- 1Department of Biological and Environmental Engineering, Cornell University, Ithaca, NY, United States
- 2Department of Microbiology and Immunology, College of Veterinary Medicine, Cornell University, Ithaca, NY, United States
- 3Sanergy, Nairobi, Kenya
- 4Center for Applied Geosciences, University of Tübingen, Tübingen, Germany
Innovative, low-cost methods for inactivating pathogens in human fecal material (HFM) are needed, particularly in expanding urban areas where conventional sewer systems and centralized wastewater treatment plants are not feasible. To address this challenge, we have developed a bioprocess that utilizes open cultures of anaerobic bacteria (i.e., microbiomes) to produce carboxylic acids using HFM as substrate. When the pH is sufficiently low, the carboxylic acids exist in the undissociated form and inactivate pathogens. Here, we used real solid waste (SW) collected from container-based, urine-diverting dry toilets (UDDT) in Nairobi, Kenya to conduct lab-scale and field-scale trials. Through these trials, we investigated operating conditions required to use carboxylic acid fermentation in sanitation waste treatment processes. We tested three different inoculum treatments and determined that the microbiome in UDDT-SW is well-suited to produce carboxylic acids without the need for an external inoculum. We also tested co-fermentation of UDDT-SW with carbohydrate-rich food waste as a means of reducing the pH. We found that when food waste was incorporated in a way that maintained the pH between 4.8 and 5.2, then the food waste was quickly converted to carboxylic acids, and the low pH created high concentrations of undissociated carboxylic acids. The resulting concentrations of undissociated carboxylic acids resulted in Ascaris inactivation within 15 days. However, we found that a temperature ≥30°C is required for carboxylic acid production to occur.
Introduction
As a global community, we struggle to manage our waste in a safe and sustainable manner. Management of human excreta is particularly concerning due to the implications it has for human health and dignity, and yet 4.5 billion people still lack access to safely managed sanitation services (WHO and UNICEF, 2017). The provision of a sanitation facility alone does not address the challenge because excreta must also be either safely contained on-site or transported, and treated off-site. Connection to a sewer system also does not guarantee access to safely managed sanitation. Of the 2.8 billion people using sewer connections, only 1.9 billion people are expected to have safely managed sanitation due to sewer lines that empty directly into the environment or wastewater treatment plants with insufficient treatment (WHO and UNICEF, 2017). Sewer systems are also frequently unfeasible due to high costs, uncertain land tenure, lack of appropriate space, and challenging topography among others (World Bank, 2019). Instead, a variety of treatment approaches and technologies are required to address the diverse challenges faced in achieving universal safe sanitation, including innovative methods for inactivating pathogens in excreta.
One method under investigation uses anaerobic fermentation of human fecal material (HFM) to produce carboxylic acids (Harroff et al., 2017). Complex organic molecules are first broken down to short-chain carboxylic acids (e.g., acetic acid, propionic acid, and n-butyric acid) through hydrolysis and primary fermentation. Oxidation of an electron donor, such as ethanol, sugars, amino acids, or lactate, is then coupled to chain elongation of the short-chain carboxylic acids to produce n-butyric acid (C4), n-caproic acid (C6), and even n-caprylic acid (C8) (Agler et al., 2011; Spirito et al., 2014). Acetic acid (C2) is often the dominant primary fermentation product, and hydrocarbon chains are elongated with two carbon molecules at a time. Therefore, molecules with even-numbered hydrocarbon chains are more common than those with odd-numbered chains, but both are produced. Previous work has shown that HFM can be used as substrate to produce up to 257 mM n-butyric acid, 11.3 mM n-valeric acid, and 27.1 mM n-caproic acid (Harroff et al., 2017).
Carboxylic acids have been used as food preservatives for decades due to their antimicrobial properties (Freese et al., 1973), and past work has demonstrated that for short-chain (one to five carbons) and medium-chain (six to eight carbons) carboxylic acids, antimicrobial activity increases as the length of the hydrocarbon chain increases (Abdul and Lloyd, 1985; Salsali et al., 2006; Royce et al., 2013). More recent work has demonstrated that carboxylic acids are also toxic to Ascaris eggs, which are commonly used as indicators for pathogen inactivation in waste treatment processes due to their high resistance to inactivation (Butkus et al., 2011; Harroff et al., 2017; Riungu et al., 2018a). The importance of hydrocarbon chain length has also been demonstrated for Ascaris inactivation. When exposure time, pH, and temperature were constant, n-caproic acid (C6) concentrations required for a 3-log reduction in Ascaris viability were more than an order of magnitude smaller than concentrations required for n-butyric acid (C4) (Harroff et al., 2017).
Carboxylic acids exist in both a dissociated (carboxylate) and undissociated (carboxylic acid) form, depending on the pH in the solution. When the pH is below the pKa (~4.8 for the carboxylic acids of interest here), a majority of the molecules are in the undissociated form with a hydrogen atom attached to the carboxyl group. At a pH above the pKa, a majority of the molecules exist as the dissociated carboxylate with the hydrogen detached. The specific concentrations can be calculated using the Henderson-Hasselbalch equation, , where [A−] represents the concentration of the dissociated form, and [HA] represents the concentration of the undissociated form. Throughout this paper, we will explicitly specify if we are referring to the undissociated form. If the undissociated form is not specified, then we are referring to the total concentration of undissociated acid plus dissociated conjugate base.
The distinction between the total concentration and the concentration of undissociated carboxylic acids is important because only the undissociated form inhibits pathogens (Butkus et al., 2011; Royce et al., 2013; Harroff et al., 2017). We previously produced carboxylic acids from HFM, but the pH remained nearly neutral throughout the experiment. Therefore, the fraction of undissociated carboxylic acids form remained relatively low, while the total carboxylic acid concentrations were promising (Harroff et al., 2017). Co-fermentation of HFM and food waste may present a solution to this problem. Many studies have shown that anaerobic fermentation or co-fermentation of carbohydrate-rich food waste results in rapid lactic acid production and pH decline (Rajagopal et al., 2014; Wang et al., 2014; Liang and Wan, 2015). In a series of recent studies, mixed food waste and HFM were co-fermented specifically to increase concentrations of undissociated carboxylic acids to inactivate pathogens, but these studies did not focus on chain elongation or report concentrations of individual carboxylic acids (Riungu et al., 2018a,b). Co-fermentation with food waste may be challenging because low pH and increased undissociated carboxylic acid concentrations can inhibit the bacteria responsible for fermentation and chain elongation, in addition to inactivating pathogens (Agler et al., 2012; Riungu et al., 2018a). Therefore, pH reduction must be carefully balanced with maximizing carboxylic acid production and chain elongation.
The primary goal of our study was to better understand how HFM can be fermented to produce undissociated carboxylic acids that cause pathogen inactivation in real sanitation and waste treatment systems. To accomplish this, we collaborated with Sanergy, which is a sanitation enterprise in Nairobi, Kenya, that maintains a network of container-based, urine-diverting dry toilets (UDDTs) and converts the collected waste to valuable reuse products. Carboxylic acid fermentation may be useful as a pre-treatment step in treatment facilities similar to Sanergy's facility, because the output has an adequately reduced pathogen load and has already undergone the often rate-limiting stages of biological degradation. It can then be used as the input for further processing into value-added products such as biogas from anaerobic digestion or livestock feed from black soldier fly larvae production. Here, we used real HFM collected from Sanergy's UDDTs, which we refer to here as UDDT-solid waste (UDDT-SW), and tested fermentation: (1) with 500-mL media bottles in the lab during Experiments 1 and 2; and (2) 45-L plastic barrels at the field-scale during Experiment 3. We tested three different inocula to determine if a specialized inoculum would be required to implement new treatment systems. We also tested the addition of banana waste at different times during the fermentation process and at different ratios to maximize concentrations of undissociated carboxylic acids. Finally, we demonstrated pathogen inactivation from co-fermentation of HFM with banana waste using Ascaris suum eggs as indicators.
