- Bioresources and Environmental Biotechnology Laboratory, Department of Environmental Studies, University of Delhi, New Delhi, India
Coal fly ash dumps represent contaminated sites that pollute the environment and affect the health of living organisms. Vegetation development at ash dumps is an ecological solution to minimize the environmental threats of ash; however, low content of nutrients, organic matter and moisture pose a challenge for plant growth at the dumps. Bacterial indole acetic acid (IAA) facilitates plant recruitment and growth, more crucially in degraded ecosystems. Bacteria with different levels of IAA determine the plant-bacterial interactions as pathogenesis or symbiosis, therefore, form microbial functional types. Understanding plant-soil feedback and identifying environmental predictors of bacterial IAA producers at ash dump would help in improving biostimulation strategies for vegetation development. Therefore, to evolve a nature-based solution for vegetational restoration of ash dumps, we analyzed the role of geochemical factors, host species and age of dump on the assembly of rhizobacterial IAA functional types of naturally colonizing grasses (Saccharum ravennae and Cynodon dactylon). Analyses showed that the rhizosphere effect on geochemical traits was distinct in the dumps, irrespective of the host plant and age of the dumps. The rhizobacterial communities from the dumps produce relatively high mean IAA levels and harbor a high micro-diversity of IAA producers as compared with the region as a whole (non-rhizosphere or bulk ash). Canonical correspondence analysis showed that the host species and specific nutrients, i.e., NO3-N, PO4-P, Fe, and Na are the significant predictors of bacterial IAA functional types. S. ravennae and C. dactylon provided evidence of driving assembly of different IAA functional types in their rhizosphere via enrichment of NO3-N and PO4-P, respectively. The identification of environmental predictors of rhizobacterial IAA functional types of S. ravennae and C. dactylon has provided basic guidelines to improve the biostimulation strategies to accelerate vegetation restoration at the ash dumps. Both controlled and field experiments involving grass species with supplementation of specific nutrients would be required to develop an effective biostimulation strategy for the on-field application.
Introduction
Fly ash dumps created during solid waste disposal of the thermal power plants are a socioeconomic and environmental load on the society (Asokan et al., 2005). Being light in weight, the fly ash quickly spreads over long distance and pollutes the environment. It has low contents of moisture, organic matter, and biologically available nutrients. In addition, it has a wide array of toxic elements (Theis and Gardner, 1990; Rau et al., 2009). Therefore, open ash dumps pollute the air, soil, and water and cause the spread of respiratory disorders among humans and livestock (Smith et al., 2006; Qadir et al., 2016). Also, the fly ash contains radioactive elements, like uranium and thorium, which poses a radioactive hazard specifically for human settlements surrounding the dumps. The leaching of radioactive elements from fly ash into the soil and water in surrounding areas, results in uptake by crop plants, consequently entering the food chain (Hvistendahl, 2007). More than 780 million tons of fly ash is produced annually, of which only 25% is used for different purposes at the global level (Ahmaruzzaman, 2010). However, the approach to remediate dumps through vegetation development and reduce risks associated with the ash dumps has received little attention (Haynes, 2009; Rau et al., 2009). Most of the restoration programs at the fly ash dumps face bottlenecks of poor knowledge of the relationship between plant-soil feedback and its impact on soil microbial functional diversity (Mohmmed et al., 2001; Bhattacharya et al., 2003; Rau et al., 2009).
Ecological theories provide basic guidelines for practicing restoration (Diaz and Cabido, 2001). Plant–soil feedback, i.e., plant-induced changes in the structure and function of soil communities that further affect the plant growth and establishment, is a key ecological process for effective and sustainable restoration of degraded ecosystems (Sharma et al., 2002; Eviner and Hawkes, 2008; Van der Putten et al., 2013; Inderjit and Cahill, 2015). Since belowground biodiversity governs the aboveground biodiversity, it is essential to understand plant-soil feedback and identify the predictors of microbial functional diversity to develop nature-based site-specific restoration plans (Eviner and Hawkes, 2008; Cadotte et al., 2011; Zhang and Chu, 2013; Xiao et al., 2016; Smith-Ramesh and Reynolds, 2017).
Poor plant recruitment is the major constraint to vegetational restoration at fly ash dumps. Indole acetic acid (IAA) producers constitute an ecologically important microbial functional group, as IAA is the plant growth regulator. IAA helps in seed germination and root growth (root hair and lateral roots) and promotes host plant colonization (Costacurta and Vanderleyden, 1995; Spaepen et al., 2007). IAA is also responsible for regulating several developmental and physiological processes in plants (Liu et al., 2016). Further, the IAA helps the host plant and microbe to adapt to diverse stress conditions (Bianco et al., 2006). The IAA levels decide the net result of plant-bacterial interactions as pathogenesis or symbiosis (Vacheron et al., 2013). The microbes produce different levels of IAA and form different functional types or functional guilds (Wilson, 1999). Microenvironmental conditions rather than the taxonomic composition is responsible for the variation in the level of each functional trait exhibited by microbial communities (Petchey and Gaston, 2002; Loucas et al., 2016).
The interaction between different genes and environment influences the level of microbial IAA (Spaepen et al., 2007). Therefore, the culture-based methods have the edge over the non-culturable methods in identifying bacterial IAA functional types (Ellis et al., 2003; Rau et al., 2009; Sharma M. et al., 2011; Sharma R. S. et al., 2011; Krause et al., 2014). Culture-based methods provide a limited view of microbial diversity but are still preferred for getting the benefits of bacterial culture for the environmental applications like restoration programs (Sipkema et al., 2011). In fact, several studies from contaminated soils showed benefits of culture-based methods over the culture-independent methods (Ellis et al., 2003; Al-Awadhi et al., 2013; Douterelo et al., 2014).
Previously, we have characterized microbial community from fly ash dumps for different functional groups involved in nutrient acquisition (Sharma et al., 2005; Rau et al., 2009). However, to develop an efficient restoration plan and promote plant recruitment at the dump the analyses of plant-soil feedback is a prerequisite. Thus, the objective of the present study was to identify the environmental factors affecting the diversity of IAA producing bacteria as a part of plant-soil feedback process at the ash dumps. Specifically, we analyzed the functional diversity among bacterial IAA producers and its relationship with host plant species, the age of dumps and environmental factors. We hypothesized that functional diversity in IAA producer results from the interactions between the host plants and/or habitat, which constitutes a plant-soil feedback process during natural attenuation process occurring at ash dump. To the best of our knowledge, this is the first study on environmental predictor of the microbial IAA functional types from fly ash environment. Understanding these microbial ecological processes in nature would provide guiding principles for improvement of vegetation restoration strategies of the fly ash dumps.
Methods
Site Description
Badarpur thermal power plant is one of the key electricity providers (~705 MW) for Delhi urban area, which also contributes to air pollution in the city (Rau et al., 2009; Qadir et al., 2016). It is located in the south eastern part of National Capital Territory of Delhi. The Badarpur fly ash dump covers over ~680 ha area, where ~3,500–4,000 metric tons of fly ash is disposed of every month through pipelines as a slurry and subsequently maintained as dry mounds. The ash dumps of different ages exist there and remain barren for years with a few vegetation patches comprised of weedy, invasive plants and very few native wild grasses (Rau et al., 2009).
We selected two fly ash dumps of different age (28°30′20″N−77°18′25″E), a relatively young dump (5-year old) and an old dump (15-year old) for the present study. The young dump was mostly barren except for few perennial grasses (S. ravennae and C. dactylon) and the rare instances of some annual tender herbs. In contrast, the old dump had rather high vegetation cover with diverse species growing there. Perennial grasses, like C. dactylon, Panicum sp., S. ravennae, and S. spontaneum were among the most dominating species at the old dump; however, a few herbs (Boerhaavia diffusa and Laggera aurita), shrubs (Abutilon indicum, Calotropis procera, and Ipomea carnea), trees (Prosopsis juliflora and Tamarix aphylla) and weeds (Parthenium hysterophorus and Xanthium strumarium) were also present. Besides this, the pressure of anthropogenic activities like cattle rearing by rural people of the surrounding villages was found at the old dump.
