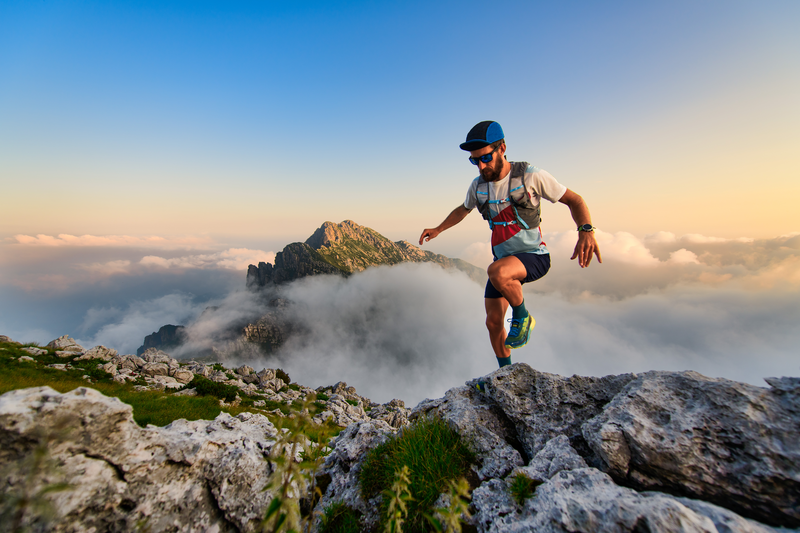
94% of researchers rate our articles as excellent or good
Learn more about the work of our research integrity team to safeguard the quality of each article we publish.
Find out more
ORIGINAL RESEARCH article
Front. Environ. Sci. , 17 December 2020
Sec. Freshwater Science
Volume 8 - 2020 | https://doi.org/10.3389/fenvs.2020.579031
This article is part of the Research Topic Balancing Hydropower and Freshwater Environments in the Global South View all 13 articles
Tropical river basins have experienced dramatically increased hydropower development over the last 20 years. These alterations have the potential to cause changes in hydrologic and ecologic systems. One heavily impacted system is the Upper Paraguay River Basin, which feeds the Pantanal wetland. The Pantanal is a Ramsar Heritage site and is one of the world's largest freshwater wetlands. Over the past 20 years, the number of hydropower facilities in the Upper Paraguay River Basin has more than doubled. This paper uses the Indicators of Hydrologic Alteration (IHA) method to assess the impact of 24 of these dams on the hydrologic regime over 20 years (10 years before and 10 years after dam installation) and proposes a method to disentangle the effects of dams from other drivers of hydrologic change using undammed “control” rivers. While most of these dams are small, run-of-the-river systems, each dam significantly altered at least one of the 33 hydrologic indicators assessed. Across all studied dams, 88 of the 256 calculated indicators changed significantly, causing changes of 5–40%, compared to undammed reaches. These changes were most common in indicators that quantify the frequency and duration of high and low pulses, along with those for the rate and frequency of hydrologic changes. Importantly, the flow regime in several undammed reaches also showed significant alterations, likely due to climate and land-use changes, supporting the need for measurements in representative control systems when attributing causes to observed change. Basin-wide hydrologic changes (in both dammed and undammed rivers) have the potential to fundamentally alter the hydrology, sediment patterns, and ecosystem of the Pantanal wetland. The proposed refinement of the IHA methods reveals crucial differences between dam-induced alteration and those assigned to other drivers of change; these need to be better understood for more efficient management of current hydropower plants or the implementation of future dams.
Growing electricity demand is a major global challenge as the need to find efficient and sustainable energy sources increases (International Energy Agency – IEA, 2019). Globally, total renewable power capacity more than doubled in the decade 2007–2017 (REN21, 2018), with a recent resurgence in focus on hydropower, especially in the developing tropics (Zarfl et al., 2015; Winemiller et al., 2016). Although hydroelectric energy is considered a source of “clean” energy (Newell et al., 2019), there are myriad environmental and social impacts related to dam operations (e.g., Fearnside, 2016; Lima et al., 2016; Latrubesse et al., 2017; Athayde et al., 2019) that must be evaluated when considering the true costs and benefits of hydropower. One critical factor is how dam operation and location alter the hydrological dynamics in the basins where impoundments are located (e.g., Magilligan and Nislow, 2005; Poff and Zimmerman, 2010).
Brazil, in particular, has an energy matrix strongly concentrated on hydroelectricity, with ~65% of its energy generated by hydropower plants1. Country-wide, there are 639 hydropower plants, including 422 small hydroelectric power plants referred to as PCH in Brazil (defined as having production potential between 5 and 30 MW and reservoirs with a surface area smaller than 3 km2) and 217 hydroelectric facilities referred to as UHE (defined as having a capacity >30 MW in operation). Over 40% of the operating hydropower plants are localized in the Brazilian Southeast and West Central regions, while planned facilities, mainly PCH (>90%) will be built in the West Central and North regions. In the Upper Paraguay Basin, where the Pantanal is found, there are 57 operating hydropower plants, of which 6 are UHE and 51 PCH (only 28 PCH with available data). Eight more hydropower plants are in construction or soon to be constructed1. An additional 80 hydropower plants are projected or planned. In 2008, there were 39 operating hydropower plants in the Upper Paraguay River Basin (Girard, 2011).
In the Upper Paraguay basin, dams are not usually built to divert water for irrigation purposes. Dams are constructed to provide electrical energy. A notable exception was the Manso dam, which was built for hydropower generation but as well to regulate droughts and flood downstream in the city of Cuiabá (Zeilhofer and Moura, 2009). This does not mean that reservoirs are not used by farmers. They do indeed use the reservoir for irrigation and also to provide drinking water for cattle, which is one of the priorities usage of water recognized by Brazilian water legislation. Consumption uses in the northern part of the basin remove 7.0% (27.4 m3/s) of the minimum flow of seven consecutive days, of which 44.0% is used for irrigation and 19.7% by animals (Agência Nacional de Águas - ANA, 2018). Withdrawals directly from reservoirs correspond to <0.01% of the available flow. Thus, despite the scenario of increased water withdrawal in the basin, these uses can be considered insignificant for changes in the flow regime on a daily scale.
Currently, many dams are constructed on the same river, one downstream of another, in cascade. Dams on the Jauru or the Juba rivers provide a good example (Figure 1). These cascades of dams can be all small hydroelectrical facilities (PCH), like in the Santana case, or include one or more large dams (UHE) like in the Juba case. Unlike the larger dams (UHE), small dams cannot control the seasonal riverine flow regime and are considered as run-of-the-river as they canalize a portion of a river, usually with little or no impoundment.
Figure 1. Dams on the river feeding the Pantanal. The upper left inset shows the Pantanal extent (yellow area) in the Upper Paraguay Basin (UPB—green area) in South America. The lower left inset shows the Brazilian Pantanal (yellow area) in the Brazilian portion of the UPB (green area) in the Brazilian States of Mato Grosso and Mato Grosso do Sul. The main figure shows the study area: the Paraguay river (red), the dammed (blue) and undammed or control rivers (yellow), of the Brazilian UPB (green shaded area) flowing into the Brazilian Pantanal wetland (yellow shaded area) and hydroelectric power plants (<30 MW: triangles; > 30 MW: stars), control stations (squares), and fluviometric stations (circles).
If hydropower development plans are fully implemented, about 40% of the flow to the Pantanal would go through one or more hydropower plants in the future (Souza Filho, 2013), which could significantly change the ecological dynamics of the Pantanal, altering not only the hydrology but also the thermal, nutrient, sediment and water chemistry regimes, geomorphology, and ecology (Olden and Naiman, 2010; Gao et al., 2012). Specific impacts resulting from impoundments include the reduction of aquatic and terrestrial productivity within the flooded areas (Burford et al., 2011; Schindler and Smits, 2017); instability of river channels (Brooks et al., 2012); reduction of available habitats and landscape alteration (Miranda et al., 2015; Aguiar et al., 2016); reduced connectivity between the river and the riparian zone; disturbance of hydrological regime (Stevaux et al., 2012); and changes in water quality (Fantin-Cruz et al., 2016; Silva et al., 2019).
Most of the recent facilities in the Upper Paraguay basin are small hydropower plants due to current government incentive policies. However, according to the World Comission on Dams - WCD (2000), small and medium-sized hydropower plants have the potential to modify vital ecosystem functions and affect water security in similar ways as large projects. The operation of small hydropower was considered to have minimal environmental impacts because they do not typically involve water storage and diversion (Small Hydro Energy Efficient Promotion Campaign Action – SHERPA, 2010; Werthessen, 2014). However, small dams can have large impacts, especially relative to their production capacity (Timpe and Kaplan, 2017); this is particularly troubling given their accelerating, widespread, and largely unregulated expansion both globally (Couto and Olden, 2018) and in Brazil (Athayde et al., 2019; Campos et al., 2020).
