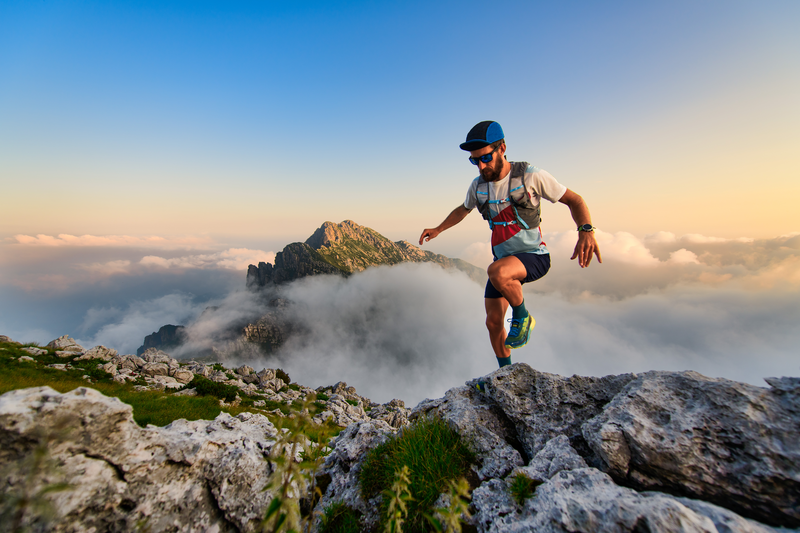
95% of researchers rate our articles as excellent or good
Learn more about the work of our research integrity team to safeguard the quality of each article we publish.
Find out more
ORIGINAL RESEARCH article
Front. Environ. Sci. , 05 August 2021
Sec. Soil Processes
Volume 9 - 2021 | https://doi.org/10.3389/fenvs.2021.574883
This article is part of the Research Topic Desertification and Rehabilitation View all 16 articles
Plant residual is of great importance in retarding soil wind erosion in the agro-pastoral ecotone. However, few studies have determined the effects of sand plant residual on wind erosion resistance. Based on field surveys, the influences of Salix residual biomass of 200, 400, 600, and 800 g m−2, soil incorporated with a residual thickness of 0.5, 1.0, and 2.0 cm, and typical proportion of residual branches and leaves (2:1, 1:1, and 1:2) on wind erosion resistance were investigated using a simulated wind tunnel. The results showed the following: 1) The soil loss amount ranged from 1.56 to 40.8 kg m−2 as Salix residual biomass decreased from 800 to 0 g m−2, with a critical residual biomass value of 400 g m−2. 2) As the thickness of soil-incorporated residual increased, the soil loss amount reduced rapidly, especially for 0–9 cm above the surface accounting for 84.6% of the total. 3) Salix branch residual is more important in resisting soil wind erosion as compared with its leaves. This kind of study may provide theoretical explanations for the optimal reconstruction of sandy vegetation in the northern wind-sand regions.
Wind erosion is an environmental concern in arid and semi-arid regions due to the emission of particulates into the atmosphere (He, 2014; Tuo et al., 2016; Zhang et al., 2018). Decades of practice showed that sandy vegetation construction is an effective method of ecological reconstruction in the northern wind-sand regions (Yu et al., 2017; Zhang et al., 2019). Most recently, research has been focused on the interactions between sandy vegetation and its influence on the soil quality in the agro-pastoral ecotone of northern China (Zhang and Zhao, 2015; Wang et al., 2020) and the relationship between plant distribution patterns and wind erosion resistance (He, 2014; Li et al., 2016, Li et al., 2017). The redistribution of leaves and fruits caused by the northwest wind is the main reason for the formation of different plant residual patterns (Zhao et al., 2003; Li et al., 2020). However, few studies have determined the effects of sand plant residual on wind erosion resistance.
Salix (Salix psammophila) is typical vegetation in the Mu Us Sandy Land, which is one of the first choices of the “Three-North Shelter Forest Program” in China. The Three-North Shelter Forest Program is a large-scale artificial forestry ecological project in the three northern areas of China (northwest, north, and northeast) (Li et al., 2016). Previous studies related to wind erosion have shown that Salix residual community can form obvious patterns in the dune slope; the spatial and temporal variation of this could strongly influence the vegetation species composition, community structure, and soil quality development due to the long-term effects of wind (Sun et al., 2016b; Liu et al., 2017). In addition, the accumulation and redistribution process of plant residual can cause significant biological effects on the surface micro-environment and is an important factor affecting soil–plant nutrient cycling as well as wind erosion resistance (Sun et al., 2016; Parwada, 2017).
