- 1Department of Energy and Technology, Swedish University of Agricultural Sciences, Uppsala, Sweden
- 2Department of Earth Sciences, Uppsala University, Uppsala, Sweden
- 3Department of Forest Biomaterials and Technology/Wood Science, Swedish University of Agricultural Sciences, Uppsala, Sweden
- 4National Institute of Chemical Physics and Biophysics, Tallinn, Estonia
This study evaluated the potential of biochar impregnated with Fe3+ or Ca2+, or mixed with Polonite®, as a filter material for removal of phosphate (PO4-P) from wastewater in onsite wastewater treatment systems (OWTS). Four treatments with biochar were investigated: unimpregnated biochar (UBC), biochar impregnated with iron Fe3+ (FBC), biochar impregnated with calcium oxide (CBC), and biochar mixed with Polonite® (PBC). In a batch experiment using phosphate solution at concentrations 0.5, 3.3, 6.5, 13, and 26 mg PO4-P L–1, adsorption of PO4-P in the different treatments was modeled using Langmuir and Freundlich isotherms. Column filters (5 diameter × 55 cm height) packed with UBC, FBC, CBC, and PBC were then furnished with raw wastewater over 148 weeks. During this experiment, adsorption of PO4-P was investigated in response to increasing hydraulic loading rate (HLR; 56, 74, and 112 L m–2 day–1) and increasing phosphate loading rate (PLR; 195, 324, 653, and 1715 mg PO4-P m–2 day–1). Among the materials, FBC had the highest maximum adsorption capacity (Qm) based on Langmuir isotherms (3.21 ± 0.01 mg g–1). FBC and CBC showed robust performance with increasing HLR, while increasing PLR increased the amount of PO4-P retained in all filters. After 148 weeks of operation, removal of PO4-P (averaged over the last 18 weeks of operation) was 13 ± 16% for UBC, 40 ± 20% for CBC, 88 ± 12% for FBC, and 30 ± 18% for PBC. The PO4-P amount retained in filters over the 148 weeks was 84.75, 221.75, 358.38, and 152.36 g m–2 in UBC, CBC, FBC, and PBC, respectively. The adsorption capacity of the filters after 148 weeks was 1.50, 4.02, 6.41, and 2.75 mg g–1 for UBC, CBC, FBC, and PBC, respectively. The adsorption capacity values and breakthrough curves showed that low concentrations (i.e., <2.6 mg L–1) of PO4-P in wastewater would allow the FBC filter to remain active for 58 months and the CBC filter for 15 months, before PO4-P removal declined to <70%. In conclusion, biochar impregnated with iron and calcium is a promising solution for removal of PO4-P from wastewater in OWTS.
Introduction
Wastewater discharge and agricultural runoff are major contributors to anthropogenic phosphorus (P) discharge to many inland aquatic systems worldwide (Smith, 2003). Agricultural activities and wastewater effluents account for the majority of annual P discharge to water bodies (HELCOM, 2018; Schellenger and Hellweger, 2019). In industrialized countries (e.g., Sweden), there are stringent requirements that regulate the quality of wastewater effluents in small scale and onsite systems in order to protect water bodies, and high-technology solutions are often used for additional P removal from wastewater to meet the environmental regulations (Havs- och vattenmyndighetens, 2016). However, wastewater quality from small-scale onsite wastewater systems (OWTS) is poor in terms of P removal (Heinonen-Tanski and Matikka, 2017), although the anthropogenic P load from OWTS is still smaller than the agricultural load. However, onsite systems can still be a relevant P source, especially in areas such as the Baltic Sea Region where reducing P loads in water bodies is high priority (Heinonen-Tanski and Matikka, 2017; HELCOM, 2018).
One environmentally friendly and effective treatment method for onsite wastewater treatment is the use of biochar filters. Biochar is produced by heating biomass at high temperature (300–800°C) in limited presence of oxygen (Downie et al., 2009), and is characterized by large specific surface and high porosity. Organic materials such as agricultural waste, sewage sludge and forest residues can act as a resource for biochar production, thereby contributing to sustainable management and re-utilization of waste products (He et al., 2016). Previous studies have demonstrated the efficiency of biochar in removing organic matter, surfactants, and nitrogen (N) from wastewater and graywater (Berger, 2012; Niwagaba et al., 2014; Dalahmeh, 2016). However, removal of P by biochar was not efficient in the latter studies, and there is therefore a need to investigate whether modification of the biochar surface by impregnating with iron (Fe) and calcium (Ca) would enhance the capacity for P removal. Modification of the surface of biochar by impregnation with different cations has been investigated previously to some extent. For example, He et al. (2017) investigated nanocomposites of iron oxide (Fe3O4) biochar for removal of different pollutants, including PO4, from wastewater. They found that Fe3O4/biochar nanocomposites not only showed significantly enhanced PO43– removal (100%), but also significantly shortened the time needed for complete removal. In a study by Chen et al. (2011), biochar powder for P removal was produced at different temperatures and impregnated with magnetite (Fe2O3) with a biochar to Fe ratio of 0.9. The modified biochar showed higher P adsorption (up to 99% removal) than unmodified control biochar. Adding iron oxides to biochar can also have structural benefits, producing larger pore volume and specific surface area (Ren et al., 2015). Ferric chloride (FeCl3) biochar was studied by Li et al. (2016), who found that an Fe to biochar ratio of 0.7 resulted in P adsorption of up to 16.58 mg g–1 biochar, compared with less than 1 mg g–1 in natural sand (Del Bubba et al., 2003). In a study testing column filters with Fe-modified biochar, Liu et al. (2012) found that 99% of the total P concentration was removed. Jung et al. (2016) analyzed fine biochar material produced by algae (as dried calcium-alginate beads) and found it had a capacity to remove 100 mg P g–1 biochar. However all these studies evaluated the performance of biochar in batch experiments and P adsorption by impregnated biochar from real wastewater was not investigated. Additionally, the performance of impregnated biochar in unsaturated column filters over extended periods and under different loading conditions was not investigated.
The main objective of the present study was to evaluate the potential of biochar impregnated with Fe3+ or Ca2+, or mixed with Polonite, as filter material for adsorption of PO4-P in OWTS. Specific objectives were to (i) determine the adsorption isotherm of PO4-P in biochar impregnated with Fe, biochar impregnated with Ca, and biochar mixed with Polonite in batch adsorption experiments, and (ii) demonstrate and assess the performance of impregnated biochar filters in PO4-P removal from wastewater under different hydraulic loading rates (HLR) and phosphate loading rates (PLR) in column experiments.
Materials and Methods
Biochar Preparation
Four treatments with biochar were tested: (i) unimpregnated biochar (UBC); (ii) biochar impregnated with calcium oxide (CaO; CBC); biochar impregnated with FeCl3 (FBC) and biochar mixed with Polonite® (PBC). For treatments FBC and CBC, pine bark (Rimbo Jord, Stockholm) of grain size 1–5 mm was soaked in FeCl3 solution (purity 97%; VWR, Stockholm) and CaO (purity 95%; VWR, Stockholm), respectively, before pyrolysis. The FeCl3 or CaO to bark ratio was 0.3 on a dry matter (DM) basis. After being mixed in the solution for 24 h in room temperature, the impregnated bark was dried at 100°C for another 24 h. Finally, the dried biomass was charred in a muffle stove at 350–400°C for 3.5 h according to Agrafioti et al. (2014). UBC was produced by pyrolysis of similar pine bark without impregnation. For treatment PBC, after pyrolysis, UBC was mixed with Polonite® gravel with a Polonite/biochar DM ratio of 0.3. Polonite® is a commercially available and reactive material which is used for phosphorus adsorption. It is produced by heating opoka rock to 900°C that results in transforming CaCO3 to the more reactive CaO (Renman and Renman, 2010). The Polonite® used in the current experiment had particle size of 1–3 mm and it was obtained from© Ecofiltration Nordic AB (Stockholm, Sweden).
Batch Adsorption Experiments
A batch experiment was carried out to assess and compare the P adsorption capacity of the different biochars (UBC, FBC, CBC, and PBC). For this, 1 g samples of biochar were added to 500 mL conical flasks containing 100 mL of PO4-P solution with concentrations 0.5, 3.3, 6.5, 13, and 26 mg PO4-P L–1, respectively. The concentrations were prepared by diluting 1000 mg PO4 L–1 stock solution based on monopotassium phosphate (KH2PO4; VWR, Stockholm) with distilled water. The PO4-P concentrations were selected based on those expected in an OWTS, and diluted according to Palm et al. (2002). Three replicates (n = 3) were prepared for each concentration and each treatment except the 0.5 mg PO4P L–1 concentration, which only had one replicate (n = 1). The flasks were shaken on a rotary table for 24 h at 130 rpm and controlled room temperature of 20 ± 2°C. Samples (6 mL) were taken from each flask after 0 min, 15 min, 75 min, 4 h, and 24 h, using a pipette. Collected samples were filtered through a 0.45 μm filter and their pH and PO4-P concentration determined. After 24 h, the residual solids were washed with deionized water, oven-dried at 80°C for 4 h, and stored in the freezer for further characterization.
Column Filter Experiments
In the column filter experiments, acrylic columns were used as filters to study the removal of chemical oxygen demand (COD), and PO4-P from wastewater. The columns (60 cm high, 5 cm diameter) were packed separately with biochar (UBC, PBC, CBC, or FBC) to form a layer of 50 cm. Single columns were prepared for each material. A 5 cm layer of coarse biochar (8–15 mm in particle size) was placed on the top and bottom of the filters to prevent clogging and to facilitate drainage (Figure 1).
