- 1Department of Animal Biology, School of Life Sciences, University of Hyderabad, Hyderabad, India
- 2School of Physics, University of Hyderabad, Hyderabad, India
Present study examined the impact of copper (Cu) as nanoparticle (Cu-NPs) and soluble form (CuSO4) in adult male catfish Clarias batrachus. Following treatment, upregulation of transcripts of several testis-related genes and increased androgen levels were evident. Though profound promotional effect was seen at gene and androgen levels, structural analysis revealed completely different scenario. Histological observations of testis showed disruption of basal lamina, distinct spermatogonium, and enlarged spermatocytes in the treated groups. Quite alarmingly, transmission electron microscopic analysis showed damage of testicular lumen with irregular basal lamina and distinctive spermatocytes. In addition, Sertoli cells and erythrocytes exhibited damage after Cu treatments. Effects of Cu treatment in catfish primary testis and TM3 Leydig cells showed cytotoxicity which is correlated well with morphological and flow cytometric analysis. Taken together, the results demonstrated that low dose of Cu/Cu-NPs can be detrimental to catfish testicular recrudescence vis-à-vis reproduction and the toxic effect manifest drastically in in vitro cultures.
Introduction
Living organisms require varying amount of certain metals which are essential for normal physiological functions. However, the same will become toxic and can cause adverse effects when exceeding the limit of exposure to organisms. Soluble heavy metals (aluminum, arsenic, cadmium, copper (Cu), iron, lead, manganese, etc.,) are part of the environmental pollutants being released into water sources from effluents. In fish, heavy metals gets accumulated in their body, and elicit toxic effects or even death (Authman et al., 2015). Simultaneously, due to biomagnification contaminated fish upon consumption will lead to serious health hazards in human (Fernandes et al., 2012). Cu is one of the major toxic heavy metal pollutants and have been widely used in alloys, coins, jewelry, and food pack container industries. It is sparingly soluble in water and binds to sediments and organic matters in and around the water bodies receiving industrial effluents. Cu contamination is seen in certain water reservoirs (Begum et al., 2009; Mane et al., 2013) where food fish such as catfish, Clarias batrachus, and common carp are wild caught or cultivated in India and certain other countries (IPCS, 1998; Damodharan, 2013; Şeker and Kutlu, 2014). According to the report submitted by World Health Organization on “Copper in drinking-water” revealed that Cu is found in complex or as particulate matter in various water bodies including drinking and sea water (ATSDR, 2002).
On the other hand, over the past decade, the usage of nanoparticles (NPs) has increased steadily in the field of biomedical sciences and engineering (Kaida et al., 2004; Wang et al., 2007) wherein metallic combination is often used in which Cu coupling seems economical. In recent years, some of the NPs have potential role in agriculture practices as it has antifungal and antimicrobial properties (Rai and Ingle, 2012). Despite several applications, NPs toxicity have been receiving much attention among researchers as it has unique property, large surface to volume ratio and highly reactive toward chemical and biological molecules (Wani et al., 2011). Intriguingly, advances in biosensor technology also focuses in detecting pesticides or nanomaterials in food and various industrial products for exercising safety (Vigneshvar et al., 2016). Several studies authenticated that NPs can cause sublethal effects in fish including reproductive and respiratory toxicity, damage to the tissues and oxidative stress (Moore, 2006; Krysanov et al., 2010; Shaw and Handy, 2011). In this context, uptake of Cu-NPs by the Nile tilapia resulted in biochemical alterations and oxidative stress (Abdel-Khalek et al., 2015). A report on different NPs exposed to zebrafish suggested that Cu and silver are more toxic to fish (Kovrižnych et al., 2013). However, Griffitt et al. (2007) demonstrated that Cu-NPs produced lethal effects on zebrafish gills when compared to soluble Cu. Zebrafish embryos exposed to different sized Cu-NPs suggested that the smaller were more toxic than the larger (Hua et al., 2014). In addition, NPs toxicity in relation to reproduction were also reported in mammals (Jia et al., 2014; Thakur et al., 2014), yet more detailed study will be more interesting if the toxicity is associated with environmental pollution.
In red seabream, exposure of Cu at early stages caused low hatching rate and growth retardation (Cao et al., 2010) while it reduced the gonadosomatic index, survival and offspring production rate in adult guppy fish (Moosavi and Shamushaki, 2015). Determination of LC50 value for copper sulfate (CuSO4) exposed to C. gariepinus (a closely related species of C. batrachus), showed a wide range between 37.47 and 44.58 ppm upon 96 h exposure (Wani et al., 2013). Studies on the Nile tilapia, the LC50 value for Cu-NPs was determined as 150 mg/L after 96 h exposure (Abdel-Khalek et al., 2015). Interestingly, several research reports validated the toxicity of different sized NPs in mammalian and piscine cell lines (Braydich-Stolle et al., 2005; Song et al., 2014). Several candidate genes and factors have been identified in teleosts that are playing essential role in gametogenesis and steroidogenesis, and interestingly most of them are interrelated to each other and also involved in gonad function (see Nagahama, 1994; Sudhakumari and Senthilkumaran, 2013). Environmental insults such as pesticides and heavy metals cause deleterious effects on fish even at minimal doses and finally affect or modulate the physiological processes which includes steroidogenesis and gametogenesis (Rajakumar et al., 2012; Authman et al., 2015; Senthilkumaran, 2015). Impact of certain heavy metals such as mercury (Kirubagaran and Joy, 1992), selenium (Lemly, 2002), and cadmium (Thompson and Bannigan, 2008) were reported in relation to reproduction using Indian fish models. On the other hand, recent studies on heavy metal toxicity with respect to genes and hormones involved in the gonadal development (Luo et al., 2015; Zhang et al., 2016) drawn reasonable attention indicating the serious concern about the question of fertility of fish and offspring survival against pollutants. Hence, comparative analysis on the levels of gonad-specific genes, hormones, and gonadal structure would be ideal to explore the impact of pollutants upon exposure.
The present study aimed to understand the effect of Cu-NPs in comparison with soluble metal form at very low concentration (100 μg/L or 0.1 ppm) using catfish, C. batrachus as an animal model as the siluriforms show high tolerance to environmental pollutants compared to other teleosts (Senthilkumaran, 2015). The dosage chosen in this study is very less compared to the LC50 value reported in a closely related species. Furthermore, the doses used are eco-relevant considering alarmingly high levels of Cu in certain rivers of India (Jain, 2004; Damodharan, 2013; Ghorade et al., 2014; Goswami and Sanjay, 2014). Quantification of mRNA transcripts of several steroidogenic enzyme genes involved in testosterone (T) and 11-ketotestosterone (11-KT) synthesis such as 3β-hydroxysteroid dehydrogenase (3β-hsd), cholesterol side-chain cleavage enzyme (cyp11a1), 11β-hydroxysteroid dehydrogenase 2 (11β-hsd2), 11β-hydroxylase (11β-h) and steroidogenic acute regulatory protein (star) and transcription factor genes such as doublesex and mab-3 related transcription factor 1 (dmrt1), SRY-related high mobility group box 9a (sox9a), Wilms' tumor suppressor 1 (wt1), steroidogenic factor 1 (ad4bp/sf-1) and GATA binding protein 4 (gata4) were chosen for analysis in this study as their involvement in testicular development/recrudescence is well demonstrated (Sudhakumari and Senthilkumaran, 2013). Further, serum T and 11-KT levels were estimated catfish to reveal the consequence. Histological and transmission electron microscopic (TEM) analysis were done in catfish testis for control and treated groups to substantially analyze the structural effects, if any. In addition, we analyzed the effects of Cu (both forms) for cytotoxicity using catfish primary testicular and TM3 Leydig cell cultures.
Materials and Methods
Preparation of Cu-NPs and Cu
Cu (II) oxide nanopowder purchased from Sigma, St. Louis, MO, USA (Catalog No. 544868) was used for the treatment. Based on the manufacturers' specifications, size of the nanopowder was determined as < 50 nm particle size by TEM analysis and the surface area was 29 m2/g. The purity of Cu nanopowder was specified as 99.5% based on the analysis report. Physiochemical properties of Cu-NPs such as pH and alkalinity were analyzed by dissolving the Cu-NPs in water before the treatment. For Cu-NPs stock preparation, 100 μg/ml of Cu-NPs was weighed and mixed in distilled water. The solution was sonicated up to 6 h and then used for the treatment. The effect of particles before and after sonication was observed through Field emission scanning electron microscopy (FESEM) and TEM. X-Ray Diffraction (XRD) and particle size distribution of Cu-NPs were also done. The percentage of Cu in CuSO4 was calculated and the equivalent concentration of Cu load was measured and chosen for the treatment.
