- 1Sanya Academy of Forestry, Sanya, China
- 2College of Forestry/Wuzhishan National Long Term Forest Ecosystem Monitoring Research Station, Hainan University, Haikou, China
- 3Guangzhou Shimen National Forest Park Management Office, Guangzhou, China
Reforestation is an effective way to alleviate deforestation and its negative impacts on ecosystem services. It is widely recognized that the most key step for reforestation is using suitable native species, but selecting suitable native tree species is much more complex and challenging than the selection of non-native tree species that have been widely used for reforestation. Here, we quantify whether the native tree species (Bombax ceiba) can be suitable for performing reforestation to restore a 0.2 km2 highly degraded tropical monsoon forest in Baopoling Mountain (BPL), Sanya, China, due to 20 years of limestone mining for cement production. We found that stomatal closure helped Bombax ceiba develop higher drought stress tolerance than the most dominant native tree species (Bridelia tomentosa) in an undisturbed tropical rainforest in BPL, thereby better adapting well to drought stress in the dry season. These characteristics in turn facilitated it to have high survival rate (92% ± 4%) and fast growth rate, after three years of monoculture in BPL. Thus, Bombax ceiba is very suitable for performing reforestation to recover highly degraded tropical forests in Hainan Island, China.
Introduction
Historic human disturbance (e.g., ore mining and agricultural use) has resulted in very high rates of deforestation and degradation worldwide, which has become a major threat to global biodiversity and ecosystem services (e.g., freshwater supply and slowing down of global warming) (Sahin and Hall, 1996; Foley et al., 2011; Lambin and Meyfroidt, 2011; Vörösmarty et al., 2015). Reforestation is assumed to be able to partly alleviate deforestation-induced consequences (Griscom et al., 2017; Taubert et al., 2018). Thus, a number of reforestation projects have been performed worldwide to alleviate these native influences (Postel and Thompson, 2005; Calder and Aylward, 2006; Grime, 2006; Calder, 2007; Jian et al., 2015). However, to date, monoculture plantations of many non-native fast-growing and commercial tree species are the main strategy for reforestation in many areas across the world (Lu et al., 2017).
Although these monoculture plantations of non-native species can quickly facilitate reforestation, they can also increase risks including high cost for maintaining soil water and its nutrients and pest and pathogen outbreaks, besides having few benefits for ecosystem services (Lamb et al., 2005; Wingfield et al., 2015). Thus, many studies emphasize the most key step for reforestation is using suitable native species but not non-native species (Miyawaki, 2004; Hall et al., 2011). However, selecting suitable native tree species is much more complex and challenging than the selection of non-native tree species, in particular (Meli et al., 2014; Stanturf et al., 2014). That is because the reforestation project usually has to be finished in a very short time and with limited economic resources. Moreover, in theory, mono-planting fast-growing tree species with high survival rates may be very effective for preventing high risks of landslide due to frequent typhoon and heavy rain in humid tropics (Stokes et al., 2009; Walker et al., 2009; Pang et al., 2018). Thus, non-native tree species which have high survival and growth rates and germination traits enabling easy propagation in the nurseries are the first choice for performing reforestation (Meli et al., 2014). Although pioneer native tree species in the early successional tropical rainforest are assumed fast-growing (Lohbeck et al., 2013; Zhu et al., 2013), their survival and growth rates and germination traits are very less investigated and thus cannot promise they are better than the non-native tree species for quickly finishing the reforestation project. Tree species in late successional or natural tropical rainforests may better adapt well to a specific environment of the highly degraded ecosystem than the non-native tree species, but they are treated as slow-growing, but with high resources competitive ability (Mason et al., 2012; Mason et al., 2013). As a result, they are also not very suitable for performing reforestation in humid tropics.
The currently developed trait-based method can open a good avenue for facilitating selection of suitable native tree species (McGill et al., 2006; Laughlin, 2014). It assumes that plant functional traits can directly reflect plants adapting well to different abiotic and biotic environments (Larjavaara and Muller-Landau, 2010; Laughlin and Laughlin, 2013; Cadotte, 2017). Thus, using the functional traits to find out native species that can not only have high survival and growth rates and germination traits enabling quick and easy propagation in the nurseries but also adapt well to the specific environment of the highly degraded ecosystem can be useful for reforestation. This goal may be easily achieved by comparing the functional traits which are highly correlated with survival and growth rates, germination, and the ability of adapting well to the environment of the highly degraded ecosystem between potential native tree species and dominant native tree species in the late successional or undisturbed old tropical rainforest.
