- 1Faculty of Nature and Life Sciences, Laboratory of Plant Biodiversity: Conservation and Valorization, Ex- ITMA, Sidi Bel Abbes, Algeria
- 2Institute of Bio- and Geosciences, Agrosphere (IBG-3), Forschungszentrum Jülich, Jülich, Germany
- 3Faculty of Nature and Life Sciences, Laboratory of Spaces Eco-Development, Ex- ITMA, Sidi Bel Abbes, Algeria
- 4Faculty of Nature and Life Sciences, Laboratory of Environmental Information Synthesis, Ex- ITMA, Sidi Bel Abbes, Algeria
Mountainous forests in Algeria are severely affected by climate change. The degradation is exacerbated by overgrazing, deforestation, and poor land management linked to the harsh topographical conditions of the mountain agrosystem. These conditions have influenced the turnover of the soil organic matter and thus the SOC stocks storage. This study aims to investigate the average of organic carbon stored in forest soils of Tessala Mount in Algeria and to examine the influence of different plant formations alongside with geographical characteristics and soil physicochemical properties on the amount of carbon stored in the soil. We estimated the critical threshold, the saturation point, and the organic carbon deficit of soils. The correlation between geographical characteristics and soil physicochemical properties and soil organic carbon stocks was determined using principal component analysis and other statistical tools. The results of the study show that the organic carbon stock in soils of Tessala Mount area has an average value of 77.4 t ha−1. The maximum average of SOC stocks (121 t ha−1) of 0–30 cm depth was noted under dense matorral of green and kermes oak followed by sparse garrigue with a value of 112 t ha−1. The soil organic carbon stock in Tessala region was positively correlated with coarse silt, elevation, and northern exposure, but negatively with calcium carbonates contents. The current carbon contents of Tessala topsoil are 22 g C kg−1 which is very low and closer to the critical threshold (11 g C kg−1) whose estimate was based on their clay and silt content. The estimated maximum storage capacity is 160 g C kg−1. The preliminary estimate of the forest soils organic carbon stock of Tessala Mount under current natural conditions indicates an alarming situation with a low rate close to the critical threshold, thus exposing this area to further and stronger degradation.
Introduction
The fight against climate change is a major challenge in the 21st century (Watson et al., 2000). Forests play a key role in controlling the accumulation of greenhouse gases in the atmosphere; by acting as carbon sinks, they absorb the equivalent of some 2 billion metric tons of carbon dioxide each year (Newell and Stavins, 2000; Canadell and Raupach, 2008; Jackson et al., 2008). The Mediterranean region is strongly affected by climate change; it experiences changes in temperatures intensification of summer, drought periods, and low and erratic precipitation rates (Moriondo et al., 2006; Gao and Giorgi, 2008; Nicault et al., 2008; Mariotti et al., 2015).
The rapid demographic population increases combined with the overuse of forest rangelands, and exacerbated by the ongoing climate change, has between 2000 and 2010 reduced the North African forest cover by more than 6.3 million hectares (FAO, 2011).
In Algeria, mountainous areas cover about 10 million hectares; with four million hectares of forest and 1.5 million hectares of agricultural lands, they are home for 25% of Algeria’s population (Tatar, 2011). Within forested regions of Algeria, 75% of the area (about 3 million hectares) does mainly consist of Aleppo pine (Pinus halepensis), cork oak (Quercus suber), holm oak (Quercus ilex), common juniper (Juniperus communis), barbary thuja (Tetraclinis articulata), common eucalyptus or southern blue gum (Eucalyptus globulus), atlas cedar (Cedrus atlantica), and maritime or cluster pine (Pinus pinaster). The other remaining 25% of the forest area (about 1 million ha) is composed of maquis and garrigues (Louni, 1994; Benderradji et al., 2006; Tatar, 2012). Algerian forests have never supplied large quantities of wood but still manage to supply a modest industrial sector, and their socioeconomic importance remains considerable for the country (Tatar, 2012).
Critically, due to direct and indirect human pressures on these mountain agroecosystems, they are currently undergoing advanced degradation. The degradation is exacerbated through overgrazing of the maquis (shrubby mostly evergreen vegetation), deforestation, and poor land management (e.g., tillage in the slope direction); these factors linked to the harsh topographical conditions of the mountain agrosystem have in turn enhanced wind and water erosion and loss of the topsoil (Veldkamp, 1994; Plaza-Bonilla et al., 2015; Belahcene, 2019).
Loss of soil is associated with soil fertility decline as a considerable amount of soil organic matter which is responsible for soil chemical, physical, and biological properties is eroded (Sánchez-Marañón et al., 2002; Miralles et al., 2007). Soil organic matter is a key component of soil fertility and quality; it plays an important role in water retention, soil aeration, biological activity, and nutrients provision (Johnston, 1991; Troeh and Thompson, 2005; Ayuke et al., 2011). Thus progressive degradation of mountainous soil and continuous loss of soil organic matter mean imbalance and disturbance of all of the above functions. Therefore, assessing soil organic matter dynamics in mountainous areas is important to estimate their resilience and sustainability.
Soil organic carbon (SOC) accumulation and distribution in mountainous agrosystem is governed by numerous factors such as the location and topographical characteristics, altitude, slope percentage, and exposure or cardinal directions (Meliho et al., 2019; Tsozué et al., 2019). In addition, vegetation type plays a crucial role in determining the organic matter inputs and thus the SOC composition and the soil physical, biological, and chemical properties especially in the upper soil layers (Fu et al., 2020; Zhang et al., 2021).
The IPCC reports indicate that several indicators have been developed to improve forecasts of the changes in soil organic C stocks (Eggleston et al., 2006). Among these indicators is the assessment of the deficit in soil organic C saturation proposed by Hassink (1997) and used by several studies. The concept of SOC saturation suggests that the quantity of stable SOC is limited and determined by fine particles (clay and fine silt) contents (Feller et al., 1991; Bonde et al., 1992; Hassink, 1997; Angers et al., 2011; Ben Hassine et al., 2012). The critical threshold of carbon represents a minimum level of OC below which the soil structure is weakened and the risks of its degradation become stronger because of the low structural stability (Loveland and Webb, 2003).
Very few detailed studies are available providing accurate regional SOC estimates based on geographical features, vegetation type, and soil physicochemical properties. In particular, there is a lack of national-scale studies on soil spatial variability in forest soils of Tessala Mount region in terms of quantity, quality, and dynamics of soil organic matter. Previous studies reported the degradation of the forest ecosystems of Tessala Mount (Kouider et al., 2011; Belahcene, 2019). Therefore, a better understanding of the soil organic matter distributions as well as its quantification and quality is necessary to forecast the status of the soil organic matter in Tessala Mount and to assess soil fertility through investigating the correspondence between geographical characteristics, soil physicochemical properties, and SOC stocks. Therefore, this preliminary study objectives are to 1) quantify the average OC stored in forest soils of Tessala Mount, 2) examine the influence of different plant formations, geographical characteristics, and soil physicochemical properties on the amount of SOC stored in the soil, and to 3) analyze the overall OC balance of forest soils of Tessala Mount and its critical threshold.