Materials and Methods
Materials
HFM Samples
HFM was obtained from UDDTs that are located in informal settlements around Nairobi, Kenya. The UDDTs have separate containers to collect urine and solid waste. The UDDT solid waste (UDDT-SW) container is 30 L and contains a mixture of HFM and sawdust, which is added by users to reduce odor in the toilets. Sawdust is expected to constitute about 20% of the total mass of the UDDT-SW. Sanergy collects the urine and UDDT-SW containers each day and replaces them with clean containers. On the starting day for Experiments 1 and 3, samples of UDDT-SW were taken from several containers that had been collected from UDDTs that same day.
Banana Waste
The pre-consumer banana waste originated from Twiga Foods, which is a food supply platform that connects smallholder farms to market vendors. Sanergy receives fresh banana waste that is unsuitable for market every few days and stores it outdoors in a pile until it is used in waste treatment and recycling processes.
Chain-Elongation-Bioreactor Inoculum
The chain-elongation-bioreactor inoculum originated from a semi-continuous lab-scale bioreactor that chain-elongated ethanol-rich fermentation beer for 4 years (Ge et al., 2015). The effluent was collected from the bioreactor and centrifuged to concentrate the biomass. The bioreactor broth was discarded, and the biomass was re-suspended in an equivalent volume of liquid that was 50% (v/v) glycerol and 50% (v/v) fresh bioreactor media. The biomass was then frozen at −80°C for ~1 year until use. After thawing, the biomass was washed twice to remove the glycerol by centrifuging and replacing the supernatant with tap water. A new culture was then started by inoculating 10% (m/m) biomass into diluted UDDT-SW. This culture was kept in an airtight 1-L glass bottle. The headspace was sparged with nitrogen gas to create anaerobic conditions, and the bottle was incubated at 30°C in the dark for 24 days with periodic manual shaking to mix. Contents from this culture were used directly as chain-elongation-bioreactor inoculum for lab-scale experiments.
Rumen Inoculum
The rumen contents were selected for testing because considerable carboxylic acid production occurs in the rumen, and rumen contents were originally used to inoculate the bioreactor that was used for the chain-elongation-bioreactor inoculum (Agler et al., 2012). The rumen contents are also easier to obtain than chain-elongation-bioreactor inoculum, which would depend on successful implementation of carboxylic acid fermentation for waste treatment. The rumen inoculum was collected from the rumen contents of a cow that had been freshly slaughtered at Dagoretti Slaughterhouse in Nairobi, Kenya. Next, the rumen contents were strained through a mesh screen and cheesecloth to remove large particulate matter before use.
Methods
Experiment 1: Determining the Importance of a Specialized Inoculum
Three inoculum treatments were tested to determine whether a specialized inoculum was necessary to achieve carboxylic acid production and chain elongation: (1) chain-elongation-bioreactor inoculum; (2) rumen inoculum; and (3) no inoculum. The chain-elongation-bioreactor inoculum and rumen inoculum were obtained as described above. For the third treatment, tap water was used in place of an inoculum, and therefore the fermentation in the media bottles for this treatment was dependent on the microbiome residing naturally in HFM. UDDT-SW was collected in the morning of the starting day of the experiment. The UDDT-SW samples were combined and homogenized in a kitchen blender before separating into treatments. Each treatment was prepared with ~65 g inoculum and 585 g UDDT-SW (Table 1). The treatment mixtures were manually mixed with a spatula, and 200 g was allocated to triplicate 500-mL glass media bottles. A 5-g sample of each treatment was also kept for pH and carboxylic acid analysis. The headspace in each media bottle was sparged with nitrogen gas for 1 min at a rate of 12 L min−1. The media bottles were then sealed with a rubber stopper and plastic ring (Figure S1) and incubated in the dark at 30°C for 26 days. Additional mixing occurred only during sample collection. The fermentation gas was vented from the media bottles on Day 1 and 2 to avoid excessive pressure buildup before the first sampling point. Venting was performed by inserting a hypodermic needle into a septum that was located in the rubber stopper of each media bottle. The samples were collected from each media bottle on Day 5, 12, 18, 21, 25, and 26 to measure pH and carboxylic acid concentrations. The rubber stopper and plastic ring were removed from the media bottle, and the contents were manually mixed with a spatula before removing a small sample (~5 g) of contents. Finally, the headspace in the media bottle was sparged with nitrogen for 1 min at 12 L min−1, and the rubber stopper and plastic ring were replaced. Carboxylic acid concentrations were measured using gas chromatography (Hewlett-Packard 5890 Series II; Wilmington, DE) with a method described previously, except nitrogen was used as the carrier gas instead of helium (Usack and Angenent, 2015).
Experiment 2: Co-fermentation With Banana Waste to Reduce pH, Increase Concentrations of Undissociated Carboxylic Acids, and Inactivate Ascaris Eggs
After Day 26 of the operating period, the media bottles from Experiment 1 were emptied. All of the remaining fermented UDDT-SW was combined, homogenized in a kitchen blender, and divided into three new treatments: (1) 0% banana-mash addition; (2) 10% banana-mash addition; and (3) 20% banana-mash addition, with all percentages on a mass basis. The banana waste had been collected from the outdoor storage pile 1 day prior to Day 0 and kept in the laboratory refrigerator overnight. The peels from the bananas were removed, and the bananas were homogenized in a kitchen blender. Finally, the resulting banana mash was combined with the fermented UDDT-SW to produce 360 g of each treatment. The mixtures were manually mixed with a spatula, and 155-g aliquots were distributed to duplicate 500-mL glass media bottles. We used duplicate treatments instead of triplicates based on limitations from the quantity of fermented UDDT-SW material available. The media bottles (Figure S1) were sparged with nitrogen, sealed, and sampled as described above, with samples collected on Day 1, 3, 6, and 15 to measure pH and carboxylic acid concentrations.
We tested Ascaris inactivation for the 0% and 20% banana-mash treatments using A. suum eggs. The eggs were collected from fecal material in the intestines of naturally infected slaughterhouse pigs and purified and stored as described previously (Harroff et al., 2017). We used plastic chambers (2.5 cm diameter, 2.5 cm height), which were sealed on both ends with 38-μm metal mesh, to contain the eggs while exposing them to the conditions inside the media bottles. The plastic chambers were initially sealed on one end, and the open end was used to fill them with the 0 and 20% banana-mash treatment mixtures and to spike them with 10,000 unembryonated A. suum eggs. A soldering iron was then used to seal the open end of the plastic chamber with a second piece of mesh (Figure S2). Nylon mesh bags have frequently been used to contain Ascaris eggs in similar experiments (Johnson et al., 1998; Nordin et al., 2009; Manser et al., 2015; Fidjeland et al., 2016), but we found that the plastic chambers were more reliable. Prior to performing the experiment, we made several nylon mesh bags and sealed the seams with a thermal sealer, as has been done before, but we found that the seams separated over time or had incomplete seals. In a study that directly compared Ascaris egg recovery from nylon mesh bags vs. plastic chambers, plastic chambers were also found to perform better and more consistently (Nelson and Darby, 2002). The mesh bags often trapped gas inside, and the seams became unsealed, possibly due to the increased gas pressure. In contrast, the fixed volume of the plastic chambers seemed to force gas out of the chamber, and the more substantial seal held throughout the experiment (Nelson and Darby, 2002).