Bombax ceiba is a native species, which is widely distributed in tropics, and it is also a high-demand plant species used for sculpture, so its seedling has been widely cultivated to get economic profits (Griffiths et al., 2003). In addition, it can adapt well to a very severe environment (e.g., drought stress), and thus, it may have high potential to be utilized for reforestation in the degraded tropical forest which has seasonal drought stress. Here, we mono-planted Bombax ceiba to restore a 0.2 km2 highly degraded tropical rainforest in Baopoling Mountain (BPL) in Sanya, Hainan Province, China, to quantify whether Bombax ceiba can be a good native tree species for reforestation in Hainan Island. It has been found that BPL can have frequent typhoon and heavy rain and very limited precipitation in the dry season (Hong et al., 2020), which may result in a high risk of landslide and drought stress during reforestation. Based on this, we use the following standards to judge whether Bombax ceiba can be a good native tree species for reforestation in extremely degraded tropical rainforests. First, Bombax ceiba can have high growth and survival rates, thereby being suitable for quickly performing reforestation. Second, landslides can be prevented after mono-planting Bombax ceiba to perform reforestation. Third, Bombax ceiba can adapt better to the seasonal drought in BPL than the most dominant native species in the undisturbed old tropical rainforest. Specifically, we survey survival and growth rates during reforestation. We also compare differences in functional traits that are highly associated with growth (i.e., photosynthesis rate) and drought stress tolerance (e.g., leaf turgor loss point) between Bombax ceiba and the most dominant native tree species in the adjacent undisturbed old tropical rainforest in BPL.
Materials and Methods
Study Sites
Our study site is located in Baopoling Mountain (BPL) which is a limestone mountain in Sanya, Hainan Province, China (109°51′01″E, 18°31′99″N) (Figure 1). It has a tropical monsoon oceanic climate with the mean annual temperature of 28°C. The average annual precipitation in Sanya is 1500 mm, with approximately 91% of the precipitation occurring between June and October; these estimates are based on the climate data form 1959 to 2015 (Luo et al., 2018). The typical vegetation is the species-rich tropical rainforest (Luo et al., 2020), but Bombax ceiba is not the native tree species in this forest but the native tree species that is widely distributed in the whole Hainan Island, China. Due to 20 years of limestone mining for producing cement, one part (0.2 km2 area) of BPL has become a highly degraded bare rocky mountain, where plants cannot be grown. Areas of BPL outside of this degraded area have not received any significant disturbance and remain as an undisturbed old tropical monsoon forest which has more than 120 tree species (Figure 1). In 2016, we have mono-planted the seedling of Bombax ceiba to perform a reforestation project in this 0.2 km2 area. The planting density was kept at 80–100 stems per hectare; we recorded their survival rates from 2016 to 2019 as
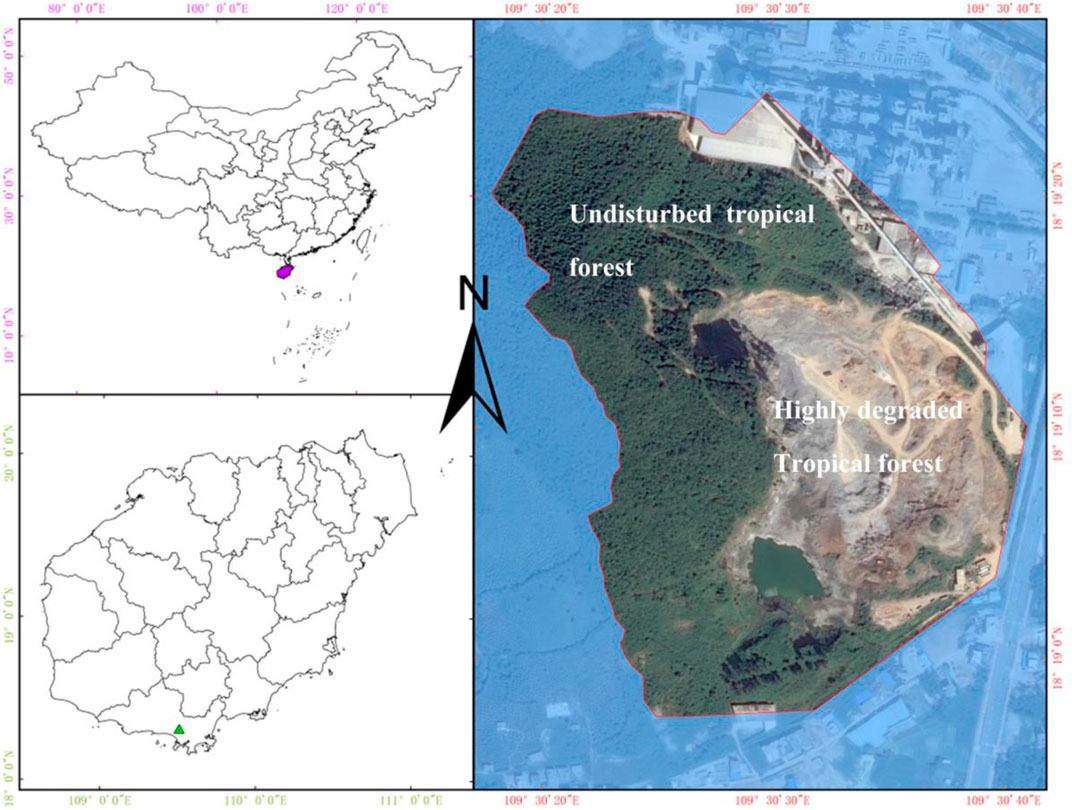
FIGURE 1. Location and landscape of our study sites (Baopoling Mountain, Sanya, China) before reforestation.