Materials and Methods
Description of the Study Area
Tessala mountains are part of the Tell Atlas of Algeria situated on a hundred kilometers between Oran to the North and the plain of Sidi Bel Abbes to the South. They give reliefs from 500 to 1,000 m of altitude, where the mount Tessala culminates to 1,061 m. The landscape draws a rugged morphology with slopes exceeding 50% in some parts, accentuated by a very marked ravine. These slopes are dissected by a large number of wadis (an Arabic term traditionally referring to a valley) and tributaries that carry fine and stony materials downstream in the plain of Sidi Bel Abbés (Pouquet, 1952). The main lithological units are represented by marls, limestone marls, and clay marl; they are met mainly in the North and North-West part (Insid, 2019). The sandstone and limestone marl of the Eocene are found mainly in the South-East. The gray marls are located in the southern part of the piedmonts. Tessala Mount has semiarid climate characteristics, characterized by a clear dry and wet season, the average annual temperature is ∼21°C, and the rainfall is low and irregular (356 mm) and occurs mainly in autumn and winter. The period from late April to mid-October is generally dry with high summer temperature and the aridity index is 12.7 (Belahcene, 2019). The vegetation cover of the forest area of Tessala mountains is composed of oak coppice (99 ha); matorral (310 ha); forest (209 ha); dense garrigue and sparse garrigue (382 ha) (Saidi, 2017).
Sites Description and Sampling Strategy
The sampling campaign involved 7 sites; each site is characterized by a distinctive vegetation formation (Supplementary Table S2 and Supplementary Figure S2). Soil samples were collected randomly from each location in May 2017 at soil depth ranging from 0 to 30 cm. Given the morphology of the terrain and the nature of the harsh rugged relief of the study area, a series of six transects with a total length of approximately 8.5 km was selected; these six series represent 7 vegetation coves including matorral (M); sparse garrigue (GC); forest (F); dense garrigue (GD); olive grove (OL); oak coppice (TC); and herbaceous vegetation (VH). A random sampling but still dependent on the relief and the distribution of plant formations was followed for each virtual line. In total 32 composite soil samples (each composite is composed of 3 samples) were collected; this gave one sample per 265 m. Soil samples were classified according to the World Reference Base for Soil Resources (WRB) (WRB, 2007); see Supplementary Table S2. The soil samples were air-dried and sieved using a 2 mm sieve for physical and chemical analyses. The mineral fractions were measured by the “Robinson” method (Gee and Or, 2002). The principle is to remove any cement such as carbonates, oxides, and organic substances by oxidation with hydrogen peroxide, the dispersion is done in sodium hexametaphosphate, the samples were pipetted at different times and different depths, following different sedimentation intervals, and the duration and the depth were calculated with Stokes’s law. All the granulometric data were expressed in the percentage of fine earth (<2 mm). The pH was measured using a pH meter (Hanna Instruments, Netherlands). For the soil samples suspensions with a ratio of 1: 2.5 (m/v) of the fine earth and water, as shown by Thomas (1996), the salinity was evaluated by the electrical conductivity (EC) in millisiemens per centimeter (mS cm−1) using a conductivity meter (Hanna Instruments, The Netherlands); a suspension of fine earth and water with a ratio 1:5 (m/v) was considered for this analysis (Rhoades, 1996). The bulk density (BD) in g cm−3 was determined by the direct sampling method using a 5.5 cm diameter and 5 cm height cylinder (Blake and Hartge, 1986). The samples taken from the cylinder were dried then passed through a sieve with a diameter of 2 mm in order to measure the content of coarse particles (expressed in %). The OC content was determined by the modified Walkley and Black method, based on the oxidation of OC by potassium dichromate (K2Cr2O7) in sulfuric acid (Gillman et al., 1986). The soil inorganic carbon (SIC, CaCO3 content) was determined for total carbonates by Bernard calcimeter method (Sparks et al., 1996) using HCl acid. The CO2 released by the reaction was measured by a gas burette (Bernard calcimeter). For active carbonates, the method described by Drouineau (1942) was used, the calcium associated with oxalates gives insoluble calcium oxalate, and the excess of ammonium oxalate is then determined by a solution of potassium permanganate in a sulfuric solution. The physical and chemical characteristics of the soil are reported in Supplementary Table S1.
Organic C Stocks, Saturation, Deficit, and Critical Threshold
The C stocks were calculated for 0–30 cm depth, with the C stocks for each sample calculated using the equation recommended (FAO, 2017; FAO, 2019) as follows:
where SOC stock is a soil organic carbon stock (t C ha−1), C is the carbon content (g C kg−1 soil), BD is the bulk density (g cm−3), T is the thickness of the soil horizon (cm), and CP is the gravel content (g g−1 soil).
The percentage of fine particles was used to calculate the stable carbon potential in soils following the equation below according to Hassink (1997):
The stable C value can be compared to the measured soil C and from that carbon saturation deficit can be calculated (Angers et al., 2011):
The critical thresholds of carbon represent a minimum level of organic C below which the soil structure is weakened and the risks of its degradation become stronger because of the low structural stability. This value was calculated after Autfray et al. (2009) as follows:
Data Analysis
An ANOVA test was performed to determine the significance of differences between measured averages. A Welch test was realized to verify if there is a difference between soil SOC stocks values under the 7 studied vegetation covers. A principal component analysis (PCA) was performed to determine if there is a correlation between variables followed by parallel hierarchical cluster analysis (HCA) (Supplementary Figure S1) to verify the information provided by the PCA. The HCA was applied to the variables and not to the individuals (represented here by the samples for the 7 sites); the aim is to group similar variables and above all to highlight the variables that have close proportions of SOC, that is, those which have more influence on the accumulation of SOC. Finally, two bivariate correlation tests with two quantitative variables were performed, one between the SOC stocks and the coarse silt and the other between SOC stocks and altitude. All data collected were processed by SPSS Statistics software version 24.0 (IBM Corp, 2016).
Results and Discussion
Variation of SOC Stocks Under Different Vegetation Covers
The average of the total OC contained in the first 30 cm of the soil varies according to the sampled sites; the highest values were attained in the topsoil under matorral and sparse garrigue vegetation covers, with rates of 3.1% and 3%. The lowest percentages were recorded under forest and herbaceous vegetation covers, with 0.8% and 1.1%. The contents recorded under dense garrigue, olive groves, and oak coppice were in the same range between 2% and 2.6% (Supplementary Table S1). The maximum value of SOC stocks averages (121 t ha−1) stored in Tessala Mount soils at 30 cm depth was noted under dense matorral of green and kermes oak followed by sparse garrigue with a value of 112 t ha−1, while the soils under olive groves contain 77 tz ha−1 and soils under dense garrigue and oak coppice have an average of 73 t ha−1. Finally, the soils under herbaceous vegetation have an average of 48 t ha−1. The minimum SOC stocks average value is recorded under the clear forest of Aleppo pine and eucalyptus with 38 t ha−1 (Table 1).