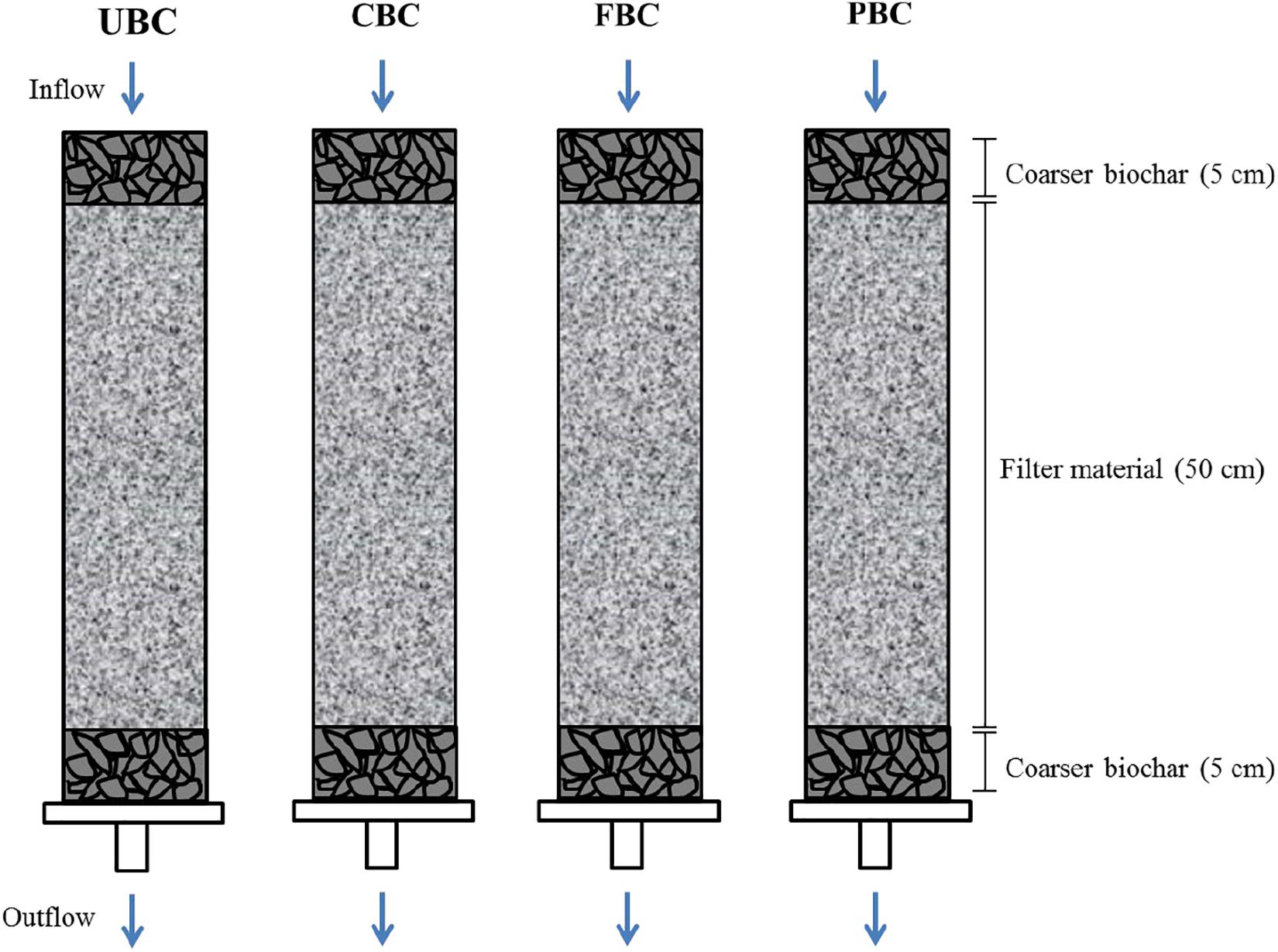
Figure 1. Set-up used for the biochar column experiment [unimpregnated biochar (UBC), calcium oxide impregnated biochar (CBC), ferric chloride impregnated biochar (FBC), and biochar mixed with Polonite® (PBC)].
Loading Conditions for the Column Filters
The column filters were tested for removal of PO4-P over an operating period from October 2016 to August 2019. During this period, the performance of filters in terms of pollutant removal was investigated in response to different HLR and PLR, as described below.
Hydraulic Loading Rate Trial
The removal of PO4-P and COD from wastewater was investigated in response to changing hydraulic loading conditions. During this trial, the filters were operated with an HLR of 56 L m–2 day–1 for 33 weeks (including the start-up period), 74 L m–2 day–1 for a further 30 weeks, and 112 L m–2 day–1 for 10 weeks. The filters were fed with wastewater at the planned HLR, without modifying the concentrations of organic matter, N, or P of the wastewater. The concentrations of PO4-P and the organic loading rate (OLR) and PLR applied during the HLR trial are summarized in Table 1.
Phosphate Loading Rate Trial
The removal of PO4-P and COD in response to changing PLR was investigated by exposing the filters to stepwise increasing PLR while the HLR was kept unchanged at 74 L m–2 day–1 (Table 2). During this trial, the filters received 194 ± 65 mg PO4-P m–2 day–1 for 18 weeks, 324 ± 27 mg PO4-P m–2 day–1 for 23 weeks, 653 ± 61 mg PO4-P m–2 day–1 for 13 weeks, and 1715 ± 39 mg PO4-P m–2 day–1 for 18 weeks. The PO4-P concentration was modified to give the target PLR by adding 15, 30, 90, and 150 mL of PO4 standard solution (1000 mg L–1) to 1 L raw wastewater. The PO4-P concentration in the raw wastewater was 2.6 ± 0.86 mg L–1. Concentrations of all other components in the raw wastewater were not modified during this trial. Inflow characteristics, HLR, OLR, and PLR of the feed wastewater during this trial are summarized in Table 2.
Preparation, Storage, and Dosage of Wastewater
All column filters were fed with raw wastewater in single-pass unsaturated downflow mode. The raw wastewater was obtained from Uppsala wastewater treatment plant (Kungsängsverket) on a weekly basis. When needed (i.e., during the PLR trial), the concentration of PO4-P was changed. The wastewater was cooled in a fridge (4 ± 2°C) when brought from Kungsängsverket. Before each dosage, the cooled wastewater was mixed by homogenization and the required dosage pumped from the refrigerated container to distribution containers placed at room temperature (20°C). After reaching room temperature, the wastewater doses were pumped from the distribution containers to the filters, using a peristaltic pump.
Chemical Analysis
The inflow wastewater and effluents from the different filters were analyzed to determine the pH, electrical conductivity (EC), COD, and PO4-P. The pH was measured using a WTW pH/ion 340i meter and EC using a Condi 330i conductivity meter (WTW, Weilheim, Germany). The COD and PO4-P concentrations were determined using Spectroquant® cell kits (numbers 14772–14773 (COD) and 14543 (PO4-P), Merck KGaA, Darmstadt, Germany). Concentrations were determined colorimetrically using a Nova 60 photometer (Merck KGaA).
Characterization of Biochar
Chemical composition of surface, particle shape, topography, and functional groups on the different biochars (UBC, PBC, CBC, and FBC) were characterized using Fourier transform infrared (FTIR), X-ray diffraction (XRD), and scanning electron microscopy (SEM). Infrared absorption spectra of the biochar samples were obtained using a Spectrum Two FTIR (Perkin-Elmer) equipped with an UATR Diamond accessory, according to Jebrane et al. (2017). The XRD patterns were obtained by a PANanalytical B.V. X’Pert3 Powder diffractometer (Netherlands) with Cu-Kα radiation (k = 1.54059 Å) within angular range of 2θ = 5–70° at 25°C. SEM was used to observe the surface morphology of the impregnated biochars. Biochar samples were deposited on stubs bearing double-sided tape and coated with a ∼6 nm layer of gold using an Emitech K550X sputter coater. Samples were observed using a Philips XL30 ESEM operated at variable kV. Images were digitalized using the microscope software.
Calculations of Adsorption Isotherms
Removal efficiency (E) was calculated from the difference in concentration between inflow and outflow of the filters as:
where E is the removal efficiency (%), Cin is the concentration in the inflow (mg L–1), and Cout is the concentration in outflow (mg L–1).
Adsorption of phosphate (Q) in the batch adsorption experiment was calculated as:
where Q is mg P adsorbed per g biochar (mg g–1), C0 is the initial concentration of solution (mg L–1), Ce is the concentration (mg L–1) after 24 h in the batch equilibrium experiment, V is the volume of solution (100 mL), and m the mass of adsorbent (1 g).
Langmuir and Freundlich adsorption isotherms were modeled for each biochar type. Langmuir isotherms were calculated according to Mead (1981):
where Qe (mg g–1) is the equilibrium adsorption capacity, Ce (mg L–1) is the concentration at equilibrium, kL (L mg–1) is the Langmuir adsorption constant, and Qm (mg g–1) is the maximum adsorption capacity.
Freundlich isotherms were calculated as:
where kF (L g–1) is the Freundlich constant and n is the dimensionless Freundlich heterogeneity exponent.