The present study undertook a simulated experiment using a wind tunnel, analyzing the amount of soil loss and its characteristics under simulated wind erosion. The main objective of this study was to quantitatively explore the effects of Salix residual on wind erosion resistance. This type of study may provide theoretical explanations for the optimal reconstruction of sandy vegetation in the agro-pastoral ecotone of northern China.
A simulated experiment was carried out in the simulation hall of the State Key Laboratory of Soil Erosion and Dryland Farming on the Loess Plateau at the Institute of Soil and Water Conservation, CAS. The soil used in the experiment was a typical silt loam soil with 0.9% clay, 8.3% silt, and 80.8% sand, collected from the top 20 cm soil in the Salix land at Dingbian County, Shaanxi Province (37°50.188′N, 107°28.941′E), China (Figure 1). The key hypothesis of this study is that wind erosion resistance of residual can be achieved by adjusting biomass, the soil–residual thickness, and the ratio of branches and leaves. According to the field investigation of 36 Salix sites in study areas (Table 1), the simulated experimental treatments examined in the present study were four residual biomass treatments of 200, 400, 600, and 800 g m−2, three soil–residual thickness treatments of 0.5, 1.0, and 2.0 cm, and three ratios of branches and leaves of 2:1, 1:1, and 1:2, which derived from true conditions in the field as shown in Figure 2. In total, 22 experiment slots (10 treatments and 1 control, with 2 repetitions) were used in the present study. The experiment treatments were arranged on April 5–9, 2017. Then, the prepared experiment slots were sprayed with 3 L tap water three times at intervals of 10 days in order to eliminate the soil water effect. After that, the experiment slots were placed for 3 months in order to provide a similar environment to the field, and the simulated experiment was carried between August 2–15, 2017.
FIGURE 1. Distribution of residual samples of Salix residual in the southeastern margin of the Mu Us Sandy Land [the survey route includes A, B, and C lines].
FIGURE 2. Schematic diagram of the experiment wind tunnel structure and entity: (A) experiment slot; (B) sand collection; (C) status after simulated wind erosion.
The soil sample was passed through a 5-mm sieve after the removal of root fragments and was then air-dried. The wind tunnel came from the simulation hall of the State Key Laboratory of Soil Erosion and Dryland Farming on the Loess Plateau at the Institute of Soil and Water Conservation, CAS. The wind tunnel measured 24 × 1.2 × 1.0 m (length × width × height) and included a fan section, sections for wind regulation and rectification, an experimental section, and sections for sand collection and diversion (Figure 2). The regulation section could produce airflow with a stable field free of turbulence. The uniformity of airflow velocity was >99%, and the gradient of axial static pressure was <0.005. The wind speed could continuously vary from 0 to 15 m s−1 using a coordinated inverter in the fan section (Tuo et al., 2016). A vane anemometer, installed 0.2 m from the experimental section and 0.2 m above the tunnel floor, accurately (±0.2 m s−1) adjusted the wind speed. The diversion section evacuated the airflow to maintain a clean laboratory environment. Soil loss above the surface was calculated as the ratio of the horizontal sediment flux to the length of the soil surface upwind of the sampler. The wind speed of 11 m s−1 and blow period of 15 min were used, which could represent a typical wind event in the Mu Us Sandy Land, China (Tuo et al., 2018). Soil collected in the sand collecting section from the surface soil to the height of the 0–30 cm wind tunnel at intervals of 3 cm collected from the bottom total amount of wind erosion. The soil loss amount was calculated by the weight difference of the experiment slot before/after simulated wind erosion. The advantage of this method is that it simulates environmental factors such as wind speed and representative composition and properties; however, the disadvantage is that the experimental plot area is limited and the marginal effect is large. This problem can be solved by increasing the sample size in the future.
The mean of two repetitions for each treatment in the present study was used for data analysis. The effects of Salix residual biomass, the thickness of soil-incorporated residual, and the different ratios of Salix residual branches and leaves on wind erosion resistance were analyzed with Office 2017 and SigmaPlot 18.0.