Animals and Treatment
C. batrachus (L.) of similar age group and size (~120 g of 21 months old; 25 ± 3.5 cm) reared in the laboratory were taken for the experiment (n = 5). Each group (control, Cu-NPs-treated and CuSO4-treated) were acclimated in glass tank (one fish/tank) contains 50 L of water and maintained at ambient light and dark cycle (20 ± 2°C; 12 h L/12 h D). During treatment, fish were daily fed commercially available pelleted fish feed, ad libitum. Catfish were separated into three groups (n = 5) such as control, CuSO4 and Cu-NPs. Adult catfish (n = 5) were exposed to Cu-NPs (100 μg/L) and CuSO4 (equivalent conc. of Cu load) for 21 days. Treatment was given every day once after the replenishment of water was done. Since the replenishment of water was done with Cu in both forms every day, the exposure of these correlates was authenticated without any measurement of dissolved Cu. Further, the histology and TEM analysis in the results section demonstrate the desired exposure of Cu in any form. The dosage selected for the experimental study was eco-relevant and also less than LC50 value reported previously, which is why a single dose Cu-NPs treatment was done with equivalent amount of Cu separately. Further, the aim of this study is not for performing dose-dependency in in vivo study, however, the same was done in in vitro. Fish behavior and water temperature were monitored for all groups and no mortality was seen during the treatment period. After 21 days, blood was drawn from dorsal vein for androgen quantification while testes were dissected out for real-time PCR (qPCR), histology and TEM analysis. All the animal (fish) experiments was performed by following the general guidelines of the Institutional Animal Ethics Committee, University of Hyderabad.
Quantification of mRNA Transcripts in Control and Treated Groups
Quantification of certain mRNA transcripts in control and treated samples were done using qPCR by SYBR® Green detection method. Total RNAs were prepared from testis of control, CuSO4 and Cu-NPs treated batches using TRI-reagent® (Sigma) as per the protocol and quantified by a NanoDrop ND-1000 spectrophotometer (Thermo Fisher Scientific, Wilmington, DE, USA). First strand synthesis was carried out for 500 ng of total RNA extracted from testis of three groups using verso cDNA synthesis kit (Thermo Scientific Inc., Waltham, MA, USA) based on the protocol followed. PCR amplification was performed for all the samples using internal control, 18S rRNA to check the efficiency of the reaction. There is no significant difference between the expression level of 18S rRNA between control and treated testis and hence it was used as a reference gene for the analysis. All qPCR primers were designed with at least one primer should spanned the junction of two exons by giving a single PCR product where there is no possibility for genomic DNA amplification. All the genes analyzed in this study were previously cloned from catfish in our laboratory and the primer sequences were listed in Supplementary Table 1. The reaction was setup in triplicates for each sample using SYBR green master mix and done on a 7500 Fast Real-Time PCR system (Applied Biosystems, Foster City, CA, USA) according to the manufacturer's recommendations. Melting-curve analysis was performed to check the efficiency of PCR product amplification while zero amplification was observed in no template control. Cycle threshold (Ct) values were calculated from the exponential phase of PCR amplification and the target gene expression was normalized against the expression of 18S rRNA to generate a ΔCt value (Ct of target gene–Ct of endogenous control). Relative expression of the target gene was then calculated according to the equation 2−ΔΔCt.
Histological Analysis
Testis of control, CuSO4 and Cu-NPs treated adult catfish (n = 5) were dissected out and fixed in Bouin's fixative (15: 5: 1 of saturated picric acid: formaldehyde: glacial acetic acid). The tissues were dehydrated using graded ethanol series, followed by xylene and finally embedded in paraplast (Sigma). On a rotatory microtome (Leitz, Wetzlar, Germany), sections of about 5 μm thickness were cut, spread and dried in a hot plate (Yorko Sales Pvt. Ltd., New Delhi, India). The tissue slides were treated with xylene for deparaffinization and rehydrated using graded ethanol series. Then, the sections were stained with hematoxylin and eosin, followed by dehydration to mount using DPX mountant (SRL, Mumbai, India). All the microphotographs were taken using Olympus CX41 bright field light microscope attached with a Micropublisher (Model-MP3.3) cooled CCD camera (Q-imaging, BC, Canada).
TEM Analysis
For TEM analysis, control and treated (CuSO4 and Cu-NPs) testis (n = 5) were fixed in 2.5% glutaraldehyde in 0.1 M phosphate buffered (PB) with pH 7.2 for 24 h at 4°C. On following day, the samples were washed for 45 min each with PB-saline (PBS) thrice. Further, it is post-fixed in 1% aqueous osmium textroxide for 2 h and rinsed with deionized water for 6 times each 45 min, dehydrated in series of graded alcohol, infiltrated and embedded in araldite 6005 resin (Spurr, 1969). The samples were then incubated at 80°C for 72 h for complete polymerization. Ultra-thin sections of about 1 μm were made using a glass knife on Ultramicrotome (Leica Ultra cut UCT-GA-D/E-1/00), mounted on Cu grids and stained with saturated aqueous Urenyl acetate and counter stained with Reynolds' lead citrate. TEM microphotographs were obtained with a FEI Technai G2 S-Twin instrument at an acceleration voltage of 200 kV. All the TEM sample processing was carried out at RUSKA Labs, Department of Veterinary Pathology, Acharya N G Ranga Agricultural University, Hyderabad.
EIA for Serum T and 11-KT Levels
T and 11-KT levels in serum were estimated by EIA kit (Cayman, Ann Arbor, MI, USA) as per the manufacturer's protocol. Blood was drawn from dorsal vein of catfish (n = 5) using a disposable syringe to obtain serum by centrifugation at 5000 g for 10 min at 4°C. The supernatant (serum) was carefully pipetted out by leaving the blood pellet and stored in −80°C for hormone analysis. The sensitivity limit for T and 11-KT measurement is 6 and 1.3 pg/ml, respectively as per the kit procedure and the assay validation was done as per Swapna et al. (2006).
Catfish Primary Testicular Culture (Mixed) and TM3 Leydig Cell Culture (Mus musculus)
Catfish primary testicular cell culture (mixed) was prepared based on the protocol followed by Song and Gutzeit (2003). Adult male catfish was anesthetized using 100 mg/dl MS222 (Sigma) and disinfected with 70% ethanol. Testes were dissected out and washed with sterile PBS. Later, the testes were kept in L-15 medium and chopped into small pieces, and then wash again with sterile PBS for 5 times. The testicular pieces were then incubated with 0.25% collagenase type I and 0.005% DNase at room temperature for 15 min. The mixture was poured into a sterile petriplate and gently pressed with blunt end of syringe to get cell suspension. The suspension was filtered through a 40 μm cell strainer and collected in a centrifuge tube. After centrifugation by 100 g at 4°C, the pellet was resuspended in Dulbecco's modified Eagle's medium (DMEM) and washed three times using DMEM. The viability of cells was assessed using trypan blue staining and about 1 × 106 cells were plated in each well of 24 well culture plate containing DMEM, 10% FBS, 1 × Glutamax and antibiotic and antimycotic and incubated at 30°C in 5% CO2. The culture was maintained for 24 h and then used for experiment.
TM3 Leydig cells (Mus musculus) purchased from NCCS, Pune, India were used for the assay. The culture was maintained as per the method described above. For cytotoxicity studies, Cu-NPs and CuSO4 were dissolved at a final concentration of 10, 25, 50, and 75 μg/ml.
Mitochondrial Function Assay
Mitochondrial function was analyzed using the CellTiter 96® AQueous One Solution Cell Proliferation Assay (Promega, Alexandria NSW) based on the procedure followed by Braydich-Stolle et al. (2005). After 24 h maintenance, increasing concentration of CuSO4 and Cu-NPs were added to 96 well cell culture plate. The cultures were kept for 48 h with supplement of 5% CO2 at 30°C and further 20 μl of Aqueous One Solution reagent was directly added to the culture wells. After 3 h incubation, the color developed was directly measured at 490 nm using a standard microplate reader (Bio-Rad, Ireland). The absorbance measured at 490 nm is the quantity of formation of formazan product which is directly proportional to the number of live cells in culture. The experiment was repeated in triplicate, independently (n = 5). The relative cell viability (%) related to control wells was expressed in percentage relative to untreated cells.
Membrane Permeability/Dead Cell Apoptosis Assay
Determination of cellular apoptosis or necrosis level was done using Vybrant Apoptosis Assay kit #4 (Molecular Probes, Eugene, OR) as per the protocol described by manufacturer. The kit supplied with YO-PRO®-1/propidium iodide, green fluorescent YO-PRO®-1 binds specifically to apoptotic cells while red-fluorescent propidium iodide stains necrotic cells. After 48 h treatment with Cu, the cells were washed with PBS and incubated with 2 ml of PBS contains 2 μl of YO-PRO®-1 and propidium iodide and further incubated for 30 min in ice. Following incubation, the cells were analyzed using BD LSRFortessa™ cell analyzer (BD Biosciences, Franklin Lakes, NJ, USA).
Statistical Analysis
The results represented for qPCR and EIA were expressed as mean ± SEM (Standard Error of the Mean). All statistical analyses were done using SigmaPlot 11.0 software (Systat Software Inc., Chicago, USA). Data passed homogeneity and normality tests and compared by one-way analysis of variance (ANOVA) followed by Student-Newman-Keuls' post-hoc test. Statistical analysis was considered significant at the level of P < 0.05.