Results
Characteristics of Biochar
FTIR Analysis and SEM
The FTIR spectra of FBC, CBC, PBC, and UBC are shown in Figure 2. The peaks observed in the region 1800–650 cm–1 in all spectra were attributed to carbonyl stretching vibrations and to -COOH symmetric stretching vibrations. The band observed around 780 cm–1 could be attributable to in-plane and out-of-plane C-H bends. The FeCl3-impregnated (FBC) filter (freshly prepared before use in wastewater treatment) caused only a slight change in the spectrum, mainly around 650 cm–1, where a new peak most probably attributable to iron oxalate was observed. In addition, a decrease in the peak around 1020 cm–1 was observed, probably as a result of shifting from COOH to COO-. After using the FBC filter for wastewater treatment, no significant changes were observed except disappearance of the peak at around 650 cm–1 characteristic of iron oxalate, possibly due to leaching of iron from the filters. The FTIR spectrum of the fresh CBC filter showed emergence of new characteristic absorption bands around 1410, 1315, 874, and 780 cm–1 which could be attributed to C-O and Ca-O bonds. After use of CBC for wastewater treatment, a slight decrease in the intensity of the C-O and Ca-O peaks was observed due to phosphate adsorption and interaction with the impregnated calcium oxide species. The FTIR spectrum of the fresh Polonite filter (PCB) exhibited strong absorption bands at 1410 and 870 cm–1, which are characteristic bands of carbonate anions, and other peaks around 1080, 980, 944, and 712 cm–1, which were attributed to the vibrations of wollastonite (β-CaSiO3). A small peak observed at 3640 cm–1 was attributed to water vibrations (Figure 2). After PCB was used for wastewater treatment, a significant decrease in the carbonate anion band intensity was observed, suggesting formation of phosphate complexes. For untreated biochar (UBC), there were no significant peaks in the FTIR spectra. The surface shape and topography of the UBC, CBC, FBC, and PBC materials are shown by SEM images in Supplementary Figure S1.
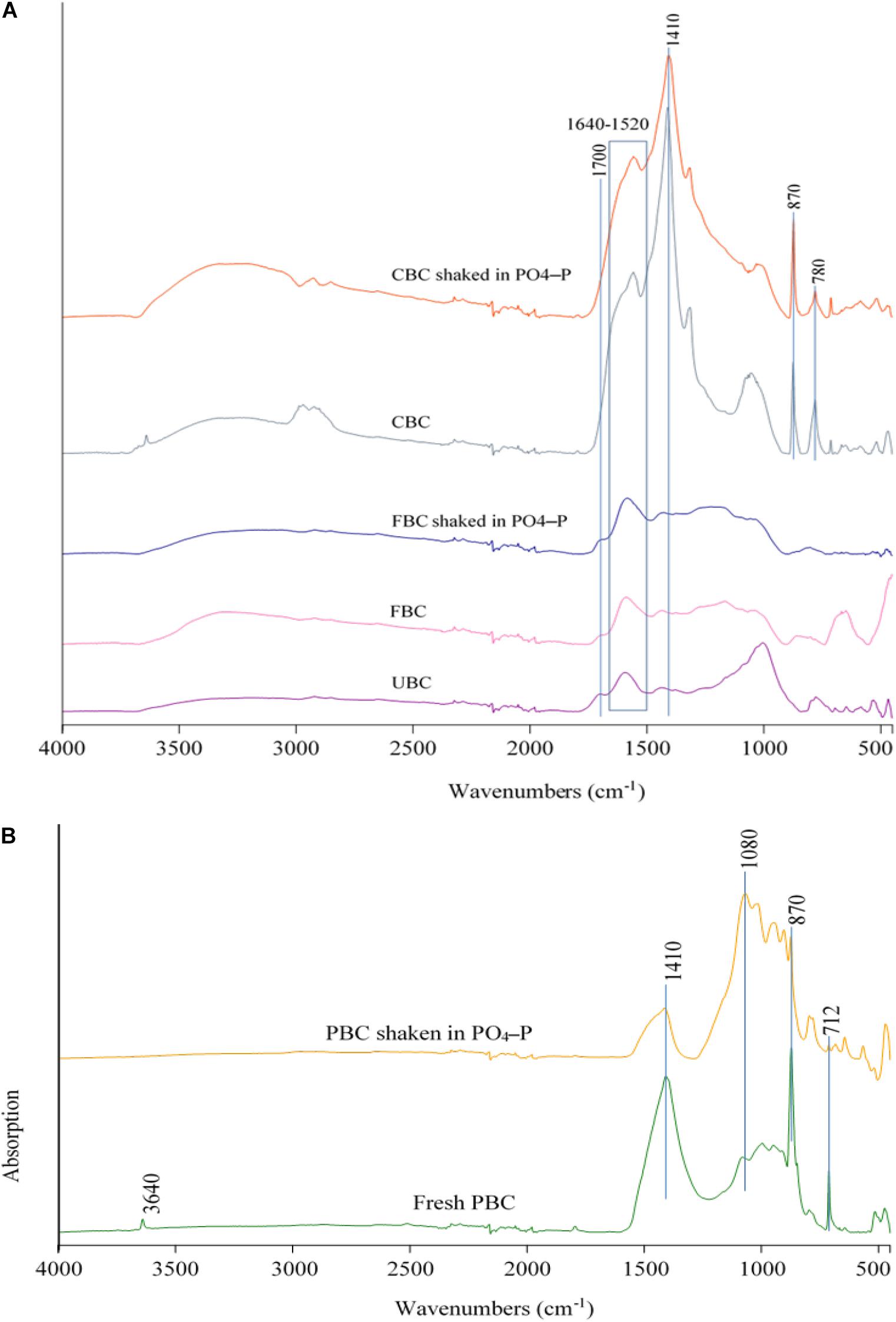
Figure 2. Fourier transform infrared (FTIR) spectra of: (A) unimpregnated biochar (UBC, purple), biochar impregnated with calcium oxide (CBC, orange), and biochar impregnated with ferric chloride (FBC, blue); and (B) biochar mixed with Polonite (PBC), fresh or shaken in PO4-P solution.
XRD Analysis
Untreated biochar contained 56% quartz, 27% albite, and 17% microcline (Table 3 and Supplementary Figures S2, S3). Impregnation of biochar with CaO resulted in a decrease in the quartz content, disappearance of microcline and albite, and formation of calcite (54%), while impregnation with FeCl3 resulted in reduction in quartz content, a slight increase in albite, and formation of akaganeite (32%). Virgin Polonite contains 52% quartz, 25% calcite, and 23% wollastonite. After using the untreated and CaO-impregnated biochar for wastewater treatment, no significant changes in the mineral content were observed. After filtration, the FeCl3-impregnated biochar showed a significant decrease in quartz content and slight decrease in akaganeite and albite, with formation of monalbite. The combination of Polonite and biochar used for Tot-P and PO4-P adsorption showed an increase in quartz content, disappearance of albite, microcline, calcite, and wollastonite, and formation of anorthite and orthoclass.
Langmuir and Freundlich Adsorption Isotherms in UBC, CBC, FBC, and PBC
FBC had a maximum adsorption capacity (Qm) according to Langmuir isotherms (3.21 ± 0.01 mg g–1) (Table 4). CBC did not reach equilibrium during the experiment. The maximum PO4-P concentration investigated for CBC was (26.7 mg L–1) and was totally adsorbed during the adsorption experiment. The Langmuir isotherms had a stronger correlation for UBC and FBC, and the Freundlich isotherms for PBC. Correlation coefficients (R2) were in the range 0.957–0.997 for Langmuir isotherms and 0.960–0.993 for Freundlich isotherms for PO4-P adsorption to the biochars (Table 4). All adsorption isotherms showed an increase in equilibrium concentration (Ce) resulted from an increase in P adsorption on the biochar surface (Qe) (Figure 3).
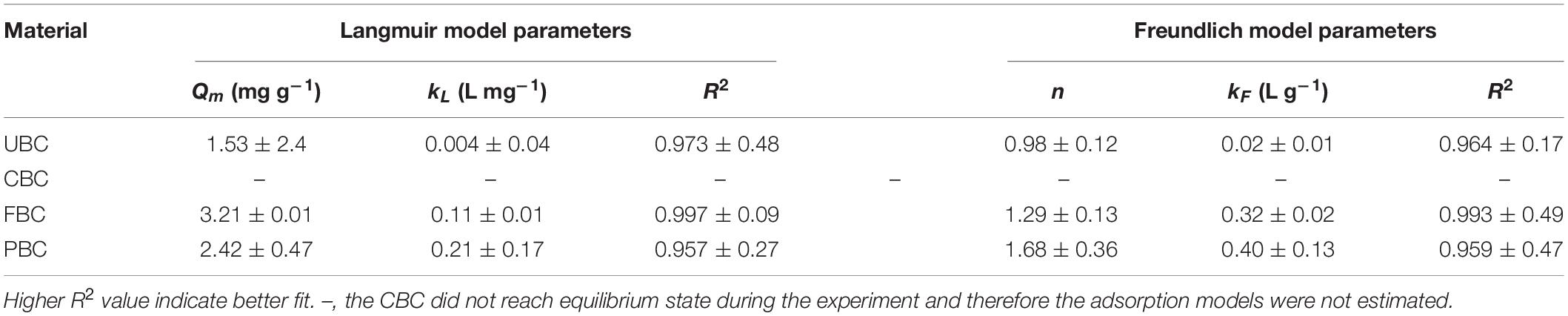
Table 4. Langmuir and Freundlich model parameters (mean ± standard deviation, n = 3) for untreated biochar (UBC), CaO-impregnated biochar (CBC), FeCl3-impregnated biochar, and untreated biochar mixed with Polonite® (PBC).