Eight plastic chambers were prepared, with two plastic chambers placed in each of the duplicate media bottles for the 0% and 20% banana-mash treatments. One plastic chamber from each media bottle was removed during sampling on Day 6 and 15. Ascaris eggs were recovered from the plastic chambers using a method adapted from several methods published previously (Nelson and Darby, 2002; Bowman et al., 2003; Trönnberg et al., 2010; Amoah et al., 2018). First, the mesh from one side was peeled off, and a metal spatula was used to divide most of the contents between two 50-mL centrifuge tubes. The empty plastic chamber was then placed in a 200-mL wide-mouth cylinder with about 75 mL of 1.5 M ammonium bicarbonate (AmBic) solution. The cylinder was sealed and shaken for 30 s to dislodge any remaining solid material. The solution in the cylinder was poured into the two centrifuge tubes, and additional AmBic solution was used to rinse the chamber and cylinder into the tubes. The two centrifuge tubes were sealed and shaken for 1 min and then centrifuged at 1,000 × g for 5 min. The supernatant was discarded, and the tubes were filled with MgSO4 (specific gravity = 1.2), vortexed, and centrifuged again at 1,000 × g for 5 min to float the Ascaris eggs. The supernatant was sieved through US 50 and US 200 sieves, before collecting the eggs on a US 400 sieve. Diluted H2SO4 (0.1 N) was used to rinse the sieve into a clean 50-mL centrifuge tube. The tube was centrifuged at 1,000 × g for 5 min to concentrate the eggs at the bottom of the tube, and a pipette was used to remove supernatant to a final volume of 5 mL. Next, the tube was vortexed for a final time, the cap was loosened to allow oxygen flow, and the tube was incubated in the dark at 28°C for 3 weeks to allow any viable A. suum eggs to develop into larvae. After 3 weeks, at least 100 eggs were observed microscopically to determine viability. Fully developed larvae were considered viable, and all others were not.
Experiment 3: Field-Scale Trial
A field-scale fermentation trial was performed on-site at Sanergy's waste processing facility located southeast of central Nairobi in Kinanie. Six 45-L screw-top plastic barrels were used as batch bioreactors to test three treatments in duplicate (Table 2, Figure S3). For Treatments 1 and 2, UDDT-SW was fermented alone for 42 days before adding banana waste because we expected the banana waste to cause a low pH that would be inhibitory to carboxylic acid production. For Treatment 3, banana waste was mixed with UDDT-SW on Day 0, and no additional banana waste was added on Day 42 of the operating period. We used cow rumen inoculum for Treatments 1 and 3, and no inoculum for Treatment 2. We obtained rumen contents from a cow that was slaughtered the same morning, and we prepared the inoculum as described above. UDDT-SW was collected from two randomly selected storage containers that had been transferred to Kinanie within the previous 2–3 days. Each storage container held UDDT-SW collected from ~3–4 UDDTs. We collected banana waste from the storage pile onsite and roughly chopped the waste with the peels left intact. The required material for each barrel was combined and manually mixed with a shovel.
Data loggers (HOBO UX100-003, Bourne, MA and Lascar Electronics EL-USB-1, Eerie, PA) were contained in sealed plastic tubes and placed in each barrel to record temperature every 15 min. The barrels were initially placed in a sunny area outside and covered with a black tarp to promote warmer temperatures. We conducted the field trial in May-July, which is the cool season for Nairobi. After 29 days of operation, the barrels were moved inside a greenhouse to achieve warmer temperatures. Throughout the operating period, samples were collected every 2–6 days from the barrels. At sampling, the lid was removed, and the barrel contents were manually mixed with a large spoon. Small samples were collected from several points in the barrel and mixed together in a plastic tube. The composite sample was used to measure pH and carboxylic acid concentrations.
We also tested Ascaris inactivation in the field-scale trial. First, we placed A. suum eggs in plastic chambers as described above. Ten chambers were prepared for each barrel, with two chambers collected from each barrel at each time point. The chambers were attached to wires and sunk in the barrels to expose the A. suum eggs to the fermentation conditions. An additional 20 chambers were prepared and filled with autoclaved UDDT-SW instead of the UDDT-SW used in the barrels. Each of these chambers was sealed in a 50-mL plastic tube that was also filled with autoclaved UDDT-SW, and the plastic tubes were sunk in the barrels for Treatment 1. Duplicate chambers with the autoclaved UDDT-SW were collected at the same time points as the other chambers and were used to isolate inactivation that occurred for reasons unrelated to the fermentation process (e.g., temperature or compounds already existing in the UDDT-SW before fermentation).
Results and Discussion
Experiment 1: Specialized Inoculum Was Not Necessary to Produce Carboxylic Acids in HFM
During 26 days of fermentation for the lab-scale trial, we found no benefit from either the chain-elongation-bioreactor inoculum or rumen inoculum compared to the no-inoculum treatment (Figures 1A,B). The n-butyric acid concentrations between the three treatments were nearly identical throughout the operating period with maximum average concentrations observed on Day 25 for all three treatments (Figure 1A). The average maximum n-butyric acid concentrations were 107, 111, and 107 mM for chain-elongation-bioreactor inoculum, rumen inoculum, and no inoculum, respectively, but the differences were within the standard deviations of the triplicate samples (Figure 1A). For n-caproic acid, the treatment with no inoculum actually outperformed the other two treatments with an average maximum concentration of 24.3 vs. 21.0 mM with chain-elongation-bioreactor inoculum and 20.0 mM with rumen inoculum (Figure 1B). We also observed that the trends for n-valeric acid concentrations were similar to the n-caproic acid concentrations (Figure S4). The treatment with no inoculum consistently produced the highest n-valeric acid concentrations, and a maximum average n-valeric acid concentration of 35.0 mM was observed on Day 25 of the operating period (Figure S4). This n-valeric acid concentration is notably larger than the 11.3 mM observed in a previously published study (Harroff et al., 2017) and may be the result of competing pathways before entry into the chain-elongating pathway. Such pathways include the acrylate pathway, which converts lactic acid into propionic acid (Kucek et al., 2016). Chain elongation of propionic acid would then result in production of n-valeric acid instead of one of the even-numbered carboxylic acids. Although we did not measure lactic acid here, it is a common product of primary fermentation in addition to acetic acid, and is quickly removed while being produced in the anaerobic food web. We observed only a small difference in pH between the three treatments and throughout the time-course of the experiment. The average pH was 6.2 with a minimum of 6.0 and maximum of 6.3 (data not shown), which is similar to the previously published study (Harroff et al., 2017).
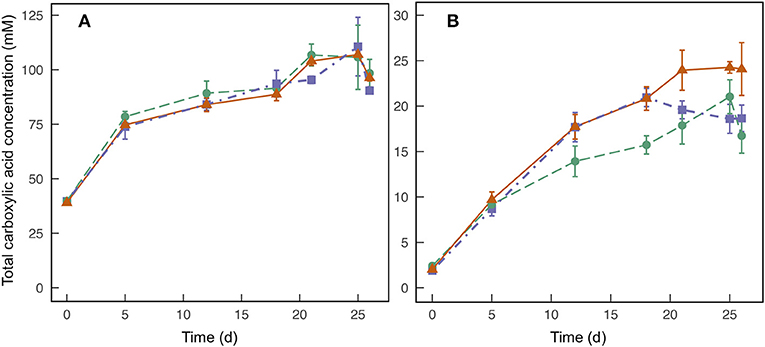
Figure 1. Average total concentrations of n-butyric acid (A) and n-caproic acid (B) found in triplicate batch-fermentation media bottles for Experiment 1, which were started with inoculum from a semi-continuous chain-elongation bioreactor (•); inoculum from rumen contents of a cow (■); and no inoculum (▲). Error bars indicate standard deviations of the measured concentrations.