Soil covered with plant residual could be especially valuable in the semi-arid regions (Zhang et al., 2017). Figure 3 shows the relationship between soil loss amount and Salix residual biomass. Compared with CK, the soil loss amount ranged from 1.56 to 40.8 kg m−2 as Salix residual biomass increased from 200 to 800 g m−2. Interestingly, there is a great difference in soil loss amount between treatments from 200 to 400 g m−2, while little difference was observed among the treatments when residual biomass increased from 400 to 800 g. These observations suggest that the natural residual biomass status (400 g m−2) may be notable for retarding soil wind erosion in study regions. Relative to the original surface soil, the soil loss amount was reduced at 37.4, 96.2, 96.6, and 97.5%, respectively, for treatments of 200, 400, 600, and 800 g m−2. These results were in agreement with those of the latest studies which found that the thick, intact litter layer is an important agent for soil protection (Liu et al., 2017). For example, He et al. (2017) studied the effect of plant residue removal at 0, 25, 50, 75, and 100% of initial height on soil wind erosion and found that crop residual removal can affect the susceptibility to soil wind erosion in Kansas. Besides, Figure 3 shows the soil loss amount was focused on 0–9 cm above the soil surface when the soil was incorporated with lower plant residual biomass (less than 400 g m−2). Therefore, as Salix residual biomass decreased from 800 to 0 g m−2, the soil loss amount ranged from 1.56 to 40.8 kg m−2, and the critical residual biomass value was 400 g m−2.
Plant residual is of great importance in retarding soil wind erosion in the agro-pastoral ecotone (Abdourhamane et al., 2019). Soil incorporated with plant residual is an important part of the ecological system, and its accumulation and redistribution could significantly affect soil surface soil–plant nutrient cycling and soil erosion resistance (Kamota et al., 2014; Guo et al., 2017). Figure 4 shows that the soil loss amount reduced rapidly with the increment of soil-incorporated residual thickness. Compared with CK, the soil loss amount was reduced at 25.3, 63.9, and 67.8%, respectively, for treatments of soil-incorporated residual at the height of 0.5, 1, and 2 cm. For different layers above the soil surface, the soil loss amount of vertical 0–9 cm accounts for 81.2–89.2% of the total soil loss amount. In addition, the soil loss amount at the layers of 12–30 cm above the surface was similar among the treatments in the present study. This result was in agreement with the fact that the application of plant residual to soil can stabilize soil against soil erosion (He et al., 2017; Pi et al., 2018). Thus, as the thickness of soil incorporated residual increased, the soil loss amount reduced rapidly, especially for 0–9 cm above the surface, accounting for 84.6% of the total.
Plant residual could influence soil erosion resistance as it can alter the chemical and/or biological composition of the soil, which may influence soil’s physical properties and/or plant growth and thus soil erosion resistance (He et al., 2017). For this reason, it is necessary to explore the contribution to retarding soil wind erosion between plant branches and leaves. Figure 5 indicates that with the increasing ratios of Salix branches and leaves, the soil loss amount showed an increasing trend. By contrast, Salix branches are the key point in resisting soil wind erosion as compared with leaves in the present study. Such results may be ascribed to the larger wind speed of 11 m s−1. Further study of the influence of near-surface wind speed on wind erosion (Li et al., 2014; Jiang et al., 2018) and the sustainability of the ecological benefits of sand plant residual needs to be conducted (Wu et al., 2019).
Based on field surveys, the influences of Salix residual biomass of 200, 400, 600, and 800 g m−2, soil incorporated with residual thickness of 0.5, 1.0, and 2.0 cm, and typical proportion of residual branches and leaves (2:1, 1:1, and 1:2) on wind erosion resistance were investigated in silt loam by a wind tunnel experiment. The results showed that, in the simulated experiment, the soil loss amount ranged from 1.56 to 40.8 kg m−2 as Salix residual biomass decreased from 800 to 0 g m−2, and a sharp decrease in the soil loss amount was found from treatments of 200 to 400 g m−2. In addition, the soil loss amount reduced rapidly as the thickness of soil-incorporated residual increased, and the soil loss amount of 0–9 cm above the surface soil accounts for 84.6% of the total. Compared with Salix leaves, the branches are more important in resisting soil wind erosion in the agro-pastoral ecotone.
The original contributions presented in this study are included in the article/Supplementary Material, and further inquiries can be directed to the corresponding author.
QL designed and wrote this article, FK and ZZ conducted the experiment, and CM and WN analyzed the data.
Financial assistance for this study was provided by the projects of the National Natural Science Foundation of China (Grant Nos. 41807521, 41867015, 41661101), the Open Program of the State Key Laboratory of Soil Erosion and Dryland Farming on the Loess Plateau, Institute of Soil and Water Conservation, CAS and MWR (A314021402-1801), and young technology stars in Shaanxi Province (2020KJXX-037).
The authors declare that the research was conducted in the absence of any commercial or financial relationships that could be construed as a potential conflict of interest.
All claims expressed in this article are solely those of the authors and do not necessarily represent those of their affiliated organizations, or those of the publisher, the editors and the reviewers. Any product that may be evaluated in this article, or claim that may be made by its manufacturer, is not guaranteed or endorsed by the publisher.
