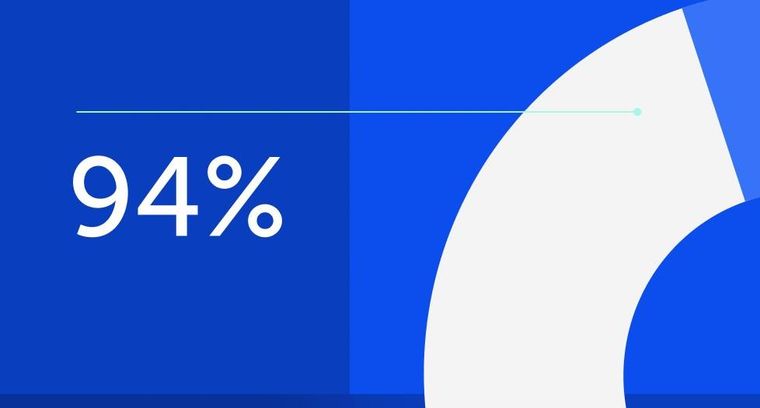
94% of researchers rate our articles as excellent or good
Learn more about the work of our research integrity team to safeguard the quality of each article we publish.
Find out more
GENERAL COMMENTARY article
Front. Environ. Sci., 07 November 2017
Sec. Microbiological Chemistry and Geomicrobiology
Volume 5 - 2017 | https://doi.org/10.3389/fenvs.2017.00074
This article is a commentary on:
Commentary: Evaluating the Role of Seagrass in Cenozoic CO2 Variations
A commentary on
Commentary: Evaluating the Role of Seagrass in Cenozoic CO2 Variations
by Macreadie, P. I., Serrano, O., Duarte, C. M., Beardall, J., and Maher, D. (2017). Front. Environ. Sci. 5:55. doi: 10.3389/fenvs.2017.00055
We would like to acknowledge Macreadie et al. (2017) for their interest in clarifying some key points on the processes involved in the balance of CO2 exerted by the seagrass ecosystems during the Cenozoic. However, their criticisms seem to be based on some misunderstandings regarding the biocalcification processes. So we take this opportunity to make clear some concepts on this topic.
Macreadie et al. (2017) criticize Brandano et al. (2016) when considering the epiphytic carbonate production to result in net CO2 sequestration, in addition to the CO2 sequestration by the growth of the seagrasses. In fact, they assert “the authors have not accounted for the fact that calcification increases pCO2 (by depleting and therefore reducing alkalinity), which facilitates the return of CO2 to the atmosphere. Specifically, for every mole of CaCO3 precipitated as carbonate, the process also consumes 2 moles of and releases 1 mole of CO2”.
This criticism is clearly questionable and contradicts themselves (Macreadie et al., 2014) when considered the C to be stored by the seagrass system not only in the organic tissues (leaves, roots and rhizomes) but also in the inorganic skeletons (carbonates). In fact they wrote “…carbonates – even though they are inorganic, and carbonate formation releases net CO2 –should be included as part of the C stock because they consist of bound C that is locked away, and might otherwise end up as CO2 in the atmosphere.”
The seagrass ecosystem uses the CO2 in two main processes: in the photosynthetic production of the organic tissues –leafs, roots and steams, as well as the organic parts of all associated organisms that live in this ecosystem, and in the carbonate precipitation forming skeletons of the epiphytic biotas. In both processes, the DIC (CO2 and ) is used. The organic matter is only a temporary sink of the CO2 because after death and decay the CO2 returns to the atmosphere.
It is well-known that photosynthetic prokaryotes since the Archean, prokaryotes and eukaryotes during the Proterozoic, and more evolved forms of photosynthetic life also during the Phanerozoic have been using the CO2 derived from the Earth Mantle outgassing. Nevertheless, following the Great Oxidation Event, around 2000 Ma ago, the oxygen started to accumulate in the atmosphere and dissolved in the oceans. This reverted the reaction and the organic matter that was not buried (isolated from the oxidizing environments) became oxidized and the carbon returned to the atmosphere in the form of CO2 (1). But, if organic matter is buried, it is isolated from oxidizing processes and becomes a real sink of C, as represented by are the hydrocarbon and coal deposits.
In alkaline waters, bicarbonate is the most abundant DIC species, but it is inaccessible without a source of protons (2). Diffusion from ambient waters can supply protons that are consumed during photosynthesis. But as diffusion is slow, the CO2 becomes depleted, photosynthesis is inhibited and the photosynthetic organisms are bathed in an alkaline microenvironment. By discharging the protons from calcification into their boundary layers (2) and Figure 1, photosynthetic organisms can maintain or even elevate CO2 concentrations despite photosynthetic CO2 uptake (3). This enhances carboxylation efficiencies (McConnaughey and Whelan, 1997; Pomar and Hallock, 2008).
Adding reactions (2) and (3) one obtains a 1:1 ratio of calcification to photosynthesis in which both calcification and organic matter are part of the same system.
Figure 1. “Cis” and “trans” calcification mechanisms. Photosynthetic alcalinization of the water causes cis calcification, while enzyme Ca++ ATPase drives the Ca++/2H+ exchange in the trans calcification (redrawn from McConnaughey and Whelan, 1997). Cis calcification occurs on the same side of the photosynthetic carbon uptake, whereas trans calcification occurs away of the site of carbon uptake.
