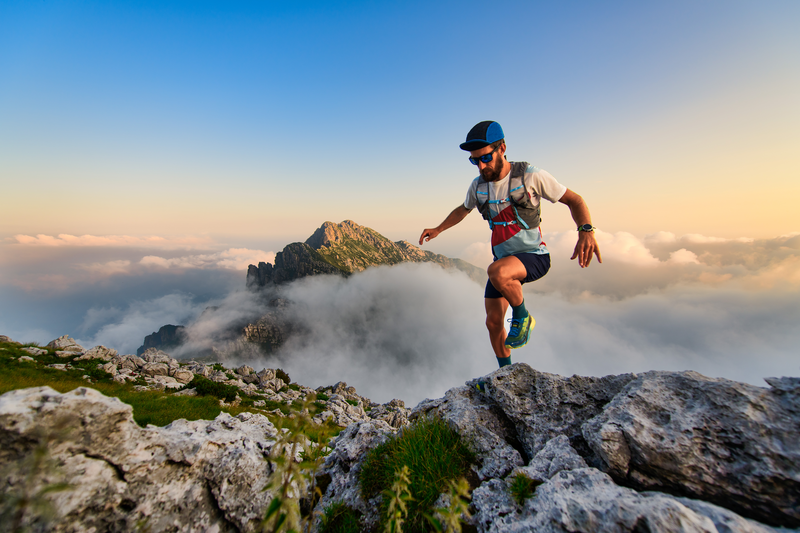
94% of researchers rate our articles as excellent or good
Learn more about the work of our research integrity team to safeguard the quality of each article we publish.
Find out more
DATA REPORT article
Front. Environ. Sci. , 13 November 2018
Sec. Soil Processes
Volume 6 - 2018 | https://doi.org/10.3389/fenvs.2018.00136
Soil is the foundation of ecosystem functioning in urban green spaces and provides key ecosystem services for a livable city (Zhu et al., 2018). Urban soils are a mixture of natural soil-forming factors and anthropogenic activities (Shuster and Dadio, 2018). Therefore, they require an adapted set of indicators for a soil quality assessment. Soil quality is one of the three constituents of environmental quality, along with water and air quality (Andrews et al., 2002). It is generally referred to as the capacity of a soil to function within ecosystems and land-use boundaries to sustain biological productivity, maintain environmental quality, and promote plant and animal health, including human health (Doran and Parkin, 1994). Here, we present a comprehensive dataset of 37 soil quality indicators measured at 170 plots in 85 urban gardens within the city of Zurich, Switzerland. They represent all major inherent and dynamic soil properties for a soil quality assessment (Bünemann et al., 2018), including eight physical, nine chemical and eleven biological soil quality indicators, plus nine soil metal properties (As, Ba, Co, Cu, Ni, Pb, Sb, V, Zn). Soil function decomposition was assessed by the tea bag index method (Keuskamp et al., 2013). Results regarding the influence of garden management, local and landscape effects on the distribution of soil quality indicators can be found in Tresch et al. (2018). This dataset is useful for future studies on urban soil quality, ecosystem services or for modeling purposes such as carbon dynamics or greenhouse gas inventory models in cities.
The dataset of 37 soil quality indicators (Figure 1) was measured at 170 plots in 85 urban gardens in the city of Zurich, Switzerland, comprising 42 allotment and 43 home gardens (Figure S1). These two garden types are the most frequent in Switzerland, but also comparatively worldwide (Lin et al., 2017). Allotment gardens represent a plot of land rented by gardeners, usually located in urban or semi-urban areas, while home gardens are often situated around private houses. The study design is part of the interdisciplinary project BetterGardens (www.bettergardens.ch) that focuses on soil quality, biodiversity, ecosystem services and human wellbeing in urban gardens in Switzerland. Two plots within each garden were selected according to garden habitat and management practices such as lawn, vegetables or flower and berry beds. Five soil samples (0–20 cm depth, 3 cm wide soil auger Eijkelkamp, NL) were collected randomly within an area of 2 × 2 m on the 170 plots, prior to the first soil gardening practices in March 2015. Soil samples were pooled for each plot, gently air dried, homogenized and sieved at 2 mm then stored at 4°C. Subsamples designated for DNA analysis were frozen and stored at -80°C until analysis. Subsamples for chemical analysis were air dried at 20°C and stored under cool and dry conditions. For physical soil quality indicators, undisturbed samples were collected with three soil cores (5 cm diameter and depth, Eijkelkamp, NL) at a depth of 10–15 cm on each plot. Soil quality analyses were performed in accordance with Swiss standard methods for soil characterization (Agroscope, 2012).
