- 1School of Life Sciences, Arizona State University, Tempe, AZ, USA
- 2Smithsonian Tropical Research Institute, Ancón, Panama
- 3Flathead Lake Biological Station, University of Montana, Polson, MT, USA
The growth rate hypothesis predicts that organisms with higher maximum growth rates will also have higher body percent phosphorus (P) due to the increased demand for ribosomal RNA production needed to sustain rapid growth. However, this hypothesis was formulated for invertebrates growing at the same temperature. Within a biologically relevant temperature range, increased temperatures can lead to more rapid growth, suggesting that organisms in warmer environments might also contain more P per gram of dry mass. However, since higher growth rates at higher temperature can be supported by more rapid protein synthesis per ribosome rather than increased ribosome investment, increasing temperature might not lead to a positive relationship between growth and percent P. We tested the growth rate hypothesis by examining two genera of Neotropical stream grazers, the leptophlebiid mayfly Thraulodes and the bufonid toad tadpole Rhinella. We measured the body percent P of field-collected Thraulodes as well as the stoichiometry of periphyton resources in six Panamanian streams over an elevational gradient spanning approximately 1,100 m and 7°C in mean annual temperature. We also measured Thraulodes growth rates using in situ growth chambers in two of these streams. Finally, we conducted temperature manipulation experiments with both Thraulodes and Rhinella at the highest and lowest elevation sites and measured differences in percent P and growth rates. Thraulodes body percent P increased with temperature across the six streams, and average specific growth rate was higher in the warmer lowland stream. In the temperature manipulation experiments, both taxa exhibited higher growth rate and body percent P in the lowland experiments regardless of experimental temperature, but growth rate and body percent P of individuals were not correlated. Although we found that Thraulodes from warmer streams grew more rapidly and had higher body percent P, our experimental results suggest that the growth rate hypothesis does not apply across temperatures. Instead, our results indicate that factors other than temperature drive variation in organismal percent P among sites.
Introduction
Somatic growth rate is a fundamental trait that integrates the effects of numerous environmental pressures (Arendt, 1997). Growth capacity is a key component of organismal fitness and as a result, is often strongly selected upon (Violle et al., 2007; Dmitriew, 2011). However, an organism's realized growth rate in nature rarely reaches its maximum capacity (Calow, 1982; Arendt, 1997). Realized growth rate varies with a number of environmental factors including predation, disturbance, food quality, and temperature (Reznick and Endler, 1982; Atkinson, 1994; Dmitriew, 2011). To achieve variation in realized growth rate over gradients in these variables, organisms must also vary in the biochemical processes that underlie growth. In most organisms except for retroviruses, new tissue is formed by the transcription of DNA into mRNA, which is then sent to ribosomes for protein synthesis. Nucleic acids are relatively phosphorus-rich molecules (8.7%; Sterner and Elser, 2002), and thus variation in their abundance in organismal cells could strongly affect phosphorus (P) demands. Although DNA concentration does not vary appreciably among individuals, RNA can vary considerably and thus represents a source of variation in whole organismal %P (Sterner and Elser, 2002). As a result, variation in growth capacity can lead to variation in organismal elemental demands.
The growth rate hypothesis (GRH) states that differences in maximum growth rate drive variation in organismal %P due to greater allocation to P-rich ribosomal RNA needed to support rapid growth (Elser et al., 1996, 2000a, 2003; Hessen et al., 2013). The GRH has been supported by empirical tests in a wide variety of organisms, particularly small invertebrates growing under the same conditions (Elser et al., 2003, 2006; Gillooly et al., 2005; see review in Hessen et al., 2013). However, the GRH was formulated specifically for differences in invertebrate consumer growth under P limitation and at constant temperature (Elser et al., 2000a; Lukas et al., 2011; Hessen et al., 2013) and has not been well-tested beyond these conditions. Ectotherms generally achieve higher realized growth rates at warmer temperatures (Atkinson, 1994); thus, their somatic %RNA and %P might be expected to increase as well (Cross et al., 2015). However, ectotherms also frequently exhibit countergradient variation in which maximum growth rate (i.e., growth capacity) is highest in organisms from cooler environments (Conover and Schultz, 1995; Arendt, 1997). Further, rapid growth at warmer temperatures is generally accomplished by more rapid protein synthesis per ribosome rather than increased ribosomal investment (Farewell and Neidhardt, 1998). As a result, %P should not increase with growth rate over a natural gradient in temperature. Supporting this hypothesis, RNA content of many organisms is actually lower when acclimated to warmer temperatures (Woods et al., 2003); thus, temperature-induced growth rate variation may not be useful in predicting consumer P demands under the growth rate hypothesis.
Changing thermal regimes could alter organismal %P through mechanisms other than the linkage between RNA and growth rate. Differences in temperature could alter P investment into other types of tissues, which may decouple growth rate and %P but could still lead to differences in somatic P storage (Sardans et al., 2012; Cross et al., 2015). This is particularly true in vertebrates, whose skeletal development involves a high P demand (Hendrixson et al., 2007). For this reason, the GRH was not expected to apply to vertebrates, a hypothesis supported by empirical tests with developing frogs (Sterner and Elser, 2002; Liess et al., 2013). However, tadpoles reared at warmer temperatures still had higher %P at metamorphosis, suggesting that developmental temperature could affect skeletal ossification (Liess et al., 2013). On the other hand, more rapidly growing fish exhibit reduced skeletal ossification (Arendt and Wilson, 2000), suggesting that warmer temperatures could reduce somatic %P in vertebrates. Elucidating these effects is important as alterations to the thermal regimes of aquatic ecosystems could lead to shifts in P cycling through the consumer-mediated storage and transport of this often limiting nutrient.
Animal consumers can play an important role in ecosystem P dynamics through the storage, transport, and recycling of P and other biologically important nutrients (Vanni et al., 2013). Much empirical work on this topic has been conducted in tropical streams, where grazers can substantially affect aquatic ecosystems through their feeding, recycling, and storage of nutrients (e.g., Taylor et al., 2006; McIntyre et al., 2008; Capps and Flecker, 2013). However, these consumer-mediated impacts are sensitive to changes in the biological community (Capps et al., 2015a). Throughout the neotropics, for example, the species richness and biomass of stream-dwelling amphibians have been drastically reduced by disease-driven amphibian declines, particularly in cooler highland streams (Lips, 1999). As a result, the dominant grazers in these affected streams are now aquatic insects, such as the immature stages of mayflies (Colón-Gaud et al., 2010; Rantala et al., 2015). This community shift has altered ecosystem function in affected streams (Connelly et al., 2008; Rugenski et al., 2012; Whiles et al., 2013), and can also affect riparian ecosystems through the movement of adults of both taxa to the terrestrial environment (Vanni et al., 2013). As a result, differential growth and P sequestration between aquatic insects and tadpoles in response to thermal variation could further alter the trajectory of stream and riparian ecosystems in the tropics affected by amphibian decline.
