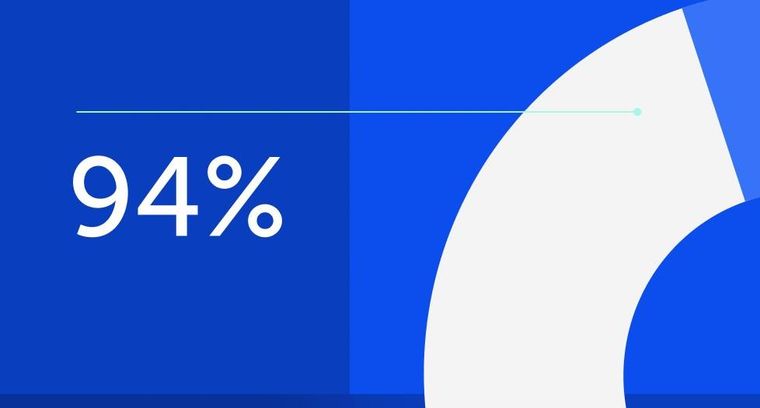
94% of researchers rate our articles as excellent or good
Learn more about the work of our research integrity team to safeguard the quality of each article we publish.
Find out more
REVIEW article
Front. Environ. Sci., 20 May 2015
Sec. Interdisciplinary Climate Studies
Volume 3 - 2015 | https://doi.org/10.3389/fenvs.2015.00038
Mountain hydrology, in particular in the European Alps, has undergone significant changes within the last decades due to climate and land use change as well as altered water consumption patterns. Climate change influences both the characteristics of droughts and floods as well as evapotranspiration, sublimation, snow-rainfall ratios, snow seasonality, and water reserves locked in glaciers. Land use change and altered water use may strongly outweigh these impacts, in particular through industrialization, urbanization, and tourism. Extreme hydrological situations such as new floods types have evolved from combined land-use and climate change and new types of water scarcity in association with accelerated and seasonally shifted water abstraction. Related water quality and pollution issues are of growing concern especially in seasonally highly populated areas. Main methodological challenges include keeping pace with recent hydrological change such as altered water inputs, water abstraction, and water quality. Emphasis should be put on the significance of small proportional changes within the total water cycle as these may have major impacts on floods, water scarcity, and general livelihood. Political challenges are strongest concerning problem reporting, initiation of monitoring programmes, and data transparency. The general lack of higher altitude hydrological data and experience with new hydrological phenomena will require an analytical approach directed more strongly toward interactions between scientists, stakeholders and decision makers encompassing local stakeholder knowledge and historical evidence. Data and innovative practices need to be exchanged more strongly between alpine regions and between the local and European level. It should be recognized that an alternative water management in mountains is fundamental for the future.
Water in mountains is a vulnerable resource that is strongly influenced by global change, especially by climate change but also by changing human behavior in different environments and over different time scales. This article focuses specifically on the European Alps due to availability of case studies and general information on highly modified catchments. Major mountain regions around the globe influenced to a varying degree by both climate and anthropogenic pressures include the Andes, Rockies, Himalayas, High Atlas, Urals, Caucasus, Altai, Carpathians, Scandes, and Pyrenees. Of these, the Alps are the mountainous zones that are most strongly influenced by urbanization due to their proximity to major agglomerations, the evolution of secondary homes, tourism resorts, and industry. Whereas, hydropower has been developed in most of these mountains ranges, the Alps have been foreriders for winter sports development and their prototype has radiated out toward many other mountain ranges worldwide. Such developments are increasingly transplanted into zones without sufficient natural snow and/or semi-arid climates leading to local and regional water-overuse. Detailed hydrological studies by scientists in other modified mountain zones are still pending.
It is generally agreed that the hydrological cycle has intensified in response to an alternating, step-wise change in global warming (Provenzale and Palazzi, 2014). As temperatures are increasing strongly over the Alps, there is a general decline in both duration and total accumulation of snow, especially at altitudes below 1500 m (Beniston, 2012). Simultaneously, there is a decreasing trend of the snowfall rainfall ratio, snow water equivalent, and snow depth during the melt season (Serquet et al., 2011; Marty and Meister, 2012). Even at higher altitudes, there is thus a clear trend toward more rain and less snow. Water reserves stored in glaciers have approximately halved since the end of the Little Ice Age and are projected to decrease by another two-thirds by 2100 due to glacier retreat in Switzerland (BAFU, 2012). For the Swiss Alps, glacierised basins are predicted to experience first increased runoff over the next few decades followed by decreased runoff, especially in the summer months (Pellicciotti et al., 2014). Rapid glacier decrease is already causing concern over drinking water supply in some smaller communities situated in inner alpine dry valleys (Gremaud and Goldschneider, 2010).
However, a major limitation for mountain hydrology is the predominant focus of classical literature on the hydrology of natural catchments. Due to the multitude of issues involved, few studies are available on the regional water balance of intensely modified catchments such as those impacted by artificial snowmaking and winter tourism (de Jong, 2009, 2013a,b; Vanham et al., 2009; Weingartner et al., 2014). Climate warming has complex impacts on mountain hydrology many of which are not yet known or adequately predictable especially when considered together with rapidly changing land-use. Whilst the dominant focus in mountain hydrology is on floods, droughts are not adequately considered. Nevertheless, statistical and dynamical downscaling of precipitation from regional climate models over the European Alps indicate that there is a strong trend toward drier conditions including a higher drought frequency and longer periods of drought (Schmidli et al., 2007; Gobiet et al., 2014). In the few hydrological studies available, droughts are investigated in mountain ranges with little human impact, such as the Cascades, Oregon (Nolin, 2012) or associated only with the forelands of mountain chains such as the Andes (Vergara et al., 2011). Increase in occurrence of low flows due to climate change in Canadian mountain basins are mentioned in Rasouli et al. (2014) and for American mountain basins in Coopersmith et al. (2014). The latter conclude that there is systematic drying of southern Appalachian basins but that for the Rockies precipitation remains steady with an overall declining snowpack. However, decreased streamflow in the Pacific Northwest United States is attributed to a decrease in mountain precipitation rather than increase in temperature (Luce et al., 2013).
Depending on the predominant geological setting, mountain hydrology can be particularly vulnerable because of its uneven distribution across catchments and because of the rapid flow response time which can produce both droughts and extreme floods over relatively short time periods. This is especially true for karst (Mathevet et al., 2004) and schist environments. Above all, the quality and quantity of mountain water is strongly influenced by upstream land use, the limited potential of subsurface water storage, precipitation, snowmelt, temperature, evapotranspiration, sublimation, and surface permeability (de Jong et al., 2009). Superimposed on strong daily and seasonal variability, the impacts of climate change on hydrology are already evident and will most likely intensify in future (Trenberth, 2011). Apart from climate change and associated land use change, direct anthropogenic influences have increasingly marked effects on water availability. Increased water demand can be associated with hydropower, tourism, artificial snow making, secondary housing, new lifestyles, and (mal)adaptation to summer droughts (de Jong, 2009). Simultaneously, land use change such as increase in forest cover due to abandonment of pasture has reduced discharge from springs, torrents and rivers and increased evapotranspiration (Dumas, 2011; Van den Bergh et al., 2014).
