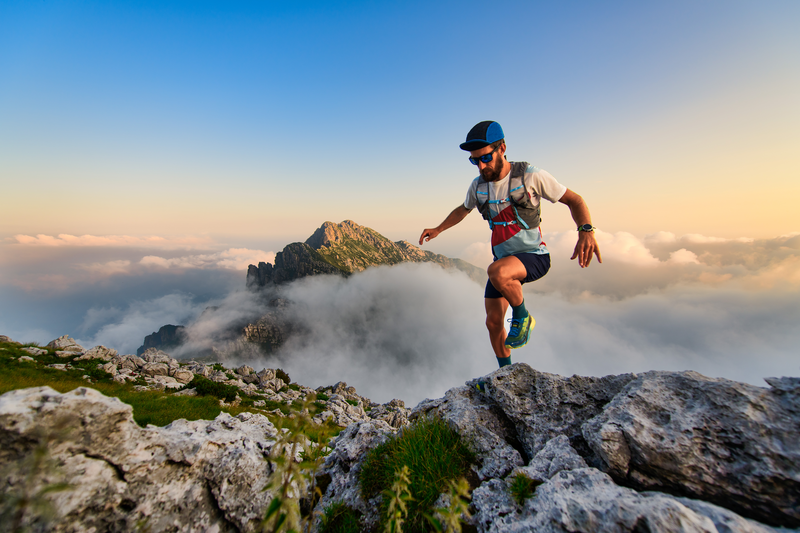
94% of researchers rate our articles as excellent or good
Learn more about the work of our research integrity team to safeguard the quality of each article we publish.
Find out more
ORIGINAL RESEARCH article
Front. Environ. Sci. , 02 September 2020
Sec. Freshwater Science
Volume 8 - 2020 | https://doi.org/10.3389/fenvs.2020.576080
This article is part of the Research Topic Advances in Biomonitoring for the Sustainability of Vulnerable African Riverine Ecosystems View all 10 articles
Studies on biomonitoring the aquatic environment using host-parasite dynamics as bio-indicators of effects and accumulators of heavy metals insults are still scarce, particularly in the tropics. In our study, we aimed at elucidating the possible use of helminth parasites of fish in monitoring and controlling heavy metal pollution. Samples were collected from an anthropogenically polluted river in north central region of Nigeria over a period of 24 months (September 2014 and October 2016). Water, fish muscle, and fish parasites samples of three dominant fish species were collected, processed, and analyzed for copper, lead, manganese, iron, zinc, and chromium. The metal concentrations in parasites of: Clarias gariepinus was in the order of Fe > Zn > Cr > Mn > Pb > Cu; Tilapia zillii was in the order of Fe > Zn > Mn > Cu > Cr > Pb; and that of Raiamas nigeriensis was in the order of Fe > Zn > Cr > Mn > Cu > Pb. The CCA ordination revealed strong relationships between fish parasites and heavy metals pollution. Generally, Fe, Zn, Mn, Cu, Cr, and Pb concentrations in the parasites of all fish species were clearly higher than those in the muscles of the fish hosts. Pb was not detected in the fish muscles of Raimas nigeriensis but was detected in the parasites of the fish, thus indicating high bioaccumulation capacity of the parasites. The close linkage between Eustrongylides sp. and zinc could mean that Eustrongylides sp. was an ideal surrogate for zinc pollution. This study revealed that intestinal helminthic parasites can be ideal surrogates for both effects and accumulation bioindication of heavy metal pollution.
Aquatic ecosystem has various sources of pollution, resulting from human activities such as industrial processes, amplified urbanization, and waste discharge (Aladaileh et al., 2020). Furthermore, processes such as weathering of rocks, human-induced emissions from mining, and other mining-related processes are ultimately likely to elevate heavy metals concentrations in water bodies, leading to increased pollution (Sankhla et al., 2016). Elevated levels of heavy metals (e.g., cadmium, lead, and mercury) are naturally found in rocks and sediments (Waheed et al., 2020). Given that a broad range of these heavy metals are persistent and are not naturally degradable, they aggregate in the sediments of streams (Sures et al., 2017). Heavy metals are biologically classified into two: the biologically essential metals (e.g., Cu, Ni, Zn and Fe) which are important for fish metabolic activities and the non-biologically essential or toxic metals (e.g., Cd, Pb and Hg) which are toxic even in trace concentrations and have no known metabolic roles in fish (Demirezen and Uruc, 2006; Mehana et al., 2020). Among the heavy metals implicated in aquatic pollution in relation to fish, lead, copper, zinc, iron, chromium, and manganese are among the most common (Afshan et al., 2014). Most of these heavy metals are essential for fish metabolism at internationally approved limits, but become very toxic when their concentrations overshoot these limits (Keke et al., 2015; Padrilah et al., 2018). Fish are distinctly in danger of heavy metal insults given that they naturally live and get nourished within the water environment, and as such exhibit certain limitations in avoiding the hazardous effects of heavy metals pollution (Ahmed et al., 2020). Fish ultimately absorb heavy metals directly from water by means of their skin and gills as well as through the intake of food that is polluted with heavy metal contaminants (Vidal-Martínez and Wunderlich, 2017; Hassan et al., 2018). Consequently, heavy metals penetrate the bloodstream of fish and bioaccumulate in their tissues or organs. The bioaccumulated concentrations of heavy metals undergo biological transformations and are either passed out by egestion or are consumed by man, with resultant deleterious outcomes on fish as well as fish consumers (Vidal-Martínez and Wunderlich, 2017), hence the consequence of the resultant deleterious effects of heavy metals on fish can be used in biomonitoring freshwater ecosystems. Among the organs of fish impacted by heavy metals, the muscle is usually preferred given its human-health deductions via consumption (Hassan et al., 2018), and its biomonitoring significance.