The actual OC measured for the first 30 cm of Tessala Mount soils records an average of 22 g OC kg−1; it varies between 31.7 g OC kg−1 under dense matorral of oak and 8.6 g OC kg−1 under the clear forest of Aleppo pine and eucalyptus (Table 2). Our results are in the range of previous findings for woodland topsoil in the mountain forest of Belezma in Algeria, where the content of SOC stocks varied from 36 g OC kg−1 under holm oak to 53 g OC kg−1 under cedar (Bensid et al., 2015). Ben Hassine et al. (2012) recorded a SOC content average equal to 0.8% (8 g OC kg−1) in Tunisia under almost similar climatic conditions. Boulmane et al. (2010) indicated that more than 80% of SOC stocks can be stored in the top 30 cm of soils of the Mediterranean oak forest of the Moroccan Middle Atlas. In their study, 63 t ha−1 of SOC stocks was recorded for the dense oak grove located at 1,670 m altitude and 47 t ha−1 for the clear oak located at 1,450 m altitude. Bounouara et al. (2017) recorded 100 t ha−1 and 168 t ha−1 of SOC stocks in Skikda region, northern east Algeria, at an altitude varying between 13 m and 140 m.
The SOC stocks at Tessala Mount vary from one site to another; this variability could be explained by the diversification in terms of vegetation, exposure, and altitude. Several studies emphasized that SOC stocks can vary regionally following environmental factors such as precipitation, temperature, elevation, slope, soil texture, and land use (Yimer et al., 2006; Liu et al., 2011; Gutiérrez-Girón et al., 2015). The type of vegetation plays a crucial role in determining SOC spatial distribution as the trees species composition can control the amount and composition of organic matter inputs especially in the upper soil layers (Díaz-Pinés et al., 2011). Aboveground vegetation properties such as stand age, leaf area index, aboveground biomass, mean tree height, tree diameter, stand density, tree basal area, and litter depth are all vegetation properties that have a potential effect on SOC stocks (Li et al., 2010).
Other studies indicated that plant formations have a profound effect on soil carbon stocks (Arasa-Gisbert et al., 2018; Yao et al., 2019; Koga et al., 2020). Boulmane et al. (2010) found that the density of a plant stand may have no significant effect on carbon sequestration potential in biomass, but it certainly has a significant effect on carbon sequestration in soils and more precisely on the layer 0–15 cm (between 52.7 t ha−1 and 33.2 t ha−1). However, a Canadian study conducted by Lagacé Banville (2009) under different climatic conditions indicates a significant correlation between plant formation and SOC stocks distribution of hardwood and closed canopy conifers with 13.4 and 12.2 kg C m−2 (134 and 122 t ha−1), respectively; the highest intakes are in sites with the highest plant density.
SOC Stocks Dynamic in Relation to Altitude
A bivariate correlation test, between two quantitative variables, the altitude and OC, was performed; the results showed a significant (p<0.05) positive correlation between the altitude and OC (Figure 1). The soils under dense matorral of holm and kermes oak have an average SOC stocks value of 121 t ha−1 at an average altitude of 955 m, whereas soils under Aleppo pine and eucalyptus forest recorded the lowest value with only 38 t ha−1 at an average altitude of 790 m (Figure 2). This difference may be explained by the influence of temperature and humidity on the decomposition rates of soil organic matter and thus the SOC accumulation. Geomorphological terrain attributes, such as slope and elevation, play an important role in controlling carbon stock variations (Chaplot et al., 2001; Moghiseh et al., 2013). The elevation is considered as one of the various important environmental factors controlling soil OC content in arid and semiarid rangelands (Shedayi et al., 2016; Sharafatmandrad, 2019). Besides, low temperatures at higher elevation may suppress the decomposition rates of soil organic matter (Yimer et al., 2006; Wang et al., 2013; Gutiérrez-Girón et al., 2015). Water is the most important key that can influence SOC storage, as microbial activity increases in well-aerated and moist soils which in turn increases the rate of organic matter decomposition, especially under warm to hot climates (Beare et al., 1994; Ogle et al., 2005). Bounouara (2018) found that, for surface horizons (0–30 cm), the stocks of OC decreased from upstream to downstream, while for depth horizons (>30 cm), the opposite can be observed; in his study the carbon stocks have gradually increased from mountain soils to the lowlands. In a similar study on natural forests in northern Iran, Moghiseh et al. (2013) have shown that slope aspect and site altitude have a great effect on SOC storage; higher SOC storage was observed at a higher altitude compared to low altitude sites.
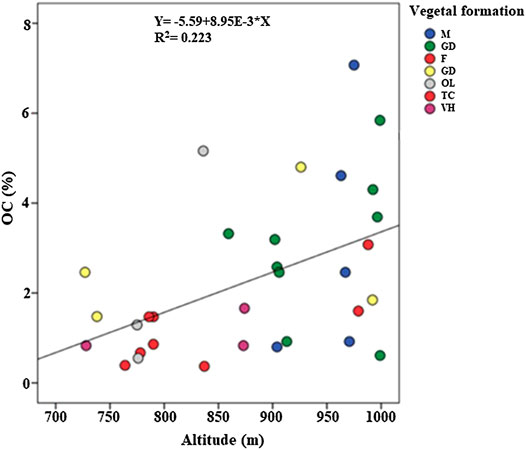
FIGURE 1. Correlation between altitude and soil organic carbon. M: matorral; GC: sparse garrigue; F: forest; GD: dense garrigue; OL: olive grove; TC: Oak coppice; VH: Herbaceous vegetation.
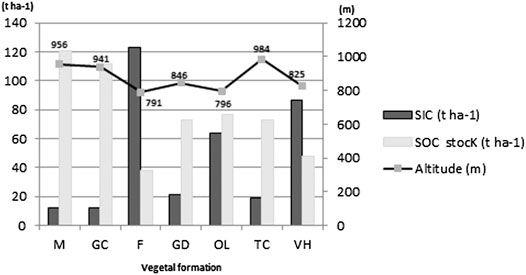
FIGURE 2. SOC stocks variation according to SIC (CaCO3) and altitude. M: matorral; GC: sparse garrigue; F: forest; GD: dense garrigue; OL: olive grove; TC: oak coppice; VH: herbaceous vegetation.