Trait Collection and Measurement
Since there is a remarkable dry season in BPL, plant species must develop high drought stress tolerance, thereby being able to survive well in BPL. Thus, in the peak of dry season (February) in 2019, we measured functional traits that are highly related to fast growth and drought stress tolerance (i.e., upper epidermis thickness (μm), palisade tissue thickness (μm), spongy tissue thickness (μm), lower epidermis thickness (μm), stomatal density (numbers mm−2), transpiration rate (mol m−2 s−1), maximum photosynthesis rate (mol m−2 s−1), stomatal conductance (mmol m−2 s−1), and leaf turgor loss point (MPa)) for Bombax ceiba and the most dominant (relative abundance >10%) plant species (Bridelia tomentosa) located in the nearby undisturbed forests. For measuring these traits, we first measured the diameter at breast height (DBH) of all individuals for both Bombax ceiba and Bridelia tomentosa to get the mean DBH for Bombax ceiba and Bridelia tomentosa, respectively (see Table 1). Then, 20 fully expanded, healthy, and sun-exposed leaves were collected from five mature individuals of Bombax ceiba and Bridelia tomentosa whose DBH values were comparable to the mean DBH values of Bombax ceiba and Bridelia tomentosa (Table 1).
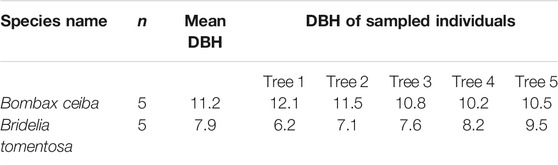
Table 1. Mean DBH (in cm) of Bombax ceiba and Bridelia tomentosa in Baopoling Mountain and DBH values (in cm) of the sampled individuals (n) for Bombax ceiba and Bridelia tomentosa, respectively.
Measurement of Leaf Anatomical Traits
Twenty mature and sun-exposed leaves were collected from five independent individuals of Bombax ceiba and Bridelia tomentosa in BPL. All leaf samples were progressively dehydrated in an ethanol series (50, 70, 85, 95, and 100%) and infiltrated with warm paraffin. Then, the thickness of the leaf, upper epidermis, lower epidermis, spongy tissue, and palisade was measured with a Leica DM2500 light microscope (Leica Microsystems GmbH, Wetzlar, Germany). The density of stomata was measured using a razor to slice the abaxial epidermis. All the sections were mounted on slides and observed under a Leica DM2500 microscope.
Measurement of Maximum Photosynthesis Rate, Transpiration Rate, and Stomatal Conductance
Measurements of the maximum photosynthesis rate, transpiration rate, and stomatal conductance were conducted between 9:00 and 11:00 on sunny days with an Li-6400 portable photosynthesis system (Li-Cor, Lincoln, Nebraska, United States). Based on preliminary trials, the photosynthetic photon flux density was set at 1500 μmol m−2 s−1 to ensure that light-saturated photosynthetic rates were measured for Bombax ceiba and Bridelia tomentosa. Ambient CO2 and air temperature were maintained at 390 μmol mol−1 and 28°C, respectively (Zhang et al., 2018a). Before data were recorded, leaves were exposed to the above conditions for about 5 min to allow photosynthetic parameters to stabilize.
Measurement of Leaf Turgor Loss Point
Leaf-bearing branches from three to five individuals of each species were harvested and transported to the laboratory where the basal ends of the branches were immersed in distilled water and re-cut. The branch samples were rehydrated until the leaf water potential was greater than −0.05 MPa. Leaves were first weighed to obtain the initial fresh mass and then immediately placed in a pressure chamber to determine the initial water potential. The leaf mass and water potential were measured periodically during slow desiccation in the laboratory. Finally, leaves were oven-dried for 72 h at 70°C to determine their dry mass. The leaf turgor loss point was determined using a pressure–volume relationship analysis program developed by Schulte and Hinckley (1985).
Statistical Methods
First, we log-transformed all measured nine functional traits (upper and lower epidermis thickness, palisade and spongy tissue thickness, stomatal conductance, stomatal density, maximum photosynthesis rate, transpiration rate, and leaf turgor loss point) for Bombax ceiba and Bridelia tomentosa in BPL. Then, we used the paired t-test to quantify whether Bombax ceiba could have higher growth rates and drought stress tolerance than Bridelia tomentosa. Then, a principal component analysis (PCA) was employed to evaluate which of the 10 traits were best at discriminating between Bombax ceiba and Bridelia tomentosa.
Results
Based on the precipitation records per month from 2016 to 2019, the mean precipitation in BPL in the dry season (February) was significantly much lower (only 1/10th) than that in the wet season (July) (Figure 2). However, after 2 years of plantation in BPL, the survival rate for all seedlings of Bombax ceiba was very high (92% ± 4%), and till now, we did not observe any destructive landslides (Figure 1). Bombax ceiba had a much higher growth rate than Bridelia tomentosa. That was because all seven traits (upper and lower epidermis thickness, palisade and spongy tissue thickness, stomatal conductance, maximum photosynthesis rate, and transpiration rate) that are highly associated with the growth of Bombax ceiba were all significantly much higher (3–5 times) than those of Bridelia tomentosa (Figure 3). In addition, Bombax ceiba had much higher drought stress tolerance than Bridelia tomentosa, as the leaf turgor loss point for Bombax ceiba was significantly much lower (merely 1/3rd) than that of Bridelia tomentosa (Figure 3). The results from the PCA indicated that only stomatal conductance was best at discriminating between Bombax ceiba and Bridelia tomentosa (Figure 4 and Table 2).
