- 1Department of Soil sciences, Soils and Fertilizers Research Institute (SFRI), Hanoi, Vietnam
- 2Centre IRD, Institute of Ecology and Environmental Sciences (UMR 242 iEES-Paris), Sorbonne Universités, Bondy, France
Soil bioturbation is associated with the production of soil macropores that influence numerous ecological functions such as those associated with water infiltration and the generation of runoff water. This impact is especially important on sloping lands in the tropics that are highly susceptible to erosion. In this study, we questioned the influence of soil biodiversity on soil macropore properties (>20 mm3) and saturated hydraulic conductivity (Ksat) on sloping land in northern Vietnam. Biostructures found at the soil surface (casts, sheetings, and soil excavated on the ground) were used to identify areas colonized either by earthworms, termites or dung beetles, respectively. The influence of soil macrofauna on Ksat was measured in situ using the Beerkan method below bioturbated zones and compared to the surrounding soil without visible biostructures at the soil surface. Undisturbed soil columns were afterwards sampled and scanned by X-ray computed tomography (X-ray CT). Properties of macropores below each biostructure depicted a large variability, revealing the complexity of the macropore network. Further, galleries made by termites, dung beetles, and earthworms were manually isolated from the rest of macroporosity. Galleries made by beetles, termites and earthworms were clearly differentiated on the basis of their diameter, verticality, sphericity, tortuosity, length and number of branches and the fraction of galleries in the top part of the column. Ksat was most increased by dung beetles (45-fold), then by termites (30-fold) and to a lesser extent by earthworms (16-fold). Relationships between total macropore properties and Ksat showed that the most important properties explaining Ksat were (i) the volume of percolating macropores, (ii) the diameter, (iii) the critical macropore diameter, and (iv) the number of macropores. In conclusion, this study confirmed not only the interest in using X-ray CT for the quantification of macroporosity but also the absence of a clear relationship between aboveground biostructures and macropore properties and functional impacts.
Introduction
Soil structure regulates many key ecological processes in soils, such as those influencing the habitat of soil organisms, the growth of roots, the protection of carbon, the release of mineral nutrients or the infiltration and diffusion of water in soil. In a recent review, Rabot et al. (2018) differentiated two complementary approaches for understanding the dynamic of soil structure: the solid and pore perspectives. From the solid-phase perspective, the dynamic of soil structure is considered through the organization and dynamic of soil aggregates. This perspective is useful for understanding the habitat of microbes and the dynamics of carbon and nutrients in soil (e.g., Six et al., 2004). Conversely, the pore-phase perspective considers soil architecture through its voids and the properties of the soil pore network (Young et al., 2001), in particular their influence on the water dynamic (e.g., Beven and Germann, 1982; Jarvis, 2007; Luo et al., 2010). Although the dynamic of soil aggregates has long been debated (e.g., Tisdall and Oades, 1982; Oades and Waters, 1991), the importance and dynamic of soil porosity on the water dynamic in soil have only recently gained in knowledge with the development of non-destructive and non-invasive scanning techniques by X-ray computerized tomography (X-ray CT). During the last decades, X-ray CT has been applied in many different studies exploring the architecture and functions of soils. The application of X-ray CT has expanded rapidly, now covering the characterization of pore space and bulk density for different land use and management systems (e.g., Anderson et al., 1990; Luo et al., 2010; Capowiez et al., 2011; Larsbo et al., 2014; Naveed et al., 2016; Jarvis et al., 2017). Furthermore, X-ray CT has been used widely to non-destructively quantify earthworm bioturbation in repacked soil cores (Joschko et al., 1991; Jégou et al., 1997; Langmaack et al., 1999; Capowiez et al., 2001, 2011; Bastardie et al., 2003) or undistributed natural soil cores (Pierret et al., 2002; Bastardie et al., 2005). The interest in X-ray CT relies on its description of the pore size distribution, connectivity, continuity, tortuosity and length, which are all considered to influence soil hydraulic properties (Perret et al., 1999; Vogel, 2000; Pierret et al., 2002).
The influence of soil biota on the properties of soil aggregates has been largely considered, especially with roots, earthworms and the production of casts or termites and the production of mounds and sheetings (e.g., Six et al., 2004; Bottinelli et al., 2015). Information about the influence of soil biodiversity on soil porosity and thus on the dynamic of water in soil remain, however, very limited to studies that have mainly been carried out in controlled conditions with earthworms (e.g., Capowiez et al., 2015; Bottinelli et al., 2017). Therefore, a clear dearth of information exists on how the other soil bioturbators influence soil porosity and the water dynamic in non-perturbed environments, which justifies the need to describe the properties of galleries produced by soil fauna. Hence, the objectives of this study were to use X-ray CT to (i) provide quantitative data of the galleries made by the most important soil engineers (sensu Jones et al., 1994, 1997) in tropical soils, namely, termites, beetles and earthworms (e.g., Lavelle et al., 1997; Jouquet et al., 2006; Filser et al., 2016) and (ii) determine how their macropores impacted water infiltration in soil.
Materials and Methods
Study Site
This study was carried out at the M-Tropics long-term observatory (46 ha) located in Dong Cao Village in the northeast of Vietnam, approximately 60 km southwest of Hanoi (20° 57′N, 105° 29′E). The annual rainfall ranges from 1,500 to 1,800 mm, and 80–85% of total rainfall is concentrated during the rainy season from April to October. The humidity is always high between 75 and 80% (Jouquet et al., 2008a). The mean daily temperature varies between 15 and 25°C (Jouquet et al., 2008b). Soils derive from the weathering of volcanic sedimentary schists of the Mesozoic age and are mainly described as Acrisols (WRB, 1998) or Ultisols (Podwojewski et al., 2008; Soil Survey Staff, 2014). The soils are dominated by clay particles (>50%, mainly kaolinite) and contain ~12 and 40% of sand and silt, respectively (Jouquet et al., 2008b). The vegetation is a deciduous forest dominated by Vernicia montana (Euphorbiaceae) and Brachiaria ruiziziensis (Poaceae) (De Rouw, unpublished data). The itinerant pasture of buffaloes in the watershed leads to the production of buffalo dung that is very attractive for dung beetles (Scarabaeidae). The study site is also characterized by high activity of earthworms (mainly Amynthas khami) and termites (mainly fungus-growing termites) (Jouquet et al., 2012). The experiment took place during the rainy season in September 2017 when the activity of soil macrofauna is considered to be the most important.
Soil Macrofauna Diversity
Soil macrofauna (>2 mm in size) were collected using the TSBF method (Anderson and Ingram, 1993) below the soil excavated by dung beetles (DB), termite sheetings (TS), and earthworm casts (EC) and in the control surrounding soil environment without visible trace of soil macrofauna (Ctrl). Soil fauna were removed by hand sorting from 25 × 25 cm wide and 30 cm deep blocks (n = 3). Individuals were preserved in 70% alcohol before counting. Regarding their occurrence, individuals were classified into 4 taxonomic groups: beetles, termites, ants, and earthworms.