Results
Characterization of Cu-NPs before and after Sonication
Characterization of Cu-NPs were done through FESEM, TEM and XRD, and distribution of Cu-NPs were provided (Figures 1A–G). Before sonication, the Cu-NPs had large size to meet the desired properties, hence sonication was performed without interruption for longer period of time (6 h) to reach the desired size and shape of NPs for biological response. Before sonication, the FESEM image of Cu-NPs showed large particle size, ~40–60 nm and the size was not uniform. Agglomerated with particle to particle and in the background tiny particle with island structure was formed which is not enough to reach the goal (Figure 1A). Thus, the phase and spherical morphology formed during sonication at 6 h of time with the same speed and the particles were well controlled in size (15–20 nm), spherical shape and particle uniformity (Figure 1B). Sonication process led to the NP size, agglomeration reduction due to dispersion of coarse agglomeration. The key observation was that the 6 h sonication time is an optimum duration to initiate the controlled size, stability and refine the particle size. TEM images before and after sonication were taken (Figures 1C–E), the particle size reduction was also evident in TEM images. Thus, the toxicity or biological response was experimented using the sample after sonication. In addition, pH and alkalinity of the water sample was unchanged after the addition of Cu-NPs into 50 L water tank during the treatment. FESEM, TEM images are conforming the shape (spherical NP), average particle size of the Cu and conformed as 40–50 nm. X-ray diffraction is one of the important techniques to determine the size of the unit cell of material. X-ray diffraction pattern of the prepared Cu-NP, the sharp and broad crystalline peaks clearly conforming the Cu-NP nature (Figure 1F). The mean Cu-NP size was measured from the full width half maxima of the most intensive peak (111) of Cu. The 2θ values of 43.29, 50.42, 74.12, and 89.92 corresponding to (111), (200), (220), and (311) planes of the face centered cubic (fcc) crystalline cubic structure of metallic Cu. The intensities of all reflections are well matched with standard data (JCPDF File No. 04-0836) and conforming that Cu-NP has high purity and other impurities was not observed. The particle size of Cu NPs were quantitatively evaluated according to Debye-Scherer formula from the broadening of intensive peak (111).
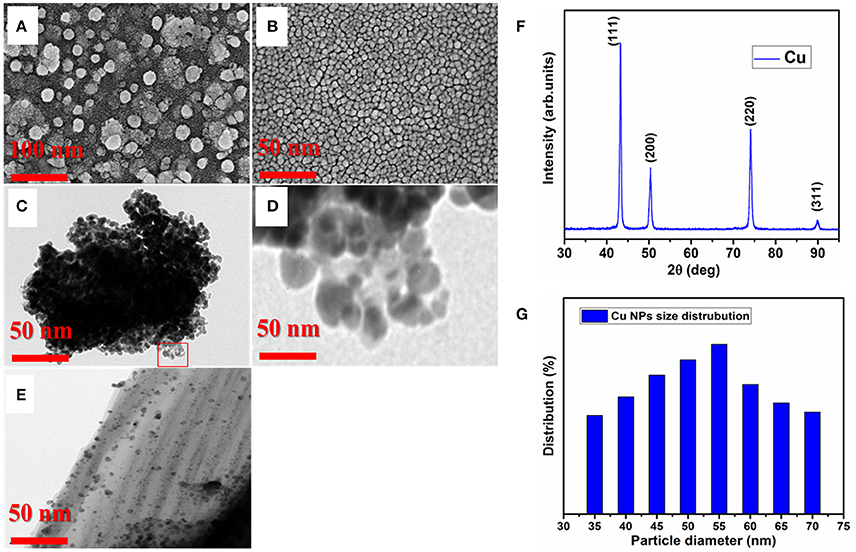
Figure 1. Particle size and distribution of Cu-NPs. (A), FESEM image of Cu-NPs before sonication (B), FESEM image of Cu-NPs after 6 h sonication (C), TEM image of Cu-NPs before sonication (D), Zoomed image of Cu-NPs from Panel (C), (E), TEM image of Cu-NPs after 6 h sonication (F), XRD pattern of Cu-NPs (G), Average particle size distribution of Cu-NPs.
Where D is the average particle size in nanometer, k is the Scherrer constant, β is the full-width half the maximum intensity (FWHM), λ is the X-ray wavelength (λ = 0.154 nm), θ is the Bragg angle. The average particle size was found as 50 nm according to Debye-Scherer formula using imageJ software based on the Cu morphology (Figure 1G). The size average size distribution was conformed as 40–60 nm which is well matched with XRD and TEM particle size analysis.
Expression Analysis of Several Steroidogenic Enzyme and Transcription Factor Genes Involved in Steroidogenesis and Testis Development
Expression level of several steroidogenic enzyme (3β-hsd, cyp11a1, 11β-hsd2, 11β-h, and star) and transcription factor genes (wt1, ad4bp/sf-1, dmrt1, sox9a, and gata4) were analyzed and quantified in control and treated (CuSO4 and Cu-NPs) groups represented by fold increase. In general, expression level of all the steroidogenic enzyme genes analyzed showed significant upregulation in both the treated groups when compared to control (Figure 2A). The expression of 3β-hsd increased significantly to 2.8 and 2.6 (P < 0.01) fold in CuSO4 and Cu-NPs treated groups, respectively when compared to control. Significant expression of cyp11a1 was found in treated batches of CuSO4 and Cu-NPs with fold increase of 2.8 and 3.8 (P < 0.01), respectively in comparison with control fish. The expression of 11β-hsd2 also showed significant increase to 3.5 (P < 0.01) and 1.3 (P < 0.05) fold, respectively in CuSO4 and Cu-NPs treated compared with control. Further, significant increase in the levels of 11β-h to 1.5 (P < 0.05) and 2.6 (P < 0.01) fold, respectively was observed in CuSO4 and Cu-NPs treated groups in comparison with control. Significant (P < 0.01) increase of star expression to 2.2 and 3.0-fold was evident in CuSO4 and Cu-NPs treated fish, respectively.
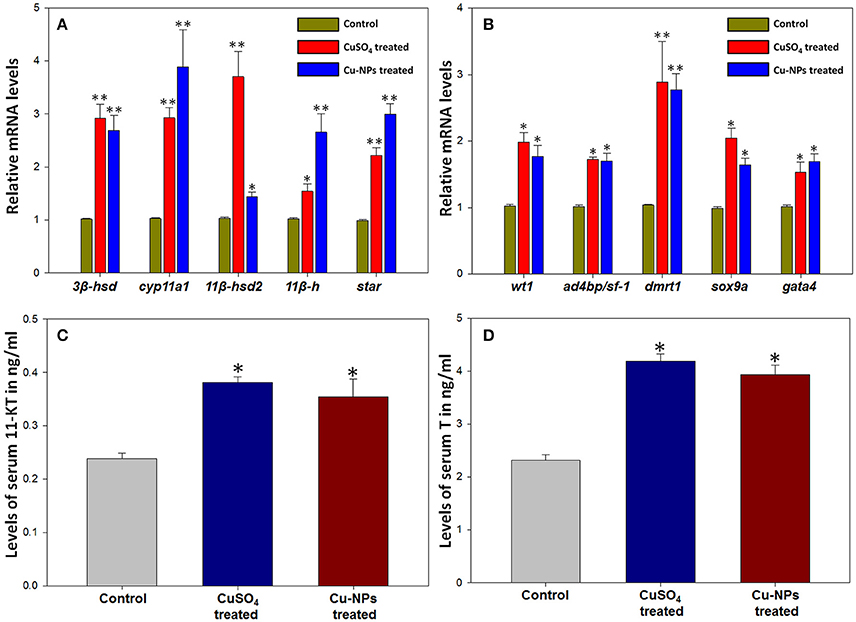
Figure 2. Changes in mRNA transcripts of several steroidogenic enzyme (A) and transcription factor (B) genes and levels of androgens, 11-ketotestosterone (C) and testosterone (D) after Cu/Cu-NPs exposure during testicular recrudescence in catfish, C. batrachus. Data (n = 5) were expressed as mean ± SEM (*, P < 0.05; ** P < 0.01; ANOVA followed by Student-Newman-Keuls' post-hoc test).
Similarly, the expression analysis of several transcription factor genes showed significant increase in expression when compared to control (Figure 2B). The expression of wt1 increased significantly (P < 0.05) to 1.9 and 1.7-fold in CuSO4 and Cu-NPs treated groups, respectively when compared to control. Significant (P < 0.05) increase of ad4bp/sf-1 to 1.7 and 1.6-fold while dmrt1 showed significant (P < 0.01) increase to 2.7 and 2.2-fold, respectively in CuSO4 and Cu-NPs treated fishes than control. Further, the expression of sox9a and gata4 levels were increased significantly (P < 0.05) to 2.0 and 1.6-fold and 1.5 and 1.6-fold in CuSO4 and Cu-NPs treated fish, respectively when compared to controls.
Effect of Serum 11-KT and T Levels in Control and Treated Catfish
Serum 11-KT and T levels estimated by EIA were compared between the control and treated groups (Figures 2C,D). The levels of 11-KT in treated groups showed significant increase (P < 0.05; Figure 2C) than the levels of control. Correspondingly, T levels in serum of treated fishes of C. batrachus (Figure 2D) were significantly elevated (P < 0.05) when compared to control.