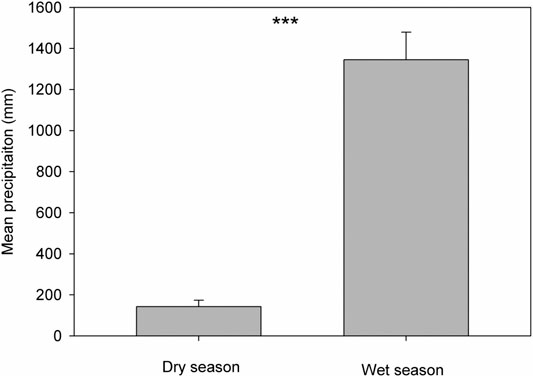
FIGURE 2. Variations in mean precipitation in the dry season (February) and wet season (July) from 2016 to 2019. *** indicates p < 0.001, based on paired t-tests.
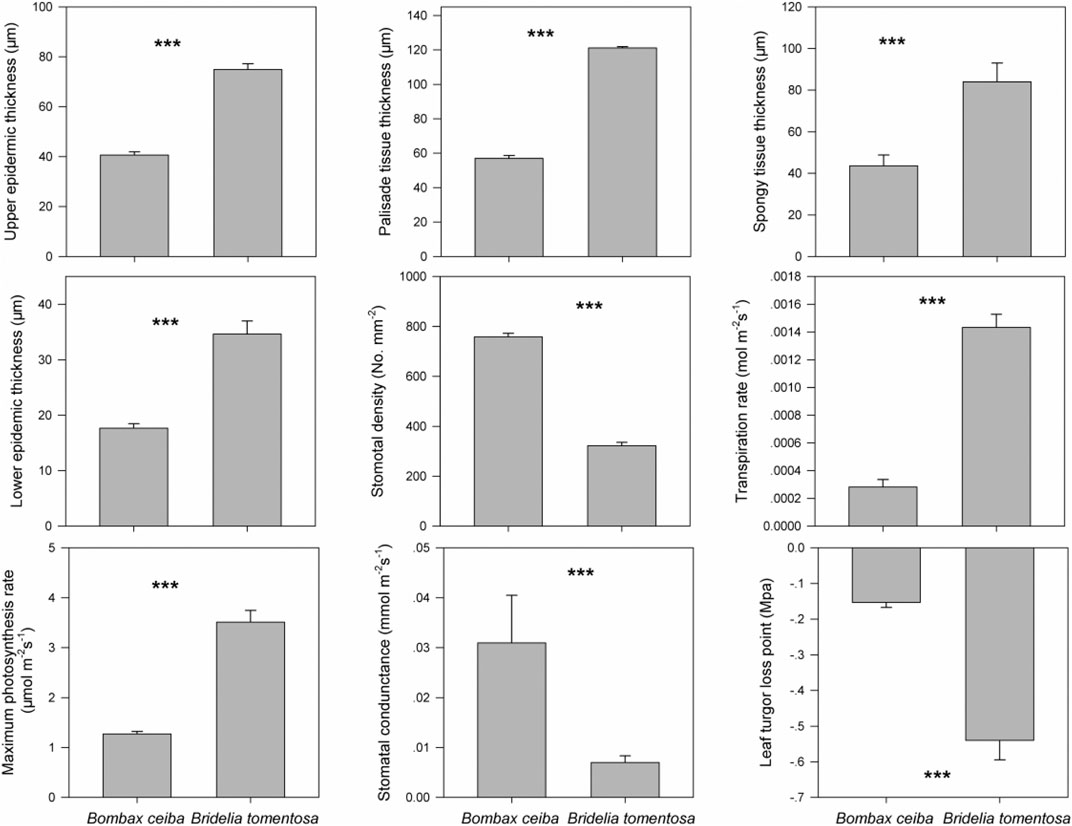
FIGURE 3. Differences in the nine functional traits (upper epidermis thickness (μm), palisade tissue thickness (μm), spongy tissue thickness (μm), lower epidermis thickness (μm), stomatal density (numbers mm−2), transpiration rate (mol m−2 s−1), maximum photosynthesis rate (mol m−2 s−1), stomatal conductance (mmol m−2 s−1), and leaf turgor loss point (MPa)) between Bombax ceiba and Bridelia tomentosa. *** indicates p < 0.001, based on paired t-tests.