Also, an experimental garden (28°41′23.4″N–77°12′41.7″E) was included in the study to compare the fly ash dumps (stressed manmade site) with semi-natural soil (unstressed man managed site). In contrast with fly ash, the garden soil lacks most of the abiotic stresses (low levels of nutrients, moisture, organic C; and high levels of different heavy metals). Therefore, garden, an unstressed site is included as a control. Experimental garden was characterized by different plant species, including grasses (Cynodon dactylon, Echinochloa, Panicum, Paspalum, Vetivaria, etc.), herbaceous and shrubby legumes (Crotalaria, Sesbania spp., Leucaena leucocephala, etc.), and other shrubby (Adhathoda vasaca, Calotropis procera, Datura innoxa, Jatropha curaera, Withania somnifera, etc.) and tree species (Cassia spp., Derris indica, Ficus spp., Leucaena leucocephala, Mimusops elengi, Polyalthia, Putranjiva, etc.). The soil in the garden was sandy loam type characterized by the frequent addition of fertilizers, farmyard manure, and periodic irrigation. There were signs of wood burning along with the growth of alien plant species (Bidens biternata, Cassia tora, Celosia argentea, Lantana camara, Malvastrum coromandelianum, Parthenium hysterophorus, Senna tora, Sonchus asper, Verbascum thapsus, Typha domingensis, etc.).
Plant Species
The grasses selected for the study, Saccharum ravennae (Ravennagrass, Ekra) and Cynodon dactylon (Indian Doab, couch grass), were the most abundant naturally colonizing species at the dumps. These grasses are excellent soil binders, prevent erosion and facilitate slope stabilization. These were also seen in the nearby surrounding areas. S. ravennae is a perennial and erect grass that grows in large, dense clumps with a deep network of large rhizomatous roots. Besides ecological advantages, it is also an integral part of the socio-economic fabric of native rural poor. C. dactylon is a perennial and prostrate grass that grows as soft, dense mat with shallow stoloniferous roots. It has a high fodder value.
Fly Ash/Soil Sampling and Study Design
The geochemical traits of ash/soil samples from different habitats (fly ash dumps and experimental garden) and plant rhizosphere were taken as explanatory factors affecting the diversity of IAA producing bacteria. The rhizosphere and non-rhizosphere (bulk) fly ash (50 g) was collected from the dumps abandoned before 5 and 15 years (stressed manmade environment). The rhizosphere (S. ravennae and C. dactylon) and non-rhizosphere ash samples (at 6 cm depth) were collected from three dumps of the same age as replicates. The dumps used as replicates were at least 150 m apart. Each replicate of the ash sample was a pooled mixture of three randomly selected sub-samples (at least 30 m apart) from the rhizosphere of plant phenotypes and non-rhizosphere ash from one dump. Similar to ash dumps, the garden soil samples (rhizosphere and non-rhizosphere) were also collected from plots of the experimental garden (unstressed manmade environment). To sample rhizosphere soil/ash, we used a sterile acid-treated polypropylene digger and carefully uprooted the plants, and collected the ash/soil loosely attached to the roots. The ash/soil samples were collected in black polyethene bags and transported to the laboratory in an ice bucket. The rhizosphere and non-rhizosphere ash/soil samples were immediately used for bacterial isolation. However, the ash/soil samples were air-dried and sieved (2.0 mm) before other analyses.
Nutrient and Elemental Analysis
Fly ash/soil samples were analyzed for chemical properties (pH, electrical conductivity), nutrients (PO4-P, NO3-N, % organic matter) and trace elements using standard analytical methods (Allen, 1989; Sharma et al., 2016). The pH and electrical conductivity of soil/fly ash were determined in distilled water suspension (1:5 wt/vol), prepared by shaking for 1 h and filtering through Whatman filter paper #1, using digital pH meter (Mettler Toledo, Switzerland) and conductivity meter (Metrex Ltd., India) (Kaur et al., 2012). Contents of PO4-P and NO3-N were determined by molybdenum-blue method (Allen, 1989) and salicylate blue method (Anderson and Ingram, 1993), respectively. Organic matter was determined using dichromate oxidation followed by titration with ferrous ammonium sulfate (Walkley and Black, 1934). Fly ash and soil samples were extracted with diethylenetriaminepentaacetic acid (DTPA) for selected trace elements (Fe, Na, Ni, Cd, Cr, Zn, Cu, Co) and their contents were estimated using atomic absorption spectrophotometric (AAS) analyses (Analytic Jena, Germany) (Gupta and Sinha, 2008). Standards of trace elements (1 M in 1.5% HNO3) were procured from E. Merck, Germany.
Isolation and Purification of Microbial Communities
Nine hundred and four bacterial isolates were purified from the rhizosphere of S. ravennae, C. dactylon and non-rhizosphere region from three different habitats, i.e., young and old fly ash dumps and garden using improved culture based technique (Sipkema et al., 2011). The isolates representing nine bacterial communities were given code having prefix representing site and host species (Sites–FAO: Fly ash dump old; FAY: Fly ash dump young; EG: Experimental garden; Fly ash/soil– Sr: Saccharum ravennae rhizosphere; Cd: Cynodon dactylon rhizosphere) and non-rhizosphere or bulk (Bs: Bulk soil; BFa: Bulk fly ash). The bacterial isolates were purified by employing 6 media, which include nutrient agar or NA (g/l: peptic digest of animal tissue 5, sodium chloride 5, beef extract 1.5, yeast extract 1.5, agar-agar 15, Himedia), Bushnell Hass agar or BHA (g/l: magnesium sulfate 0.2, calcium chloride 0.02, mono-potassium phosphate 1, di-potassium phosphate 1, ammonium nitrate 1, ferric chloride 0.05, agar-agar 20, Himedia), R2A Agar (g/l: casein acid hydrolysate 0.5, yeast extract 0.5, proteose peptone 0.5, dextrose 0.5, starch, soluble 0.5, di-potassium phosphate 0.3, magnesium sulfate 0.024, sodium pyruvate 0.3, agar-agar 15, Himedia) and 10 times dilutions of NA, BHA and R2A. Morphologically distinct bacterial colonies developed on the media plates until 3 days of incubation at 30°C were purified. Colony morphology of bacterial isolates was analyzed, and glycerol stocks of all the 904 bacterial isolates were prepared in triplicates and stored at −80°C.
Estimation of IAA Levels and Categorization of Bacteria into IAA Functional Types
Variation in IAA production among the isolates of all bacterial communities was determined using standard Salkowsky's reagent spectrophotometric assay (Patten and Glick, 2002). Three biological replicates of each of the 904 isolates were grown in LB medium (g/l: tryptone 10, yeast extract 5, NaCl 10, pH 7.5) supplemented with L-tryptophan (2 mg/ml) for 48 h at 30°C. The culture was centrifuged at 15,000 × g for 2 min, and the supernatant was collected. An aliquot (50 μl) of the supernatant was poured in a 300 μl microplate well, and 200 μl of Salkowsky's reagent (2% 0.5M FeCl3 and 35% perchloric acid) was added, and the mixture was kept at RT for 20 min. The OD535nm was measured using microplate reader (BioTek, USA) and the concentration of IAA was determined by comparing with IAA standard (Sigma, USA).
After reviewing the literature for a useful range of IAA (Spaepen et al., 2007), we grouped the isolates into five different functional types. These include: Very low (<2 μg/ml)-, Low (2.01–6 μg/ml)-, Moderate (6.01–10 μg/ml)-, Slightly high (10.01–14 μg/ml)-, and High (>14.01 μg/ml)- IAA producer. The mean IAA production by each bacterial community was also determined.
Statistical Analysis
Z-test was used to identify any significant difference in relative abundance of each bacterial IAA functional type from the rhizosphere of each plant species and the non-rhizosphere region of each habitat. BOX-plot analysis was used to determine the variability in different IAA producing bacterial communities.