Histology of Control and Treated Testis of Catfish
Control testis of catfish stained with hematoxylin and eosin (Figures 3A–F) displayed normal basal lamina consisting of interstitial/Leydig cells (Figure 3D), spermatogonia with dark nuclei and spermatocytes with large oval nuclei (Figures 3C,E,F). The spermatids and/or sperm were detected in the lumen of testis in control (Figure 3C). In the treated groups, reduction in the size of testicular lumen with disrupted basal lamina was seen (Figures 3G–R). Spermatocytes were completely separated from the basal lamina integration with distinct shape were observed in each lumen of testis (Figures 3G,J,K,M,O,Q). Spermatogonia displayed abnormal structure with signs of disrupted nuclei (Figures 3K,Q) when compared to the control. The Cu-NPs treated group showed disorganization in testis lumen (Figures 3M,N) with irregular and enlarged spermatocytes (Figure 3Q). Interestingly, the interstitial cell layer is not affected as the testicular architecture was not altered, which is even evident at low magnification in the treated groups (Figures 3G,H,M,N). However, both the treated groups showed corroborative effects when exposed to Cu as a soluble metal and NPs.
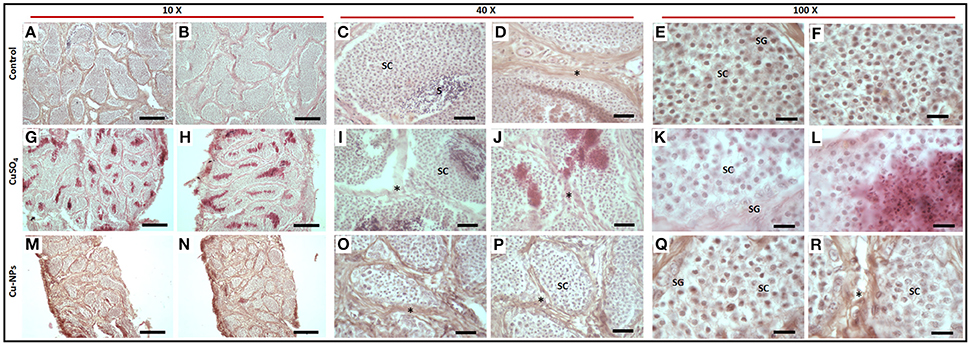
Figure 3. Histology of representative control (A–F), CuSO4 (G–L) and CU-NPs (M–R) treated testis of catfish stained with hematoxylin and eosin. * indicates basal lamina (BL); Spermatocytes (SC); Spermatogonium (SG); Sperm and/or spermatids (S). Scale bar indicates (10 × : 120 μm; 40 × : 25 μm and 100 × : 10 μm).
TEM Analysis of Control and Treated Testis of Catfish
TEM analysis of control testis showed normal testicular organization with basal lamina consists of interstitial/Leydig or myoid cells and regular shaped spermatocytes (Figures 4A,D,G). Spermatocyte of control testis displayed spherical/oval shaped nuclei with well-defined nuclear membrane which is attached to endoplasmic reticulum (Figure 4G). Cytoplasm of the spermatocyte consists of ribonucleic protein granules. Sertoli cell comprises of thick nucleolus which is present near basal lamina facing toward the testis lumen (Figure 4J). Erythrocyte of control testis showed normal nucleated uniform structure located inside the lumen of capillary blood vessel (Figure 4M).
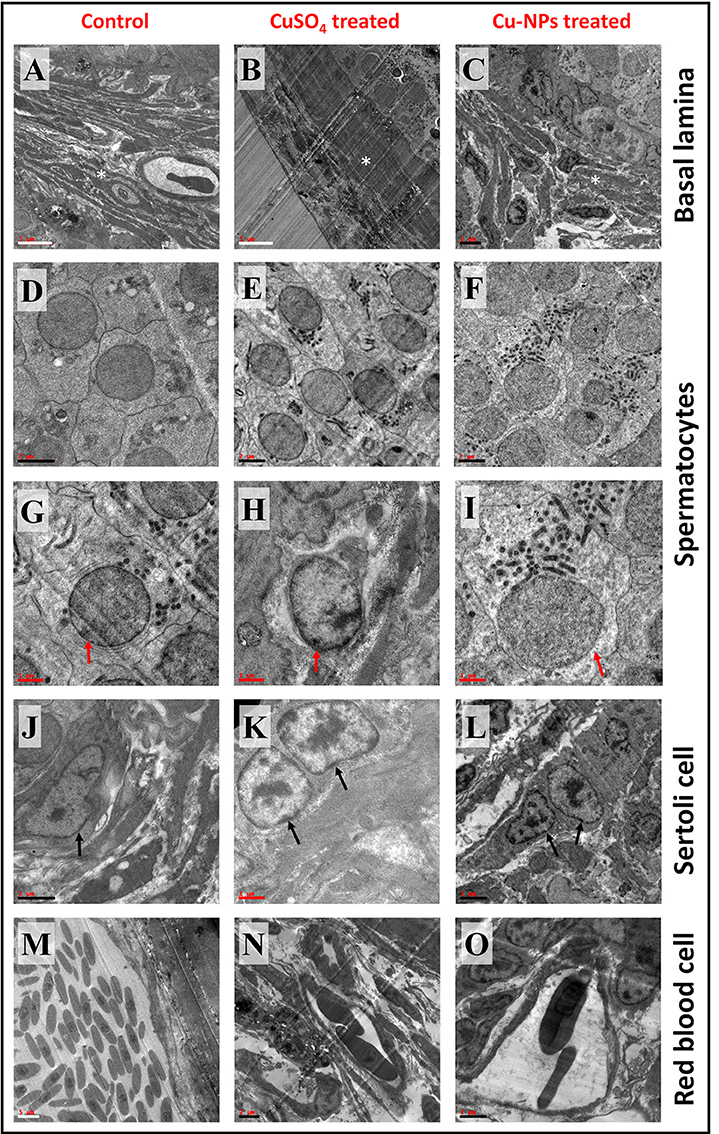
Figure 4. Transmission electron microscope (TEM) representative images of basal lamina (BL), Spermatocyte (SC), Sertoli (ST) cell and red blood cell (RBC) of control and treated catfish. Control testis (A,D,G,J,M) indicates normal appearance of BL, SC, ST, and RBC. CuSO4 treated testis (B,E,H,K,N) showing disruption in BL, irregular SC and ST with disruption in nuclear membrane and distinct RBC. Cu-NPs treated testis (C,F,I,L,O) displayed degenerated BL, apoptotic SC and ST with disappearance of nuclear membrane and deformability of RBC. * indicates BL, red and black arrow indicates SC and ST, respectively. Scale bar: white bar—5 μm; black bar—2 μm and red bar—1 μm.
TEM images from treated testis (both groups) exhibited degeneration of basal lamina with the presence of apoptotic cells (Figures 4B,C). Distinct shaped spermatocytes with degeneration of nuclear membrane were seen in both groups (Figures 4E,F,H,I). Sertoli cells of treated groups showed chromatin marginalization with disintegration of nuclear membrane and electron dense bodies (Figures 4K,L). Treated groups of testis displayed erythrocyte deformability inside the capillary. In addition, the capillary walls showed some damage after the treatment (Figures 4N,O).
Spermatocyte with vacuolated cytoplasm was observed in the treated group (Figure 5A). Interestingly, bio-distribution of Cu-NPs with apoptotic cells were observed in the treated testis (Figures 5B–F).
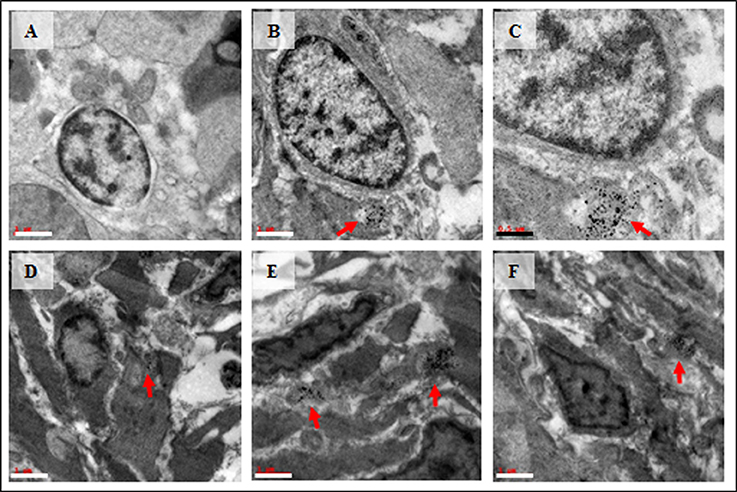
Figure 5. TEM images of vacuolated spermatocyte (A) and bio-distribution of Cu-NPs in testis with apoptotic cells (B–F) in treated testis. Red arrows showed the presence of Cu-NPs in testis. Scale bar: white bar—1 μm; black bar—0.5 μm.
Cytotoxic and Morphological Analysis of Catfish Primary Testicular Culture and TM3 Leydig Cells upon Cu Treatments
Relative cell viability decreased with the increasing concentrations of CuSO4 and Cu-NPs exposure to cell cultures. For catfish primary testicular cells, the LC50 value was calculated at 20.5 and 30.0 μg/ml for Cu-NPs and CuSO4, respectively (Figure 6Ai). In the case of TM3 Leydig cell culture, the LC50 value was noted at 37.5 and 25.3 μg/ml for Cu-NPs and CuSO4, respectively (Figure 6Aii). In comparison, the cytotoxic effects were almost similar for both type of cells.