Soil Inorganic Carbon Stocks
The maximum average of SIC stocks (123 t ha−1) stored in Tessala Mount soils at 0–30 cm was noted under a clear forest of Aleppo pine and eucalyptus, followed by herbaceous vegetation with a value of 87 t ha−1, while the soils under olive groves contain 84 t ha−1 and soils under dense garrigue and oak coppice have, respectively, an average of 21 t ha-1 and 19 t ha−1. The minimum SIC stocks average value was recorded under sparse garrigue and dense matorral of green and kermes oak with 12 t ha−1 for each (Table 1). Our results indicated that SIC has a negative correlation with SOC stocks (p < 0.05); soil with higher values of SIC exhibited less SOC accumulation and vice versa (Figure 3). For example, at SIC of 3% a maximum SOC stocks value was recorded (121 t ha−1), while the soil with 22% of the total carbonates content only recorded 38 t ha−1 of SOC stocks (Figure 2). The results came in contradiction to previous studies findings, which indicate that SIC has a positive effect on soil organic matter stabilization and soil structure (Álvaro-Fuentes et al., 2009; Shi et al., 2017; Martí-Roura et al., 2019; Quijano et al., 2020). Exchangeable Ca correlates positively with the concentration of SOC and its resistance to oxidation due to the positive effect on the aggregation and structural stability of the soil and by the indirect influence on the accumulation and occlusion of OC (Rowley et al., 2018). However, the exact mechanisms behind this relationship and the actual consequences on occluded SOC stocks remain unclear as many factors such as soil texture, pH, organic inputs, and distribution may interfere with these mechanisms. Martí-Roura et al. (2019) found that microbial carbon use efficiency was more closely associated with land use than with carbonate content.
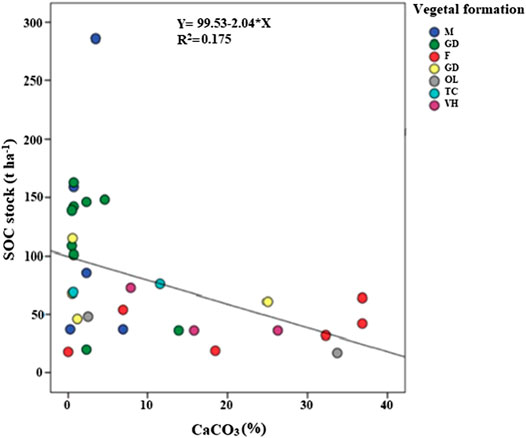
FIGURE 3. Correlation between soil carbonates (CaCO3) and SOC stocks. M: matorral; GC: sparse garrigue; F: forest; GD: dense garrigue; OL: olive grove; TC: oak coppice; VH: herbaceous vegetation.
SOC Stocks Variation in Relation to Coarse Silt Texture
A bivariate correlation test, between coarse silt and SOC stocks, was performed to show the relationship between the coarse silt and SOC accumulation; the results showed a significant marginal correlation of coarse silt with SOC stocks (p < 0.05) (Figure 4). Increasing in coarse silt contents would tend to relatively increase SOC stocks. For example, under dense matorral of holm and kermes oak, an average value of 121 t ha−1 for SOC stocks under 41.3% of coarse silt content was noted, whereas under Aleppo pine and eucalyptus forests the lowest value of SOC stocks was recorded with only 38 t ha−1 and an average percentage of coarse silt of 14.6% (Supplementary Table S1). Soil texture has a distinct influence on organic carbon accumulation because of the physical protection of SOM induced by the aggregation of fine particles, such as clay and fine silt (Feller et al., 1991; Jobbágy and Jackson, 2001). Previous studies showed that forests soils with high contents of silt ranging from fine to coarse aggregates are essential for OC stabilization (Balesdent et al., 1998). Coarse silts and sands fractions ranging from 20 to 2000 µm have the potential to associate plant debris at various stages of decomposition (Feller et al., 1991; Schulten et al., 1993). Forest soils contain particularly large amounts of SOM associated with the coarser soil fraction (Martí-Roura et al., 2019).
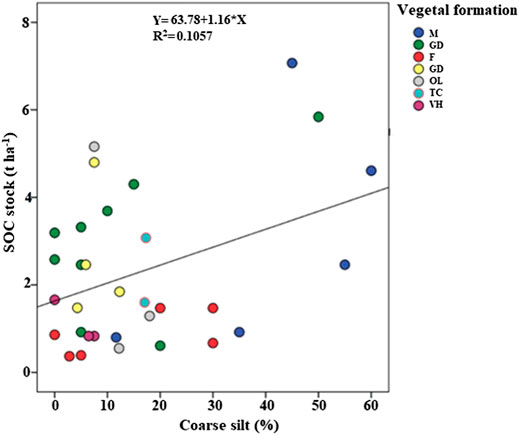
FIGURE 4. Correlation between coarse silt content and soil organic carbon. M: matorral; GC: sparse garrigue; F: forest; GD: dense garrigue; OL: olive grove; TC: oak coppice; VH: herbaceous vegetation.
Correlation Between Location, Soil Properties, and SOC Stocks (PCA)
The principal components selected are the F1 axis, which provides the most important statistical information with an inertia rate of 37.05% and the F2 axis with 15.69%. These two axes restore 52.74% of the initial information and highlight two groups confirmed by parallel hierarchical cluster analysis (HCA) (Figure S1). The choice of these groups was made based on the principal component analysis (PCA) (Figure 5) and HCA.
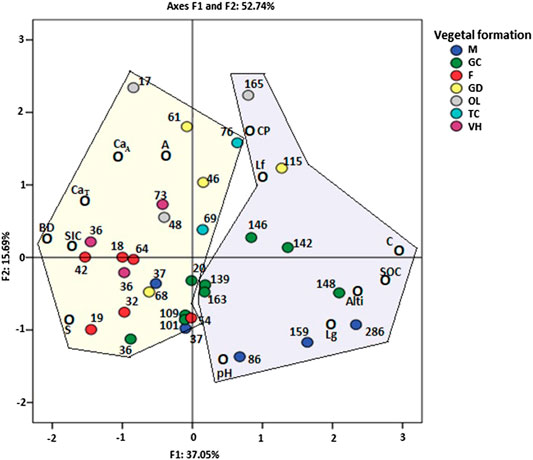
FIGURE 5. Factorial plan representing the intersection of edaphic variables measured for the sample’s locations and different vegetation covers (PCA). A: clay, Lf: fine silt, Lg: coarse silt, S: silt, CP: coarse particle, BD: bulk density CaT: total carbonates, CaA: active carbonates, C: carbon, SOC: soil organic carbon stock, SIC: soil inorganic carbon, Alt.: altitude. M: matorral; GC: sparse garrigue; F: forest; GD: dense garrigue; OL: olive grove; TC: oak coppice; VH: herbaceous vegetation.
Group 1: SOC, C, altitude, coarse silt, pH; Group 2: coarse particles, fine silt, clay, bulk density, sand, total and active CaCO3, SIC.
Based on the information provided by the PCA, it was noted that elevation, OC, pH, and coarse silt correlate with stocks; PCA shows as variable; OC, altitude, pH, and coarse silt are factors that would influence SOC stocks.