Soil Hydraulic Conductivity
Saturated hydraulic conductivity (Ksat) was measured in situ using the Beerkan method (Lassabatère et al., 2006) below DB, TS, and EC and in the Ctrl (n = 3 per treatment). PVC cylinders (14 cm height and 13 cm in diameter) were positioned at the soil surface and inserted to a depth of approximately 1 cm to avoid lateral loss of the ponded water at the soil surface. A fixed volume of water (100 ml, corresponding to a water layer of 1 cm) was poured into the cylinder, and the time needed for the water to infiltrate was measured. The procedure was repeated between 7 and 10 times to reach a steady state of infiltration. Soil cores (100 cm3) were used to determine the soil bulk density and the initial water content in the surrounding soil (0–5 cm depth). The results were analyzed with the original BEST algorithm (Lassabatère et al., 2006) in order to estimate Ksat.
Quantification of Macropores and Galleries
After measuring water infiltration, soil cores were excavated by gently inserting the PVC pipes into the soil to a depth of 10 cm (n = 3). All cores were scanned using medical X-ray CT (Siemens Somatom® Definition Flash) at the Bach Maï hospital (Hanoï, Vietnam) to obtain a set of 0.6 mm thick images with a pixel size of 0.3 mm. The X-ray beam was operated at 93 mA and 120 kV. Images (16-bit DICOM format, 512 × 512 pixels) were transformed into 8-bits TIFF format and rendered isotropic with a resolution of 0.3 mm. Prior to segmentation, a 3-D Median filter with a radius of two voxels size was applied in order to reduce noise and scatter. Since the gray-level of histograms was bi-modal, the automatic Otsu thresholding method was used (Otsu, 1979). Image processing and quantification were conducted with the open-source software ImageJ version 1.51 (Schneider et al., 2012).
After the images were preprocessed, soil macrofauna macropores inside each core were selected by removing pores <20 mm3 in order to reduce noise and exclude roots. Characteristics of total macropores were then described based on their number, volume (largest volume, volume of the pores connected to the surface, volume of the pores connected to the bottom and the percolating volume), diameter (the mean diameter and the critical diameter of the percolating macropores) and global connectivity (Γ), which reflects the probability of two randomly chosen pore voxels to belong to the same macropore cluster (Renard and Allard, 2013). Macropores were then reconstructed and visualized using AvizoFire 8.1.
Field observations revealed that beetles produced larger galleries (~5–6 mm in diameter) than termites (<3 mm in diameter), while earthworms produced intermediate galleries (~3–4 mm). Further, anecic earthworm species are also well-known to make large and vertical burrows open to the surface (Capowiez et al., 2011). From these observations, galleries made by earthworms, beetles, and termites were manually isolated from the total macroporosity based on their body size and shape (Figure 1) and using the option “volume edit” in the Avizo 8.1 software. Galleries were then described by measuring their (i) diameter, (ii) verticality (orientation or angle between the maximum Feret diameter of the object and the XY plane), (iii) tortuosity (the ratio between the actual branch length >10 mm of the object and the Euclidean distance along the skeleton), (iv) sphericity (the ratio between the volume and surface of the object), (v) total length of galleries (sum of branches with length >10 mm after skeletonization), (vi) number of branches (number of branches with length >10 mm after skeletonization), and (vii) fraction of galleries volume in upper part (fraction of the galleries volume in the top part of the column).
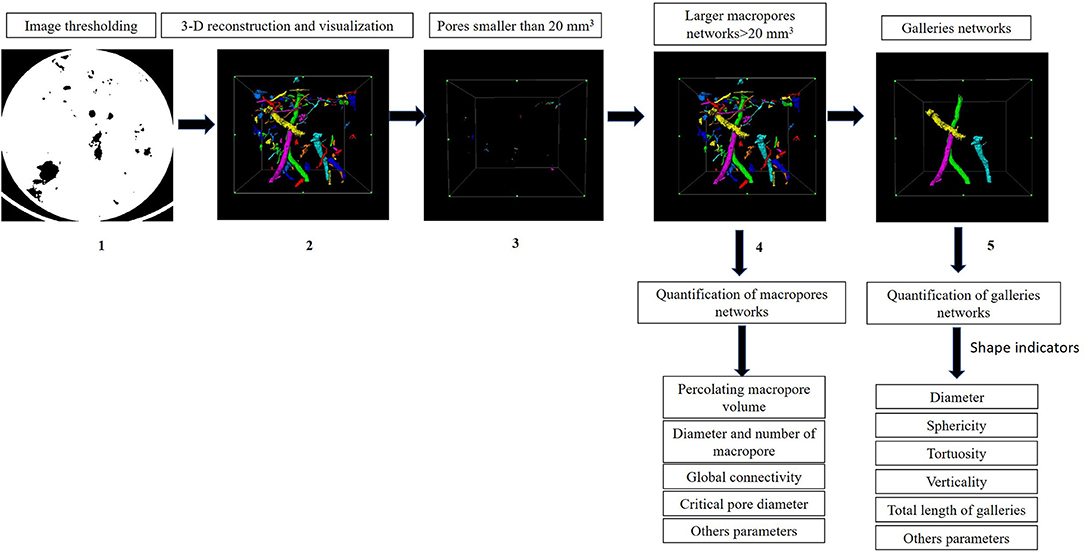
Figure 1. Procedure used for image analysis and quantification of macropores below soil macrofauna biostructures and the differentiation of macropores and galleries. (1) Segmentation of the image. (2) Reconstruction and visualization of the total macroporosity. (3) Representation of the pores <20 mm3 that were discarded to reduce noise. (4) Representation of macropores >20 mm3. (5) Galleries manually isolated from macropores.
Statistical Analyses
Prior to analysis, the homogeneity of variances was inspected using Levene's test, and data were log-transformed if needed. One-way analysis of variance (ANOVA) and least significant difference (LSD) tests were performed to assess differences between means. To visually resume the information from the soil total macroporosity and galleries properties, an ordination method (principal component analysis, PCA) was applied using “ade4” packages in R. Partial least squares regression (PLSR) analysis was performed to predict important total macropores variables associated with soil hydraulic conductivity using the “pls” package (Mevik and Wehrens, 2007). All statistical calculations were carried out using R version 3.5.1. Differences among treatments were declared significant at the <0.05 probability level.