Figure 1. Concept of assessing urban soil quality through the measurements of soil quality indicators related to soil functions. Details about soil quality indicators measured in urban gardens of Zurich, Switzerland can be found in Table S4.
Soil texture was measured by a combined sieving and sedimentation technique (Schinner et al., 1996). Soil water holding capacity (WHC) was determined by a cylinder method on a sand bath (Schinner et al., 1996). Soil aggregate stability (SA) was determined from aggregates in the 1–2 mm fraction by wet sieving for 5 min on a multi-sieve device (42 cycles min-1) and subtracting the sand fraction (Schinner et al., 1996). Soil bulk density (BD) and pore space volume (PV) were determined with the undisturbed soil cores following Swiss reference methods (Agroscope, 2012). Penetration resistance was measured with a Penetrologger (cone type: 100 mm2, penetration speed: 0.002 ms-1; Eijkelkamp, NL), recording the penetration resistance every 1 cm down to a soil depth of 80 cm. Ten replicated measurements were taken and mean values from 0 to 20 cm soil depth were calculated for the penetration resistance.
Soil pH and electrical conductivity (EC) were measured in a soil suspension with deionized water (1:2.5 w/v). Soil nutrient contents (P, K, Mg, Cu, B, Mn, Fe) were measured at an external certified laboratory with ammonium acetate-EDTA.
Total organic carbon (TOC) and nitrogen (TON) were determined by a CHN analyzer (Thermo Scientific Flash EA 1112, NL) after removing carbonates with 2 M HCl. Dissolved organic carbon (DOC) and nitrogen (DON) as well as mineralized N (Nmin), were measured in an extract with 0.01 M CaCl2 (1:4 w/v) (Krauss et al., 2017). Soil microbial biomass carbon (Cmic) and nitrogen (Nmic) contents were determined by chloroform-fumigation-extraction (CFE) (Fliessbach et al., 2007). Soil basal respiration and C mineralization (Cmin) were assessed by measuring CO2 evolution in defined intervals from soil samples using a gas chromatograph (TCD detector; 7890A, Agilent Technologies, USA) as described in Tresch et al. (2018). Basal respiration rates were recorded after 1 week and Cmin after 4 weeks of soil incubation at 20°C. Community level physiological profiles (CLPP) were assessed with the MicroResp technique (Campbell et al., 2003) using 18 different carbon substrates and water (see Table S2). The carbon substrates represent a cross-section of root exudates, ranging from sugars to amino acids, carboxylic acids, amino sugars and hemicellulose typically occuring in soil (Campbell et al., 1997). Quantitative real-time PCR (qPCR) was performed to estimate the bacterial (16S rRNA; BactQuant assay, Liu et al., 2012) and fungal (18S rRNA; FR1/FF390 primer, Vainio and Hantula, 2000 validated by Chemidlin Prévost-Bouré et al., 2011) gene copy numbers (see Table S3).
Metals contents were measured as total element concentrations analyzed in dried and ball milled soil samples pressed with wax to tablets on an X-Ray Fluorescence device (XRF, X-lab 2000, SPECTRO Analytical Instruments, DE). Although the measurement corresponds to standard soil metal concentrations (Horta et al., 2015), metal concentrations are probably overestimated by 10–20% (Christl et al., 2004) compared to the standard method (i.e., aqua regia). Cu.m refers to copper metal values.
Belowground decomposition was measured by using standardized commercial tea bags following the tea bag index method (Keuskamp et al., 2013). Green tea bags were used as a rapid and rooibos tea as a slowly decomposable material. In total, four tea bags per tea type (170 plots × 2 tea types × 4 replicates = 1,360 tea bags) were burried at a depth of 8 cm for 90 days (mid-October until mid-January 2016). Decomposition, expressed as percentage change in mass before and after decomposition, was calculated after drying at 60°C and subtracting soil particles subsequent to incineration of the tea bags.
All statistics were performed using R software version 3.4.2 (R Core Team, 2017). Descriptive statistics (dplyr) and visualization (ggplot2) were obtained with the data manipulation package tidyverse (Wickham and Grolemund, 2016) and the spatial plot (Figure S1) with the package ggmap (Kahle and Wickham, 2013). Example R codes (R project files) including the raw data (csv file) are provided in the Supplementary Material.