Biomonitoring is the use of biological indicators (bioindicators) i.e., aquatic organisms to determine the impact level of human influences on the ecological balance of aquatic ecosystems (Edegbene et al., 2020). Bioindicators are organisms whose presence, absence, or physiological conditions are indicative of environmental quality (Sures, 2003; Arimoro and Keke, 2017). They can either be effect indicators or accumulation indicators. Effect bioindicators reflect alterations in the physiology, molecules, functions, or number of organisms; while accumulation bioindicators (sentinels) are able to effectively accumulate materials from the surroundings to concentrations that are appreciably higher than those in the surroundings without manifesting deleterious outcomes (Sures, 2003; Tellez and Merchant, 2015).
Historically, the assessment of water quality using bioindicator organisms has been through the use of free-living biota such as fish, macroinvertebrates, and plankton (Ortega-Álvarez and MacGregor-Fors, 2011; Tweedley et al., 2014; Keke et al., 2017). Nevertheless, the use of free-living biota as bioindicators is characterized by several constraints; for example: (1) the sampling processes are sophisticated and require loads of funds; (2) sampling routine requires considerable amounts of samples to make any meaningful inference; (3) the composition of the organisms is affected by seasonal and temporal dynamics; (4) the large-scale heterogeneity in interpreting results occasioned by the use of sophisticated analytic tools and methods (Wright, 2010; Vidal-Martínez and Wunderlich, 2017).
Currently, emphasis is shifting to the use of parasites as both effect indicators and accumulation indicators, given that they respond differently to a variety of pollutants in the environment (Vidal-Martínez et al., 2014; Vidal-Martínez and Wunderlich, 2017; Hassan et al., 2018; Al-Hasawi, 2019; Mehana et al., 2020). Helminth parasites of fish (e.g., trematodes, nematodes, cestodes, and acanthocephalans) have been employed as ideal tools for biomonitoring of heavy metal insults in aquatic ecosystem studies (Bush et al., 2001; Sures, 2003; Hassan et al., 2018; Al-Hasawi, 2019). Some of the advantages of using parasites as bioindicators of effects and accumulation include, but not limited to, the following: (1) they do not have complex species richness, particularly in benthic freshwater ecosystem; (2) their taxonomic attributes and life-history are very familiar; (3) each individual host forms a sampling domain with its own group of parasite taxa (Kuris, 1980); (4) given that parasites of high-level predators are regarded as high-level consumers, they inevitably represent ideal indicators of trophic level accumulation (Tellez and Merchant, 2015; Vidal-Martínez and Wunderlich, 2017).
Studies on bioremediating the aquatic environment using host-parasite dynamics as bio-indicators of effects and accumulators of heavy metals insults are still scarce, particularly in the tropics. Due to the strategic location of Chanchaga River, it is believed to be contaminated with heavy metals largely from mining, agriculture, construction works, fishing, domestic and industrial wastes from water processing for municipal supplies (Amadi et al., 2012; Edegbene et al., 2015; Mgbemena et al., 2020). Anthropogenic emissions from crude and artisanal mining activities and metal fetching from underground that are overwhelmingly on the increase around the river would consequently lead to further increase in heavy metals pollution of the river. This present study fills an important gap in the knowledge of the ecotoxicology field by evaluating the possible use of parasites as bio-monitors of heavy metals pollution.
A total of 195 samples of the most dominant species of fish (72 Claris gariepinus, 58 Tilapia zillii and 65 Raiamas nigeriensis) in Chanchaga River were caught by the use of a trawling net over a period of 24 months (September 2014 and October 2016). Chanchaga River lies between latitude 8043′N to 9040′N and longitude 6012′E to 6047′E of the equator (Figure 1; Edegbene et al., 2015). Chanchaga River is massively polluted given various forms of human-influenced activities ranging from gold mining, crop farming and the presence of Niger State Water Board Authority along the river course (Amadi et al., 2012; Edegbene et al., 2019). Samples of water and fish were collected monthly for 24 months from four (4) different locations along the river (named Stations 1 to 4) based on contrasting degrees of anthropogenic disturbances.
Figure 1. Map of the study area showing the sampling stations, major towns. Source: Remote sensing laboratory, Department of Geography, Federal University of Technology, Minna.
This station is located at Zhabyala village (latitude 9040′N and longitude 6046′E). Human activities are numerous and most intense in this station when compared to other stations. Some of the obvious human activities include welding processes as well as their related discharges, washing of motorcycles and vehicles, fishing by folks, agricultural processing, wood-fetching and cuttings especially of baboon tress, laundry, bathing alongside some small-scale industries like block-making, crude mining, and mechanic workshops. The riparian vegetation is an open vegetation because of the degree of disturbance by locals, and is characterized by mostly bamboo trees (Bambusa vulgaris) and some species of epiphytic plants.
This station is located at Tunga Waya community of Bosso Local Government Area (latitude 9035′N and longitude 6039′E). Human activities here are reduced and not as much as those of Stations 1 and 4, and consist mostly farming of a number of cash crops like melon, cowpea, okra etc., Domestic activities like bathing, washing and defecation are intermittently observed here. The vegetation here is a dense canopy cover with shrubs and grass dominating the surroundings given the limited disturbances by locals.