Flood characteristics are changing too. Claps (2010) and Allamano et al. (2009) have noticed a distinct (threefold) increase in the unit flood discharge in 27 mountain basins in southern Switzerland since the 1970s. According to Allamano et al. (2009), in future the 100 year flood in Switzerland will experience a 5-fold increase in frequency assuming scenario with a 2°C increase in temperature. The flood recurrence interval will be reduced to once in every 20 years. Increased frequency of intense precipitation (and resulting floods) experienced in the Alps over the last 40 years is producing more damage and casualties when coupled with increasing urbanization and river rectification (Faccini et al., 2014). Growing urbanization not only leads to an increase in impermeable surfaces at the cost of floodplains but also encourages related engineering works. For example, there has been a 50–75% reduction in bridge width since the Nineteenth century in many Italian alpine and pre-alpine rivers (Faccini et al., 2014) increasing the risk of jams, new flood routing, and bridge destruction. This has been observed for other alpine rivers as well. Widespread loss of river floodplain accentuates flow velocity and flood peaks due to the absence of meanders, flow expansion zones, vegetation and other obstacles ensuring flow retardation or buffering. Only an estimated 5–10% of floodplain surface is still available in alpine rivers (Litschauer, 2014) and 10–20% in Switzerland (SAEFL, 2004). Floods with the same discharge have therefore become more devastating nowadays than compared with 100 years ago. Moreover, a loss of floodplain decreases social resilience i.e., the capacity of a system to recover from an external shock to floods (Liao, 2012). The common emphasis is on technical resistance to floods encouraging so-called flood resistant design (such as dams, dikes, and canals), leaving only a small margin of acceptable risk and a false sense of security to society. Such flood design is geared toward a flood with a certain recurrence interval, commonly 100 years as determined by state law. When a larger flood than designed for occurs, resilience is often very poor due to lack of preparedness. Those regions that have created a mix between social and technical resilience to floods have a higher margin of acceptable risk and are better off during extreme events. In future, it would be recommendable to increase flood resilience by increasing flood and social buffering zones in view of larger and more frequent floods (Liao, 2012). Building resilience in cooperation with stakeholders pays off the political and economic risk in the long term (Dufty, 2012).
A major challenge remains understanding the impacts of land-use change such as bridges, roads, canalization, rectification and damming of rivers on the local and regional flood hydrology. Many new constructed phenomena in the landscape (such as tunnels, quarries, ski slopes etc) influence flood routing, velocity, and magnitude. Coupled with unusual meteorological phenomena (such as a rapid switch-over from precipitation to snowmelt on unusually warm soils) and extreme precipitation from local sub-catchments, new types of flash floods are created whose results may be unforeseeable.
On the other hand, the seasonal decrease in precipitation observed in many parts of the Alps (Casty et al., 2005) is translated into more droughts and an increase in low flows of streams and rivers (EEA, 2009; de Jong and Biedler, 2012; de Jong, 2013a). For example, during the last 500 years, the five hottest summers in Europe, including the Alps, were all recorded after 2001 (Barriopedro et al., 2011). The annual number of dry days is directly linked to this change. In the region of Piedmont, Italy, there has been an increase of 15 dry days since 1960, from 215 to 230 on average annually (ARPA, 2011)1. This corresponds to one extra dry day every 3 years. This rhythm is very similar for the French Alps (Casty et al., 2005).
As mountain water resources can become seasonally scarce, water quality may suffer. Still, water quality and water pollution are not frequently associated with mountain hydrology. Due to the vulnerable and episodic nature of water resources in many mountain catchments, water pollution is becoming a rising issue when impacted by land-use change, such as urbanization, road infrastructural development, tourism, and artificial snowmaking. This includes the impacts of fuel from cars, snow grooms and their fueling stations on ski runs and contaminated water from ski resorts and artificial snow from ski runs.
The general lack of higher altitude hydrological and meteorological data at the basin scale in mountains and the lack of experience with new hydrological phenomena will require an analytical approach directed more strongly toward interactions between scientists, stakeholders and decision makers. Local stakeholder knowledge and historical evidence will need to be systematically categorized using a scientific methodology based on intensive mutual exchange between scientists and mountain stakeholders. Water management should be recognized as a necessity encompassing all water uses regardless of their political and economic influence.
Therefore, the purpose of this article is to investigate advances and challenges of monitoring, predicting, and managing mountain water resources related to water quantity and quality in natural and modified catchments with special emphasis on local stakeholders and historical evidence.
Understanding the dynamics of mountain hydrology with relation to climate and anthropogenic change requires both scientific, anthropogenic, and historical evidence. Long-term hydro-meteorological data from measuring stations representative for specific catchments and altitudes should be supplemented by data on anthropogenic water abstraction. Current hydro-meteorological information from stakeholders and local newspapers should complement archives and historical documents. In future methodological approaches should encompass both natural and social sciences. This should span both climatology, hydrology, geography, engineering, agriculture, medicine, history, archeology as well as philosophy, sociology, anthropology, and psychology.
To begin with, a recurrent problem is the lack of higher altitude measuring stations providing precise short-term and long-term hydrological forecasting data (de Jong et al., 2005a). The lack in observational networks is due to logistical and financial reasons as well as lacking political will. Instead of increasing their density in data sparse areas many of the few existing measuring stations have been closed down in recent years in mountain areas. In the past, mountain settlements were built to survive hydrological extremes in the absence of (automatic) measuring stations. Inhabitants were far more autonomous and trained in observation and transmission of experience than nowadays. They were driven toward disaster resilience rather than resistance and in doing so their lifestyle was adapted to local conditions as far as possible. Geographical avoidance of disaster was important and risk tolerance was generally higher e.g., toward periodic flooding of fields or cellars or drying of springs. Citizens were sensitive to changing meteorological and hydrological conditions as well as natural risks and knew how to best evade them. Local inhabitants disposed of a high degree of flood preparedness and handling risk. If the risk became too high, they re-settled to safer areas. This is more difficult nowadays since the nature and scale of impact has changed and the individual sense of responsibility has decreased at the cost of centralized flood or drought governance.
Today, methodological approaches in mountain hydrology are much more focused on avoiding or reducing disaster through forecasting and protective engineering. Risk tolerance toward hydrological disasters has reduced together with the sense of personal responsibility in society for exposure to hazards such as floods and droughts. Methodological challenges are therefore strongly related to preventing, postponing, diverting, or storing water resources during floods or droughts. Forecasting and early-warning has become more centralized under the auspices of administration and government, demanding less involvement and responsibility from individual citizens. The emphasis has moved away from distributed, regional field observations to single automatic meteorological and river gauging stations accompanied by spatial information by remote sensing and hydro-meteorological modeling. It is aimed at enabling a fast and efficient prognosis of flood events or to create early warning against droughts.
At the same time, society is increasingly confronted with decision-making under the uncertainty of science, new anthropogenic pressures and incompleteness of technology (Noe, 2014) with regard to changing event characteristics. In recent years, climate adaptation experts have been required to include factors other than climate when considering the vulnerability of systems (Füssel, 2007 in Daron et al., 2014). Furthermore, Daron et al. (2014) state that “we cannot, and probably never will, accurately predict the future climate at regional and local scales, let alone predict the evolution of complex dynamic socio-ecological systems” and that in future approaches related to socio-ecological systems management decisions “cannot simply extrapolate from current practices which largely focus on data provision and a one-way flow of information.” Looking back in history at philosophical interpretations of disaster, the Japanese physicist and poet Terada (Terada, 1934 in Noe, 2014) observed nearly a century ago that “The more civilization progresses, the more the degree of severity of natural disaster increases.” and further that most disasters are man-made: “As civilization advanced, human beings gradually had an ambition to conquest nature. They built many kinds of structures which resist gravity, wind pressure and the power of water. When they start obstructing the violence of nature, nature eventually begins to rampage around like a troop of wild animals escaping from a cage. It is not unjust to say that the origin of such disaster is nothing but human beings' work to resist nature” (passage modified after Noe, 2014).
Efforts therefore need to be concentrated on ameliorating data and information relevant for hydrology where best available i.e., from medium altitudes (de Jong et al., 2005a). These should be compared where possible to higher altitude alpine stations in their vicinity according to the PUB (Predictions in Ungauged Catchments) approach (Whitfield et al., 2013). The PUB approach identifies catchments with similar characteristics (geology, geomorphology, vegetation, land use etc) in order to provide a comparison of possible hydrological behavior of ungauged or poorly gauged catchments with fully gauged catchments. Apart from these regional perspectives, the upcoming challenge is to distinguish between short term spatial and temporal hydrological fluctuations and longer term trends due to climate and anthropogenic change. Concerning droughts and water scarcity, the challenge is to provide more efficient measurement and estimation of evapotranspiration and sublimation (Körner, 2003; de Jong et al., 2005b, 2009).
In situations where hydrological and meteorological data is not available or too scarce, information from local newspapers and informed, local stakeholders, e.g., on the drying of wells, situations of water scarcity or the extent of flooding should be documented (de Jong, 2013a). Impacts of climate change and land-use change are being observed even in medium altitude mountains. Taking the example of the Chartreuse mountains, French Alps, local stakeholders in small hamlets are confronted not only with climate change, that modifies the quantity and seasonality of snow, but also with droughts and low flow conditions of higher frequency and longer duration coupled with an increase in household water consumption and a reduction in available discharge due to the encroachment of forest (de Jong, 2013a). Simultaneously, flood regimes are becoming more flashy.