Results
Soil Macrofauna
Four dominant soil macrofauna groups were identified across the study area, namely, earthworms, beetles, ants and termites. The abundance of soil macrofauna was influenced by the different treatments (Table 1). Ants were in all the treatments and in large numbers, especially in the Ctrl treatment, although they could not be clearly associated with any specific galleries. Termites were mainly found in the TS treatment and to a lesser extent in the Ctrl treatment. Termites belonged to soil-feeding termites in Ctrl, while they belonged to the fungus-growing termite taxon in TS (subfamily Macrotermitinae, Odontotermes spp.). Beetles were exclusively found below DB, while endogeic earthworms (small-sized and non-pigmented) were only found beneath EC. In total, 62.4, 29.1, 4.2, and 4% of the total number of individuals (n = 165) were ants, termites, beetles or earthworms, respectively.
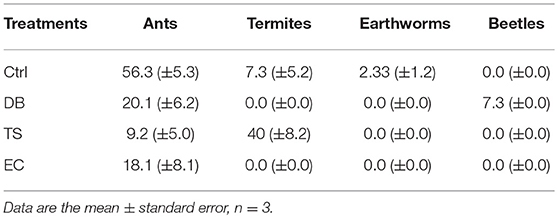
Table 1. Abundance of soil macrofauna (ind m−2) (n = 3) collected below termite sheetings (TS), dung beetles (DB), and earthworm casts (EC) and in control (Ctrl) treatments.
Visualization and Quantification of the Macropore Network
The three-dimensional visualization of the macroporosity within the columns is shown in Supplementary File 1. Macropore characteristics were obviously different among the different treatments. We observed also different macropores with different origins. Figure 2 shows the PCA obtained from the properties of the macropores (data used for computing the PCA are shown in Table 2). Treatments were mainly differentiated along the first axis of the PCA that explained 61.7% of the total variability, while variability within treatment was mainly evident on the second axis of the PCA (17% of the total variability). The DB treatment was clearly differentiated from the Ctrl treatment, while overlaps were observed among EC, TS and Ctrl treatments. From the different variables, only two were significantly influenced by the treatments (i.e., the volume of the total macroporosity and the volume of the largest pore) (ANOVA test, P < 0.05 in both cases). The volume of the total soil macroporosity was highest in DB and EC (P > 0.05 between the two) and lowest in Ctrl, while an intermediate value was reached in TS (P > 0.05 with EC and Ctrl). The largest pores were also measured in DB in comparison with those in the other treatments (P > 0.05 between them).
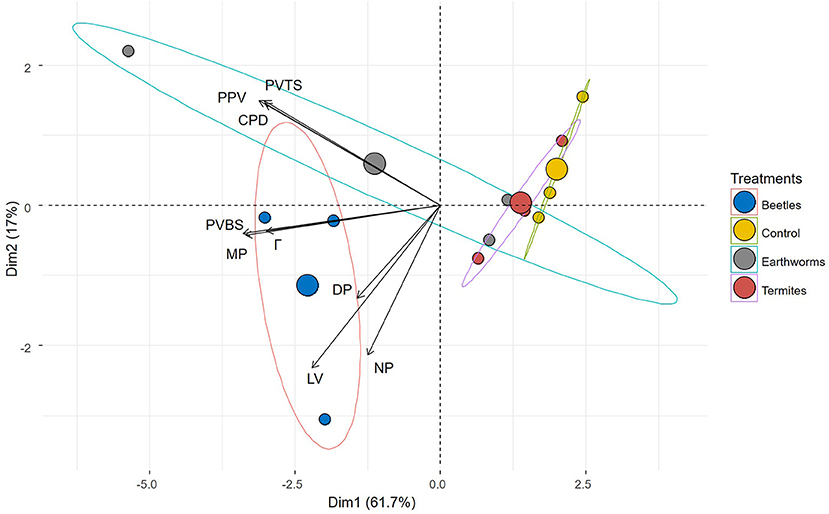
Figure 2. Biplot showing the principal components analysis (PCA) from variables describing soil macroporosity for the different treatments (control in yellow, termite sheetings in red, dung beetles in blue and earthworm casts in gray) in the plane defined by axes 1 and 2 of the PCA. Variables are number of macropores (NP), macroporosity (%) (MP), pore diameter (mm) (DP), macropore volume connected to the surface (mm3) (PVTS), macropore volume connected to the bottom surface (mm3) (PVBS), percolating macropore volume (mm3) (PPV), largest volume (mm3) (LV), critical pore diameter (mm) (CPD), and connection probability (Γ).
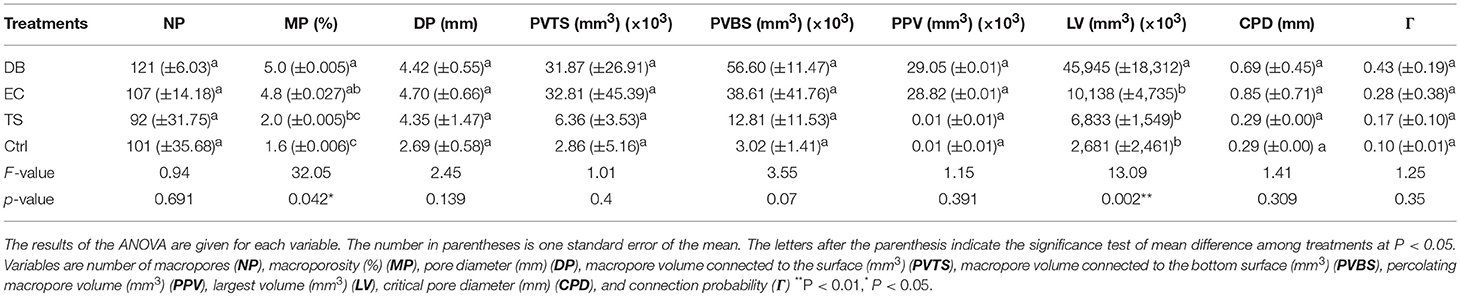
Table 2. Influence of the treatments [termite sheetings (TS), dung beetles (DB), earthworm casts (EC) and control (Ctrl)] on soil macroporosity.
Geometrical Properties of Soil Macrofauna Galleries
Galleries made by beetles, termites and earthworms are shown in Figure 3. Treatments were clearly differentiated along the first and second axes of the PCA, which explained 62.3 and 16.1% of the total variability, respectively (Figure 4). Galleries made by beetles were characterized by their large diameter (5.8 mm on average) and their verticality (52° on average) (Table 3). Conversely, TS galleries were relatively small (~2 mm in diam.). Galleries were also markedly horizontal (~32°). Finally, earthworm galleries had intermediate size with a diameter of 4 mm on average and were markedly vertical (51°) in comparison with those made by termites. The total length of galleries network and the number of branches were calculated based on their skeletons. Galleries of beetles were longer with more branches than those of termites and earthworms. No significant difference was found among treatments in terms of sphericity, tortuosity and fraction of the galleries volume contained in the top part of the column (P > 0.05 in both cases).