Station 3 is located in Chanchaga area of Minna town close to the Niger State Water Board which is where the name of river originates from (Latitude 9032′N and Longitude 6034′E). This site is approximately 4.23 km from Tunga Waya community. Like Station 2, human disturbances are greatly reduced here in comparison to Stations 1 and 4 with intense human disturbances. However, most activities occurring downstream include fishing, laundry, bathing, and passing of human wastes by locals. A canopy cover of mostly mango trees (Mangifera indica) is dominant in this station but the area is surrounded by moderately portioned lands. The riparian vegetation here is mostly Rubiaceae (Nauclea latifolia).
This area is called the Koropan Community (Latitude 9031′N and Longitude 6032′E). It is about 1.5 km from the Water Board site. It is a community that harbors quite a number of residential structures and because of this human activities here are relatively high when compared to Stations 2 and 3. Agriculture practices, mining and numerous domestic activities including car washing, laundry and even fishing are also carried out on daily basis near the water body, but not as intense as those of Station 1. The riparian vegetation here is quite sparse or almost absent due to the presence of dense human activities.
Samples of muscles and middle intestines were taken from each fish and kept frozen at –20°C until being processed for heavy metal analysis.
The parasites were initially pooled alongside the fish hosts according to the different stations given that the information would be employed on station-basis for Canonical correspondence analysis (CCA). Carefully dissected fish from each of the stations were examined microscopically for endo-parasites, and helminths parasites found in the intestines of the infected fish were carefully collected using a dropper and washed severally in petri-dishes containing saline solution. Parasites were identified to their taxonomic groups according to their morphological features using relevant taxonomic keys (Hoffman, 1999). Collected helminthic parasites were carefully homogenized into a composite, and kept frozen at –20°C until being processed for heavy metals analysis. Helminth parasitic pool was then made for each of the fish species prior to heavy metals analysis.
Water samples from the four stations were filtered through a 0.45 μm membrane filter and acidified with suprapure HNO3 to pH < 2, and then analyzed directly for Cu, Pb, Mn, Fe, Zn, and Cr heavy metals by Flame Atomic Absorption Spectrometry (Centre of Genetic Engineering laboratory in Federal University of Technology, Minna Nigeria), using Varian 5-AAS analytic Jena Spectrometer.
Fish muscle samples and parasite tissue samples were analyzed according to the method prescribed by Zimmermann et al. (2001). Firstly, the frozen samples were allowed to thaw, after which 150 mg by wet weight of the homogenized fish tissues or 50 mg of parasites were transferred to a 150 mL perfluoralkoxy (PFA) vessel. A solution containing a mixture of 2 mL HNO3 (65%, suprapure) and 2.5 mL H2O2 (30%, suprapure) was added to the vessel and heated for 90 minutes at approximately 170°C in a microwave digestion system (CEM GmbH, Kamp-Lintfort, Germany; Model MDS-2000). As soon as digestion was over, the resulting solution was diluted to 5 mL with high-quality deionized water in a volumetric glass flask, and then analyzed for heavy metals (Cu, Pb, Mn, Fe, Zn, and Cr) by Flame Atomic Absorption Spectrometry (Centre of Genetic Engineering laboratory in Federal University of Technology, Minna Nigeria), using Varian 5-AAS analytic Jena Spectrometer. The optimization of the flame wavelength as well as the sample aspiration rate was performed in line with recommendations from manufacturers. The calibration was performed using four aqueous standards with analytical concentrations that are comparable to the linear response extent of the instrument, and also comprising similar concentrations of nitric acid as that of the sample. The analysis of the samples, standards, and blanks were conducted by the use three 10-s integration. The final concentration of heavy metals was achieved by preparing the reagent blank and its value was subtracted (Hassan et al., 2018).
The range, mean and standard deviation for each parameter and Station were calculated. One-way Analysis of Variance (ANOVA) at 95% level of significance was used to compare means of concentrations of heavy metals among the four collection stations. Significant ANOVAs (P < 0.05) were followed by Tukey’s post hoc HSD tests to identify differences among the means. The range, mean, standard deviation, ANOVA, and post hoc were conducted using SPSS software program (version 20). The student t test was used to evaluate significant difference between the heavy metal concentrations of the fish muscle and parasites. t test analysis was performed using PAST statistical package (Hammer et al., 2001).
The bioaccumulation factor (BAF) was calculated for each species of fish with parasites as recommended by Sures et al., 1999 as the ratio of metal concentration in the parasite and the host tissue (C[parasite]/C[host tissue]).
Canonical correspondence analysis (CCA) was used to evaluate the relationships between the parasites abundance (composition) and the heavy metals. CCA was conducted using PAST statistical package (Hammer et al., 2001). Prior to CCA, the test of assumptions of unimodality computed using DCA returned a gradient length of >3.0 (ter Braak, 1995), therefore we performed the ordination using CCA. CCA is a robust mechanism for unraveling complex datasets, and, being a direct gradient analysis, it enables combined analysis of both taxa and environmental data (ter Braak and Smilauer, 2002). The correlation coefficients between the species and the environment provided an estimate of the description of community pattern by individual environmental variables. The significance of the first three canonical axes was tested using a Monte Carlo permutation test with 999 permutations (Jckel, 1986).
The summary of heavy metals concentration from the four study stations in Chanchaga River is shown in Table 1. These values are compared with standard values of guidelines of USEPA (2010) for environmental health. As shown in this table, the heavy metals concentrations of the four stations were significantly different (P < 0.05) among the stations. The concentrations of Cu, Zn, and Cr were significantly highest at Station 1 while Pb concentration was significantly highest in Station 4. Stations 2, 3 and 4 were generally more similar in terms of heavy metals concentrations. The comparison with USEPA (2010) guideline indicated clear pollution of the river along the stations as Pb, Mn, and Fe were well above the safe limit of USEPA (2010); but Cr, Cu, and Zn values were either lower or equal to the safe limit.