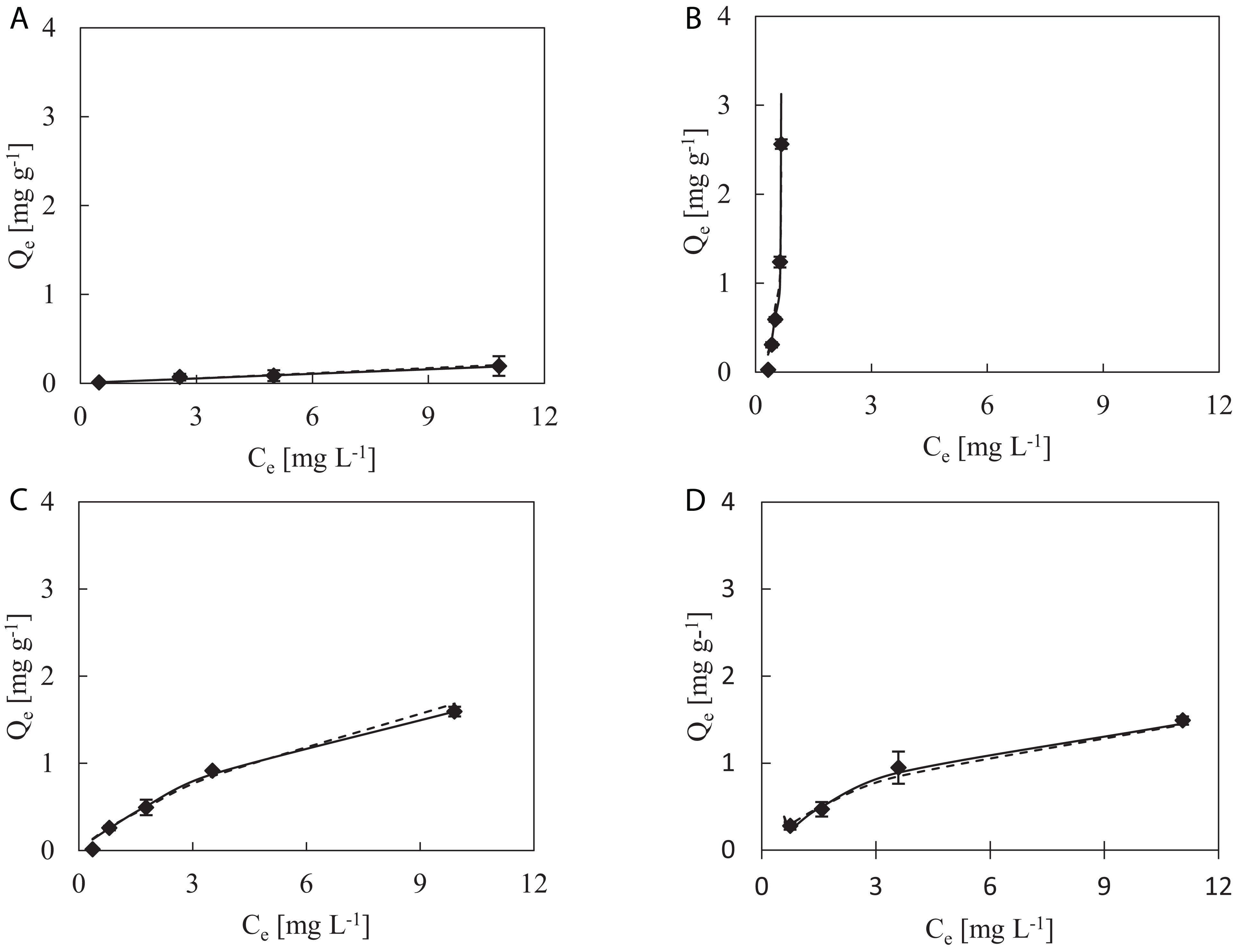
Figure 3. Equilibrium adsorption capacity (Qe; mg/g) as function of equilibrium concentration of PO4-P (Ce; mg/L) as modeled using Langmuir (——) and Freundlich (————–) isotherms, compared to the measured adsorption capacities (◆) in (A) untreated biochar (UBC), (B) CaO-impregnated biochar (CBC), (C) FeCl3-impregnated biochar, and (D) untreated biochar mixed with Polonite® (PBC). Bars indicate mean ± standard deviation, n = 3.
Adsorption Capacity of Phosphate in UBC, CBC, FBC, and PBC Filters
The PO4-P adsorption capacity was highest in FBC and lowest in UBC, with the filters following the order FBC > < cps:it > CBC < /cps:it≫ < cps:it > PBC < /cps:it≫UBC, with overall PO4-P adsorption capacity of the different filters 1.50, 4.02, 6.41, and 2.75 mg per g biochar for UBC, CBC, FBC, and PBC, respectively (Figure 4). The corresponding surface removal rate of PO4-P was 358.36, 221.75, 152.36, and 84.75 g m–2 of the filter surface, in the same order. At the end of the operation period of the filter, removal of PO4-P (averaged over the last 18 weeks of operation) was 13 ± 16% for UBC, 30 ± 18% for PBC, 88 ± 12% for FBC, and 40 ± 20% for CBC (Figure 4).
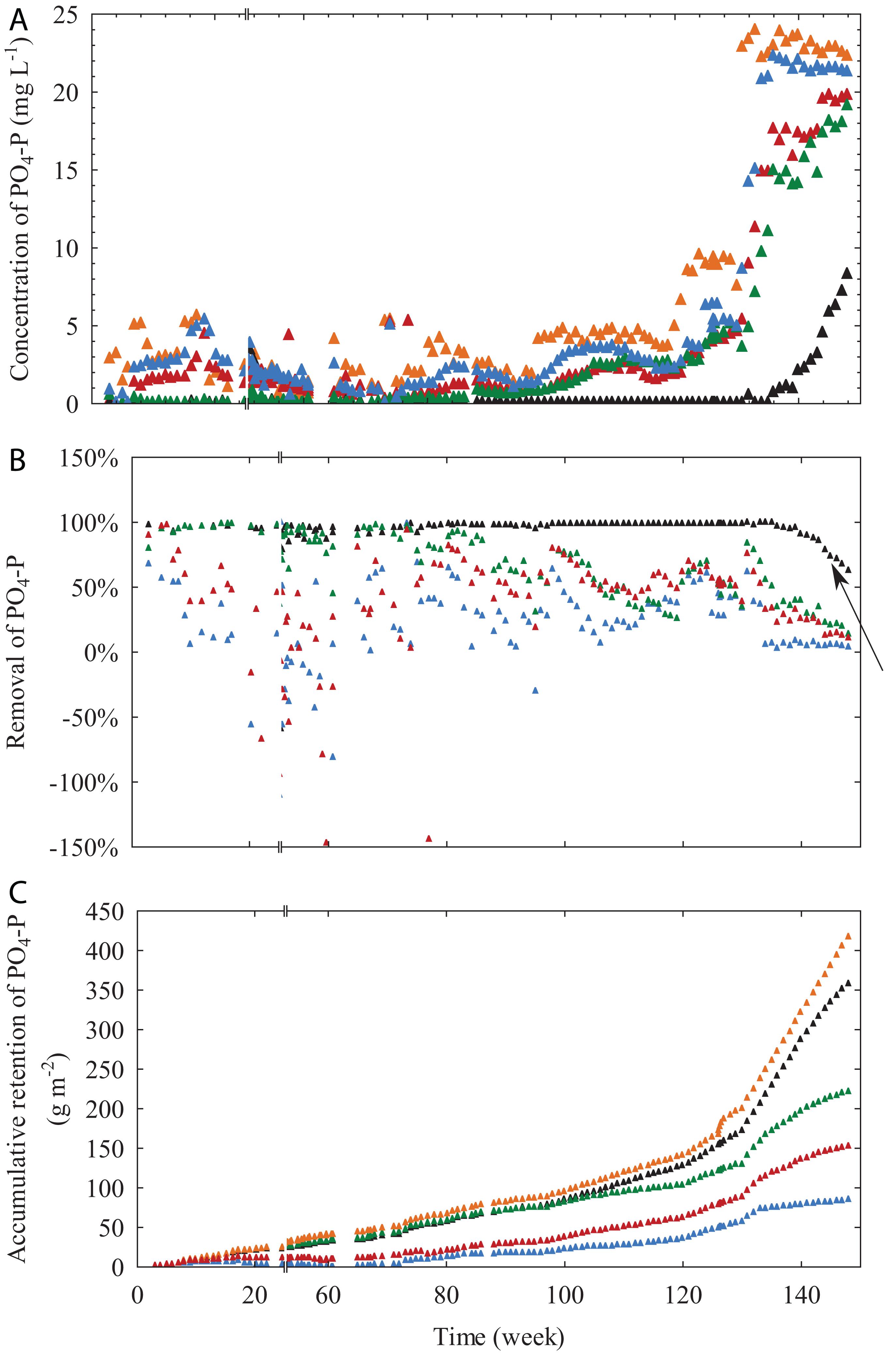
Figure 4. (A) Concentration of phosphate-phosphorus (PO4-P) in the inflow to filters, (B) removal of PO4-P in filters, and (C) cumulative amount of PO4-P retained (mg m–2) from start to end of the experiment in: unimpregnated biochar filter (UBC; blue), CaO-impregnated biochar filter (CBC; green), FeCl3-impregnated biochar filter (FBC; black), and biochar filter mixed with Polonite® (PBC; red). The orange line in panel (C) shows cumulative amount of PO4-P (g m–2) applied to the filters.
The low removal in UBC (4–6%) of PO4-P toward the end of the period indicated that UBC may have reached its ultimate PO4-P retention capacity during the experimental period (148 weeks) (Figures 4B,C). Removal of PO4-P in CBC (14–23%) and PBC (11–15%) was also low by the end of the experiment. In contrast, PO4-P removal in the FBC filter declined to almost 63% by the end of the experimental period (week 148).