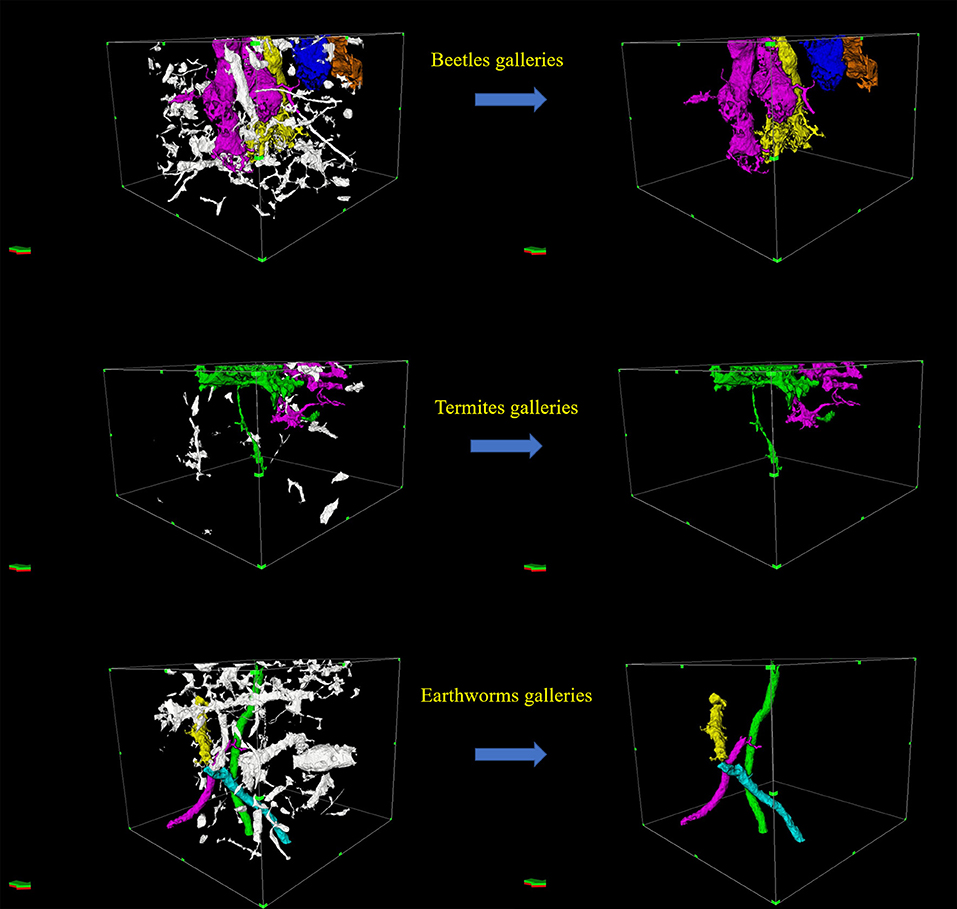
Figure 3. Examples of three-dimensional images of galleries made by beetles, termites and earthworms (Right) manually isolated from macropores (in white: Left).
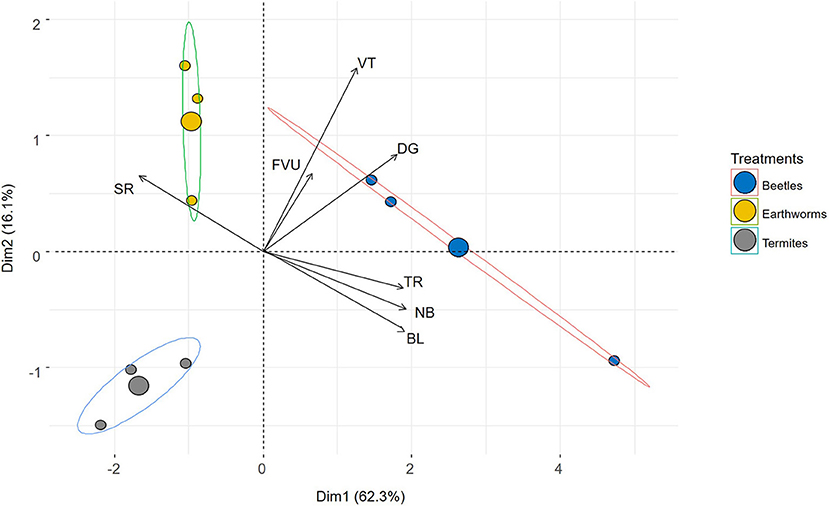
Figure 4. Biplot showing the PCA made from the 3-D characteristics of galleries made by beetles, termites and earthworms. Variables include the diameter of galleries (mm) (DG), their tortuosity (–) (TR), sphericity (SR) (–), verticality (°) (VT), total length of galleries 10 (mm) (BL), number of branches 10 (NB) (–), and fraction of galleries volume in upper part (FVU). The correlation circle is also given on the right side. Ordination of the samples is in the plane defined by axes 1 and 2 of the PCA.
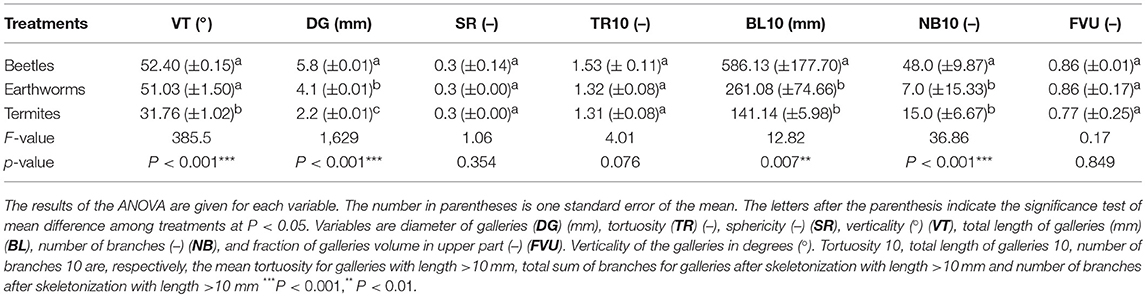
Table 3. Influence of the treatments on the morphological characteristics of galleries created by beetles, earthworms and termites derived from X-ray CT image analysis.
Impact of Soil Macrofauna on Ksat
Figure 5 shows that Ksat was significantly influenced by soil macrofauna activity [ANOVA test, F(3, 8) = 6.39, P = 0.03], and Ksat increased by 45 and 30-fold in DB and TS in comparison with that in Ctrl (P < 0.05). Earthworm activity also increased Ksat by 16-fold in comparison with that in Ctrl, although this difference was not significant (P > 0.05). The best model was obtained when only the diameter and the number of pores, the volume of the percolating pores and the critical pore diameter were considered (RMSEP = 0.55, Q2 = 0.68) (Table 4).