Principal component analysis (PCA) was used to recognize the pattern of distribution of sites based on the environmental variables. Based on the PCA biplot, we analyzed the effect of plant species (S. ravennae vs. C. dactylon), rhizosphere (rhizosphere vs. non-rhizosphere), the age of dump (young dump vs. old dump) and the habitat (ash dump vs. experimental garden) on environmental variables. Thirteen environmental variables (PO4-P, NO3-N, % organic matter, pH, electrical conductivity, Fe, Na, Ni, Cd, Cr, Zn, Cu, Co) were included in PCA analysis (Table S2). The data were log-transformed before conducting PCA to standardize the variables.
Canonical correspondence analysis (CCA) (XLSTAT ver 2014) was used to explain the relationship of IAA functional types with host plant species (S. ravennae and C. dactylon), habitat type (young and old fly ash dumps, and experimental garden) and environmental variables. Only significant environmental variables (having VIF inflation value <10) were included in the CCA triplot (pH, NO3-N, PO4-P, Na, and Fe) (Table S3). CCA helped to visualize the relationship between IAA functional types with environmental variables of different sites (age of dump, plant host) in two-dimensional scale.
Independent sample t-test was used to ascertain any significant difference between soil geochemical traits of fly ash and mean IAA levels of bacterial communities of different host plants and habitats. The analyses include pair-wise comparison of non-rhizosphere vs. rhizosphere, fly ash vs experimental garden, young dump vs old dump; and S. ravennae rhizosphere vs C. dactylon rhizosphere with respect to the geochemical traits of fly ash and soil (chemical properties: pH, electrical conductivity; nutrients: PO4-P, NO3-N, % organic matter; and trace elements: Fe, Na, Ni, Cd, Cr, Zn, Cu, Co); and mean IAA level of the bacterial communities (Figure 2 and Figures S1–S4). The comparison also meant to strengthen the results of the multivariate analysis (CCA), i.e., specific association of a particular environmental variable (NO3-N, PO4-P, Fe, Na) with particular plant species/site and IAA functional type. XLSTAT ver 2014 was used to carry out Z-test, PCA, and CCA. SPSS ver 16 was used to carry out independent samples t-test.
Results
Geochemical Characteristics of Rhizosphere and Non-rhizosphere Fly Ash
The rhizosphere represents a contrasting environment as compared with the non-rhizosphere region, specifically due to high content of NO3-N, organic matter, PO4-P, Na, Cd, Cu, and Zn (Figures S1, S3). The rhizosphere of S. ravennae showed enrichment of NO3-N at both young (t-test, p = 0.019) and old dumps (t-test, p = 0.014), whereas rhizosphere of C. dactylon showed enrichment of PO4-P at the old dump (t-test, p = 0.007) (Figures S2, S3; Table S1). Though macronutrient levels at fly ash dumps were limiting, both the grass rhizosphere showed enrichment of nutrients with the age of the dump. Specifically, C. dactylon showed enrichment of organic matter content (t-test, p = 0.011) and S. ravennae showed enrichment of NO3-N (t-test, p < 0.000) (Table S1, Figure S2). Rhizosphere of both grass species showed an increase of PO4-P with the age of the dump, however, the difference was not significant.
In PCA, S. ravennae rhizosphere was separated from that of C. dactylon along the secondary axis (Figure 1) suggesting a noticeable effect of plant species on geochemical traits of fly ash rather than that of dump age. However, non-rhizosphere (bulk) region at both the dumps separated out from the rhizosphere of both grasses considerably, depicting substantial differences in geochemical traits between rhizosphere and non-rhizosphere regions (Table S2). Unlike ash, the non-rhizosphere and rhizosphere soil of the garden grouped together as the garden soil has sufficient nutrients and moisture and periodic mixing of soil was common. The three-component solution in PCA explained 86.69% of the total variance.
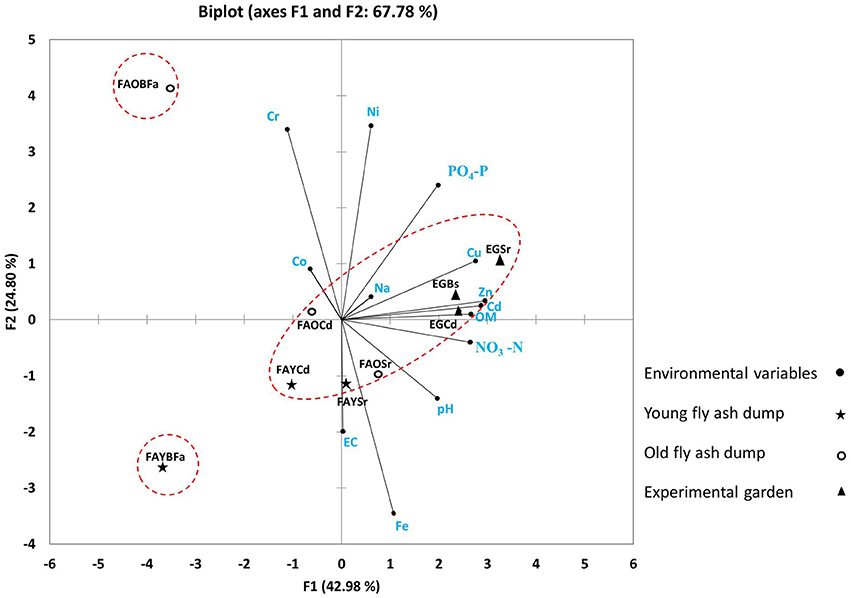
Figure 1. Principal component analysis showing the positions of rhizosphere ash/soil samples of S. ravennae and C. dactylon and non-rhizosphere (bulk) ash/soil from fly ash dumps of different ages and experimental garden. Based on geochemical properties, the rhizosphere and non-rhizosphere fly ash are separated depicting substantial differences. S. ravennae rhizosphere samples separated out from C. dactylon rhizosphere samples along the secondary axis (in this figure) suggesting a noticeable effect of plant species on geochemical traits of fly ash than the age of dump. FAO and FAY, Old and young fly ash dumps; Sr, S. ravennae rhizosphere; Cd, C. dactylon rhizosphere; EG, experimental garden; Bs/BFa, Bulk soil/fly ash.
Variation in IAA Functional Types in Bacterial Communities from Rhizosphere and Non-rhizosphere Ash
The rhizobacterial communities of fly ash produced the significantly higher level of IAA (t-test, p < 0.05) as compared with the non-rhizosphere or bulk communities, however, the trend was opposite in the garden (Figure 2A). The rhizobacterial communities of old dumps from both plant species produced significantly high mean IAA levels (t-test, p < 0.05) as compared with young dumps (Figure 2B).
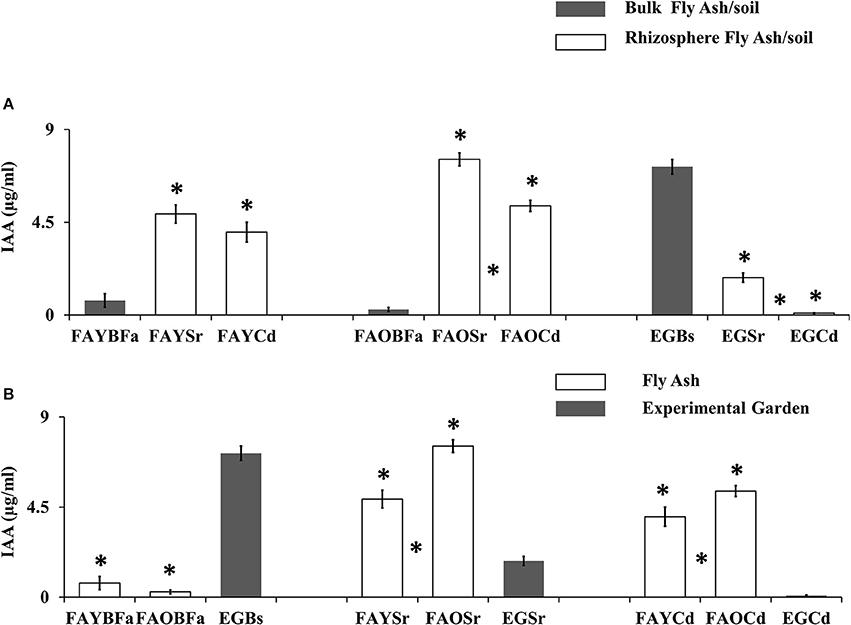
Figure 2. (A) Comparison of microbial IAA production from non-rhizosphere (bulk) and rhizosphere (S. ravennae and C. dactylon) from fly ash dumps and experimental garden. “*” Above the bar indicates significant difference from the non-rhizosphere region. “*” Between the bars indicates a significant difference between S. ravennae and C. dactylon rhizosphere ash/soil samples. (B) Comparison of microbial IAA production from old fly ash dump, young ash dump and experimental garden. “*” Above the bar indicates significant difference from the experimental garden. “*” Between the bars indicates a significant difference between a young dump and old dump. All data are presented as mean ± SE of IAA (μg/ml) production by bacterial communities; Significant differences were calculated using Independent sample t-test (p < 0.05).