Understanding dam-induced changes to the flow regime in the Upper Paraguay River Basin is of regional and international relevance, as the Pantanal, a vast floodplain wetland, is considered to be one of the most diverse terrestrial biomes in the world. The Pantanal is a UNESCO World Heritage Site (Junk and Cunha, 2005), and the ecosystem produces environmental services of great economic value to the region, including maintenance of regional microclimates, regulation of river discharge, fishing, native pasture, habitat for threatened species, and wintering ground for migratory species (Wantzen et al., 2008; Tomas et al., 2019; Campos et al., 2020). The provision of these services depends on the flood pulse from upstream regions which delivers nutrients, sediments, fauna, and plant propagules to the floodplain and maintains large areas flooded for long periods (Girard et al., 2010). This natural behavior of flood-pulsed rivers conditions multiple ecological processes in the river-floodplain system, including the richness, abundance, and distribution of fauna, flora, and human activities in the region (Junk et al., 1989; Oliveira et al., 2019; Silveira et al., 2020). Constructed and planned dams in the Upper Paraguay Basin have the potential to alter nutrient cycling, sedimentation processes downstream, and the flood pulse (Ivory et al., 2019). Other potential dam impacts in the Pantanal floodplain are blocking fish migration routes (Campos et al., 2020) and thus reducing fisheries production; progressive water loss, especially in permanent water bodies, which is likely to provoke changes in the composition and structure of their biological assemblages (Silio-Calzada et al., 2017); and repercussions for the livelihoods of local communities who depend on fishing and the collection of other natural resources (Schulz et al., 2019).
Many studies have been carried out to evaluate the ecohydrological consequences of dam construction and operation (Costigan and Daniels, 2012). More than 170 hydrological indices have been developed to identify the various components of the flow regime and assess their contributions to the ecology of rivers (Olden and Poff, 2003). To assess hydrologic alterations in aquatic ecosystems, Richter et al. (1996) proposed a method for evaluating 33 different indicators of the flow regime, called Indicators of Hydrologic Alteration (IHA). This method has been widely used all over the world (Richter et al., 1996, 1997; Magilligan and Nislow, 2005; Rocha, 2010; Li and Qiu, 2016; Zhang et al., 2016; Sabino, 2017; Timpe and Kaplan, 2017). The type and magnitude of dam-induced hydrologic change found across these studies vary greatly as a function of climate, geography, dam type, and reservoir operation protocols, suggesting that regional analyses are needed to characterize relevant aspects of flow alteration in specific areas.
Environmental impact assessments commonly compare post-impact outcomes with what “would have happened” if the impact had not occurred (referred to as the “counterfactual”) (Valle and Kaplan, 2019). A common approach for testing the counterfactual is to compare variables of interest before and after the impact of a dam under the assumption that the counterfactual would be similar to the pre-impact observations. However, this assumes stationarity of all other relevant drivers. Multiple studies have used his before/after approach to quantify how dams alter riverine hydrology (e.g., Forsberg et al., 2017; Sabo et al., 2017; Gierszewski et al., 2020) despite this limitation. Stationarity is assumed by hydrologic indicator methods, including IHA, but this assumption may not be valid when other variables (e.g., climate, land use, and management regimes) are dynamic or directional, which can be the case when assessing long-term hydrological trends (Milly et al., 2008; Salas and Obeysekera, 2014).
Given this limitation, we sought to explicitly adjust for other drivers of change to quantify impacts that are related to the dam, rather than other changes such as land use or climate (Fantin-Cruz et al., 2015). This was accomplished by comparing flow variation in rivers impacted by hydropower plants to flow variation in undammed rivers, which were used as controls (see Singer, 2007; Rheinheimer and Viers, 2015; Meitzen, 2016).
The overarching goal of our study was to quantify the effects of hydropower plants on the hydrological regimes of rivers feeding the Pantanal. We used the IHA (Richter et al., 1996) to assess how the construction of dams changed the hydrological parameters in the dammed rivers. We then identified which IHA parameters were most affected by hydropower plant operation in the Upper Paraguay River Basin.
The study area encompasses the portion of the Upper Paraguay River Basin within the State of Mato Grosso (Figure 1). The Upper Paraguay River Basin has a humid tropical climate, with a well-defined rainy season between October and March and a dry season between April and September. Mean annual precipitation varies between 800 and 2,000 mm, with an average of 1,368 mm/year (Agência Nacional de Águas - ANA, 2018). The Upper Paraguay River Basin comprises a drainage area of ~600,000 km2 (Bravo et al., 2012), with the Brazilian portion totaling 362,380 km2. Forty-eight percent of the Brazilian basin is within the State of Mato Grosso with the remainder in the State of Mato Grosso do Sul (Instituto Brasileiro de Geografia e Estatística – IBGE, 2016). About 150,000 km2 of the Brazilian portion of the Upper Paraguay River Basin is classified as floodplains, where the Pantanal is located, and the rest is described as the upper plateau (Bergier et al., 2018) (Figure 1). The plateau region has an average altitude above 200 m and a maximum elevation of 1,400 m in the eastern portion of the basin with well-defined and convergent drainage (Agência Nacional de Águas - ANA, 2018). The Mato Grosso upper plateau is considered an important region for the Pantanal supply since the rivers that arise in this portion of the Upper Paraguay River Basin contribute more than two-thirds of the average annual flow into the Pantanal (Girard, 2011). Dams are built in the plateau region, and most of the dams in this study are located in the upper half of the plateau region closer to river headwaters where gradients are generally steeper. Notable exceptions are the Itiquira and Ponte de Pedra large hydropower plants built, respectively, on the Itiquira and Correntes rivers which are located in the lower half of the upper plateau region (Figure 1). Note that The Pantanal is fed by the upper plateau rivers. It has an average altitude of 80–150 m and is characterized by an intricate drainage system of large lakes, divergent watercourses, and areas of seasonal drainage and flooding.
The initial goal of the study was to use all hydropower plants in the study area, but many did not have sufficient flow data for IHA analysis. The hydropower plants retained for analysis were selected according to the extent of the available fluviometric record before and after dam construction in monitoring stations located down-river of the dam or dam cascade. Ideally, monitoring flow stations downstream of the hydropower plants should have at least 10 years of data available before and after the operational start dates (Richter et al., 1997). The Cuiabá (6628000) and São Lourenço (66400000) monitoring stations did not meet this requirement for the post-operation time series, as the dams were recently built (Table 1). However, the length of record required to support IHA analysis in the tropics vary widely, as low as 2–7 years for rivers with strongly repeated seasonality (Timpe and Kaplan, 2017). These dams were thus included as they had 7 and 9 years of post-operation fluviometric data, respectively.
Table 1. Characteristics of the hydropower plants used to study hydrological alterations in the Upper Paraguay Basin.
Sufficient hydrologic information was available for 24 operational hydropower plants in the Mato Grosso State Upper Paraguay River Basin (Table 1). Several of these are in cascades of dams on the same river, such as dams on the Juba (4 hydropower plants), Jauru (6 hydropower plants), and Santana (2 hydropower plants) rivers (Table 1, Figure 1). One gage station downstream from each one of these cascades of hydropower plants was available for IHA analysis. In other cases, the only available monitoring fluviometric station was downstream from a group of dams in the same drainage basin, such as the arrangement of 5 hydropower plants in the São Lourenço River Basin and of 4 hydropower plants built in the Piquiri basin. In the São Lourenço River arrangement, the São Lourenço hydropower plant is downstream from the other four on the São Lourenço main channel. It is also the only one with a significant reservoir (5 km2). The Sucupira and Pequi hydropower plants are installed on the Saia Branca River, the two other hydropower plants are installed on the Ibó stream and the Tenente Amaral River. The Saia Branca, Tenente Amaral, and Ibó are all tributaries of the São Lourenço.
In the Piquiri River Basin, the arrangement is composed of the Itiquira hydropower plant, built on the Itiquira River, and the Ponte de Pedra, Aquárius, and São Gabriela hydropower plants are built on the Correntes River. Both the Correntes and the Itiquira River are tributaries of the Piquiri River, where the monitoring station is located. As the Itiquira hydropower plant is the largest in this arrangement, it will be referred to as Itiquira hydropower plants. In the cases of the Manso, São Tadeu, and Poxoréo hydropower plants, there is only one hydropower plant on each river with a downstream fluviometric station (Figure 1, Table 1). For each hydropower plant, cascade, or arrangement of hydropower plants, the operation period starts when the first dam was built. We did not consider the construction period as only the operation start date was known with certainty. All data before the operation start date were taken as the pre-operation period. All data concerning hydropower plant characteristics (installed power, reservoir area, dam locations, start of operation dates) were obtained from the Brazilian Agency for Electrical Energy—ANEEL2. All data regarding monitoring fluviometric stations (location, contributing area, daily discharges, length of record) were obtained from the National Water Agency -ANA web site3 Hidroweb – Portal Hidroweb. http://www.snirh.gov.br/hidroweb/ [Accessed June 6, 2020]. Distances between downstream fluviometric stations and the dam, or the closest dam in a cascade or arrangement were obtained from Google Earth and correspond to the river path distances.
All hydropower plants with installed power ≤ 30 MW are operating as run-of-the-river systems; even if the dam elevates the water line to produce an impoundment (generally lower than 3 km2). Most of these hydropower plants do not have floodgates and consequently, the dam is not equipped to actively change the seasonal flow regime of the river. The hydropower plants over 30 MW (Juba I and II, Jauru, Manso, Itiquira, and Ponte de Pedra), have floodgates which allow for the active alteration of the flow regime downriver. However, most of these have small reservoirs (< 8 km2) which limit this capacity. Only Manso and Ponte de Pedra hydropower plants have large reservoirs. The Manso hydropower plant has a 427 km2 reservoir, roughly equivalent to 3 years of mean discharge (Zeilhofer and Moura, 2009). The Pontes de Pedra hydropower plant reservoir area is 17 km2, storing a volume equivalent to about 1 month of discharge (Fantin-Cruz et al., 2016).