For longer term reconstructions and dynamics, historical markers on buildings, paintings, photos, historical documents, articles, and chronicles should be studied (Faccini et al., 2014). Our understanding of impacts of hydrological extremes in mountains needs improvement. Certain floods are accelerated or even created by bottlenecks such as bridges and canals. These should be identified and described by local inhabitants and witnesses and fed into water management and risk plans. It is particularly important for stakeholders or observers to provide information on the exact timing of overbank flow or changes in flood routing.
In future, the challenge for floods is to improve understanding of infiltration characteristics on anthropogenically modified surfaces, especially in urbanized areas such as ski resorts and large industrial zones located in valleys. Infiltration is strongly modified in ski resorts, on ski runs, parking lots, roads and dirt roads frequently used by heavy machines and lorries. Results show that infiltration on ski runs located between 1800 and 2200 m in the French and Italian Alps can be 5–20 times lower than on natural soils on limestone, schist, and crystalline rocks, thereby substantially increasing surface run-off (de Jong et al., 2014). Despite re-greening, intensively compacted ski runs have been observed to remain 100% impermeable. Altered infiltration and extra water inputs by artificial snow melt not only affect the pattern and timing of daily and seasonal melt but also peak flood runoff (Figure 1) which can augment by up to 20% (de Jong and Barth, 2007).
Figure 1. Model of influence of artificial snow melt input (yellow line) superimposed on normal flood discharge (blue line) during an extreme rainfall flood reconstructed for 12th June 2003 for the torrent Villards, ski resort Les Arcs 1600, Savoy, France (de Jong and Barth, 2007). The flood peak is increased by approximately 18%. It is assumed that all natural snow has already melted.
One of the most difficult tasks in mountain environments is identifying, understanding, and quantifying anthropogenic water abstraction from agriculture, hydropower, industry, and tourism, including individual consumption, hotels, and artificial snow production. This requires a sound understanding of social behavior, which may be sporadic and independent of climatological and hydrological conditions. An interdisciplinary and inter-sectorial approach should be applied in order to understand and define the choices and issues surrounding water consumption and water management (de Jong, 2013a,b). This should include feedback from water agencies, politicians, farmers, fishers, foresters, tourism representatives, and teachers but also citizens (both permanent and those with secondary homes). Useful information can be obtained from those citizens directly impacted by water issues in particular those with a long experience, for example retired persons and mountain and outdoor guides etc. However, the more political water use becomes, the more difficult it is to obtain data.
Land-use and associated water use have considerably changed over the past century in the Alps. The most severe hydrological impacts are those associated with hydro-electricity, river straightening and winter and summer tourism. Of these, the fastest ongoing increase concerns water abstraction for production of snow for winter sports. Nevertheless, due to its political dimension, it is very difficult to obtain correct and up-to-date data on water abstraction and consumption for artificial snow making in the Alps. There is virtually no proxy information that could be used from other similarly intensively exploited ski resorts, with the exception of some water quality studies that are not readily transferrable to case studies in the Alps. For example; ski resorts in northern and northeast Finland have strong impacts on the water quality of boreal lakes, increasing nitrogen and phosphorous levels (Kangas et al., 2012) and ski resorts in Big Sky, Rockies, Montana have increased nitrogen loading in streams, in particular in winter, mainly due to septic and waste water inputs (Gardner and McGlynn, 2009). In eastern Serbia, ski resorts have triggered more frequent extreme events in the Zubska River headwater due to deforestation and enhanced surface-runoff on ski runs and access roads (Ristic et al., 2012).
In principle, all alpine and pre-alpine lakes are controlled at their outlets, either for optimizing lake levels for tourism or extracting water for artificial snow or hydro-electric production, including Lake Garda, Lake Como, Lake Maggiore and Lake Geneva as well as smaller lakes such as Lake Annecy and Lake Bourget (de Jong, 2013b). As a result, both the daily, monthly and annual water level fluctuations are smoothed. Water abstraction for hydropower and agriculture has a particularly strong influence on reservoir levels, groundwater and stream flow during droughts (Figure 2). This situation is amplified by lacking rainfall and snowfall inputs as well as high evaporation rates from the reservoir.
Figure 2. Impacts of the 2003 drought and water overuse on the Mis dam reservoir near Belluno, region Veneto, Italy. The reservoir is only filled to 5% of its capacity. Photo: Veccellio.
Apart from lakes, wetlands, and floodplains have also been the victim of river straightening and water diversions. In the Davos area, several water bodies have disappeared over the past 80 years, for example the large Palüda swamp (latin “paludosus” = swampy), the ice-skating pond at the confluence of the Flüela- and Sertig torrents, the floodplains around the River Landwasser (due to the diversion of Lake Davos water into the River Landquart, formerly flowing into the River Landwasser) and the high moor at the south end of Lake Davos (Stöckli, 2012). In ski areas, wetlands are most commonly destroyed or reduced in size due to construction of water reservoirs for snowmaking, drainage of ski runs, construction of parking lots and shopping areas (de Jong, 2013b). Snowmaking reservoirs either totally extinguish wetlands or greatly marginalize them, eventually drying them (Figure 3). Names such as Adret des Tuffes (= wetland) above $Les Arcs, 2000, French Alps (de Jong and Barth, 2007) are the only indicators remaining of their existence apart from aerial photos and satellite images when available at the right resolution.
Figure 3. Remnants of a wetland (2225 ma.s.l.) between a water reservoir for snowmaking and a ski run under the Roc du Diable above Belle Plagne near La Plagne Ski Resort, Savoie, France in 2011. The original wetland has been replaced by an impermeable, artificially regulated reservoir. Photo: de Jong.
Some river training works, diversions, and drainage schemes, in particular those in upper catchments and smaller torrents, rivers and wetlands have experienced a deterioration of their good ecological status and have been carried out against EU regulations such as the WFD (de Jong, 2014a).
Growing urbanization and industrialization in mountain areas not only has major impacts on surface and groundwater flow but also on water quality. Climate change increases water temperatures of different water bodies (SAEFL, 2004) and decreases water quantity in many regions, thereby increasing the risk of pollution and decreasing water quality. We are now confronted with a new speed and dimension of water pollution and the systematic measurement of water quality needs to be addressed. Mountainous terrain increases the costs of water pumping and treatment, therefore not all households or tourist amenities are joined to wastewater networks. In addition, water treatment plants are often under-dimensioned for cost-benefit reasons and thus cannot cope with the quantities of waste water produced by ten thousands of tourists in the winter season.
In alpine valleys, increased urbanization causes increased impermeabilisation of the ground, causing faster and more direct runoff into the canalization. Since the filtering effects of soils and vegetation is often reduced or missing, contaminants from diverse sources are either directly channeled away or when produced at higher altitudes can find their way into drinking water perimeters. The loss of wetlands aggravates this situation (de Jong, 2013b).
Pollution and water quality degradation is an issue that is rapidly evolving. Today, the principal challenge is locating and identifying sources of pollution. In mountain catchments water quality measurements are usually restricted for logistical and political reasons. Water pollution by substances such as bacteria (Lagriffoul et al., 2010), diesel oil, nitrogen, ammonium, and other heavy metals can both directly affect drinking water or water bodies in general. Pollution can be linked to tourism, agriculture, industry, and transport. Those regions that are dominated by winter sports and heavy seasonal tourist frequentation should include pollution by tourism as a separate category in water management plans. Pollution from winter tourism should be distinguished according to coli-bacteria, pH, conductivity, ammonium, nitrogen, and heavy metals from (1) pipelines for artificial snowmaking, (2) water storage reservoirs for snowmaking, (3) artificial snowmelt runoff from newly constructed and re-vegetated ski runs and from pipelines and outlets below ski resorts. In future, poor quality water that may develop during peaks of tourist frequentation during the winter season should be anticipated and treated.