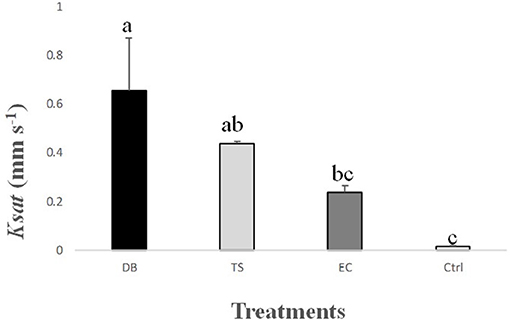
Figure 5. Influence of the treatments on Ksat (mm s−1). Treatments are “DB” for dung beetles, “TS” for termite sheetings, “EC” for earthworm casts, and “Ctrl” for control. Error bars represent the standard error of the mean. Histograms with the same letters are not significantly different at P = 0.05, n = 3.
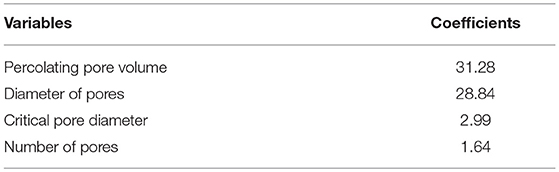
Table 4. Coefficient values from the most relevant variables used for the PLSR describing the evolution of soil hydraulic conductivity at saturation (Ksat).
Discussion
Influence of Soil Macrofauna Activity on Soil Porosity
Anecic earthworms and fungus-growing termites produce specific casts and sheetings on the ground in the Dong Cao watershed (Jouquet et al., 2008b, 2009, 2012), while dung beetles excavate an important quantity of soil. Our study showed that these soil biogenic aggregates (sensu Bullock, 1985) were associated with complex macropore networks in soil. Despite specific signatures on the PCA, treatments were characterized by an important variability, most likely due to the low number of replicates (n = 3) and the presence of galleries that could be attributed to a variety of soil organisms. Since only macropores >20 mm3 were considered in this study, it is unlikely that these macropores corresponded to roots, while they could result from ant, termite and earthworm activities, which were abundantly found in soil. Although the morphological properties of ant nest chambers have been previously described (e.g., Mikheyev and Tschinkel, 2004), a clear lack of information exists concerning the shape of their galleries. The morphological properties of ant galleries remain unknown and most likely difficult to differentiate from those made by earthworms and termites in the field. Moreover, as highlighted by Cheik et al. (2018b), the lifetimes of galleries made by soil invertebrates are difficult to estimate, making it difficult to estimate the origin, age and functional impact of the numerous macropores that were observed in our study.
Regarding the variability of the macropore networks, galleries made by earthworms, beetles and termites were visually distinguished from the rest of the macroporosity and manually extracted from the images. Although the accuracy of this approach is likely to be site-dependent, and the approach probably minimizes the influence of these soil invertebrates on soil architecture, a clear distinction was revealed among the gallery types. Beetle galleries were significantly larger than those of the others (~6 mm in diameter) and marked by their verticality. Beetles had also the longest galleries networks and the highest number of branches. Although the size of their galleries is likely to vary depending on the size and functional group of beetle species (e.g., Slade et al., 2007), our result is in line with that of Mikus and Uchman (2013) who found that beetles make vertical galleries ranging from 6 to 11 mm in diameter in temperate ecosystems. Conversely, termite galleries were more connected to the upper part of the soil column with small galleries (~2 mm in diameter) mainly markedly horizontal. Although our study is the first to quantify the complexity of termite galleries using X-ray CT technology, our findings are in line with those of Kooyman and Onck (1987) who manually measured in the field gallery diameters ranging from 2 to 5 mm. Our study also confirms results obtained by Léonard and Rajot (2001) who found that galleries made by Odontotermes sp. (Macrotermitinae) are mainly horizontal and shallow within the first cm of soil in west Africa. The complexity of termite galleries was especially important in comparison with that of earthworm galleries, which were larger (~4 mm in diameter), mainly vertical and with the highest elongation index. Earthworm galleries were produced by A. khami, which was not found during the soil macrofauna sampling. This species might be very long (up to 70 cm) and goes down very quickly to the deep soil layers (>1 m, Jouquet pers. com.). This species is also considered to belong to the anecic functional group because its globular casts have similar isotopic signatures to those of the litter (e.g., Hong et al., 2011). Consistent with the properties of its casts, our study showed that its galleries are also characteristic of the anecic earthworm functional group, with vertical and percolating galleries open on the soil surface, as shown in laboratory conditions (e.g., Bastardie et al., 2003; Capowiez et al., 2015; Bottinelli et al., 2017).
Influence of Soil Macrofauna on Water Infiltration
Despite high variability in soil macroporosity, our treatments led to significant differences in Ksat with the highest values below DB (45-fold) followed by those of TS (30-fold) and EC (16-fold) in comparison with those in Ctrl. These results underline the importance of differentiating the influence of macropores made by earthworms, termites and beetles on water dynamic in our study site. The highest Ksat values measured for DB can be explained by the largest size of the galleries, most likely because of the harder and larger body diameter of beetles than that of earthworms and termites.
The positive influence of termites on water infiltration is mainly evident in dry environments such as in west Africa in comparison with the control surrounding environment (1.5- to 10.5-fold) (e.g., Mando et al., 1996, 1999; Léonard et al., 2001, 2004; Kaiser et al., 2017) and more recently in India (3–12-fold, Cheik et al., 2018a,b). Our findings showed that termite foraging activity also increased water infiltration in the humid tropical environment of Southeast Asia. However, these results have to be considered in light of another study carried out in the same study site by Jouquet et al. (2012), who showed that the fragmentation of termite sheeting on the ground by the rain leads to the production of soil crusts that reduce water infiltration and increase soil erosion. Consequently, it can be concluded that the impact of termite foraging activity on water infiltration results from a balance between two antagonistic processes (increasing water infiltration through the production of galleries vs. reducing water infiltration through the production of soil sheetings and then soil crusts on the surface), making any simple conclusion on the functional impact of termites difficult to establish.
Finally, our study confirmed the positive impact of anecic earthworm galleries on water infiltration (e.g., Fischer et al., 2014; Andriuzzi et al., 2015). Although the positive influence of earthworms on Ksat has been previously demonstrated, especially in temperate environments (van Schaik et al., 2014) or more specifically in our study site (e.g., Jouquet et al., 2008b, 2012), our study showed that earthworms only slightly improved Ksat in comparison with beetles and termites. Hydraulic conductivity strongly depends on the number and diameter of connected flow pathways. The results from the PLSR showed that four variables were used for the prediction of Ksat: the diameter and the number of pores, the volume of the percolating pores and the critical pore diameter. Interestingly, these variables were not influenced by the treatments, and a higher Ksat would have been expected with EC than that with TS because of the larger, more vertical and more elongated galleries of earthworms than those of termites. We explain these results by the fact that galleries made by termites and earthworms represented only a small proportion of the efficient macroporosity. However, regarding the importance of earthworm activity in our study site and the comparatively low and sporadic activity of beetles and termites, we assume that the earthworm species A. khami plays a very important role in favoring water infiltration and then reducing soil erosion in the watershed (Podwojewski et al., 2008).