In addition to pre- post-dam analysis, each dammed river was evaluated against one station in an undammed river (Figure 1, Table 2). Each station on an undammed river was chosen so that it was on a reach with no dams upstream and no downstream dams nearby. Ideally, several additional criteria to help select undammed river stations were followed whenever possible: (1) it should be in the same drainage basin as the hydropower plant; (2) the river reach should be geographically close to the station monitoring the hydropower plants; (3) and the station should be in the same river section position (e.g., if the monitoring station on a dammed river was mid-basin, the undammed river one should be too), so that river regimes would be similar. By doing so, it is also likely to reduce hydrologic changes due to the geographical variations in geology, geomorphology, and topography. Due to data availability, several of the ideal criteria could not be met for each river assessed here. Specifically, the São Tadeu undammed station is far from the dammed station; the São Lourenço undammed station is not in the same drainage basin as the undammed river monitoring gage; the Itiquira undammed station is up basin while the dammed river station is down basin (Figure 1). Note, however, that all gage stations, either for dammed or undammed rivers are located in the upper plateau region.
The Indicators of Hydrologic Alteration (IHA) is based on the analysis of hydrologic data available either from existing measurement points, such as stream gauges or wells (Richter et al., 1996). It uses 33 indicators to statistically characterize hydrologic variation within each year. These indicators inform on ecologically significant features of surface and groundwater regimes influencing aquatic, wetland, and riparian ecosystems. The 33 hydrologic alteration indicators were evaluated by comparing the hydrological regime before and after the start of operation of each hydropower plant using IHA 7.1 software (The Nature Conservancy - TNC, 2009) which produces measures of central tendency and dispersion for each parameter between defined “pre-dam” and “post-dam” time frames, allowing to quantify dam impacts assuming stationarity of other drivers. The IHA organizes these 33 indicators into 5 groups: (i) magnitude of flows (median discharge) in each month; (ii) magnitude and duration of annual extreme flow conditions (medians minima and maxima discharge of 1, 3, 7, 30, and 90 days); (iii) timing of annual extreme flow conditions (date of the 1-day minima and maxima); (iv) frequency and duration of high and low pulses (thresholds were set as the median flow plus or minus 25%), and; (v) rate and frequency of hydrologic changes (obtained by dividing the hydrologic record into “rising” and “falling” periods, which correspond to periods in which daily changes in flows are either positive or negative, respectively). Each group of parameters is associated with ecosystem functions listed in Table 3; a thorough description of each indicator is provided by Richter et al. (1997).
Table 3. Summary of hydrological parameters used in the Indicator of Hydrologic Alteration Software (IHA) and their characteristics.
One further step was included before the calculation of the hydrological alteration. To adjust for hydrological alterations due to other drivers of change (land use and climate) and estimate only alterations provoked by hydropower plants, hydrological changes in dammed rivers were compared with those found in undammed rivers. The hydrological alteration in the undammed rivers was calculated using the same procedure as for dammed rivers. Impacts in dammed rivers that were not deemed different from those encountered in undammed rivers were not included in the calculation of the hydrologic alteration.
The IHA software (The Nature Conservancy - TNC, 2009) calculates a deviation factor by comparing post to pre-impact periods for 33 hydrologic indicators. For each dammed river, an undammed river was chosen to monitor the extent of parameter deviation in the absence of hydropower plants. In this study, the deviation factor was called the hydrologic alteration factor (HA) (Timpe and Kaplan, 2017). The HAi for each IHA parameter i was the relative change of the median of the indicator of the post-impact period (Mi,post) to the pre-impact period (Mi,pre) in percent:
HAi was calculated for dammed and undammed rivers. In any undammed river, the pre and post-impact periods were the same as in the dammed river. The IHA software provided its test of significance, called significance count (SC). The SC was calculated by randomly shuffling data across the entire period of record and regenerating pre- and post-impact medians 1,000 times. The SC was the fraction of those 1,000 iterations for which calculated HAi (Equation 1) values were greater than those for the unshuffled data and could be likened to a p-value in parametric statistics (The Nature Conservancy - TNC, 2009). Whenever SC was > 0.05 in dammed or undammed rivers, HAi was considered not significant.
Next, a step-wise screening algorithm was used to assess whether a given HAi was different between dammed (d) (HAid) and its undammed control (c) rivers (HAic). This algorithm removed any changes that were statistically similar between dammed and undammed rivers. First, the HAid was tested for significance from zero. If it was not significant, its value was set to zero. Otherwise, HAid was compared to the deviation of the same IHA parameter on the undammed river (HAic). If HAic was not significant, then HAid was conserved for calculation of overall alteration. Otherwise, HAid was further screened as follows: when HAic was significant, but its direction was opposite of HAid (HAid/HAic < 0), then HAid was conserved for calculation. Otherwise, HAid was further screened as follows: if HAic was significant and its direction the same as HAid (HAid/HAic > 0), then HAid was conserved for further calculation only if HAid/HAic ≥ 1.25 (i.e., the proportional change in the IHA parameter in the dammed river was more than 25% greater than that in the undammed river); otherwise HAid was set to 0 (zero).
Equation 1 yielded both positive and negative values of HA, corresponding to an increase or decrease in IHA parameters between the pre- and post-operation periods, respectively. IHA results for dammed and undammed rivers were summarized by calculating the arithmetic average (HAmean) of the absolute value of HAi, following Timpe and Kaplan (2017). HAmean was calculated either using unadjusted HAi provided by the IHA software (unadjusted HAmean) or using only the ones adjusted by the previously described step-wise screening algorithm (adjusted HAmean). All other parameters not retained by the step-wise screening algorithm were set to zero (0).
Discharge time series at fluviometric stations on dammed and undammed rivers indicated that dams were installed on seasonal river reaches, some highly variable. The fluvial regime also varied markedly between rivers (Figure 2). The dammed and undammed Jauru river stations (Figure 2B) are more typical of mid-reach regimes with amplitude to median ratios around 5. In contrast, the dammed Poxoréo River station and its control on the undammed Jorigue River, (Supplementary Figure 1A) illustrated flow regimes of the upper basin reaches. The amplitude (maximum-minimum discharge) of the Poxoréo river was more than 60 times its median flow, while for the Jorigue River, the same ratio is over a hundred. In these two cases, the regimes of the dammed and undammed river stations were well-paired. Other such well-paired stations included the Manso hydropower plant (Supplementary Figure 1B, ratios of 11 and 8.5, for dammed and undammed stations, respectively), the Juba hydropower plant (Supplementary Figure 1E, both ratios around 5), and Santana hydropower plant (Supplementary Figure 1C, ratios of 16 and 31).
Figure 2. Discharge hydrographs for the Jauru cascade of hydropower plants. Median flow (horizontal blue lines) for dammed and undammed river gages are also shown. Maximum flows are also shown in purple. (A) Hydrograph of the dammed Jauru River. The starting operation date for each hydropower plant is marked by a vertical red dash line and the name of the hydropower plant is written or numbered (see Table 1 for details): 1 Antonio Brennand; 2 Jauru; 3 Indiavaí; 4 Ombreiras; 5 Salto; 6 Figueirópolis. (B) The undammed Jauru control river (Aguapei River).
Other dammed and undammed gage stations were less well-matched. At the Itiquira hydropower plant, the dammed river station (Supplementary Figure 1F), located in the down-basin reach (Figure 1), had a ratio of the amplitude to the median flow of 3 compared to 24 at its undammed control station in the upper mid-basin reach. At the São Tadeu hydropower plant (Supplementary Figure 1D) stations, these ratios were 5,3, and 28. The most extreme case was the São Lourenço hydropower plant (Supplementary Figure 1G) where these ratios were ~7 (dammed river) and 143 (undammed river).
It was sometimes possible to detect clear temporal variations in the flow regime associated with the installation of the hydropower plants. For example, for the Jauru hydropower plants cascade (Figure 2A) the number of low pulses increased, and the corresponding HA parameter reached 537.5% after dam operation (Table 4). Low-flow pulses were also observed at the undammed river control station, but less frequently, and the corresponding HA was also lower (Supplementary Table 1). The progressive diminution in base flow, after the start of the Jauru hydropower plant operation, was observed in both dammed and undammed river hydrographs (Figures 2A,B). The decrease in the undammed river was steeper than in the dammed river. The base flow index on the undammed river was more than 5 times higher than on the dammed river (Table 4, Supplementary Table 1), indicating that the hydropower plant operation may not have been the cause of this decline.
Table 4. Hydrologic alteration in % of the IHA parameters of the hydropower plants as calculated from equation 1.
For the Manso hydropower plant (Supplementary Figure 1B), there was an increase in base flow, as well as the diminution in the number of maximum annual flows, but no similar changes occurred on the undammed control river where there was a progressive decrease in baseflow. Changes in base flow were common on dammed and undammed river stations, both before and after hydropower plant installation. In the São Tadeu (Supplementary Figure 1D), there was an increase in baseflow around 2001, which preceded the operation of the São Tadeu I dam in 2009. Increasing and decreasing base flows were also observed at both the Juba dammed and undammed stations, as well as at the Itiquira and São Lourenço dammed stations, before and after the beginning of the hydropower plant operation.
The unadjusted HAmean and the contribution from each IHA parameter group for dammed and undammed river stations varied (Figure 3). Unadjusted HAmean of dammed rivers varied from 61.6% for the Poxoréo hydropower plant to 17.6% for the São Lourenço basin hydropower plants. Hydrological alteration for the undammed control rivers was generally lower than in the regulated rivers, varying from 32.5% at the São Lourenço arrangement to 12% at the Juba cascade. For example, HAmean on the dammed Poxoréo station was more than three times larger than at its undammed control station. The alteration was about 2 times higher for the Manso hydropower plant, 1.5 times higher for the Jauru and Juba hydropower plants, and 1.3 times higher for the São Tadeu hydropower plant. For the Itiquira hydropower plants, dammed, and undammed HAmean were roughly equal. Unexpectedly for the São Lourenço and Santana hydropower plants, HAmean of the undammed rivers was larger than for dammed rivers. Overall, hydrological alterations in the frequency and duration of high and low pulses (group 4 parameters) and the rate and frequency of hydrologic changes (group 5 parameters) constituted more than half of the observed HAmean in both dammed and undammed rivers, with the exception for the Manso hydropower plant, where group 4 and 5 hydrological alterations were about 40% of the total dammed river HAmean.