Concerning transport, pollution inputs include diesel and oil from roads and small airports, diesel leaking from snow grooms in runoff from ski runs, diesel depots, and supply truck accidents. During the winter season 2014, a supply truck loaded with 18,000 liters of diesel plunged off the road at 1310 m altitude in the Zillertal, Tirol, Austria (Tiroler Tageszeitung, 2014). It was to deliver diesel for the middle ski station of the Zillertal Arena for the snow grooms. Although the diesel had to be unloaded into another truck and most of the leakage could be prevented, it is unlikely that none of it escaped into the snow and ground.
Pollution from industry and urbanization mainly concerns alpine streams and lakes as well as dam reservoirs. Alpine and sub-alpine lakes are particularly impacted since they act as final pollution traps. For example, the Lake Bourget has experienced a 1.8°C temperature increase in only 62 years between 1950 and 2012 and a precipitation decrease between 10 and 30% over the last 10 years (CISALB, 2012). The decrease in water volume has concentrated pollution mostly during low flow and drought periods (Serraz, 2012). In contrast, during heavy rainfall, there is a risk of development of cyanobacteria from overflow of waste water plants. Some pollutants can be remobilized after several decades when deposited in lake sediments. This has been the case for substances such as PCB from ancient factories causing a ban on the consumption of certain fish species (CISALB, 2012). Dam reservoirs are also more prone to pollution during droughts and low level periods. Increased temperature and reduced water dilution increase methane and algae production (Delsontro et al., 2010).
Other sources of pollutants can be released from runoff originating from rapid glacier ice melting in association with climate change and glacier retreat. Toxic substances deposited in glacier ice several decades ago (such as PCBs) can be released over relatively short periods of time during spring and summer melt runoff.
Water quality measurements can be wide-ranging and expensive and need to be preceded by a hypothesis of potential contamination. Methodologically this is complex because both historical data of contaminant sources as well as upcoming sources have to be considered. In order to derive pollution sources, information from local stakeholders is essential. Seasonality issues also need to be taken into account. Some pollution may be difficult to detect in winter when water is frozen and may only re-appear during the snowmelt or glacier melt phase. Sites may also be difficult to access due to topographic and weather conditions.
After identification of potential pollutant sources, a regionally and temporally representative campaign should be initiated. For large ski resorts, campaigns should be carried before during and after frequentation peaks and artificial snow production.
Flood characteristics have been observed to change in European mountain catchments, both due to climate as well as anthropogenic impacts. While climate change is influencing flood timing and magnitude as a result of changing precipitation and snow melt patterns, human impacts such as river rectification, damming and land use change is influencing flood routing, flood timing and flood magnitude and may outweigh natural impacts.
Urbanisation and land-use change can have major impacts on flood magnitude and routing. This requires careful monitoring of both land use changes in the upper catchment as well as modifications to the main river channels, constraining infrastructure such as bridges, extent of urbanization and impermeable surfaces, extend of floodable fan surfaces and amount of artificial water inputs. Apart from regularly monitoring of these changes it is also necessary to combine different potential scenarios in order to predict future situations. This requires joining knowledge from other events developed under similar conditions or in similar catchments. It is also necessary to add “soft” expert knowledge to existing models and monitoring results in order to attempt to predict the “unknown.” The expert knowledge should be closely supported by stakeholder accounts of extreme events and historical evidence from photos and paintings.
On the 10th/11th of October 2011, a devastating flood occurred in the River Kander in the canton of Bern, Switzerland following a prolonged rain/snow and intense snowmelt event whose impacts were amplified by road and river infrastructure. The flood resulted from two precipitation phases recorded over the Kander region: a first phase lasting for 3 days with approximately 100 mm of precipitation, 60–80% of which fell as snow and a second phase lasting for 2 days (10–11th October) with another 60–90 mm of precipitation accompanied by intensive snowmelt due to a rapid temperature increase to 10°C (Geo7, 2012; BAFU, 2014). Strikingly, in the sub-catchment of the Kander-Eggeschwand alone, 120 mm of precipitation were recorded during the first phase, increasing to 200 mm on the 10th of October. A record October peak discharge of 262 m3/s, equivalent to a 300 year flood, was measured at the gauging station of the River Kander at Hondrich 15 km further downstream (Badoux et al., 2013). This recurrence interval was extrapolated from a 107 year discharge series after the extreme value theory. Only 6 years before, a near identical peak discharge was reached at the same gauge. In future it would therefore be recommendable to present this event in terms of annual exceedance probability, i.e., 0.33% (Pe = 0.0033). In contrast, the precipitation only had a 10 year return period. Peak discharge at the gauging station was reached within 11 h and increased 15-fold to its pre-flood level (Figure 4). The obvious challenge is to correctly model the rising limb of such an extreme event (Badoux et al., 2013). Unlike, previous extreme floods such as in 2005, this flood had a very flashy regime, making it extremely difficult for the catchment to buffer the flow and for humans to prepare and react on time. During the rising flood limb the narrow bridge over the River Kander near Büel was blocked and the torrent subsequently followed a new path, flowing over an inclined agricultural surface around a farm, down a short and steep metaled road, along a main road, through the Mitholz avalanche protection tunnel only to reappear with full power in a hydraulic jump at the other end (Figure 5). The flood was able to gather momentum along its way since it had few obstructions and flowed over highly impermeable surfaces. Emerging from the avalanche tunnel, the flood caused large-scale erosion, destroying the road and flooding the urbanized areas of Mitholz (5 km below Kandersteg). As for other flood events, it would be important to define the exact timing of the new route into the tunnel flow from stakeholder feedback and relate this to the flood hydrograph, taking into account the time lag between this site and the stage gauge further downstream. One possibility is via the automatic radar installed in the tunnel that could detect the first water flow but it would be tedious to obtain the information e.g., via the traffic administrations or public questionnaires.
Figure 4. Comparison of the 2005 and 2011 extreme flood hydrographs (>100 year recurrence interval) in the River Kander, Switzerland at the Hondrich stage gauge [modified after BAFU (Federal Office for the Environment) 2014].
Figure 5. Reconstructed flood route of the River Kander, Switzerland for the extreme flood event of 10th/11th of October 2011. Due to obstruction of a bridge, the flood changed its flood route following road infrastructure and encroaching an anti-avalanche tunnel (de Jong, 2014b).
A recent debris flow/flood event in the torrent Saint-Antoine in Modane, Savoie, French Alps occurred between the 1st and 2nd of August 2014 only 27 years after a similarly destructive event. Local inhabitants noted a rapid sequence of debris flow pulses during the night (Briancon-Marjollet, 2014). During the flood, the industrial zone and houses that had been previously destroyed on the sedimentary fan during the 1987 flood event and reconstructed again were once more destroyed. Thus, the area impacted by the two major flood events was similar (Figures 6A,B). Local inhabitants had observed that the narrow bridge over the torrent on the lower fan was under-dimensioned and had already caused excessive flooding and flow deviation when obstructed in the past. Since the bridge was destroyed in the flood, it was decided not to rebuild it. This time all buildings on the floodable zone of the fan will be deconstructed. With over 55 check dams in the upper catchment (extending from 1100 to 3086 m), it is questionable to what extent flood protection was effective. Most probably, these check dams were filled close to capacity with sediments and were therefore ineffective during the event. As is the case in most parts of the Alps, maintenance of check dams has greatly declined since the 1950s, thus causing them to become structurally weakened and congested with sediments. Whilst the flood/debris flow of 1987 transported 80,000 m3 of sediments in only 30 min, the 2014 event had the same duration but transported somewhat less (between 40 and 60,000 m3) because of a gravel trap constructed upstream of the fan (Gominet, 2014). The recurrence interval of the first event is estimated at 100 years, and the second at 30–50 years.
Figure 6. Flood/debris flow in the torrent Saint-Antoine in Modane, Savoie, France destroying approximately the same urbanized area on (A) 24th August 1987. (Photo: Jean-Marie Jeudy) and (B) on 1st August 2014. Photo: Alain Favre.