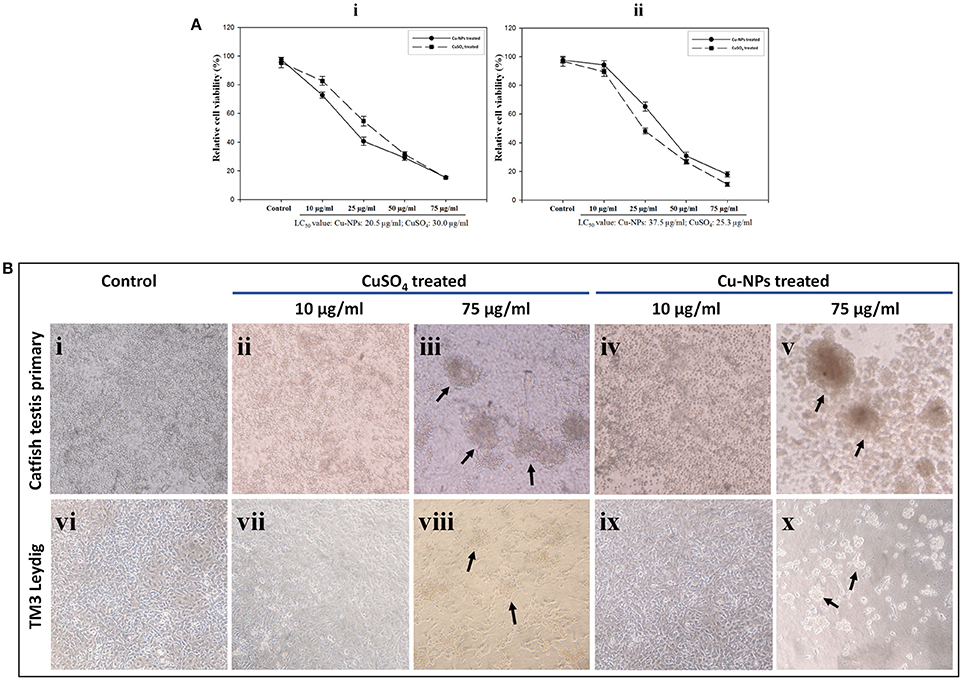
Figure 6. Cytotoxic effect of CuSO4 and Cu-NPs with different concentrations exposed to catfish testis primary culture (Ai) and TM3 Leydig cell line (Aii) for 48 h incubation. Relative cell viability (%) was determined for cells exposed to PBS (control) and CuSO4 and Cu-NPs (treated) by MTT assay as described in Section Mitochondrial function assay (Ai,ii). All results were expressed as total percentage of viable cells with mean ± SEM of three independent determinations with (n = 5) for each point (P < 0.05; statistically significant difference compared with control). Microcopy based assessment of catfish testis primary culture and TM3 Leydig cell line of control (Bi,vi), CuSO4 (Bii,iii,vii,viii) and Cu-NPs (Biv,v,ix,x), respectively. Indicated doses of CuSO4 (equivalent amount of copper load) and Cu-NPs were exposed to the cells for 48 h in serum free DMEM. Arrows highlight loss of adhesion and cell clumping as signs for toxicity. Cells exposed to other indicated concentrations showed the same morphology and hence, we provided the low and high doses studied in the experiment.
By examining the cell morphology, control groups of both the cultures showed normal adherent nature throughout the experiment period (Figures 6Bi,vi). Upon treatment, the cells exhibited dramatic change in the morphology. In 10 μg/ml treatment group, cells started to show signs of clumping in culture dishes (Figures 6Bii,iv,vii,ix). However, cell toxicity was not evident. Loss of adhesion, cell clumping and shrinkage were more in Cu-NPs and CuSO4 of 75 μg/ml treated batches (Figures 6Biii,v,viii,x). However, similar results were observed in rest of the batches analyzed (photos not shown). Distinct morphological changes during treatment indicate the occurrence of apoptosis/necrosis.
Flow Cytometric Analysis of Catfish Testicular Culture and TM3 Leydig Cells Exposed to Cu
To further investigate the Cu-induced cellular toxicity, control and treated cells of catfish primary testicular culture (Figure 7A) and TM3 Leydig cell (Figure 7B) were added with YO-PRO-1 and PI dyes and the difference in normal and apoptotic/necrotic cells were analyzed using flow cytometry. Results indicated that percentage of apoptotic/necrotic cells were increased with the increasing concentration of Cu-NPs and CuSO4 exposure (Figures 7A,B) when compared to the control (low right quadrant). The percentage of cells appeared in the positively stained with YO-PRO-1 which represented early apoptotic cells (lower right quadrant) and cells stained with both YO-PRO-1 and PI (upper right quadrant) which denoted late apoptotic cells were calculated and compared with the control. However, the percentage of dead cell population was evaluated from the upper left quadrant. The percentage of population in each quadrant was given in Supplementary Table 2.
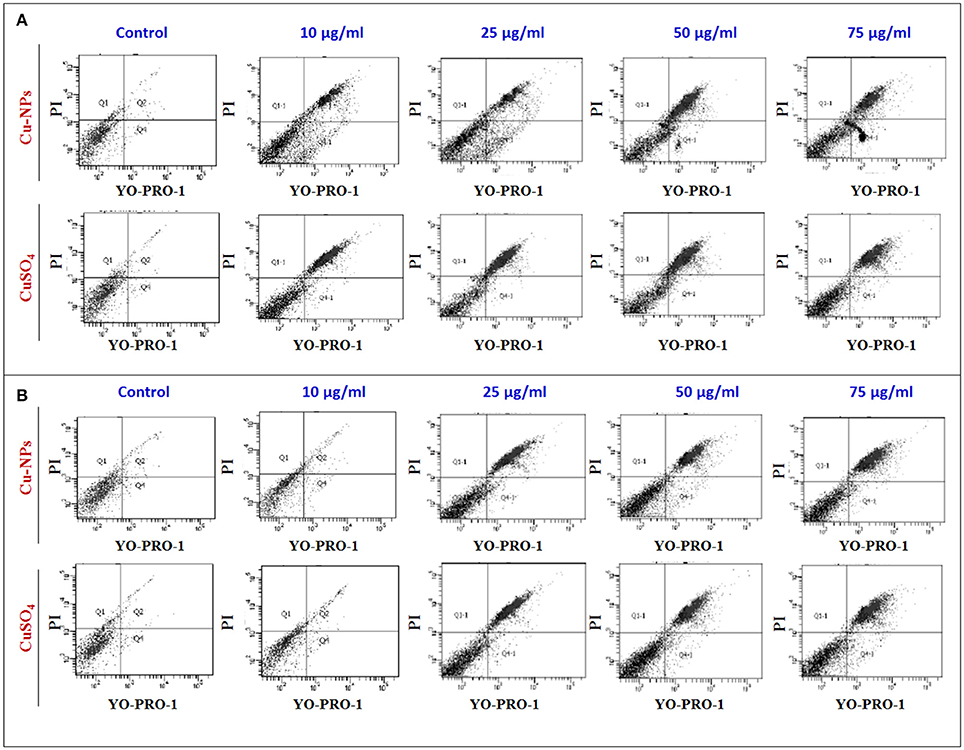
Figure 7. Flow cytometry analysis of catfish primary testicular culture (6A) and TM3 Leydig cell culture (6B) exposed to increasing concentration of Cu-NPs and CuSO4 and evaluated using YO-PRO-1 and PI labeled dye. All figures stained with YO-PRO-1 which represented early apoptotic cells (lower right quadrant) and cells stained with both YO-PRO-1 and PI (upper right quadrant) which denoted late apoptotic cells were calculated and compared with the control. The percentage of cells were calculated and listed in Supplementary Table 2.
Discussion
In nature, due to its quantum size, NPs can easily enter through several ports, interact with biomolecules, causing adverse effect on organisms. Available scientific evidence suggests that NPs can enter via lungs or gills, skin or oral route and can cause oxidative stress-dependent toxic effects (Yah et al., 2012; Gupta et al., 2016). Interestingly, several studies have authenticated that NPs can cross the blood-testis barrier, leading to testicular accumulation and translocation to testicular cells and/or nucleus to generate adverse effects (Lan and Yang, 2012). However, present study provided comparative analysis on the impact of Cu-NPs with a comparison on soluble Cu. Interestingly, Cu also has physiological relevance and is an essential element involved in normal homeostasis in fish (Miura and Miura, 2011). In this regard, present study analyzed the impact of Cu (both forms) in catfish in relation to male reproduction at molecular, histological and ultra-structural level which revealed differential level of effects. In addition, cytotoxic effect of Cu was analyzed in catfish primary testicular cells (mixed) and TM3 Leydig cells. Ion leaching will occur in the process of metal oxide NPs synthesis in which metal oxide surface will release the metal cations and dissolve in the medium. Cu-NPs are very sensitive to the biological samples due to the toxicity of Cu2+ ion presence (Borgmann and Ralph, 1983). In order to check this, we performed TEM analysis to validate the size and shape of Cu-NPs before and after sonication. Based on the results, we presume that after a 6 h of sonication, the toxicity was evident due to leaching of Cu ions from the surface of Cu-NPs.