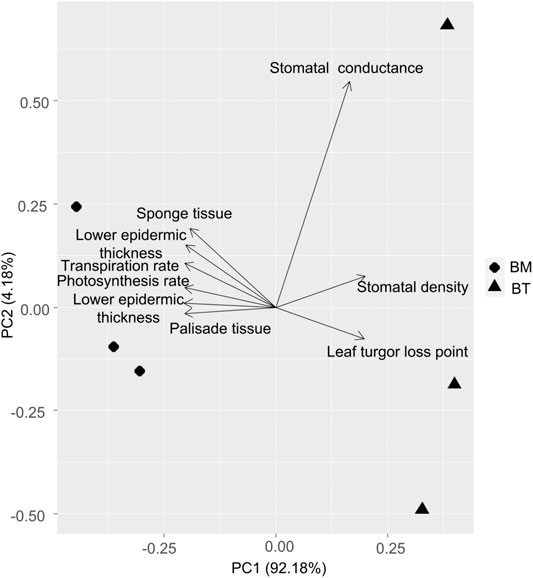
FIGURE 4. Principal component analysis (PCA) of the nine functional traits between Bombax ceiba (BM) and Bridelia tomentosa (BT). Diamonds and triangles represent BM and BT respectively.
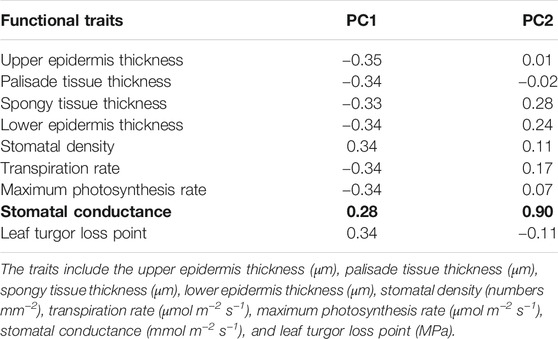
Table 2. The first two axes of a principal component analysis (PCA) for Bombax ceiba and Bridelia tomentosa, based on nine plant traits. Bold indicates significant.
Discussion
Our results clearly revealed that when planting Bombax ceiba in Baopoling Mountain (BPL) for performing reforestation, we found that Bombax ceiba had a very high survival rate (92% ± 4%) and that mono-planting Bombax ceiba can indeed prevent landslides. Moreover, Bombax ceiba had a higher growth rate and drought stress tolerance than the most dominant native tree species (Bridelia tomentosa) in the nearby undisturbed tropical rainforest when facing the limited water supply in the dry season. Thus, Bombax ceiba can be suitable for performing reforestation to restore the highly degraded tropical forests in BPL.
We found that the photosynthetic rate for Bombax ceiba was much higher (3 times) than that of Bridelia tomentosa. The photosynthetic rate impacts the energy balance of a plant, where, typically, a high photosynthetic rate is linked to a fast-growing rate (Kirschbaum, 2011; Zhang et al., 2018b). This indicated that Bombax ceiba could have a higher growth rate than Bridelia tomentosa, even though it had to face drought stress in the dry season. Higher photosynthesis rates will also result in higher leaf anatomy traits (He et al., 2017; Hua et al., 2017), stomatal traits (McAusland et al., 2016; Lawson and Vialet-Chabrand, 2019), and leaf transpiration rates (Santos et al., 2018). That was why we observed these traits for Bombax ceiba are all much higher (from 3 to 5 times) than those of Bridelia tomentosa. These results indicated that Bombax ceiba had developed appropriate functional traits to have very high growth rates even when facing the limited water supply.
We found that the mean precipitation in the dry season was merely 1/10th of that in the wet season, indicating high drought stress in the dry season. We also found Bombax ceiba in BPL had a much lower leaf turgor loss point than the native dominant plant species (Bridelia tomentosa) in the dry season. A lower leaf turgor loss point is usually observed when leaf cannot get enough water supply (Bartlett et al., 2012). Moreover, plants with a lower leaf turgor loss point can maintain higher stomatal conductance, photosynthesis rates, and higher transpiration rates, when facing very limited water supply (Sack et al., 2003; Mitchell et al., 2008; Blackman et al., 2010). Thus, these results indicated that Bombax ceiba had developed higher drought stress tolerance than Bridelia tomentosa and thereby can maintain higher growth rates than Bridelia tomentosa even when facing the limited water supply. This also demonstrated that Bridelia tomentosa can even adapt better to the seasonal drought than the most dominant native species (Bridelia tomentosa) in the undisturbed tropical rainforest in the dry season.
Our PCA results revealed that Bombax ceiba and Bridelia tomentosa were significantly distinguished merely by stomatal conductance. Bombax ceiba had much lower stomatal conductance, compared to Bridelia tomentosa. Lower stomatal conductance is highly correlated with stomatal closure, which results in a lower leaf turgor point to tolerate drought stress (Brodribb and Holbrook, 2003; Farrell et al., 2017; Trueba et al., 2019). As a result, a higher intensity of stomatal closure in the dry season should be the key to help Bombax ceiba to possess higher drought stress tolerance and growth rates than the most dominant native tree species (Bridelia tomentosa) in the undisturbed tropical rainforest (Bartlett et al., 2012).