Conclusions
Properties of the soil macroporosity and galleries made by beetles, termites, and earthworms were studied using X-ray CT, thereby providing evidence of the impact of soil invertebrate biodiversity on soil architecture. A conclusion of this study is that most of the macroporosity in soil can be viewed as a heritage of the activity of many other soil invertebrates, such as ants or endogeic earthworms, which do not leave traces of activity on the soil surface. We confirmed the positive impact, although taxon-specific, of soil fauna on water infiltration (beetles ≥ termites ≥ earthworms), and we confirmed that macroporosity measured by X-ray CT provides an accurate prediction of Ksat (Rachman et al., 2005; Kim et al., 2010; Luo et al., 2010). This finding confirms also the interest in this approach for quantifying the impact of soil fauna on the dynamic of water in soil and highlights the need for a better understanding of the dynamic of these galleries in terms of production and degradation.
Data Availability
The datasets for this manuscript are not publicly available because the datasets generated during and/or analyzed during the current study are available from the corresponding author upon request. Requests to access the datasets should be directed to c291Z3VlaC5jaGVpa0BpcmQuZnI=.
Author Contributions
SC, NB, and PJ conceived and designed the research and analyzed the X-ray CT images, analyzed and interpreted the data, and wrote the manuscript. TM and TD provided access to the laboratory, medical scanner, and the study site. SC, PJ, and NB wrote the manuscript.
Funding
This work was supported by the French Institute for Research for Development (IRD) through a Ph.D. grant awarded to SC from Allocations de Recherche pour une Thèse au Sud (ARTS).
Conflict of Interest Statement
The authors declare that the research was conducted in the absence of any commercial or financial relationships that could be construed as a potential conflict of interest.
Acknowledgments
We acknowledge the staff at the Soils and Fertilizers Research Institute (SFRI, Hanoi) in Vietnam who maintained the M-tropics long-term observatory. Computational resources of this study were made available through the support of Dr. Yvan Capowiez from the department Environnement Méditerranéen et Modélisation des Agro-Hydrosystèmes (EMMAH) at French National Institute for Agricultural Research (INRA). We are grateful to Dr. Nguyen Ngoc Hie, vice director of Bach Maï Hospital for providing us with access to the medical scanner. We also thank Lucian Elles and Hien for their help during fieldwork.
Supplementary Material
The Supplementary Material for this article can be found online at: https://www.frontiersin.org/articles/10.3389/fenvs.2019.00031/full#supplementary-material
Supplementary File 1. Three-dimensional images of soil macropores (>20 mm3) obtained below treatments DB: “dung beetles,” EC: “earthworm casts,” and TS: “termite sheetings” and in Ctrl: control.
References
Anderson, J. M., and Ingram, J. S. I. (1993). Tropical soil biology and fertility: a handbook of methods. J. Ecol. 78:547. doi: 10.2307/2261129
Anderson, S. H., Peyton, R. L., and Gantzer, C. J. (1990). Evaluation of constructed and natural soil macropores using X-ray computed tomography. Geoderma 46, 13–29. doi: 10.1016/0016-7061(90)90004-S
Andriuzzi, W. S., Pulleman, M. M., Schmidt, O., Faber, J. H., and Brussaard, L. (2015). Anecic earthworms (Lumbricus terrestris) alleviate negative effects of extreme rainfall events on soil and plants in field mesocosms. Plant Soil 397, 103–113. doi: 10.1007/s11104-015-2604-4
Bastardie, F., Capowiez, Y., and Cluzeau, D. (2005). 3D characterisation of earthworm burrow systems in natural soil cores collected from a 12-year-old pasture. Appl. Soil Ecol. 30, 34–46. doi: 10.1016/j.apsoil.2005.01.001
Bastardie, F., Capowiez, Y., de Dreuzy, J.-R., and Cluzeau, D. (2003). X-ray tomographic and hydraulic characterization of burrowing by three earthworm species in repacked soil cores. Appl. Soil Ecol. 24, 3–16. doi: 10.1016/S0929-1393(03)00071-4
Beven, K., and Germann, P. (1982). Macropores and water flow in soils. Water Resources Res. 18, 1311–1325. doi: 10.1029/WR018i005p01311
Bottinelli, N., Angers, D. A., Hallaire, V., Michot, D., Le Guillou, C., Cluzeau, D., et al. (2017). Tillage and fertilization practices affect soil aggregate stability in a Humic Cambisol of Northwest France. Soil Tillage Res. 170, 14–17. doi: 10.1016/j.still.2017.02.008
Bottinelli, N., Jouquet, P., Capowiez, Y., Podwojewski, P., Grimaldi, M., and Peng, X. (2015). Why is the influence of soil macrofauna on soil structure only considered by soil ecologists? Soil Tillage Res. 146, 118–124. doi: 10.1016/j.still.2014.01.007
Bullock, P. (ed.). (1985). Handbook for Soil Thin Section Description. Albrighton: Waine Research Publications.