Next, we analyzed the impact of Cu leaching by the CuSO4 and Cu-NPs treatments on the regulation of testicular steroidogenesis and architecture. Testicular steroidogenesis is a complex event wherein androgens are synthesized through steroidogenic enzyme reaction cascade. The most potent androgens, T and 11-KT are primarily involved in testicular development and the normal maintenance of spermatogenesis (Nagahama, 1994; Tokarz et al., 2015). Transcription factors are proteins that control the regulation of gene expression directly and/or indirectly through intracellular signaling pathway. Interestingly, most of the transcription factors play critical role in regulating steroidogenesis (Morohashi and Omura, 1996; Tremblay and Viger, 2003). Reports in relation to the effect of NPs on the expression of transcription factors related to testicular development/recrudescence are scarce in teleosts. Reports from our laboratory demonstrated the importance of certain transcription factors like Dmrt1 and sox9a due to their predominance in preparatory to pre-spawning phases when spermatogonia and spermatocytes are abundant (Raghuveer and Senthilkumaran, 2009, 2010). Further, wt1, ad4bp/sf-1, and gata4 were found to be essential factors for spermatogenesis and Sertoli cell function (Nakayama et al., 1998; Lei and Heckert, 2004; Ijiri et al., 2007; Murugananthkumar and Senthilkumaran, 2016). To this end, present analysis on expression pattern of steroidogenic enzyme (3β-hsd, cyp11a1, 11β-hsd2, 11β-h, and star) and transcription factor (wt1, ad4bp/sf-1, dmrt1, sox9a, and gata4) genes revealed up-regulation at different levels with concomitant increase of androgens (T and 11-KT) in the treated groups. These changes can be attributed to the entry of Cu into testis in both the treated groups.
Several reports on fish suggest that exposure of Cu poses serious threat, resulting in altered gene expression and cellular damage (Craig et al., 2009). Incidentally, exposure of NPs in mice resulted in the increased expression of several steroidogenic enzyme genes and T synthesis. While the above finding may be considered as a favorable effect, histological analysis showed entry of NPs cause severe damage to testicular cells which further alters hormone levels (Zhang et al., 2015). Supplementation of silver NPs subcutaneously in mice resulted in significant upregulation in Cyp11a1 and 3β-hsd expression with increased testosterone levels (Garcia et al., 2014). Similarly, in vitro supplement of carbon black and diesel exhaust particles showed upregulation of StAR in mouse Leydig cells (Komatsu et al., 2008). In contrast, titanium dioxide exposure on mice decreased steroidogenic enzyme genes expression and T synthesis (Jia et al., 2014). In zebrafish, different NPs exposure on gills did not produce the same effect (Griffitt et al., 2009). As explained earlier, effect caused due to NPs is purely based on its nature and properties which can cause adverse reaction. Interestingly, in the present report elevated levels of steroidogenic enzyme and transcription factor genes and androgens signifies the promotional effect on Cu on testicular development in catfish while histological and TEM analysis showed a contrasting deleterious effect.
Histological analysis revealed severe cellular damage in both the treated groups. Findings of damaged basal lamina correlated with the disruption of blood-testis barrier, affecting the micro environment (lumen) for sperm formation. Treated groups showed disrupted spermatocytes, apoptosis and/or necrosis of spermatocytes with distinct spermatogonium. Further, the study was extended for ultra-structural analysis on basal lamina, spermatocytes, Sertoli cell and erythrocytes. Both the treated groups displayed degenerative changes in basal lamina and distinct spermatocytes. Apoptotic Sertoli cell with nuclear membrane collapse, chromatin marginalization, and erythrocyte deformability were seen in the treated groups implicating targeted effects on spermatogenic cells. Spermatogenesis is highly coordinated process where spermatozoa are produced inside the lumen by the regulation of various transcription factors and hormones including androgens (Nagahama, 1994). Blood-testis barrier is formed between the blood vessels and tight junctions between adjoining Sertoli cells. Unlike birds and mammals, less evolved blood-testis barrier in fish provides only a marginally effective internal compartmentation for spermatid/spermatozoa to prevent autoimmune damage in testis. Present report revealed treatment of CuSO4 and Cu-NPs disrupted the basal lamina which further affected Sertoli cell barrier and spermatocytes. Histological and ultra-structural study on rat by Thakur et al. (2014) indicated that the treatment of NPs, orally, affected the testicular morphology by causing Sertoli cell damage and cytoplasmic degeneracy with several vacuoles. Further, exposure of Cu resulted in damage to the tissues and cause oxidative stress (Varanka et al., 2001). Interestingly, the results also showed accumulation of NPs near the basement membrane, vacuolated spermatocytes and bio-distribution of Cu-NPs in testicular lumen. Though damages were seen in histological and TEM analysis, exposure of Cu might have induced certain transcripts in relation to androgens as the disruptive effects are limited to germ and Sertoli cells but not to interstitial cells. It is also possible to presume as a compensatory mechanism by interstitial cells to restore the testicular lumen by increased production of androgens. This phenomenon needs to be analyzed further yet plausible impairment of Cu-NPs toxicity is fairly evident in spite of elevation of androgens in catfish. It is also plausible that the increased levels of testosterone results in negative impact on testis which was also evident in the Atlantic salmon with high androgens (Antonopoulou et al., 2009). In view of this, cytotoxic analysis in both testicular primary and TM3 Leydig cell cultures were performed with high doses, (from 75 μg/ml onwards) which exposed the potential of Cu to cause cellular toxicity and apoptosis. Toxicity effect was not evident in 10 μg/ml dose which confirms the normal response of interstitial cells after in vivo treatment. Based on this, it is possible to suggest that the interstitial/Leydig cells susceptibility to toxicity happens only at high doses but not in the lower doses as observed in the in vivo study resulting in high T and 11-KT levels. Supporting the above finding, zebrafish exposed to Cu have been found to have increased expression of genes involved in the intrinsic and extrinsic pathways of apoptosis (Luzio et al., 2013). Concentration dependent cytotoxicity was observed in mouse fibroblasts cells treated with Cu (Cao et al., 2012). In addition, ROS mediated cytotoxic effect were seen in exposure of Cu against human glioma cells (Recio Despaigne et al., 2014). The above evidence suggest and support that Cu can induce apoptosis in catfish, which was observed in the in vitro analysis, revealing morphological features characteristic of apoptosis. Taken together eco-relevant dose under in vivo acts mildly when compared to high in vitro doses which eventually implicate differential deleterious effects of Cu-NPs.
In fish, though CuSO4 and Cu-NPs treatment upregulated steroidogenic enzyme and transcription factor genes with corroborative elevation of androgens (T and 11-KT), recrudescing testicular lumen architecture could not cope up these changes, resulting in differential cellular disarray and damage as depicted by histology and TEM analysis. Further, in vitro results warranted the cytotoxic effect of Cu on cell cultures. Taken together, the findings warrant reproductive toxicity effects of Cu and Cu-NPs even if the levels of contaminants in the environment are low.
Conclusion
To our knowledge, this is the first report with comprehensive analysis of the impact of Cu-NPs in a male teleost focusing reproduction. The exposure of Cu as soluble metal and NPs in catfish testis resulted in upregulation of several genes and androgens related to testicular growth. Histology and TEM images exhibited damage in testicular cells and defect in spermatogenesis. In vitro studies showed Cu is very sensitive to the cells and can induce cytotoxicity. Taken together, the present findings suggested that exposure of Cu (both forms) even at minimum concentration affect reproductive health in catfish.
Author Contributions
RM and BS conceived and designed the experiments. DR performed particle characterization while RM and BS analyzed, interpreted, and wrote the manuscript. BS supervised the work overall.
Conflict of Interest Statement
The authors declare that the research was conducted in the absence of any commercial or financial relationships that could be construed as a potential conflict of interest.
Acknowledgments
This work was completely supported by Department of Biotechnology (DBT), India through DBT-TATA innovation fellowship (BT/HRD/35/01/02/2013) awarded to BS. RM is thankful to the Council of Scientific and Industrial Research, India for Junior and Senior Research fellowships. We sincerely thank Dr. M. Lakshman, College of Veterinary Science, Hyderabad for TEM sample preparation. We also acknowledge the Centre for Nanotechnology, University of Hyderabad for TEM facility and DST-FIST (level 1, phase-II) to the Department of Animal Biology at School of Life Sciences, University of Hyderabad.
Supplementary Material
The Supplementary Material for this article can be found online at: http://journal.frontiersin.org/article/10.3389/fenvs.2016.00067/full#supplementary-material
References
Abdel-Khalek, A. A., Kadry, M. A. M., Badran, S. R., and Marie, M. A. S. (2015). Comparative toxicity of copper oxide bulk and nano particles in Nile Tilapia; Oreochromis niloticus: biochemical and oxidative stress. JOBAZ 72, 43–57. doi: 10.1016/j.jobaz.2015.04.001
Antonopoulou, E., Swanson, P., and Borg, B. (2009). Effects of aromatase inhibitors and different doses of testosterone on gonadotropins in one year old male Atlantic salmon (Salmo salar). Comp. Biochem. Physiol. 153A, 408–416. doi: 10.1016/j.cbpa.2009.03.019
ATSDR (2002). Toxicological Profile for Copper (Draft for Public Comment). Atlanta, GA: US Department of Health and Human Services, Public Health Service, Agency for Toxic Substances and Disease Registry (Subcontract No. ATSDR-205-1999-00024).