We also express our gratitude to the reviewers and editors for their constructive comments and suggestions.
Abdourhamane Touré, A., Tidjani, A. D., Rajot, J. L., Marticorena, B., Bergametti, G., Bouet, C., et al. (2019). Dynamics of Wind Erosion and Impact of Vegetation Cover and Land Use in the Sahel: A Case Study on sandy Dunes in southeastern Niger. Catena 177, 272–285. doi:10.1016/j.catena.2019.02.011
Guo, Z., Chang, C., Wang, R., and Li, J. (2017). Comparison of Different Methods to Determine Wind-Erodible Fraction of Soil with Rock Fragments under Different Tillage/management. Soil Tillage Res. 168, 42–49. doi:10.1016/j.still.2016.12.008
He, W. (2014). Vegetation Characteristics and its Response to Soil Erosion in Mu Us Desert. Hohhot, China: Inner Mongolia Agricultural University.
He, Y., Presley, D. A. R., Tatarko, J., and Blanco-Canqui, H. (2017). Crop Residue Harvest Impacts Wind Erodibility and Simulated Soil Loss in the central Great plains. GCB Bioenergy 10, 3–10. doi:10.1111/gcbb.12483
Jiang, Y., Gao, Y., Dong, Z., Liu, B., and Zhao, L. (2018). Simulations of Wind Erosion along the Qinghai-Tibet Railway in north-central Tibet. Aeolian Res. 32, 192–201. doi:10.1016/j.aeolia.2018.03.006
Kamota, A., Muchaonyerwa, P., and Mnkeni, P. N. S. (2014). Decomposition of Surface-Applied and Soil-Incorporated Bt Maize Leaf Litter and Cry1Ab Protein during Winter Fallow in South Africa. Pedosphere 24, 251–257. doi:10.1016/s1002-0160(14)60011-4
Li, J., Jia, H., Han, X., Zhang, J., Sun, P., and Lu, M. (2016). Selection of Reliable Reference Genes for Gene Expression Analysis under Abiotic Stresses in the Desert Biomass Willow, salix Psammophila. Front. Plant Sci. 7, 11–13. doi:10.3389/fpls.2016.01505
Li, Q., Liu, G.-B., Zhang, Z., Tuo, D.-F., Bai, R.-r., and Qiao, F.-f. (2017). Relative Contribution of Root Physical Enlacing and Biochemistrical Exudates to Soil Erosion Resistance in the Loess Soil. Catena 153, 61–65. doi:10.1016/j.catena.2017.01.037
Li, Q., Liu, G., Zhang, Z., Tuo, D., and Miao, X. (2016). Structural Stability and Erodibility of Soil in an Age Sequence of Artificial Robinia Pseudoacacia on a Hilly Loess Plateau. Pol. J. Environ. Stud. 25, 1595–1601. doi:10.15244/pjoes/62390
Li, Q., Liu, N., Zhang, Z., Ma, C. Y., Yu, W. J., and Nan, W. G. (2020). Features of the Distribution of Salix Residual in the South Edge of Mu Us Sandy Land of China. Drought meteorology 38 (2), 313–318.
Li, X., Niu, J., and Xie, B. (2014). The Effect of Leaf Residual Cover on Surface Runoff and Soil Erosion in Northern China. Plos One 9, 77–89. doi:10.1371/journal.pone.0107789
Liu, W., Luo, Q., Lu, H., Wu, J., and Duan, W. (2017). The Effect of Litter Layer on Controlling Surface Runoff and Erosion in Rubber Plantations on Tropical Mountain Slopes, SW China. Catena 149, 167–175. doi:10.1016/j.catena.2016.09.013
Pi, H., Sharratt, B., Schillinger, W. F., Bary, A. I., and Cogger, C. G. (2018). Wind Erosion Potential of a winter Wheat-Summer Fallow Rotation after Land Application of Biosolids. Aeolian Res. 32, 53–59. doi:10.1016/j.aeolia.2018.01.009
Sun, L., Zhang, G.-h., Liu, F., and Luan, L.-l. (2016). Effects of Incorporated Plant Litter on Soil Resistance to Flowing Water Erosion in the Loess Plateau of China. Biosyst. Eng. 147, 238–247. doi:10.1016/j.biosystemseng.2016.04.017
Sun, L., Zhang, G.-h., Luan, L.-l., and Liu, F. (2016). Temporal Variation in Soil Resistance to Flowing Water Erosion for Soil Incorporated with Plant Litters in the Loess Plateau of China. Catena 145, 239–245. doi:10.1016/j.catena.2016.06.016
Tuo, D., Xu, M., and Gao, G. (2018). Relative Contributions of Wind and Water Erosion to Total Soil Loss and its Effect on Soil Properties in Sloping Croplands of the Chinese Loess Plateau. Sci. Total Environ. 633, 1032–1040. doi:10.1016/j.scitotenv.2018.03.237