Capowiez, Y., Bottinelli, N., Sammartino, S., Michel, E., and Jouquet, P. (2015). Morphological and functional characterisation of the burrow systems of six earthworm species (Lumbricidae). Biol. Fertil. Soils 51, 869–877. doi: 10.1007/s00374-015-1036-x
Capowiez, Y., Monestiez, P., and Belzunces, L. (2001). Burrow systems made by Aporrectodea nocturna and Allolobophora chlorotica in artificial cores: morphological differences and effects of interspecific interactions. Appl. Soil Ecol. 16, 109–120. doi: 10.1016/S0929-1393(00)00110-4
Capowiez, Y., Sammartino, S., and Michel, E. (2011). Using X-ray tomography to quantify earthworm bioturbation non-destructively in repacked soil cores. Geoderma 162, 124–131. doi: 10.1016/j.geoderma.2011.01.011
Cheik, S., Bottinelli, N., Soudan, B., Harit, A., Chaudhary, E., Sukumar, R., et al. (2018a). Effects of termite foraging activity on topsoil physical properties and water infiltration in Vertisol. Appl. Soil Ecol. 133, 132–137. doi: 10.1016/j.apsoil.2018.10.001
Cheik, S., Bottinelli, N., Sukumar, R., and Jouquet, P. (2018b). Fungus-growing termite foraging activity increases water infiltration but only slightly and temporally impacts soil physical properties in southern Indian woodlands. Eur. J. Soil Biol. 89, 20–24. doi: 10.1016/j.ejsobi.2018.09.001
Filser, J., Faber, J. H., Tiunov, A. V., Brussaard, L., Frouz, J., De Deyn, G., et al. (2016). Soil fauna: key to new carbon models. Soil 2, 565–582. doi: 10.5194/soil-2-565-2016
Fischer, C., Roscher, C., Jensen, B., Eisenhauer, N., Baade, J., Attinger, S., et al. (2014). How do earthworms, soil texture and plant composition affect infiltration along an experimental plant diversity gradient in grassland? PLoS ONE 9:e98987. doi: 10.1371/journal.pone.0098987
Hong, H. N., Rumpel, C., Henry des Tureaux, T., Bardoux, G., Billou, D., Tran Duc, T., et al. (2011). How do earthworms influence organic matter quantity and quality in tropical soils? Soil Biol. Biochem. 43, 223–230. doi: 10.1016/j.soilbio.2010.09.033
Jarvis, N., Larsbo, M., and Koestel, J. (2017). Connectivity and percolation of structural pore networks in a cultivated silt loam soil quantified by X-ray tomography. Geoderma 287, 71–79. doi: 10.1016/j.geoderma.2016.06.026
Jarvis, N. J. (2007). A review of non-equilibrium water flow and solute transport in soil macropores: principles, controlling factors and consequences for water quality. Eur. J. Soil Sci. 58, 523–546. doi: 10.1111/j.1365-2389.2007.00915.x
Jégou, D., Cluzeau, D., Wolf, H. J., Gandon, Y., and Tréhen, P. (1997). Assessment of the burrow system of Lumbricus terrestris, Aporrectodea giardi, and Aporrectodea caliginosa using X-ray computed tomography. Biol. Fertil. Soils 26, 116–121. doi: 10.1007/s003740050353
Jones, C. G., Lawton, J. H., and Shachak, M. (1994). Organisms as ecosystem engineers. Oikos 69, 373–386. doi: 10.1007/978-1-4612-4018-1_14
Jones, C. G., Lawton, J. H., and Shachak, M. (1997). Positive and negative effects of organisms as physical ecosystem engineers. Ecology 78, 1946–1957. doi: 10.1890/0012-9658(1997)078[1946:PANEOO]2.0.CO;2
Joschko, M., Graff, O., Müller, P. C., Kotzke, K., Lindner, P., Pretschner, D. P., et al. (1991). A non-destructive method for the morphological assessment of earthworm burrow systems in three dimensions by X-ray computed tomography. Biol. Fertil. Soils 11, 88–92. doi: 10.1007/BF00336369
Jouquet, P., Bottinelli, N., Podwojewski, P., Hallaire, V., and Tran Duc, T. (2008a). Chemical and physical properties of earthworm casts as compared to bulk soil under a range of different land-use systems in Vietnam. Geoderma 146, 231–238. doi: 10.1016/j.geoderma.2008.05.030
Jouquet, P., Dauber, J., Lagerlöf, J., Lavelle, P., and Lepage, M. (2006). Soil invertebrates as ecosystem engineers: intended and accidental effects on soil and feedback loops. Appl. Soil Ecol. 32, 153–164. doi: 10.1016/j.apsoil.2005.07.004
Jouquet, P., Janeau, J.-L., Pisano, A., Sy, H. T., Orange, D., Minh, L. T. N., et al. (2012). Influence of earthworms and termites on runoff and erosion in a tropical steep slope fallow in Vietnam: a rainfall simulation experiment. Appl. Soil Ecol. 61, 161–168. doi: 10.1016/j.apsoil.2012.04.004
Jouquet, P., Podwojewski, P., Bottinelli, N., Mathieu, J., Ricoy, M., Orange, D., et al. (2008b). Above-ground earthworm casts affect water runoff and soil erosion in Northern Vietnam. Catena 74, 13–21. doi: 10.1016/j.catena.2007.12.006
Jouquet, P., Zangerle, A., Rumpel, C., Brunet, D., Bottinelli, N., and Tran Duc, T. (2009). Relevance and limitations of biogenic and physicogenic classification: a comparison of approaches for differentiating the origin of soil aggregates. Eur. J. Soil Sci. 60, 1117–1125. doi: 10.1111/j.1365-2389.2009.01168.x
Kaiser, D., Lepage, M., Konaté, S., and Linsenmair, K. E. (2017). Ecosystem services of termites (Blattoidea: Termitoidae) in the traditional soil restoration and cropping system Zaï in northern Burkina Faso (West Africa). Agric. Ecosyst. Environ. 236, 198–211. doi: 10.1016/j.agee.2016.11.023
Kim, H., Anderson, S. H., Motavalli, P. P., and Gantzer, C. J. (2010). Compaction effects on soil macropore geometry and related parameters for an arable field. Geoderma 160, 244–251. doi: 10.1016/j.geoderma.2010.09.030
Kooyman, C., and Onck, R. F. M. (1987). Distribution of termite (Isoptera) species in southwestern Kenya in relation to land use and the morphology of their galleries. Biol. Fertil. Soils 3, 69–73. doi: 10.1007/BF00260581
Langmaack, M., Schrader, S., Rapp-Bernhardt, U., and Kotzke, K. (1999). Quantitative analysis of earthworm burrow systems with respect to biological soil-structure regeneration after soil compaction. Biol. Fertil. Soils 28, 219–229. doi: 10.1007/s003740050486
Larsbo, M., Koestel, J., and Jarvis, N. (2014). Relations between macropore network characteristics and the degree of preferential solute transport. Hydrol. Earth Syst. Sci. 18, 5255–5269. doi: 10.5194/hess-18-5255-2014
Lassabatère, L., Angulo-Jaramillo, R., Soria Ugalde, J. M., Cuenca, R., Braud, I., and Haverkamp, R. (2006). Beerkan estimation of soil transfer parameters through infiltration experiments—BEST. Soil Sci. Soc. Am. J. 70:521. doi: 10.2136/sssaj2005.0026
Lavelle, P., Bignell, D., and Lepage, M. (1997). Soil function in a changing world: the role of invertebrate ecosystem engineers. Eur. J. Soil Sci. 33, 159–193.