Authman, M. M. N., Zaki, M. S., Khallaf, E. A., and Abbas, H. H. (2015). Use of fish as bio-indicator of the effects of heavy metals pollution. J. Aquac. Res. Dev. 6, 328. doi: 10.4172/2155-9546.1000328
Begum, A., Ramaiah, M., Harikrishna Khan, I., and Veena, K. (2009). Heavy metal pollution and chemical profile of Cauvery river water. E J. Chem. 6, 47–52. doi: 10.1155/2009/154610
Borgmann, U., and Ralph, K. M. (1983). Complexation and toxicity of copper and the free metal bioassay technique. Water Res. 17, 1697–1703. doi: 10.1016/0043-1354(83)90030-1
Braydich-Stolle, L., Hussain, S., Schlager, J. J., and Hofmann, M. C. (2005). In vitro cytotoxicity of nanoparticles in mammalian germline stem cells. Toxicol. Sci. 88, 412–419. doi: 10.1093/toxsci/kfi256
Cao, B., Zheng, Y., Xi, T., Zhang, C., Song, W., Burugapalli, K., et al. (2012). Concentration-dependent cytotoxicity of copper ions on mouse fibroblasts in vitro: effects of copper ion release from TCu380A vs TCu220C intra-uterine devices. Biomed. Microdevices 14, 709–720. doi: 10.1007/s10544-012-9651-x
Cao, L., Huang, W., Liu, J., Ye, Z., and Dou, S. (2010). Toxicity of short-term copper exposure to early life stages of red sea bream, Pagrus major. Environ. Toxicol. Chem. 29, 2044–2052. doi: 10.1002/etc.247
Craig, P. M., Hogstrand, C., Wood, C. M., and McClelland, G. B. (2009). Gene expression endpoints following chronic waterborne copper exposure in a genomic model organism, the zebrafish, Danio rerio. Physiol. Genomics 40, 23–33. doi: 10.1152/physiolgenomics.00089.2009
Damodharan, U. (2013). “Bioaccumulation of heavy metals in contaminated river Water-Uppanar, Cuddalore, South East Coast of India,” in Perspectives in Water Pollution, eds I. Ahmad and M. A. Dar (Rijeka: Croatia-European Union; InTech), 23–34. doi: 10.5772/53374
Fernandes, A. C., Medeiros, C. O., Bernardo, G. L., Ebone, M. V., Pietro, P. F. D., de Assis, M. A. A., et al. (2012). Benefits and risks of fish consumption for the human health. Rev. Nutr. 25, 283–295. doi: 10.1590/S1415-52732012000200010
Garcia, T. X., Costa, G. M., França, L. R., and Hofmann, M. C. (2014). Sub-acute intravenous administration of silver nanoparticles in male mice alters Leydig cell function and testosterone levels. Reprod. Toxicol. 45, 59–70. doi: 10.1016/j.reprotox.2014.01.006
Ghorade, I. B., Lamture, S. V., and Patil, S. S. (2014). Assessment of heavy metal content in godavari river water. Int. J. Res. Appl. Nat. Soc. Sci. (IMPACT: IJRANSS) 2, 23–26.
Goswami, D. N., and Sanjay, S. S. (2014). Determination of heavy metals, viz. cadmium, copper, lead and zinc in the different matrices of the Ganges River from Rishikesh to Allahabad through differential pulse anodic striping voltametry. Int. J. Adv. Res. Chem. Sci. (IJARCS) 1, 7–11.
Griffitt, R. J., Hyndman, K., Denslow, N. D., and Barber, D. S. (2009). Comparison of molecular and histological changes in zebrafish gills exposed to metallic nanoparticles. Toxicol. Sci. 107, 404–415. doi: 10.1093/toxsci/kfn256
Griffitt, R. J., Weil, R., Hyndman, K. A., Denslow, N. D., Powers, K., Taylor, D., et al. (2007). Exposure to copper nanoparticles causes gill injury and acute lethality in zebrafish (Danio rerio). Environ. Sci. Technol. 41, 8178–8186. doi: 10.1021/es071235e
Gupta, Y. R., Durai, S., Monica, K., Deepa, S., Senthilkumaran, B., and Basavaraju, Y. (2016). Effect of copper nanoparticles exposure in the physiology of the common carp (Cyprinus carpio): biochemical, histological and proteomic approaches. Aquac. Fish. doi: 10.1016/j.aaf.2016.09.003
Hua, J., Vijver, M. G., Ahmad, F., Richardson, M. K., and Peijnenburg, W. J. (2014). Toxicity of different-sized copper nano- and submicron particles and their shed copper ions to zebrafish embryos. Environ. Toxicol. Chem. 33, 1774–1782. doi: 10.1002/etc.2615
Ijiri, S., Kaneko, H., Kobayashi, T., Wang, D. S., Sakai, F., Paul-Prasanth, B., et al. (2007). Sexual dimorphic expression of genes in gonads during early differentiation of a teleost fish, the Nile tilapia Oreochromis niloticus. Biol. Reprod. 78, 333–341. doi: 10.1095/biolreprod.107.064246
IPCS (1998). Copper. Geneva: World Health Organization, International Programme on Chemical Safety (Environmental Health Criteria 200).
Jain, C. K. (2004). Metal fractionation study on bed sediments of River Yamuna, India. Water Res. 38, 569–578. doi: 10.1016/j.watres.2003.10.042
Jia, F., Sun, Z., Yan, X., Zhou, B., and Wang, J. (2014). Effect of pubertal nano-TiO2 exposure on testosterone synthesis and spermatogenesis in mice. Arch. Toxicol. 88, 781–788. doi: 10.1007/s00204-013-1167-5
Kaida, T., Kobayashi, K., Adachi, M., and Suzuki, F. (2004). Optical characteristics of titanium oxide interference film and the film laminated with oxides and their applications for cosmetics. J. Cosmet. Sci. 55, 219–220.
Kirubagaran, R., and Joy, K. P. (1992). Toxic effects of mercury on testicular activity in the freshwater teleost, Clarias batrachus (L.). J. Fish Biol. 41, 305–315. doi: 10.1111/j.1095-8649.1992.tb02659.x
Komatsu, T., Tabata, M., Kubo-Irie, M., Shimizu, T., Suzuki, K., Nihei, Y., et al. (2008). The effects of nanoparticles on mouse testis Leydig cells in vitro. Toxicol. In Vitro 22, 1825–1831. doi: 10.1016/j.tiv.2008.08.009
Kovrižnych, J. A., Sotníková, R., Zeljenková, D., Rollerová, E., Szabová, E., and Wimmerová, S. (2013). Acute toxicity of 31 different nanoparticles to zebrafish (Danio rerio) tested in adulthood and in early life stages - comparative study. Interdiscip. Toxicol. 6, 67–73. doi: 10.2478/intox-2013-0012
Krysanov, E. Y., Pavlov, D. S., Demidova, T. B., and Dgebuadze, Y. Y. (2010). Effect of nanoparticles on aquatic organisms. Biol. Bull. 37, 406–412. doi: 10.1134/S1062359010040114
Lan, Z., and Yang, W. X. (2012). Nanoparticles and spermatogenesis: how do nanoparticles affect spermatogenesis and penetrate the blood-testis barrier. Nanomedicine 7, 579–596. doi: 10.2217/nnm.12.20
Lei, N., and Heckert, L. L. (2004). Gata4 regulates testis expression of Dmrt1. Mol. Cell. Biol. 24, 377–388. doi: 10.1128/MCB.24.1.377-388.2004
Lemly, A. D. (2002). Symptoms and implications of selenium toxicity in fish: the Belews Lake case example. Aquat. Toxicol. 57, 39–49. doi: 10.1016/S0166-445X(01)00264-8
Luo, Y., Shan, D., Zhong, H., Zhou, Y., Chen, W., Cao, J., et al. (2015). Subchronic effects of cadmium on the gonads, expressions of steroid hormones and sex-related genes in tilapia Oreochromis niloticus. Ecotoxicology 24, 2213–2223. doi: 10.1007/s10646-015-1542-5
Luzio, A., Monteiro, S. M., Fontaínhas-Fernandes, A. A., Pinto-Carnide, O., Matos, M., and Coimbra, A. M. (2013). Copper induced upregulation of apoptosis related genes in zebrafish (Danio rerio) gill. Aquat. Toxicol. 128–129, 183–189. doi: 10.1016/j.aquatox.2012.12.018
Mane, A. V., Pardeshi, R. G., Gore, V. R., Walave, R. L., Manjrekar, S. S., and Sutar, G. N. (2013). Water quality and sediment analysis at selected locations of Pavana river of Pune district, Maharashtra. J. Chem. Pharm. Res. 5, 91–102.