Figure 3. Comparative mean hydrological alteration (HAmean) for dammed and undammed rivers. HAmean was calculated using unadjusted HAi. The contribution of each parameter group to the overall HA is also illustrated. Dash lines separate each pair of dammed and undammed rivers. In each pair, the left bar column is HAmean for the hydropower plants dammed river and the right one is the HAmean at the control undammed river. The values of HAmean are indicated. Group 1: magnitude of monthly flow conditions; group 2: Magnitude and duration of annual extreme flow conditions; group 3: Timing of annual extreme flow conditions; group 4: Frequency and duration of high and low pulses; group 5: Rate and frequency of hydrologic changes.
Widespread and significant alterations of the flow regime occurred across many rivers in the Upper Paraguay River Basin before and after the initiation of hydropower operations (Table 4). On dammed rivers, the IHA parameters that most consistently differed from their undammed counterparts were August median discharge, the 1-day minimum flow, and the low pulse count, each with 5 occurrences. Conversely, the April median discharge, the 30-day maximum, and the low pulse duration were never found to be different in dammed and their undammed river controls (Table 4).
The Poxoréo hydropower plant, Jauru River hydroelectric cascade, and Manso hydropower plant had the largest changes, with adjusted HAmean of 43.8, 34.9, and 26.1% respectively (Figure 4). In these systems 16, 19 and 14 parameters were different from their undammed controls, respectively. For the other hydropower plants, the number of parameters different from their undammed controls varied from 1 to 10 (Table 4). The smallest adjusted HAmean values were for the Itiquira and São Lourenço River complexes: 8.0 and 4.9%, respectively, and only five and one of 33 IHA indicators differed from their undammed rivers controls (Table 4).
Figure 4. Adjusted mean hydrologic alteration (HAmean) of the studied hydropower systems. HAmeanwas calculated using adjusted HAi obtained from the step-wise screening algorithm described in section Hydropower Plants, Dammed, and Undammed Rivers Gage Stations. The contribution of each parameter group to the overall HA is also illustrated. Values of HAmean are indicated. Group 1: magnitude of monthly flow conditions; group 2: Magnitude and duration of annual extreme flow conditions; group 3: Timing of annual extreme flow conditions; group 4: Frequency and duration of high and low pulses; group 5: Rate and frequency of hydrologic changes.
The adjusted mean hydrologic alteration of the frequency and duration of high and low pulses (group 4 parameters) and the rate and frequency of hydrologic changes (group 5) were generally highest across all systems (Figure 4). However, dramatic changes were also observed in the timing of annual extreme flow conditions (group 3) at the Manso hydropower plant and for the magnitude of monthly flow conditions (group 1) at Manso and Poxoréo. The average adjusted HAmean for frequency and duration of high and low pulses was 55.8%, while the average adjusted HAmean rate and frequency of hydrologic changes was 22.5%. In both cases, the highest values of HAmean for groups 4 and 5 were in the Jauru system, with values of 152.1 and 95.7%, respectively.
For group 4 (frequency and duration of high and low pulses), changes to the low and high pulse counts drove the largest variation in all but two hydropower plants (Table 4). Differences in low pulse count were highest for the Jauru (537.5%) and Juba (166.7%) series of hydropower plants. In Poxoréo and São Lourenço hydropower plants, the high pulse durations increased by 322.2 and 158.3%, respectively. In group 5 (rate and frequency of water condition changes), rise and fall rates were the largest observed change between pre- and post-operation.
Since some HAi were set to zero to perform the calculation of HAmean with the step-wise screening algorithm, it is by definition lower than the mean hydrological alteration calculated without it, as can be seen by comparing the adjusted HAmean of Figure 4 to the unadjusted HAmean of Figure 3 (for dammed rivers). Adjusted HA varied from 4.9 to 43.8%. In some cases, as for the Jauru hydropower plants, the adjusted HAmean (34.9%) was similar to the unadjusted HAmean (38.4%), while in others like the Manso hydropower plant (44.9 vs. 26.1%) or the São Lourenço arrangement (22.9 vs. 4.9%), the differences were quite large (Table 4).
In dammed rivers, of the 256 calculated HAi, 88 (34.4%) were found to be significant (Supplementary Table 2). However, when HAi was significant, it was almost always (83 times out of 88) found to be different than in undammed rivers (Table 4). The number of significant HAi on undammed control rivers was 57 (22.3%), but only 27 (10.5%) of the HAi were significant at both the dammed and undammed stations (Supplementary Tables 1, 2). Of these 27, 16 changes occurred in opposite directions, indicating that these were different. Of the remaining, 11 occurrences where the same parameter was significant and varied in the same direction, only in 6 cases was the ratio of the HA of the dammed river to the undammed river >1.25. These results indicate that the vast majority of significantly altered parameters in dammed rivers differed from those of undammed rivers. The HAmean value adjusted by the step-wise algorithm devised in this study was able to capture these changes and is therefore useful for identifying flow-alteration effects from hydropower plants, even where the HA of the undammed control river was equally (or more) altered compared to the dammed river (e.g., São Lourenço, Santana, Itiquira; 3 out of the 8 studied hydropower plants).
The adjusted HA provided a different view on the severity of impacts than with the unadjusted HA (Figures 3, 4). Using the unadjusted HA, the impact ranking from highest to lowest was: Poxoreu, Manso, Jauru, Santana, São Lourenço, São Tadeu, Itiquira, and Juba. Using the adjusted HA, the impact ranking from highest to lowest was: Poxoreu, Jauru, Manso, Santana, São Tadeu, Juba, Itiquira, and São Lourenço.
As with the unadjusted HA, adjusted HA of parameters related to the frequency and duration of high and low pulses (group 4) and rate and frequency of hydrologic changes (group 5) were usually the largest, except in the Manso case where they were absent. Furthermore, with the adjusted HA, alterations of the timing of annual extreme flow conditions (group 3) are only seen in Manso, Santana, and Juba. For the Itiquira hydropower plants, the adjusted HA showed that these dams would only influence parameters of groups 4 and 5, while in the São Lourenço case, only parameters of group 4 would be affected.
At the parameter level, substantial differences also existed between unadjusted and adjusted HA. For example, in the Poxoreu group 4, low pulse count did not contribute to the adjusted HA even when its magnitude was comparable to high pulse count. The same can be said of the 1- and 3-day maximum in Manso group 2, the number of reversals in São Tadeu group 5, the 7-day maximum in Juba group 2, and the low pulse duration of São Lourenço group 5 (Supplementary Table 1).
From what precedes it is clear that many hydrological alterations in this study were not only dam-induced but that other drivers of change modified the regimes of the studied rivers. However, the analysis revealed that hydrologic alterations likely due to changes in land use, irrigation, and climate in the Upper Paraguay River Basin were different than those provoked by hydropower plants, as significantly altered parameters in undammed rivers were different from those in dammed rivers.
The hydrologic alterations found in this paper were consistent with those found in other studies that used IHA to evaluate the variation in the river flow regime caused by the operation of hydropower plants. There was an HA of 56.3% for the Porto Primavera hydropower plants in the adjacent Paraná River basin (Rocha, 2010). In the Brazilian Amazon region, mean HA varied between 8 and 108% (Timpe and Kaplan, 2017), including a mean HA of 29% for the Jauru hydropower plants cascade (38.4% in this study), 62% for the Manso Dam (44.9% in this study), 21% for the Juba hydropower plants cascade (17.6%), and 18% for the Itiquira hydropower plants arrangement (18.4%). The different time windows for pre- and post-dam operation, and sometimes different monitoring stations probably explains these differences.
As in other studies, the frequency and duration of high and low pulses (group 4 parameters) and the rate and frequency of hydrologic changes (group 5) were often the most affected elements of the hydrologic regime. For example, Zhang et al. (2016) reported greater variations for the IHA parameters of groups 4 and 5, especially for the number and duration of low pulses, in studies in southwest China. Richter et al. (1996) also observed the greatest alteration to elements of the frequency and duration elements of the flow regime captured by IHA groups 4 and 5. Timpe and Kaplan (2017) observed that for all of the 33 hydropower plants they evaluated in Amazonian rivers, the hydrological alteration was also generally highest for groups 4 and 5. This was related to the capacity of flow regulation by hydropower plants which reduces extreme flows (maximum and minimum) to maximize the energy generation; significantly altering the rates and durations of the maximum and minimum peaks. Richter et al. (1996) reinforce this thesis, showing that the operation of hydropower plants severely affects the behavior of river flow pulses, increasing the number of occurrences and reducing the duration of these pulses (i.e., group 4) and increasing the rates of rise and fall in the flow records (i.e., group 5).