On the basis of the information provided by the sampled sites and the measured edaphic variables, the result of the analysis confirms that the variables of group 1 are correlated with axis 1 which essentially indicates the geographical and physicochemical characteristics of the sites studied represented by northern exposure, high elevation, high CO, and high coarse silt ratio; all of these factors positively contributed to the increase in SOC stock.
On the contrary, the variables of group 2 are not correlated with axis 1; this can be explained by the geographical and physicochemical characteristics of these sites, with a southern exposure, a low altitude, and a low CO content; all these factors are not conducive to the storage of CO in soils. It has been noted that, in addition to the physical and chemical properties of topsoil sites, other geographical factors appear to influence OC storage, such as exposure and altitude. The northern slopes in Tessala area benefit from milder climatic conditions, with lower temperatures compared to the southern slopes. In addition, the sea breeze in summer on the northern slopes, with low evaporation rates and significant rainfall, has created a favorable environment for the accumulation of the organic matter and thus influenced the OC storage (Saidi, 2017). Also, the high altitude of the northern slopes is associated with cooler local climate conditions compared to the southern exposure which has low altitude and thus warmer climate. Soil carbon budget tends to increase when moving from a warmer climate to a cooler climate; this is attributed to the accelerated decomposition of organic matter in warmer and dry climates compared to cold and wet climates (Schimel et al., 1994; Bachelet et al., 2001).
Saturation Rate and Critical Soil Carbon Thresholds
The calculation of soil carbon saturation levels has made it possible to estimate the soil carbon storage capacity. Table 2 (values are presented in g kg−1) shows that the maximum soil storage capacity is noted under olive grove with 23.1% of carbon, in the second position the oak coppice with 21%, then under dense garrigue with 16.7%, and under herbaceous vegetation and sparse garrigue with 13.8% and 13.7%, respectively. Lastly, forest and scrubland recorded 12% and 11%, respectively. The critical threshold of carbon varies between 0.7% and 1.7%. The average rate of SOC concentration in the study area is 22 g C kg−1, and the average critical threshold is 11 g kg−1 (Table 2). The average saturation rate is 160 g C kg−1; this means that it is possible to increase the average carbon content to 138 g C kg−1. In Tunisia, under nearly similar pedoclimatic conditions to our study area (Ben Hassine et al., 2012), a critical threshold was recorded that varies between 2.7% and 1.79% (27 g C kg−1 and 17.9 g C kg−1). The average deficit of Tessala Mount topsoils is 137 g C kg−1. For agricultural topsoils Angers et al. (2011) note an average deficit of 8.1 g C kg−1, with a maximum of up to 500 g C kg−1. The lowest deficit recorded of 78 t C ha−1 was for the soils under dense matorral located at a high altitude with a North exposure. As described in this study smaller deficits occurred at higher altitudes, probably due to the combined effect of the cooler temperature and the presence of meadows. Table 2 shows that the current carbon content (Cactual) of Tessala Mount soils is very low and closer to the critical threshold (Ccriticalthreshold) than its maximum storage capacity represented by saturation carbon (Csat). The carbon deficit (C.sat. deficit) in soils of the study area is very alarming because it is very close to saturation levels.
Conclusion
The SOC stocks in Tessala Mount are affected by vegetation type, topographical features, and physicochemical characteristics of the soil. The maximum average of SOC stocks stored in Tessala Mount soils at 0–30 cm is flowing in this order: dense matorral of green and kermes oak > sparse garrigue > olive groves > dense garrigue and oak coppice > herbaceous vegetation > Aleppo pine and eucalyptus. The geographical and physicochemical characteristics represented by northern exposure, high altitude, high OC contents, and high coarse silt ratio are factors that have favorably influenced SOC storage in Tessala Mount. Generally, the current C content of Tessala topsoil is very low and closer to the critical threshold and a maximum storage capacity of 160 g C kg−1. The findings indicate further loss of C under the current natural conditions and management conditions.
Data Availability Statement
The datasets generated for this study are available on request to the corresponding author.
Author Contributions
FF contributed to the conception of the study. FF and MM contributed to the establishment of the work plan. MM and FF organized the soil database, field trips, and physicochemical analyses in the laboratory. AL took care of the part related to plant formations and provided information necessary for the field study. MM and RD performed statistical analysis. MM, FF, and RD interpreted the statistical data. MM wrote the first draft of the manuscript. FF made the first corrections and wrote the second draft. RB and RM corrected the second draft and contributed to its improvement. RB, RM, and FF wrote sections of the manuscript. RB did scientific evaluation of the data. RB and RM proceeded to the final revision of the manuscript and wrote sections of manuscript. All authors contributed to the revision of the manuscript and read and approved the submitted version.
Funding
This study is part of a research project (PRFU project number; D00L02UN220120190005) funded by the Plant Biodiversity Laboratory: Conservation and Valorization of Sidi Bel Abbes University, and the Ministry of Higher Education and Scientific Research (Algeria), but the publication costs are not covered; the corresponding author is responsible for publication costs.
Conflict of Interest
The authors declare that the research was conducted in the absence of any commercial or financial relationships that could be construed as a potential conflict of interest.
Acknowledgments
Special thanks are due to the financial support from the Ministry of Higher Education and Scientific Research, through the associated PRFU project (D00L02UN220120190005) and to the Department of Environmental Sciences of Sidi Bel Abbès University for facilitating access to the soil laboratory.
Supplementary Material
The Supplementary Material for this article can be found online at: https://www.frontiersin.org/articles/10.3389/fenvs.2020.520284/full#supplementary-material.
References
Álvaro-Fuentes, J., Cantero-Martínez, C., López, M., Paustian, K., Denef, K., Stewart, C., et al. (2009). Soil aggregation and soil organic carbon stabilization: effects of management in semiarid Mediterranean agroecosystems. Soil Sci. Soc. Am. J. 73 (5), 1519–1529. doi:10.2136/sssaj2008.0333
Angers, D. A., Arrouays, D., Saby, N. P. A., and Walter, C. (2011). Estimating and mapping the carbon saturation deficit of French agricultural topsoils. Soil Use Manag. 27 (4), 448–452. doi:10.1111/j.1475-2743.2011.00366.x
Arasa-Gisbert, R., Vayreda, J., Román-Cuesta, R. M., Villela, S. A., Mayorga, R., and Retana, J. (2018). Forest diversity plays a key role in determining the stand carbon stocks of Mexican forests. For. Ecol. Manage. 415–416, 160–171. doi:10.1016/j.foreco.2018.02.023
Autfray, P., Guillaume, P., Forest, F., Chabanne, A., and Husson, O. (2009). “Outils de gestion des matières organiques en agriculture de conservation en Tunisie, [Tools for the management of organic matter in conservation agriculture in Tunisia],” in Conference: Journée Nationale La gestion du stock organique dans les sols de Tunisie, El Kef, Tunisia, Le Kef, jeudi juin 04, 2009 (s.n.), 10.