The promising performance of the treatment with no inoculum indicates that the gut microbiome, which is inherent to HFM, can be used to produce carboxylic acids. This finding is not surprising because the human-gut microbiome is already known to produce n-butyric acid and n-caproic acid within the digestive system (Cummings et al., 1987; Miller and Wolin, 1996). While not surprising, the finding is important for implementing the proposed treatment method in sanitation systems because the system becomes less complex and less expensive if a specialized inoculum is not required. However, human diet is known to affect the gut microbial community and resulting fermentation products (Flint et al., 2015). Therefore, variability in rates of carboxylic acid production and final concentrations should be expected when relying on the gut microbiome.
Experiment 2: Co-fermentation With Banana Mash and Ascaris Inactivation
Co-fermentation With Banana Mash Reduces pH
We observed only small changes in pH throughout the operating period when solely UDDT-SW was fermented for Experiment 1 (average pH of 6.2), which continued during Experiment 2 for the 0% banana-mash treatment (Figure 2A). A pH value of 6.2 causes only 4.2% of the total carboxylic acid concentration to exist in the undissociated form (Figures 2B,C, Figures S5, S6). After banana mash was mixed with the fermented UDDT-SW at a rate of 20% by mass, the pH dropped from 6.1 to 4.8 within 6 days of the operating period (Figure 2A). At such a pH value, half of the total carboxylic acid concentration was in the undissociated form (Figures 2B,C, Figures S5, S6). In the 10% banana-mash treatment, the pH decreased to 5.2 within 6 days (Figure 2A), resulting in 32% of the total carboxylic acid concentration in the undissociated form (Figures 2B,C, Figures S5, S6). A previous study co-fermented mixed food waste and UDDT-SW at a series of substrate ratios and observed similar pH values compared to our study (Riungu et al., 2018a). When food waste made up 20% of the total mass, the pH decreased from 5.7 to 4.9 in 4 days (Riungu et al., 2018a).
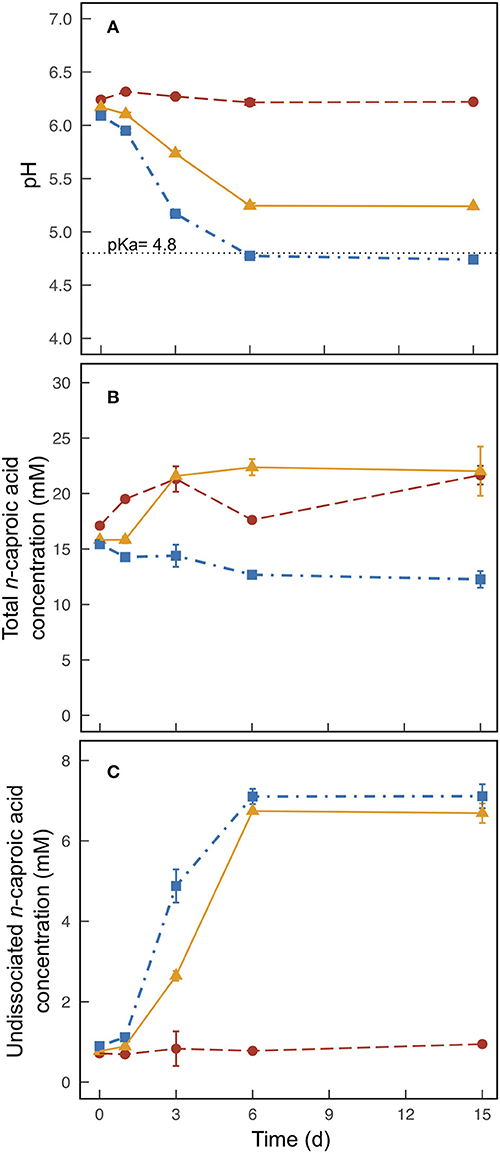
Figure 2. Average pH (A), total n-caproic acid concentration (undissociated plus dissociated) (B), and undissociated n-caproic acid concentration (C) found in duplicate batch fermentation media bottles for Experiment 2 with banana mash added at 0 % (m/m) (•), 10 % (m/m) (▲), and 20 % (m/m) (■). Error bars indicate the range of the measured values between the duplicate media bottles. The dotted horizontal line at pH = 4.8 indicates the pKa of carboxylic acids.
Low pH Causes Inhibition of Carboxylic Acid Production
On Day 0 for Experiment 2, carboxylic acid concentrations were already 73.5–88.7 mM n-butyric acid, 23.3–27.3 mM n-valeric acid, and 15.4–17.1 mM n-caproic acid due the pre-fermentation during Experiment 1. The addition of banana mash lowered these concentrations slightly due to dilution (Figure 2B, Figures S5A, S6A). When no banana mash was added, carboxylic acid concentrations continued to increase for the first 3 days of the operating period before tapering off (Figure 2B, Figures S5A, S6A). This result is similar to the result we would have anticipated if we had allowed the Experiment 1 treatments to continue fermenting. We observed maximum average concentrations for the 0% banana-mash treatment of 101 mM n-butyric acid, 31.4 mM n-valeric acid, and 21.7 mM n-caproic acid. The n-caproic acid concentration was similar to the maximum average concentration we observed in a previous fermentation experiment, but the n-butyric acid concentration was less than half of the concentration we observed (Harroff et al., 2017). Similar to Experiment 1, concentrations of n-valeric acid were higher than those seen previously (Figure S6), and this may partly account for the lower concentrations of n-butyric acid. In addition, a higher conversion efficiency of n-butyric acid to n-caproic acid may also have contributed to a relatively low n-butyric acid concentration.
For the 20% banana-mash treatment, no additional carboxylic acid production occurred (Figure 2B, Figures S5A, S6A), which can be attributed to a combination of inhibition from: (1) the low pH directly; and (2) the high concentration of undissociated carboxylic acids, which was considerably increased due to the low pH (Figure 2C, Figures S5B, S6B) (Agler et al., 2012; Riungu et al., 2018a). For the 10% banana-mash treatment, production rates of carboxylic acids were slightly higher than those observed for the 0% banana-mash treatment (Figure 2B, Figures S5A, S6A). While the pH declined for the 10% banana-mash treatment, it remained above 5 (Figure 2A), which did not cause noticeable inhibition compared to the 0% banana-mash treatment. Therefore, the added banana mash provided a readily degradable carbon course that contributed to the increased production rates of carboxylic acids compared to the 0% banana-mash treatment.
A Low pH Must Be Balanced With Carboxylic Acid Production to Produce High Concentrations of Undissociated Carboxylic Acids
Although no additional carboxylic acid production occurred after banana mash was added in the 20% treatment, this treatment still resulted in the highest concentrations of undissociated carboxylic acids due to the lower pH value compared to the 0 and 10% treatments (Figure 2C, Figures S5B, S6B). The difference in undissociated n-caproic acid concentrations between the 20% treatment and the 10% treatment was minor (Figure 2C), but it was more pronounced for undissociated n-valeric acid and undissociated n-butyric acid concentrations (Figures S5B, S6B). The concentration of undissociated carboxylic acids is a function of the total carboxylic acid concentration and the pH value. The pH relationship is exponential and has a greater effect than the total carboxylic acid concentrations. This is clearly visualized by the much higher concentrations of undissociated n-caproic acid in the 10% banana-mash treatment compared to the 0% banana-mash treatment, while the total concentrations of n-caproic acid were somewhat equivalent between the two treatments (Figures 2B,C). However, comparing the 10% and 20% banana-mash treatments demonstrates that a high total n-caproic acid concentration is also important. The difference in undissociated concentrations of n-caproic acid between these two treatments was minor because the 10% treatment had a larger concentration of total n-caproic acid (22.3 mM in the 10% treatment and vs. 12.3 mM in the 20% treatment) (Figures 2B,C).