Other challenges include changing flood regimes from dam water release which affects about 28% of alpine rivers or 16,000 km of 58,000 km rivers assessed in the Alps (Litschauer, 2014). In many alpine rivers with hydro-power, hydro-peaking (the periodic, mostly daily release of water from dams for hydro-electric production) causes extremely sudden and regular daily flood peaks, which can be dangerous for local stakeholders since the rivers are otherwise dry or carry low flow. On the River Arc, Savoy, French Alps, for example, annual dam release is estimated to be the equivalent of a 1-year flood (Jaballah and Jodeau, 2012). On the other hand, flood release due to a combination of heavy precipitation and large storage in dam reservoirs can significantly augment the flood peak and create an artificially destructive flood. Even though it may involve only a relatively small increase in the percentage of the discharge, the effects can be disastrous due to heavy additional flooding and destruction. In addition, extreme floods (with a 100 year R.I.) produced below dams are more likely to flood and erode nowadays than in the pre-dam era due to altered bed morphology resulting from a lack in bedload transport (DDTS, 2013).
Water scarcity evolving both due to climate change and human impacts has accelerated over the last 15 years. It has neither kept springs, torrents, rivers, lakes, wetlands nor groundwater untouched. For example, the alpine country Steiermark (Austria) was confronted with such severe water scarcity after prolonged summer drought in July 2013 that wells had to be manually re-filled with water (Steininger et al., 2015).
Lake water volumes are another important issue. Lake levels over the past 10–15 years are increasingly controlled throughout the Alps for artificial snowmaking with obvious hydrological impacts. The water level of Lake Davos at an altitude of 1560 m in Grisons, Switzerland, is lowered by up to 28 m for artificial snow production during the winter season (Stöckli, 2012 and Figures 7A,B) corresponding to more than 50% of its total depth. This is because the Parsenn and Jakobshorn ski areas consume approximately 600,000 m3 of water for snow production together annually. Although this is the equivalent of only 0.2% of the annual water cycle it represents 33% of the annual drinking water. The abrupt lowering of the lake level independent of seasonality has caused the disappearance of Sparganium angustifolium that normally grows along the shoreline of ponds and lakes. Since the lake is already subject to annual lowering for hydropower since 1937, this species is considerably endangered. Such practices shed considerable doubt on the sustainability of mountain water.
Figure 7. Comparison of water levels of Lake Davos, Grisons, Switzerland (A) near maximum after the summer season on 11th October 2009 and (B) after massive lowering following water withdrawal for snowmaking during the winter season on the 8th February 2010. The lake is lowered by up to 28 m during winter. Copyright Stöckli (2012).
In 2014, water abstraction was allowed from Lake Montriond situated at 1072 m within the Geopark Chablais, Upper Savoy, France for snowmaking in the ski resort of Avoriaz. Water is pumped to higher elevations between 1100 and 2466 m. According to the private water companies and snow production companies, the lake level will drop by only 15–20 cm (for an average water abstraction of 120,000 m3 per year) and up to approximately 40 cm for droughts with an estimated 5 year recurrence interval (Alpes Ingé et al., 2013). This lowering can have considerable effects during low discharge periods in winter and during droughts since the natural lake level variations would be increased by a factor of 2. Additional pressures on lake water volume from increased evaporation during droughts are not taken into account in these projections. Avoriaz is a ski resort that already suffers from water scarcity due to seasonal water overuse for snowmaking. As for many other alpine ski resorts, water abstraction for snowmaking causes further anxiety as the recent development of large water worlds with swimming pool complexes are superimposed on rising drinking and household water use. Despite such concerns, the expansion of artificial snow making in alpine ski resorts is not limited relative to water availability in catchments and the problems are further intensified by long-distance pumping from lower altitudes or adjacent catchments (Le Messager, 2014).
For other lakes the inverse is true. For example, the water level of Lake Moro situated at 2235 m altitude in the ski area of Foppolo-Corona in the Bergamo Alps, Italy has been increased by approximately 2 m through the construction of a small dam. Approximately 60,000 m3 of water are thus stored for snow production. The decreased discharge into the surface and sub-surface below its outlet due to flow diversion into the other catchments may well endanger the wetlands downstream. Alpine lake levels may also simply be controlled to ensure high enough water levels for lake tourism, e.g., Lake Annecy or Lake Bourget. In the meantime it has been recognized that the dampening of annual water level fluctuations (for example 30 cm instead of 1.75 m in Lake Bourget) has major repercussions on aquatic species, leading to the near disappearance of reed (de Jong, 2013b).
Unfortunately, the environmental impacts of lake control for snowmaking at relatively high altitudes (mostly between 1500 and 2500 m altitude) has not been directly addressed or integrated into water management plans. Economic interests are usually very strong and water is controlled by private companies. Environmental impacts assessment is both commissioned and carried out by private companies and/or the snow producing companies themselves (Alpes Ingé et al., 2013). Scientific studies are missing and there are no precise and continuous measurements of water abstraction.
Alpine lake level control for hydropower has a longer history—e.g., Lake Aiguebelette in Savoie or Lake Paladru in Isere, whose water level can be lowered by up to 3.7 m during droughts relative to an average lake depth of 25 m (SIBF, 2011).
Another issue is direct water abstraction from springs, streams and rivers for winter tourism. Water use for snowmaking as well as drinking and household water is increasing local and regional water stress in many ski resorts (de Jong, 2013a,b). In exclusive ski resorts such as Courchevel (France), water consumption can reach 850–1000 L per person per day for 4–5 star hotels due to water use for swimming pools, Jacuzzis, baths, water walls etc. This is approximately 6–10 times higher than the average water consumption for a normal household in industrialized Europe.
The Crans-Montana ski area situated in the dry, inner alpine valley of Wallis, Switzerland, is already under considerable water stress to supply its 200 snow guns for artificial snow production. From the beginning of December onwards, water demand (both for snowmaking as well as increased tourist drinking water) exceeds availability and by the end of December, water demand can reach up to double of that available (after the Intercommunal Master Plan 2008 in Bréthaut, 2012). Water therefore has to be transferred from a hydropower reservoir, springs, boreholes and water catchments to fulfill the seasonal needs of artificial snow production (Weingartner et al., 2014). It is questionable how sustainable this interbasin and interregional water transfer really is. For the drought year of 2011, water consumption for artificial snow in the Crans-Montan region made up 3% of the total water consumption excluding hydropower and 5% of drinking water (Weingartner et al., 2014). If tourist drinking water needs are considered together with artificial snow, the proportions are much more significant. As for other alpine ski resorts, a rapid expansion of snowmaking is envisaged. A “business as usual” expansion of winter sports would translate into nearly 80% increase in water consumption for artificial snow (Weingartner et al., 2014). Supplying sufficient water could be quite difficult to maintain given the existing long distance water transfers.
It is therefore important to reconsider the meaning of proportions in the context of local ecological values. Even a very low overall percentage of total water use at an inappropriate time of the year can have disastrous repercussions on the survival of water-dependent ecosystems since mountain water resources play a crucial spatial and temporal role (e.g., small wetlands, small streams). Inversely, the Crans-Montana study shows that the minimal residual water in torrents could be doubled in a “moderation scenario,” corresponding to a regional population decrease, a qualitative new orientation of tourism without artificial snow production, an overall decrease in winter tourism and development of soft tourism (Weingartner et al., 2014). This demonstrates how sensitive ecosystems can be to very small proportional changes in water use. The authors conclude that present management of surface water quantity in their alpine catchment is least sustainable followed closely by weak resource efficiency, justice and willingness to learn.
It can be observed that many recent water issues related to the ski industry remain silenced both due to inadequate hydrological measurement as well as for political reasons. For example, in 2014, the Austrian ski resort of St. Johann, Pongau used three times the amount of water permitted in the year 2002 (42,000 m3 instead of 14,000 m3) for snowmaking as a reaction to climate change-induced natural snow deficits. This illegal water use was counteracted by partially closing the water pipeline for snowmaking (ORF, 2014). In the same year, the nature protection association Pro Natura filed a charge for illegal water use for snowmaking at the departmental police and water agency for the ski resort of Kleine Scheidegg-Männlichen, Berner Oberland, Switzerland (Der Bund, 2014). It has been confirmed that water has been withdrawn illegally for years from streams by the lift companies through fixed installations (Fritschi, 2015). Permissions for water abstraction were issued in 2008. Water demand for snow production in large ski resorts such as Kitzbühel, Austria (with more than 750 snow canons) has increased by approximately 30% annually between 2004 and 2010 (Bergbahnen Kitzbühel, 2012) and continues to do, reaching a projected four-fold increase of more than 2 million m3 in less than 10 years. Since other ski resorts have similar projected increases in water demand, it is important to integrate this water use in water management plans.