Here we investigate the relationships of consumer somatic %P with growth rate of grazing mayflies and development rate of grazing tadpoles across a thermal gradient in Panamá to test whether the growth rate hypothesis applies to temperature-induced growth rate differences. We conducted a survey of periphyton and consumer stoichiometry across a natural thermal gradient in combination with controlled temperature manipulation experiments. We predicted that organismal %P would not increase with growth rate over a thermal gradient based on the biochemical mechanisms by which more rapid growth is achieved at warmer temperatures. We especially did not expect this relationship in tadpoles, whose developing bones represent a substantial P reservoir. This work expands upon our understanding of the applicability of the growth rate hypothesis and sheds light on the ways in which temperature-induced growth rate differences may violate its assumptions.
Methods
Study Sites
We conducted this study in six streams in Panamá ranging in elevation from 52 to 1,156 m and in mean temperature from approximately 18–25°C (Figure 1, Table 1, Table S1). The three lowland streams were located in Parque Nacional Soberanía near Gamboa (Mendoza, Macho, and Frijolito), while the three higher elevation streams were located in Parque Nacional Omar Torrijos (Guabal), Bosque Protector Palo Seco (Castillo), and Reserva Forestal Fortuna (Chorro). We measured temperature and light incidence hourly during the dry season of 2014 (February-May) at all sites using HOBO Pendant loggers (Onset Computer Corporation; Bourne, MA). Organisms from two streams, Río Frijolito and Quebrada Chorro, were studied more extensively through in situ growth measurements and temperature manipulation experiments.
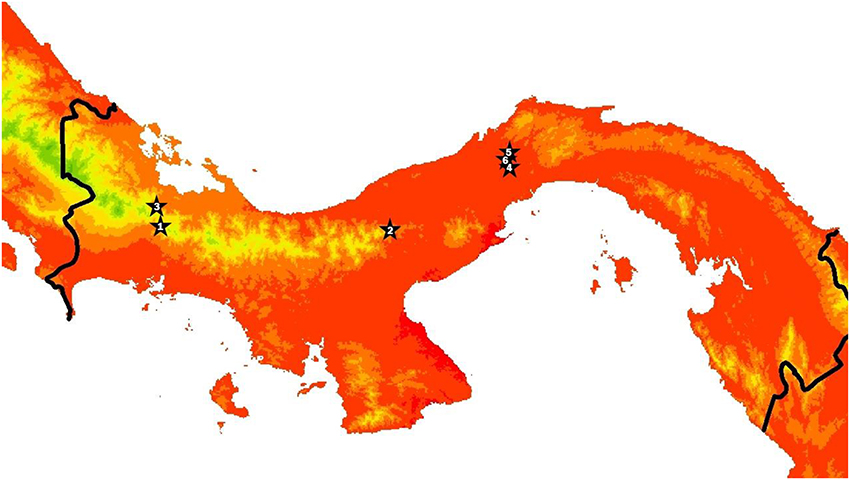
Figure 1. Map of Panamá with stars indicating the locations of the six study streams. The color gradient shows mean annual air temperature from WORLDCLIM at 30 arcsecond resolution, with green to red indicating colder to warmer. Sites are ranked from coldest to warmest and correspond to site numbers in Tables 1, 3. Site 1 corresponds to where organisms were collected for Fortuna and Site 4 corresponds to where organisms were collected for Soberanía temperature manipulation experiments.
Natural Thermal Gradient
At least six (range: 6–14) Thraulodes mayflies were collected from each stream by a combination of kick sampling with a D-frame net and hand searching on large rocks in riffles. The organisms were transported on ice and then frozen prior to determination of body %P. To examine whether variation in consumer %P could be driven by variation in food quality, we also measured carbon (C), nitrogen (N), and P concentrations of periphyton growing on rocks from all streams. Periphyton was collected from five replicate Loeb samples in both riffles and pools of each stream, and values were then averaged using methods described in Connelly et al. (2008) and Whiles et al. (2013).
We then measured in situ growth rates of Thraulodes using clear acrylic cylindrical chambers with fine mesh (500 μm) covering each end (described in Rugenski et al., 2012) in the two streams that provided the source populations for our experiments described below, Río Frijolito in Parque Nacional Soberanía and Quebrada Chorro in Reserva Forestal Fortuna. Mayflies were collected by hand by turning over rocks from stream riffles and then were placed into chambers containing four or five similar-sized individuals each with periphyton-covered rocks from the stream. We deployed five chambers per stream. Chambers were left in the stream for 4 days, after which mayflies were collected and re-measured. The total body length excluding cerci was measured to the nearest 0.1 mm from photographs taken immediately before and after deploying and retrieving the chambers using imageJ. Photographs were taken with a digital camera (Olympus TG-620) mounted above a petri dish on a flat surface. We then converted these values to dry mass using a length-mass regression for Leptophlebiidae (Benke et al., 1999). From these values we calculated specific growth rates (SGR) per day using the formula:
Final sample size varied between sites based on number of mayflies recovered. We recovered approximately 60% of the mayflies initially placed into chambers at each site, but we excluded two chambers from the Frijolito site from further analysis because their corresponding labels were lost upon recovery.
Temperature Manipulation
We conducted temperature manipulation experiments at two sites with both Thraulodes and bufonid tadpoles in the genus Rhinella from the lowland Río Frijolito and the highland Quebrada Chorro. We collected organisms from each stream with hand dip nets and transported them in buckets of stream water via automobile to coolers filled with 45 L of aged tap water (Frijolito) or aged stream water (Chorro). Water was aged by filling coolers at least 24 h before adding organisms. For Frijolito experiments, coolers were housed in a climate-controlled room in Gamboa (ambient air temperatures were too high to maintain cooler temperatures in pilot trials) with a 12:12 light:dark cycle and on a covered outdoor patio at the Jorge L. Araúz research station for Chorro experiments (Figure 2). Both locations were located approximately 20 min driving from the source streams. Light recordings inside coolers were also somewhat higher than those recorded in-stream, where zero light was recorded for 13–14 h per day depending on weather.
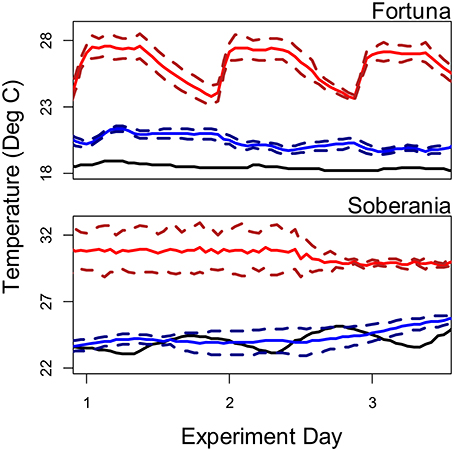
Figure 2. Temperature regimes in source streams (black lines), ambient treatments (blue lines), and heated treatments (red lines) during the course of temperature manipulation experiments. For experimental treatments, solid lines represent average temperatures and dashed lines represent ± one standard deviation.