The dataset comprises a collection of soil quality indicators for urban garden soils including standardized tea bags representing the soil function decomposition. We measured eight physical, nine chemical, eleven biological soil quality indicators as well as nine metal values (Figure 1), that are necessary for a comprehensive soil quality analysis in an urban context. The dataset was sampled at a scale of an entire representative medium-sized European city (Figure S1). The data is split according to the two most common urban garden types: allotment and home gardens (Table S1). Furthermore, sample plots were assigned one of three garden habitat types: vegetable beds (i.e., annual vegetable plants), flower beds and berry cultivations (i.e., perennial flowers, roses, and berry shrubs), and lawn (i.e., meadows and turf). Descriptive statistics are given in Tables S4–S6. A graphical representation of percentage deviations from the overall mean value split by garden and habitat type is given in Figure 2. In summary, this dataset provides information about a city-wide soil quality assessment of urban gardens. This data can be used for comparing soil properties among different cities or land use types. Moreover, our study may help to analyze the effect of garden management or urbanization on soil quality (see Tresch et al., 2018) or provide data for modeling of carbon dynamics in urban soils or other soil based ecosystem services.
Figure 2. Soil quality indicators per garden type (left) and habitat type (right) consisting of (A) eight physical indicators, (B) nine chemical indicators, (C) eleven biological indicators, (D) nine metal measurements. Data points represent the relative deviation [%] from the grand mean (Table S4) that is set to 100%. Standard error bars are shown with lines.
AF, MM, R-CL, PM, DF, and ST conceived and designed the research. ST, DF, and AZ performed the field work. Lab work was done by ST, AK, AM, and BS. ST analyzed the data. All authors contributed to the writing of the manuscript.
This work was part of the interdisciplinary project BetterGardens (www.bettergardens.ch), funded by the Swiss National Science Foundation in the frame of the Sinergia program (CRSII1_154416).
The authors declare that the research was conducted in the absence of any commercial or financial relationships that could be construed as a potential conflict of interest.
We thank the project coordinator Dr. Robert Home for his integrative work. We are grateful to Prof. Dr. Rainer Schulin and Björn Studer for the XRF-analysis at ETH and Roger Köchlin for the soil texture analysis at WSL. We acknowledge in particular the willingness and interest of the 85 participating gardeners to give us access to their gardens and to have us dig and sample soils on their property.
The Supplementary Material for this article can be found online at: http://journal.frontiersin.org/article/10.3389/fenvs.2018.00136/full#supplementary-material
Agroscope (2012). Referenzmethoden der Forschungsanstalten Agroscope: Band 1, Bodenuntersuchungen zur Düngeberatung. Zurich: Forschungsanstalt Agroscope Reckenholz-Tänikon (ART) and Changins-Wädenswil (ACW).
Andrews, S. S., Karlen, D. L., and Mitchell, J. P. (2002). A comparison of soil quality indexing methods for vegetable production systems in Northern California. Agricult. Ecosyst. Environ. 90, 25–45. doi: 10.1016/S0167-8809(01)00174-8
Bünemann, E. K., Bongiorno, G., Bai, Z., Creamer, R. E., De Deyn, G., de Goede, R., et al. (2018). Soil quality A critical review. Soil Biol. Biochem. 120, 105–125. doi: 10.1016/j.soilbio.2018.01.030
Campbell, C., Grayston, S., and Hirst, D. (1997). Use of rhizosphere carbon sources in sole carbon source tests to discriminate soil microbial communities. J. Microbiol. Methods 30, 33–41. doi: 10.1016/S0167-7012(97)00041-9
Campbell, C. D., Chapman, S. J., Cameron, C. M., Davidson, M. S., and Potts, J. M. (2003). A rapid microtiter plate method to measure carbon dioxide evolved from carbon substrate amendments so as to determine the physiological profiles of soil microbial communities by using whole soil. Appl. Environ. Microbiol. 69, 3593–3599. doi: 10.1128/AEM.69.6.3593-3599.2003
Chemidlin Prévost-Bouré, N., Christen, R., Dequiedt, S., Mougel, C., Lelièvre, M., Jolivet, C., et al. (2011). Validation and application of a PCR primer set to quantify fungal communities in the soil environment by real-time quantitative PCR. PLoS ONE 6:e24166. doi: 10.1371/journal.pone.0024166
Christl, I., Gulz, P. A., Kretzschmar, R., and Schulin, H. R. (2004). Umgang mit Bodenbelastungen in Familiengärten der Stadt Zürich. Technical report, ETH Zürich.
Doran, J. W., and Parkin, T. B. (1994). “Dening and assessing soil quality,” in Dening Soil Quality for a Sustainable Environment, Vol. 35, eds J. Doran, D. C. Coleman, D. Bezdicek, and B. Stewart (Madison, WI: Soil Science Society of America), 3–21.