Phosphate Removal in Response to Changes in Hydraulic Loading Rate
From the filters tested, FBC showed highest removal of PO4-P (96%) and was least sensitive to changes in HLR, followed by CBC (77–95% removal) (Figure 5 and Table 5). UBC was least efficient in PO4-P removal (−9 to 46%) and the least robust to changes in HLR.
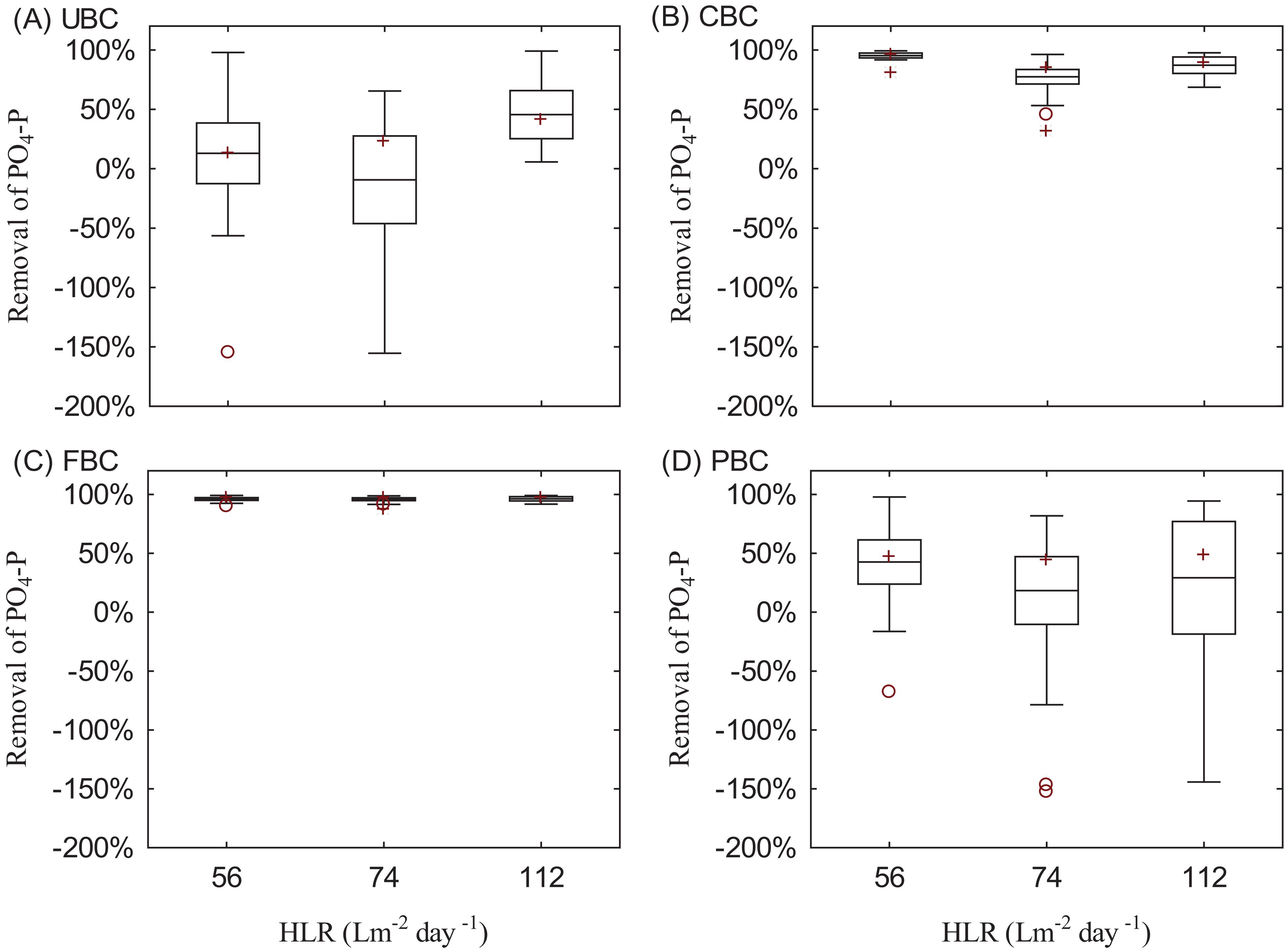
Figure 5. Boxplots showing removal of phosphate-phosphorus (PO4-P, %) in (A) unimpregnated biochar filter (UBC), (B) CaO-impregnated biochar filter (CBC), (C) FeCl3-impregnated biochar filter (FBC), and (D) biochar filter mixed with Polonite® (PBC) for different hydraulic loading rates (HLR). The middle line (–) of the box is the mean, the cross (+) is the median, the box is the confidence interval, the whiskers are the non-outlier range, and the red dots are outliers and extreme values.
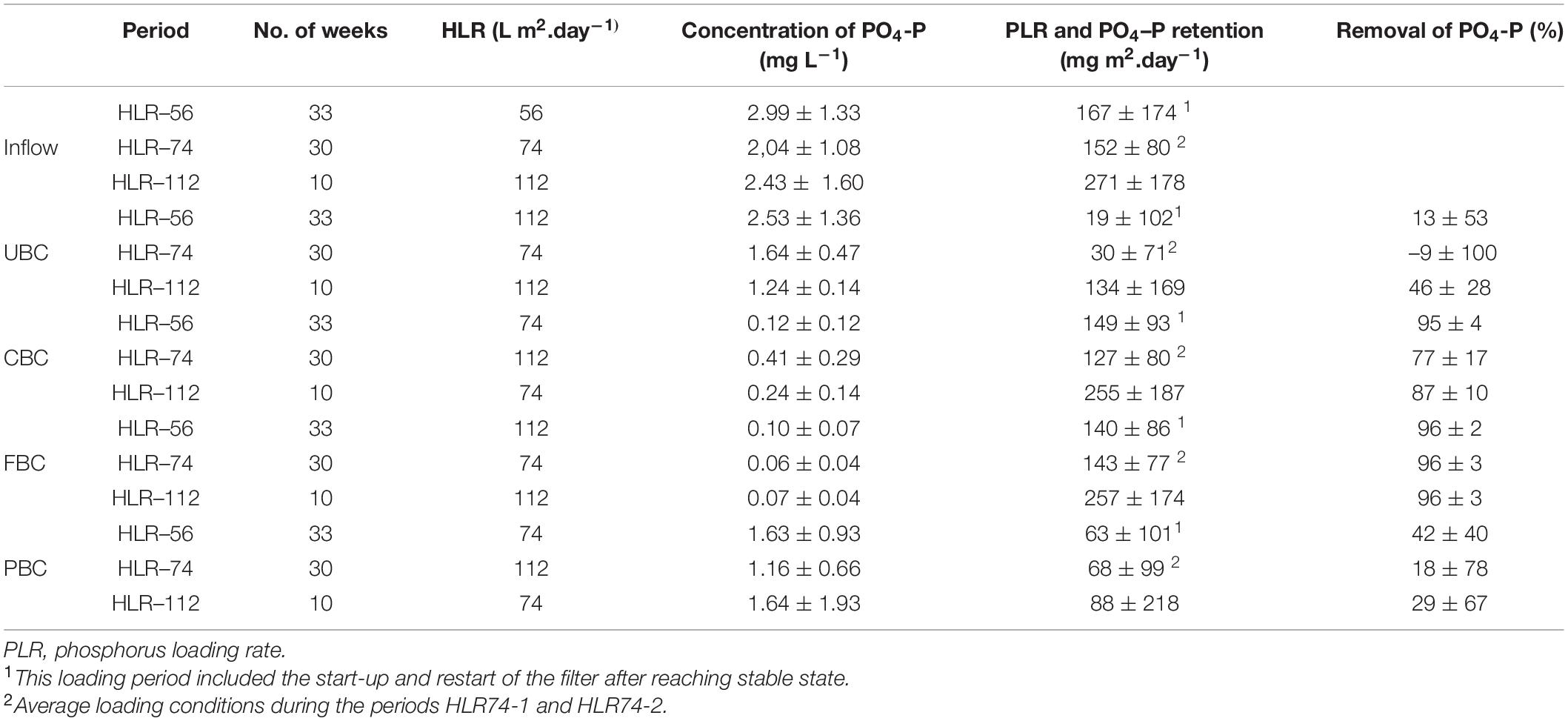
Table 5. Concentrations of phosphate-phosphorus (PO4-P) in filter inflow and outflow, PO4-P retention (mg m–2 day–1) and PO4-P removal in the unimpregnated biochar filter (UBC), CaO-impregnated biochar filter (CBC), FeCl3-impregnated biochar filter (FBC), and biochar filter mixed with Polonite® (PBC) at different hydraulic loading rates (HLR).
With the UBC filter, the average percentage PO4-P removal was lower for HLR-74 than HLR-56 and the filter performed best when HLR was 112 L m–2 day–1 (Figure 5A and Table 5). UBC showed a decline in PO4-P removal when HLR was increased from 56 to 74 L m–2 day–1 (Figure 5A). Another decline in removal in UBC (from 46 to 27%) was observed when HLR was decreased from 112 to 74 L m–2 day–1. In the CBC filter, increasing the HLR from 74 to 112 L m–2 day–1 enhanced PO4-P removal and CBC showed a decline (from 87% to 71%) in PO4-P removal when HLR decreased from 112 to 74 L m–2 day–1 (Figure 5B).
The average percentage of removal of PO4-P in the FBC filter was not influenced by HLR (96% for all HLRs) (Table 5 and Figure 5C). The trend in PO4-P removal in the PBC filter in response to changes in HLR was similar to that observed for UBC, but the levels of removal were higher in PBC (42 ± 40, 18 ± 78 and 29 ± 76% for HLR-56, HLR-74, and HLR-12, respectively) (Figure 5D and Table 5).