Conclusion
Taken all results together, when planting Bombax ceiba to restore the highly degraded tropical forest in BPL, Bombax ceiba tends to trigger higher stomatal closure to have higher drought stress tolerance than the most dominant native tree species in the undisturbed tropical rainforest, thereby better adapting well to the drought stress in the dry season and having higher growth and survival rates. Moreover, consistent with previous observations (Stokes et al., 2009; Walker et al., 2009; Pang et al., 2018), mono-planting fast-growing tree species (in our study, Bombax ceiba) with high survival rate can indeed help prevent landslides due to frequent typhoon and heavy rain. As a result, Bombax ceiba can indeed be a good candidate native species for performing reforestation to restore the degraded tropical rainforest in the whole Hainan Island, China.
Data Availability Statement
The raw data supporting the conclusions of this article will be made available by the authors, without undue reservation.
Author Contributions
JL, WH, JY, KJ, and HZ designed the research. JL, WH, KJ, ZT, QH, and JC performed the research. ZT, QH, HZ, and JC analyzed the data. All authors have contributed to the writing of this manuscript.
Funding
This work was funded by the scientific research project of ecological restoration of Baopoling Mountain in Sanya, China, and a start-up fund from Hainan University (KYQD (ZR) 1876).
Conflict of Interest
The authors declare that the research was conducted in the absence of any commercial or financial relationships that could be construed as a potential conflict of interest.
References
Bartlett, M. K., Scoffoni, C., and Sack, L. (2012). The determinants of leaf turgor loss point and prediction of drought tolerance of species and biomes: a global meta-analysis. Ecol. Lett. 15 (5), 393–405. doi:10.1111/j.1461-0248.2012.01751.x
Blackman, C. J., Brodribb, T. J., and Jordan, G. J. (2010). Leaf hydraulic vulnerability is related to conduit dimensions and drought resistance across a diverse range of woody angiosperms. New Phytol. 188 (4), 1113–1123. doi:10.2307/40960863
Brodribb, T. J., and Holbrook, N. M. (2003). Stomatal closure during leaf dehydration, correlation with other leaf physiological traits. Plant Physiol. 132 (4), 2166–2173. doi:10.1104/pp.103.023879
Cadotte, M. W. (2017). Functional traits explain ecosystem function through opposing mechanisms. Ecol. Lett. 20 (8), 989–996. doi:10.1111/ele.12796
Calder, I. R., and Aylward, B. (2006). Forest and floods. Water Int. 31 (1), 87–99. doi:10.1080/02508060608691918
Calder, I. R. (2007). Forests and water - ensuring forest benefits outweigh water costs. For. Ecol. Manag. 251 (1), 110–120. doi:10.1016/j.foreco.2007.06.015
Farrell, C., Szota, C., and Arndt, S. K. (2017). Does the turgor loss point characterize drought response in dryland plants? Plant Cell Environ. 40 (8), 1500–1511. doi:10.1111/pce.12948
Foley, J. A., Ramankutty, N., Brauman, K. A., Cassidy, E. S., Gerber, J. S., Johnston, M., et al. (2011). Solutions for a cultivated planet. Nature 478 (7369), 337–342. doi:10.1038/nature10452
Griffiths, A. D., Philips, A., and Godjuwa, C. (2003). Harvest of Bombax ceiba for the aboriginal arts industry, central arnhem land, Australia. Biol. Conserv. 113 (2), 295–305. doi:10.1016/S0006-3207(02)00419-6
Grime, J. P. (2006). Trait convergence and trait divergence in herbaceous plant communities: mechanisms and consequences. J. Veg. Sci. 17 (2), 255–260. doi:10.1111/j.1654-1103.2006.tb02444.x
Griscom, B. W., Adams, J., Ellis, P. W., Houghton, R. A., Lomax, G., Miteva, D. A., et al. (2017). Natural climate solutions. P. Natl. Acad. Sci. USA. 114 (44), 11645–11650. doi:10.1073/pnas.1710465114
Hall, J. S., Ashton, M. S., Garen, E. J., and Jose, S. (2011). The ecology and ecosystem services of native trees: implications for reforestation and land restoration in Mesoamerica. For. Ecol. Manag. 261 (10), 1553–1557. doi:10.1016/j.foreco.2010.12.011
He, N., Liu, C., Miao, T., Li, M., Hao, Y., Yu, G., et al. (2017). Variation in leaf anatomical traits from tropical to cold-temperate forests and linkage to ecosystem functions. Funct. Ecol. 32 (8), 1175–1181. doi:10.1111/1365-2435.12934
Hong, W. J., Yang, J. D., Luo, J. H., Jiang, K., Xu, J. Z., and Zhang, H. (2020). Reforestation based on mono-plantation of fast-growing tree species make it difficult to maintain high soil water content in tropics, a case study in Hainan island, China. Water. 12 (11), 3077. doi:10.3390/w12113077