Table 1. Summary of heavy metals (mg L–1) concentrations of water in Chanchaga River study stations.
The result of fish species collected from each station is presented in Table 2. The lowest numbers of Clarias gariepinus and Tilapia zillii were collected from Station 1 while the lowest number of Raimas nigeriensis was collected from Station 4. Station 2 contributed the overall highest number of individuals (65) followed by Station 3 with 54, while the lowest number of fishes were collected from Station 1 with 36 individuals. A total of 152 (77.95%) of the 195 different fish species examined were infected with various helminthic parasites. In terms of percentage infection rate by species, 80.6% (58 out of 72 individuals), 74.1% (43 out of 58 individuals), and 78.5% (51 out of 65 individuals) were recorded for Clarias gariepinus, Tilapia zillii, and Raimas nigeriensis, respectively. The distribution of the infected fishes as per stations was 44, 41, 32.
A total of 1,310 parasites were encountered and identified, and the majority of these parasites were made up of the class Nematodae, which accounted for 97.3% (1274 parasites) of the entire endo-parasites. Other parasites that made up the remaining 2.7% included the classes Monogenea (Dactylogyrus sp.), Cestoda (Polyonchobothrium clarias), and Acanthocephala (Acanthocephalus sp.), and they contributed only 36 endo-parasites across the study stations. The class Nematoda was represented by Camallanus sp., Capillaria sp., Eustrongylides sp., Cucullanus sp. and Alvinocaris markensis.
The CCA ordination revealed strong degrees of relationships between fish parasites abundance and measured heavy metals. The first two canonical axes accounted for as considerable as 92.62% of the variation in the parasite data set. An unrestricted Monte Carlo permutation test indicated that the first three canonical axes were significant (P < 0.05). As revealed by the convex hulls of the CCA, most of the heavy metals (Pb, Zn, Fe, and Cu) and majority of the parasites were associated to Station 1 that had the highest concentrations of the heavy metals sampled across the stations (Figure 2, Tables 1, 3). Axis 1, which was strongly associated with most of the parasites, was mostly explained by Pb, Mn, and Fe. Samples from Station 1 were positioned on the left, close to the center point of the plot. Most of the samples taken from Stations 4 were positioned on the left, whereas those from Stations 2 and 3 were on the right. Axis 2 of the CCA plot was associated mainly with Cu and Mn. Alvinocaris markensis was particularly associated with station 2. Station 3 had no very close relationship with majority of the fish parasites. Conversely, many of the fish parasites were found to have very close relationship with station 1 with the highest concentrations of these heavy metals. Similarly, from the CCA ordination plot, species such as Capillaria sp., Acantocephalo sp., Dactylogyrus sp., Camallanus sp., Eustrongylides sp., and Cucullanus sp were characteristic indicators of the highest concentrations of heavy metals at station 1. These species were closely associated with increased heavy metal. The heavy metal, Zn has the closest relationship with the prevalence and abundance of these fish parasites and seemed a perfect indicator for most of the heavy metal insults. In specifics, the fish parasite, Eustrongylides sp., is heavily linked to Zn pollution.
Figure 2. Canonical Correspondence Analysis (CCA) ordination plots of Pb, Cu, Mn, Zn, Fe, and Cr association with helminths parasites metrics of the fish samples collected in Chanchaga River.
Table 3. Correlations of importance of the heavy metals in the prevalence of the parasites with the first three axes of canonical correspondence analysis (CCA) in Chanchaga River, Niger State.
The heavy metal concentrations in fish muscle and parasite of Clarias gariepinus, Tilapia zillii, and Raimas nigeriensis are shown in Table 4. Generally, Fe, Zn, Mn, Cu, Cr, and Pb concentrations in the parasites of all fishes were clearly higher than those in the muscles of the fish hosts. However, no significant relationships (P < 0.05) were found between the heavy metals of the parasites and those of the muscles of the fish hosts. The metal concentrations in parasites of: Clarias gariepinus was in the order of Fe > Zn > Cr > Mn > Pb > Cu; Tilapia zillii was in the order of Fe > Zn > Mn > Cu > Cr > Pb; and that of Raimas nigeriensis was in the order of Fe > Zn > Cr > Mn > Cu > Pb. Again, for Raimas nigeriensis, Pb was not detected in the fish muscle but was detected in the parasites, and this further showed the bioaccumulation capacity of these parasites.
Table 4. Heavy metal concentration (mg kg–1) in fish muscle and parasite of Clarias gariepinus, Tilapia zillii, and Raimas nigeriensis.
As shown in Table 5, the parasites showed relatively high abilities to accumulate heavy metals from the host fishes as the BAF of all metals of all species of fish were high (>1). The overall highest BAF (BAF = Concentration in parasite/Concentration in fish tissues) was obtained for Cu (BAF = 4.60) which presented accumulation ratio between parasites and fish muscle higher than other metals. While Cu (BAF = 4.60) showed highest BAF between parasites and Tilapia zillii, Mn (BAF = 2.80) showed highest accumulation factor between parasites and Clarias gariepinus; and Zn (BAF = 3.77) showed BAF factor between parasites and Raimas nigeriensis.
Table 5. BAF for the analyzed metals in parasites and fish tissues of Clarias gariepinus, Tilapia zillii, and Raimas nigeriensis.
