- 1Laboratory of Hetero-Organic Compounds and Nanostructured Materials (LR18ES11), Department of Chemistry, Faculty of Sciences of Bizerte, University of Carthage, Zarzouna, Tunisia
- 2Water, Environmental and Food Chemistry, Department of Environmental Chemistry, IDAEA-CSIC, Barcelona, Spain
Contamination by classic (polybromodiphenyl ethers, PBDEs) and emerging halogenated flame retardants (HFRs) like pentabromobenzene (PBEB), hexabromobenzene (HBB), decabromodiphenyl ethane (DBDPE), and halogenated norbornenes (HNs), as well as organophosphate flame retardants (OPFRs) were investigated in sediment and eel (Anguilla Anguilla) samples from Bizerte Lagoon, northern Tunisia. For sediment samples, HFR levels ranged from 3.30 to 28.5 ng/g dry weight (dw), with a mean value of 10.6 ± 4.36 ng/g dw, while OPFR levels ranged between 9.77 and 164 ng/g dw, with a mean value of 53.6 ± 10.5 ng/g dw. As regards levels in fish, concentration of HFRs ranged between 4.72 and 151 ng/g lipid weight (lw) (mean value of 36.5 ± 28.5 ng/g lw), and OPFR levels ranged between 19.7 and 2,154 ng/g lw (mean value of 404 ± 367 ng/g lw). This is the first time that OPFR levels have been reporting in this area, being higher than those for HFRs. Statistical analysis of the relationship between OPFR and HFR concentrations in sediment and total organic carbon (TOC) was examined. Results suggested that OPFR levels were significantly correlated with TOC, whereas no correlation was found for HFRs and TOC. The health risk associated by the consumption of eel in Bizerte city was assessed and posed no threat to public health concerning PBDE and OPFR intakes.
Introduction
The growth of the human industrial activities has resulted in the contamination of many ecosystems. An increasing advance in technology and global population lead to increase of chemical production, and therefore, their release into the environment. The production and use of chemical additives known as flame retardants (FRs) have also increased. FRs are applied to plastics, electronics, vehicles, textiles, etc. (De Wit, 2002; Alaee et al., 2003). There are several types of FRs: halogenated flame retardants (HFRs) including brominated (BFRs) and chlorinated (CFRs), and organophosphorus flame retardants (OPFRs) which represent 20% of FRs consumption in 2006 in Europe (Van der Veen and de Boer, 2012). The most widely used group of HFRs were polybromodiphenyl ethers (PBDEs). PBDEs have been found in numerous environmental and biological matrices, such as sediment (Hu et al., 2010; Barón et al., 2014a; Herrero et al., 2018), air (Castro-Jiménez et al., 2017; Reche et al., 2019; Yadav and Nevi, 2019), biota (McHugh et al., 2010; Ben Ameur et al., 2013; Barhoumi et al., 2014a; Polder et al., 2014) and human samples (Norén and Meironyté, 2000; Zhou et al., 2002; Ben Hasine et al., 2015). Due to their adverse effect on the environment and human health, PBDEs were banned and added to the Stockholm Convention on Persistent Organic Pollutants (POPs) (SC, 2008).
Special consideration was given to new FRs which have been considered as an alternative to PBDEs, such as emerging BFRs, hexabromobenzene (HBB), pentabro-moethylbenzene (PBEB) and decabromodiphenyl ethane (DBDPE), and halogenated norbonenes (HNs), such as Dechlorane 602 (Dec 602), Dechlorane 603 (Dec 603), Dechlorane 604 (Dec 604), and Dechlorane plus (DP). HNs seem to have similar properties as PBDEs, such as persistence, high hydrophobicity and toxicity (Santín et al., 2016). In addition, many reports showed their presence in environmental and biological matrices, such as sediment (Sverko et al., 2008; Yu et al., 2015; Giulivo et al., 2017), fish (Santín et al., 2013; Widelka et al., 2016; Guo et al., 2017), bird eggs (Gauthier et al., 2007; Barón et al., 2014b).
The family of FRs encompasses OPFRs, which are organic compounds used as FRs but also as plasticizers, antifoaming agents and hydraulic fluids (Wei et al., 2015). OPFRs are proposed as alternative for PBDEs and they used in plastics, textile, electronic equipment, and furniture (Van der Veen and de Boer, 2012; Ma et al., 2017). Moreover, OPFRs are resistant to degradation and can stay persistently in the environment (Wei et al., 2015; Zhang et al., 2016). The occurrence of OPFRs was observed in various environmental compartments like air (Van der Veen and de Boer, 2012), sediment (Cao et al., 2012; Cristale and Lacorte, 2013; Wu et al., 2016) and biota (Giulivo et al., 2016; Greaves et al., 2016; Sala et al., 2019).
One example of an ecologic area submitted by several anthropogenic pressures is the Bizerte Lagoon, located in northern Tunisia, and placed in a heavily industrialized area. It supported many anthropogenic pressures, such as industrial, urbanization and agricultural activities (metallurgical industry, cement works, tire pollution factories, etc.). Previous research described the occurrence of different POPs in this area: polychlorinated biphenyl (PCBs) (Derouiche et al., 2004; Barhoumi et al., 2014b), polycyclic aromatic hydrocarbons (PAHs) (Trabelsi and Driss, 2005; Barhoumi et al., 2014c, 2016), and PBDEs and their methoxylated analogs (MeO-PBDEs) (Ben Ameur et al., 2013; Mekni et al., 2019). In this area, POPs appear with high concentration in surface sediment (∑PAHs from 16.9 to 394 ng/g dw) (Barhoumi et al., 2014c), in fish (∑PBDEs: 171 ng/g lw) (Ben Ameur et al., 2013), and clams (∑PBDEs: 95.6 ng/g lw) (El Megdiche et al., 2017).
European eels (Anguilla Anguilla) were chosen as bioindicator species for organic pollution due to their specific physical and behavioral characteristics (McHugh et al., 2010). Eels are long-lived and they migrate for a large distance to reach their spawning sites (Malarvannan et al., 2014). Moreover, eels have benthic live style. PBDE, HN, and OPFR occurrence in eel was previously reported in some works (Sühring et al., 2016; Zacs et al., 2016). However, to date, there is no information on contamination levels of FRs in eels from Bizerte Lagoon. The aim of this study is to evaluate the occurrence of HFRs and OPFRs in both abiotic (sediment) and biotic (eel) samples from Bizerte Lagoon (northern Tunisia).
Materials and Methods
Standards and Reagents
Native standards of OPFRs, including tris(2-butoxyethyl) phosphate (TBOEP), tris(chloroethyl) phosphate (TCEP), tris(2-chloroisopropyl) phosphate (TClPP), trihexyl phosphate (THP), and tris(2-ethylhexyl) phosphate (TEHP), were obtained from Santa Cruz Biotechnology (Santa Cruz, CA, USA). Tris(phenyl) phosphate (TPHP), triphenylphosphine oxide (TPPO) and tris(1,3-dichloro-2-propyl) phosphate (TDClPP) were purchased from Sigma-Aldrich (St. Louis, MO, USA). Isodecyldiphenyl phosphate (IDPP) and 2-ethylhexyldiphenyl phosphate (EHDPP) were purchased from AccuStandard (New Haven, CT, USA). Diphenyl cresyl phosphate (DCP), tri-n-butyl phosphate (TNBP) and tricresyl phosphate (TMCP) were provided by Dr. Ehrenstorfer (Augsburg, Germany). Tris(isopropyl-phenyl) phosphate (IPPP) was purchased from Chiron (Trondheim, Norway). d15-TDClPP, d27-TNBP, d12-TCEP, and 13C2-TBOEP were purchased from Wellington Laboratories Inc. (Guelph, ON, Canada). d15-TPHP was obtained from Cambridge Isotope Laboratories Inc. (Andover, MA, USA).
PBEB, HBB, DBDPE were purchased from Wellington Laboratories Inc. (Guelph, ON, Canada). Native and 13C-labeled standards mixtures of PBDEs (BDE-28, BDE-47, BDE-99, BDE-100, BDE-153, BDE-154, BDE-183, and BDE-209), syn- and anti-DP isomers and 13C-syn-DP were obtained from Cambridge Isotope Laboratories Inc. Dec 602 (95%), Dec 603 (98%), and Dec 604 (98%) were purchased from Toronto Research Chemical Inc. (Toronto, ON, Canada).
Dichloromethane (DCM), hexane, toluene, sulfuric acid, alumina (0.063–0.2 mm) and copper (<63 μm) were provided from Merck (Darmstadt, Germany). Al-N cartridges were obtained by Biotage (Uppsala, Sweden).