Miura, C., and Miura, T. (2011). Analysis of spermatogenesis using an Eel model. Aqua BioSci. Monogr. 4, 105–129. doi: 10.5047/absm.2011.00404.0105
Moore, M. N. (2006). Do nanoparticles present ecotoxicological risks for the health of the aquatic environment? Environ. Int. 32, 967–976. doi: 10.1016/j.envint.2006.06.014
Moosavi, M. J., and Shamushaki, V. A. J. (2015). Effects of different levels of copper sulfate on growth and reproductive performances in guppy (P. reticulate). J. Aquac. Res. Dev. 6, 305. doi: 10.4172/2155-9546.1000305
Morohashi, K. I., and Omura, T. (1996). Ad4BP/SF-1, a transcription factor essential for the transcription of steroidogenic cytochrome P450 genes and for the establishment of the reproductive function. FASEB J. 10, 1569–1577.
Murugananthkumar, R., and Senthilkumaran, B. (2016). Expression analysis and localization of wt1, ad4bp/sf-1 and gata4 in the testis of catfish, Clarias batrachus: impact of wt1-esiRNA silencing. Mol. Cell. Endocrinol. 431, 164–176. doi: 10.1016/j.mce.2016.05.006
Nakayama, Y., Yamamoto, T., Matsuda, Y., and Abé, S. I. (1998). Cloning of cDNA for newt WT1 and the differential expression during spermatogenesis of the Japanese newt, Cynops pyrrhogaster. Dev. Growth Differ. 40, 599–608. doi: 10.1046/j.1440-169X.1998.00400.x
Raghuveer, K., and Senthilkumaran, B. (2009). Identification of multiple dmrt1s in catfish: localization, dimorphic expression pattern, changes during testicular cycle and after methyltestosterone treatment. J. Mol. Endocrinol. 42, 437–448. doi: 10.1677/JME-09-0011
Raghuveer, K., and Senthilkumaran, B. (2010). Isolation of sox9 duplicates in catfish: localization, differential expression pattern during gonadal development and recrudescence, and hCG-induced up-regulation of sox9 in testicular slices. Reproduction 140, 477–487. doi: 10.1530/REP-10-0200
Rai, M., and Ingle, A. (2012). Role of nanotechnology in agriculture with special reference to management of insect pests. Appl. Microbiol. Biotechnol. 94, 287–293. doi: 10.1007/s00253-012-3969-4
Rajakumar, A., Singh, R., Chakrabarty, S., Murugananthkumar, R., Laldinsangi, C., Prathibha, Y., et al. (2012). Endosulfan and flutamide impair testicular development in the juvenile Asian catfish, Clarias batrachus. Aquat. Toxicol. 110–111, 123–132. doi: 10.1016/j.aquatox.2011.12.018
Recio Despaigne, A. A., Da Silva, J. G., da Costa, P. R., Dos Santos, R. G., and Beraldo, H. (2014). ROS-mediated cytotoxic effect of copper (II) hydrazone complexes against human glioma cells. Molecules 19, 17202–17220. doi: 10.3390/molecules191117202
Senthilkumaran, B. (2015). Pesticide- and sex steroid analogue-induced endocrine disruption differentially targets hypothalamo-hypophyseal-gonadal system during gametogenesis in teleosts - a review. Gen. Comp. Endocrinol. 219, 136–142. doi: 10.1016/j.ygcen.2015.01.010
Shaw, B. J., and Handy, R. D. (2011). Physiological effects of nanoparticles on fish: a comparison of nanometals versus metal ions. Environ. Int. 37, 1083–1097. doi: 10.1016/j.envint.2011.03.009
Şeker, S., and Kutlu, B. (2014). Determination of copper (Cu) levels for rivers in Tunceli Turkey. World Environ. 4, 168–171. doi: 10.5923/j.env.20140404.02
Song, L., Connolly, M., Fernández-Cruz, M. L., Vijver, M. G., Fernández, M., Conde, E., et al. (2014). Species-specific toxicity of copper nanoparticles among mammalian and piscine cell lines. Nanotoxicology 8, 383–393. doi: 10.3109/17435390.2013.790997
Song, M., and Gutzeit, H. O. (2003). Primary culture of medaka (Oryzias latipes) testis: a test system for the analysis of cell proliferation and differentiation. Cell Tissue Res. 313, 107–115. doi: 10.1007/s00441-003-0729-x
Spurr, A. R. (1969). A low-viscosity epoxy resin embedding medium for electron microscopy. J. Ultrastruct. Res. 26, 31–43. doi: 10.1016/S0022-5320(69)90033-1
Sudhakumari, C. C., and Senthilkumaran, B. (2013). “Expression profiling of various marker genes involved in gonadal differentiation of teleosts: molecular understanding of sexual plasticity,” in Sexual Plasticity and Gametogenesis in Fishes, ed B. Senthilkumaran (New York, NY: Nova Biomedical), 401–421.
Swapna, I., Rajasekhar, M., Supriya, A., Raghuveer, K., Sreenivasulu, G., Rasheeda, M. K., et al. (2006). Thiourea-induced thyroid hormone depletion impairs testicular recrudescence in the air-breathing catfish, Clarias gariepinus. Comp. Biochem. Physiol. 144A, 1–10. doi: 10.1016/j.cbpa.2006.01.017
Thakur, M., Gupta, H., Singh, D., Mohanty, I. R., Maheswari, U., Vanage, G., et al. (2014). Histopathological and ultra structural effects of nanoparticles on rat testis following 90 days (Chronic study) of repeated oral administration. J. Nanobiotechnol. 12, 42. doi: 10.1186/s12951-014-0042-8
Thompson, J., and Bannigan, J. (2008). Cadmium: toxic effects on the reproductive system and the embryo. Reprod. Toxicol. 25, 304–315. doi: 10.1016/j.reprotox.2008.02.001
Tokarz, J., Möller, G., Hrabě de Angelis, M., and Adamski, J. (2015). Steroids in teleost fishes: a functional point of view. Steroids 103, 123–144. doi: 10.1016/j.steroids.2015.06.011
Tremblay, J. J., and Viger, R. S. (2003). Novel roles for GATA transcription factors in the regulation of steroidogenesis. J. Steroid Biochem. Mol. Biol. 85, 291–298. doi: 10.1016/S0960-0760(03)00211-5
Varanka, Z., Rojik, I., Varanka, I., Nemcsók, J., and Abrahám, M. (2001). Biochemical and morphological changes in carp (Cyprinus carpio L.) liver following exposure to copper sulfate and tannic acid. Comp. Biochem. Physiol. 128C, 467–478. doi: 10.1016/s1532-0456(01)00166-1
Vigneshvar, S., Sudhakumari, C. C., Senthilkumaran, B., and Prakash, H. (2016). Recent advances in biosensor technology for potential applications - an overview. Front. Bioeng. Biotechnol. 4:11. doi: 10.3389/fbioe.2016.00011
Wang, J. J., Sanderson, B. J., and Wang, H. (2007). Cyto- and genotoxicity of ultrafine TiO2 particles in cultured human lymphoblastoid cells. Mutat. Res. 628, 99–106. doi: 10.1016/j.mrgentox.2006.12.003
Wani, A. A., Sikdar-Bar, M., and Khan, H. A. (2013). Acute toxicity of copper sulphate to African catfish, (Clarias gariepinus). GERF Bull. Biosci. 4, 14–18.
Wani, M. Y., Hashim, M. A., Nabi, F., and Malik, M. A. (2011). Nanotoxicity: dimensional and morphological concerns. Adv. Phys. Chem. 2011:450912. doi: 10.1155/2011/450912
Yah, C. S., Simate, G. S., and Iyuke, S. E. (2012). Nanoparticles toxicity and their routes of exposures. Pak. J. Pharm. Sci. 25, 477–491.
Zhang, Q. F., Li, Y. W., Liu, Z. H., and Chen, Q. L. (2016). Reproductive toxicity of inorganic mercury exposure in adult zebrafish: histological damage, oxidative stress, and alterations of sex hormone and gene expression in the hypothalamic-pituitary-gonadal axis. Aquat. Toxicol. 177, 417–424. doi: 10.1016/j.aquatox.2016.06.018
Keywords: spermatocytes, qPCR, histology, transmission electron microscopy, testis primary culture, cytotoxicity, copper nanoparticle
Citation: Murugananthkumar R, Rajesh D and Senthilkumaran B (2016) Copper Nanoparticles Differentially Target Testis of the Catfish, Clarias batrachus: In vivo and In vitro Study. Front. Environ. Sci. 4:67. doi: 10.3389/fenvs.2016.00067
Received: 04 August 2016; Accepted: 03 October 2016;
Published: 02 November 2016.
Edited by:
Abdel-Tawab H. Mossa, National Research Centre, EgyptReviewed by:
Srikanth Koigoora, University of Aveiro, PortugalAtef Mohamed Khedr Nassar, Damanhour University, Egypt
Copyright © 2016 Murugananthkumar, Rajesh and Senthilkumaran. This is an open-access article distributed under the terms of the Creative Commons Attribution License (CC BY). The use, distribution or reproduction in other forums is permitted, provided the original author(s) or licensor are credited and that the original publication in this journal is cited, in accordance with accepted academic practice. No use, distribution or reproduction is permitted which does not comply with these terms.
*Correspondence: Balasubramanian Senthilkumaran, bsksl@uohyd.ernet.in; senthilkumaranb@yahoo.com
†Present Address: Desapogu Rajesh, Department of Electronic Engineering, National Chiao Tung University, Hsinchu, Taiwan