We kept coolers either at ambient water temperature or heated them using 100 watt aquarium heaters (Eheim; Deizisau, Germany) to increase mean temperature by approximately 5°C for 3 days. To prevent acute thermal stress, temperature was slowly increased over the course of 12 h after adding organisms to heated treatments. We employed recirculating aquarium pumps (Technological Aquatic Associated Manufacturing; Taiwan) to homogenously heat the water and simulate stream flow in both ambient and heated coolers. Heaters were unplugged several hours after dark to mimic the slow overnight cooling of water experienced by the ambient treatments and the natural streams and then restarted in the morning after sunrise while pumps ran continuously throughout the experiments (Figure 2). The magnitude of the temperature manipulation was chosen based on the average sensitivity of stream temperature to changes in air temperature of 0.51/°C (Luce et al., 2014) and the range of predicted mean temperatures for Panamá under the A2 climate change scenarios (28–31°C, ensemble low and high, bottom and top 10% of all model predictions respectively, 2080–2099, World Bank Climate Change Knowledge Portal). Therefore, the experiment represents a short-term exposure to thermal regimes that these organisms may face for longer durations in the next century. We monitored water temperatures in the coolers using HOBO pendant loggers as above.
We placed five organisms in each cooler, conducting all treatments for a given species and site simultaneously. Organisms were fed periphyton ad libitum using either pre-colonized unglazed ceramic tiles from the stream of collection (Soberanía) or periphyton-covered rocks from the stream of collection (Fortuna). We replaced these with fresh tiles or rocks before starting with a new species and if periphyton appeared to have been substantially consumed during an experiment so that organisms could feed ad libitum and so the elemental composition of periphyton would not differ substantially from that in-stream. After 3 days, we measured change in body length as above, and for tadpoles, Gosner developmental stage (Gosner, 1960). The Gosner developmental stage is based on the presence and developmental extent of limb buds, limbs, and toes, which we assessed visually under a dissecting scope after euthanizing tadpoles. We held all organisms without food for approximately 1 h to minimize the effect of food content on body %P to approximate the same methods used in field collections. We then euthanized organisms using an overdose of MS-222 and preserved them for determination of %P as described above. Half of the organisms were preserved for P analysis, while half were preserved for transcriptomic analysis (not included in this manuscript). Recovery rates were somewhat lower than field growth chambers. We recovered approximately 35% of mayflies and 60% of tadpoles at the Soberanía site and 50% of mayflies and 90% of tadpoles at the Fortuna site. Our observations indicated that both mortality and emergence of adults contributed to our failure to recover all organisms. Most observed mortalities occurred within 12 h of transport to coolers, thus we believe these were likely due to handling stress rather than unfavorable conditions within coolers.
Laboratory Analyses
To measure body %P, tissue was dried to a constant weight at 50°C. For mayflies and periphyton collected from streams, we digested homogenized tissues in 1N hydrochloric acid and then used inductively coupled plasma mass spectrometry to measure %P (Thermo iCAP 6300; ThermoFisher Scientific, Waltham, MA). Carbon and N concentrations of periphyton were measured using a CHN analyzer (Thermo Flash 2000 CHNS/O Elemental Analyzer; CE Elantech, New Brunswick, NJ). Body percent phosphorus of experimental organisms was measured using a similar protocol, but we instead used the colorimetric acid molybdate method following acid digestion (APHA, 2005).
Statistical Analysis
We used Spearman's rank correlation tests to test for differences in periphyton stoichiometry with temperature among streams. We then employed ANCOVA with body length as a covariate to test how mayfly body %P varied with stream temperature. As specific growth rate is age- and size-dependent, we also used ANCOVA with body length as a covariate to test whether it varied in mayflies grown in chambers between the high-elevation cool stream and the low-elevation warm stream. For the temperature manipulation experiment, we tested whether consumer %P varied among taxa, temperatures, sites, and the interaction of the latter two using ANOVA. We used separate ANOVAs for tadpoles and mayflies to test whether growth or development rates varied with temperature, site, and/or their interaction as the growth metrics were not directly comparable between the two genera. Although the metric for tadpoles is a measure more of development rate than growth rate, we could not reliably measure specific growth rate in developing tadpoles as they lost size and mass at later Gosner stages. As faster rates of development also necessitate faster rates of protein synthesis, we believe this metric should produce results consistent with growth rate for the responses of interest. We also tested for a correlation between final Gosner stage and tadpole %P to test whether ontogenetic variation could explain variation in %P. Finally, we tested for significance of correlations between consumer %P and specific growth rate (in mayflies) or development rate (in tadpoles) by testing whether Pearson's correlation coefficients differed from zero using t-tests. For all analyses, we visually assessed normality and heterogeneity of variance of model residuals using normal quantile and residuals vs. fitted value plots, respectively, and log-transformed data to meet assumptions as needed. All statistical analyses were performed using R version 3.2.2 (R Core Team, 2015).
Results
Natural Thermal Gradient
Mean water temperature during the dry season generally increased with decreasing elevation among the study sites, although the lowest site (Río Frijolito) was slightly cooler than the other two lowland sites (Table 1). The standard deviation of water temperature varied somewhat among streams but not systematically with elevation or the mean temperature (Table 1). Mean incident light also varied among streams but again there was no evident pattern related to temperature or elevation (Table 1).
Among the six study streams, Thraulodes body %P did not vary with body size [F(2, 42) = 0.98, p = 0.382] but increased with mean water temperature [F(1, 42) = 6.81, p = 0.013] (Figure 3). Periphyton %C, %N, and %P were all negatively correlated with water temperature (p < 0.05; Figure 4). Periphyton C:P was positively correlated with water temperature (r = 0.39, S = 17,951, p = 0.003), but periphyton C:N and N:P were not significantly correlated with water temperature (p > 0.05). In the in situ growth chambers, mean specific growth rate of mayflies decreased with initial body size [Size, F(1, 11) = 17.08, p = 0.002], but for a given size did not differ among sites [Site, F(1, 11) = 4.09, p = 0.068] (Figure 5). However, since mayflies were smaller on average in Río Frijolito, their average specific growth rate (0.059 mg*d−1) was higher than those in Quebrada Chorro (0.035 mg*d−1). We found no interactive effect of body size and stream site on specific growth rate [F(1, 11) = 0.03, p = 0.872].
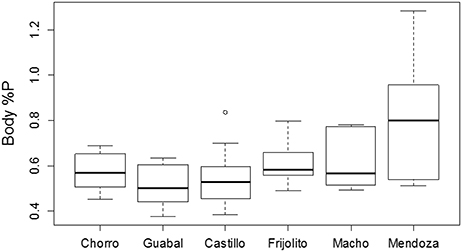
Figure 3. Body %P by dry mass of six populations of Thraulodes mayflies in Panamá. Streams are ordered from coolest to warmest. Ln-transformed body %P did not vary with mayfly size [F(2, 42) = 0.98, p = 0.382] but did increase with increasing average water temperature [F(1, 42) = 6.81, p = 0.013]. This plot shows untransformed data for easier biological interpretation. In the boxplot, the thick line represents the median, the edges of the boxes represent the upper and lower quartiles, and the whiskers represent 1.5 times the interquartile range above and below the upper and lower quartiles.