Hydrological alterations were observed in all undammed rivers, with HAmean ranging from 12.0 to 32.5%, and 22.3% of the parameters significantly different between post and pre-operation periods. However, HAmean for the undammed rivers controlling the Santana and the São Lourenço arrangement was larger than HAmean of their corresponding dammed rivers (Figure 3). Furthermore, in the undammed controls for the Manso and Itiquira, more hydrological parameters were significantly altered than their respective dammed rivers (Supplementary Table 2). In the Upper Paraguay River Basin, the replacement of native vegetation with pastures and crops is ubiquitous in the basin. It is estimated that deforestation of 15% of the native vegetation in the floodplain area and 60% of the upper plateau has occurred in the Upper Paraguay River Basin (World Wide Fund for Nature – WWF, 2015). Existing studies on the conversion of natural soils to agriculture indicate resulting modifications to the hydrological regime. These modifications include changes in many important hydrologic parameters, including mean runoff and sediment concentration (Tucci, 2002; Rocha, 2010; Nobrega, 2014), siltation of river beds (Galdino et al., 2002), and interannual variability of minimum flows (Rocha, 2010; Rocha and Tommaselli, 2012).
Elsewhere in the world, changes in land use, irrigation, and climate have altered hydrology. For example, on the Cimarron River in Oklahoma, a variety of land use and cover changes changed a historically flashy river to a more stable river. There, HA parameters related to the magnitude of monthly flow conditions (group 1 parameters), the timing of annual extreme flow conditions (group 3), and frequency and duration of high and low pulses (group 4) were more pronounced (Dale et al., 2015). In the Mediterranean, water abstraction for irrigation purposes strongly affected the flow regime in irrigated catchments. The parameters related to the timing of annual extreme flow conditions (group 3), frequency and duration of high and low pulses (group 4), and rate and frequency of hydrologic changes (group 5) were strongly impacted (Stefanidis et al., 2016). In the Geba catchment, Ethiopia, the expansion of agricultural and grazing land at the expense of natural vegetation increased almost all hydrological parameters from 1972 to 2014 (Gebremicael et al., 2019).
In the Upper Paraguay River Basin, the largest hydrological alterations at control stations were observed for parameters related to frequency and duration of high and low pulses (group 4) and rate and frequency of hydrologic changes (group 5), whose combined relative importance varies from 50 to 84% of the total HAmean (Figure 3). As for the magnitude of monthly flow conditions (group 1), the magnitude and duration of annual extreme flow conditions (group 2), and the timing of annual extreme flow conditions (group 3), HAmean of undammed rivers were either larger (Jauru, Santana, São Lourenço) or quite similar (Juba and Itiquira) compared to those of dammed rivers (Figure 3).
For most of the studied dammed (Figure 4) and undammed rivers (Figure 3), except for the case of Manso, the larger hydrological impacts were related mainly to the frequency and duration of high and low pulses (group 4 parameters) and to a lesser extent to the rate and frequency of hydrologic changes (group 5). According to Richter (1996 – Table 3), these hydrological impacts would generally change the stress level for terrestrial plants, the availability of habitat for aquatic organisms, water birds and other terrestrial animals, the desiccation stress for stream edge organisms, amongst others (Table 3). For the pantaneiros, these may result in harsher conditions for the pasture sustaining cattle farming and lower fish catches (Schulz et al., 2019). In the case of the Manso dam, the alteration of the magnitude of monthly flow conditions (group 1), the magnitude and duration of annual extreme flows conditions (group 2), and the timing of annual extreme flow conditions (group 3) of the Manso River would result in changes in habitat availability for aquatic organisms, changes on oxygen levels in the water column, dehydration in animals, change the duration of stressful conditions in aquatic environments, change the access to special habitats during reproduction or to avoid predation and disturb spawning cues for migratory fishes.
Many studies relating the floodplain ecosystem functions to the hydrological regime link flood pulse and aquatic organisms (Petrere et al., 2002; Bailly et al., 2008; Costa and Mateus, 2009; Lourenço et al., 2012; Pinho and Marini, 2012; Ziober et al., 2012; Scanferla and Súarez, 2016; Barzotto and Mateus, 2017; Penha et al., 2017; Tondato et al., 2018; Pereira and Súarez, 2019; Santana et al., 2019). However, in most of these studies, the hydrological conditions were not sufficiently detailed to make direct correspondence with the observed HA in the upper plateau rivers feeding the Pantanal. In some cases, however, it is possible to make inferences between the upper plateau river regimes and the ecological functions in the floodplain. For example, Wantzen et al. (2016) viewed the most severe environmental problems currently threatening floodplain invertebrates as alteration of the natural rhythm of the flood pulse. This change affects all flood pulse-adapted species, not only invertebrates, which lose their habitats when the frequency and duration of high or low pulse (group 4 parameters) are altered as we observed in all dammed rivers except for the Manso case (Table 3, Figure 4).
The intensity, frequency, and amplitude of the flood and drought phases also can modify connectivity and affect the biodiversity of the benthic assemblages in the Pantanal (Marchese et al., 2005). The long duration of hydrological connectivity acts to unify effects on the physical and biological characteristics of neighboring water bodies in river-floodplain systems. Isolating or drying water bodies, which occurs with long droughts, reduce benthic diversity. Similarly, Catella and Petrere (1996) found that the floodplain lakes function as a dry season feeding ground for small-sized species of fish, which are potential prey for the more highly valued larger species of fish. The number and connectivity of these lakes can be linked to the magnitude and duration of annual extreme flow conditions as well as to the frequency and duration of high low pulses. With the operation of the Manso dam, the peak of the drought (timing of annual extreme conditions) not only arrived sooner than before the dam but all parameters related to the magnitude and duration of annual minima increased (magnitude and duration of annual extreme flow conditions, Table 4).
Even though the dam impacts on fish and fisheries in the Pantanal are not still clearly visible or demonstrated, in the nearly located Paraná River basin, such impacts are now well-established. There, dam construction started in the 1980s, and large dams are more numerous than in the Upper Paraguay River Basin. Their impacts on river regimes have reduced the extent and duration of flood events, limiting the reproductive processes of several fish species (Agostinho et al., 2004) and impacting fish populations (Agostinho et al., 2004, 2007, 2016).
The step-wise screening algorithm presented here retains a specific HA parameter based on several conditions. The “removal” of land use and climate-induced changes in hydrology is, in the end, not a complete removal. The hydrologic alteration factor (HA) of the dammed river is only conserved if significant. The HA of the dammed river is further considered if, in comparison, the HA in the undammed river (1) is not significant, (2) is of the opposite direction as in the dammed river, or (3) if the HA of the dammed river is at least 25% larger than in the undammed river. In the latter case, the HA due to land uses or climate change could still contribute to the “overall” HA in the dammed river. However, in this specific study, this is unlikely to substantially change our findings. Of the 83 HA retained for the calculation of the adjusted HAmean, only 6 (7.2%) were conserved because of this last criterion.
The definition of pre and post-impact period to assess the HA of other drivers of changes at the undammed control river is one main weakness of the proposed method. For example, the undammed Jorigue River, due to its location, was used as a control for both the Poxoréo and São Lourenço hydropower plants (Table 2). When the Jorigue River station was used as the Poxoréo control, the year defining the pre/post-operation period was 1998, yielding HAmean of 17.8%. When it was used as São Lourenço control, the defining year was 2008, and HAmean was 32.5% (Figure 3). Not only was the overall HA larger, but the relative contributions of the parameter groups also varied. When the undammed Jorigue River was used as a control for the Poxoreu dammed river, HA related to the magnitude and duration of annual extreme flow conditions (group 2) was the least important. When used as a control for the São Lourenço hydropower plants, the HA of group 2 was third in importance, almost as much as the rate and frequency of hydrologic changes (group 5) (Figure 3, Supplementary Table 1).
Dams are built relatively quickly unlike increasing irrigation, land use, and climate change which occur progressively over much longer periods that could be equivalent to the temporal length of the fluviometric time series used to assess the HA. For the Jorigue River, the larger HA found when using it as the São Lourenço control might have reflected the fact that the length of record of the post-impact period was relatively short (10 years from 2008 to 2018) and prone to the influence of stochastic events such as large floods that were infrequent during this time span (2008–2018), while relatively frequent before (Figure 2A). Alternatively, it might capture better the fact that irrigation in the Cerrado region increased dramatically after 2000 (Martins and Santos, 2017). There is no simple alternative to choosing the start of operation date to assess HA from other drivers of change. An alternative would be to analyze independent of stationarity, such as proposed by Valle and Kaplan (2019), who suggested using a Gaussian Copula model to predict the counterfactual in the presence of substantial data gaps through the integration of data from multiple sources. These models have been widely applied in hydrology to quantify the association between multiple hydrological variables, such as drought duration, affected area, and severity, annual maxima of stream flows or rainfalls, and to predict associations among climate and flows.
Finally, the choice of the undammed river gage station is linked to the availability of data. As noted in section Hydropower Plants, Dammed and Undammed Rivers Gage Stations, these gages were selected so that dammed and dammed river regimes would be similar, and that hydrological variations due to geology, geomorphology, and topography would be reduced. However, hydrologic changes due to these factors, namely gage positions in the drainage basin, hydrological variations in geology, geomorphology, and topography, could not be controlled and were not quantified.
Despite these drawbacks, using the proposed step-wise algorithm to assess HA has several advantages for the management of dams and the conservation of the Pantanal. It selects only dam-induced alteration and reduces the number of parameters that management would have to focus on to diminish HA. For example, in the Manso case, the efforts to reduce impacts to the Cuiabá River flow would have to concentrate on parameters related to the magnitude of monthly flow conditions, the magnitude, and duration of annual extreme flows conditions, and the timing of annual extreme flow conditions since no parameters related to the frequency and duration of high and low pulses nor the rate and frequency of hydrologic changes significantly differed from their undammed river control. Specifically, median flows of June to October significantly differed from their control and these could be targets for restituting a more natural flow to the Cuiabá River and its flooding regime in the Pantanal as already proposed by Zeilhofer and Moura (2009). Regarding flow extremes, operation targets would only be on minima as no maxima significantly differed from their control. Regarding timing, dam operation affected the date of the minimum flow but not the maximum, so operation targets could be set in direction of the pre-operation period minimum date.