BOX plot analysis showed a high variability in IAA functional types in rhizobacterial communities from fly ash dumps as compared with their respective non-rhizosphere communities and the rhizobacterial community from the garden (Figure 3). The rhizobacterial community of S. ravennae and C. dactylon showed a high variability in bacterial IAA types at the young and old dumps respectively (Figure 3).
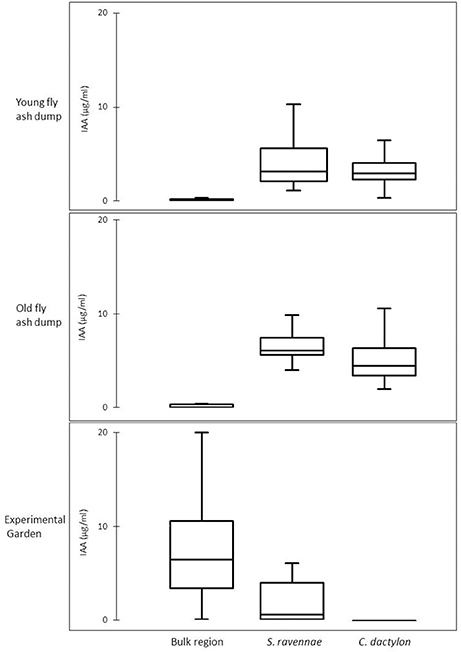
Figure 3. Variability in IAA production of bacterial communities from non-rhizosphere (bulk) and rhizosphere ash/soil samples of S. ravennae and C. dactylon from fly ash dumps of different age and experimental garden.
Relative abundance of different IAA functional types in bacterial communities in the rhizosphere of two different grass species at three habitats differed significantly. For example, the rhizobacterial communities of ash dumps showed a high variability of IAA functional types (Figure 4A, Table S4). The non-rhizosphere (bulk) region had a significantly higher relative abundance of Very low IAA producers in comparison to rhizosphere (S. ravennae and C. dactylon) at both the dumps (Z-test, p < 0.05). On the other hand, S. ravennae harbors a significantly higher relative abundance of Low, Moderate and High IAA producers at the young dump (Z-test, p < 0.05). In addition to this, C. dactylon rhizosphere had a higher relative abundance of Low and Moderate IAA producers at the young dump. Similarly, at the old dumps both S. ravennae and C. dactylon rhizosphere had a high relative abundance of low, moderate and slightly high IAA producers (Z-test, p < 0.05).
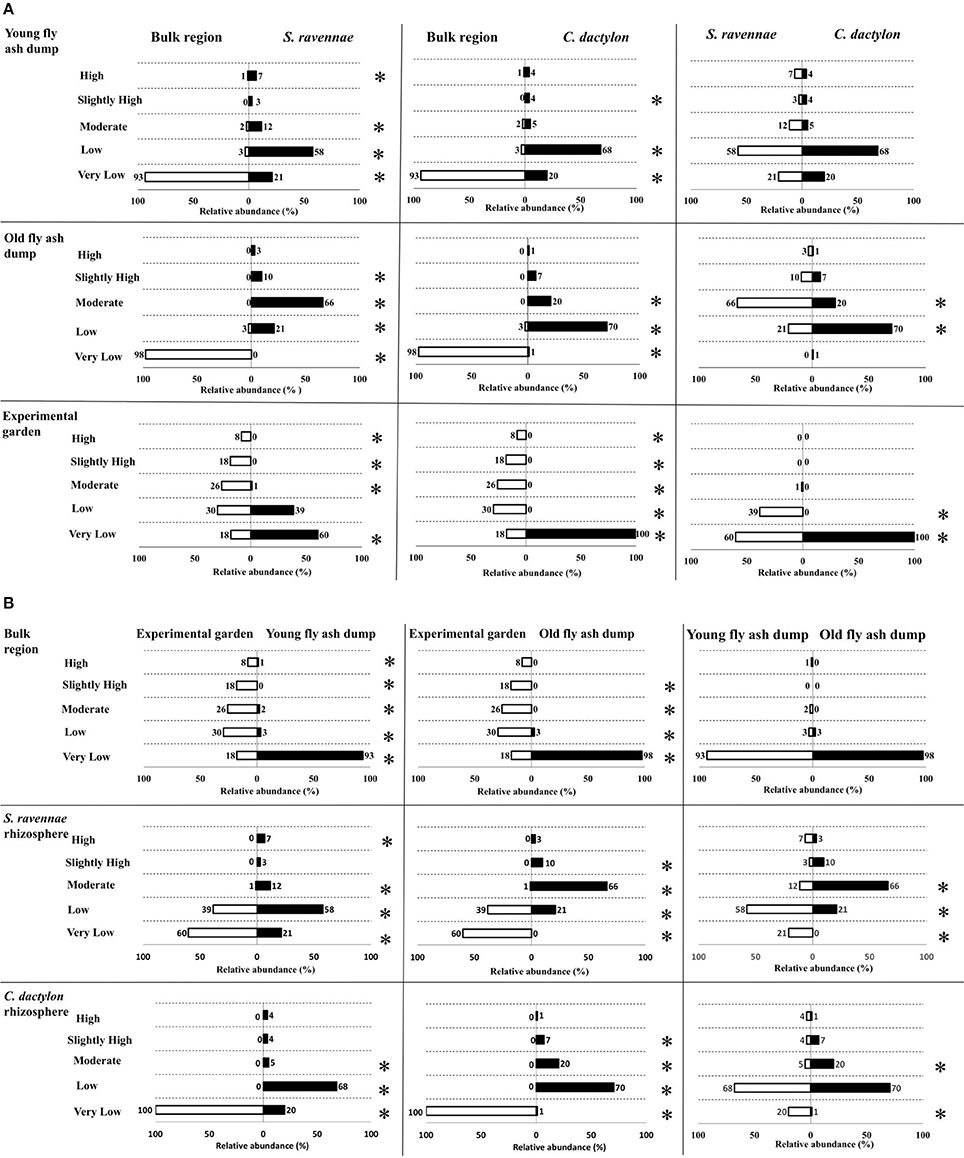
Figure 4. Comparison of the relative abundance of IAA functional types from bacterial communities from non-rhizosphere (bulk) and rhizosphere ash/soil samples of S. ravennae and C. dactylon (A), and different habitats, i.e., fly ash dumps of different age and experimental garden (B). Numbers above the horizontal bars represent the proportion of isolates belonging to each functional type; “*” given on right side of the chart denotes the significant difference (Z-test, p < 0.05) between the relative abundance of IAA functional types.
While comparing both the dumps non-rhizosphere region had no significant differences in terms of relative abundance of IAA functional types (Figure 4B). S. ravennae rhizosphere had a significantly high relative abundance of Very low and Low IAA producers at the young dump (Z-test, p < 0.05). However, at the old dumps, it had a significantly higher relative abundance of Moderate and Slightly high IAA producers (Z-test, p < 0.05). Similarly, C. dactylon rhizosphere at the young dump had a significantly higher relative abundance of Very low IAA producers, but at the old dump, it had a significantly higher relative abundance of Moderate IAA producers (Z-test, p < 0.05).