Ayuke, F. O., Brussaard, L., Vanlauwe, B., Six, J., Lelei, D. K., Kibunja, C. N., et al. (2011). Soil fertility management: impacts on soil macrofauna, soil aggregation and soil organic matter allocation. Appl. Soil Ecol. 48 (1), 53–62. doi:10.1016/j.apsoil.2011.02.001
Bachelet, D., Neilson, R. P., Lenihan, J. M., and Drapek, R. J. (2001). Climate change effects on vegetation distribution and carbon budget in the United States. Ecosystems 4 (3), 164–185. doi:10.1007/s10021-001-0002-7
Balesdent, J., Besnard, E., Arrouays, D., and Chenu, C. (1998). The dynamics of carbon in particle-size fractions of soil in a forest-cultivation sequence. Plant Soil 201 (1), 49–57. doi:10.1023/A:1004337314970
Beare, M., Hendrix, P., and Coleman, D. (1994). Water‐stable aggregates and organic matter fractions in conventional‐and no‐tillage soils. Soil Sci. Soc. Am. J. 58 (3), 777–786. doi:10.2136/sssaj1994.03615995005800030020x
Belahcene, F. (2019). Mise en valeur des potentialités agricole des zones de montagne; cas des monts de Tessala et Beni Chougrane dans la wilaya de Sidi Bel Abbes (Algérie occidentale) [Development of the agricultural potential of mountain areas; case of the mountains of Tessala and Beni Chougrane in the wilaya of Sidi Bel Abbes]. Master’s thesis. Sidi Bel Abbes (Algeria): Dspace de universite Djillali Liabes de SBA.
Ben Hassine, B., Karbout, N., Kridane, K., Sanaa, M., and Jedidi, N. (2012). Caractérisation des fractions colloïdales minérales et organiques des horizons superficiels des sols d’une toposéquence en zone semi-aride de la Tunisie [Characterization of the mineral and organic colloidal fractions of the superficial horizons of soils of a toposquence in a semi-arid zone of Tunisia]. Etudes et Gestion des Sols 19 (2), 105–118. doi:10.13140/RG.2.1.4581.5121
Benderradji, M. E. H., Alatou, D., Arfa, A. M. T., and Benachour, K. (2006). Problèmes de dégradation de l'environnement par la désertification et la déforestation Impact du phénomène en Algérie [Problems of environmental degradation through desertification and deforestation Impact of the phenomenon in Algeria]. New Med. 4, 15–22. Available at: https://newmedit.iamb.it/edizioni_new_medit,229,229,2006,20,80,problemes-de-degradation-de-lenvironnement-par-la-desertification-et-la-deforestation:-impact-du-phenomene-en-algerie.htm
Bensid, Z., Halitat, T., Sbih, M., and Messaadia, H. (2015). Status of soil organic matter of cedar stands in forest mountains of Belezma (Aures, Algeria). Res. J. For 9 (1), 6–21. doi:10.3923/rjf.2015.6.21
Blake, G. R., and Hartge, K. (1986). Bulk density. Methods Soil Analys 5, 363–375. 10.2136/sssabookser5.1.2ed.c13
Bonde, T. A., Christensen, B. T., and Cerri, C. C. (1992). Dynamics of soil organic matter as reflected by natural 13C abundance in particle size fractions of forested and cultivated oxisols. Soil Biol. Biochem. 24 (3), 275–277. doi:10.1016/0038-0717(92)90230-U
Boulmane, M., Makhloufi, M., Bouillet, J.-P., Saint-André, L., Satrani, B., Halim, M., et al. (2010). Estimation du stock de carbone organique dans la chênaie verte du Moyen Atlas marocain [Estimating the stock of organic carbon in the holm oak forest of the Moroccan Middle Atlas Mountains]. Acta Bot. Gall 157 (3), 451–467. doi:10.1080/12538078.2010.10516222
Bounouara, Z., Chevallier, T., Balesdent, J., Toucet, J., Sbih, M., Bernoux, M., et al. (2017). Variation in soil carbon stocks with depth along a toposequence in a sub-humid climate in North Africa (Skikda, Algeria). J. Arid Environ. 141, 25–33. doi:10.1016/j.jaridenv.2017.02.001
Bounouara, Z. (2018). Origine et évolution de la matière organique dans les sols des zones sub-hmide cas de la région de Skikda [Origin and evolution of organic matter in soils of sub-moist areas in the Skikda region case study]. Master’s thesis. Batna (Algeria): Université de Batna 1-hadj lakhder.
Canadell, J. G., and Raupach, M. R. (2008). Managing forests for climate change mitigation. Science 320 (5882), 1456–1457. doi:10.1126/science.1155458
Chaplot, V., Bernoux, M., Walter, C., Curmi, P., and Herpin, U. (2001). Soil carbon storage prediction in temperate hydromorphic soils using a morphologic index and digital elevation model. Soil Sci. 166 (1), 48–60. doi:10.1097/00010694-200101000-00008
Díaz-Pinés, E., Rubio, A., Van Miegroet, H., Montes, F., and Benito, M. (2011). Does tree species composition control soil organic carbon pools in Mediterranean mountain forests? For. Ecol. Manage. 262 (10), 1895–1904. doi:10.1016/j.foreco.2011.02.004
Drouineau, G. (1942). Dosage rapide du calcaire actif du sol: nouvelles données sur la separation et la nature des fractions calcaires. Ann. Agron. 12, 441–450. doi:10.1007/BF00748590
Eggleston, S., Buendia, L., Miwa, K., Ngara, T., and Tanabe, K. (2006). IPCC guidelines for national greenhouse gas inventories. Japan: Institute for Global Environmental Strategies Hayama, 20.
FAO (2019). “Measuring and modeling soil carbon stocks and stock changes in livestock production systems,” in Guidelines for assessment ". (Livestock environmental assessment and performance (LEAP) partnership. Rome, Italy: FAO, 170. Available: http://www.fao.org/3/ca2934en/CA2934EN.pdf.
FAO (2017). Soil organic carbon: the hidden potential. Rome, Italy: Food and Agriculture Organization of the United Nations Available: http://www.fao.org/3/a-i6937e.pdf.
FAO (2011). State of the world’s forests. Rome, Italy: Food and Agriculture Organization of the United NationsAvailable: http://www.fao.org/3/i2000e/i2000e00.htm.
Feller, C., Fritsch, E., Poss, R., and Valentin, C. (1991). Effet de la texture sur le stockage et la dynamique des matières organiques dans quelques sols ferrugineux et ferrallitiques (Afrique de l’Ouest, en particulier) [Effect of texture on the storage and dynamics of organic matter in some ferrous and ferrallitic soils, west Africa, in particular]. Cah. ORSTOM, sér. Pédol XXVI (1), 25–36.