Since most of the studied dams (21 out of 24) were part of cascades or arrangements, reducing impacts might not be as straightforward as in the single Manso hydropower plant. However, the HA analysis reveals crucial differences in the most-impacted parameters that ought to be understood to better manage actual hydropower plants or the implementation of future ones. For example, the bulk of the impact in the Jauru, Juba, Itiquira, and São Lourenço was related to the frequency and duration of high and low pulses (Figure 4). However, in the São Lourenço case, it was only the high-pulse duration which was affected, while for the other three hydropower plants, the low and high pulse counts were most impacted (Supplementary Table 1).
In this paper, we refined the widely used IHA method to adjust for the effects of other hydrologic drivers such as land-use and climate change to estimate the impacts due to damming. These impacts differ from those of other drivers. It is compelling to assert what are effectively the alterations provoked by the operation of hydropower plants on river systems to make the right decisions to diminish these impacts. Further research is needed to assess hydrological alterations relevant to different drivers of change. In the Pantanal region, where the number of hydropower plants is growing fast, this is essential to strike a balance between the benefits of hydropower and its impacts on fluvial ecosystem services.
Publicly available datasets were analyzed in this study. This data can be found at: http://www.snirh.gov.br/hidroweb/.
PE and PG conceived the idea and curated and organized the data. PE, PG, and IF-C discussed the idea. PE ran the IHA software and wrote the original manuscript draft. PG, PE, HT, IF-C, and DK discussed the results. IF-C did the project administration. PG supervised the manuscript. PE, IF-C, HT, PG, and DK reviewed and edited the writing. All authors contributed to the article and approved the submitted version.
The authors declare that the research was conducted in the absence of any commercial or financial relationships that could be construed as a potential conflict of interest.
The authors thank CAPES for the granting of master's scholarships. Further thanks to CNPQ (Case 435543/2018-0) and FAPEMAT (Case 0249639/2017) for financial support. Thanks to the Brazilian National Water Agency (ANA) for providing the basin database.
The Supplementary Material for this article can be found online at: https://www.frontiersin.org/articles/10.3389/fenvs.2020.579031/full#supplementary-material
1. ^Agência Nacional de Energia Elétrica – ANEEL (2020a) Sistema de Informações de Geração da ANELL SIGA – Empreendimentos por Sub-bacia. https://clck.ru/NpGrX [Accessed June 6, 2020].
2. ^Agência Nacional de Energia Elétrica – ANEEL (2020b) SIGEL - Sistema de Informações Georreferenciadas do Setor Elétrico SIGEL– Download. https://sigel.aneel.gov.br/Down/ [Accessed June 6, 2020].
Agência Nacional de Águas - ANA (2020). Portal Hidroweb. Available online at: https://www.snirh.gov.br/hidroweb/ (accessed June 6, 2020).
Agência Nacional de Águas - ANA. (2018). Plano de Recursos Hídricos da Região Hidrogr?fica do Paraguai – PRH Paraguai. Relatório final. Brasília– DF. 379 p.
Agência Nacional de Energia Elétrica – ANEEL (2020a). Banco de Informações de Geração – BIG. Available online at: http://www2.aneel.gov.br/aplicacoes/capacidadebrasil/capacidadebrasil.cfm (accessed June 3, 2020).
Agência Nacional de Energia Elétrica – ANEEL (2020b). Sistema de Informações Georreferenciadas do Setor Elétrico – SIGEL. Available online at: http://www2.aneel.gov.br/aplicacoes/capacidadebrasil/capacidadebrasil.cfm (accessed June 3, 2020).
Agostinho, A. A., Gomes, L. C., Santos, N. C. L., Ortega, J. C. G., and Pelicice, F. M. (2016). Fish assemblages in neotropical reservoirs: colonization patterns, impacts and management. Fish. Res. 173, 26–36. doi: 10.1016/j.fishres.2015.04.006
Agostinho, A. A., Marques, E. E., Agostinho, C. S., Almeida, D. A. D., Oliveira, R. J. D., and Melo, J. R. B. D. (2007). Fish ladder of Lajeado Dam: migrations on one-way routes? Neotrop. Ichthyol. 5, 121–130. doi: 10.1590/S1679-62252007000200005
Agostinho, A. A., Thomaz, S. M., and Gomes, L. C. (2004). Threats for biodiversity in the floodplain of the Upper Paraná river: effects of hydrological regulation by dams. Ecohydrol. Hydrobiol. 4, 255–256. Available online at: http://repositorio.uem.br:8080/jspui/bitstream/1/5287/1/242.pdf
Aguiar, F. C., Martins, M. J., Silva, P. C., and Fernandes, M. R. (2016). Riverscapes downstream of hydropower dams: effects of altered flows and historical land-use change. Landsc. Urban Plan. 153, 83–98. doi: 10.1016/j.landurbplan.2016.04.009
Athayde, S., Mathews, M., Bohlman, S., Brasil, W., Doria, C. R., Dutka-Gianelli, J., et al. (2019). Mapping research on hydropower and sustainability in the Brazilian Amazon: advances, gaps in knowledge and future directions. Curr. Opin. Environ. Sustain. 37, 50–69. doi: 10.1016/j.cosust.2019.06.004
Bailly, D., Agostinho, A. A., and Suzuki, H. I. (2008). Influence 545 of the flood regime on the reproduction of fish species with different reproductive strategies in the Cuiabá River, Upper Pantanal, Brazil. River Res. Appl. 24, 1218–1229. doi: 10.1002/rra.1147
Barzotto, E., and Mateus, L. (2017). Reproductive biology of the migratory freshwater fish Salminus Brasiliensis (Cuvier. 1816). in the Cuiabá River basin, Brazil. J. Appl. Ichthyol. 33, 415–422. doi: 10.1111/jai.13262
Bergier, I., Assine, M. L., McGlue, M. M., Alho, C. J. R., Silva, A., Guerreiro, R. L., et al. (2018). Amazon rainforest modulation of water security in the Pantanal wetland. Sci. Total Environ. 619-620, 1116–1125. doi: 10.1016/j.scitotenv.2017.11.163
Bravo, J. M., Allasia, D., Paz, A. R., Collischonn, W., and Tucci, C. E. M. (2012). Coupled hydrologic-hydraulic modeling of the upper paraguay River Basin. J. Hydrol. Eng. 17, 635–646. doi: 10.1061/(ASCE)HE.1943-5584.0000494
Brooks, K. N., Ffolliott, P. F., and Magner, J. A. (2012). Hydrology and the Management of Watersheds. 4th Edn. (New Jersey, NY: Wiley & Sons). doi: 10.1002/9781118459751
Burford, M. A., Revill, A. T., Palmer, D. W., Clementson, L., Robson, B. J., and Webster, I. T. (2011). River regulation alters drivers of primary productivity along a tropical river-estuary system. Marine Freshwater Res. 62, 141–151. doi: 10.1071/MF10224
Campos, M. M., Tritico, H., Girard, P., Zeilhofer, P., Hamilton, S., and Fantin-Cruz, I. (2020). Predicted impacts of proposed hydroelectric facilities on fish migration routes upstream from the Pantanal wetland (Brazil). River Res. Appl. 36, 452–464. doi: 10.1002/rra.3588
Catella, A. C., and Petrere, M. Jr. (1996). Feeding patterns in a fish community of Baia da Onça, afloodplain lake of the Aquidauana River, Pantanal, Brazil. Fish. Manag. Ecol. 3, 229–237. doi: 10.1111/j.1365-2400.1996.tb00150.x
Costa, R. M. R. D., and Mateus, L. A. D. F. (2009). Reproductive biology of pacu Piaractus mesopotamicus (Holmberg. 1887). (Teleostei: Characidae). in the Cuiabá River Basin, Mato Grosso, Brazil. Neotropic. Ichthyol. 7, 447–458. doi: 10.1590/S1679-62252009000300012
Costigan, K. H., and Daniels, M. D. (2012). Damming the prairie: human alteration of great plains river regimes. J. Hydrol. 444–445, 90–99. doi: 10.1016/j.jhydrol.2012.04.008
Couto, T. B., and Olden, J. D. (2018). Global proliferation of small hydropower plants - science and policy. Front. Ecol. Environ. 16, 91–100. doi: 10.1002/fee.1746
Dale, J., Zou, C. B., Andrews, W. J., Long, J. M., Liang, Y., and Qiao, L. (2015). Climate, water use, and land surface transformation in an irrigation intensive watershed—streamflow responses from 1950 through 2010. Agric. Water Manag. 160, 144–152. doi: 10.1016/j.agwat.2015.07.007
Fantin-Cruz, I., Pedrollo, O., Girard, P., Zeilhofer, P., and Hamilton, S. K. (2015). Effects of a diversion hydropower facility on the hydrological regime of the correntes river, a tributary to the Pantanal floodplain, Brazil. J. Hydrol. 531, 810–820. doi: 10.1016/j.jhydrol.2015.10.045
Fantin-Cruz, I., Pedrollo, O., Girard, P., Zeilhofer, P., and Hamilton, S. K. (2016). Changes in river water quality caused by a diversion hydropower das bordering the Pantanal floodplain. Hydrobiologia 768, 223–238. doi: 10.1007/s10750-015-2550-4
Fearnside, P. M. (2016). Environmental and social impacts of hydroelectric dams in brazilian Amazonia: implications for the Aluminum industry. World Dev. 77, 48–65. doi: 10.1016/j.worlddev.2015.08.015
Forsberg, B. R., Melack, J. M., Dunne, T., Barthem, R. B., Goulding, M., Paiva, R. C. D., et al. (2017). The potential impact of new Andean dams on Amazon fluvial ecosystems. PLoS ONE 12:e0182254. doi: 10.1371/journal.pone.0182254
Galdino, S., Vieira, L., Oliveira, H., and Cardoso, E. (2002). Circular Técnica 37 - Impactos da Agropecuária nos Planaltos sobre o Regime Hidrológico do Pantanal. (Brasília: Embrapa).