The baseline concentrations of carboxylic acids produced during Experiment 1 were also important here. Without it, the 20% treatment would have had very low total carboxylic acid concentrations (and therefore low undissociated concentrations) due to inhibition from low pH after banana-mash addition. Therefore, 20% banana-mash addition may be appropriate if fermentation is completed in two stages, as was done here. However, it is simpler to conduct the fermentation in a single stage with UDDT-SW and banana mash mixed upfront. For this scenario, a lower fraction of banana mash may be desired, which would maintain a moderate pH (~5) that does not inhibit carboxylic acid production but still maintains a large portion of the total concentration in the undissociated form, as we saw in the 10% banana-mash addition treatment.
Ascaris Eggs Are Inactivated Faster When Banana Mash Is Used to Produce a Higher Concentration of Undissociated Carboxylic Acids
We only compared Ascaris egg inactivation for the treatments containing 20% banana mash and 0% banana mash. For both treatments, we observed large decreases in Ascaris viability after 6 days and 15 days, but viability below detection was only achieved for the 20% banana-mash treatment after 15 days (Figure 3). The inactivation rates were also faster for the 20% banana-mash treatment compared to the 0% banana-mash treatment, with 1.4% viability observed after 6 days compared to 53% viability, respectively (Figure 3). The observed inactivation for the 0% banana-mash treatment was unexpected because the undissociated carboxylic acid concentrations were very low (Figure 2C, Figures S5B, S6B). However, similar inactivation rates have been observed previously for treatments with low undissociated carboxylic acid concentrations, and they were attributed to additional fermentation products that were not measured (Harroff et al., 2017). One fermentation product that we thought might be important, particularly with added banana waste, was lactic acid. However, during a preliminary experiment we exposed Ascaris eggs to concentrations of lactic acid up to 1 M (at pH 2, for 1–15 days at 30°C), and we observed less than a 10% decrease in viability for all treatments (data not shown). Previous studies that performed similar batch fermentations with food waste found lactic acid concentrations in the range of 0.1–0.2 M (Wang et al., 2014; Liang and Wan, 2015). Therefore, we can reasonably assume that lactic acid did not cause the Ascaris inactivation we observed in the present study.
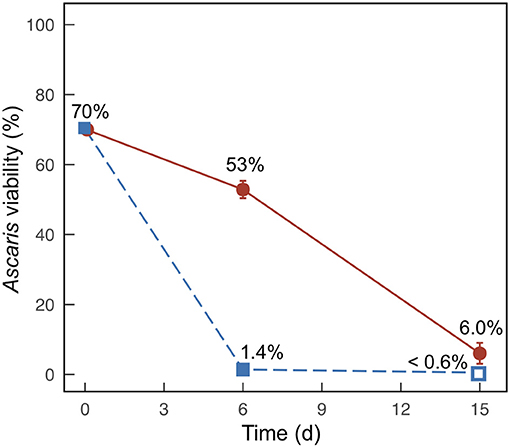
Figure 3. Average percent viability of Ascaris eggs collected from duplicate batch fermentation media bottles for Experiment 2 with banana mash added at 0% (m/m) (•) and 20% (m/m) (■). Error bars indicate the range between the two samples. Open symbols indicate that viability was below the detection limit (0.6%).
Riungu et al. (2018a) also tested Ascaris inactivation related to fermentation of UDDT-SW and food waste. The results are difficult to compare because individual carboxylic acid concentrations were not measured in the Riungu et al. study; however, inactivation below detection limits was observed within 4 days for many treatments (Riungu et al., 2018a). These inactivation rates were even faster than in our study, which can be partly attributed to the higher temperature of 35°C for the Riungu et al. study. Indeed, temperature has been shown to have a significant impact on Ascaris inactivation rates by ammonia (Fidjeland et al., 2015). We anticipate a similar effect for carboxylic acids because both methods rely on the ability of the chemicals to cross the lipid membrane of the eggs (Fidjeland et al., 2015).
Experiment 3: Field-Scale Trial
Cow Rumen Inoculum Did Not Affect Carboxylic Acid Concentrations
The effect of cow rumen inoculum was tested in Treatment 1 (rumen inoculum) and Treatment 2 (no inoculum). Although we already demonstrated that inoculum was not necessary in Experiment 1 (Figure 1), these results were not available when we started Experiment 3 because Experiment 1 was not fully completed. In Experiment 3, we further confirmed that the use of inoculum did not improve carboxylic acid concentrations (Figure 4A, Figure S8A).
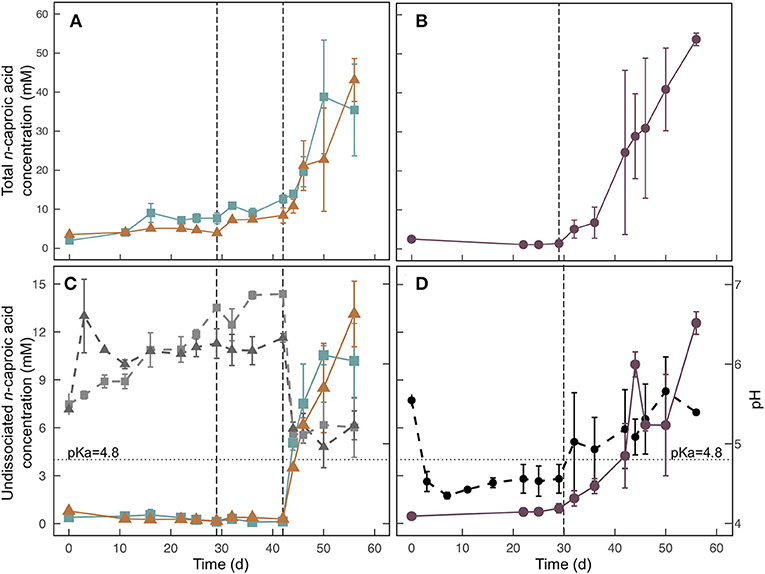
Figure 4. Total n-caproic acid concentrations (A,B), undissociated n-caproic acid concentrations, (C,D) and pH (C,D) for the field-scale trial during Experiment 3. For (C) and (D), undissociated n-caproic acid concentrations (solid colored lines) are plotted against the left axis, and pH (dashed, ray-scale lines) is plotted against the right axis. Treatments (■) and 2 (▲), which were conducted in two stages are shown on the left (A,C). Treatment 3 (•), which had banana waste mixed with UDDT-SW at initial startup is shown on the right (B,D). Error bars show the range between duplicate barrels. The dashed vertical line at Day 29 indicates the time that barrels were moved into the greenhouse, and the average temperature increased from 22.1 to 26.9°C. The dashed vertical line at Day 42 in (A) and (C) indicates the time that banana waste was added to Treatments 1 and 2. The dotted horizontal line at pH = 4.8 indicates the pKa of carboxylic acids.
Warm Temperatures Are Needed to Produce Carboxylic Acids
We used data loggers to measure and record the temperature every 15 min in each of the 6 barrels that were used for the field-scale trial. Two of the loggers failed during the experiment, and the data was not retrievable. However, we can assume that the temperatures in all barrels were the same because the temperatures measured from the remaining four loggers were not statistically different (p = 0.235) (Figure S7). During the first 29 days of the trial, the barrels were kept outside under a black tarp. The average temperature during this period was 22.1°C with a range of 17.7 − 29.0°C and standard deviation of 1.8°C. The observed temperatures were lower than we had originally anticipated, which resulted in very little carboxylic acid production during this period (Table 3, Figure 4, Figure S8). Previous fermentation trials that produced carboxylic acids from HFM or UDDT-SW, including the lab-scale trials here, were performed at higher temperatures of 30°C (Harroff et al., 2017) and 35°C (Riungu et al., 2018a). Therefore, we moved the barrels to a greenhouse on Day 29 of the operating period. From Day 29 until the end of the trial on Day 56, the average temperature was 26.9°C with a range of 18.7–34.5°C and standard deviation of 2.2°C. This temperature increase resulted in modest increases in n-caproic acid concentrations between days 29 and 42 (Figures 4A,B), but concentrations remained lower than seen previously, likely because the average temperature remained below 30°C.