Tuo, D., Xu, M., Gao, L., Zhang, S., and Liu, S. (2016). Changed Surface Roughness by Wind Erosion Accelerates Water Erosion. J. Soils Sediments 16, 105–114. doi:10.1007/s11368-015-1171-x
Wang, Y., Dong, Y., Su, Z., Mudd, S. M., Zheng, Q., Hu, G., et al. (2020). Spatial Distribution of Water and Wind Erosion and Their Influence on the Soil Quality at the Agropastoral Ecotone of North China. Int. Soil Water Conservation Res. 8, 253–265. doi:10.1016/j.iswcr.2020.05.001
Wu, Z., Wang, M., Zhang, H., and Du, Z. (2019). Vegetation and Soil Wind Erosion Dynamics of Sandstorm Control Programs in the Agro-Pastoral Transitional Zone of Northern china. Front. Earth Sci. 13 (2), 430–443. doi:10.1007/s11707-018-0715-y
Yu, X., Huang, Y., Li, E., Li, X., and Guo, W. (2017). Effects of Vegetation Types on Soil Water Dynamics during Vegetation Restoration in the Mu Us sandy Land, Northwestern China. J. Arid Land 9, 188–199. doi:10.1007/s40333-017-0054-y
Zhang, G., Azorin-Molina, C., Shi, P., Lin, D., Guijarro, J. A., Kong, F., et al. (2019). Impact of Near-Surface Wind Speed Variability on Wind Erosion in the Eastern Agro-Pastoral Transitional Zone of Northern China, 1982-2016. Agric. For. Meteorology 271, 102–115. doi:10.1016/j.agrformet.2019.02.039
Zhang, J.-Q., Zhang, C.-L., Chang, C.-P., Wang, R.-D., and Liu, G. (2017). Comparison of Wind Erosion Based on Measurements and Sweep Simulation: a Case Study in Kangbao County, Hebei Province, china. Soil Tillage Res. 165, 169–180. doi:10.1016/j.still.2016.08.006
Zhang, J., Yang, M., Deng, X., Liu, Z., Zhang, F., and Zhou, W. (2018). Beryllium-7 Measurements of Wind Erosion on Sloping fields in the Wind-Water Erosion Crisscross Region on the Chinese Loess Plateau. Sci. Total Environ. 615, 240–252. doi:10.1016/j.scitotenv.2017.09.238
Zhang, Y., and Zhao, W. (2015). Vegetation and Soil Property Response of Short-Time Fencing in Temperate Desert of the Hexi Corridor, Northwestern China. Catena 133, 43–51. doi:10.1016/j.catena.2015.04.019
Keywords: wind tunnel, soil loss amount, simulated wind erosion, Salix litter, Mu Us sandy land
Citation: Li Q, Kang F, Zhang Z, Ma C and Nan W (2021) Simulated Experiment on Wind Erosion Resistance of Salix Residual in the Agro-Pastoral Ecotone. Front. Environ. Sci. 9:574883. doi: 10.3389/fenvs.2021.574883
Received: 30 June 2020; Accepted: 28 June 2021;
Published: 05 August 2021.
Edited by:
Xian Xue, Northwest Institute of Eco-Environment and Resources (CAS), ChinaReviewed by:
Na Li, Northeast Institute of Geography and Agroecology (CAS), ChinaCopyright © 2021 Li, Kang, Zhang, Ma and Nan. This is an open-access article distributed under the terms of the Creative Commons Attribution License (CC BY). The use, distribution or reproduction in other forums is permitted, provided the original author(s) and the copyright owner(s) are credited and that the original publication in this journal is cited, in accordance with accepted academic practice. No use, distribution or reproduction is permitted which does not comply with these terms.
*Correspondence: Qiang Li, bXIubGlfcWlhbmdAMTYzLmNvbQ==
Disclaimer: All claims expressed in this article are solely those of the authors and do not necessarily represent those of their affiliated organizations, or those of the publisher, the editors and the reviewers. Any product that may be evaluated in this article or claim that may be made by its manufacturer is not guaranteed or endorsed by the publisher.
Research integrity at Frontiers
Learn more about the work of our research integrity team to safeguard the quality of each article we publish.