Gao, B., Yang, D., Zhao, T., and Yang, H. (2012). Changes in the eco-flow metrics of the Upper Yangtze River from 1961 to 2008. J. Hydrol. 448–449, 30–38. doi: 10.1016/j.jhydrol.2012.03.045
Gebremicael, T. G., Mohamed, Y. A., and Van der Zaag, P. (2019). Attributing the hydrological impact of different land use types and their long-term dynamics through combining parsimonious hydrological modelling, alteration analysis and PLSR analysis. Sci. Total Environ. 660, 1155–1167. doi: 10.1016/j.scitotenv.2019.01.085
Gierszewski, P. J., Habel, M., Szmanda, J., and Malgorzata, L. (2020). Evaluating effects of dam operation on flow regimes and riverbed adaptation to those changes. Sci. Total Environ. 710:136202. doi: 10.1016/j.scitotenv.2019.136202
Girard, P., Fantin-Cruz, I., Oliveira, S. M. L., and Hamilton, S. K. (2010). Small-scale spatial variation of inundation dynamics in a floodplain of the Pantanal (Brazil). Hydrobiologia 638, 223–233. doi: 10.1007/s10750-009-0046-9
Girard, P. (2011). “Hydrology of surface and ground waters in the Pantanal floodplains,” in The Pantanal: Ecology, Biodiversity and Sustainable Management of a Large Neotropical Seasonal Wetland, ed. Sofia (Pensoft Publishers), 103–126.
Instituto Brasileiro de Geografia e Estatística – IBGE (2016). Estimativas Populacionais Para os Municípios e Para as Unidades da Federação. Available online at: https://ww2.ibge.gov.br/home/estatistica/populacao/estimativa2016/estimativa_dou.shtm (accessed January 14, 2019).
International Energy Agency – IEA (2019). World Energy Outlook 2019. Available online at: https://www.iea.org/reports/world-energy-outlook-2019 (accessed June 6, 2020).
Ivory, S. J., McGlue, M. M., Spera, S., Silva, A., and Bergier, I. (2019). Vegetation, rainfall, and pulsing hydrology in the Pantanal, the world's largest tropical wetland. Environ. Res. Lett. 14:124017. doi: 10.1088/1748-9326/ab4ffe
Junk, W., Bayley, P. B., and Sparks, R. E. (1989). The flood pulse concept in river-floodplain systems. Canad. J. Fish. Aquat. Sci. 106, 110–127.
Junk, W. J., and Cunha, C. N. (2005). Pantanal: a large South American wetland at a crossroads. Ecol. Eng. 24, 391–401. doi: 10.1016/j.ecoleng.2004.11.012
Latrubesse, E. M., Arima, E. Y., Dunne, T., Park, E., Baker, V. R., D'Horta, F. M., et al. (2017). Damming the rivers of the Amazon basin. Nature 546, 363–369. doi: 10.1038/nature22333
Li, F. F., and Qiu, J. (2016). Incorporating ecological adaptation in a multi-objective optimization for the three gorges reservoir. J. Hydroinform. 18, 564–578. doi: 10.2166/hydro.2015.045
Lima, J. M. T., Valle, D., Moretto, E. M., Pulice, S. M. P., Zuca, N. L., Roquetti, D. R., et al. (2016). A social-ecological database to advance research on infraestructure development in the Brazilian Amazon. Sci. Data 3:160071. doi: 10.1038/sdata.2016.71
Lourenço, L. S., Fernandes, I. M., Penha, J., and Mateus, L. A. (2012). Persistence and stability of cichlid assemblages in neotropical floodplain lagoons. Environ. Biol. Fish. 93, 427–437. doi: 10.1007/s10641-011-9933-9
Magilligan, F. J., and Nislow, K. H. (2005). Changes in hydrologic regime by dams. Geomorphology 71, 61–78. doi: 10.1016/j.geomorph.2004.08.017
Marchese, M. R., Wantzen, K. M., and De Drago, I. E. (2005). Benthic invertebrate assemblages and species diversity patterns of the Upper Paraguay River. River Res. Appl. 21, 485–499. doi: 10.1002/rra.814
Martins, R. A., and Santos, E. V. (2017). Expansão do agrohidronegócio do pivô central no cerrado goiano e a ineficiência do poder público na gestão dos recursos hídricos. Anais SNCMA 8:p. 1.Available online at: http://anais.unievangelica.edu.br/index.php/sncma/article/view/14
Meitzen, K. M. (2016). Stream flow changes across North Carolina (USA). 1955-2012 with implications for environmental flow management. Geomorphology 252, 171–184. doi: 10.1016/j.geomorph.2015.06.019
Milly, P. C. D., Betancourt, J., Falkenmark, M., Hirsch, R. M., Kundzewicz, Z. W., Lettenmaier, D. P., et al. (2008). Stationarity Is Dead: Whither Water Management? Science 319, 573–574. doi: 10.1126/science.1151915
Miranda, R. B., Scarpinella, G. D. A., da Silva, R. S., and Mauad, F. F. (2015). Water erosion in Brazil and in the World: a brief review. Modern Environ. Sci. Eng. 1, 17–26. doi: 10.15341/mese(2333-2581)/01.01.2015/003
Newell, R. G., Pizer, W. A., and Raimi, D. (2019). U.S. federal government subsidies for clean energy: design choices and implications. Energy Econ. 80, 831–841. doi: 10.1016/j.eneco.2019.02.018
Nobrega, R. S. (2014). Impactos do desmatamento e de mudanças climáticas nos recursos hídricos na Amazônia ocidental utilizando o modelo SLURP. Revista Brasileira de Meteorol. 29, 111–120. doi: 10.1590/0102-778620130024
Olden, J. D., and Naiman, R. J. (2010). Incorporating thermal regimes into environmental flows assessments: modifying dam operations to restore freshwater ecosystem integrity. Freshw. Biol. 55, 86–107. doi: 10.1111/j.1365-2427.2009.02179.x
Olden, J. D., and Poff, N. L. (2003). Redundancy and the choice of hydrologic indices for characterizing streamflow regimes. River Res. Appl. 19, 101–121. doi: 10.1002/rra.700
Oliveira, M. D., Calheiros, D. F., and Hamilton, S. K. (2019). Mass balances of major solutes, nutrients and particulate matter as water moves through the floodplains of the Pantanal (Paraguay River, Brazil). Revista Brasileira de Recursos Hídricos (RBRH). 24:e1. doi: 10.1590/2318-0331.231820170169
Penha, J., Landeiro, V. L., Ortega, J. C., and Mateus, L. (2017). Interchange between flooding and drying, and spatial connectivity control the fish metacommunity structure in lakes of the Pantanal wetland. Hydrobiologia 797, 115–126. doi: 10.1007/s10750-017-3164-9
Pereira, M. J., and Súarez, Y. R. (2019). Reproductive ecology of Otocinclus vittatus (Regan. 1904). in the Pantanal floodplain, upper Paraguay River basin. Brazil. J. Biol. 79, 735–741. doi: 10.1590/1519-6984.191560
Petrere, M. Jr, Agostinho, A. A., Okada, E. K., and Júlio, H. F. Jr (2002). Review of the fisheries in the Brazilian portion of the Paraná/Pantanal basin. Manag. Ecol. Lake Reserv. Fish. 123–143. doi: 10.1002/9780470995679.ch11
Pinho, J. B., and Marini, M. A. (2012). Using birds to set conservation priorities for Pantanal wetland forests, Brazil. Bird Conserv. Int. 22, 155–169. doi: 10.1017/S0959270911000207
Poff, N. L., and Zimmerman, J. K. H. (2010). Ecological responses to altered flow regimes: a literature review to inform the science and management of environmental flows. Freshw. Biol. 55, 194–205. doi: 10.1111/j.1365-2427.2009.02272.x
Rheinheimer, D. E., and Viers, J. H. (2015). Combined effects of reservoir operations and climate warming on the flow regime of hydropower bypass reaches of California's Sierra Nevada. River Res. Appl. 31, 269–279. doi: 10.1002/rra.2749
Richter, B. D., Baumgartner, J. F., Powell, J., and Braun, D. (1996). A method for assessing hydrologic alteration with ecosystems. Conserv. Biol. 10, 1163–1174. doi: 10.1046/j.1523-1739.1996.10041163.x
Richter, B. D., Baumgartner, J. F., Wigington, R., and Braun, D. (1997). How much does a river need? Freshw. Biol. 37, 231–249. doi: 10.1046/j.1365-2427.1997.00153.x
Rocha, P. C. (2010). Indicadores de alteração hidrológica no Alto Rio Paraná: intervenções humanas e implicações na dinâmica do ambiente fluvial. Soc. Nat. 22, 191–211. doi: 10.1590/S1982-45132010000100014
Rocha, P. C., and Tommaselli, J. T. G. (2012). Variabilidade hidrológica nas bacias dos rios aguapeí e peixe, região do oeste paulista. Revista Brasileira de Climatol. 10, 69–84. doi: 10.5380/abclima.v10i1.30588
Sabino, A. A. (2017). Verificação do Impacto de Reservatórios de Usinas Hidrelétricas no Regime Hidrológico de Bacias Hidrográficas Utilizando o Software IHA. (Ph.D. Dissertation/master's thesis), Universidade Federal do Paraná, Curitiba, Brazil.