Fu, D., Wu, X., Duan, C., Chadwick, D. R., and Jones, D. L. (2020). Response of soil phosphorus fractions and fluxes to different vegetation restoration types in a subtropical mountain ecosystem. Catena 193, 104663. doi:10.1016/j.catena.2020.104663
Gao, X., and Giorgi, F. (2008). Increased aridity in the Mediterranean region under greenhouse gas forcing estimated from high resolution simulations with a regional climate model. Global Planet. Change 62 (3), 195–209. doi:10.1016/j.gloplacha.2008.02.002
Gee, G., and Or, D. (2002). “Particle size analysis,” in Methods of soil analysis, Part 4. Physical methods. Madison, WI: Soil Science Society of America Book Series, 5, 255–293.
Gillman, G. P., Sinclair, D. F., and Beech, T. A. (1986). Recovery of organic carbon by the walkley and black procedure in highly weathered soils. Commun. Soil Sci. Plant Anal. 17 (8), 885–892. doi:10.1080/00103628609367759
Gutiérrez-Girón, A., Díaz-Pinés, E., Rubio, A., and Gavilán, R. G. (2015). Both altitude and vegetation affect temperature sensitivity of soil organic matter decomposition in Mediterranean high mountain soils. Geoderma 237–238, 1–8. doi:10.1016/j.geoderma.2014.08.005
Hassink, J. (1997). The capacity of soils to preserve organic C and N by their association with clay and silt particles. Plant Soil 191 (1), 77–87. doi:10.1023/A:1004213929699
Insid (2019). Available at: http://insid.dz/index.php?option=com_contact&view=contact&id=1&Itemid=103 (Accessed December 07, 2019).
Jackson, R. B., Randerson, J. T., Canadell, J. G., Anderson, R. G., Avissar, R., Baldocchi, D. D., et al. (2008). Protecting climate with forests. Environ. Res. Lett. 3 (4), 044006. doi:10.1088/1748-9326/3/4/044006
Jobbágy, E. G., and Jackson, R. B. (2001). The distribution of soil nutrients with depth: global patterns and the imprint of plants. Biogeochemistry 53 (1), 51–77. doi:10.1023/A:1010760720215
Johnston, A. (1991). Soil fertility and soil organic matter. Advances in soil organic matter research: the impact on agriculture and the environment. Melksham, Wiltshire: The Royal Society of Chemistry, 299–314.
Koga, N., Shimoda, S., Shirato, Y., Kusaba, T., Shima, T., Niimi, H., et al. (2020). Assessing changes in soil carbon stocks after land use conversion from forest land to agricultural land in Japan. Geoderma 377, 114487. doi:10.1016/j.geoderma.2020.114487
Kouider, C., Zoheir, M., Ali, L., and Salah Eddine Bachir, B. (2011). Impact de l’action anthropozoogène sur l’écosystème forestier du mont de Tessala (Algérie occidentale) [Impact of anthropogenic action on the forest ecosystem of Mount Tessala (Western Algeria)]. Sci. Chang. Planétaires Sécher 22 (3), 197–206. doi:10.1684/sec.2011.0310
Lagacé Banville, J. (2009). Caractérisation des stocks de carbone de 5 types de formations végétales dans un secteur du bassin versant de la rivière Eastmain [Characterization of the carbon stocks of 5 types of plant formations in a sector of the Eastmain River Watershed]. Master’s thesis. Montreal (Canada): Université du Québec à Montréal.
Li, P., Wang, Q., Endo, T., Zhao, X., and Kakubari, Y. (2010). Soil organic carbon stock is closely related to aboveground vegetation properties in cold-temperate mountainous forests. Geoderma 154 (3), 407–415. doi:10.1016/j.geoderma.2009.11.023
Liu, Z., Shao, M. a., and Wang, Y. (2011). Effect of environmental factors on regional soil organic carbon stocks across the Loess Plateau region, China. Agric. Ecosyst. Environ. 142 (3), 184–194. doi:10.1016/j.agee.2011.05.002
Loveland, P., and Webb, J. (2003). Is there a critical level of organic matter in the agricultural soils of temperate regions: a review. Soil. Till. Res. 70 (1), 1–18. doi:10.1016/S0167-1987(02)00139-3
Mariotti, A., Pan, Y., Zeng, N., and Alessandri, A. (2015). Long-term climate change in the Mediterranean region in the midst of decadal variability. Clim. Dynam. 44 (5–6), 1437–1456. 10.1007/s00382-015-2487-3
Martí-Roura, M., Hagedorn, F., Rovira, P., and Romanyà, J. (2019). Effect of land use and carbonates on organic matter stabilization and microbial communities in Mediterranean soils. Geoderma 351, 103–115. doi:10.1016/j.geoderma.2019.05.021
Meliho, M., Nouira, A., Benmansour, M., Boulmane, M., Khattabi, A., Mhammdi, N., et al. (2019). Assessment of soil erosion rates in a Mediterranean cultivated and uncultivated soils using fallout 137Cs. J. Environ. Radioact. 208–209, 106021. doi:10.1016/j.jenvrad.2019.106021
Miralles, I., Ortega, R., Sánchez-Marañón, M., Soriano, M., and Almendros, G. (2007). Assessment of biogeochemical trends in soil organic matter sequestration in Mediterranean calcimorphic mountain soils (Almería, Southern Spain). Soil Biol. Biochem. 39 (10), 2459–2470. doi:10.1016/j.soilbio.2007.04.017
Moghiseh, E., Heidari, A., and Ghannadi, M. (2013). Impacts of deforestation and reforestation on soil organic carbon storage and CO2 emission. Soil Environ 32 (1), 1–13. Available at: https://www.researchgate.net/publication/286200544_Impacts_of_deforestation_and_reforestation_on_soil_organic_carbon_storage_and_CO2_emission
Moriondo, M., Good, P., Durao, R., Bindi, M., Giannakopoulos, C., and Corte-Real, J. (2006). Potential impact of climate change on fire risk in the Mediterranean area. Clim. Res. 31 (1), 85–95. doi:10.3354/cr031085
Newell, R. G., and Stavins, R. N. (2000). Climate change and forest sinks: factors affecting the costs of carbon sequestration. J. Environ. Econ. Manag. 40 (3), 211–235. doi:10.1006/jeem.1999.1120
Nicault, A., Alleaume, S., Brewer, S., Carrer, M., Nola, P., and Guiot, J. (2008). Mediterranean drought fluctuation during the last 500 years based on tree-ring data. Clim. Dynam. 31 (2–3), 227–245. doi:10.1007/s00382-007-0349-3
Ogle, S. M., Breidt, F. J., and Paustian, K. (2005). Agricultural management impacts on soil organic carbon storage under moist and dry climatic conditions of temperate and tropical regions. Biogeochemistry 72 (1), 87–121. doi:10.1007/s10533-004-0360-2
Plaza-Bonilla, D., Arrúe, J. L., Cantero-Martínez, C., Fanlo, R., Iglesias, A., and Álvaro-Fuentes, J. (2015). Carbon management in dryland agricultural systems. A. Review. Agron. Sustain. Dev. 35 (4), 1319–1334. doi:10.1007/s13593-015-0326-x
Pouquet, J. (1952). Les monts du Tessala:(chaînes sud-telliennes d'Oranie) essai morphogénétique [The mountains of Tessala: (south-tellian chains of Oranie) morphological essay]. Master’s thesis. Paris (France): Société d'édition d'enseignement supérieur.