Hua, L., Chen, Y., Zhang, H., Fu, P., and Fan, Z. (2017). Stronger cooling effects of transpiration and morphology of the plants from a hot dry habitat than from a hot wet habitat. Funct. Ecol. 31 (12), 2202–2211. doi:10.1111/1365-2435.12923
Jian, S., Zhao, C., Fang, S., and Yu, K. (2015). Effects of different vegetation restoration on soil water storage and water balance in the Chinese Loess Plateau. Agr. For. Meteorol. 206, 85–96. doi:10.1016/j.agrformet.2015.03.009
Kirschbaum, M. U. (2011). Does enhanced photosynthesis enhance growth? Lessons learned from CO2 enrichment studies. Plant Physiol. 155 (1), 117–124. doi:10.1104/pp.110.166819
Lamb, D., Erskine, P. D., and Parrotta, J. A. (2005). Restoration of degraded tropical forest landscapes. Science 310 (5754), 1628–1632. doi:10.1126/science.1111773
Lambin, E. F., and Meyfroidt, P. (2011). Global land use change, economic globalization, and the looming land scarcity. P. Natl. Acad. Sci. USA. 108 (9), 3465–3472. doi:10.1073/pnas.1100480108
Larjavaara, M., and Muller-Landau, H. C. (2010). Rethinking the value of high wood density. Funct. Ecol. 24 (4), 701–705. doi:10.1111/j.1365-2435.2010.01698.x
Laughlin, D. C. (2014). Applying trait-based models to achieve functional targets for theory-driven ecological restoration. Ecol. Lett. 17 (7), 771–784. doi:10.1111/ele.12288
Laughlin, D. C., and Laughlin, D. E. (2013). Advances in modeling trait-based plant community assembly. Trends Plant Sci. 18 (10), 584–593. doi:10.1016/j.tplants.2013.04.012
Lawson, T., and Vialet-Chabrand, S. (2019). Speedy stomata, photosynthesis and plant water use efficiency. New Phytol. 221 (1), 93–98. doi:10.1111/nph.15330
Lohbeck, M., Poorter, L., Lebrija-Trejos, E., Martínez-Ramos, M., Meave, J. A., Paz, H., et al. (2013). Successional changes in functional composition contrast for dry and wet tropical forest. Ecology 94 (6), 1211–1216. doi:10.1890/12-1850.1
Lu, Y., Ranjitkar, S., Harrison, R. D., Xu, J., Ou, X., Ma, X., et al. (2017). Selection of native tree species for subtropical forest restoration in southwest China. PLoS ONE 12 (1), e0170418. doi:10.1371/journal.pone.0170418
Luo, H. X., Dai, S. P., Li, M. F., and Xi, Z. H. (2018). The climate characteristics of Hainan Island from 1959-2015. Jiangsu Agr. Sci. 46 (15), 261–268. doi:10.15889/j.issn.1002-1302.2018.15.066
Luo, J. H., Cui, J., Pandey, S. P., Jiang, K., Tan, Z. Y., He, Q. F., et al. (2020). Seasonally distinctive growth and drought stress functional traits enable Leucaena Leucocephala to successfully invade a Chinese tropical forest. Trop. Conserv. Sci. 13, 1–7. doi:10.1177/1940082920949176
Mason, N. W., Bello, F., Mouillot, D., Pavoine, S., and Dray, S. (2013). A guide for using functional diversity indices to reveal changes in assembly processes along ecological gradients. J. Veg. Sci. 24, 794–806. doi:10.1111/jvs.12013
Mason, N. W., Richardson, S. J., Peltzer, D. A., de Bello, F., Wardle, D. A., and Allen, R. B. (2012). Changes in coexistence mechanisms along a long-term soil chronosequence revealed by functional trait diversity. J. Ecol. 100, 678–689. doi:10.1111/j.1365-2745.2012.01965.x
McAusland, L., Vialet-Chabrand, S., Davey, P., Baker, N. R., Brendel, O., and Lawson, T. (2016). Effects of kinetics of light-induced stomatal responses on photosynthesis and water-use efficiency. New Phytol. 211 (4), 1209–1220. doi:10.1111/nph.14000
McGill, B. J., Enquist, B. J., Weiher, E., and Westoby, M. (2006). Rebuilding community ecology from functional traits. Trends Ecol. Evol. 21 (4), 178–185. doi:10.1016/j.tree.2006.02.002
Meli, P., Martínez-Ramos, M., Rey-Benayas, J. M., Carabias, J., and Ewald, J. (2014). Combining ecological, social and technical criteria to select species for forest restoration. Appl. Veg. Sci. 17 (4), 744–753. doi:10.1111/avsc.12096
Mitchell, P. J., Veneklaas, E. J., Lambers, H., and Burgess, S. S. (2008). Leaf water relations during summer water deficit: differential responses in turgor maintenance and variation in leaf structure among different plant communities in south-western Australia. Plant Cell Environ. 31 (12), 1791–1802. doi:10.1111/j.1365-3040.2008.01882.x
Miyawaki, A. (2004). Restoration of living environment based on vegetation ecology: theory and practice. Ecol. Res. 19 (1), 83–90. doi:10.1111/j.1440-1703.2003.00606.x