Heavy metals are important and acute markers of both fish well-being and the aquatic ecosystem at large (Padrilah et al., 2018). Assuredly, heavy metals being natural components of the aquatic ecosystem are important cofactor for most enzymes that are useful in fish metabolism (Jan et al., 2015). Human-mediated processes such as industrial, mining, and agricultural processes have eventually magnified their concentrations beyond recommended safe limits; with adverse consequences on human health given that the fish consumed by man have the capacity to bioaccumulate these heavy metals (Mehana et al., 2020). In our present study, the heavy metals concentrations revealed obvious pollution of Chanchaga River along the sampled stations – especially at Station 1 with the highest heavy metal values. The increased concentrations of heavy metals in Station 1 with the lowest number of fishes sampled among stations may not be unconnected with the heightened human disturbances in this station occasioned by the presence of artisanal miners, welding works, agricultural activities, among others from locals at this station. Apart from Cr, Cu, and Zn the values of all other heavy metals measured in this present study (Pb, Mn, and Fe) were well above the recommended safe limits of USEPA (2010) for environmental health and this could be attributed to a wide range of anthropogenic activities within the river, being a municipal stream. The consequences of this polluted status of the river is the impaired use of the water and its resources by both man and other aquatic biota – especially fish loss and eventual human-health implications. This could therefore be important in aquatic biomonitoring exercise as pollution effect indicators. Similarly, concentrations of As, Mn, Zn, Cu, Cr, Pb, Cd, and Fe above recommended safe limits have been reported recently in Chad Bath region, Jeddah coast, and elsewhere as ideal effect indicators of fish ecosystem degradation attributed to shipping industry, agricultural discharges, mining and other anthropogenic activities (Oumar et al., 2018; Rajeshkumar and Li, 2018; Mehana et al., 2020). Furthermore, the study of Kim et al. (2018) had reported higher concentrations of heavy metals in fish-based meals than its poultry-based counterpart.
The CCA ordination also showed that the fish parasites were significantly related with the heavy metals of Chanchaga River along the different degrees of pollution. Most of the measured heavy metals (Cu, Mn, Zn and Cr) were highest at Station 1. The combination of these variables might be used to identify and describe the multiple-scale stressor. The correlation of many individual heavy metals with the axes were relatively high for CCA but were not statistically significant. Perhaps, these estimated significances may be the results of the unmeasured heavy metals. Station 1 had very close relationship with majority of the fish parasites. The dominance of most parasites (Capillaria sp, Acantocephalo, Dactylogyrus sp., Camallanus sp., Eustrongylides sp., and Cucullanus sp.) at Stations 1 is indicative of the highest deteriorating biotic and overall ecological health of the river by heavy metals pollution at this station – and could be important in biomonitoring exercise. Zinc has the closest relationship with most of the fish parasites, and therefore, could be used as indicator of fish parasitic infestation. In specifics, the close linkage between Eustrongylides sp. and zinc could mean that Eustrongylides sp. was an ideal surrogate for zinc pollution, and could serve as early warning signal for possible zinc pollution of aquatic ecosystem. These helminthic parasites (Eustrongylides sp., Capillaria sp, Acantocephalo, Dactylogyrus sp., Camallanus sp., and Cucullanus sp.) have been employed as ideal effects indicators of heavy metal insults (Vidal-Martínez et al., 2014; Sures et al., 2017; Vidal-Martínez and Wunderlich, 2017; Hassan et al., 2018; Mehana et al., 2020). In like manner, Ashmawy et al., 2018 demonstrated that the elevated values of heavy metals were related to some helminthic parasites, such as Monogena, Nematodes, and Acanthocephala.
Fish parasites of the helminth group support the survival of their host amidst heavy metals pollution by accumulating higher concentrations of these heavy metals, and by that means act as metal sinks (Marcogliese et al., 2006; Eissa et al., 2012). Among the helminth group, the intestinal parasites access added metals in comparison to parasites that inhabit other body areas of the fish (Nachev et al., 2013). In the present study, the BAF for all heavy metals confirmed the high accumulation capacity of parasites given that heavy metal concentrations were higher in parasites of all fish species sampled in comparison to the fish muscles. Furthermore, the fact that Pb values were below detection limit in the muscle of Raimas nigeriensis but was found in the parasites inhabiting its intestine gives even more credence to the ability of these parasites to accumulate heavy metals from the fish body and serve as their heavy metal sink. This result also revealed the possibility of parasites accumulating a significant value of heavy metals from their fish hosts, enabling the fish hosts deal with elevated values of pollutants. Heavy metals ingested by the fish (through intestines or their gills) are transported through the blood to the fish liver, where majority of the metals are extracted to manufacture organo-metallic complexes which are conveyed with bile to either the intestine, to continue the liver-intestinal cycles, or taken out of the fish body by egestion (Sures, 2001 and 2003; Al-Hasawi, 2019; Mehana et al., 2020). Intestinal parasites do not have the capacity to manufacture their own cholesterols and fatty acids and, due to lipophilicity, they meddle in the cycle by easily ingesting the organo-metallic-bile complexes from their host, and this results to a reduction in the capacity of the fish host to accumulate metals (Al-Hasawi, 2019; Mehana et al., 2020). This could possibly suffice to define the higher concentrations of heavy metals in parasites in comparisons to the fish muscles in our study; and could also explain the reason Pb was not recorded from the muscle of Raimas nigeriensis but was found in the parasites. Our results are implicitly consistent with reports from some previous studies that helminth parasites (nematodes, cestodes, Acanthocephala) are ideal bioindicators of heavy metal insults in the aquatic ecosystem (Khaleghzadeh-Ahangar et al., 2011; Eissa et al., 2012; Mazhar et al., 2014; Tellez and Merchant, 2015; Sures et al., 2017; Vidal-Martínez and Wunderlich, 2017; Hassan et al., 2018). In the quest to achieve fast growth and development, the larva of nematodes absorbs biologically essential metals (Nachev et al., 2013; Hassan et al., 2016). The absence of digestive tract in cestodes necessitates their ability to accumulate more heavy metals relative to their hosts; and utilize the bile salts in reproduction (Hassan et al., 2018). Similarly, Acanthocephalans and nematodes have wide range of capacity to extract bile than the intestine of their host fish (Sures et al., 2017; Al-Hasawi, 2019). Bamidele and Kuton (2016) reported that intestinal nematodes accumulated more metals (Cu, Cr, Ni, Pb and Fe) than their fish host muscle (Clarias gariepinus and Parachanna obscura) in Lekki lagoon. Hassan et al. (2018) also reported that Cestoda parasites contained much more heavy metals than their fish host tissues.