Relationship between Microbial IAA Functional Types, Environmental Variables, and Host Plant Species
The bacterial IAA producers from S. ravennae, C. dactylon and non-rhizosphere (bulk) region formed three distinct groups regardless of the habitat in CCA triplot (Figure 5). Also, the three separate groups housed distinct bacterial IAA functional types. For example, Group 1 consisted of rhizobacteria of C. dactylon with Low, Slightly high and High IAA functional types. On the other hand, Group 2 consisted of rhizobacteria of S. ravannae with Moderate IAA functional type. Group 3 consisted of non-rhizosphere isolates with Very low IAA functional type. Interestingly, the rhizobacterial isolates of C. dactylon from the garden grouped with bacterial communities of the non-rhizosphere region.
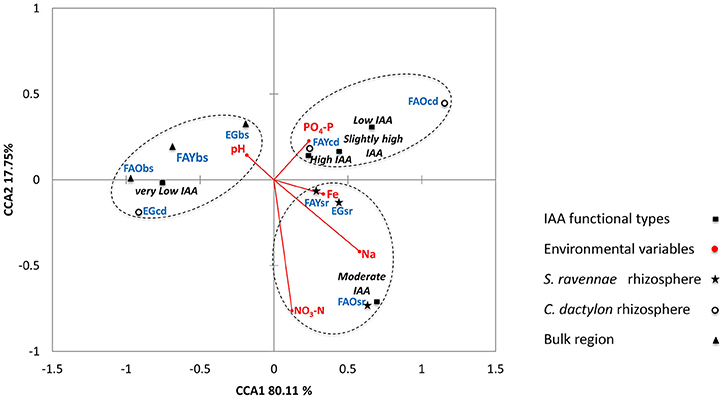
Figure 5. Triplot of canonical correspondence analysis relating the IAA functional types (Very low, Low, Moderate, Slightly high, and High) from the rhizosphere of S. ravennae and C. dactylon, and non-rhizosphere (bulk) region from fly ash dumps of different ages and experimental garden, with environmental variables.
CCA demonstrated that Fe, PO4-P, NO3-N, Na, and pH are the major predictors of the micro-variations in IAA producers (Figure 5). Environmental variables and habitat explained 97.86% of micro-variations in IAA producers (Table S3). The rhizobacterial communities separated out from that of the non-rhizosphere (bulk) region along the second canonical axis explaining 17.75% variation in IAA functional types. The rhizobacterial communities from S. ravennae and C. dactylon were separated from each other, regardless of the habitats, along with the first canonical axis that explained 80.11% variation in IAA functional types. The first axis positively correlated with Fe, Na, and PO4-P and negatively correlated with pH. On the other hand, the second axis negatively correlated with NO3-N. Based on the length of the arrows in CCA triplot, we suggest NO3-N as the major predictor with a strong positive influence on S. ravennae rhizobacterial communities (Figure 5), whereas PO4-P as the major predictor with a positive influence on C. dactylon rhizobacterial communities.
Discussion
In this study, we identified the environmental predictors of micro-variations in bacterial IAA producers. We purified the non-rhizosphere and rhizobacterial communities of different grasses from fly ash dumps of different ages and the experimental garden and estimated the bacterial IAA levels. Based on multivariate analyses, the study showed a significant impact of host plants on the functional variation of IAA producers with specific nutrient as a major predictor (S. ravennae–NO3-N; C. dactylon– PO4-P). Fe, Na, NO3-N, and PO4-P were the major predictors of IAA functional types.
The separate grouping of rhizosphere and non-rhizosphere (bulk) fly ash in PCA provided evidence on the role of the wild grasses and associated IAA producers in habitat processing that convert the dumps into the biologically productive habitats. The significant accumulation of functionally diverse IAA producers in rhizosphere ash (Figure 5) indicates the role of plant-soil feedback in plant colonization and survival under the contaminated and nutrient stressed environment of the dumps (Figures S1, S2). The diverse IAA types enhance the growth of host plant in degraded soil (Bianco et al., 2006). In fact, the specific environmental stress selects a particular functional group of bacteria at the site (Aislabie et al., 2004). However, limited information is available on how plant-microbe interactions affect the soil geochemical traits and in turn influence the microbial functional diversity (Cong et al., 2015). In contrast with fly ash, the garden sample lacked rhizosphere effect where periodic mixing of soil, the addition of fertilizer and frequent irrigation were common. Other researchers have also shown fertilization-induced alteration in microbial diversity in soil (Koyama et al., 2014).
S. ravennae and C. dactylon provided evidence of driving the assembly of IAA producers in their rhizosphere via enrichment of NO3-N and PO4-P, respectively (Figure 5). Host plant influences assembly of microbial communities through environmental filtering (Hartmann et al., 2009). In fact, N and P facilitate the spread of grasses over other herbs (Xia and Wan, 2008; Xu et al., 2015). In fact, the response of N and P substantially varies among grass species (Ryser et al., 1997). van der Heijden (2002) have shown that arbuscular mycorrhizal fungi assist the coexistence of plant species through the allocation of N and P. However, the influence of N and P on bacterial IAA producers of grasses naturally colonizing a degraded ecosystem has not yet been examined. Our results suggest an important but differential role of N and P on IAA functional types in the rhizosphere of different grass species, which also represents an important plant-soil feedback process.
Among other soil geochemical factors, Fe, Na, and pH were the important drivers that explained the variation in microbial IAA functional types. To our knowledge, this is first evidence from the environment to show the Fe and Na as predictors of microbial IAA functional types. It may be noted that Fe serves as a regulator of IAA biosynthesis (Costacurta and Vanderleyden, 1995; Kamnev et al., 2001). IAA producers also alleviate salinity stress in host plants (Egamberdieva, 2009).
High levels of weathering and leaching of ash due to annual precipitation are presumed to have resulted in low salinity in the non-rhizosphere (bulk) ash of the old dump (Figure S2). However, high biological availability and retention of ions caused a significant increase in salinity in the rhizosphere of S. ravennae at the old dump. The rhizosphere of S. ravnnae also showed an increase in Na content (Figure S2). S. ravennae is a native species of floodplains and is well adapted to soils with a high level of ions deposited through the periodic addition of silt from the river (Rau et al., 2009). In contrast, the lack of similar pattern in the rhizosphere of C. dactylon is due to the combination of factors viz., shallow roots of the grass, high leaching, and role of other ions besides Na in electrical conductivity.
pH represents another important geochemical factor in shaping bacterial IAA types at the ash dumps (Figure 5). pH serves as a major predictor of microbial functional diversity in different vegetation types (Shen et al., 2013). Typically, high pH in fly ash limits biologically available Fe, Cu, Zn, and P, therefore these elements featured in differentiating non-rhizosphere and rhizosphere soil (Figure 1 and Figure S1). pH and bioavailable nutrients have reciprocal effects on each other. Therefore, it is important to examine the complex relationship between pH and PO4-P, NO3-N, Fe, and Na and their cumulative impact on bacterial IAA functional types.
The influence of heavy metals on IAA producers is complex. For example, Cu II and Cr II reduce the IAA production in Azospirillum brasilense (Kirchhof et al., 1997). In Bacillus JH 2-2 from a mine site, different levels of Cr VI stimulated or inhibited the IAA production, which also influenced bacterial potential to promote plant growth (Shim et al., 2015). The organic matter reduces heavy metal bioavailability. Consequently, the impact of heavy metals of fly ash on IAA producers becomes more complex in the presence of organic matter. Therefore, the role of these elements (Cd, Cu, and Zn) (Figures S1, S3) on soil microbes belonging to different IAA types requires additional research.
The severe deficiency of N remains a major challenge for ash management (Tropek et al., 2014). Our results show nitrogen enrichment in S. ravennae rhizosphere with the age of dump (Figure S1). Saccharum spp. with both endophytic and rhizosphere microbes fix nitrogen (Momose et al., 2009; Rau et al., 2009). Also, in extreme N-deprivation condition, IAA also serves as an alternate source of nitrogen (Scott et al., 2013). Therefore, S. ravennae is one of the ecologically important species that accelerate the revegetation of N-deficient fly ash dump.