It is interesting to follow the political evolution of the analysis of Water in the Alps between 2008 and 2014 from Alpine Convention reports concerning sensitive issues such as water use and water quality in ski areas. Whereas, the 2008 report (Alpine Convention, 2008) authors hydrologists from water agencies to summarize present and possible future challenges, the 2010 report (Alpine Convention, 2011) omits such experts and addresses a reduced set of issues mainly from a political point of view.
While the 2008 report details seasonal problems of water quality related to ski resorts, the 2010 no longer addresses this issue: “Point sources of pollution linked either to local communities or to industrial sites have given rise to important investments in sewage collection and treatment as required by legislation, in particular the European directives. Still, not all facilities have been completed or they do not always allow to attain the necessary degree of treatment. Ski resorts, for example, are behind schedule. They are confronted with seasonal pollution peaks at times when water levels are low in the receiving water bodies.” (Bouffard, in Alpine Convention, 2008 report).
Not surprisingly, the 2010 report contradicts both itself and the 2008 report in terms of artificial snow-related water problems and the need for water management. For example “Identified regional and local water-related problems such as water scarcity due to snow making in winter or low water levels left due to the use of hydropower are issues for sound local or regional water resources management” (Grambow in Alpine Convention, 2008 report) against “Artificial snowmaking has been addressed by just one country (Italy) as a relevant issue, whereas for four countries this is not deemed a relevant water management issue at regional and national level” (p. 69 in the 2010 report). This is contradicted later in the same report by “…. water requirements for snowmaking can be substantial at a local level, using a considerable share of the annual water abstraction and can lead to water conflicts especially in the winter season in areas where snowmaking stations are connected to the drinking supply network; this can cause temporary water shortages.” (p. 92, 2010 report) and again by “However, from the point of view of the regional and Alpine-wide water resource balance, artificial snowmaking is not a significant issue.” (p. 92, 2010 report). The inconsistency between the existence of water stress/scarcity and its apparent insignificance within the water cycle resurges several times in the report: “Even if considering the water cycle for the entire Alpine region the water volumes used for artificial snowmaking are insignificant … ” against “In fact, artificial snowmaking can imply temporal water stress rising conflicts among users… ” (p. 49 in 2010 report). It is recognized that “… 3 the conflict with tourism and artificial snow making that has become a central issue” (p. 72, 2010 report).
Furthermore, in the 2008 report, Götz in Alpine Convention (2008) criticizes that “The EU Framework Directive does not include problems relating to artificial snow installations such as the impact of chemical snow-making additives on water quality or the tapping of water to create artificial snow in times of water shortage.” However, the 2010 report no longer addresses this issue. Such shortcomings have been mentioned by de Jong (2014a) proposing a new EU tourist directive that is to restrict increasing water use for tourist resorts and carefully monitor environmental impacts from an interdisciplinary point of view to avoid opposing the EU Water and EU Soils directive.
The reports for the 2012 and 2014 conferences are still pending, however there does not seem to be any specific treatment of water scarcity and winter sports. In the meantime, a report on sustainable tourism in the Alps has been published but it also neglects water quality issues related to ski resorts and only briefly addresses water quantity issues (Alpine Convention, 2013).
This evolution shows that the strongest political challenge concerns problem reporting and with this the initiation of monitoring programmes and data transparency. Given rising tensions on water availability, water abstraction for leisure activities should be regulated and restricted in future.
It is clear that the foremost challenges of mountain hydrology in the third millennia concern anthropogenic impacts and their interactions with climate change. The main issues are as follows:
- Changing flood characteristics due to land-use change and engineering structures and evolving water scarcity due to altered water abstraction need to be both recognized and predicted under climate change conditions.
- A holistic approach to mountain hydrology is necessary in the future, encompassing the state of springs, torrents, lakes and wetlands as well as evapotranspiration and sublimation in order to complement the present-day restrictive valley discharge approach.
- As long as local hydrographs reflecting antecedent conditions in higher altitude sub-catchments are not available, flood or drought prediction tools remain inadequate. Similarly, we have limited experience with new hydrological phenomena. Therefore, both scientific and societal knowledge should be included in measuring and modeling programmes with stronger interactions between stakeholders, scientists and decision-makers.
- It is essential to move away from the concept of percentages as an indicator of the total water cycle and instead recognize the relative significance and ecological meaning of water resources. Small percentages can be as consequential for local water scarcity and water conflicts as for increased flood discharge and damage.
- Causes of water quality deterioration and new pollution sources, especially from winter tourism and industry, should be established in close contact with local stakeholders where measuring networks are too sparse and stricter regulations established.
- A more stringent distinction should be made between water consumption and water use and its ensuing quality with the help of scientists before integrating into water management plans.
- Water management should be recognized as a necessity encompassing all water uses regardless of their political and economic influence. An alternative water management at the catchment and sub-catchment is essential in the future. Political challenges remain strongest with respect to problem recognition, reporting, initiation of monitoring programmes, and data transparency.
In future, simultaneous observation and measuring programmes, data and innovative practices need to be exchanged more strongly between alpine regions and between the local and European level to ensure a more sustainable use of mountain water.
The author declares that the research was conducted in the absence of any commercial or financial relationships that could be construed as a potential conflict of interest.
1. ^ARPA. (2011). Piemonte. http://www.arpa.piemonte.it. MeteoClima50, Long Term Trends in Precipitation.
Allamano, P., Claps, P., and Laio, F. (2009). Global warming increases flood risk in 10 mountainous areas. Geophys. Res. Lett. 36, L24404–L24411. doi: 10.1029/2009GL041395
Alpes Ingé Alp'Pagès My Neige, and Fafournoux, P. Y. (2013). SERMA - Commune de Montriond (74). Pompage Dans le lac de Montriond Pour la Fabrication de Neige de Culture (Pumping Water from Lake Montriond for Production of Artificial Snow). Programme de travaux d'enneigement, Etude d'impact. (Les Menuires: Work Programme for snowmaking, Impact study). 98.
Alpine Convention. (2008). “Water balance in the Alps,” in Proceedings of the 2nd Conference, Report on the State of the Alps Water (RSA II-Water) (Munich).
Alpine Convention. (2011). “Water in the alps; striking the balance,” in 3rd International Conference and Preparatory Workshops 2010 (Rome), 104.
Alpine Convention. (2013). Sustainable Tourism in the Alps. Report on the State of the Alps, Alpine Signals–Special Edition (Innsbruck), 4, 48.
Badoux, A., Hofer, M., and Jonas, T. (2013). Hydrometeorologische Analyse des Hochwasser-ereignisses vom 10 Oktober 2011. (Birmensdorf: WSL; Davos: SLF; Zürich: MeteoSchweiz; Bern: Geo7; Bern: BAFU), 94.
BAFU. (2012). “Auswirkung der Klimaänderung auf Wasserressourcen und Gewässer (Impacts of Climate Change on Water Ressources and Water Bodies),” in Synthesis Report for the Project “Klimaänderung und Hydrologie in der Schweiz” (Climate Change and Hydrology in Switzerland) (CCHydro). Bern: Bundesamt für Umwelt (Federal Office for the Environment), Umwelt-Wissen Nr. 1217, 76.
BAFU. (2014). Hochwasserereignis vom 10./11. Oktober 2011 (The flood event of 10th/11th October 2011), Hydrologischer Spezialbericht des Bundesamtes für Umwelt (Special hydrological Report for the Federal Office of the Environment), Report, BAFU (Federal Office for the Environment) Available online at: http://www.bafu.admin.ch/hydrologie/01834/02041/11745/index.html?lang=de
Barriopedro, D., Fischer, E. M., Luterbacher, J., Trigo, R. M., and Garcia-Herrera, R. (2011). The Hot Summer of 2010: Redrawing the Temperature Record Map of Europe. Science 332, 220–224. doi: 10.1126/science.1201224
Beniston, M. (2012). Focus Article. Is snow in the Alps receding or disappearing? WIREs Clim. Change 3, 349–358. doi: 10.1002/wcc.179
Bergbahnen Kitzbühel (2012). Geschäftsbericht 2010/11 (2010/11 Working Report of the Kitzbühel Lift Companies) (Kitzbühel).