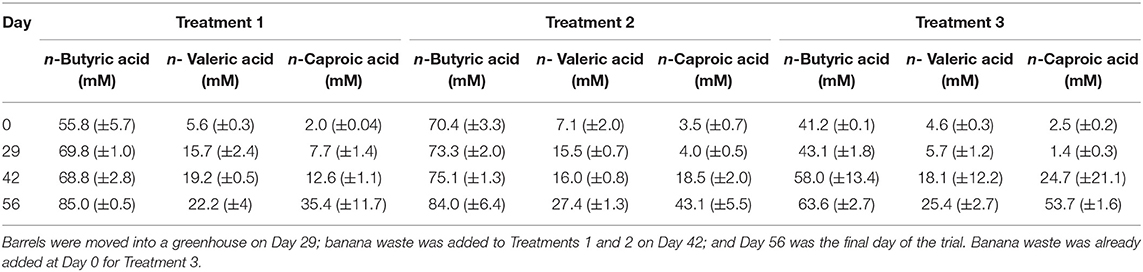
Table 3. Carboxylic acid concentrations at transitional time points during the field-scale trial for Experiment 3.
If pH >5 Is Maintained, Then Banana Waste Is Rapidly Converted to Carboxylic Acids, and High Undissociated Concentrations Can Be Achieved Without Experiencing Product Inhibition
We added banana waste to Treatments 1 and 2 on Day 42 of the operating period as 20% of the total mass, which was after the temperature had been increased. As anticipated, the pH decreased within 2 days from 6.9 to 5.1 for Treatment 1 and from 6.3 to 5.2 for Treatment 2 (Figure 4C). However, the pH never decreased below 5.0 for either treatment. Based on the 20% banana-mash treatment during Experiment 2, we had anticipated a lower pH. We are attributing the higher pH for Experiment 3 to two possible reasons: (1) the different composition between banana mash without peel (Experiment 2) and banana waste with peel (Experiment 3); and (2) ammonia production from protein breakdown during the longer fermentation period compared to Experiment 2 (ammonia data not available), which would have caused a higher buffering capacity of the UDDT-SW (Procházka et al., 2012; Rose et al., 2015). For Treatment 3, we added banana waste from the beginning of the operating period (Day 0), and the pH was reduced to 4.5 within the first 3 days of the trial (Figure 4D), even though the temperature had been relatively low. The pH consistently remained below the carboxylic acid pKa of 4.8 until Day 29 of the operating period (Figure 4D). However, after moving the barrels to the greenhouse on Day 29, the pH for Treatment 3 increased slightly to above 5.0 (Figure 4D), likely due to increased ammonia production coinciding with increased microbial activity. However, ammonia concentration data is not available to confirm this theory. From Day 32 until the end of the trial, the average pH further increased, but remained below 6 (Figure 4D).
For all three treatments, n-caproic acid concentrations increased rapidly when the pH was >5.0 and banana mash was available as substrate. Similarly, Riungu et al. (2018b) found that co-digestion of UDDT-SW and food waste with a pH >5 resulted in higher total volatile fatty acid concentrations than when UDDT-SW was digested alone. Here, we achieved average final n-caproic acid concentrations for Treatments 1, 2, and 3 of 35.4, 43.1, and 53.7 mM, respectively (Table 3). If we had not stopped the trial on Day 56 of the operating period, the longer fermentation period would likely have resulted in even higher concentrations because the concentrations were still increasing. During Experiments 1 and 2, we observed a maximum average n-caproic acid concentration of 22.4 mM (Figures 1B, 2B), while the maximum average n-caproic acid concentration previously had been 27.1 mM (Harroff et al., 2017). The likely reason for the highest concentrations of n-caproic acid during Experiment 3, is the relatively high conversion efficiency of n-butyric acid into n-caproic acid, explaining the lower concentrations of n-butyric acid during Experiment 3 than what we have observed previously (Table 3, Figure S8) (Harroff et al., 2017).
After increasing the temperature, we observed a larger difference in results between the two barrels per treatment than before for both the carboxylic acid concentrations and the pH (Figure 4, Figure S8). Particularly for Treatment 3, one barrel lagged behind the other one (Figure 4B). For one barrel, the pH immediately increased from 4.7 to 5.6 between Day 29 and Day 32, and the total concentration of n-caproic acid increased simultaneously. For the second Treatment 3 barrel, the pH increased more slowly after Day 29 and was not >4.8 until Day 44. Accordingly, the total concentration of n-caproic acid in that bioreactor increased from 3.6 mM on Day 42 to 18.0 mM on Day 44. The pH remained above 4.8 after that point, and the total n-caproic acid concentration increased steadily until it reached the same concentration as the duplicate barrel on Day 56 (Figures 4B,D). Regardless of the precise onset of carboxylic acid production, banana waste was rapidly converted to carboxylic acids when the pH was above 5.0 at the higher temperature (Figures 4A,B, Figures S8A,B).
Undissociated n-caproic acid concentrations were also higher than we have observed previously (Figures 4C,D). The average pH in all treatments was above the pKa by the end of this experiment, which caused <50% of the total concentration to be in the undissociated form. However, a pH of 5 combined with the relatively high total concentrations of n-caproic acid resulted in undissociated n-caproic acid concentrations of 10.2, 13.1, and 12.6 mM for Treatments 1, 2, and 3, respectively (Figures 4C,D). The highest undissociated n-caproic acid concentration observed, thus far, was 7.1 mM in the 20% banana-mash treatment from Experiment 2. For Treatment 3, an unexpected trend for undissociated n-caproic acid was observed around Day 44 of the operating period because the total concentration of n-caproic acid steadily increased as anticipated, but the undissociated concentration increased between Day 42 and 44 and then decreased between Day 44 and 46 (Figure 4D). This inconsistency occurred because of non-homogenous conditions within the barrels, resulting in changes in the average pH between sampling days. The pH on Day 44 was slightly lower than on Day 46 (Figure 4D), and small differences in pH result in large differences in undissociated carboxylic acid concentrations due to the exponential relationship between the two. This result is noteworthy because it demonstrates that non-homogenous conditions within a bioreactor can result in large differences in efficacy for pathogen inactivation.
Additional Work Is Needed to Optimize Co-fermentation Conditions
The results here suggest that banana waste (or alternative food waste) co-fermentation rates should be optimized to result in a pH of about 5, which will maximize undissociated carboxylic acid concentrations by promoting a large fraction of the total concentration to be in the undissociated form, while avoiding inhibition that appears to result at pH values below 4.8. One goal of the current study was to determine whether a two-stage (Treatments 1 and 2) or one-stage (Treatment 3) process was preferred for accomplishing this goal. The final concentrations of undissociated n-caproic acid were similar between the three treatments, but they were also still increasing when the experiment ended on Day 56 (Figures 4C,D). With additional fermentation time, more differences may have been observed between the treatments. Therefore, it is difficult for us to make a firm conclusion about which treatment performed better. If the experiment is repeated with temperatures at 30°C during the entire experiment, fermentation should occur faster, and differences between the treatments may become apparent with less time required. When resulting performances in the one-stage and two-stage processes are determined to be equivalent, then a one-stage process is strongly preferred because it is simpler to operate and implement. Additional work should also be performed to determine optimal ratios of food waste to UDDT-SW that will result in a pH of ~5.