Response of Filters to Increasing Phosphate Loading Rate
The average amount of PO4-P retained in the media, increased as response to increase in PLR for all filters (Figure 6). Regarding PO4-P removal in UBC as function of time, intermediate removal (∼60%) was observed at the beginning of PLR-194, PLR-652, and PLR-1715, after which it then declined over time (Supplementary Figure S4A). For CBC, the average removal of PO4-P decreased from 72% at PLR-194 to ∼40% at PLR-324 and PLR-1715 (Supplementary Figure S4B).
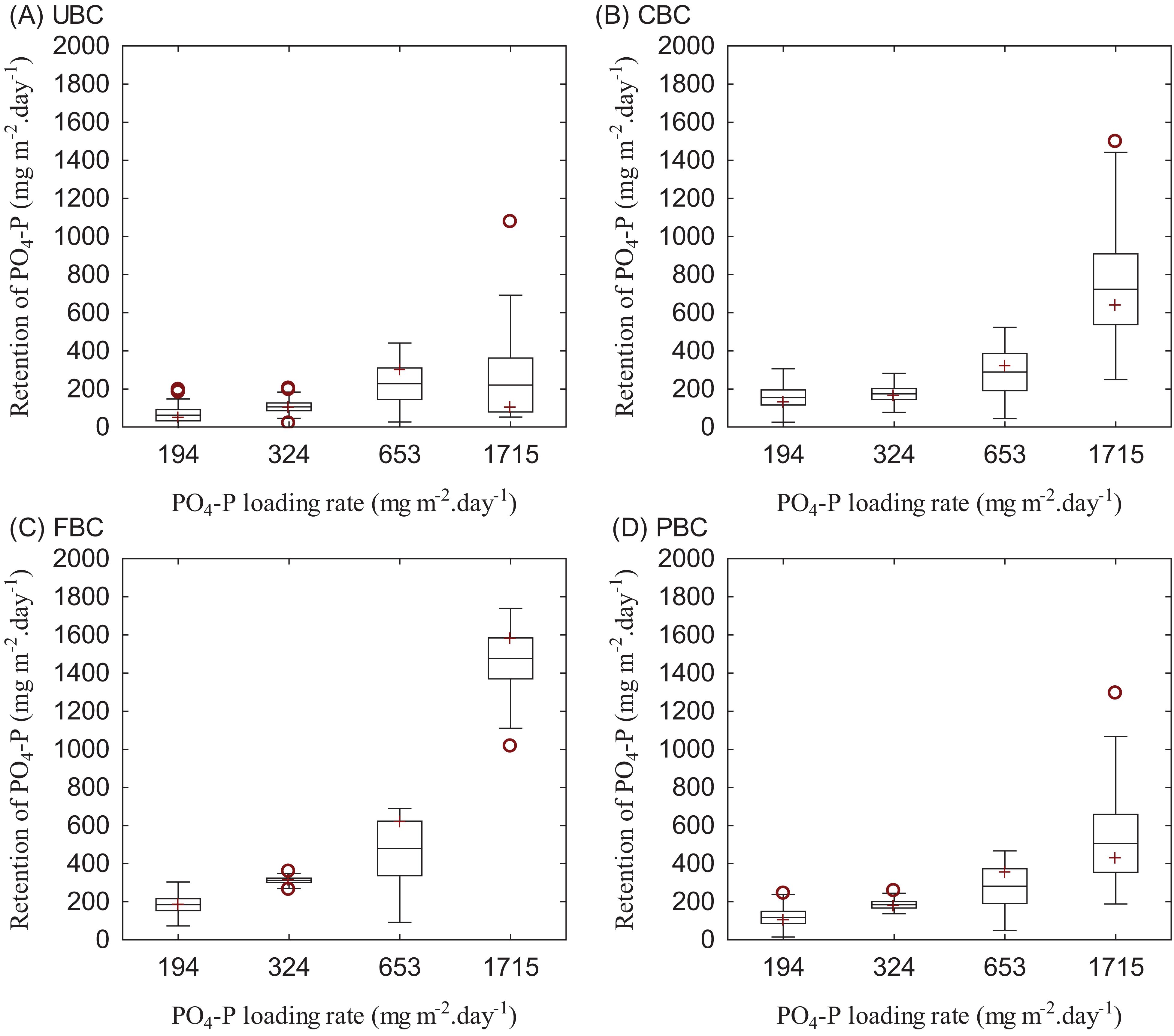
Figure 6. Boxplots of retention of phosphate-phosphorus (PO4-P, mg m–2 day–1) in (A) unimpregnated biochar filter (UBC), (B) CaO-impregnated biochar filter (CBC), (C) FeCl3-impregnated biochar filter (FBC), and (D) biochar filter mixed with Polonite® (PBC), in response to increasing phosphate loading rate (PLR). The middle line (–) of the box is the mean, the cross (+) is the median, the box is the confidence interval, the whiskers are the non-outlier range, and the red dots are outliers and extreme values.
In contrast, FBC showed high average removal of PO4-P (>99%) at all PLRs until week 5 in PLR-1715, when removal began to decline (Supplementary Figure S4C). The average removal of PO4-P in PBC was around 57% at PLR-194 to PLR-653, but declined drastically in PLR-1715 to reach 30% (Supplementary Figure S4D).
Discussion
Adsorption Capacity of UBC, CBC, FBC, and PBC
In the batch adsorption experiment, the four biochar types were shaken with solutions of five different PO4-P concentrations. CBC showed greatest P adsorption capacity, PBC and FBC both had lower but similar adsorption, and untreated biochar (UBC) adsorbed least P. The shape of the adsorption isotherm for CBC (Figure 3) showed that when the equilibrium concentrations were low, the increase in equilibrium adsorption was also low. This may indicate involvement of dissolved organic compounds in P adsorption at low concentrations (Essington, 2004). The Freundlich model best fitted PO4-P adsorption to CBC and PBC biochars, which means the adsorption to these materials can best be described as non-uniform. Adsorption to FBC biochar correlated better with the Langmuir adsorption model, which indicates that their adsorption can be modeled as homogeneous and in a monolayer over the biochar surface. Similarly, Li et al. (2016) found that P removal using wheat straw biochar impregnated with FeCl3 fitted well to a Langmuir model. In contrast, Chen et al. (2011) found that P adsorption by untreated and magnetite-coated biochar made from orange peel fitted the Freundlich model better.
The Langmuir adsorption constant kF was higher for adsorption on PBC than for the other biochar types. This indicates that the affinity between P and PBC was highest for this material. The Langmuir maximum adsorption Qm for CBC was negative, which is unrealistic, indicating that this model was not suitable for describing adsorption on CBC (Table 4). FBC had the highest Qm (3.2 mg g–1), but this is still lower than the 16.58 mg g–1 reported by Liu et al. (2015) for Fe-impregnated biochar made from wheat straw. The biochar in the study by Li et al. (2016) had smaller-diameter particles than the biochar used in this experiment (<1 mm compared with 1–7 mm) and higher iron to biochar ratio (0.7 compared with 0.3), which can explain the difference. The maximum adsorption capacity obtained in column filters (1.5, 4.02, 6.41, and 2.75 mg g–1, for UBC, CBC, FBC, and PBC, respectively) was higher in some cases (CBC and FBC) than in the batch experiment (1.53, −0.41, 3.21 ± 0.01, and 2.42 mg g–1, respectively). Besides physical-chemical adsorption in the column filters, other factors may have contributed to this behavior, e.g., when PLR increased, more bacterial growth occurred in the filters and thus more PO4-P was assimilated. The bacterial growth coupled with the competitive adsorption of organics can drastically affect the removal of phosphates (Dalahmeh et al., 2014). Moreover the complexation of organics with phosphates is also possible.
Retention of Phosphate in Impregnated Biochar and Response to Loading Conditions
In filters designed for P removal, the capacity of the filter to retain PO4-P depends on pH and the availability of surfaces rich in metal oxides such as Al-, Fe-, and Ca- oxides (Arias et al., 2001; Hylander et al., 2006). In this study, biochar filters impregnated with Ca (CBC) and Fe (FBC) showed better removal of PO4-P than UBC filters (UBC and PBC). The PO4-P adsorbed to the FBC and CBC filters reacted with Fe and Ca minerals to form strong precipitates or surface complexes. The FBC filter maintained low pH (<4.5) during the entire experimental period, indicating continuous formation of Fe oxides in FBC and hence enhanced PO4-P adsorption to these oxides. This was confirmed by XRD tests of FBC surfaces after 100 weeks of operation, which showed a high content (26%) of akaganeite [a chloride-containing Fe (III) oxide-hydroxide mineral]. This indicated success in achieving long-lasting impregnation of biochar using FeCl3. In contrast, with the CBC filter and its higher pH, the PO43+ ions formed complexes with Ca ions, so CaHPO4⋅2H2O and Ca4H(PO4)⋅3H2O could have been formed at the beginning of filter operation (US EPA, 2002). Adsorption of PO4-P in CBC deteriorated over time. When the surface of the biochar was examined using XRD after 100 weeks of operation, CaO was not among the minerals deposited on the biochar. This shows that impregnation of biochar with CaO was reversed during operation of the filter. PBC showed less retention of PO4-P than FBC and CBC, despite its high content of anorthite and orthoclase, which are known to bind phosphate (Figure 4). The amount of Polonite mixed with the biochar to produce PBC was only 30% of its weight and this was probably not enough to efficiently remove PO4-P from wastewater over long periods. The UBC filter showed poor retention of PO4-P and low removal (5–50%) during the experiment, probably due to PO43+ assimilation to bacterial biofilms and to weak adsorption to silica and aluminum in the quartz, albite, and microcline deposited on the surface of UBC. These minerals likely originated from soil particles in wastewater.