The culture-based approach investigates only a fraction of the total bacterial community. However, such an approach is important to analyze plant-soil feedback process as it considers the cellular activity (Lebaron et al., 2001) and avoids common but dormant forms of bacteria (Kell et al., 1998). Measuring IAA production helps analyze ecologically important and metabolically active bacteria from the dumps, not the inactive cells, which do not contribute to ecosystem function. Therefore, the culture-based methods were found more suitable for contaminated soils, to relate environmental conditions and a microbial community as compared with the culture-independent methods (Ellis et al., 2003; Al-Awadhi et al., 2013; Douterelo et al., 2014). The culture-based approach also provides live bacterial culture for inoculation to assist the plant growth.
The IAA helps the host plant and a microbial community to adapt to diverse stress conditions (Bianco et al., 2006). Therefore, characterization of IAA functional types in rhizobacteria of ecologically successful species from degraded sites and identification of their environmental predictors have significance in the development of biostimulation and bioremediation methods for vegetation restoration. In fact, microbiologists have already revealed importance of variations in IAA level in microbes to promote plant growth, protect plants from stresses, and increase productivity for sustainable agriculture (Liu et al., 2016). Depending on the level of IAA, the yeast showed an inhibitory effect on specific strains competing for resources, and a stimulatory effect on other yeasts or their growth. Further, high diversity of microbial functional types is a representation of a resilient microbial community (Sharma et al., 2008; Sharma R. S. et al., 2011). The increase in resilience is the primary goal to develop a self-sustaining microbial community in a degraded environment. Therefore, identification of environmental predictors, which influence the variability in microbial functional types, would be useful not only for biostimulation practices but also to increase the resilience of the microbial community.
Environmental predictors of microbial IAA functional types at fly ash dumps will be useful to evolve remediation and revegetation plans. In our case, the use of culture-based approach is necessary to develop in situ bioremediation at dumps. The microbial community of grass species irrespective of habitat formed different groups, suggesting that different strategies will biostimulate indigenous IAA producers of different grass species. Specifically, S. ravennae accumulated nitrogen, and C. dactylon accumulated phosphorus in their rhizosphere fly ash. Therefore, depending on the grass species, we may need to supplement nitrogen and phosphorus to accelerate vegetation development and phytostabilize the fly ash dumps. However, understanding the interactions among the environmental variables identified in the study will play a key role in developing nature-based in situ bioremediation and vegetation restoration strategies for the dumps.
To conclude, the present study identifies the NO3-N, PO4-P, pH, Fe, and Na as environmental predictors of functional diversity of rhizobacterial IAA producers of S. ravennae and C. dactylon colonizing fly ash environment. Based on the multivariate analyses, we propose N or P amendment to stimulate rhizobacterial IAA producers and facilitate the spread of S. ravennae and C. dactylon, respectively. Such a biostimulation approach based on plant-soil feedback process would serve as a nature-based solution for vegetation restoration at fly ash dumps. The mats of C. dactylon would help in the surface stabilization of dumps preventing the spread of ash, whereas large clumps of S. ravennae would help in the enrichment of organic matter. Our findings suggest that a better understanding of the interactions between N and P with other soil-geochemical factors, such as pH, Fe, and Na and their impact on microbial communities will help to develop efficient revegetation plans at the ash dumps. Further studies, both in controlled and field environment involving grass species with supplementation of specific nutrients would be required to develop an effective biostimulation strategy for the on-field application.
Author Contributions
VM, RS, SM conceived and designed the research; SM performed the experiments, SK isolated and purified the bacteria; SM, VM, RS analyzed the data, wrote and edited the manuscript.
Conflict of Interest Statement
The authors declare that the research was conducted in the absence of any commercial or financial relationships that could be construed as a potential conflict of interest.
Acknowledgments
Authors thank the reviewers and editors for their constructive and extensive comments to improve the quality of MS. VM thanks, Department of Science and Technology (DST), Govt. of India for the SERB Women Excellence Award grant. VM and RS thank the University of Delhi for Faculty Research Grant and DU-DST PURSE Grant Phase II. SK thanks, University Grant Commission (UGC) for providing Junior Research Fellowship and SM thanks the University of Delhi for Non-NET Fellowship.
Supplementary Material
The Supplementary Material for this article can be found online at: http://journal.frontiersin.org/article/10.3389/fenvs.2017.00059/full#supplementary-material
Figure S1. Effect of Rhizosphere (rhizosphere vs. non-rhizosphere or bulk) and plant species (S. ravennae vs. C. dactylon) showed significant impact on soil geochemical characteristics. All data are presented as mean ± SE calculated from 3 replicates. The full name of each site is provided in Figure 1. (Note: “*” above the bars: Significant difference (t-test, p < 0.05) between rhizosphere and non-rhizosphere region; “*” between the bars: Significant difference (t-test, p < 0.05) between S. ravennae vs. C. dactylon).
Figure S2. Effect of habitat (fly ash dumps vs. garden) and age of the fly ash dump (young vs. old dump) on soil geo-chemical characteristics. All data are presented as mean ± SE calculated from 3 replicates. The full name of each site is provided in Figure 1. (Note: “*” above the bars: Significant difference (t-test, p < 0.05) between fly ash dumps and experimental garden; “*” between the bars: Significant difference (t-test, p < 0.05) between the young and old fly ash dump).
Figure S3. Effect of rhizosphere (rhizosphere vs non-rhizosphere or bulk) and plant species (S. ravennae vs C. dactylon) on trace and essential elements in fly ash/soil. All data are presented as mean ± SE calculated from 3 replicates. The full name of each site is provided in Figure 1. (Note: “*” above the bars: Significant differences (t-test, p < 0.05) between rhizosphere and non-rhizosphere region; “*” between the bars: Significant differences (t-test, p < 0.05) between S. ravennae vs. C. dactylon).
Figure S4. Effect of habitat (fly ash dumps vs. garden) and age of the fly ash dump (young vs. old dump) on trace and essential elements in fly ash/soil. All data are presented as mean ± SE calculated from 3 replicates. The full name of each site is provided in Figure 1. (Note: “*” above the bars: Significant difference (t-test, p < 0.05) between fly ash dumps and experimental garden; “*” between the bars: Significant difference (t-test, p < 0.05) between the young and old fly ash dump).
References
Ahmaruzzaman, M. (2010). A review on the utilization of fly ash. Prog. Energy Combust. Sci. 36, 327–363. doi: 10.1016/j.pecs.2009.11.003
Aislabie, J. M., Balks, M. R., Fogh, J. M., and Waterhouse, E. J. (2004). Hydrocarbon spills on Antarctic soils: effects and management. Environ. Sci. Technol. 38, 1265–1274. doi: 10.1021/es0305149
Al-Awadhi, H., Dashti, N., Khanafer, M., Al-Mailem, D., Ali, N., and Radwan, S. (2013). Bias problems in culture-independent analysis of environmental bacterial communities: a representative study on hydrocarbonoclastic bacteria. Springerplus 2:369. doi: 10.1186/2193-1801-2-369
Allen, S. E. (1989). Chemical Analysis of Ecological Materials. London: Blackwell Scientific Publication.
Anderson, J. M., and Ingram, J. S. I. (1993). Tropical Soil Biology and Fertility: A Handbook of Methods. Wallingford, CT: CABI Publishing.