Sampling
Bizerte lagoon is the second largest lagoon in Tunisia. It is located in an important very economically part of northern Tunisia (latitude 37°80′-37°14′N; longitude 9°46′-9°56′E), and it covers an area of 138 km2 with a mean depth of 7 m (Figure 1). Menzel Abderahmen (S1), Menzel Jemil (S2), Menzel Bourguiba (S3), Faroua (S4), Maghraoua (S5), and Channel (S6) were chosen to be sediment sampling stations, whereas S1, S2, S3 and Sea Mediterranean (SM) sites were selected for eel samples. They were selected based on possible differences in contamination levels, as well as depending on the availability of fish. Three contaminated zones (S1, S2 and S3) clearly exposed to anthropogenic pressure, as well as a non-urbanized area (SM), were selected for eel sampling. S1 is the most populated station (10,000 inhabitants) receiving chronic inputs from urban runoff, and it is also surrounded by industrial units, such as electronic industries, textile fabrics, etc… S2, located near to industrial and mussel farming area, receives massive effluents from many industrial units, such as textile fabrics and electronic industries located in the city. Also, it receives chronic discharges from sewerage and urban runoff from the town of Menzel Jemil. S3 is influenced by the discharge from Ichkeul Lake. This site is characterized by the intensive activity, such as naval construction, tire production and metallurgical industry, and also it receives industrial effluents from the steel complex “El fouladh.” S4, situated in southeast part of Bizerte Lagoon, is far from industrial source of pollution, but it is influenced by agricultural inputs. S5, situated in the northwestern part of the lagoon, is also characterized by the intensive agricultural activities. Finally, S6 is characterized by industrial activities including the passage of ships and cement manufacturing. This area is affected by the urban effluents from the Bizerte city.
Sediment and eel (Anguilla anguilla) samples were collected in March 2017. Two replicates of surface sediments were collected at the six stations mentioned above, reaching 12 samples. The top 0–10 cm of sediment was collected using a stainless steel grab sampler. Sediments were freeze-dried, filtered and sieved using a 2-mm mesh and stored at 4°C until their analysis. Physicochemical parameters of sediment samples are described in Table 1. As regards eel samples, the sampling procedure and handling was largely based on European Union guidelines (OJEU, 2010). They were euthanized by cervical dislocation, and the muscle tissues of each fish were rapidly dissected and stored separately at −20°C until they were freeze-dried and homogenized with a blender. Only four stations were selected, and six specimens were sampled at each site, reaching a total of 24 eel samples. The average ± SD length (centimeter) and weight (gram) of eel collected from Bizerte lagoon were, respectively: S1 (59.6 ± 11.1; 762 ± 395), S2 (54.6 ± 6.18; 260 ± 93.7), S3 (60.4 ± 6.06; 464 ± 110), and SM (57.8 ± 13.9; 328 ± 229).
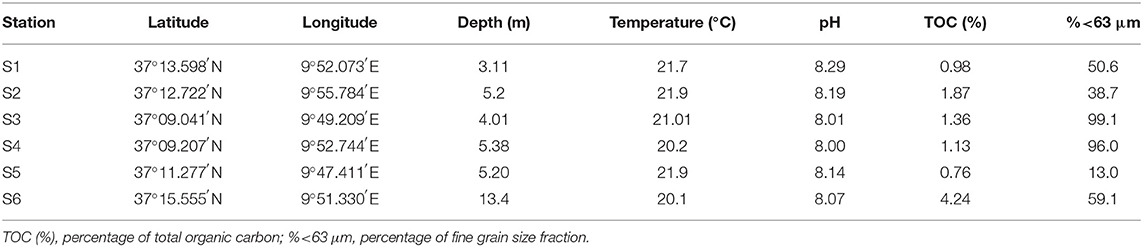
Table 1. Sampling site locations and physico-chemical properties in costal superficial sediments of Bizerte lagoon, Tunisia.
This study was carried out in accordance with the principles of the Basel Declaration and recommendations of European Union guidelines (OJEU, 2010). The fish sampling procedures and protocols have been used previously by Barhoumi et al. (2016). Before taking any sample we took the authorization from “National Agency for Navigation and Fishing Bizerte Tunisia.” The sampling was done respecting and following the instructions of the Directive 2010/63/EU of the European Parliament and of the Council of 22 September 2010 on the protection of animals for specific purposes “articles 24 and 25.” The number of collected samples was 24 fish and that was the exact number needed for our experiences and it was preapproved by the “National Agency for Navigation and Fishing Bizerte Tunisia” to collect fish from four different sampling sites to not affect the number of fish in the area. In addition, the number of eel (Anguilla anguilla) is high during the period of our sampling (March of every year) knowing that it's its period of immigration of Bizerte Lagoon.
Sample Preparation
HFR analyses in sediments were carried out with a selective pressurized liquid extraction (PLE) method, in which extraction and purification were done simultaneously (De la Cal et al., 2003). One gram dry weight (dw) of sediment was spiked with internal standards (13C-PBDE mixture and 13C-syn-DP). Spiked samples were kept overnight to equilibrate. Spiked samples were ground with alumina and copper (1:2:2) and loaded into 22 mL cell extraction. Solvent extraction was DCM:hexane (1:1). Extracts were concentrated to incipient dryness and re-dissolved with toluene until a final volume of 40 μL prior to instrumental analysis.
For OPFRs, sediment extraction was also carried out by PLE. One gram dw of sample was loaded in 22 mL extraction cell with hydromatrix and copper and extracted with hexane: acetone (1:1). Extracts were concentrated to incipient dryness and re-dissolved with methanol for obtaining 500 μL as a final volume. Prior to instrumental analysis, labeled compounds (d12-TCEP, d15-TDClPP, d27-TNBP, d15-TPHP, and 13C2-TBOEP) were added as internal standards.
Regarding HFR analyses in eel samples, 1.5 g dw was spiked with 13C-PBDE mixture and 13C-syn-DP. Samples were kept overnight in the fridge to equilibrate. Spiked samples were loaded into 11 mL extraction cell and ground with copper (1:2). A PLE was performed out using a previous optimized method (Barón et al., 2012, 2014c). After extraction, extracts were concentrated to dryness, and kept in the oven at 95°C for 2 h. Then, lipid content was determined gravimetrically. Extracts were re-dissolved in hexane and threated with sulfuric acid to remove lipids. Afterwards the organic phase was cleaned by solid phase extraction (SPE) using alumina cartridges (5g) eluted with hexane:DCM (1:1). The extracts were concentrated and reconstituted with toluene for a final volume of 40 μL.
Extraction of OPFRs from eel samples was performed by ultrasound-assisted extraction method according to Giulivo et al. (2017). 0.5 g dw were extracted twice with 15 mL of an hexane:acetone mixture (1:1). Extracts were combined and evaporated to dryness under a purified nitrogen stream, and reconstituted with hexane:methanol (1:3) up to a volume of 5 mL. Then, the solution was centrifuged and 200 μL were collected and spiked with a mixture of labeled compound (d12-TCEP, d15-TDClPP, d27-TNBP, d15-TPHP, and 13C2-TBOEP) prior to the instrumental analysis.
Total organic carbon (TOC) is an important parameter to evaluate the environmental status of aquatic ecosystems in marine sediment (Hu et al., 2008). Properties, such as TOC or the presence of other substances in the sediment are able to decrease or increase the bioavailability of the contaminants (Santín et al., 2016). Thus, the TOC value can partly influence the distribution of organic pollutants in a coastal environment. In this work, sediment TOC was calculated by colorimetry using a 702 coulomat after decarbonisation of sediment with HCl-2N at 60°C (Ouertani et al., 2006). The percentage of fine grain fraction sizes (% <63 μm) for each sediment sample was determined gravimetrically after wet sieving (Savinov et al., 2000) (see Table 1).
Chemical Analysis
Analysis of PBDEs and emerging BFRs (PBEB, HBB, and DBDPE) was carried by an Agilent 7890 gas chromatograph coupled to an Agilent 7000A GC/MS triple quadrupole. Chromatographic separation was performed with a DB-5ms column (15 m × 0.25 mm × 0.1 μm film thickness). Gas chromatography coupled to tandem mass spectrometry (GC-MS-MS) using electron ionization (EI) was applied. More details on instrumental conditions were previously published (Eljarrat et al., 2002, 2007; Barón et al., 2014c). Due to the low sensibility obtained for decabrominated compounds, BDE-209 and DBDPE were determined by GC-NCI-MS using an Agilent 6890A gas chromatograph connected to an Agilent 5975A network mass spectrometry (Eljarrat et al., 2004). HNs were also analyzed by GC-MS-MS, but using negative chemical ionization (NCI) with as reagent gas (Barón et al., 2012).
For OPFRs, an online sample purification and analysis by turbulent flow chromatography (TFC) coupled to tandem mass spectrometry (MS-MS) was performed with a Thermo Scientific TurboFlow™ system (Giulivo et al., 2016). CycloneTM−P (0.5 × 50 mm) and C18-XL (0.5 × 50 mm) columns were used for purification, whereas Purosphere Star RP-18 (125 × 0.2 mm) column was used for chromatographic separation. Spectrometric analysis was carried with a triple quadrupole with a heated-electrospray ionization source.
Selective reaction monitoring (SRM) mode was used with two transitions monitored for each compound. The most intense transitions were used for quantification purposes, and the second ones for confirmation criteria.
Quality Control
Identification of analytes was based on the following criteria: (i) simultaneous responses for the two monitored transitions must be obtained at the same retention time than those of available standards; (ii) signal-to-noise ratios must be >3; and (iii) relative peak intensity ratio must be within ±20% of the theoretical values obtained with standard solutions.
Analytical parameters, such as recoveries, limits of detection (LODs) and limits of quantification (LOQ) are summarized in Table SI 1.