Ascaris Inactivation in Field-Scale Trial
We only collected Ascaris data at two time points (Days 11 and 29) during the field-scale trial and only during the low temperature period (Table 4). One of the duplicate Treatment 1 barrels caused lower viability than any of the others for unknown reasons. With the exception of this barrel, viabilities for the Ascaris eggs exposed in the barrels were similar to the eggs contained in tubes of autoclaved UDDT-SW. The similarity between the barrels and autoclaved UDDT-SW are supported by the slow fermentation rates observed during this time period. The barrels did not have high undissociated carboxylic acid concentrations until after Day 42 (Figures 4C,D, Figures S8C,D). Therefore, we expect that the conditions in the barrels were similar to the autoclaved UDDT-SW between Day 0 and Day 29.
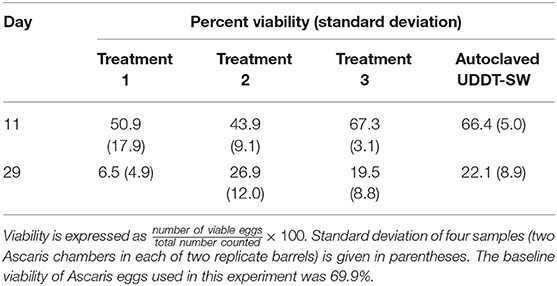
Table 4. Average percent viability of Ascaris eggs collected for field-scale trial during Experiment 3.
The viability of Ascaris eggs in all of the barrels plus the autoclaved UDDT-SW decreased considerably between Day 11 and Day 29 (Table 4). Because undissociated carboxylic acid concentrations were still low at this time, we expect that the decline in viability was related to confounding factors in the UDDT-SW that were not measured here. Therefore, we did not collect additional Ascaris samples beyond 29 days because we recognized that we would not be able to identify the cause of inactivation or to determine the effect of undissociated carboxylic acid concentrations.
Conclusions
Several findings were made in this study that improve our understanding of how carboxylic acid fermentation can be used as part of a sanitation waste treatment system to inactive pathogens:
1. Fermentation of UDDT-SW in combination with food waste can be used to inactivate Ascaris eggs in a real sanitation setting when the carboxylic acid product spectrum is shifted toward longer-chain carboxylic acids, including n-butyric acid, n-valeric acid, and n-caproic acid, rather than just acetic acid.
2. A specialized inoculum of carboxylic acid-producing bacteria is not necessary because the gut microbiome found in UDDT-SW is already capable of producing carboxylic acids with longer carbon chains.
3. Fermentation temperatures should be higher than 30°C to promote reasonable rates of carboxylic acid production. Lower temperatures will result in longer required fermentation times or no carboxylic acid production at all. For waste treatment systems, long fermentation times require larger and more expensive bioreactors.
4. When easily degraded food waste, such as banana waste, is co-fermented with UDDT-SW in a way that maintains a pH between 4.8 and 5.2, we can accomplish our two main goals of: (1) producing high total concentrations of carboxylic acids; and (2) reducing the pH to cause a greater fraction of the total concentration to exist in the undissociated form. If the pH is below 4.8, then carboxylic acid production is inhibited. If the pH is above ~5.2, the fraction of carboxylic acids in the undissociated form is too low for the process to effectively inactivate pathogens.
5. The timing of food waste addition and ratio of food waste to UDDT-SW can both be optimized in a two-stage process to maintain an appropriate pH. However, for practical implementation of waste treatment systems, it is simpler to mix food waste and UDDT-SW when the fermentation is started. Therefore, an appropriate ratio of food waste should be found that could maintain the appropriate pH. This ratio will need to be adapted to individual systems based on composition and buffering capacity of the UDDT-SW and food waste used.
6. Ascaris eggs were inactivated faster when food waste was used to increase concentrations of undissociated carboxylic acids, but more investigation will be needed to determine appropriate operating conditions to inactivate pathogens, including Ascaris eggs.
Data Availability Statement
The datasets generated for this study are available on request to the corresponding author.
Author Contributions
LA directed the experimental design and overall concept and provided guidance to LH. DB and JL guided Ascaris methodology and experimental design. TE and EW assisted in design and implementation of the field-scale trial. LH designed and conducted the experiments, collected samples, and analyzed data. LH wrote the manuscript together with LA, with further revisions from DB, TE, EW, and JL.
Funding
LH acknowledges support from the Cross-Scale Biogeochemistry and Climate NSF IGERT program (Award #1069193) and the NSF Graduate Research Fellowship Program (DGE-1650441). LA acknowledges support from the Alexander von Humboldt Foundation in the framework of the Alexander von Humboldt Professorship endowed by the Federal Ministry of Education and Research in Germany. We acknowledge support by the Deutsche Forschungsgemeinschaft and Open Access Publishing Fund of University of Tübingen.
Conflict of Interest
EW and TE were employed by company Sanergy, Nairobi.
The remaining authors declare that the research was conducted in the absence of any commercial or financial relationships that could be construed as a potential conflict of interest.
Acknowledgments
We thank Daniel Kyalo, Davis Ireri, Clifford Odhiambo, Eunice Muthui, Ricky Ojwang, and Jimmy Kirui at Sanergy for valuable assistance in organizing logistics, assisting in setup, and sampling for the field-scale trial. We also thank Lucinda Li for her work investigating Ascaris inactivation by lactic acid.