To synthesize the major outcomes of this study, the result showed that intestinal helminths were able to reduce the concentrations of heavy metals in the fish tissues by accumulating them. Second, it further confirmed the possible application of helminthic parasites as early warning effect indicators of heavy metal pollution, as most of the parasites were associated to the station with the overall highest heavy metal concentrations.
The raw data supporting the conclusions of this article will be made available by the authors, without undue reservation.
The animal study was reviewed and approved by Environmental Protection Agency of Nigeria.
UK performed data analysis and wrote the manuscript. FA designed the study and wrote the manuscript. AM and IO designed the study and performed the sample collection and analysis. All authors read and approved the final manuscript.
The authors declare that the research was conducted in the absence of any commercial or financial relationships that could be construed as a potential conflict of interest.
Afshan, S., Ali, S., Ameen, U. S., Farid, M., Bharwana, S. A., Hannan, F., et al. (2014). Effect of different heavy metal pollution on fish. Res. J. Chem. Environ. Sci. 2, 74–79.
Ahmed, N. F., Sadek, K. M., Soliman, M. K., Khalil, R. H., Khafaga, A. F., Ajarem, J. S., et al. (2020). Moringa oleifera leaf extract repairs the oxidative misbalance following sub-chronic exposure to sodium fluoride in nile tilapia oreochromis niloticus. Animals 10:626. doi: 10.3390/ani10040626
Aladaileh, S. H., Khafaga, A. F., El-Hack, M. E. A., Al-Gabri, N. A., Abukhalil, M. H., Alfwuaires, M. A., et al. (2020). Spirulina platensis ameliorates the sub chronic toxicities of lead in rabbits via anti-oxidative, anti-inflammatory, and immune stimulatory properties. Sci. Total Environ. 701:134879. doi: 10.1016/j.scitotenv.2019.134879
Al-Hasawi, Z. M. (2019). Environmental Parasitology: intestinal helminth parasites of the siganid fish Siganus rivulatus as bioindicators for trace metal pollution in the Red Sea. Parasite 26:12. doi: 10.1051/parasite/2019014
Amadi, A. N., Yisa, J., Ogbonnaya, I. C., Jacob, J. O., and Alkali, Y. B. (2012). Quality evaluation of river chanchaga using metal pollution index and principal component analysis. J. Geography Geol. 4:2. doi: 10.5539/jgg.v4n2p13
Arimoro, F. O., and Keke, U. N. (2017). The intensity of human-induced impacts on the distribution and diversity of macroinvertebrates and water quality of Gbako River. North Central, Nigeria. Energy, Ecol. Environment 2, 143–154. doi: 10.1007/s40974-016-0025-8
Ashmawy, K. I., Hiekal, F. A., Abo-Akadda, S. S., and Laban, N. E. (2018). The inter-relationship of water quality parameters and fish parasite occurrence. Alex. J. Vet. Sci. 59, 97–106. doi: 10.5455/ajvs.299584
Bamidele, A., and Kuton, M. P. (2016). Parasitic diseases and heavy metal analysis in Parachanna obscura (Gunther 1861) and Clarias gariepinus (Burchell 1901) from Epe Lagoon, Lagos, Nigeria. Asian Pac. J. Trop. Dis. 6, 685–690. doi: 10.1016/s2222-1808(16)61110-6
Bush, A. O., Fernandez, J. C., Esch, G. W., and Seed, J. R. (2001). Parasitism; The diversity and Ecological of Animal Parasites. Cambridge: Cambridge University Press. doi: 10.1016/s2222-1808(16)61110-6
Demirezen, D., and Uruc, K. (2006). Comparative study of trace elements in certain fish, meat and meat products. Meat Sci. 74, 255–260. doi: 10.1016/j.meatsci.2006.03.012
Edegbene, A. O., Arimoro, F. O., Odoh, O., and Ogidiaka, E. (2015). Effects of anthropogenicity on the composition and diversity of aquatic insects of a municipal river in North Central Nigeria. Biosci. Res. Todays World 1, 55–66.
Edegbene, A. O., Arimoro, F. O., and Odume, O. N. (2019). Developing and applying a macroinvertebrate-based multimetric index for urban rivers in the Niger Delta. Nigeria. Ecol. Evol. 9, 12869–12885. doi: 10.1002/ece3.5769
Edegbene, A. O., Arimoro, F. O., and Odume, O. N. (2020). Exploring the distribution patterns of macroinvertebrate signature traits and ecological preferences and their responses to urban and agricultural pollution in selected rivers in the Niger Delta ecoregion. Nigeria. Aquatic Ecol. 54, 553–573. doi: 10.1007/s10452-020-09759-9
Eissa, I. A. M., Gehan, I. S., Wafeek, M., and Nashwa, A. S. (2012). Bioremediation for heavy metals in some Red Sea fishes in Suez, Egypt. SCVMJ 17, 341–356.