Increasing the HLR from 56 to 74 L m–2 day–1 decreased retention of PO4-P in UBC and PBC. In fact, UBC experienced breakthrough at HLR-74 and PO4-P desorbed/washed out from the filter, indicating low robustness of UBC to sudden increases in HLR (Figure 5). The PBC filter did not show breakthrough when the HLR increased, but the removal rate of PO4-P decreased substantially (from 42% to 18%). High hydraulic loads increase the infiltration rate and thereby reduce exchanges between mobile water in macropores and water retained in micropores (Boller et al., 1993). When HLR increases, the contact time between PO4-P and the filter medium decreases and less adsorption occurs. For CBC, the first increase in HLR (HLR-56 to HLR-74) enhanced removal of PO4-P due to enhanced surface wetting, and hence more PO4-P reaching CaO hidden in biochar particles. However, when HLR-74 increased to HLR-112, removal of PO4-P declined due to the washing-out of retained PO4-P. Calcium is also likely to have been washed off the biochar surface in CBC when the HLR increased, and hence less PO4-P could be bound to CaO. This was confirmed by XRD analysis after HLR-112, in which CaO could not be detected in the CBC material. FBC was not influenced by HLR, because PO4-P was mostly removed by chemical adsorption, in which the bonds between the PO4 and iron oxides are strong. Leaching of PO4-P in this form will be triggered by e.g., changes in pH or oxidation-reduction (redox) potential. During the entire HLR trials, the pH in the FBC treatment was about 4 SU, with very low fluctuations.
With all filters except FBC, adsorption (retention) of PO4-P in the filters increased as the PLR increased (Figure 6 and Supplementary Figure S4). This was due to UBC, CBC, and PBC developing new equilibrium conditions each time the PLR changed. This behavior was also observed in the batch adsorption trial, in which the increase in equilibrium concentrations (Ce) resulted in an increase in P adsorbed on the surface. Moreover, the average concentration of PO4-P in the outflow increased in response to increasing PLR. At the beginning of each PLR trial, the concentration of PO4-P in the outflow from UBC, CBC, and PBC was initially low and increased with time, which indicated that PO4-P removal was high at the beginning of each PLR trial and decreased thereafter (Supplementary Figure S4). However, this was due to old water with low levels of PO4-P draining from the filters for several days after the onset of the new PLR. The hydraulic retention times were not determined for these filters.
In contrast, FBC maintained low PO4-P concentrations (<0.05 mg L–1) in outflow at high and low PLR (194–1715 g m2 L–1 day–1). This indicates that the adsorption capacity of the FBC material was mainly limited by chemical adsorption of PO4 onto Fe oxides available on the surface of the filter. Removal of PO4-P declined in FBC at PLR-1715 and this was accompanied by an increase in pH from 4–4.5 to 5.0–5.4 SU, which showed that Fe oxides in FBC started to be consumed after formation of FeOOH-PO4. Siwek et al. (2019) reported that, with increasing pH, the sorption affinity of Fe compounds to PO43+ drops, whereas the solubility of their combinations with P increases. The same study reported the optimal pH for creating durable complexes of FeOOH-PO4 is below 5–7.
In general, PO4-P retention capacity was highest in FBC and lowest in UBC, with the filters following the order FBC > < cps:it > CBC < /cps:it≫ < cps:it > PBC < /cps:it≫UBC, with overall PO4-P retention of 358.36, 221.75, 152.36, and 84.75 g m–2, respectively. Based on retention capacities, it was possible to estimate the service life of the FBC filter for different concentrations of PO4-P in wastewater (Table 6). The service life defined here is the time during which a filter can be successfully used to remove >70% of PO4-P from wastewater. This threshold was chosen because the Swedish regulations require 70% removal of PO4-P in areas classified as non-sensitive recipients (Havs- och vattenmyndighetens, 2016). Theoretically, having a low concentration (<2.6 mg L–1) of PO4-P in wastewater would allow the FBC filter to remain active for about 4.8 years and CBC to remain active for 1.25 years, before their PO4-P removal declined to <70%. The wastewater in OWTS usually contains high concentrations (6–12 mg L–1) of PO4-P. If a FBC filter is used to treat wastewater without any pre-treatment (i.e., average PO4-P concentration 8.8 mg L–1), its service life may decline to 17 months, after which it may fail to meet the 70% PO4-P removal limit. The service life of UBC and PBC could not be estimated for the 70% removal limit, because these filters did not achieve efficient removal at the start of filter operation.
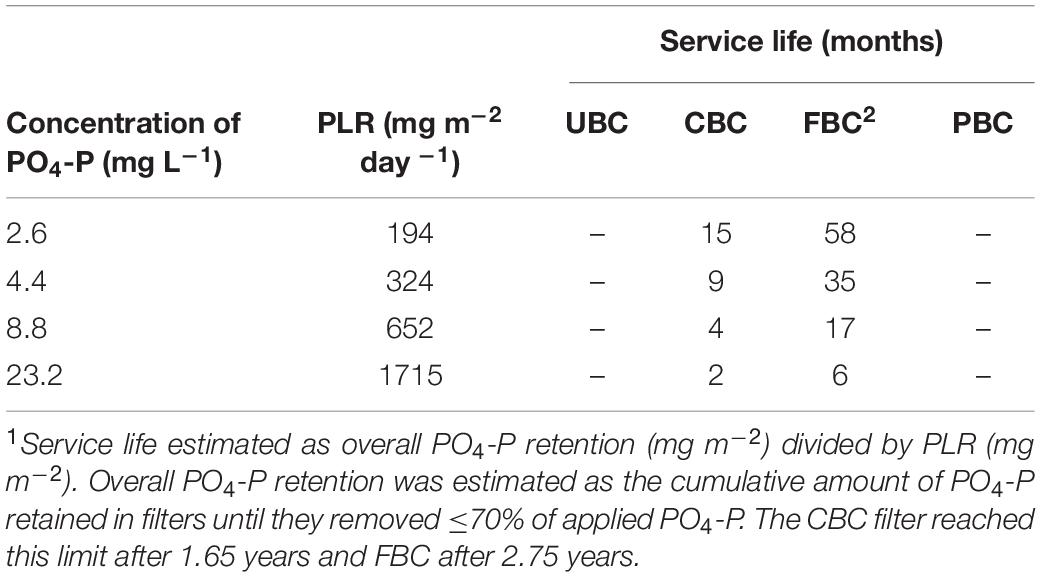
Table 6. Estimated service life1 (in months) of unimpregnated biochar filter (UBC), CaO-impregnated biochar filter (CBC), FeCl3-impregnated biochar filter (FBC), and biochar filter mixed with Polonite® (PBC) under different phosphorus loading rates (PLR) at a fixed hydraulic loading rate of 74 L m–2.
The column filters received wastewater, with all ingredients included (solids, organics, nutrient, micropollutants, etc.). It is likely that some of these elements might adsorb to biochar (e.g., NH4, pharmaceuticals, bacteria, viruses, and heavy metals), competing for removal of PO4-P. Biochar impregnated with Fe (FBC) can apparently be an effective filter material for removal of PO4-P from wastewater in OWTS. However, in full-scale implementation, it is recommended to use the FBC filter as a final treatment (or post-treatment) step for removal of PO4-P after organic matter and other competing elements have been removed from wastewater. This is likely to ensure effective utilization of the impregnated surfaces during planned use and to elongate the service life of the filter.
Conclusion
1. The Freundlich model best fitted PO4-P adsorption to biochar mixed with Polonite (PBC), while adsorption to iron-impregnated filter material (FBC) biochar correlated better with the Langmuir adsorption model.
2. The enrichment of the FBC and CBC with Fe+3 and Ca+2 lead to effective adsorption of PO4-P through formation of strong precipitates and surface complexes.
3. FBC showed the best performance in phosphorus removal from wastewater. FBC showed high phosphate adsorption capacity and can achieve high retention over long periods, especially if wastewater contains <3 mg PO4-P L–1. This filter was robust and not sensitive to changes in hydraulic loading rate or phosphorus loading rate.
4. It is recommended that iron-impregnated biochar is used in a separate phosphorus filter module (post-treatment step) after organic matter and solids are removed from wastewater. With this approach, all adsorption sites on the impregnated biochar material will be available for phosphate removal.
Data Availability Statement
The datasets generated for this study are available on request to the corresponding author.
Author Contributions
SD and YS collected the data and wrote the article. MJ did FTIR analyses and revised the article. LH did the pyrolyses of biochar and revised the article. GD did the SEm analyses and IH did the XRD analyses. All authors contributed to the article and approved the submitted version.
Funding
This work was funded by the Swedish Agency for Water and Marine Management (Havs- och vattenmyndigheten) (Grant Number 864/2017), the Swedish Foundation for international cooperation in research and education (Grant Number PT2016–6875), Swedish research council for sustainable development (FORMAS) (Grant Numbers 2018-00261 and 2019-01257).
Conflict of Interest
The authors declare that the research was conducted in the absence of any commercial or financial relationships that could be construed as a potential conflict of interest.
Acknowledgments
We gratefully acknowledge Almoyied Assayed and Anas Al-Mustafa for their efforts in the laboratory work.
Supplementary Material
The Supplementary Material for this article can be found online at: https://www.frontiersin.org/articles/10.3389/fenvs.2020.538539/full#supplementary-material
Supplementary Figure 1 | Scanning electron microscope images of (A) unimpregnated biochar filter (UBC), (B) CaO-impregnated biochar filter (CBC), (C) FeCl3-impregnated biochar filter (FBC), and (D) biochar filter mixed with Polonite® (PBC). All images are shown at 10 μm resolution except Polonite (20 μm).
