandegehuchte, M. B., and Janssen, C. R. (2015). Microplastics are taken up by mussels (Mytilus edulis) and lugworms (Arenicola marina) living in natural habitats. Environ. Pollut. 199, 10–17. doi:10.1016/j.envpol.2015.01.008
Vandermeersch, G., Van Cauwenberghe, L., Janssen, C. R., Marques, A., Granby, K., Fait, G., et al. (2015). A critical view on microplastic quantification in aquatic organisms. Environ. Res. 143, 46–55. doi:10.1016/j.envres.2015.07.016
Veiga, J. M., Fleet, D., Kinsey, S., Nilsson, P., Vlachogianni, T., Werner, S., et al. (2016). Identifying sources of marine litter. In JRC Technical Report (Issue January 2017). doi:10.2788/018068
Von Moos, N., Burkhardt-Holm, P., and Köhler, A. (2012). Uptake and effects of microplastics on cells and tissue of the blue mussel Mytilus edulis L. after an experimental exposure. Environ. Sci. Technol. 46 (20), 11327–11335. doi:10.1021/es302332w
Wang, T., Zou, X., Li, B., Yao, Y., Li, J., Hui, H., et al. (2018). Microplastics in a wind farm area: a case study at the Rudong offshore wind farm, yellow sea, China. Mar. Pollut. Bull. 128 (November 2017), 466–474. doi:10.1016/j.marpolbul.2018.01.050
Watts, A. J., Lewis, C., Goodhead, R. M., Beckett, S. J., Moger, J., Tyler, C. R., et al. (2014). Uptake and retention of microplastics by the shore crab carcinus maenas. Environ. Sci. Technol. 48 (15), 8823–8830. doi:10.1021/es501090e
Weis, J. S. (2020). Aquatic microplastic research—a critique and suggestions for the future.Water 12(5) 1475. doi:10.3390/w12051475
White, E., Clark, S., Manire, C. A., Crawford, B., Wang, S., Locklin, J., et al. (2018). Ingested micronizing plastic particle compositions and size distributions within stranded post-hatchling sea turtles. Environ. Sci. Technol. 52 (18), 10307–10316. doi:10.1021/acs.est.8b02776
Woods, M. N., Stack, M. E., Fields, D. M., Shaw, S. D., and Matrai, P. A. (2018). Microplastic fiber uptake, ingestion, and egestion rates in the blue mussel (Mytilus edulis). Mar. Pollut. Bull. 137 (November), 638–645. doi:10.1016/j.marpolbul.2018.10.061
Wright, S. L., Rowe, D., Thompson, R. C., and Galloway, T. S. (2013). Microplastic ingestion decreases energy reserves in marine worms. Curr. Biol. 23 (23), R1031–R1033. doi:10.1016/j.cub.2013.10.068
Keywords: microplastics, plastic pollution, mussels, peppery furrow shell, polychaetes, horse mackerel, atlantic chub mackerel, coastal waters
Citation: Pequeno J, Antunes J, Dhimmer V, Bessa F and Sobral P (2021) Microplastics in Marine and Estuarine Species From the Coast of Portugal. Front. Environ. Sci. 9:579127. doi: 10.3389/fenvs.2021.579127
Received: 01 July 2020; Accepted: 18 January 2021;
Published: 24 February 2021.
Edited by:
Cristina Panti, University of Siena, ItalyReviewed by:
Jesus Javier Ojeda, Swansea University, United KingdomCatherine Tsangaris, Hellenic Center for Marine Research (HCMR), Greece
Copyright © 2021 Pequeno, Antunes, Dhimmer, Bessa and Sobral. This is an open-access article distributed under the terms of the Creative Commons Attribution License (CC BY). The use, distribution or reproduction in other forums is permitted, provided the original author(s) and the copyright owner(s) are credited and that the original publication in this journal is cited, in accordance with accepted academic practice. No use, distribution or reproduction is permitted which does not comply with these terms.
*Correspondence: João Pequeno, j.pequeno@fct.unl.pt