Léonard, J., Perrier, E., and de Marsily, G. (2001). A model for simulating the influence of a spatial distribution of large circular macropores on surface runoff. Water Resources Res. 37, 3217–3225. doi: 10.1029/2001WR000337
Léonard, J., Perrier, E., and Rajot, J. L. (2004). Biological macropores effect on runoff and infiltration: a combined experimental and modelling approach. Agric. Ecosyst. Environ. 104, 277–285. doi: 10.1016/j.agee.2003.11.015
Léonard, J., and Rajot, J. L. (2001). Influence of termites on runoff and infiltration: quantification and analysis. Geoderma 104, 17–40. doi: 10.1016/S0016-7061(01)00054-4
Luo, L., Lin, H., and Li, S. (2010). Quantification of 3-D soil macropore networks in different soil types and land uses using computed tomography. J. Hydrol. 393, 53–64. doi: 10.1016/j.jhydrol.2010.03.031
Mando, A., Brussaard, L., and Stroosnijder, L. (1999). Termite- and mulch-mediated rehabilitation of vegetation on crusted soil in West Africa. Restoration Ecol. 7, 33–41. doi: 10.1046/j.1526-100X.1999.07104.x
Mando, A., Stroosnijder, L., and Brussaar, L. (1996). Effects of termites on infiltration into crusted soil. Geoderma 74, 107–113. doi: 10.1016/S0016-7061(96)00058-4
Mevik, B., and Wehrens, R. (2007). The pls Package: Principal Component and Partial Least Squares Regression in R. Journal of Statistical Software. doi: 10.18637/jss.v018.i02
Mikheyev, A. S., and Tschinkel, W. R. (2004). Nest architecture of the ant Formica pallidefulva : structure, costs and rules of excavation. Insectes Sociaux 51, 30–36. doi: 10.1007/s00040-003-0703-3
Mikus, P., and Uchman, A. (2013). Beetle burrows with a terminal chamber: a contribution to the knowledge of the trace fossil Macanopsis in continental sediments. Palaios 28, 403–413. doi: 10.2110/palo.2012.p12-129
Naveed, M., Schjønning, P., Keller, T., de Jonge, L. W., Moldrup, P., and Lamandé, M. (2016). Quantifying vertical stress transmission and compaction-induced soil structure using sensor mat and X-ray computed tomography. Soil Tillage Res. 158, 110–122. doi: 10.1016/j.still.2015.12.006
Oades, J., and Waters, A. (1991). Aggregate hierarchy in soils. Aust. J. Soil Res. 29:815. doi: 10.1071/SR9910815
Otsu, N. (1979). A threshold selection method from gray-level histograms. IEEE Trans. Syst. Man Cybernet. 9, 62–66. doi: 10.1109/TSMC.1979.4310076
Perret, J., Prasher, S. O., Kantzas, A., and Langford, C. (1999). Three-dimensional quantification of macropore networks in undisturbed soil cores. Soil Sci. Soc. Am. J. 63:1530. doi: 10.2136/sssaj1999.6361530x
Pierret, A., Capowiez, Y., Belzunces, L., and Moran, C. (2002). 3D reconstruction and quantification of macropores using X-ray computed tomography and image analysis. Geoderma 106, 247–271. doi: 10.1016/S0016-7061(01)00127-6
Podwojewski, P., Orange, D., Jouquet, P., Valentin, C., Nguyen, V. T., Janeau, J. L., et al. (2008). Land-use impacts on surface runoff and soil detachment within agricultural sloping lands in Northern Vietnam. Catena 74, 109–118. doi: 10.1016/j.catena.2008.03.013
Rabot, E., Wiesmeier, M., Schlüter, S., and Vogel, H.-J. (2018). Soil structure as an indicator of soil functions: a review. Geoderma 314, 122–137. doi: 10.1016/j.geoderma.2017.11.009
Rachman, A., Anderson, S. H., and Gantzer, C. J. (2005). Computed-tomographic measurement of soil macroporosity parameters as affected by stiff-stemmed grass hedges. Soil Sci. Soc. Am. J. 69:1609. doi: 10.2136/sssaj2004.0312
Renard, P., and Allard, D. (2013). Connectivity metrics for subsurface flow and transport. Adv. Water Resources 51, 168–196. doi: 10.1016/j.advwatres.2011.12.001
Schneider, C. A., Rasband, W. S., and Eliceiri, K. W. (2012). NIH Image to ImageJ: 25 years of image analysis. Nat. Methods 9, 671–675. doi: 10.1038/nmeth.2089
Six, J., Bossuyt, H., Degryze, S., and Denef, K. (2004). A history of research on the link between (micro)aggregates, soil biota, and soil organic matter dynamics. Soil Tillage Res. 79, 7–31. doi: 10.1016/j.still.2004.03.008
Slade, E. M., Mann, D. J., Villanueva, J. F., and Lewis, O. T. (2007). Experimental evidence for the effects of dung beetle functional group richness and composition on ecosystem function in a tropical forest. J. Anim. Ecol. 76, 1094–1104. doi: 10.1111/j.1365-2656.2007.01296.x
Soil Survey Staff (2014). Keys to Soil Taxonomy, 12th Edn. Washington, DC: USDA-Natural Resources Conservation Service.
Tisdall, J. M., and Oades, J. M. (1982). Organic matter and water-stable aggregates in soils. J. Soil Sci. 33, 141–163. doi: 10.1111/j.1365-2389.1982.tb01755.x
van Schaik, L., Palm, J., Klaus, J., Zehe, E., and Schröder, B. (2014). Linking spatial earthworm distribution to macropore numbers and hydrological effectiveness: linking spatial earthworm distribution to macropore numbers and hydrology. Ecohydrology 7, 401–408. doi: 10.1002/eco.1358
Vogel, H. J. (2000). A numerical experiment on pore size, pore connectivity, water retention, permeability, and solute transport using network models: pore structure and hydraulic properties. Eur. J. Soil Sci. 51, 99–105. doi: 10.1046/j.1365-2389.2000.00275.x
WRB (1998). World Reference Base for Soil Resources. World soil resources Reports, Vol. 84. Rome: FAO.
Keywords: soil, X-ray computed tomography, soil macrofauna, galleries networks, saturated hydraulic conductivity
Citation: Cheik S, Bottinelli N, Minh TT, Doan TT and Jouquet P (2019) Quantification of Three Dimensional Characteristics of Macrofauna Macropores and Their Effects on Soil Hydraulic Conductivity in Northern Vietnam. Front. Environ. Sci. 7:31. doi: 10.3389/fenvs.2019.00031
Received: 11 December 2018; Accepted: 20 February 2019;
Published: 19 March 2019.
Edited by:
Philippe C. Baveye, AgroParisTech Institut des Sciences et Industries du Vivant et de l'Environnement, FranceReviewed by:
Andrey S. Zaitsev, University of Giessen, GermanyFernando San José Martínez, Polytechnic University of Madrid, Spain
Copyright © 2019 Cheik, Bottinelli, Minh, Doan and Jouquet. This is an open-access article distributed under the terms of the Creative Commons Attribution License (CC BY). The use, distribution or reproduction in other forums is permitted, provided the original author(s) and the copyright owner(s) are credited and that the original publication in this journal is cited, in accordance with accepted academic practice. No use, distribution or reproduction is permitted which does not comply with these terms.
*Correspondence: Sougueh Cheik, c291Z3VlaC5jaGVpa0BpcmQuZnI=