Quijano, L., Kuhn, N. J., and Navas, A. (2020). Effects of interrill erosion on the distribution of soil organic and inorganic carbon in different sized particles of Mediterranean Calcisols. Soil. Till. Res. 196, 104461. doi:10.1016/j.still.2019.104461
Rhoades, J. (1996). Salinity: electrical conductivity and total dissolved solids. Methods Soil Analys. 5, 417–435. 10.2136/msa2015.0039
Rowley, M. C., Grand, S., and Verrecchia, É. P. (2018). Calcium-mediated stabilisation of soil organic carbon. Biogeochemistry 137 (1), 27–49. doi:10.1007/s10533-017-0410-1
Sánchez-Marañón, M., Soriano, M., Delgado, G., and Delgado, R. (2002). Soil quality in mediterranean mountain environments. Soil Sci. Soc. Am. J. 66 (3), 948–958. doi:10.2136/sssaj2002.9480
Saidi, B. (2017). Dynamique de la phytodiversité dans les monts de tessala (Algerie occidentale) [Dynamics of phytodiversity in the Tessala Mountains (Western Algeria)]. MS dissertation. Sidi Bel Abbes (Algeria): Université Djillali Liabes de Sidi Bel Abbes.
Schimel, D. S., Braswell, B. H., Holland, E. A., McKeown, R., Ojima, D. S., Painter, T. H., et al. (1994). Climatic, edaphic, and biotic controls over storage and turnover of carbon in soils. Global Biogeochem. Cycles 8 (3), 279–293. doi:10.1029/94GB00993
Schulten, H. R., Leinweber, P., and Sorge, C. (1993). Composition of organic matter in particle‐size fractions of an agricultural soil. J. Soil Sci. 44 (4), 677–691. doi:10.1111/j.1365-2389.1993.tb02332.x
Sharafatmandrad, M. (2019). Soil carbon in arid and semiarid rangelands: controlling factors. Arab. J. Geosci. 12 (9), 310. doi:10.1007/s12517-019-4489-7
Shedayi, A. A., Xu, M., Naseer, I., and Khan, B. (2016). Altitudinal gradients of soil and vegetation carbon and nitrogen in a high altitude nature reserve of Karakoram ranges. Springer Plus 5 (1), 320. doi:10.1186/s40064-016-1935-9
Shi, H. J., Wang, X. J., Zhao, Y. J., Xu, M. G., Li, D. W., and Guo, Y. (2017). Relationship between soil inorganic carbon and organic carbon in the wheat-maize cropland of the North China Plain. Plant Soil 418 (1), 423–436. doi:10.1007/s11104-017-3310-1
Sparks, D., Page, A., Helmke, P., Loeppert, R., Soltanpour, P., Tabatabai, M., et al. (1996). Methods of soil analysis, part 3. Chem. Methods 1085–1121. doi:10.2136/sssabookser5.3
Tatar, H. (2011). La perennite des forets et les pratiques conservatoires en algerie [The preservation of forests and conservatory practices in Algeria]. Sci. Technol. D. Sciences de la terre 34, 39–46.
Tatar, H. (2012). Production forestière, exploitation et valorisation en Algérie [Forestry production, exploitation and valorization in Algeria]. Forêt méditerranéenne 33 (4), 361–368. Available at: http://hdl.handle.net/2042/48983
Thomas, G. W. (1996). Soil pH and soil acidity. Methods Soil Analys 5, 475–490. doi:10.2136/sssabookser5.3.c16
Tsozué, D., Nghonda, J. P., Tematio, P., and Basga, S. D. (2019). Changes in soil properties and soil organic carbon stocks along an elevation gradient at Mount Bambouto, Central Africa. Catena 175, 251–262. doi:10.1016/j.catena.2018.12.028
Veldkamp, E. (1994). Organic carbon turnover in three tropical soils under pasture after deforestation. Soil Sci. Soc. Am. J. 58 (1), 175–180. doi:10.2136/sssaj1994.03615995005800010025x
Wang, G., Zhou, Y., Xu, X., Ruan, H., and Wang, J. (2013). Temperature sensitivity of soil organic carbon mineralization along an elevation gradient in the Wuyi Mountains, China. PloS One 8 (1), e53914. doi:10.1371/journal.pone.0053914
Watson, R. T., Noble, I. R., Bolin, B., Ravindranath, N., Verardo, D. J., and Dokken, D. J. (2000). IPCC special report on land use, land-use change, and forestry. Cambridge, UK: Cambridge University Press, 375.
Yao, X., Yu, K., Deng, Y., Zeng, Q., Lai, Z., and Liu, J. (2019). Spatial distribution of soil organic carbon stocks in Masson pine (Pinus massoniana) forests in subtropical China. Catena 178, 189–198. doi:10.1016/j.catena.2019.03.004
Yimer, F., Ledin, S., and Abdelkadir, A. (2006). Soil organic carbon and total nitrogen stocks as affected by topographic aspect and vegetation in the Bale Mountains, Ethiopia. Geoderma 135, 335–344. doi:10.1016/j.geoderma.2006.01.005
Keywords: soil organic carbon, forest soils, Tessala Mount, plant formations, critical threshold, saturation point, organic carbon deficit, Algeria
Citation: Merabtene MD, Faraoun F, Mlih R, Djellouli R, Latreche A and Bol R (2021) Forest Soil Organic Carbon Stocks of Tessala Mount in North-West Algeria-Preliminary Estimates. Front. Environ. Sci. 8:520284. doi: 10.3389/fenvs.2020.520284
Received: 14 December 2019; Accepted: 07 December 2020;
Published: 28 January 2021.
Edited by:
Frank Hagedorn, Swiss Federal Institute for Forest, Snow and Landscape Research (WSL), SwitzerlandReviewed by:
Daniela Businelli, University of Perugia, ItalyJinyang Wang, Nanjing Agricultural University, China
Copyright © 2021 Merabtene, Faraoun, Mlih, Djellouli, Latreche, and Bol. This is an open-access article distributed under the terms of the Creative Commons Attribution License (CC BY). The use, distribution or reproduction in other forums is permitted, provided the original author(s) and the copyright owner(s) are credited and that the original publication in this journal is cited, in accordance with accepted academic practice. No use, distribution or reproduction is permitted which does not comply with these terms.
*Correspondence: Fatiha Faraoun, ZmFyYW91bi5mQGdtYWlsLmNvbQ==