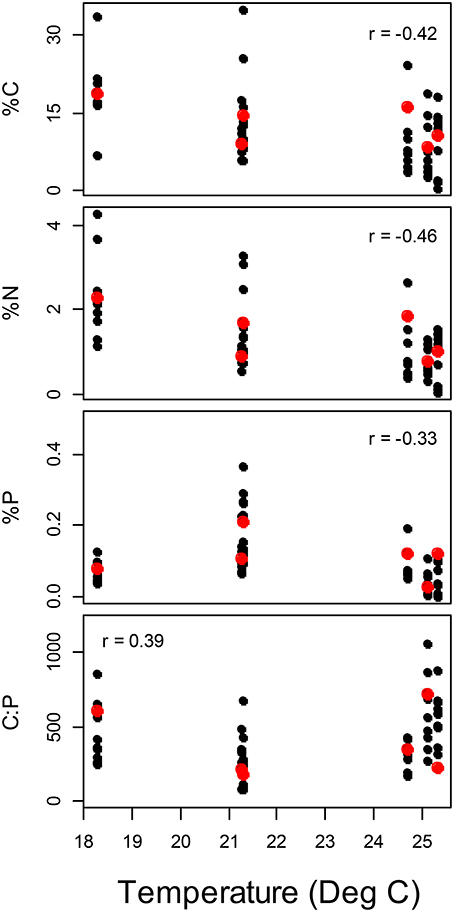
Figure 4. Variation in periphyton elemental concentrations with temperature among streams. Spearman rank correlation coefficients significantly different from zero are presented. Red points represent the average value for each stream.
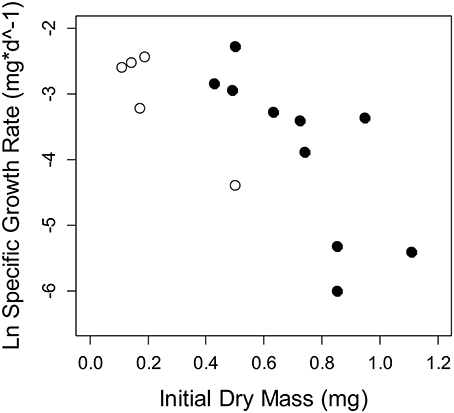
Figure 5. Specific growth rate of Thraulodes measured from in situ chambers at two sites. Points in white are the lowland stream, Frijolito, and points in black are the highland stream, Chorro. Each point is an individual mayfly. There was a significant effect of the covariate initial dry mass [F(1, 11) = 17.08, p = 0.002], but no site effect on specific growth rate after controlling for body size [F(1, 11) = 4.09, p = 0.068].
Temperature Manipulation
In mayflies, specific growth rate was higher at the warmer lowland site Frijolito [Site, F(1, 20) = 8.93, p = 0.007) and in the warmer temperature treatment [Treatment, F(1, 20) = 8.56, p = 0.008]. Tadpoles used in the experiments were initially at later Gosner developmental stages at the lowland Frijolito site, and thus ultimately reached later Gosner stages by the end of the experiment (Table 2). In tadpoles, development rate was also higher at the lowland Frijolito site [Site, F(1, 35) = 63.21, p < 0.001] as well as in the warmed treatments [Treatment, F(1, 35) = 35.84, p < 0.001], and there was also a significant interaction effect of site and temperature [Interaction, F(1, 35) = 39.73, p < 0.001] such that tadpoles developed more rapidly in warmer water at Frijolito, but developmental rate did not vary with temperature at Chorro.
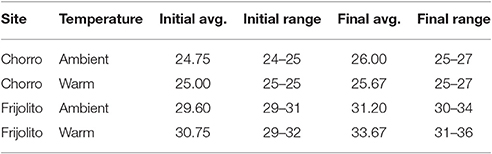
Table 2. Gosner developmental stages of Rhinella tadpoles used in the temperature manipulation experiments.
Body %P was not significantly correlated with final Gosner stage in tadpoles among sites and treatments (r = 0.40, t13 = 1.59, p = 0.137). Similar to specific growth rate, body %P was higher at the lowland Frijolito site in both taxa [Site, F(1, 25) = 20.83, p < 0.001]. In contrast, body %P did not vary with temperature manipulation [Treatment, F(1, 25) = 2.72, p = 0.112] nor did it vary between Thraulodes and Rhinella [Species, F(1, 25) = 0.01, p = 0.904; Figure 6]. Body %P was not significantly correlated with ln-transformed development rate in tadpoles (r = 0.32, t13 = 1.40, p = 0.238) or ln-transformed specific growth rate in mayflies (r = 0.42, t10 = 1.47, p = 0.173; Figure 7).
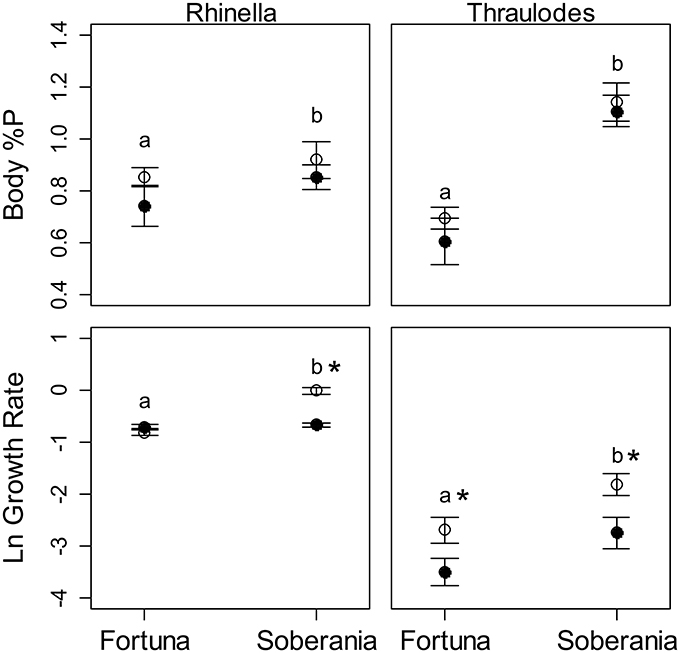
Figure 6. Average body %P by dry mass and growth rate of Rhinella (left panels) and Thraulodes (right panels). Growth of Rhinella is represented as Δ Gosner stage * d−1, and for Thraulodes as specific growth rate based on body mass (mg * d−1). White points are heated temperature treatments and black points are ambient temperature treatments. Error bars are ± one standard error. Letters above points represent significant differences in the response variable among sites and asterisks next to letters represent significant effects of temperature treatment at that site.
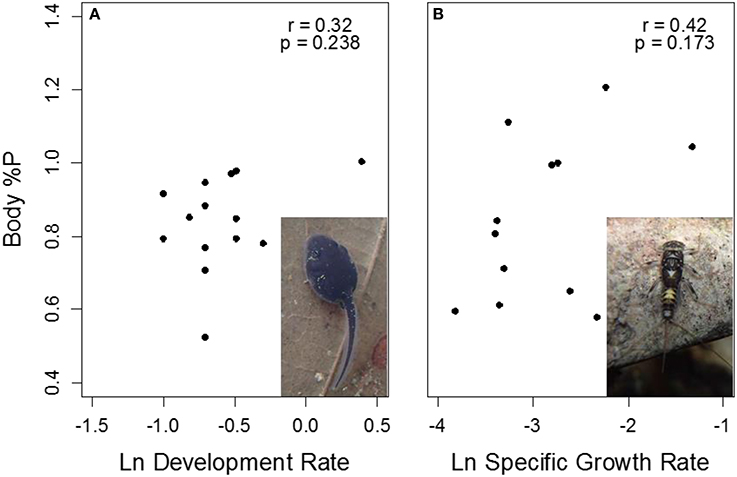
Figure 7. Correlations of body %P with growth rate in Rhinella (A) and Thraulodes (B) from temperature manipulation experiments. For Rhinella, growth is represented as Δ Gosner stage * day−1, while in Thraulodes it is represented as specific growth rate based on body mass. Neither correlation coefficients were significantly different from zero (p > 0.05).