Pang, C. C., Ma, X. K. -K., Lo, J. P. -L., Hung, T. T. -H., and Hau, B. C. -H. (2018). Vegetation succession on landslides in Hong Kong: plant regeneration, survivorship and constraints to restoration. Glob. Ecol. Conserv. 15 (2), e00428. doi:10.1016/j.gecco.2018.e00428
Postel, S. L., and Thompson, B. H. (2005). Watershed protection: capturing the benefits of nature's water supply services. Nat. Resour. Forum. 29 (2), 98–108. doi:10.1111/j.1477-8947.2005.00119.x
Sack, L., Cowan, P., Jaikumar, N., and Holbrook, N. (2003). The ‘hydrology’ of leaves: co-ordination of structure and function in temperate woody species. Plant Cell Environ. 26 (8), 1343–1356. doi:10.1046/j.0016-8025.2003.01058.x
Sahin, V., and Hall, M. J. (1996). The effects of afforestation and deforestation on water yields. J. Hydrol. 178 (1–4), 293–309. doi:10.1016/0022-1694(95)02825-0
Santos, V. A. H. F. D., Ferreira, M. J., Rodrigues, J. V. F. C., Garcia, M. N., Ceron, J. V. B., Nelson, B. W., et al. (2018). Causes of reduced leaf-level photosynthesis during strong El Niño drought in a Central Amazon forest. Glob. Change Bio. 24 (9), 4266–4279. doi:10.1111/gcb.14293
Schulte, P., and Hinckley, T. (1985). A comparison of pressure-volume curve data analysis techniques. J. Exp. Bot. 36 (10), 1590–1602. doi:10.1093/jxb/36.10.1590
Stanturf, J. A., Palik, B. J., and Dumroese, R. K. (2014). Contemporary forest restoration: a review emphasizing function. For. Ecol. Manag. 331, 292–323. doi:10.1016/j.foreco.2014.07.029
Stokes, A., Atger, C., Bengough, A. G., Fourcaud, T., and Sidle, R. C. (2009). Desirable plant root traits for protecting natural and engineered slopes against landslides. Plant Soil. 324 (1), 1–30. doi:10.1007/s11104-009-0159-y
Taubert, F., Fischer, R., Groeneveld, J., Lehmann, S., Müller, M. S., Rödig, E., et al. (2018). Global patterns of tropical forest fragmentation. Nature 554, 519–522. doi:10.1038/nature25508
Trueba, S., Pan, R., Scoffoni, C., John, G. P., Davis, S. D., and Sack, L. (2019). Thresholds for leaf damage due to dehydration: declines of hydraulic function, stomatal conductance and cellular integrity precede those for photochemistry. New Phytol. 223 (1), 134–149. doi:10.1111/nph.15779
Vörösmarty, C. J., Hoekstra, A. Y., Bunn, S. E., Conway, D., and Gupta, J. (2015). Fresh water goes global. Science 349 (6247), 478–479. doi:10.1126/science.aac6009
Walker, L. R., Velázquez, E., and Shiels, A. B. (2009). Applying lessons from ecological succession to the restoration of landslides. Plant Soil. 324 (1), 157–168. doi:10.1007/s11104-008-9864-1
Wingfield, M. J., Brockerhoff, E. G., Wingfield, B. D., and Slippers, B. (2015). Planted forest health: the need for a global strategy. Sci. 349 (6250), 832–836. doi:10.1126/science.aac6674
Zhang, H., Chen, H. Y. H., Lian, J., John, R., Ronghua, L., Liu, H., et al. (2018a). Using functional trait diversity patterns to disentangle the scale-dependent ecological processes in a subtropical forest. Funct. Ecol. 32, 1379–1389. doi:10.1111/1365-2435.13079
Zhang, H., John, R., Zhu, S., Liu, H., Xu, Q., Qi, W., et al. (2018b). Shifts in functional trait–species abundance relationships over secondary subalpine meadow succession in the Qinghai Tibetan Plateau. Oecologia. 188 (2), 547–557. doi:10.1007/s00442-018-4230-3
Keywords: drought stress tolerance, fast-growing, functional trait, native species, non-native species, reforestation
Citation: Luo J, Hong W, Yang J, Jiang K, Tan Z, He Q, Zhang H and Cui J (2021) Bombax ceiba is a Good Native Tree Species for Performing Reforestation to Restore Highly Degraded Tropical Forests in Hainan Island, China. Front. Environ. Sci. 9:569428. doi: 10.3389/fenvs.2021.569428
Received: 04 June 2020; Accepted: 01 February 2021;
Published: 15 April 2021.
Edited by:
Guangyu Wang, University of British Columbia, CanadaReviewed by:
Hongfang Lu, South China Botanical Garden (CAS), ChinaWenxing Long, Hainan University, China
Copyright © 2021 Luo, Hong, Yang, Jiang, Tan, He, Zhang and Cui. This is an open-access article distributed under the terms of the Creative Commons Attribution License (CC BY). The use, distribution or reproduction in other forums is permitted, provided the original author(s) and the copyright owner(s) are credited and that the original publication in this journal is cited, in accordance with accepted academic practice. No use, distribution or reproduction is permitted which does not comply with these terms.
*Correspondence: Jie Cui, amllX19jdWlAMTYzLmNvbQ==; Hui Zhang, NDQ2MDU2OTYyQHFxLmNvbQ==
†These authors have contributed equally to this work.