Asokan, P., Saxena, M., and Asolekar, S. R. (2005). Coal combustion residues—environmental implications and recycling potentials. Resour. Conserv. Recy. 43, 239–262. doi: 10.1016/j.resconrec.2004.06.003
Bhattacharya, D., Sarma, P. M., Krishnan, S., Mishra, S., and Lal, B. (2003). Evaluation of genetic diversity among Pseudomonas citronellolis strains isolated from oily sludge-contaminated sites. Appl. Environ. Microb. 69, 1435–1441. doi: 10.1128/AEM.69.3.1435-1441.2003
Bianco, C., Imperlini, E., Calogero, R., Senatore, B., Amoresano, A., Carpentieri, A., et al. (2006). Indole-3-acetic acid improves Escherichia coli's defences to stress. Arch. Microbiol. 185, 373–382. doi: 10.1007/s00203-006-0103-y
Cadotte, M. W., Carscadden, K., and Mirotchnick, N. (2011). Beyond species: functional diversity and the maintenance of ecological processes and services. J. Appl. Ecol. 48, 1079–1087. doi: 10.1111/j.1365-2664.2011.02048.x
Cong, J., Yang, Y., Liu, X., Lu, H., Liu, X., Zhou, J., et al. (2015). Analyses of soil microbial community compositions and functional genes reveal potential consequences of natural forest succession. Sci. Rep. 5:10007. doi: 10.1038/srep10007
Costacurta, A., and Vanderleyden, J. (1995). Synthesis of phytohormones by plant-associated bacteria. Crit. Rev. Microbiol. 21, 1–18. doi: 10.3109/10408419509113531
Diaz, S., and Cabido, M. (2001). Vive la difference: plant functional diversity matters to ecosystem processes. Trends Ecol. Evol. 16, 646–655. doi: 10.1016/S0169-5347(01)02283-2
Douterelo, I., Boxall, J. B., Deines, P., Sekar, R., Fish, K. E., and Biggs, C. A. (2014). Methodological approaches for studying the microbial ecology of drinking water distribution systems. Water Res. 65, 134–156. doi: 10.1016/j.watres.2014.07.008
Egamberdieva, D. (2009). Alleviation of salt stress by plant growth regulators and IAA producing bacteria in wheat. Acta Physiol. Plant 31, 861–864. doi: 10.1007/s11738-009-0297-0
Ellis, R. J., Morgan, P., Weightman, A. J., and Fry, J. C. (2003). Cultivation-dependent and -independent approaches for determining bacterial diversity in heavy-metal-contaminated soil. Appl. Environ. Microb. 69, 3223–3230. doi: 10.1128/AEM.69.6.3223-3230.2003
Eviner, V. T., and Hawkes, C. V. (2008). Embracing variability in the application of plant-soil interactions to the restoration of communities and ecosystems. Restor. Ecol. 16, 713–729. doi: 10.1111/j.1526-100X.2008.00482.x
Gupta, A. K., and Sinha, S. (2008). Decontamination and/or revegetation of fly ash dykes through naturally growing plants. J. Hazard. Mater. 153, 1078–1087. doi: 10.1016/j.jhazmat.2007.09.062
Hartmann, A., Schmid, M., van Tuinen, D., and Berg, G. (2009). Plant-driven selection of microbes. Plant Soil 321, 235–257. doi: 10.1007/s11104-008-9814-y
Haynes, R. J. (2009). Reclamation and revegetation of fly ash disposal sites - challenges and research needs. J. Environ. Manage. 90, 43–53. doi: 10.1016/j.jenvman.2008.07.003
Hvistendahl, M. (2007). Coal ash is more radioactive than nuclear waste. Sci. Am. 13:112. Available online at: http://www.scientificamerican.com/article/coal-ash-is-more-radioactive-than-nuclear-waste/ (Accessed August 31, 2017).
Inderjit and Cahill, J. F. (2015). Linkages of plant-soil feedbacks and underlying invasion mechanisms. AoB Plants 7:plv022. doi: 10.1093/aobpla/plv022
Kamnev, A., Shchelochkov, A., Perfiliev, Y., Tarantilis, P., and Polissiou, M. (2001). Spectroscopic investigation of indole-3-acetic acid interaction with iron(III). J. Mol. Struct. 563–564, 565–572. doi: 10.1016/S0022-2860(00)00911-X
Kaur, R., Malhotra, S., and Inderjit (2012). Effects of invasion of Mikania micrantha on germination of rice seedlings, plant richness, chemical properties and respiration of soil. Biol. Fertil. Soils 48, 481–488. doi: 10.1007/s00374-011-0645-2
Kell, D. B., Kaprelyants, A. S., Weichart, D. H., Harwood, C. R., and Barer, M. R. (1998). Viability and activity in readily culturable bacteria: a review and discussion of the practical issues. Anton. Leeuw. 73, 169–187. doi: 10.1023/A:1000664013047
Kirchhof, G., Schloter, M., Abmus, B., and Hartmann, A. (1997). Molecular microbial ecology approaches applied to diazotrophs associated with non legumes. Soil Biol. Biochem. 29, 853–862. doi: 10.1016/S0038-0717(96)00233-7
Koyama, A., Wallenstein, M. D., Simpson, R. T., and Moore, J. C. (2014). Soil bacterial community composition altered by increased nutrient availability in Arctic tundra soils. Front. Microbiol. 5:516. doi: 10.3389/fmicb.2014.00516
Krause, S., Le Roux, X., Niklaus, P. A., van Bodegom, P. M., Lennon, T. J. T., Bertilsson, S., et al. (2014). Trait-based approaches for understanding microbial biodiversity and ecosystem functioning. Front. Microbiol. 5:251. doi: 10.3389/fmicb.2014.00251
Lebaron, P., Servais, P., Agogué, H., Courties, C., and Joux, F. (2001). Does the high nucleic acid content of individual bacterial cells allow us to discriminate between active cells and inactive cells in aquatic systems? Appl. Environ. Microbiol. 67, 1775–1782. doi: 10.1128/AEM.67.4.1775-1782.2001
Liu, Y. Y., Chen, H. W., and Chou, J. Y. (2016). Variation in indole-3-acetic acid production by wild Saccharomyces cerevisiae and S. paradoxus strains from diverse ecological sources and its effect on growth. PLoS ONE 11:e0160524. doi: 10.1371/journal.pone.0160524
Loucas, S., Parfrey, L. W., and Doebeli, M. (2016). Decoupling function and taxonomy in the global ocean microbiome. Science 353, 1272–1277. doi: 10.1126/science.aaf4507
Mohmmed, A., Sharma, R. S., Ali, S., and Babu, C. R. (2001). Molecular diversity of the plasmid genotypes among Rhizobium gene pools of sesbanias from different habitats of a semi-arid region (Delhi). FEMS Microbiol. Lett. 205, 171–178. doi: 10.1111/j.1574-6968.2001.tb10943.x
Momose, A., Ohtake, N., Sueyoshi, K., Sato, T., Nakanishi, Y., Akao, S., et al. (2009). Nitrogen fixation and translocation in young sugarcane (Saccharum officinarum L.) plants associated with endophytic nitrogen-fixing bacteria. Microbes Environ. 24, 224–230. doi: 10.1264/jsme2.ME09105
Patten, C. L., and Glick, B. R. (2002). Role of Pseudomonas putida indoleacetic acid in development of the host plant root system. Appl. Environ. Microb. 68, 3795–3801. doi: 10.1128/AEM.68.8.3795-3801.2002
Petchey, O., and Gaston, K. (2002). Functional diversity (FD), species richness and community composition. Ecol. Lett. 5, 402–411. doi: 10.1046/j.1461-0248.2002.00339.x
Qadir, S. U., Raja, V., and Siddiqui, W. A. (2016). Morphological and biochemical changes in Azadirachta indica from coal combustion fly ash dumping site from a thermal power plant in Delhi, India. Ecotox. Environ. Safe. 129, 320–328. doi: 10.1016/j.ecoenv.2016.03.026
Rau, N., Mishra, V., Sharma, M., Das, M. K., Ahaluwalia, K., and Sharma, R. S. (2009). Evaluation of functional diversity in rhizobacterial taxa of a wild grass (Saccharum ravennae) colonizing abandoned fly ash dumps in Delhi urban ecosystem. Soil Biol. Biochem. 41, 813–821. doi: 10.1016/j.soilbio.2009.01.022