The CaCO3 accumulates in the lithosphere, being the limestones and dolostones a permanent storage form of CO2. The same applies to the skeletons of calcifying organisms. The only process releasing the CO2 trapped in the carbonate rocks is the recycling of the lithosphere in the subduction zones and the associated volcanism.
When Macreadie et al. (2017) use the reaction (5) to illustrate calcification as a source of CO2, they ignore the stoichiometry of the complete system (6):
As it is obvious, to release one CO2 from carbonate precipitation (5), two CO2 are needed to produce the two required bicarbonates (6). So, the balance of the carbonate precipitation is a net CO2 sink. This is well illustrated in the different calcification processes (Figure 1)
Macreadie et al. (2017) opinion that calcification represents a global CO2 source to the atmosphere, and therefore seagrass meadows could represent a significant net CO2 source is just the vision of one half of the processes, and cannot be sustained when looking at the entire carbon cycle.
As result of these biased misconceptions, the authors enter in continuous contradictions. Their estimations from Mediterranean case histories made them to conclude that calcification in P. oceanica meadows could be responsible for the emission of 0.7 to 4.2 Tg C yr−1 to the atmosphere (Mateo and Serrano, 2012). So why are people promoting to restore and protect P. oceanica meadows, in order to fulfill their key role in carbon cycle and storage by adopting efficient regulation of human pressures such as fishing? Some of the coauthors of the Macreadie et al. (2017) commentary (e.g., Fourqurean et al., 2012) state that the rapid loss of seagrasses resulted in a substantial decrease in C sequestration by seagrass ecosystems of 6 to 24 Tg C yr−1. So the authors of the Macreadie et al. (2017) paper have to take a decision: are seagrasses a sink or a source of CO2? If they conclude seagrasses are a source, then we should review the entire evolution of Earth and Life.
MB, LP, and GM led the paper. All authors contributed to the discussion.
The authors declare that the research was conducted in the absence of any commercial or financial relationships that could be construed as a potential conflict of interest.
Criticisms by reviewer Hildegard Westphal and Associate Editor Cody Sheik greatly improved the manuscript.
Brandano, M., Cuffaro, M., Gaglianone, G., Pettricca, P., Stagno, V., and Mateu-Vicens, G. (2016). Evaluating the role of seagrass in Cenozoic CO2 variations. Front. Environ. Sci. 4:72. doi: 10.3389/fenvs.2016.00072
Fourqurean, J. W., Duarte, C. M., Kennedy, H., Marbà, N., Holmer, M., Mateo, M. A., et al. (2012). Seagrass ecosystems as a globally significant carbon stock. Nature Geosci. 5:505–509. doi: 10.1038/ngeo1477
Macreadie, P. I., Baird, M. E., Trevathan-Tackett, S. M., Larkum, A. W. D., and Ralph, P. J. (2014). Quantifying and modelling the carbon sequestration capacity of seagrass meadows: a critical assessment. Mar. Pollut. Bull. 83, 430–439. doi: 10.1016/j.marpolbul.2013.07.038
Macreadie, P. I., Serrano, O., Duarte, C. M., Beardall, J., and Maher, D. (2017). Commentary: Evaluating the Role of Seagrass in Cenozoic CO2 Variations. Front. Environ. Sci. 5:55. doi: 10.3389/fenvs.2017.00055
Mateo, M. A., and, O., and Serrano (2012). The Carbon Sink Associated to Posidonia oceanica. Switzerland and Málaga: IUCN, Gland.
McConnaughey, T. A., and Whelan, J. F. (1997). Calcification generates protons for nutrient and bicarbonate uptake. Earth Sci. Rev. 42, 95–117.
Keywords: biocalcification, seagrass, CO2, carbonate, biosequestration
Citation: Brandano M, Pomar L, Mateu-Vicens G, Cuffaro M, Gaglianone G, Petricca P and Stagno V (2017) Response: Commentary: Evaluating the Role of Seagrass in Cenozoic CO2 Variations. Front. Environ. Sci. 5:74. doi: 10.3389/fenvs.2017.00074
Received: 01 September 2017; Accepted: 19 October 2017;
Published: 07 November 2017.
Edited by:
Cody Sheik, University of Minnesota Duluth, United StatesReviewed by:
Hildegard Westphal, Leibniz Centre for Tropical Marine Research (LG), GermanyCopyright © 2017 Brandano, Pomar, Mateu-Vicens, Cuffaro, Gaglianone, Petricca and Stagno. This is an open-access article distributed under the terms of the Creative Commons Attribution License (CC BY). The use, distribution or reproduction in other forums is permitted, provided the original author(s) or licensor are credited and that the original publication in this journal is cited, in accordance with accepted academic practice. No use, distribution or reproduction is permitted which does not comply with these terms.
*Correspondence: Marco Brandano, bWFyY28uYnJhbmRhbm9AdW5pcm9tYTEuaXQ=
Disclaimer: All claims expressed in this article are solely those of the authors and do not necessarily represent those of their affiliated organizations, or those of the publisher, the editors and the reviewers. Any product that may be evaluated in this article or claim that may be made by its manufacturer is not guaranteed or endorsed by the publisher.
Research integrity at Frontiers
Learn more about the work of our research integrity team to safeguard the quality of each article we publish.