Bréthaut, C. (2012). Analyse Comparée des Régimes Institutionnels des Services Urbains de l'eau dans les Stations Touristiques: Le cas de Crans-Montana (Suisse), une Illustration du Modèle de gestion “Public Local Fort” (A Comparative Analysis of the Institutional Regimes of Urban Water Services in Ski Resorts: The Case of Crans-Montana (Switzerland), an Illustration of a “Local Strong Public” Management Model). (Mondes du tourisme), 106–108.
Briancon-Marjollet, A. (2014). Modane, Trois Mois Après la Crue (Modane, 3 Months After the Flood). Dauphiné Libéré (Grenoble: Dauphiné Libéré).
Casty, C., Wanner, H., Luterbacher, J., Esper, J., and Böhm, R. (2005). Temperature and precipitation variability in the European Alps since 1500. Int. J. Climatol. 25, 1855–1880. doi: 10.1002/joc.1216
CISALB. (2012). Comité Intersyndical Pour l'assainissement du lac du Bourget (Intercommunal Syndicate for the Water Sanitation of the Lake Bourget). Available online at: http://www.cisalb.com/main.php
Claps, P. (2010). “European Directives and flood evaluation methods in alpine environments,” Third Alpine Water Conference, Preparatory Workshop n. 1 (Trento).
Coopersmith, E. J., Minsker, B. S., and Sivapalan, M. (2014). Patterns of regional hydroclimatic shifts: an analysis of changing hydrologic regimes. Water Resour. Res. 50, 1960–1983. doi: 10.1002/2012WR013320
Daron, J. D., Sutherland, K., Jack, C., and Hewitson, B. C. (2014). The role of regional climate projections in managing complex socio-ecological systems. Reg. Environ. Change 15, 1–12. doi: 10.1007/s10113-014-0631-y
DDTS. (2013). Direction Départementale des Territoires de la Savoie, Service Sécurité Risques, Unité Risques, Plan de Prévention des Risques Inondation de l'Arc, Tronçon d'Aiton à St. Marie de Cuines (19 communes), Ministere de l'écologie, du Developpement Durable et de L'energie, Report. (Departmental Directorate of the Territories of Savoie, Risk Security Service, Risk Unit, Plan for Flood Risk Prevention of the River Arc, Reach from Aiton to St. Marie de Cuines, 19 Communities, Ministry for Ecology, Sustainable Development and Energy). (Chambery: Direction Départementale des Territoires de la Savoie).
de Jong, C. (2009). Chapter 5.5. “Savoy – balancing water demand and water supply under increasing climate change pressures” in Regional Climate Change and Adaptation. The Alps facing the challenge of changing Water Resources. EEA (European Environment Agency) report, N. 8, 81–84.
de Jong, C. (2013a). Linking ICT and society in early warning and adaptation to hydrological extremes in mountains. Nat. Hazard. Earth Syst. Sci. 13, 2253–2270. doi: 10.5194/nhess-13-2253-2013
de Jong, C. (2013b). (Über)Nutzung des Wassers in den Alpen (Water (Over)use in the Alps). Jahrbuch Verein zum Schutz der Bergwelt 78, 19–44.
de Jong, C. (2014a). “A white decay of winter tourism. climate change adaptation manual,” in Lessons Learned from European and Other industrialized Countries, eds A. Prutsch, S. McCallum, T. Grothmann, R. Swart, I. Schauser, and R. Swart (London; New York, NY: Routledge), 226–233.
de Jong, C. (2014b). “The role of society in flood risk assessment and disaster resilience in mountains,” in International Innovation Workshop in Tsunami, Snow Avalanche and Flash Flood Energy Dissipation, Conference Proceedings (University of Tohoku and University of Lyon, Chamonix), 5–15.
de Jong, C., and Barth, T. (2007). “Challenges in hydrology of mountain ski resorts under changing climate and human pressures. ESA,” Proceedings of the 2nd Space for Hydrology Workshop, “Water Storage and Runoff: Modeling, In-Situ data and Remote Sensing” (Geneva).
de Jong, C., Carletti, G., and Previtali, F. (2014). “Assessing impacts of climate change, ski slope, snow and hydraulic engineering on slope stability in ski resorts (French and Italian Alps),” in Engineering Geology for Society and Territory - Climate Change and Engineering Geology, eds G. Lollino, A. Manconi, J. Clague, W. Shan, and M. Chiarle (Springer: Cham), 51–55.
de Jong, C., Lawler, D., and Essery, R. (2009). Mountain Hydroclimatology and Snow seasonality—perspectives on climate impacts, snow seasonality and hydrological change in mountain environments. Hydrol. Process. 23, 955–961. doi: 10.1002/hyp.7193
de Jong, C., Mundelius, M., and Migala, K. (2005b). “Comparison of evapotranspiration and condensation measurements between the Giant Mountains and the Alps,” Climate and Hydrology in Mountain Areas, eds C. de Jong, D. Collins, and R. Ranzi (Chichester: J. Wiley & Sons), 161–184.
de Jong, C., Whelan, F., and Messerli, B. (2005a). The importance of a hydrological research framework for water balance studies in high mountain basins: Special Issue Mountain Hydrology. Hydrol. Process. 19, 2323–2328. doi: 10.1002/hyp.5886
Delsontro, T., McGinnis, D. F., Sobek, S., Ostrovsky, I., and Wehrli, B. (2010). Extreme Methane Emissions from a Swiss Hydropower reservoir: contribution from bubbling sediments. Environ. Sci. Technol. 44, 2419–2425. doi: 10.1021/es9031369
Der Bund (2014, November 15). Anzeige wegen illegaler Wasserentnahme. Im Skigebiet Kleine Scheidegg-Männlichen wird Illegal Wasser für die Beschneiung Genutzt. (Charge for Illegal Water Use. In the Ski Resort Kleine Scheidedd-Männlichenwater is Beiung Used Illegally for Artificial Snow Production), Der Bund. Available online at: http://www.derbund.ch/bern/kanton/Anzeige-wegen-illegaler-Wasserentnahme------/story/29904590
Dufty, N. (2012). Using social media to build community disaster resilience. Aust. J. Emerg. Manage. 27, 40–45.
Dumas, D. (2011). The impact of forests on the evolution of water resources in the mid-altitude Alps from the middle of the 19th Century (Chartreuse massif, France). J. Alp. Res. 99, 2–14. doi: 10.4000/rga.1632
EEA. (2009). Water Resources Across Europe—Confronting Water Scarcity and Drought, eds R. Collins, P. Kristensen, and N. Thyssen (Copenhagen: European Environment Agency Report Nr. 2), 60.
Faccini, F., Luino, F., Sacchini, A., and Turconi, L. (2014). “Flash flood events and urban development 155 in Genoa (Italy): Lost in Translation,” in Engineering Geology for Society and Territory, Urban Geology, Sustainable Planning and Landscape Exploitation, Vol. 5, eds G. Lollino, A. Manconi, F. Guzetti, M. Culshaw, P. Bobrowsky, and F. Luino (Cham: Springer), 797–801.
Fritschi, H. (2015). Für den Beschneiungswahn werden Bäche angezapft (Torrents are tapped for snowmaking madness), Pro Nat. Mag. 2, 23.
Füssel, H. M. (2007). Vulnerability: a generally applicable conceptual framework for climate change research. Glob. Environ. Change 17, 155–167. doi: 10.1016/j.gloenvcha.2006.05.002
Gardner, K. K., and McGlynn, B. L. (2009). Seasonality in spatial variability and influence of land use/land cover and watershed characteristics on streamwater nitrate concentrations in a developing watershed in the rocky mountain west. Water Res. Res. 45. doi: 10.1029/2008WR007029
Geo7. (2012). Hydrometeorologische Analyse des Ereignisses vom 10./11. Oktober 2011, Teilbericht Modul 2 “Schneehydrologie,” Bern: WSL; Geo7 AG.
Gobiet, A., Kotlarski, S., Beniston, M., Heinrich, G., Rajczak, J., and Stoffel, M. (2014). 21st century climate change in the European Alps-A review. Sci. Total Environ. 493, 1138–1151. doi: 10.1016/j.scitotenv.2013.07.050
Gominet, G. (2014). Deux Laves Torrentielles dans le Torrent du Saint-Antoine qui se Ressemblent… Presque ! (Two Debris Flows in the Torrent of Saint-Antoine that Resemble Each Other - Nearly!). Grenoble: Institute of Major Risks (IMRS).