Sabo, J. L., Ruhi, A., Holtgrieve, G. W., Elliott, V., Arias, M. E., and Ngor, P. B. (2017) Designing river flows to improve food security futures in the Lower Mekong Basin. Science 358:eaao1053. doi: 10.1126/science.aao1053.
Salas, J. D., and Obeysekera, J. (2014). Revisiting the concepts of return period and risk for nonstationary hydrologic extreme events. J. Hydrol. Eng. 19, 554–568. doi: 10.1061/(ASCE)HE.1943-5584.0000820
Santana, C. A., Tondato, K. K., and Súarez, Y. R. (2019). Reproductive biology of Hyphessobrycon eques (Characiformes: Characidae) in Southern Pantanal, Brazil. Brazil. J. Biol. 79, 70–79. doi: 10.1590/1519-6984.176273
Scanferla, A. F., and Súarez, Y. R. (2016). Flood pulse are the main determinant of feeding dynamics and composition of Odontostilbe pequira (Characiformes: Characidae) in southern Pantanal, Brazil. Acta Limnol. Bras. 28:e19. doi: 10.1590/s2179-975x3316
Schindler, D. E., and Smits, A. P. (2017). Subsidies of aquatic resources in terrestrial ecosystems. Ecosystems 20, 78–93. doi: 10.1007/s10021-016-0050-7
Schulz, C., Whitney, B. S., Rossetto, O. C., Neves, D. M., Crabb, L., Oliveira, E. C., et al. (2019). Physical, ecological and human dimensions of environmental change in Brazil's Pantanal. Sci. Total Environ. 687, 1011–1027. doi: 10.1016/j.scitotenv.2019.06.023
Silio-Calzada, A., Barquín, J., Huszar, V. L. M., Mazzeo, N., Méndez, F., and Álvarez-Martínez, J. M. (2017). Long-term dynamics of a floodplain shallow lake in the Pantanal wetland: is it all about climate? Sci. Total Environ. 605–606, 527–540. doi: 10.1016/j.scitotenv.2017.06.183
Silva, A. C. C., Fantin-Cruz, I., Lima, Z. M., and Figueiredo, D. M. (2019). Cumulative changes in water quality caused by six cascading hydroelectric dams on the Jauru River, tributary of the Pantanal floodplain. Revista Brasileira de Recursos Hídricos. 24:e18. doi: 10.1590/2318-0331.241920180047
Silveira, R., Leão-Neto, W. M., and Silva, F. H. B. (2020). Small-sized fish as possible seed dispersers: disclosing novel fish and plant species interactions in the Pantanal wetland. Stud. Neotrop. Fauna Environ. 55, 36–43. doi: 10.1080/01650521.2019.1669422
Singer, M. B. (2007). The influence of major dams on hydrology through the drainage network of the Sacramento River Basin, California. River Res. Appl. 23, 55–72. doi: 10.1002/rra.968
Small Hydro Energy Efficient Promotion Campaign Action – SHERPA (2010). Hydropower and Environment: Technical and Operational Procedures to Better Integrate Small Hydropower Plants in the Environment. (Brussels).
Souza Filho, E. E. (2013). As barragens na bacia do Rio Paraguai e a possível influência sobre a descarga fluvial e o transporte de sedimentos. Boletim de Geografia. 31, 117–133. doi: 10.4025/bolgeogr.v31i1.13638
Stefanidis, K., Panagopoulos, Y., Psomas, A., and Mimikou, M. (2016). Assessment of the natural flow regime in a Mediterranean river impacted from irrigated agriculture. Sci. Total Environ. 573, 1492–1502. doi: 10.1016/j.scitotenv.2016.08.046
Stevaux, J. C., Corradini, F. A., and Aquino, S. (2012). Connectivity process and riparian vegetation of the Upper Paraná River, Brazil. J. S. Am. Earth Sci. 46, 113–121. doi: 10.1016/j.jsames.2011.12.007
The Nature Conservancy - TNC (2009). Indicators of Hydrologic Alteration: Version. 7.1. Arlington, VA:TNC worldwilde office. Available online at: https://www.conservationgateway.org/Documents/IHAV7.pdf
Timpe, K., and Kaplan, D. (2017). The changing hydrology of a dammed Amazon. Sci. Adv. 3:1700611. doi: 10.1126/sciadv.1700611
Tomas, W. M., De Oliveira Roque, F., Morato, R. G., Medici, P. E., Chiaravalloti, R. M., Tortato, F. R., et al. (2019). Sustainability agenda for the Pantanal wetland: perspectives on a collaborative interface for science, policy, and decision-making. Trop. Conserv. Sci. 12, 1–30. doi: 10.1177/1940082919872634
Tondato, K. K., Súarez, Y. R., de Fátima Mateus, L. A., Vicentin, W., and Fialho, C. B. (2018). Life history characteristics and recruitment of fish under the effect of different hydrological regimes in a tropical floodplain. Environ. Biol. Fishes. 101, 1369–1384. doi: 10.1007/s10641-018-0784-5
Tucci, C. (2002). Impactos da Variabilidade Climática e do Uso do solo Nos Recursos Hídricos. Fórum Brasileiro de Mudanças Climáticas. Câmara Temática sobre Recursos Hídricos. (Brasília: Agência Nacional de Águas – ANA).
Valle, D., and Kaplan, D. (2019). Quantifying the impacts of dams on riverine hydrology under non-stationary conditions using incomplete data and Gaussian copula models. Sci. Total Environ. 677, 599–611. doi: 10.1016/j.scitotenv.2019.04.377
Wantzen, K. M., Cunha, C. N., Junk, W. J., Girard, P., Rossetto, O. C., Penha, J. M., et al. (2008). Towards a sustainable management concept for ecosystem services of the Pantanal wetland. Ecohydrol. Hydrobiol. 8, 115–138. doi: 10.2478/v10104-009-0009-9
Wantzen, K. M., Marchese, M. R., Marques, M. I., and Battirola, L. D. (2016). “Invertebrates in neotropical floodplains,” in Invertebrates in Freshwater Wetlands, eds. D. Batzer, and D. Boix (Cham: Springer), p. 493–524. doi: 10.1007/978-3-319-24978-0_14
Werthessen, D. (2014). Environmental considerations of small-scale hydroelectric power plants in Himachal Pradesh, India. Undergrad. Rev. 10, 178–185.
Winemiller, K. O., Mcintyre, P. B., Castello, L., Fluet-Chouinard, E., Giarrizzo, T., Nam, S., et al. (2016). Balancing hydropower and biodiversity in the Amazon, Congo, and Mekong. Science 351, 128–129. doi: 10.1126/science.aac7082
World Wide Fund for Nature – WWF (2015). Bacia do Alto Paraguai - cobertura vegetal: monitoramento das alterações da cobertura vegetal e uso do solo na Bacia do Alto Paraguai porção brasileira. Período de Análise: 2012 a. 2014. Brasília: WWF.
World Comission on Dams - WCD. (2000). Dams and Development: A New Framework for Decision-Making. (London: London and Sterling Scan Publications).
Zarfl, C., Lumsdon, A. E., Berlekamp, J., Tydecks, L., and Tockner, K. (2015). A global boom in hydropower dam construction. Aquat. Sci. 77, 161–170. doi: 10.1007/s00027-014-0377-0
Zeilhofer, P., and Moura, R. M. (2009). Hydrological changes in the northern Pantanal caused by the Manso dam: impact analysis and suggestions for mitigation. Ecol. Eng. 35, 105–117. doi: 10.1016/j.ecoleng.2008.09.011
Zhang, Z., Huang, Y., and Huang, J. (2016). Hydrologic alteration associates with dam construction in a medium-sized coastal watershed of Southeast China. Water 8:317. doi: 10.3390/w8080317
Keywords: hydrologic alteration, IHA, Upper Paraguay Basin, hydropower plants, dams, rivers, Pantanal, Brazil
Citation: Ely P, Fantin-Cruz I, Tritico HM, Girard P and Kaplan D (2020) Dam-Induced Hydrologic Alterations in the Rivers Feeding the Pantanal. Front. Environ. Sci. 8:579031. doi: 10.3389/fenvs.2020.579031
Received: 01 July 2020; Accepted: 20 November 2020;
Published: 17 December 2020.
Edited by:
Sergi Sabater, University of Girona, SpainReviewed by:
Christiane Zarfl, University of Tübingen, GermanyCopyright © 2020 Ely, Fantin-Cruz, Tritico, Girard and Kaplan. This is an open-access article distributed under the terms of the Creative Commons Attribution License (CC BY). The use, distribution or reproduction in other forums is permitted, provided the original author(s) and the copyright owner(s) are credited and that the original publication in this journal is cited, in accordance with accepted academic practice. No use, distribution or reproduction is permitted which does not comply with these terms.
*Correspondence: Pierre Girard, cGllcnJlZ2lyYXJkMTMwMUBnbWFpbC5jb20=
Disclaimer: All claims expressed in this article are solely those of the authors and do not necessarily represent those of their affiliated organizations, or those of the publisher, the editors and the reviewers. Any product that may be evaluated in this article or claim that may be made by its manufacturer is not guaranteed or endorsed by the publisher.
Research integrity at Frontiers
Learn more about the work of our research integrity team to safeguard the quality of each article we publish.