Estimated Daily Intake and Risk Evaluation
The human exposure to HFRs and OPFRs through the consumption of eels was evaluated by calculating the estimated daily intake (EDI), expressed in ng/kg body weight/day. The calculation was based on a body weight (BW) of 60.0 kg (Ben Ameur et al., 2013) and a daily fish consumption (DFC) of 27 g/day, a value obtained from the International Institute of Nutrition and Food Technology (http://www.institutdenutrition.rns.tn). The hazard quotient (HQ) and the cancer risk (CR) were calculated for the target compounds using the methodology described by Staskal et al. (2008) and by Ni et al. (2012).
Statistical Analysis
Statistical treatment of obtained data was performed with STATISTICA (version 6). Pearson's correlation coefficient was calculated to examine the possible relationship between parameters; statistical significance was accepted at p < 0.05. In box plot figures, outliers (×) were calculated as values above Q3−1.5 IQR and below Q1−1.5 IQR (Q3 = third quartile, IQR = interquartile range, Q1 = first quartile). Two sample t-tests were carried out, using the GraphPad prism 5 program, to determine significant difference (p ≤ 0.05).
Results and Discussion
Sediment Samples
Table SI 2 summarizes the concentration levels, expressed in ng/g dw, of HFRs and OPFRs detected in sediment samples from Bizerte Lagoon, Tunisia. For results of individual compounds in each sediment sample, see Table SI 3.
HFRs
HFRs were detected in all sediment samples at concentration levels ranging from 3.30 to 28.5 ng/g dw, being S5 and S6 the most contaminated sites. PBDEs were detected in all analyzed samples, between 1.77 and 19.1 ng/g dw, whereas emerging BFRs were not detected in any sample. HNs were also detected in all the samples, except for one, at contamination levels between not detected (nd) and 14.2 ng/g dw.
PBDE concentrations
Mean value of ∑PBDEs in sediment samples was 7.86 ng/g dw. The highest concentration value corresponded to S5 (mean value of 14.0 ng/g dw), followed by S6 (mean value of 8.04 ng/g dw), and then by S3, S2 and S1 (mean values of 7.55, 7.19, and 5.38 ng/g dw, respectively). Relatively low concentration was detected at the selected station far from urban and industrial areas, S4 (mean value of 5.05 ng/g dw). Of the 8 different PBDE congeners included in the study, 7 were detected in samples: BDE-28, −47, −99, −100, −154, −153, and −209, with ∑PBDE levels ranging from 5.05 to 14.0 ng/g lw. PBDE congener pattern was dominated by BDE-209, with an average contribution of 83% over total PBDEs. Similar PBDE pattern in sediment samples has been previously described in different works (Lee et al., 2018; Zhu et al., 2018).
There is a lack of information regarding occurrence of PBDEs in sediments from the Bizerte Lagoon. There are some published studies focused on other pollutants, such as PCBs (Derouiche et al., 2004) or PAHs (Barhoumi et al., 2014c), from the same area. PCB levels varied from 0.89 to 6.63 ng/g dw being similar to those obtained in our study for PBDEs (1-79-19.1 ng/g dw), whereas concentrations of PAHs were higher ranging from 16.9 to 394 ng/g dw. In our work, the analysis of PBDEs in surface sediment show a difference on concentration values with a recent published study reported by Mekni et al. (2019), which focused on the analysis of the same compound in the same sampling points. The concentration levels obtained in the previous work (∑PBDEs mean value, 5.51 ng/g dw), were similar but slightly lower than those obtained in this study (∑PBDEs mean value, 7.86 ng/g dw). The increase of level of PBDEs is probably explained by the appearance of other source of pollution, such as urban or industrial in the studied area.
If we compare our results with those recently reported in sediments from other locations around the world, similar levels were found in Danshui river basin (Taiwan) (2.30–10.5 ng/g dw) and Awash river basin (Ethiopia) (3.71–19.0 ng/g dw) (Cheng and Ko, 2018; Chai et al., 2019). However, our results are lower compared to samples collected in South China sea, with levels between 8.09 and 596 ng/g dw (Zhu et al., 2018), and in Peal river estuary, with levels ranging from 1.25 to 206 ng/g dw (Zhang et al., 2015).
HN concentrations
Dec 602, Dec 603, Dec 604, and both DP isomers (syn-DP and anti-DP) were detected in all sediment, with ∑HNs mean value of 2.69 ng/g dw. Similarly to PBDEs, S4 was the least contaminated site by HNs confirming that this station is located far of industrial and agricultural sources of pollution. S6 represented the most polluted site (mean value of 9.06 ng/g dw) followed by S1 (mean value of 3.16 ng/g dw), S2 and S3 (mean values of 1.60 and 1.57 ng/g dw, respectively) and S5 (mean value of 0.54 ng/g dw). Dec 602 was the most dominant compound, with levels up to 3.99 ng/g dw. In previous studies, Dec 602, Dec 603, Dec 604, and DP were also detected in all surface sediment samples from the Great Lakes (Shen et al., 2010) and from the Arctic Circle (Na et al., 2015). In case of both DP isomers were detected, Fanti defined as concentration of anti-DP divided by the sum of concentration of syn-DP and anti-DP, was calculated. Fanti values ranged from 0.23 to 0.64, being lower than value in commercial DP products, between 0.64 and 0.80 (Hoh et al., 2006) and suggesting a stereo selective enrichment of syn-DP isomer in the environment (Zhu et al., 2018).
Our ∑HNs ranged between nd and 14.2 ng/g dw, being comparable to values obtained in sediment from Chenab River, Pakistan (0.1–12.5 ng/g dw) (Mahmood et al., 2015), and slightly lower than in surface sediment from Jiulong River Estuary, southeast China (9.3–36.2 ng/g dw) (Chen et al., 2018).
OPFRs
OPFRs were also detected in all sediment samples at concentration levels ranging from 9.77 to 164 ng/g dw, with a mean value of 53.6 ng/g dw. S6 is the most contaminated site (mean value of 136 ng/g dw), followed by S2 (mean value of 52.0 ng/g dw), and then by S1, S5, S3, and S4 (mean values of 41.0, 38.6, 28.1, and 26.3 ng/g dw, respectively).
The fourteen OPFRs included in our analytical methodology were detected in sediment samples, with the exception of IDPP which was not detected in any sample. Moreover, THP was detected, but always at concentration levels below limit of quantification. TPPO presented the highest concentration values (mean value of 15.3 ng/g dw), followed by TPHP (mean value of 12.3 ng/g dw) and then TClPP, TNBP, and EHDPP (mean value of 8 ng/g dw, each one).
Until now, only limited studies have reported OPFR levels in sediments. Our results were higher than those published in sediments from Hainan Island, south China (0.74–60 ng/g dw) (Mo et al., 2019) or sediments from Bohai and Yellow Seas, China (0.08–4.55 ng/g dw) (Zhong et al., 2018). Lower OPFR values were found in ocean sediments from the North Pacific to the Arctic Ocean (0.16–0.46 ng/g dw) (Ma et al., 2017).
If we compare our sediments FR results, we observed that OPFR levels were higher than HFR concentrations (Figure 2). Moreover, student's t-test showed significant statistical differences between OPFRs and HFR (t = 3.83, df = 17, p < 0.05). There are not many published studies with HFR and OPFR values in the same series of samples. Similar findings were observed by Brandsma et al. (2015) in their study on the Western Scheldt estuary (The Netherlands). In the abiotic compartments (sediment and suspended particular matter) they found that OPFR concentrations were often higher than those of PBDEs. Similarly, Giulivo et al. (2017) analyzed sediments collected from three European river basins, Evrotas, Adige and Sava. HFR levels ranged between 0.25 and 34.0 ng/g dw, whereas OPFR concentrations were between 0.31 and 549 ng/g dw.
Sediment Geochemistry and HFR and OPFR Accumulation
Some sediment parameters, such as TOC and fine fraction percentages can influence the concentration levels of organic contaminants. The TOC and fine fraction percentages in surface sediments from the Bizerte Lagoon ranged from 0.76 to 4.24% and from 13.0 to 99.1%, respectively (Table 1). S6 showed the highest content of TOC (4.24%), which can be related to the massive occurrence of industrial and urban wastewater discharges, that are rich in organic matter. Regression analysis was carried out to examine the relationship between the percentage of TOC and the concentrations of ∑HFRs and ∑OPFRs. No significant correlation was observed between ∑HFRs and TOC (p > 0.05), suggesting that HFR distribution is more influenced by direct industrial discharge rather than organic matter content in sediment.
As regards OPFRs, significant correlation was observed between ∑OPFRs and TOC (p-values < 0.05), suggesting that OPFR distribution is influenced by the organic matter content in sediment, or/and the distribution of OPFRs may be controlled mainly by the distribution of organic matter in the sediments, which is in agreement with previous reports (He et al., 2019).
Eel Samples
Table SI 4 summarizes the concentration levels, expressed in ng/g lw, of HFRs and OPFRs obtained in eel sample from Bizerte Lagoon, Tunisia. For results of individual compounds in each eel sample, see Table SI 5.
HFRs
HFRs were detected in all eel samples at concentration levels ranging from 4.72 to 151 ng/g lw, being S1 the most contaminated site. The high concentration in this site can be attributed to the total length and weight of eel, which was significantly greater in this station. PBDEs were detected in all analyzed samples, between 3.03 and 59.0 ng/g lw, whereas emerging BFRs were not detected in any sample. HNs were also detected in some samples, at contamination levels between nd and 103 ng/g lw.