Supplementary Figure 2 | X-ray diffraction patterns of untreated biochar (UBC) and biochar impregnated with CaO (CBC), before and after use for wastewater filtration.
Supplementary Figure 3 | X-ray diffraction patterns of biochar treated with FeCl3 (FBC) and biochar mixed with Polonite, before and after use for wastewater filtration.
Supplementary Figure 4 | Percentage removal of phosphate-phosphorus (PO4-P) in (A) unimpregnated biochar filter (UBC), (B) CaO-impregnated biochar filter (CBC), (C) FeCl3-impregnated biochar filter (FBC), and (D) biochar filter mixed with Polonite (PBC), as a function of time at P loading rate (PLR)-194 (•), PLR-324 (■), PLR-652 (▲) and PLR-1715 (◆).
References
Agrafioti, E., Kalderis, D., and Diamadopoulos, E. (2014). Ca and Fe modified biochars as adsorbents of arsenic and chromium in aqueous solutions. J. Environ. Manag. 146, 444–450. doi: 10.1016/j.jenvman.2014.07.029
Arias, C. A., Del Bubba, M., and Brix, H. (2001). Phosphorus removal by sands for use as media in subsurface flow constructed reed beds. Water Res. 35, 1159–1168. doi: 10.1016/s0043-1354(00)00368-7
Berger, C. (2012). “Biochar and activated carbon filters for greywater treatment: comparison of organic matter and nutrient removal,” in Energy and Technology, (Uppsala: Swedish University of Agricultural Sciences).
Boller, M., Schwager, A., Eugster, J., and Mottier, V. (1993). Dynamic bahavior of intermittent buried filters. Water Sci. Technol. 28, 99–107. doi: 10.2166/wst.1993.0213
Chen, B., Chen, Z., and Lv, S. (2011). A novel magnetic biochar efficiently sorbs organic pollutants and phosphate. Bioresour. Technol. 102, 716–723. doi: 10.1016/j.biortech.2010.08.067
Dalahmeh, S. (2016). Capacity of Biochar Filters for Wastewater Treatment in Onsite Systems – Technical Report: Report 2016-90. Gothenburg: Havs och vattenmyndighet.
Dalahmeh, S. S., Pell, M., Hylander, L. D., Lalander, C., Vinnerås, B., and Jönsson, H. (2014). Effects of changing hydraulic and organic loading rates on pollutant reduction in bark, charcoal and sand filters treating greywater. J. Environ. Manag. 132, 338–345. doi: 10.1016/j.jenvman.2013.11.005
Del Bubba, M., Arias, C. A., and Brix, H. (2003). Phosphorus adsorption maximum of sands for use as media in subsurface flow constructed reed beds as measured by the Langmuir isotherm. Water Res. 37, 3390–3400. doi: 10.1016/S0043-1354(03)00231-8
Downie, A., Krosky, A., and Munroe, P. (2009). “Physical properties of biochar,” in Biochar for Environmental Management Science and Technology, eds J. Lehmann and S. Joseph, (London: Earthscan).
Essington, M. E. (2004). Soil and Water Chemistry: An Integrative Approach. Boca Raton: CRC Press LLC.
Havs- och vattenmyndighetens, (2016). Havs- Och Vattenmyndighetens Allmänna råd om små Avloppsanordningar för Hushållsspillvatten;, (Ed.) H.-o. Vattenmyndigheten, Vol. 17. Gothenburg: Havs- och vattenmyndigheten.
He, S., Zhong, L., Duan, J., Feng, Y., Yang, B., and Yang, L. (2017). Bioremediation of wastewater by iron oxide-biochar nanocomposites loaded with photosynthetic bacteria. Front. Microbiol. 8:823. doi: 10.3389/fmicb.2017.00823
He, Z., Uchimiya, S. M., and Guo, M. (2016). “Production and characterization of biochar from agricultural by-products: overview and use of cotton biomass residues,” in Agricultural and Environmental Applications of Biochar: Advances and Barriers, eds M. Guo, Z. He, and S. M. Uchimiya, (Madison, WI: Soil Science Society of America, Inc).
Heinonen-Tanski, H., and Matikka, V. (2017). Chemical and microbiological quality of effluents from different on-site wastewater treatment systems across Finland and Sweden. Water 9:47. doi: 10.3390/w9010047
HELCOM, (2018). State of the Baltic Sea – Second HELCOM Holistic Assessment 2011-2016. Helsinki: HELCOM.
Hylander, L. D., Kietlinska, A., Renman, G., and Simán, G. (2006). Phosphorus retention in filter materials for wastewater treatment and its subsequent suitability for plant production. Bioresour. Technol. 97, 914–921. doi: 10.1016/j.biortech.2005.04.026
Jebrane, M., Terziev, N., and Heinmaa, I. (2017). Biobased and sustainable alternative route to long-chain cellulose esters. Biomacromolecules 18, 498–504. doi: 10.1021/acs.biomac.6b01584
Jung, K.-W., Jeong, T.-U., Kang, H.-J., and Ahn, K.-H. (2016). Characteristics of biochar derived from marine macroalgae and fabrication of granular biochar by entrapment in calcium-alginate beads for phosphate removal from aqueous solution. Bioresour. Technol. 211, 108–116. doi: 10.1016/j.biortech.2016.03.066
Li, J. H., Lv, G. H., Bai, W. B., Liu, Q., Zhang, Y. C., and Song, J. Q. (2016). Modification and use of biochar from wheat straw (Triticum aestivum L.) for nitrate and phosphate removal from water. Desalinat. Water Treat. 57, 4681–4693. doi: 10.1080/19443994.2014.994104
Liu, P., Liu, W.-J., Jiang, H., Chen, J.-J., Li, W.-W., and Yu, H.-Q. (2012). Modification of bio-char derived from fast pyrolysis of biomass and its application in removal of tetracycline from aqueous solution. Bioresour. Technol. 121, 235–240. doi: 10.1016/j.biortech.2012.06.085
Liu, F., Zuo, J., Chi, T., Wang, P., and Yang, B. (2015). Removing phosphorus from aqueous solutions by using iron-modified corn straw biochar. Front. Environ. Sci. Eng. 9, 1066–1075. doi: 10.1007/s11783-015-0769-y
Mead, J. (1981). A comparison of the Langmuir, Freundlich and Temkin equations to describe phosphate adsorption properties of soils. Soil Res. 19, 333–342. doi: 10.1071/sr9810333
Niwagaba, C. B., Dinno, P., Wamala, I., Dalahmeh, S. S., Lalander, C., and Jönsson, H. (2014). Experiences on the implementation of a pilot grey water treatment and reuse based system at a household in the slum of Kyebando-Kisalosalo, Kampala. J. Water Reuse Desal. 4, 294–307. doi: 10.2166/wrd.2014.016
Palm, O., Malmén, L., and Jönsson, H. (2002). Robusta, Uthålliga små Avloppsystem. Stockholm: Naturvårdsverket.
Ren, J., Li, N., Li, L., An, J.-K., Zhao, L., and Ren, N.-Q. (2015). Granulation and ferric oxides loading enable biochar derived from cotton stalk to remove phosphate from water. Bioresour. Technol. 178, 119–125. doi: 10.1016/j.biortech.2014.09.071
Renman, A., and Renman, G. (2010). Long-term phosphate removal by the calcium-silicate material Polonite in wastewater filtration systems. Chemosphere 79, 659–664. doi: 10.1016/j.chemosphere.2010.02.035
Schellenger, F. L., and Hellweger, F. L. (2019). Phosphorus loading from onsite wastewater systems to a lake (at long time scales). Lake Reserv. Manag. 35, 90–101. doi: 10.1080/10402381.2018.1541031
Siwek, H., Bartkowiak, A., and Włodarczyk, M. (2019). Adsorption of phosphates from aqueous solutions on alginate/goethite hydrogel composite. Water 11:633. doi: 10.3390/w11040633
Smith, V. H. (2003). Eutrophication of freshwater and coastal marine ecosystems: a global problem. Environ. Sci. Pollut. Res. 10, 126–139.
Keywords: adsorption, biochar, iron impregnation, calcium impregnation, onsite wastewater treatment, phosphate retention
Citation: Dalahmeh SS, Stenström Y, Jebrane M, Hylander LD, Daniel G and Heinmaa I (2020) Efficiency of Iron- and Calcium-Impregnated Biochar in Adsorbing Phosphate From Wastewater in Onsite Wastewater Treatment Systems. Front. Environ. Sci. 8:538539. doi: 10.3389/fenvs.2020.538539
Received: 13 March 2020; Accepted: 20 October 2020;
Published: 20 November 2020.
Edited by:
Alberto Tiraferri, Politecnico di Torino, ItalyReviewed by:
Xiaofei Tan, Hunan University, ChinaKonstantinos Plakas, Centre for Research and Technology Hellas (CERTH), Greece
Copyright © 2020 Dalahmeh, Stenström, Jebrane, Hylander, Daniel and Heinmaa. This is an open-access article distributed under the terms of the Creative Commons Attribution License (CC BY). The use, distribution or reproduction in other forums is permitted, provided the original author(s) and the copyright owner(s) are credited and that the original publication in this journal is cited, in accordance with accepted academic practice. No use, distribution or reproduction is permitted which does not comply with these terms.
*Correspondence: Sahar S. Dalahmeh, sahar.dalahmeh@slu.se; sahar.dalahmeh@geo.uu.se