Discussion
Variation in the somatic %P of invertebrates is well-explained by variation in rRNA production needed for protein synthesis to achieve somatic growth under the growth rate hypothesis (GRH), but the limits to its applicability, particularly in regards to developing vertebrates, have not been fully tested. In this study we sought to test the GRH in vertebrate and invertebrate benthic grazers over a thermal gradient in Panamá. Although our results meet predictions of the growth rate hypothesis, they do not support its application in explaining somatic %P variation with temperature. Across the six streams, average %P of Thraulodes increased with average water temperature. Since average SGR of Thraulodes were also higher in the warmer lowland Río Frijolito than the cooler highland Quebrada Chorro, these data conform to the predicted relationship between growth rate and somatic %P under the GRH. However, body %P did not increase with temperature in the manipulative experiment even though growth and developmental rates did in most cases. In fact, both body %P and growth/developmental rates were higher in the lowland Frijolito experiments regardless of temperature treatment (Figure 6). Our results suggest, at least in Thraulodes, body %P increases with growth rate among sites but thermal effects on growth rate did not drive this effect. This result supports the assumption of the GRH that it does not apply to growth rate variation driven by varying temperatures. Instead, we suggest that other factors drive variation in growth rate and body %P among sites.
The GRH was formulated with an assumption that organisms were growing at the same temperature (Elser et al., 1996, 2000a). We know from previously published work examining temperature-induced growth variation that it might not lead to variation in somatic %P if individual ribosomes synthesize proteins more rapidly, requiring a lower amount of ribosomal RNA to support rapid protein synthesis at higher temperatures (Farewell and Neidhardt, 1998; Gillooly et al., 2005). In fact, eukaryotic RNA content tends to decrease in warm-acclimated organisms (Woods et al., 2003). Therefore, in taxa for which RNA is the primary pool of somatic P, including the mayflies and early-stage tadpoles we studied, it is unlikely that increasing growth rates with warming temperatures will lead to higher somatic %P. If grazer P requirements do not change with warming temperatures, this will buffer them from potential P-limitation due to increased algal C:P with warming (Yvon-Durocher et al., 2010), a phenomenon we also observed with increasing temperature among streams in our study (Figure 4). Further, the storage of P by consumers can represent a substantial P sink in aquatic ecosystems, particularly when those consumers have biphasic life cycles and migrate to terrestrial ecosystems as adults (Vanni et al., 2013; Tiegs et al., 2016); thus, this finding has implications for ecosystem-scale fluxes of P in these streams. However, growth rate and %P of these grazers did vary among streams both in field collections and in our experiment; thus, it is important to discuss what may have driven this variation.
While it may be clear that temperature-driven effects did not affect the somatic %P of the grazers we studied, it is less clear why body %P and growth rate of both taxa varied among sites. As site-specific differences in %P were consistent in the temperature manipulation experiment in both Thraulodes and Rhinella, they may reflect some other important difference between lowland (<200 m) and highland (>500 m) streams (Figure 3). For example, the amphibian pathogen Batrachochytridium dendrobatidis is present across this entire elevational gradient, but has only led to catastrophic amphibian declines in highland streams (La Marca et al., 2005; Kilburn et al., 2010). If insect grazer densities increase in highland streams following amphibian declines, more intense intraspecific competition could lead to reduced individual growth rates. However, after reductions in tadpole densities following declines in one of our study streams, Río Guabal, densities of Thraulodes and other grazing invertebrate taxa did not significantly change (Colón-Gaud et al., 2010). Since Thraulodes should have less competition for algal resources in post-decline streams, we believe this would lead to increased growth rates if it affected them at all. Differences in the severity of amphibian decline do not effectively explain Thraulodes growth differences among sites, but other differences, such as disturbance frequency, resource quality, and predation pressure among streams may provide more insights.
The presence or absence of fishes was a defining characteristic between the upland and lowland streams in this study. The lowland streams in our study host diverse insectivorous fish communities including the aquatic insect-feeding genera Astyanax, Brycon, Andinoacara, Geophagus, Pimelodella, Trichomycterus, and Gobiomorus (Kramer and Bryant, 1995), while the upland streams either lack fish (Chorro and Castillo) or host only the primarily terrestrial insectivore Brachyrhaphis (Guabal) (Colón-Gaud et al., 2010). While all streams host predatory invertebrates such as pseudothelphusid crabs, dragonflies, and dobsonflies (Colón-Gaud et al., 2010; Múrria et al., 2015), fish and invertebrate predators can often invoke contrasting responses in prey due to differences in predator feeding strategies (Peckarsky and McIntosh, 1998; Touchon and Warkentin, 2008). Many fishes are visual predators and feed more actively during daylight hours while many of the invertebrate predators such as crabs and odonates are nocturnally active and rely more heavily on olfactory and tactile cues (Flecker, 1992; Peckarsky and McIntosh, 1998; Maitland, 2003). Unfortunately, which predators have greater effects on prey fitness is highly prey species-specific (e.g., Touchon and Vonesh, 2016), and we have insufficient data to infer whether fish predators have stronger fitness costs than invertebrate predators in Thraulodes and Rhinella.
Elevated predation pressure selects for more rapid growth and development to maturity in many organisms including mayflies and amphibians (Reznick and Endler, 1982; Peckarsky et al., 2001; Vonesh and Warkentin, 2006), so variation in predation pressure could explain higher growth rate and %P of lowland taxa if fish predators induce stronger selection for rapid growth. Selection for rapid growth and development to maturity in Arctic Daphnia populations developing under short growing seasons explained higher %P in those populations relative to temperate populations when reared at the same temperature (Elser et al., 2000b), and a similar scenario induced by predation risk may have occurred in our study. Although predation pressure is not a strong predictor of variation in body stoichiometry in Trinidadian guppies (El-Sabaawi et al., 2012), its effects on somatic %P via growth rate may be stronger in invertebrates and tadpoles. The latter develops P-rich bones only at later Gosner stages. As the streams we studied host genetically isolated populations of Thraulodes (Múrria et al., 2015), these effects could represent a potential evolutionary tradeoff in response to exposure to fish predators and merit further study.