Supplementary Material
The Supplementary Material for this article can be found online at: https://www.frontiersin.org/articles/10.3389/fenvs.2019.00139/full#supplementary-material
References
Abdul, P., and Lloyd, D. (1985). Pathogen survival during anaerobic digestion: fatty acids inhibit anaerobic growth of Escherichia coli. Biotechnol. Lett. 7, 125–128. doi: 10.1007/BF01026683
Agler, M. T., Werner, J. J., Iten, L. B., Dekker, A., Cotta, M. A., Dien, B. S., et al. (2012). Shaping reactor microbiomes to produce the fuel precursor n-butyrate from pretreated cellulosic hydrolysates. Environ. Sci. Technol. 46, 10229–10238. doi: 10.1021/es302352c
Agler, M. T., Wrenn, B. A., Zinder, S. H., and Angenent, L. T. (2011). Waste to bioproduct conversion with undefined mixed cultures: the carboxylate platform. Trends Biotechnol. 29, 70–78. doi: 10.1016/j.tibtech.2010.11.006
Amoah, I. D., Reddy, P., Seidu, R., and Stenström, T. A. (2018). Concentration of soil-transmitted helminth eggs in sludge from South Africa and Senegal: a probabilistic estimation of infection risks associated with agricultural application. J. Environ. Manage. 206, 1020–1027. doi: 10.1016/j.jenvman.2017.12.003
Bowman, D. D., Little, M. D., and Reimers, R. S. (2003). Precision and accuracy of an assay for detecting Ascaris eggs in various biosolid matrices. Water Res. 37, 2063–2072. doi: 10.1016/S0043-1354(02)00597-3
Butkus, M. A., Hughes, K. T., Bowman, D. D., Liotta, J. L., Jenkins, M. B., and Labare, M. P. (2011). Inactivation of Ascaris suum by short-chain fatty acids. Appl. Environ. Microbiol. 77, 363–366. doi: 10.1128/AEM.01675-10
Cummings, J., Pomare, E., Branch, W., Naylor, C., and Macfarlane, G. (1987). Short chain fatty acids in human large intestine, portal, hepatic and venous blood. Gut 28, 1221–1227. doi: 10.1136/gut.28.10.1221
Fidjeland, J., Nordin, A., Pecson, B. M., Nelson, K. L., and Vinnerås, B. (2015). Modeling the inactivation of Ascaris eggs as a function of ammonia concentration and temperature. Water Res. 83, 153–160. doi: 10.1016/j.watres.2015.06.030
Fidjeland, J., Nordin, A., and Vinnerås, B. (2016). Inactivation of Ascaris eggs and Salmonella spp. in fecal sludge by treatment with urea and ammonia solution. J. Water Sanit. Hyg. Dev. 6, 465–473. doi: 10.2166/washdev.2016.017
Flint, H. J., Duncan, S. H., Scott, K. P., and Louis, P. (2015). Links between diet, gut microbiota composition and gut metabolism. Proc. Nutr. Soc. 74, 13–22. doi: 10.1017/S0029665114001463
Freese, E., Sheu, C. W., and Galliers, E. (1973). Function of lipophilic acids as antimicrobial food additives. Nature 241, 321–325. doi: 10.1038/241321a0
Ge, S., Usack, J. G., Spirito, C. M., and Angenent, L. T. (2015). Long-term n-caproic acid production from yeast-fermentation beer in an anaerobic bioreactor with continuous product extraction. Environ. Sci. Technol. 49, 8012–8021. doi: 10.1021/acs.est.5b00238
Harroff, L. A., Liotta, J. L., Bowman, D. D., and Angenent, L. T. (2017). Inactivation of Ascaris eggs in human fecal material through in situ production of carboxylic acids. Environ. Sci. Technol. 51, 9729–9738. doi: 10.1021/acs.est.7b02014
Johnson, P., Dixon, R., and Ross, A. (1998). An in-vitro test for assessing the viability of Ascaris suum eggs exposed to various sewage treatment processes. Int. J. Parasitol. 28, 627–633. doi: 10.1016/S0020-7519(97)00210-5
Kucek, L. A., Nguyen, M., and Angenent, L. T. (2016). Conversion of L-lactate into n-caproate by a continuously fed reactor microbiome. Water Res. 93, 163–171. doi: 10.1016/j.watres.2016.02.018
Liang, S., and Wan, C. (2015). Carboxylic acid production from brewer's spent grain via mixed culture fermentation. Bioresour. Technol. 182, 179–183. doi: 10.1016/j.biortech.2015.01.082
Manser, N. D., Wald, I., Ergas, S. J., Izurieta, R., and Mihelcic, J. R. (2015). Assessing the fate of Ascaris suum ova during mesophilic anaerobic digestion. Environ. Sci. Technol. 49, 3128–3135. doi: 10.1021/es505807a
Miller, T. L., and Wolin, M. J. (1996). Pathways of acetate, propionate, and butyrate formation by the human fecal microbial flora. Appl. Environ. Microbiol. 62, 1589–1592.
Nelson, K. L., and Darby, J. L. (2002). Determination of the inactivation rate of Ascaris eggs in wastewater stabilization pond sludge using dialysis chambers and sludge cores. Water Environ. Res. 74, 362–369. doi: 10.2175/106143002X140125
Nordin, A., Nyberg, K., and Vinnerås, B. (2009). Inactivation of Ascaris eggs in source-separated urine and feces by ammonia at ambient temperatures. Appl. Environ. Microbiol. 75, 662–667. doi: 10.1128/AEM.01250-08
Procházka, J., Dolejš, P., Máca, J., and Dohányos, M. (2012). Stability and inhibition of anaerobic processes caused by insufficiency or excess of ammonia nitrogen. Appl. Microbiol. Biotechnol. 93, 439–447. doi: 10.1007/s00253-011-3625-4
Rajagopal, R., Ahamed, A., and Wang, J.-Y. (2014). Hydrolytic and acidogenic fermentation potential of food waste with source segregated feces-without-urine as co-substrate. Bioresour. Technol. 167, 564–568. doi: 10.1016/j.biortech.2014.06.024
Riungu, J., Ronteltap, M., and van Lier, J. B. (2018a). Build-up and impact of volatile fatty acids on E. coli and A. lumbricoides during co-digestion of urine diverting dehydrating toilet (UDDT-F) faeces. J. Environ. Manage. 215, 22–31. doi: 10.1016/j.jenvman.2018.02.076
Riungu, J., Ronteltap, M., and van Lier, J. B. (2018b). Volatile fatty acids build-up and its effect on E. coli inactivation during excreta stabilisation in single-stage and two-stage systems. J. Water Sanit. Hyg. Dev. 8, 257–267. doi: 10.2166/washdev.2018.160
Rose, C., Parker, A., Jefferson, B., and Cartmell, E. (2015). The characterization of feces and urine: a review of the literature to inform advanced treatment technology. Crit. Rev. Environ. Sci. Technol. 45, 1827–1879. doi: 10.1080/10643389.2014.1000761
Royce, L. A., Liu, P., Stebbins, M. J., Hanson, B. C., and Jarboe, L. R. (2013). The damaging effects of short chain fatty acids on Escherichia coli membranes. Appl. Microbiol. Biotechnol. 97, 8317–8327. doi: 10.1007/s00253-013-5113-5
Salsali, H. R., Parker, W. J., and Sattar, S. A. (2006). Impact of concentration, temperature, and pH on inactivation of Salmonella spp. by volatile fatty acids in anaerobic digestion. Can. J. Microbiol. 52, 279–286. doi: 10.1139/w05-125
Spirito, C. M., Richter, H., Rabaey, K., Stams, A. J., and Angenent, L. T. (2014). Chain elongation in anaerobic reactor microbiomes to recover resources from waste. Curr. Opin. Biotechnol. 27, 115–122. doi: 10.1016/j.copbio.2014.01.003
Trönnberg, L., Hawksworth, D., Hansen, A., Archer, C., and Stenström, T. A. (2010). Household-based prevalence of helminths and parasitic protozoa in rural KwaZulu-Natal, South Africa, assessed from faecal vault sampling. Trans. R. Soc. Trop. Med. Hyg. 104, 646–652. doi: 10.1016/j.trstmh.2010.06.009
Usack, J., and Angenent, L. (2015). Comparing the inhibitory thresholds of dairy manure co-digesters after prolonged acclimation periods: part 1–Performance and operating limits. Water Res. 87, 446–457. doi: 10.1016/j.watres.2015.05.055
Wang, K., Yin, J., Shen, D., and Li, N. (2014). Anaerobic digestion of food waste for volatile fatty acids (VFAs) production with different types of inoculum: effect of pH. Bioresour. Technol. 161, 395–401. doi: 10.1016/j.biortech.2014.03.088
Keywords: carboxylic acids, volatile fatty acids, Ascaris, sanitation, pathogen inactivation
Citation: Harroff LA, Liotta JL, Wangolo EE, Egan TJS, Bowman DD and Angenent LT (2019) Field-Scale Co-fermentation of Solid Waste From Urine-Diverting Dry Toilets (UDDT-SW) and Banana Waste to Produce Undissociated Carboxylic Acids to Inactivate Ascaris Eggs. Front. Environ. Sci. 7:139. doi: 10.3389/fenvs.2019.00139
Received: 04 July 2019; Accepted: 05 September 2019;
Published: 24 September 2019.
Edited by:
Alberto Tiraferri, Politecnico di Torino, ItalyCopyright © 2019 Harroff, Liotta, Wangolo, Egan, Bowman and Angenent. This is an open-access article distributed under the terms of the Creative Commons Attribution License (CC BY). The use, distribution or reproduction in other forums is permitted, provided the original author(s) and the copyright owner(s) are credited and that the original publication in this journal is cited, in accordance with accepted academic practice. No use, distribution or reproduction is permitted which does not comply with these terms.
*Correspondence: Largus T. Angenent, l.angenent@uni-tuebingen.de