PBDE concentrations
Mean concentration of ∑PBDEs was 10.8 ng/g lw. Eight different PBDEs (BDE-28, BDE-47, BDE-100, BDE-99, BDE-154, BDE-153, BDE-183, and BDE-209) were detected in eel samples. BDE-47 was the predominant congener, followed by BDE-99 and BDE-100. This PBDE congener pattern was consistent with Penta-BDE commercial mixture contamination. The predominance BDE-47 is consistent with the general pattern found in other published works with eel samples (see references in Table 2), with the exception of eel samples from Irish waters (McHugh et al., 2010), which were exposed to Octa-BDE mixture rather than Penta-BDE. BDE-209, which represented the most prevalent PBDE congener in sediments, was not detected or present at low levels (from nq to 4.58 ng/g lw) in eel samples due to their very low bioavailability (log KOW ≈ 10). However, some studies reported the predominance of this high brominated congener in bivalves from Korean coastal waters (Moon et al., 2007), or high levels (from 10 to 87 ng/g lw) in eels from Flanders (Belgium) (Roosens et al., 2010).
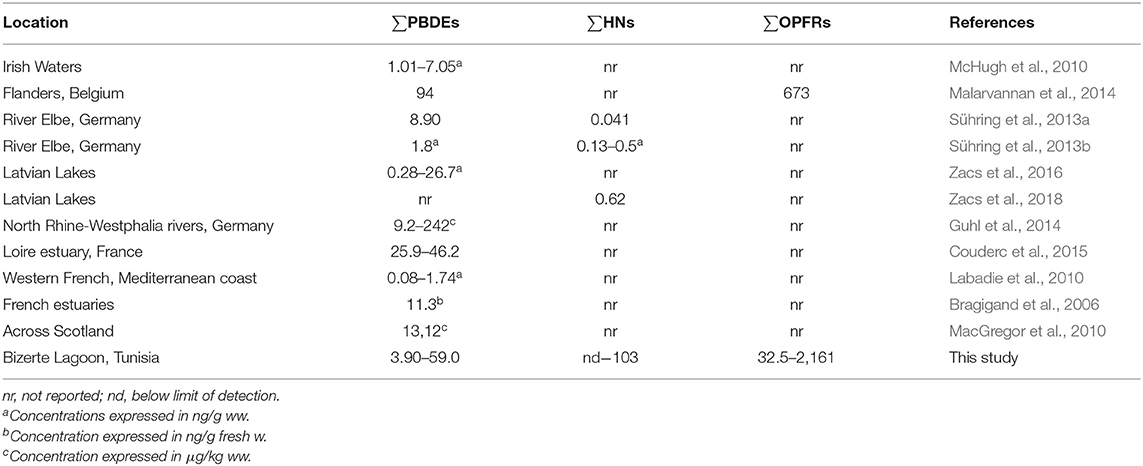
Table 2. PBDE, HN, and OPFR range of concentrations or mean values (expressed in ng/g lw) found in European eel samples collected around the world.
∑PBDE levels observed in this study were compared with previous data in eel samples collected around the world (Table 2). Our results were similar to those found in samples collected in Loire estuary (between 25.9 and 46.2 ng/g lw) (Couderc et al., 2015), whereas they were lower than those reported by Guhl et al. (2014) in North Rhine-Westphalia rivers, with values up to 242 ng/g lw, or those published by Malarvannan et al. (2014) in Belgium, with a mean value of 94 ng/g lw.
HN concentrations
As regards HNs, their frequency of detection was slightly lower than that of PBDEs: PBDEs were detected in the 100% of analyzed samples, whereas HNs were found in the 88% of samples. The four compounds included in the analytical work were detected being Dec 604 the most times detected (75%), followed by DP (67%) and finally Dec 602 and Dec 603 (54%). However, the highest concentrations corresponded to Dec 602, with values between nd and 85.4 ng/g lw. HN concentrations varied depending on the sampling station, with S1 and S2 presenting the higher concentrations with mean values of 57.4 and 25.6 ng/g lw, respectively. Levels in SM were lower, with a mean value of 16.7 ng/g lw, corresponding with the situation of this site located far from industrial and agricultural areas. On the other hand, and despite his position near to urban and industrial areas, S3 has the lowest HN levels with a mean value of 2.41 ng/g lw, proving the limited use of HNs in this area.
There are few available studies reporting HN levels in eel samples collected in different locations of the world (Table 2). However, our results were always much higher than those previously published for samples collected in Germany (mean values between 0.04 and 0.13 ng/g lw) (Sühring et al., 2013a, 2016) or samples from Latvian lakes (mean value of 0.62 ng/g lw) (Zacs et al., 2018).
In addition for sediment samples, Fanti values have been also calculated for eel samples. Fanti values ranged from 0.50 to 0.53, being lower than values in commercial DP products, between 0.64 and 0.80 (Hoh et al., 2006) and suggesting that anti-DP can be easily degradable or that syn-DP is more bioaccumulative than anti-DP.
OPFRs
The average ± SD (∑OPFRs) concentration in eel samples from Bizerte lagoon was 404 ng/g lw (± 367). OPFRs were detected in all eel samples at concentration levels ranging from 19.7 to 2,154 ng/g lw, being S2 the most contaminated site (mean value of 842 ng/g lw), followed by S1 (mean value of 574 ng/g lw) and S3 (mean value of 111 ng/g lw). Finally, the lower concentrations were found at site SM (mean value of 90.7 ng/g lw).
Thirteen of the 14 OPFRs included in our analytical methodology were detected in at least some eel sample. However, THP was not detected in any sample. TClPP, TDClPP, TPHP, TNBP and DCP were detected in all analyzed eel samples. However, TMCP presented the highest levels (mean value of 436 ng/g lw), followed by EHDPP (mean value of 309 ng/g lw), IDPP (mean value of 290 ng/g lw), DCP (mean value of 206 ng/g lw), IPPP (mean value of 111 ng/g lw), TPHP (mean value of 106 ng/g lw), and TPPO (mean value of 88.2 ng/g lw).
Until now, only one study has reported OPFR levels in eel samples collected in Flanders, Belgium (Malarvannan et al., 2015), with values slightly higher than those found in our study, with a mean value of 673 ng/g lw.
Similarly to sediment samples, OPFRs were significantly higher than those HFRs in eel (t = 3.47, df = 17, p < 0.05) (Figure 2). The ratio between mean values of OPFRs and HFRs in eels from different sites ranged between 3.1 and 26, whereas for sediments these ratios were slightly lower, between 2.7 and 8.0. Higher bioaccumulation potential of HFRs vs. OPFRs has been previously described (Giulivo et al., 2017), as well as limited OPFR biomagnification through food web (Hallanger et al., 2015) probably due to biotransformation processes (Strobel et al., 2018). Thus, the higher ratio observed in eel samples could be a result of an additional OPFR source of exposure. Recently, two other studies in marine environment showed the same behavior, with OPFR levels higher than those of PBDEs in dolphin tissue samples from the Mediterranean (Sala et al., 2019) and Indian Ocean (Aznar-Alemany et al., 2019). One hypothesis would be related to the fact that OPFRs are also used as plasticizers, antifoaming agents and performance additives in consumer products. Precisely, its use as plasticizers as well as the large amount of microplastics both in continental and marine waters could also contribute to the OPFR levels found in eel samples. Microplastics come from their manufacturing for various applications, such as their use in cosmetics, toothpaste, hand soap and cleaning products. On the other hand, when plastics reach the sea, they are fragmented into microplastics by the action of sunlight and waves. Once the microplastics are ingested by the organism, the animal can accumulates the chemical compounds associated with the plastic in their tissues. However, additional studies are needed to evaluate the extent to which plastics transfer additives to organisms after ingestion and to verify if high OPFR levels in biota are due to this source of contamination.
Risk Assessment of Human Exposure
In order to understand the magnitude of the contamination in eel samples through consumption, we calculated the EDI values for the target compounds and for the general population in Bizerte. EDI values of 0.92, 2.18, and 34.5 ng/kg/day for PBDE, HNs, and OPFRs, respectively, were obtained (Table 3). In order to put the results in a more concrete context, it is important to calculate HQ and CR to screen the potential health risk associated to the consumption of eels from the Bizerte Lagoon.
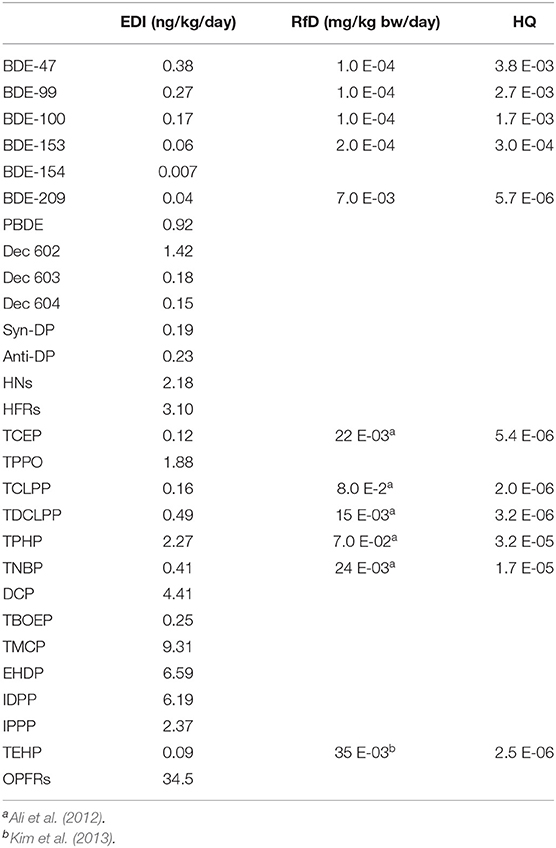
Table 3. Calculated of estimated daily intakes (EDI) and hazard quotient (HQ) by consumption of eel (Anguilla Anguilla) from Bizerte Lagoon.