Another possibility for the elevated %P of consumers at the lowland Frijolito site is that it is an artifact of our experimental design. Unfortunately, some methodological differences did occur among sites due to the remote location of the highland streams. In particular, our experiments at the highland Chorro site were kept on an open patio as opposed to in a climate-controlled room, which allowed temperatures to vary more than at the lowland Frijolito site (Figure 2). Thermal fluctuations often lead to more rapid development in ectotherms (e.g., Czarnoleski et al., 2013), yet we observed lower growth and developmental rates in organisms from Chorro. As a result, we do not believe this strongly affected our results. A further difference is that tadpoles at the lowland Frijolito site developed more rapidly and reached later stages by the end of our study (Table 2; Figure 6); thus, ontogenetic variation could explain variation in %P (Main et al., 1997; Pilati and Vanni, 2007). This is particularly true of developing Anurans, which exhibit extreme ontogenetic changes in %P as bone ossification begins at later Gosner stages (Tiegs et al., 2016). In the related European toad (Bufo bufo), tadpole bones begin ossification at Gosner stage 38 (Dunlap and Sanchiz, 1996). Some tadpoles at the lowland Frijolito site reached Gosner stage 38 by the end of the experiment, but we only included %P values for tadpoles at stages <38 in our analysis. The stage at which Rhinella alata or R. marina begin ossification is not known, but the fact that %P was not significantly correlated with final Gosner stage indicates that minimal ossification may occur before stage 38 in these species. Even if ontogenetic variation did not strongly influence our results, however, these changes are likely to affect the %P of developing tadpoles in natural ecosystems.
While our results provide insight into stoichiometric theory, they also have implications for Neotropical stream ecosystems facing disease-driven amphibian declines and future climatic change. The effects of the loss of amphibian communities on aquatic ecosystem functions have been well-studied (Connelly et al., 2008; Rugenski et al., 2012; Whiles et al., 2013; Rantala et al., 2015), but both amphibians and many aquatic insects have terrestrial adult stages. The movement of organisms from aquatic ecosystems to the terrestrial environment can represent a significant nutrient sink to aquatic ecosystems and an important subsidy to their surrounding terrestrial counterparts (Sabo and Power, 2002; Vanni et al., 2013; Capps et al., 2015b). Variation in %P among populations of Rhinella and Thraulodes can lead to variation in P export to the surrounding terrestrial ecosystem. However, the biphasic life cycles of these organisms could lead to dramatic changes in the %P of adults (Tiegs et al., 2016), which we did not consider in this study. Rhinella metamorphs in particular may exhibit strong differences in %P from pre-ossification tadpoles if bone density is unrelated to tadpole growth rates. Further, since the total consumer biomass remains significantly lower in post-decline streams (Whiles et al., 2013), this variation is unlikely to offset the P fluxes lost to amphibian extirpations.
One final caveat is that dietary stoichiometry of the study organisms likely varied among sites following variation in periphyton elemental concentrations (Table 3, Figure 4). The GRH is only successful at predicting somatic %P variation under P-limited consumer growth, because consumers can store P in tissues aside from ribosomal RNA when excess P is available (Hessen et al., 2013). This is particularly true of some vertebrates, in which bone tissues may serve as a flexible pool of body P (Benstead et al., 2014). Although periphyton C:P generally increased with water temperature (Figure 4), the highland Chorro site also had a relatively high average periphyton C:P. As a result, the particularly high C:P ratio of periphyton in the highland Chorro experiments relative to that in the lowland Frijolito experiments could explain why somatic %P of both taxa was not significantly correlated with growth rate (Figure 7) if consumer growth were limited by P in Chorro experiments and C in Frijolito experiments. A more detailed investigation of how threshold elemental ratios (TERs; Sterner and Hessen, 1994) vary with temperature is required to examine this mechanism. Variation in ontogeny and resource stoichiometry contributed to variation in consumer %P among sites in this study; thus, a carefully controlled study examining the effects of reduced competition for resources and predation pressure on the %P of these grazers is needed to understand the mechanisms behind the patterns we observed.
Although global climate change is expected to have a number of effects on stream ecosystems (Cross et al., 2015), our results do not provide any evidence that warming temperatures will alter nutrient storage and export by individual consumers. Instead, a mechanistic understanding of the drivers of variation in these stoichiometric traits is needed to predict how they will respond over environmental gradients. We suggest that more work investigating the drivers of growth rate variation and the relationship between juvenile growth and adult elemental composition in these taxa is needed to elucidate how consumers with biphasic life cycles such as these will alter nutrient dynamics in changing ecosystems.
Ethics Statement
This study was carried out in accordance with the recommendations of the Smithsonian Tropical Research Institute Institutional Animal Care and Use Committee. The protocol was approved by the Smithsonian Tropical Research Institute Institutional Animal Care and Use Committee (Protocol 2013-1201-2016).
Author Contributions
Conceived and designed the study: EM, AR, JS, BT, JE. Performed the study: EM, AR, BT. Analyzed the data: EM, AR. Wrote the paper: EM, AR, JS, BT, JE.
Conflict of Interest Statement
The authors declare that the research was conducted in the absence of any commercial or financial relationships that could be construed as a potential conflict of interest.
Acknowledgments
We thank the Smithsonian Tropical Research Institute and Arizona State University for funding this research and providing logistical support through a collaborative grant to AR, JE, JS, and BT and a fellowship to EM. We specifically thank Dayana Agudo, Carlos Espinosa, Luis Fernando de León, and Diana Sharpe for their assistance with logistics in Panamá. Michael Angilletta, Krista Capps, James Collins, and Ren Ze provided feedback on earlier versions of this manuscript. Scott Brovarney and Ashley Sanders helped with laboratory work. This work was conducted under ANAM scientific collection permit SC/A-2-14. Work with Rhinella was approved by the STRI animal care use committee (STRI IACUC protocol 2013-1201-2016).
Supplementary material
The Supplementary Material for this article can be found online at: http://journal.frontiersin.org/article/10.3389/fenvs.2017.00014/full#supplementary-material
References
APHA (2005). Standard Methods for the Examination of Water and Wastewater. Washington, DC: APHA-AWWA-WEF.
Arendt, J. D. (1997). Adaptive intrinsic growth rates: an integration across taxa. Q. Rev. Biol. 72, 149–177. doi: 10.1086/419764
Arendt, J., and Wilson, D. S. (2000). Population differences in the onset of cranial ossification in pumpkinseed (Lepomis gibbosus), a potential cost of rapid growth. Can. J. Fish. Aquat. Sci. 57, 351–356. doi: 10.1139/f99-250
Atkinson, D. (1994). Temperature and organism size – a biological law for ectotherms? Adv. Ecol. Res. 25, 1–58.