Ryser, P., Verduyn, B., and Lambers, H. (1997). Phosphorus allocation and utilisation in three grass species with contrasting response to N and P supply. New Phytol. 137, 293–302. doi: 10.1046/j.1469-8137.1997.00807.x
Scott, J. C., Greenhut, I. V., and Leveau, J. H. J. (2013). Functional characterization of the bacterial iac genes for degradation of the plant hormone indole-3-acetic acid. J. Chem. Ecol. 39, 942–951. doi: 10.1007/s10886-013-0324-x
Sharma, M., Mishra, V., Rau, N., and Sharma, R. S. (2011). Functionally diverse rhizobacteria of Saccharum munja (a native wild grass) colonizing abandoned morrum mine in Aravalli hills (Delhi). Plant Soil 341, 447–459. doi: 10.1007/s11104-010-0657-y
Sharma, M., Mishra, V., Rau, N., and Sharma, R. S. (2016). Increased iron-stress resilience of maize through inoculation of siderophore-producing Arthrobacter globiformis from mine. J. Basic Microb. 56, 719–735. doi: 10.1002/jobm.201500450
Sharma, R. S., Mishra, V., Mohmmed, A., and Babu, C. R. (2008). Phage specificity and lipopolysaccarides of stem- and root-nodulating bacteria (Azorhizobium caulinodans, Sinorhizobium spp., and Rhizobium spp.) of Sesbania spp. Arch. Microbiol. 189, 411–418. doi: 10.1007/s00203-007-0322-x
Sharma, R. S., Mishra, V., Mohmmed, A., and Babu, C. R. (2011). Variations in outer-membrane characteristics of two stem-nodulating bacteria of Sesbania rostrata and its role in tolerance towards diverse stress. Curr. Microbiol. 63, 81–86. doi: 10.1007/s00284-011-9946-9
Sharma, R. S., Mohmmed, A., and Babu, C. R. (2002). Diversity among rhizobiophages from rhizospheres of legumes inhabiting three ecogeographical regions of India. Soil Biol. Biochem. 34, 965–973. doi: 10.1016/S0038-0717(02)00030-5
Sharma, R. S., Mohmmed, A., Mishra, V., and Babu, C. R. (2005). Diversity in a promiscuous group of rhizobia from three Sesbania spp. colonizing ecologically distinct habitats of the semi-arid Delhi region. Res. Microbiol. 156, 57–67. doi: 10.1016/j.resmic.2004.08.009
Shen, C., Xiong, J., Zhang, H., Feng, Y., Lin, X., Li, X., et al. (2013). Soil pH drives the spatial distribution of bacterial communities along elevation on Changbai mountain. Soil Biol. Biochem. 57, 204–211. doi: 10.1016/j.soilbio.2012.07.013
Shim, J., Kim, J. W., Shea, P. J., and Oh, B. T. (2015). IAA production by Bacillus sp. JH 2-2 promotes Indian mustard growth in the presence of hexavalent chromium. J. Basic Microb. 55, 652–658. doi: 10.1002/jobm.201400311
Sipkema, D., Schippers, K., Maalcke, W. J., Yang, Y., Salim, S., and Blanch, H. W. (2011). Multiple approaches to enhance the cultivability of bacteria associated with the marine sponge Haliclona (gellius) sp. Appl. Environ. Microb. 77, 2130–2140. doi: 10.1128/AEM.01203-10
Smith, K. R., Veranth, J. M., Kodavanti, U. P., Aust, A. E., and Pinkerton, K. E. (2006). Acute pulmonary and systemic effects of inhaled coal fly ash in rats: comparison to ambient environmental particles. Toxicol. Sci. 93, 390–399. doi: 10.1093/toxsci/kfl062
Smith-Ramesh, L. M., and Reynolds, H. L. (2017). The next frontier of plant-soil feedback research: unraveling context dependence across biotic and abiotic gradients. J. Veg. Sci. 28, 484–494. doi: 10.1111/jvs.12519
Spaepen, S., Vanderleyden, J., and Remans, R. (2007). Indole-3-acetic acid in microbial and microorganism-plant signaling. FEMS Microbiol. Rev. 31, 425–448. doi: 10.1111/j.1574-6976.2007.00072.x
Theis, T. L., and Gardner, K. H. (1990). Environmental assessment of ash disposal. Crit. Rev. Env. Contr. 20, 21–42. doi: 10.1080/10643389009388388
Tropek, R., Cerna, I., Straka, J., Kadlec, T., Pech, P., Tichanek, F., et al. (2014). Restoration management of fly ash deposits crucially influence their conservation potential for terrestrial arthropods. Ecol. Eng. 73, 45–52. doi: 10.1016/j.ecoleng.2014.09.011
Vacheron, J., Desbrosses, G., Bouffaud, M.-L., Touraine, B., Moënne-Loccoz, Y., Muller, D., et al. (2013). Plant growth-promoting rhizobacteria and root system functioning. Front. Plant Sci. 4:356. doi: 10.3389/fpls.2013.00356
van der Heijden, M. G. A. (2002). “Arbuscular mycorrhizal fungi as a determinant of plant diversity: in search of underlying mechanisms and general principles,” in Mycorrhizal Ecology, eds M. G. A. van der Heijden and I. R. Sanders (Berlin; Heidelberg: Springer Berlin Heidelberg), 243–265.
Van der Putten, W. H., Bardgett, R. D., Bever, J. D., Bezemer, T. M., Casper, B. B., Fukami, T., et al. (2013). Plant-soil feedbacks: the past, the present and future challenges. J. Ecol. 101, 265–276. doi: 10.1111/1365-2745.12054
Walkley, A., and Black, I. A. (1934). An examination of the Degtjareff method for determining soil organic matter, and proposed modification of the chromic acid titration method. Soil Sci. 37, 29–38. doi: 10.1097/00010694-193401000-00003
Wilson, J. B. (1999). Guild, functional type and ecological groups. Oikos 86, 507–522. doi: 10.2307/3546655
Xia, J. Y., and Wan, S. Q. (2008). Global response patterns of terrestrial plant species to nitrogen addition. New Phytol. 179, 428–439. doi: 10.1111/j.1469-8137.2008.02488.x
Xiao, L., Liu, G.-B., Zang, J.-Y., and Xue, S. (2016). Long-term effects of vegetational restoration on soil microbial communities on the loess plateau of China. Restor. Ecol. 24, 794–804. doi: 10.1111/rec.12374
Xu, D., Fang, X., Zhang, R., Gao, T., and Bu, H. (2015). Influences of nitrogen, phosphorus and silicon addition on plant productivity and species richness in an alpine meadow. AoB Plants 7, 1–12 doi: 10.1093/aobpla/plv125
Keywords: ecological restoration, fly ash, functional diversity, indole acetic acid (IAA), microbial ecology, environmental predictor, soil biology
Citation: Malhotra S, Mishra V, Karmakar S and Sharma RS (2017) Environmental Predictors of Indole Acetic Acid Producing Rhizobacteria at Fly Ash Dumps: Nature-Based Solution for Sustainable Restoration. Front. Environ. Sci. 5:59. doi: 10.3389/fenvs.2017.00059
Received: 13 April 2017; Accepted: 11 September 2017;
Published: 28 September 2017.
Edited by:
Philippe C. Baveye, ÉcoSys Écologie Fonctionnelle et Écotoxicologie des Agroécosystèmes (INRA), FranceReviewed by:
Andrey S. Zaitsev, Justus Liebig Universität Gießen, GermanyRaymon Shange, Tuskegee University, United States
Copyright © 2017 Malhotra, Mishra, Karmakar and Sharma. This is an open-access article distributed under the terms of the Creative Commons Attribution License (CC BY). The use, distribution or reproduction in other forums is permitted, provided the original author(s) or licensor are credited and that the original publication in this journal is cited, in accordance with accepted academic practice. No use, distribution or reproduction is permitted which does not comply with these terms.
*Correspondence: Radhey S. Sharma, cmFkczI2QGhvdG1haWwuY29t
Vandana Mishra, bWlzdGxldG9lX2hAaG90bWFpbC5jb20=
†These authors have contributed equally to this work.