The literature about the dietary intakes of the selected pollutants are very scarce. In this study, dietary intakes for PBDEs was 55.2 ng/day, moderately lower than those obtained for fish (Solea solea) consumption in the same area of study (64.3 ng/day) (Ben Ameur et al., 2013). However, EDI of PBDEs in Bizerte are higher than those from USA, 8.94–15.7 ng/day (Schecter et al., 2006), Italy 20.9 ng/day (Martellini et al., 2016), or Finland, 23 ng/day (Kiviranta et al., 2004). On the other hand, a previous published work also reported lower dietary intake in swordfish from Spain (0.06 ng/day) (Domingo et al., 2006). Regarding HNs, our dietary intake of 131 ng/day was higher than that reported by Mekni et al. (2019) in sea urchin (42 ng/day) from the same area of Bizerte lagoon, Tunisia, and in fish (144 pg/day) from Japan (Kakimoto et al., 2012). Finally, our dietary intake for OPFRs was 2,070 ng/day, a value higher than that obtained by Soumis et al. (2003) in fish from Amazon River (990 ng/day).
The RfD is an estimate dose of a daily exposure to the human population that is likely to be without an appreciable risk of deleterious effects, and it is expressed in mg/kg/day units. EDI values obtained in this investigation ranged between 7.0 E-03 and 0.38 ng/kg/day for PBDEs, between 0.15 and 1.42 ng/kg/day for NHs and between 9.0 E-02 and 9.31 ng/kg/day for OPFRs. The U.S. EPA (2008) proposed an oral RfD between 2.0 E-04 and 7.0 E-03 mg/kg/day, for BDE-47, BDE-99, BDE 100, BDE-153, and BDE-209 congeners (Table 3). As regards OPFRs, oral RfD lying between 35 E-03 and 8.0 E-02 mg/kg/day for TCEP, TCLPP, TDCLPP, TPHP, TNBP and TEHP congeners. Oral RfD values are much higher than our EDI values, suggesting that the consumption of eels will not likely pose any health risks to consumers.
As far as, when the HQ value >1, indicate the potential risk of exposure for humans (Asante et al., 2011; Ni et al., 2012). In this present study, the HQ results are all below 1 suggesting a low deleterious risk to PBDEs and OPFRs through the consumption of eel from Bizerte city. Similar results were recently obtained by Mekni et al. (2019) for sea urchin (Paracentrotus lividus), clams (Ruditapes decussatus) (El Megdiche et al., 2017), and in fish (Solea solea) (Ben Ameur et al., 2013) from the Bizerte Lagoon, Tunisia. Around the word, our HQ values are also similar to those reported in fish and mussels from Italy (Martellini et al., 2016) and in seafood from chine (Miyake et al., 2008; Ni et al., 2012).
BDE-209 presented the only congener for which information is available to evaluate its carcinogenicity. In this case, our CR values ranged from 4.7 × 10−9 and 6.3 × 10−8, being slightly lower than those obtained in sea urchin (Mekni et al., 2019), and in clam (El Megdiche et al., 2017) from the Bizerte Lagoon. According to CR values obtained in this study, PBDEs in eel from Bizerte Lagoon had no threat to the human health [acceptable increased cancer risk ranges between 1 × 10−6 and 1 × 10−4, (Ni et al., 2012)]. From all these results, it can be concluded no adverse health effects (carcinogenic or non-carcinogenic) are associated with the consumption of eel from the Bizerte lagoon.
Conclusions
This work is one of few studies including the occurrence of two families of FRs, halogenated and organophosphates, in sediment and eel samples (Anguilla anguilla) collected from Bizerte Lagoon. In sediment samples, PBDEs were the main contributors to HFRs of contamination. While, in eel the levels of HNs were higher than PBDEs. As regards OPFRs, they were found in all samples with higher levels than HFRs in both, sediment and eel samples. Our statistically significant correlation between TOC content and OPFRs demonstrated that sediment has the capacity to accumulate more OPFRs than HFRs. The higher ratio between OPFR and HFR levels observed in eel samples could be a result of an additional OPFR source of exposure, probably due to their use also as plasticizers and the large amount of microplastics both in continental and marine waters. Our risk evaluation of eel consumption suggests that there is not significant human health risk. Dietary exposures to PBDEs and OPFRs were much lower than the respective health based guidance values.
Data Availability Statement
All datasets generated for this study are included in the article/Supplementary Material.
Author Contributions
SM conceived the study and wrote the manuscript with inputs from BB, ST, MD, and EE. EE provided the methodology, validation, resources, supervision, and funding. All authors contributed to manuscript revision, read, and approved the submitted version.
Funding
This study was supported by the Spanish Ministry of Science and Innovation (Project CEX2018- 000794-S), by the Generalitat de Catalunya (Consolidated Research Group Water and Soil Quality Unit 2017 SGR 01404), and by the Tunisian Ministry of Higher Education and Scientific Research.
Conflict of Interest
The authors declare that the research was conducted in the absence of any commercial or financial relationships that could be construed as a potential conflict of interest.
Supplementary Material
The Supplementary Material for this article can be found online at: https://www.frontiersin.org/articles/10.3389/fenvs.2020.00067/full#supplementary-material
References
Alaee, M., Arias, P., Sjödin, A., and Bergman, A. (2003). An overview of commercially used brominated flame retardants, their applications, their use patterns in different countries/regions and possible modes of release. Environ. Int. 29, 683–689. doi: 10.1016/S0160-4120(03)00121-1
Ali, N., Dirtu, A. C., den Eede, N. V., Goosey, E., Harrad, S., Neels, H., et al. (2012). Occurrence of alternative flame retardants in indoor dust from New Zealand: indoor sources and human exposure assessment. Chemosphere 88, 1276–1282. doi: 10.1016/j.chemosphere.2012.03.100
Asante, K. A., Adu-Kumi, S., Nakahiro, K., Takahashi, S., Isobe, T., Sudaryanto, A., et al. (2011). Human exposure to PCBs, PBDEs and HBCDs in Ghana: temporal variation, sources of exposure and estimation of daily intakes by infants. Environ. Int. 37, 921–928. doi: 10.1016/j.envint.2011.03.011
Aznar-Alemany, O., Sala, B., Plön, S., Bouwman, H., Barceló, D., and Eljarrat, E. (2019). Halogenated and organophosphorus flame retardants in cetaceans from the southwestern Indian ocean. Chemosphere 226, 791–799. doi: 10.1016/j.chemosphere.2019.03.165
Barhoumi, B., El Megdiche, Y., Clérandeau, C., Ben Ameur, W., Mekni, S., Bouabdallah, S., et al. (2016). Occurrence of polycyclic aromatic hydrocarbons (PAHs) in mussel (Mytilus galloprovincialis) and eel (Anguilla anguilla) from bizerte lagoon, Tunisia, and associated human health risk assessment. Cont. Shelf Res. 124, 104–116. doi: 10.1016/j.csr.2016.05.012
Barhoumi, B., Le Menach, K., Clérandeau, C., Ben Ameur, W., Budzinski, H., Driss, M. R., et al. (2014a). Assessment of pollution in the bizerte lagoon (Tunisia) by the combined use of chemical and biochemical markers in mussels, Mytilus galloprovincialis. Mar. Pollut. Bull. 84, 379–390. doi: 10.1016/j.marpolbul.2014.05.002
Barhoumi, B., Le Menach, K., Devier, M-H., Elmegdiche, Y., Hammami, B., Ben Ameur, W., et al. (2014b). Distribution and ecological risk of polychlorinated biphenyls (PCBs) and organochlorine pesticides (OCPs) in surface sediments from the Bizerte Lagoon, Tunisia. Environ. Sci. Pollut. Res.21, 6290–6302. doi: 10.1007/s11356-013-1709-7
Barhoumi, B., Le Menach, L., Devier, M.H., Ben Ameur, W., Etcheber, H., Budzinski, H., et al. (2014c). Polycyclic aromatic hydrocarbons (PAHs) in surface sediments from the bizerte lagoon, Tunisia: levels, sources, and toxicological significance. Environ. Monit. Assess. 186, 2653–2669. doi: 10.1007/s10661-013-3569-5
Barón, E., Eljarrat, E., and Barceló, D. (2012). Analytical method for the determination of halogenated norbornene flame retardants in environmental and biota matrices by gas chromatography coupled to tandem mass spectrometry. J. Chromatogr. A 1248, 154–160. doi: 10.1016/j.chroma.2012.05.079