Hammer, Ø, Harper, D. A. T., and Ryan, P. D. (2001). PAST: paleontological statistics software package for education and data analysis. Palaeontologia Electronica 4:9.
Hassan, A., Al-Zanbagi, N., and Al-Nabati, E. (2016). Impact of nematode helminths on metal concentrations in the muscles of koshar fish, Epinephelus summana, in Jeddah, Saudi Arabia. J. Basic Appl. Zool. 74, 56–61. doi: 10.1016/j.jobaz.2016.09.001
Hassan, A., Moharram, S., and El Helaly, H. (2018). Role of parasitic helminths in bioremediating some heavy metal accumulation in the tissues of Lethrinus mahsena. Turk. J. Fish. Aquat. Sci. 2018, 435–443.
Hoffman, G. L. (1999). Parasite of North American Freshwater fishes. Poland. Cornell University Press.
Jan, A., Azam, M., Siddiqui, K., Ali, A., Choi, I., and Haq, Q. (2015). Heavy metals and human health: mechanistic insight into toxicity and counter defense system of antioxidants. Int. J. Mol. Sci. 16, 29592–29630. doi: 10.3390/ijms161226183
Jckel, K. (1986). Finite sample properties and asymptotic efficiency of Monte Carlo tests. J. Appl. Econometr. 14, 85–118.
Keke, U. N., Arimoro, F. O., Auta, Y. I., and Ayanwale, A. V. (2017). Temporal and spatial variability in Macroinvertebrate community structure in relation to environmental variables in Gbako River. Niger State, Nigeria. Tropical Ecol. 58, 229–240.
Keke, U. N., Arimoro, F. O., Ayanwale, A. V., and Aliyu, S. M. (2015). Physicochemical parameters and heavy metals content of surface water in downstream Kaduna River, Zungeru, Niger state, Nigeria. Appl. Sci. Res. J. 3, 46–57.
Khaleghzadeh-Ahangar, H., Malek, M., and McKenzie, K. (2011). The parasitic nematodes Hysterothylacium sp. type MB larvae as bioindicators of lead and cadmium: a comparative study of parasite and host tissues. Parasitology 138, 1400–1405. doi: 10.1017/s0031182011000977
Kim, H. T., Loftus, J. P., Mann, S., and Wakshlag, J. J. (2018). Evaluation of arsenic, cadmium, lead and mercury contamination in over-the-counter available dry dog foods with different animal ingredients (Red Meat, Poultry, and Fish). Front. Vet. Sci. 5:264. doi: 10.3389/fvets.2018.00264
Marcogliese, D. J., Gendron, A. D., Plante, C., Fournier, M., and Cyr, D. (2006). Parasites of potential shiners (Notropis hudsonius) in the St. Lawrence River: effects of municipal effluents and habitat. Can. J. Zool. 84, 1461–1481. doi: 10.1139/z06-088
Mazhar, R., Shazili, N. A., and Harrison, F. S. (2014). Comparative study of the metal accumulation in Hysterothalycium reliquens (nematode) and Paraphilometroides nemipteri (nematode) as compared with their doubly infected host, Nemipterus peronii (Notched threadfin bream). Parasitol. Res. 113, 3737–3743. doi: 10.1007/s00436-014-4039-x
Mehana, E. E., Khafaga, A. F., Elblehi, S. S., Abd El-Hack, M. E., Naiel, M. A., Bin-Jumah, M., et al. (2020). Biomonitoring of heavy metal pollution using acanthocephalans parasite in ecosystem: an updated overview. Animals 10:811. doi: 10.3390/ani10050811
Mgbemena, A., Arimoro, F. O., Omalu, I. C. J., and Keke, U. N. (2020). Prevalence of helminth parasites of Clarias gariepinus and Tilapia zillii in relation to age and sex in an afrotropical stream. Egyptian J. Aquatic Biol. Fish. 24, 1–11. doi: 10.21608/ejabf.2020.102364
Nachev, M., Schertzinger, G., and Sures, B. (2013). Comparison of the metal accumulation capacity between the acanthocephalan Pomphorhynchus laevis and larval nematodes of the genus Eustrongylides sp. infecting barbel (Barbusbarbus). Paras. Vectors 6, 1–8.
Ortega-Álvarez, R., and MacGregor-Fors, I. (2011). Dusting-off the file: a review of knowledge on urban ornithology in Latin America. Landsc. Urban Plann. 101, 1–10. doi: 10.1016/j.landurbplan.2010.12.020
Oumar, D. A., Flibert, G., Tidjani, A., Rirabe, N., Patcha, M., Bakary, T., et al. (2018). Risks assessments of heavy metals bioaccumulation inwater and tilapia nilotica fish from maguite island of fitri lake. Curr. J. Appl. Sci. Technol. 26, 1–9. doi: 10.9734/cjast/2018/39384
Padrilah, S. N., Shukor, M. Y. A., Yasid, N. A., Ahmad, S. A., Sabullah, M. K., and Shamaan, N. A. (2018). Toxicity effects of fish histopathology on copper accumulation. Pertanika J. Trop. Agric. Sci. 2018, 519–540.