Benke, A. C., Huryn, A. D., Smock, L. A., and Wallace, J. B. (1999). Length-mass relationships for freshwater macroinvertebrates in North America with particular reference to the Southeastern United States. J. North Am. Benthol. Soc. 18, 308–343. doi: 10.2307/1468447
Benstead, J. P., Hood, J. M., Whelan, N. V., Kendrick, M. R., Nelson, D., Hanninen, A. F., et al. (2014). Coupling of dietary phosphorus and growth across diverse fish taxa: a meta-analysis of experimental aquaculture studies. Ecology 95, 2768–2777. doi: 10.1890/13-1859.1
Capps, K. A., Atkinson, C. L., and Rugenski, A. T. (2015a). Implications of species addition and decline for nutrient dynamics in fresh waters. Freshw. Sci. 34, 485–496. doi: 10.1086/681095
Capps, K. A., Berven, K. A., and Tiegs, S. D. (2015b). Modelling nutrient transport and transformation by pool-breeding amphibians in forested landscapes using a 21-year dataset. Freshw. Biol. 60, 500–511. doi: 10.1111/fwb.12470
Capps, K. A., and Flecker, A. S. (2013). Invasive aquarium fish transform ecosystem nutrient dynamics. Proc. R. Soc. Lond. B 280:20131520. doi: 10.1098/rspb.2013.1520
Colón-Gaud, C., Whiles, M. R., Brenes, R., Kilham, S. S., Lips, K. R., Pringle, C. M., et al. (2010). Potential functional redundancy and resource facilitation between tadpoles and insect grazers in tropical headwater streams. Freshw. Biol. 55, 2077–2088. doi: 10.1111/j.1365-2427.2010.02464.x
Connelly, S., Pringle, C. M., Bixby, R. J., Brenes, R., Whiles, M. R., Lips, K. R., et al. (2008). Changes in stream primary producer communities resulting from large-scale catastrophic amphibian declines: can small-scale experiments predict effects of tadpole loss? Ecosystems 11, 1262–1276. doi: 10.1007/s10021-008-9191-7
Conover, D. O., and Schultz, E. T. (1995). Phenotypic similarity and the evolutionary significance of countergradient variation. Trends Ecol. Evol. 10, 248–252. doi: 10.1016/S0169-5347(00)89081-3
Cross, W. F., Hood, J. M., Benstead, J. P., Huryn, A. D., and Nelson, D. (2015). Interactions between temperature and nutrients across levels of ecological organization. Glob. Chang. Biol. 21, 1025–1040. doi: 10.1111/gcb.12809
Czarnoleski, M., Cooper, B. S., Kierat, J., and Angilletta, M. J. (2013). Flies developed small bodies and small cells in warm and thermally fluctuating environments. J. Exp. Biol. 216, 2896–2901. doi: 10.1242/jeb.083535
Dmitriew, C. M. (2011). The evolution of growth trajectories: what limits growth rate? Biol. Rev. 86, 97–116. doi: 10.1111/j.1469-185X.2010.00136.x
Dunlap, K. D., and Sanchiz, B. (1996). Temporal dissociation between the development of the cranial and appendicular skeletons in Bufo bufo (Amphibia: Bufonidae). J. Herpetol. 30, 506–513. doi: 10.2307/1565693
El-Sabaawi, R. W., Zandonà, E., Kohler, T. J., Marshall, M. C., Moslemi, J. M., Travis, J., et al. (2012). Widespread intraspecific organismal stoichiometry among populations of the Trinidadian guppy. Funct. Ecol. 26, 666–676. doi: 10.1111/j.1365-2435.2012.01974.x
Elser, J. J., Acharya, K., Kyle, M., Cotner, J., Makino, W., Markow, T., et al. (2003). Growth rate-stoichiometry couplings in diverse biota. Ecol. Lett. 6, 936–943. doi: 10.1046/j.1461-0248.2003.00518.x
Elser, J. J., Dobberfuhl, D. R., MacKay, N. A., and Schampel, J. H. (1996). Organism size, life history, and N:P stoichiometry. Bioscience 46, 674–684. doi: 10.2307/1312897
Elser, J. J., O'Brien, W. J., Dobberfuhl, D. R., and Dowling, T. E. (2000b). The evolution of ecosystem processes: growth rate and elemental stoichiometry of a key herbivore in temperate and arctic habitats. J. Evol. Biol. 13, 845–853. doi: 10.1046/j.1420-9101.2000.00215.x
Elser, J. J., Sterner, R. W., Gorokhova, E., Fagan, W. F., Markow, T. A., Cotner, J. B., et al. (2000a). Biological stoichiometry from genes to ecosystems. Ecol. Lett. 3, 540–550. doi: 10.1046/j.1461-0248.2000.00185.x
Elser, J. J., Watts, T., Bitler, B., and Markow, T. A. (2006). Ontogenetic coupling of growth rate with RNA and P contents in five species of Drosophila. Funct. Ecol. 20, 846–856. doi: 10.1111/j.1365-2435.2006.01165.x
Farewell, A., and Neidhardt, F. C. (1998). Effect of temperature on in vivo protein synthetic capacity in Escherichia coli. J. Bacteriol. 180, 4704–4710.
Flecker, A. S. (1992). Fish predation and the evolution of invertebrate drift periodicity: evidence from Neotropical streams. Ecology 73, 438–448. doi: 10.2307/1940751
Gillooly, J. F., Allen, A. P., Brown, J. H., Elser, J. J., Martínez del Río, C., Savage, V. M., et al. (2005). The metabolic basis of whole-organism RNA and phosphorus content. Proc. Natl. Acad. Sci. U.S.A. 102, 11923–11927. doi: 10.1073/pnas.0504756102
Gosner, K. L. (1960). A simplified key for staging anuran embryos and larvae with notes on identification. Herpetologica 16, 183–190.
Hendrixson, H. A., Sterner, R. W., and Kay, A. D. (2007). Elemental stoichiometry of freshwater fishes in relation to phylogeny, allometry, and ecology. J. Fish Biol. 70, 121–140. doi: 10.1111/j.1095-8649.2006.01280.x
Hessen, D. O., Elser, J. J., Sterner, R. W., and Urabe, J. (2013). Ecological stoichiometry: an elemental approach using basic principles. Limnol. Oceanogr. 58, 2219–2236. doi: 10.4319/lo.2013.58.6.2219
Kilburn, V. L., Ibáñez, R., Sanjur, O., Bermingham, E., Suraci, J. P., and Green, D. M. (2010). Ubiquity of the pathogenic chytrid fungus, Batrachochytridium dendrobatidis, in Anuran communities in Panamá. Ecohealth 7, 537–548. doi: 10.1007/s10393-010-0634-1
Kramer, D. L., and Bryant, M. J. (1995). Intestine length in the fishes of a tropical stream: 2. Relationships to diet – the long and short of a convoluted issue. Environ. Biol. Fish. 42, 129–141. doi: 10.1007/BF00001991
La Marca, E., Lips, K. R., Lötters, S., Puschendorf, R., Ibáñez, R., Rueda-Almonacid, J. V., et al. (2005). Catastrophic population declines and extinctions in neotropical harlequin frogs (Bufonidae: Atelopus). Biotropica 37, 190–201. doi: 10.1111/j.1744-7429.2005.00026.x
Liess, A., Rowe, O., Guo, J. W., Thomsson, G., and Lind, M. I. (2013). Hot tadpoles from cold environments need more nutrients – life history and stoichiometry reflects latitudinal adaptation. J. Anim. Ecol. 82, 1316–1325. doi: 10.1111/1365-2656.12107
Lips, K. R. (1999). Mass mortality and population declines of Anurans at an upland site in Western Panama. Conserv. Biol. 13, 117–125. doi: 10.1046/j.1523-1739.1999.97185.x