Barón, E., Eljarrat, E., and Barceló, D. (2014c). Gas chromatography/tandem mass spectrometry method for the simultaneous analysis of 19 brominated compounds in environmental and biological samples. Anal. Bioanal. Chem. 406, 7667–7676. doi: 10.1007/s00216-014-8196-7
Barón, E., Máñez, M., Andreu, A., Sergio, F., Hiraldo, F., Eljarrat, E., et al. (2014b). Bioaccumulation and biomagnification of emerging and classical flame retardants in bird eggs of 14 species from doñana natural space and surrounding areas (South-western Spain). Environ. Int. 68, 118–126. doi: 10.1016/j.envint.2014.03.013
Barón, E., Santín, G., Eljarrat, E., and Barceló, D. (2014a). Occurrence of classical and emerging flame retardants in sediment and sludge samples from the Ebro and Llobregat river basins (Spain). J. Hazard. Mat. 265, 288–295. doi: 10.1016/j.jhazmat.2013.10.069
Ben Ameur, W., El Megdiche, Y., Eljarrat, E., Ben Hassine, S., Badreddine, B., Trabelsi, S., et al. (2013). Organochlorine and organobromine compounds in a benthic fish (Solea solea) from bizerte lagoon (NorthernTunisia): implications for human exposure. Ecotox. Environ. Saf. 88, 55–64. doi: 10.1016/j.ecoenv.2012.10.021
Ben Hasine, S., Ben Ameur, W., Eljarrat, E., Barceló, D., Touil, S., and Driss, M. R. (2015). Methoxylated polybrominated diphenyl ethers (MeO-PBDE) in human milk from Bizerte, Tunisia. Environ. Res. 138, 32–37. doi: 10.1016/j.envres.2015.01.016
Bragigand, B., Amiard-Triquet, C., Parlier, E., Boury, P., Marchand, P., and El Hourch, M. (2006). Influence of biological and ecological factors on the bioaccumulation of polybrominated diphenyl ethers in aquatic food webs from French estuaries. Sci. Total Environ. 368, 615–626. doi: 10.1016/j.scitotenv.2006.04.001
Brandsma, S. H., Leonards, P. E., Leslie, H. A., and De Boer, J. (2015). Tracing organophosphorus and brominated flame retardants and plasticizers in an estuarine food web. Sci. Total Environ. 505, 22–31. doi: 10.1016/j.scitotenv.2014.08.072
Cao, S., Zeng, X., Song, H., Li, H., Yu, Z., Sheng, G., et al. (2012). Levels and distributions of organophosphate flame retardants and plasticizers in sediment from Taihu lake, China. Environ. Toxicol. Chem. 31, 1478–1484. doi: 10.1002/etc.1872
Castro-Jiménez, J., Barhoumi, B., Paluselli, A., Tedetti, M., Jimenez, B., Munoz-Arnanz, J., et al. (2017). Occurrence, loading, and exposure of atmospheric particle-bound POPs at the African and European edges of the Western Mediterranean sea. Environ. Sci. Technol. 51, 13180–13189. doi: 10.1021/acs.est.7b04614
Chai, M., Ding, H., Shen, X., and Li, R. (2019). Contamination and ecological risk of polybrominated diphenyl ethers (PBDEs) in surface sediments of mangrove wetlands: a nationwide study in China. Environ. Pollut. 249, 992–1001. doi: 10.1016/j.envpol.2019.02.044
Chen, X., Zhu, Y., Huang, Q., Liu, J., Liu, B., Zhang, Y., et al. (2018). Distributions, influencing factors, and risk assessment of dechlorane plus and related compounds in surficial water and sediment from the Jiulong river Estuary, Southeast China. Environ. Sci. Pollut. Res. 25, 30292–30300. doi: 10.1007/s11356-018-2874-5
Cheng, J. O., and Ko, F. C. (2018). Occurrence of PBDEs in surface sediments of metropolitan rivers: sources, distribution pattern, and risk assessment. Sci. Total Environ. 637-638, 1578–1585. doi: 10.1016/j.scitotenv.2018.05.075
Couderc, M., Poirier, L., Zalouk-Vergnoux, A., Kamari, A., Blanchet-Letrouvé, I., Marchand, P., et al. (2015). Occurrence of POPs and other persistent organic contaminants in the European eel (Anguilla anguilla) from the Loire estuary, France. Sci. Total Environ. 505, 199–215. doi: 10.1016/j.scitotenv.2014.09.053
Cristale, J., and Lacorte, S. (2013). Development and validation of a multi residue method for the analysis of polybrominated diphenyl ethers, new brominated and organophosphorus flame retardants in sediment, sludge and dust. J. Chromatogr. A 1305, 267–275. doi: 10.1016/j.chroma.2013.07.028
De la Cal, A., Eljarrat, E., and Barceló, D. (2003). Determination of 39 polybrominated diphenyl ether congeners in sediment samples using fast selective pressurized liquid extraction and purification. J. Chromatogr. A 1021, 165–173. doi: 10.1016/j.chroma.2003.09.023
De Wit, C. A. (2002). An overview of brominated flame retardants in the environment. Chemosphere 46, 583–624. doi: 10.1016/S0045-6535(01)00225-9
Derouiche, A., Sanda, Y. G., and Driss, M. R. (2004). Polychlorinated biphenyls in sediments from Bizerte lagoon, Tunisia. Bull. Environ. Contam. Toxicol. 73, 810–817. doi: 10.1007/s00128-004-0499-5
Domingo, J. L., Bocio, A., Falcó, G., and Llobet, J. M. (2006). Exposure to PBDEs and PCDEs associated with the consumption of edible marine species. Environ. Sci. Technol. 40, 4394–4399. doi: 10.1021/es060484k
El Megdiche, Y., Ben Ameur, W., Hammami, B., Ben Hassine, S., Barhoumi, B., Touil, S., et al. (2017). Anthropogenic (PBDE) and naturallyproduced (MeO-PBDE) brominated compound levels in Bizerte lagoon clam (Ruditapes decussatus): levels and human health risk assessment. Mar. Pollut. Bull. 125, 176–185. doi: 10.1016/j.marpolbul.2017.08.005
Eljarrat, E., de la Cal, A., and Barceló, D. (2004). Determination of decabromodiphenyl ether in sediments using selective pressurized liquid extraction followed by GC-NCI-MS. Anal. Bioanal. Chem. 378, 610–614. doi: 10.1007/s00216-003-2220-7
Eljarrat, E., Labandeira, A., Marsh, G., Raldúa, D., and Barceló, D. (2007). Decabrominated diphenyl ether in river fish and sediment samples collected downstream an industrial park. Chemosphere 69, 1278–1286. doi: 10.1016/j.chemosphere.2007.05.052
Eljarrat, E., Lacorte, S., and Barceló, D. (2002). Optimization of congener-specific analysis of 40 polybrominated diphenyl ethers by gas chromatography/mass spectrometry. J. Mass Spectrom. 37, 76–84. doi: 10.1002/jms.262
Gauthier, L. T., Hebert, C. E., Weseloh, D. V. C., and Letcher, R. J. (2007). Current-use flame retardants in the eggs of herring gulls (Larus argentatus) from the Laurentian great lakes. Environ. Sci. Technol. 41, 4561–4567. doi: 10.1021/es0630487
Giulivo, M., Capri, E., Eljarrat, E., and Barceló, D. (2016). Analysis of organophosphorus flame retardants in environmental and biotic matrices using on-line turbulent flow chromatography-liquid chromatography-tandem mass spectrometry. J. Chromatogr. A 1474, 71–78. doi: 10.1016/j.chroma.2016.10.042
Giulivo, M., Capri, E., Kalogianni, E., Milacic, R., Majone, B., Ferrari, F., et al. (2017). Occurrence of halogenated and organophosphate flame retardants in sediment and fish samples from three European river basins. Sci. Tot. Environ. 586, 782–791. doi: 10.1016/j.scitotenv.2017.02.056
Greaves, A. K., Letcher, R. J., Chen, D., McGoldrick, D. J., Gauthier, L. T., and Backus, S. M. (2016). Retrospective analysis of organophosphate flame retardants in herring gull eggs and relation to the aquatic food web in the Laurentian great lakes of North America. Environ. Res. 150, 255–263. doi: 10.1016/j.envres.2016.06.006
Guhl, B., Stürenberg, F. J., and Santora, G. (2014). Contaminant levels in the European eel (Anguilla anguilla) in North Rhine-Westphalia rivers. Environ. Sci. Eur. 26:26. doi: 10.1186/s12302-014-0026-1
Guo, J., Venier, M., Salamova, A., and Hites, R. A. (2017). Bioaccumulation of dechloranes, organophosphate esters, and other flame retardants in Great lakes fish. Sci. Total Environ. 583, 1–9. doi: 10.1016/j.scitotenv.2016.11.063
Hallanger, I. G., Sagerup, K., Evenset, A., Kovacs, K. M., Leonards, P., Fuglei, E., et al. (2015). Organophosphorous flame retardants in biota from Svalbard, Norway. Mar. Pollut. Bull. 101, 442–447. doi: 10.1016/j.marpolbul.2015.09.049
He, J., Li, J., Ma, L., Wu, N., Zhang, Y., and Niu, Z. (2019). Large-scale distribution of organophosphate esters (flame retardants and plasticizers) in soil from residential area across China: implications for current level. Sci. Total Environ. 697, 1339–13397. doi: 10.1016/j.scitotenv.2019.133997
Herrero, A., Vila, J., Eljarrat, E., Ginebreda, A., Sabater, S., Batalla, R., et al. (2018). Transport of sediment borne contaminants in a Mediterranean river during a high flow event. Sci. Total Environ. 633, 1392–1402. doi: 10.1016/j.scitotenv.2018.03.205
Hoh, E., Zhu, L., and Hites, R. A. (2006). Dechlorane plus, a chlorinated flame retardant, in the Great lakes. Environ. Sci. Technol. 40, 1184–1189. doi: 10.1021/es051911h