Rajeshkumar, S., and Li, X. (2018). Bioaccumulation of heavy metals in fish species from the Meiliang Bay, Taihu Lake, China. Toxicol. Rep. 5, 288–295. doi: 10.1016/j.toxrep.2018.01.007
Sankhla, M. S., Kumari, M., Nandan, M., Kumar, R., and Agrawal, P. (2016). Heavy metals contamination in water and their hazardous effect on human health—A review. Int. J. Curr. Microbiol. Appl. Sci. 5, 759–766. doi: 10.20546/ijcmas.2016.510.082
Sures, B. (2001). The use of fish parasites as bioindicators of heavy metals in aquatic ecosystems: a review. Aquat. Ecol. 35, 245–255.
Sures, B. (2003). Accumulation of heavy metals by intestinal helminths in fish: an overview and perspective. Parasitology 126, 53–60.
Sures, B., Nachev, M., Selbach, C., David, J., and Marcogliese, D. J. (2017). Parasite responses to pollution: what we know and where we go in ‘Environmental Parasitology’. Paras. Vectors 10:65.
Sures, B., Siddall, R., and Taraschewski, H. (1999). Parasites as accumulation indicators of heavy metal pollution. Parasitol. Today 15, 16–21. doi: 10.1016/s0169-4758(98)01358-1
Tellez, M., and Merchant, M. (2015). Biomonitoring heavy metal pollution using an aquatic apex predator, the American alligator, and its parasites. PLoS One 10:e0142522. doi: 10.1371/journal.pone.0142522
ter Braak, C. J. F. (1995). “Ordination,” in Data Analysis in Community and Landscape Ecology, eds R. H. G. Jongman, C. J. F. ter Braak, and O. F. R. van Tongeren (Cambridge: Cambridge University Press), 346–399.
ter Braak, C. J. K., and Smilauer, P. (2002). CANOCO Reference Manual and Canoco Draw for Windows User’s Guide: Software for Canonical Community Ordination (Version 4.5). New York, NY: Microcomputer Power, Ithaca.
Tweedley, J. R., Warwick, R. M., Clarke, K. R., and Potter, I. C. (2014). Family-level AMBI is valid for use in the north-eastern Atlantic but not for assessing the health of microtidal Australian estuaries. Estuar. Coast. Shelf Sci. 141, 85–96. doi: 10.1016/j.ecss.2014.03.002
USEPA (2010). Lists of Contaminants and Their Maximum Contaminant Levels (MCLs). Washington, DC: USEPA.
Vidal-Martínez, V. M., Pal, P., Aguirre-Macedo, M. L., May Tec, A. L., and Lewis, J. W. (2014). Temporal variation in the dispersion patterns of metazoan parasites of a coastal fish species from the Gulf of Mexico. J. Helminthol. 88, 112–122. doi: 10.1017/s0022149x12000843
Vidal-Martínez, V. M., and Wunderlich, A. C. (2017). Parasites as bioindicators of environmental degradation in Latin America: a meta-analysis. J. Helminthol. 91, 165–173. doi: 10.1017/S0022149X16000432
Waheed, R., El Asely, A. M., Bakery, H., El-Shawarby, R., Abuo-Salem, M., Abdel-Aleem, N., et al. (2020). Thermal stress accelerates mercury chloride toxicity in Oreochromis niloticus viaup-regulation of mercury bioaccumulation and HSP70 mRNA expression. Sci. Total Environ. 718:137326. doi: 10.1016/j.scitotenv.2020.137326
Wright, J. (2010). Biomonitoring with Aquatic Benthic Macroinvertebrates in Southern Costa Rica in Support of Community Based Watershed Monitoring. M.Sc. thesis, York University, Toronto, ON.
Zimmermann, S., Menzel, C., Berner, Z., Eckhardt, J. D., Stüben, D., et al. (2001). Trace analysis of platinum in biological samples: a comparison between high resolution inductively coupled plasma mass spectrometry (HR-ICP-MS) following microwave digestion and adsorptive cathodic stripping voltammetry (ACSV) after high pressure ashing. Analytica Chimica Acta 439, 203–209. doi: 10.1016/s0003-2670(01)01041-8
Keywords: aquatic pollution, bioaccumulation, bioindication, biomonitoring, ecotoxicology, heavy metals, parasites
Citation: Keke UN, Mgbemena AS, Arimoro FO and Omalu ICJ (2020) Biomonitoring of Effects and Accumulations of Heavy Metals Insults Using Some Helminth Parasites of Fish as Bio-Indicators in an Afrotropical Stream. Front. Environ. Sci. 8:576080. doi: 10.3389/fenvs.2020.576080
Received: 25 June 2020; Accepted: 14 August 2020;
Published: 02 September 2020.
Edited by:
Vinicius Fortes Farjalla, Federal University of Rio de Janeiro, BrazilReviewed by:
Daniele Kasper, Federal University of Rio de Janeiro, BrazilCopyright © 2020 Keke, Mgbemena, Arimoro and Omalu. This is an open-access article distributed under the terms of the Creative Commons Attribution License (CC BY). The use, distribution or reproduction in other forums is permitted, provided the original author(s) and the copyright owner(s) are credited and that the original publication in this journal is cited, in accordance with accepted academic practice. No use, distribution or reproduction is permitted which does not comply with these terms.
*Correspondence: Unique N. Keke, bi5rZWtlQGZ1dG1pbm5hLmVkdS5uZw==; dW5pcXVla2tuQGdtYWlsLmNvbQ==
Disclaimer: All claims expressed in this article are solely those of the authors and do not necessarily represent those of their affiliated organizations, or those of the publisher, the editors and the reviewers. Any product that may be evaluated in this article or claim that may be made by its manufacturer is not guaranteed or endorsed by the publisher.
Research integrity at Frontiers
Learn more about the work of our research integrity team to safeguard the quality of each article we publish.