Luce, C., Staab, B., Kramer, M., Wenger, S., Isaak, D., and McConnell, C. (2014). Sensitivity of summer stream temperatures to climate variability in the Pacific Northwest. Water Resour. Res. 50, 3428–3443. doi: 10.1002/2013WR014329
Lukas, M., Frost, P. C., and Wacker, A. (2011). The neonate nutrition hypothesis: early feeding affects the body stoichiometry of Daphnia offspring. Freshw. Biol. 58, 2333–2344. doi: 10.1111/fwb.12213
Main, T. M., Dobberfuhl, D. R., and Elser, J. J. (1997). N: P stoichiometry and ontogeny of crustacean zooplankton: a test of the growth rate hypothesis. Limnol. Oceanogr. 42, 1474–1478. doi: 10.4319/lo.1997.42.6.1474
Maitland, D. P. (2003). Predation on snakes by the freshwater land crab Eudaniela garmani. J. Crustacean Biol. 23, 241–246. doi: 10.1163/20021975-99990331
McIntyre, P. B., Flecker, A. S., Vanni, M. J., Hood, J. M., Taylor, B. W., and Thomas, S. A. (2008). Fish distributions and nutrient cycling in streams: can fish create biogeochemical hotspots? Ecology 89, 2335–2346. doi: 10.1890/07-1552.1
Múrria, C., Rugenski, A. T., Whiles, M. R., and Vogler, A. P. (2015). Long-term isolation and endemicity of Neotropical aquatic insects limit the community responses to recent amphibian decline. Divers. Distrib. 21, 938–949. doi: 10.1111/ddi.12343
Peckarsky, B. L., and McIntosh, A. R. (1998). Fitness and community consequences of avoiding multiple predators. Oecologia 113, 565–576. doi: 10.1007/s004420050410
Peckarsky, B. L., Taylor, B. W., McIntosh, A. R., McPeek, M. A., and Lytle, D. A. (2001). Variation in mayfly size at metamorphosis as a development response to risk of predation. Ecology 82, 740–757. doi: 10.1890/0012-9658(2001)082[0740:VIMSAM]2.0.CO;2
Pilati, A., and Vanni, M. J. (2007). Ontogeny, diet shifts, and nutrient stoichiometry in fish. Oikos 116, 1663–1674. doi: 10.1111/j.0030-1299.2007.15970.x
Rantala, H. M., Nelson, A. M., Fulgoni, J. N., Whiles, M. R., Hall, R. O., Dodds, W. K., et al. (2015). Long-term changes in structure and function of a tropical headwater stream following a disease-driven amphibian decline. Freshw. Biol. 60, 575–589. doi: 10.1111/fwb.12505
R Core Team (2015). R: A Language and Environment for Statistical Computing. Vienna: R Foundation for Statistical Computing. Available online at: http://www.R-project.org/
Reznick, D. N., and Endler, J. A. (1982). The impact of predation on life history evolution in Trinidadian guppies (Poecilia reticulata). Evolution 36, 160–177. doi: 10.2307/2407978
Rugenski, A. T., Múrria, C., and Whiles, M. R. (2012). Tadpoles enhance microbial activity and leaf decomposition in a neotropical headwater stream. Freshw. Biol. 57, 1904–1913. doi: 10.1111/j.1365-2427.2012.02853.x
Sabo, J. L., and Power, M. E. (2002). River-watershed exchange: effects of riverine subsidies on riparian lizards and their terrestrial prey. Ecology 83, 1860–1869. doi: 10.2307/3071770
Sardans, J., Rivas-Ubach, A., and Peñuelas, J. (2012). The elemental stoichiometry of aquatic and terrestrial ecosystems and its relationships with organismic lifestyle and ecosystem structure and function: a review and perspectives. Biogeochemistry 111, 1–39. doi: 10.1007/s10533-011-9640-9
Sterner, R. W., and Elser, J. J. (2002). Ecological Stoichiometry. Princeton, NJ: Princeton University Press.
Sterner, R. W., and Hessen, D. O. (1994). Algal nutrient limitation and the nutrition of aquatic herbivores. Annu. Rev. Ecol. Syst. 25, 1–29. doi: 10.1146/annurev.es.25.110194.000245
Taylor, B. W., Flecker, A. S., and Hall, R. O. (2006). Loss of a harvested fish species disrupts carbon flow in a diverse tropical river. Science 313, 833–836. doi: 10.1126/science.1128223
Tiegs, S. D., Berven, K. A., Carmack, D. J., and Capps, K. A. (2016). Stoichiometric implications of a biphasic life cycle. Oecologia 180, 853–863. doi: 10.1007/s00442-015-3504-2
Touchon, J. C., and Vonesh, J. R. (2016). Variation in abundance and efficacy of tadpole predators in a Neotropical pond community. J. Herpetol. 50, 113–119. doi: 10.1670/14-111
Touchon, J. C., and Warkentin, K. M. (2008). Fish and dragonfly nymph predators induce opposite shifts in color and morphology of tadpoles. Oikos 117, 634–640. doi: 10.1111/j.0030-1299.2008.16354.x
Vanni, M. J., Boros, G., and McIntyre, P. B. (2013). When are fish sources vs. sinks of nutrients in lake ecosystems? Ecology 94, 2195–2206. doi: 10.1890/12-1559.1
Violle, C., Navas, M.-L., Vile, D., Kazakou, E., Fortunel, C., Hummel, I., et al. (2007). Let the concept of trait be functional! Oikos 116, 882–892. doi: 10.1111/j.0030-1299.2007.15559.x
Vonesh, J. R., and Warkentin, K. M. (2006). Opposite shifts in size at metamorphosis in response to larval and metamorph predators. Ecology 87, 556–562. doi: 10.1890/05-0930
Whiles, M. R., Hall, R. O., Dodds, W. K., Verburg, P., Huryn, A. D., Pringle, C. M., et al. (2013). Disease-driven amphibian declines alter ecosystem processes in a tropical stream. Ecosystems 16, 146–157. doi: 10.1007/s10021-012-9602-7
Woods, H. A., Makino, W., Cotner, J. B., Hobbie, S. E., Harrison, J. F., Acharya, K., et al. (2003). Temperature and the chemical composition of poikilothermic organisms. Funct. Ecol. 17, 237–245. doi: 10.1046/j.1365-2435.2003.00724.x
Keywords: ecological stoichiometry, elemental phenotype, thermal gradient, Panamá, neotropical stream, leptophlebiidae
Citation: Moody EK, Rugenski AT, Sabo JL, Turner BL and Elser JJ (2017) Does the Growth Rate Hypothesis Apply across Temperatures? Variation in the Growth Rate and Body Phosphorus of Neotropical Benthic Grazers. Front. Environ. Sci. 5:14. doi: 10.3389/fenvs.2017.00014
Received: 28 January 2017; Accepted: 29 March 2017;
Published: 18 April 2017.
Edited by:
Sergi Sabater, University of Girona, SpainReviewed by:
Daniel von Schiller, University of the Basque Country, SpainClaudia Feijoó Feijoó, National University of Luján, Argentina
Copyright © 2017 Moody, Rugenski, Sabo, Turner and Elser. This is an open-access article distributed under the terms of the Creative Commons Attribution License (CC BY). The use, distribution or reproduction in other forums is permitted, provided the original author(s) or licensor are credited and that the original publication in this journal is cited, in accordance with accepted academic practice. No use, distribution or reproduction is permitted which does not comply with these terms.
*Correspondence: Eric K. Moody, ZXJpY2ttb29keUBnbWFpbC5jb20=