Hu, G., Xu, Z., Dai, J., Mai, B., Cao, H., Wang, J., et al. (2010). Distribution of polybrominated diphenyl ethers and decabromodiphenyl ethane in surface sediments from Fuhe river and Baiyangdian lake, North China. J. Environ. Sci. 22, 1833–1839. doi: 10.1016/S1001-0742(09)60328-4
Hu, J., Liu, W. X., Chen, J. L., Fan, Y. S., Xing, B. S., Kang, H., et al. (2008). Distribution and property of polycyclic aromatic hydrocarbons in littoral surface sediments from the Yellow sea, China. J. Environ. Sci. Health 43, 382–389. doi: 10.1080/10934520701795582
Kakimoto, K., Nagayoshi, H., Yoshida, J., Akutsu, K., Konishi, Y., Toriba, A., et al. (2012). Detection of dechlorane plus and brominated flame retardants in marketed fish in Japan. Chemosphere 89, 416–419. doi: 10.1016/j.chemosphere.2012.05.072
Kim, J. W., Isobe, T., Sudaryanto, A., Malarvannan, G., Chang, K. H., Muto, M., et al. (2013). Organophosphorus flame retardants in house dust from the Philippines: occurrence and assessment of human exposure. Environ. Sci. Pollut. Res. 20, 812–822. doi: 10.1007/s11356-012-1237-x
Kiviranta, H., Ovaskainen, M-L., and Vartiainen, T. (2004). Market basket study on dietary intake of PCDD/Fs, PCBs, and PBDEs in Finland. Environ. Int. 30, 923–932. doi: 10.1016/j.envint.2004.03.002
Labadie, P., Alliot, F., Bourges, C., Desportes, A., and Chevreuil, M. (2010). Determination of polybrominated diphenyl ethers in fish tissues by matrix solid-phase dispersion and gas chromatography coupled to triple quadrupole mass spectrometry: case study on European eel (Anguilla anguilla) from Mediterranean coastal lagoons. Anal. Chim. Acta. 675, 97–105. doi: 10.1016/j.aca.2010.07.010
Lee, H. J., Jeong, H. J., Jang, Y. L., and Kim, J. B. (2018). Distribution, accumulation, and potential risk of polybrominated diphenyl ethers in the marine environment receiving effluents from a sewage treatment plan. Mar. Pollut. Bull.129, 364–369. doi: 10.1016/j.marpolbul.2018.02.050
Ma, Y., Xie, Z., Lohmann, R., Mi, W., and Gao, G. (2017). Organophosphate ester flame retardants and plasticizers in ocean sediments from the North Pacific to the Arctic ocean. Environ. Sci. Technol. 51, 3809–3815. doi: 10.1021/acs.est.7b00755
MacGregor, K., Oliver, I. W., Harris, L., and Ridgway, I. M. (2010). Persistent organic pollutants (PCB, DDT, HCH, HCB & BDE) in eels (Anguilla anguilla) in Scotland: current levels and temporal trends. Environ. Pollut. 158, 2402–2411. doi: 10.1016/j.envpol.2010.04.005
Mahmood, A., Malik, R. N., Li, J., and Zhang, G. (2015). Distribution, congener profile, and risk of polybrominated diphenyl ethers and dechlorane plus in water and sediment from two tributaries of the Chenab river, Pakistan. Environ. Contam. Toxicol. 68, 83–91. doi: 10.1007/s00244-014-0074-y
Malarvannan, G., Belpaire, C., Geeraerts, C., Eulaers, I., Neels, H., and Covaci, A. (2014). Assessment of persistent brominated and chlorinated organic contaminants in the European eel (Anguilla anguilla) in Flanders, Belgium: levels, profiles and health risk. Sci. Total Environ. 482–483, 222–233. doi: 10.1016/j.scitotenv.2014.02.127
Malarvannan, G., Belpaire, C., Geeraerts, C., Eulaers, I., Neels, H., and Covaci, A. (2015). Organophosphorus flame retardants in the European eel in Flanders, Belgium: occurrence, fate and human health risk. Environ. Res. 140, 604–610. doi: 10.1016/j.envres.2015.05.021
Martellini, T., Diletti, G., Scortichini, G., Lolini, M., Lanciotti, E., Katsoyiannis, A., et al. (2016). Occurrence of polybrominated diphenyl ethers (PBDEs) in foodstuffs in Italy and implications for human exposure. Food Chem. Toxicol. 89, 32–38. doi: 10.1016/j.fct.2015.12.026
McHugh, B., Poole, R., Corcoran, J., Anninou, P., Boyle, B., Joyce, E., et al. (2010). The occurrence of persistent chlorinated and brominated organic contaminants in the European eel (Anguilla anguilla) in Irish waters. Chemosphere 79, 305–313. doi: 10.1016/j.chemosphere.2010.01.029
Mekni, S., Barhoumi, B., Aznar-Alemany, O., Touil, S., Driss, M. R., Barceló, D., et al. (2019). Occurrence of halogenated flame retardants in sediments and sea urchins (Paracentrotus lividus) from a North African Mediterranean coastal lagoon (Bizerte, Tunisia). Sci. Total Environ. 654, 1316–1325. doi: 10.1016/j.scitotenv.2018.11.139
Miyake, Y., Jiang, Q., Yuan, W., Hanari, N., Okazawa, T., Wyrzykowska, B., et al. (2008). Preliminary health risk assessment for polybrominated diphenyl ethers and polybrominated dibenzo-p-dioxins/furans in seafood from Guangzhou and Zhoushan, China. Mar. Pollut. Bull. 57, 357–364. doi: 10.1016/j.marpolbul.2008.03.009
Mo, L., Zheng, J., Wang, J., S, Y-G., Chen, B-J., Liu, B., et al. (2019). Legacy and emerging contaminants in coastal surface sediments around Hainan island in South China. Chemosphere 125, 133–141. doi: 10.1016/j.chemosphere.2018.10.022
Moon, H. B., Kannan, K., Lee, S. J., and Choi, M. (2007). Polybrominated diphenyl ethers (PBDEs) in sediment and bivalves from Korean coastal waters. Chemosphere 66, 243–351. doi: 10.1016/j.chemosphere.2006.05.025
Na, G., Wei, W., Zhou, S., Gao, H., Ma, X., Qiu, L., et al. (2015). Distribution characteristics and indicator significance of dechloranes in multi-matrices at Ny-Alesund in the Arctic. J. Environ. Sci. 28, 8–13. doi: 10.1016/j.jes.2014.07.019
Ni, H-G., Ding, C., Lu, S-Y., Yin, X-L., and Samuel, S. O. (2012). Food as a main route of adult exposure to PBDEs in Shenzhen, China. Sci. Total Environ. 437, 10–14. doi: 10.1016/j.scitotenv.2012.07.056
Norén, K., and Meironyté, D. (2000). Certain organochlorine and organobromine contaminants in Swedish human milk in perspective of past 20–30 years. Chemosphere 40, 1111–1123. doi: 10.1016/S0045-6535(99)00360-4
OJEU (2010). Directive 2010/63/EU of the European Parliament and of the Council of 22 September 2010 on the protection of animals used for scientific purposes. Off. J. Eur. Union 276, 33–79.
Ouertani, N., Hamouda, R., and Belayouni, H. (2006). Study of the organic matter buried in recent sediments of an increasing anoxic environment surrounded by an urban area: the “Lac sud de Tunis”. Geo. Eco. Trop. 2, 21–34. Available online at: http://www.geoecotrop.be/uploads/publications/pub_302_848449.pdf
Polder, A., Müller, M., Lyche, J., Mdegela, R., Nonga, H., Mabiki, F., et al. (2014). Levels and patterns of persistent organic pollutants (POPs) in tilapia (Oreochromis sp.) from four different lakes in Tanzani: a geographical differences and implications for human health. Sci. Total Environ. 488, 252–260. doi: 10.1016/j.scitotenv.2014.04.085
Reche, C., Viana, M., Querol, X., Corcellas, C., Barceló, D., and Eljarrat, E. (2019). Concentrations and sources of legacy and novel flame retardants in outdoor and indoor environments. Sci. Total Environ. 649, 1541–1552. doi: 10.1016/j.scitotenv.2018.08.408
Roosens, L., Geeraerts, L., Belpaire, C., Van Pelt, I., Neels, H., and Covaci, A. (2010). Spatial variations in the levels and isomeric patterns of PBDEs and HBCDs in the European eel in Flanders. Environ. Int. 36, 415–423. doi: 10.1016/j.envint.2010.03.001
Sala, B., Giménez, J., de Stephanis, R., Barceló, D., and Eljarrat, E. (2019). First determination of high levels of organophosphorus flame retardants and plasticizers in dolphins from Southern European waters. Environ. Res. 172, 289–295. doi: 10.1016/j.envres.2019.02.027
Santín, G., Barón, E., Eljarrat, E., and Barceló, D. (2013). Emerging and historical halogenated flame retardants in fish samples from Iberian rivers. J. Hazard. Mater. 263, 116–121. doi: 10.1016/j.jhazmat.2013.08.068
Santín, G., Eljarrat, E., and Barceló, D. (2016). Bioavailability of classical and novel flame retardants: effect of fullerene presence. Sci. Total Environ. 565, 299–305. doi: 10.1016/j.scitotenv.2016.04.155
Savinov, V. M., Savinova, T. N., Carroll, J., Mathisov, G. G., Dahle, S., and Naes, K. (2000). Polycyclic aromatic hydrocarbons (PAHs) in sediments of the White sea, Russia. Mar. Pollut. Bull. 40, 807–818. doi: 10.1016/S0025-326X(00)00004-7
SC (2008). Stockholm Convention on Persistent Organic Pollutants. Available online at: http://chm.pops.int/ (accessed August 2017).