Fliessbach, A., Oberholzer, H.-R., Gunst, L., and Mader, P. (2007). Soil organic matter and biological soil quality indicators after 21 years of organic and conventional farming. Agricult. Ecosyst. Environ. 118, 273–284. doi: 10.1016/j.agee.2006.05.022
Horta, A., Malone, B., Stockmann, U., Minasny, B., Bishop, T., McBratney, A., et al. (2015). Potential of integrated field spectroscopy and spatial analysis for enhanced assessment of soil contamination: a prospective review. Geoderma 241–242, 180–209. doi: 10.1016/j.geoderma.2014.11.024
Keuskamp, J. A., Dingemans, B. J. J., Lehtinen, T., Sarneel, J. M., and Hefting, M. M. (2013). Tea Bag Index: a novel approach to collect uniform decomposition data across ecosystems. Methods Ecol. Evol. 4, 1070–1075. doi: 10.1111/2041-210X.12097
Krauss, M., Krause, H.-M., Spangler, S., Kandeler, E., Behrens, S., Kappler, A., et al. (2017). Tillage system affects fertilizer-induced nitrous oxide emissions. Biol. Fertil. Soils 53, 49–59. doi: 10.1007/s00374-016-1152-2
Lin, B. B., Philpott, S. M., Jha, S., and Liere, H. (2017). “Urban agriculture as a productive green infrastructure for environmental and social well-being,” in Greening Cities Forms and Functions (Singapore: Springer), 155–179. doi: 10.1007/978-981-10-4113-6_8
Liu, C. M., Aziz, M., Kachur, S., Hsueh, P.-R., Huang, Y.-T., Keim, P., et al. (2012). BactQuant: an enhanced broad-coverage bacterial quantitative real-time PCR assay. BMC Microbiol. 12:56. doi: 10.1186/1471-2180-12-56
Schinner, F., Öhlinger, R., Kandeler, E., and Margesin, R. (1996). Methods in Soil Biology. Berlin; Heidelberg: Springer.
Shuster, W. D., and Dadio, S. (2018). “An applied hydropedological perspective on the rendering of ecosystem services from urban soils,” in Urban Soils, 1st Edn., eds B. A. Stewart and R. Lal (Boca Raton, FL: CRC Press), 261–274.
Tresch, S., Moretti, M., Le Bayon, R.-C., Mäder, P., Zanetta, A., Frey, D., et al. (2018). A Gardener's influence on urban soil quality. Front. Environ. Sci. 6:25. doi: 10.3389/fenvs.2018.00025
Vainio, E. J., and Hantula, J. (2000). Direct analysis of wood-inhabiting fungi using denaturing gradient gel electrophoresis of amplified ribosomal DNA. Mycol. Res. 104, 927–936. doi: 10.1017/S0953756200002471
Wickham, H., and Grolemund, G. (2016). R for Data Science: Import, Tidy, Transform, Visualize, and Model Data. Sebastopol, CA: O'Reilly Media, Inc.
Keywords: urban soil quality assessment, soil quality indicators, heavy metal contents in urban soils, urbanization, urban gardening, allotment gardens, home gardens, private gardens
Citation: Tresch S, Moretti M, Le Bayon R-C, Mäder P, Zanetta A, Frey D, Stehle B, Kuhn A, Munyangabe A and Fliessbach A (2018) Urban Soil Quality Assessment—A Comprehensive Case Study Dataset of Urban Garden Soils. Front. Environ. Sci. 6:136. doi: 10.3389/fenvs.2018.00136
Received: 06 August 2018; Accepted: 24 October 2018;
Published: 13 November 2018.
Edited by:
Dionisios Gasparatos, Aristotle University of Thessaloniki, GreeceReviewed by:
Andrey S. Zaitsev, Justus Liebig Universität Gießen, GermanyCopyright © 2018 Tresch, Moretti, Le Bayon, Mäder, Zanetta, Frey, Stehle, Kuhn, Munyangabe and Fliessbach. This is an open-access article distributed under the terms of the Creative Commons Attribution License (CC BY). The use, distribution or reproduction in other forums is permitted, provided the original author(s) and the copyright owner(s) are credited and that the original publication in this journal is cited, in accordance with accepted academic practice. No use, distribution or reproduction is permitted which does not comply with these terms.
*Correspondence: Simon Tresch, c2ltb24udHJlc2NoQGZpYmwub3Jn
Disclaimer: All claims expressed in this article are solely those of the authors and do not necessarily represent those of their affiliated organizations, or those of the publisher, the editors and the reviewers. Any product that may be evaluated in this article or claim that may be made by its manufacturer is not guaranteed or endorsed by the publisher.
Research integrity at Frontiers
Learn more about the work of our research integrity team to safeguard the quality of each article we publish.