Schecter, A., Papke, O., Harris, T. R., Tung, K. C., Musumba, A., Olson, J., et al. (2006). Polybrominated diphenyl ether (PBDE) levels in an expanded market basket survey of US food and estimated PBDE dietary intake by age and sex. Environ. Health Perspect. 114, 1515–1520. doi: 10.1289/ehp.9121
Shen, L., Reiner, E. J., Macpherson, K. A., Kolic, T. M., Sverko, E., Helm, P. A., et al. (2010). Identification and screening analysis of halogenated norbornene flame retardants in the Laurentian great lakes: dechloranes 602, 603, and 604. Environ. Sci. Technol. 44, 760–766. doi: 10.1021/es902482b
Soumis, N., Lucotte, M., Samalo, D., Almeida, D. R., Girous, D., Morais, S., et al. (2003). Presence of organopsphate insecticides in fish of the Amazon river. Amazonica Acta 33, 325–338. doi: 10.1590/1809-4392200332338
Staskal, D. F., Scott, L. L. F., Haws, L. C., Luksemburg, W. J., Birnbaum, L. S., Urban, J. D., et al. (2008). Assessment of polybrominated diphenyl ether exposures and health risks associated with consumption of Southern Mississippi catfish. Environ. Sci. Technol. 42, 6755–6761. doi: 10.1021/es800613k
Strobel, A., Willmore, W. G., Sonne, C., Dietz, R., and Letcher, R. J. (2018). Organophosphate esters in East Greenland polar bears and ringed seals: adipose tissue concentrations and in vitro depletion and metabolite formation. Chemosphere 196, 240–250. doi: 10.1016/j.chemosphere.2017.12.181
Sühring, R., Busch, F., Fricke, N., Kötke, D., Wolschke, H., and Ebinghaus, R. (2016). Distribution of brominated flame retardants and dechloranes between sediments and benthic fish–a comparison of a freshwater and marine habitat. Sci. Total Environ. 542, 578–585. doi: 10.1016/j.scitotenv.2015.10.085
Sühring, R., Byer, J., Freese, M., Pohlmann, J. D., Wolschke, H., Möller, A., et al. (2013b). Brominated flame retardants and dechloranes in European and American eels from glass to silver life stages. Chemosphere 116, 104–111. doi: 10.1016/j.chemosphere.2013.10.096
Sühring, R., Möller, A., Freese, F., Pohlmann, J. D., Wolschke, H., Sturm, R., et al. (2013a). Brominated flame retardants and dechloranes in eels from German rivers. Chemosphere 90, 118–124. doi: 10.1016/j.chemosphere.2012.08.016
Sverko, E.d., Tomy, G. T., Marvin, C. H., Zaruk, D., Reiner, E., Helm, P. A., et al. (2008). Dechlorane plus levels in sediment of the lower great lakes. Environ. Sci. Technol. 42, 361–366. doi: 10.1021/es0710104
Trabelsi, S., and Driss, M. R. (2005). Polycyclic aromatic hydrocarbons in superficial coastal sediments from Bizerte lagoon, Tunisia. Mar. Pollut. Bull. 50, 344–348. doi: 10.1016/j.marpolbul.2004.11.031
U.S. EPA, 2008. Toxicological review of 2,20,4,40 tetrabromodiphenyl ether (BDE-47). Support of Summary Information on the Integrated Risk Information System (IRIS). Available online at: http://cfpub.epa.gov/ncea/cfm/recordisplay-cfm?deidS) 161845 (accessed June 2008); Toxicological Review of 2,20′,4,40,5-Pentabromodiphenyl Ether (BDE-99): In Support of Summary Information on the Integrated Risk Information System (IRIS). Available online at: http://cfpub.epa.gov/ncea/cfm/recordisplay-cfm?deidS) 161846. (accessed June 2008); Toxicological Review of 2,20,4,40,5,50 Hexabromodiphenyl Ether (BDE-153): In Support of Summary Information on the Integrated Risk Information System (IRIS). Available online at: http://cfpub.epa.gov/ncea/cfm/recordisplay-.cfm?deidS) 161847 (accessed June 2008).
Van der Veen, I., and de Boer, J. (2012). Phosphorus flame retardants: properties, production, environmental occurrence, toxicity and analysis. Chemosphere 88, 1119–1153. doi: 10.1016/j.chemosphere.2012.03.067
Wei, G. L., Li, D. Q., Zhuo, M. N., Liao, Y. S., Xie, Z. Y., Guo, T. L., et al. (2015). Organophosphorus flame retardants and plasticizers: sources, occurrence, toxicity and human exposure. Environ. Pollut. 196, 29–46. doi: 10.1016/j.envpol.2014.09.012
Widelka, M., Lydy, M. J., Wu, W., and Chen, D. (2016). Statewide surveillance of halogenated flame retardants in fish in Illinois, USA. Environ. Pollut. 214, 627–634. doi: 10.1016/j.envpol.2016.04.063
Wu, C., Bao, L. J., Tao, S., and Zeng, E. Y. (2016). Mediated distribution pattern of organic compounds in estuarine sediment by anthropogenic debris. Sci. Total Environ. 565, 132–139. doi: 10.1016/j.scitotenv.2016.04.141
Yadav, C. I., and Nevi, N. L. (2019). Data relating to fate and transport of organophosphate ester flame retardants in indoor air and dust from Nepal. Data Brief. 25:104287. doi: 10.1016/j.dib.2019.104287
Yu, D., Yang, J., Li, T., Feng, J., Xian, Q., Zhu, J. (2015). Levels and distribution of dechloranes in sediments of Lake Taihu, China. Environ. Sci. Pollut. Res. 22, 6601–6609. doi: 10.1007/s11356-014-3794-7
Zacs, D., Ikkere, L. E., and Bartkevics, V. (2018). Emerging brominated flame retardants and dechlorane-related compounds in European eels (Anguilla anguilla) from Latvian lakes. Chemosphere 197, 680–690. doi: 10.1016/j.chemosphere.2018.01.105
Zacs, D., Rjabova, J., Fernandes, A., and Bartkevics, V. (2016). Brominated, chlorinated and mixed brominated/chlorinated persistent organic pollutants in European eels (Anquilla anguilla) from Latvian lakes. Food Addit. Contam. 33, 460–472. doi: 10.1080/19440049.2015.1136436
Zhang, X., Sühring, R., Serodio, D., Bonnell, M., Sundin, N., and Diamond, M. L. (2016). Novel flame retardants: estimating the physical-chemical properties and environmental fate of 94 halogenated and organophosphate PBDE replacements. Chemosphere 144, 2401–2407. doi: 10.1016/j.chemosphere.2015.11.017
Zhang, Z. W., Sun, Y. X., Sun, F. K., Xu, R. X., Yu, S., Zheng, T. L., et al. (2015). Brominated flame retardants in mangrove sediments of the Pearl river estuary, South China: spatial distribution, temporal trend and mass inventory. Chemosphere 123, 26–32. doi: 10.1016/j.chemosphere.2014.11.042
Zhong, M., Wu, H., Mi, W., Li, F., Ji, C., Ebinghaus, R., et al. (2018). Occurrences and distribution characteristics of organophosphate ester flame retardants and plasticizers in the sediments of the Bohai and Yellow seas, China. Sci. Total Environ. 615, 1305–1311. doi: 10.1016/j.scitotenv.2017.09.272
Zhou, T., Taylor, M. M., DeVito, M. J. U., and Crofton, K. M. (2002). Developmental exposure to brominated diphenyl ethers results in thyroid hormone disruption. Toxicol. Sci. 66, 105–116. doi: 10.1093/toxsci/66.1.105
Keywords: Bizerte Lagoon, HFRs, OPFRs, sediment, eel, TOC, risk assessment
Citation: Mekni S, Barhoumi B, Touil S, Driss MR and Eljarrat E (2020) Occurrence of Halogenated and Organophosphate Flame Retardants in Sediments and Eels (Anguilla anguilla) From Bizerte Lagoon, Tunisia. Front. Environ. Sci. 8:67. doi: 10.3389/fenvs.2020.00067
Received: 03 July 2019; Accepted: 07 May 2020;
Published: 05 June 2020.
Edited by:
Ravi Naidu, University of Newcastle, AustraliaReviewed by:
Bhabananda Biswas, University of South Australia, AustraliaOladele Ogunseitan, University of California, Irvine, United States
Copyright © 2020 Mekni, Barhoumi, Touil, Driss and Eljarrat. This is an open-access article distributed under the terms of the Creative Commons Attribution License (CC BY). The use, distribution or reproduction in other forums is permitted, provided the original author(s) and the copyright owner(s) are credited and that the original publication in this journal is cited, in accordance with accepted academic practice. No use, distribution or reproduction is permitted which does not comply with these terms.
*Correspondence: Ethel Eljarrat, eeeqam@cid.csic.es