Gremaud, V., and Goldschneider, N. (2010). “Climate Change Effects on Aquifer Recharge in a Glacierised Karst Aquifer System, Tsanfleuron-Sanetsch, Swiss Alps,” in Advances in Research in Karst Media, eds F. Carrasco, D. Valsero, J. José, and J. W. LaMoreaux (Heidelberg: Springer), 31–36.
Jaballah, M., and Jodeau, M. (2012). “2-D numerical modeling of water flow over a gravel bar,” in River Flow, ed F. Murillo Munoz (London: Taylor and Francis) 139–145.
Kangas, K., Vuori, K. M., Määttä-Juntunen, H., and Siikamäki, P. (2012). Impacts of ski resorts on water quality of boreal lakes: a case study in northern Finland. Boreal Environ. Res. 17, 313–325.
Körner, C. (2003). Alpine Plant Life, Functional Plant Ecology of High Mountain Ecosystems. Heidelberg: Springer
Lagriffoul, A., Boudene, J. L., Absi, R., Ballet, J. J., Berjeaud, J. M., Chevalier, S., et al. (2010). Bacterial-based additives for the production of artificial snow: what are the risks to human health? Sci. Total Environ. 408, 1659–1666. doi: 10.1016/j.scitotenv.2010.01.009
Le Messager. (2014). Montriond. Pompage au Lac pour la Neige Artificielle (Pumping water from Lake Montriond for artificial snow). Available online at: http://www.lemessager.fr/Actualite/Chablais/2014/04/24/article_montriond_pompage_au_lac_pour_la_neige_a.shtml#.VLY8tXuTWDk
Liao, K. H. (2012). A theory on urban resilience to floods—a basis for alternative planning practices. Ecol. Soc. 17:48. doi: 10.5751/ES-05231-170448
Litschauer, C. (2014). Save the Alpine Rivers! WWF Eur. Alp. Program. Available online at: http://d2ouvy59p0dg6k.cloudfront.net/downloads/wwf_study_save_the_alpine_rivers__c__wwf_2014_1.pdf
Luce, C. H., Abatzoglou, J. T., and Holden, Z. A. (2013). The missing mountain water: slower westerlies decrease orographic enhancement in the Pacific Northwest USA. Science 342, 1360–1364. doi: 10.1126/science.1242335
Marty, C. H., and Meister, R. (2012). Long-term snow and weather observations at Weissfluhjoch and its relation to other high-altitude observatories in the Alps. Theor. Appl. Climatol. 110, 573–583. doi: 10.1007/s00704-012-0584-3
Mathevet, T., Lepiller, M., and Mangin, A. (2004). Application of time-series analyses to the hydrological functioning of an Alpine karstic system: the case of Bange-l'Eau Morte. Hydrol. Earth Sys. Sci. 8, 1051–1064. doi: 10.5194/hess-8-1051-2004
Noe, K. (2014). “Science and Technology after 3.11: from a philosophical point of view,” in International Innovation Workshop on Tsunami, Snow Avalanche and Flash Flood Energy Dissipation (Tohoku University and University of Lyon, Chamonix).
Nolin, A. (2012). Perspectives on climate change, mountain hydrology, and water resources in the Oregon Cascades, USA. Mt. Res. Dev. 32, 35–46. doi: 10.1659/mrd-journal-d-11-00038.s1
ORF. (2014). Obertauern: Behörde verplombt Beschneiung (Obertauern. Agency seals snowmaking), ORF. Salzburg. Available online at: http://salzburg.orf.at/news/stories/2682339/
Pellicciotti, F., Carenzoa, M., Bordoya, R., and Stoffel, M. (2014). Changes in glaciers in the Swiss Alps and impact on basin hydrology: Current state of the art and future research. Sci. Total Environ. 493, 1152–1170. doi: 10.1016/j.scitotenv.2014.04.022
Provenzale, A., and Palazzi, E. (2014). “Assessing climate change risks under uncertain conditions,” in Engineering Geology for Society and Territory - Climate Change and Engineering Geology, eds G. Lollino, A. Manconi, J. Clague, W. Shan, and M. Chiarle (Cham: Springer), 1–5.
Rasouli, K., Pomeroy, J. W., Janowicz, R., Carey, S. K., and Williams, T. J. (2014). Hydrological sensitivity of a northern mountain basin to climate change. Hydrol. Process. 28, 4191–4208. doi: 10.1002/hyp.10244
Ristic, R., Kasanin-Grubin, M., Radic, B., Nikic, Z., and Vasiljevic, N. (2012). Land degradation at the stara planina ski resort. Environ. Manage. 49, 580–592. doi: 10.1007/s00267-012-9812-y
SAEFL. (2004). Riverine Floodplain use and Environmental Damage. Nature and Landscape Life Cycle Assessments. Final Report, Environmental Series 361.
Schmidli, J., Goodess, C. M., Frei, C., Haylock, M. R., Hundecha, Y., Ribalaygua, J., et al. (2007). Statistical and dynamical downscaling of precipitation: an evaluation and comparison of scenarios for the European Alps. J. Geophys. Res. 112, D4. doi: 10.1029/2005JD007026
Serquet, G., Marty, C. H., Dulew, J. P., and Rebetez, M. (2011). Seasonal trends and temperature dependence of the snowfall / precipitation day ratio in Switzerland. Geophys. Res. Lett. 38, 1–5. doi: 10.1029/2011GL046976
Serraz, G. (2012, September 9). The Lake Bourget has lost one quarter of its water resources. Les Echos. Available online at: http://www.lesechos.fr/20/09/2012/LesEchos/21274-027-ECH_le-lac-du-bourget-a-perdu-le-quart-de-ses-ressources-en-eau.htm#sXeV8rIQwJ5L0zO1.99
SIBF (2011). Syndicat Intercommunal du Bassin de la Fure. Etude d'opportunité pour la Mise en Place d'une Politique de Gestion Global de l'eau et des Milieux Aquatiques. Bassins Paladru, Fure, Morge et Olon. (Intercommunal Union of the Fure Basin). Feasability Study for the implementation of a global water and aquatic environment management policy" Rapport de Phase 1 - Etat des lieux et diagnostiques. Report. Burgeap. St. Martin d'Heres. 173.
Steininger, K. W., Haas, W., König, M., Prettenthaler, F., Prutsch, A., Themessi, M., et al. (2015). Die Folgeschäden des Klimawandels in Österreich. Dimensionen unserer Zukunft in zehn Bildern für Österreich. Wien: Sonderheft COIN, Austrian Climate Research Programme.
Stöckli, V. (2012). Wasserwirtschaft in Davos—eine Kurze Bilanz Ihrer Nachhaltigkeit, Alpine Schnee- und Wasserressourcen Gestern, Heute, Morgen (Water Management in Davos - a Short Summary of ist Sustainability. Alpine Snow and Water Resources Yesterday, Today and Tomorrow), Forum für Wissen (Knowledge Forum) 2012. Eidgenössische Forschungsanstalt WSL (Hrsg.), (Birmensdorf).
Tiroler Tageszeitung. (2014). A supply tank with 18,000 L diesel plunged off road at 1310m altitude in the Zillertal on the 21st March 2014, Tiroler Tageszeitung, Tirol. Available online at: http://www.tt.com/panorama/unfall/8127039-91/tankwagen-mit-18.000-liter-diesel-st%C3%BCrzte-auf-1310-meter-seeh%C3%B6he-ab.csp?tab=article
Trenberth, K. E. (2011). Changes in precipitation with climate change. Clim. Res. 47, 123–138. doi: 10.3354/cr00953
Van den Bergh, T., Hiltbrunner, E., and Körner, C. (2014). “Land Abandonment Increases Evapotranspiration in Mountain Grassland, abstract 1887374,” in 3rd International Symposium, Evapotranspiration: Challenges in Measurement and Modeling, from Leaf to the Landscape Scale and Beyond (Raleigh).
Vanham, D., Fleischhacker, E., and Rauch, W. (2009). Impact of snowmaking on alpine water resources management under present and climate change conditions. Water Sci. Technol. 59, 1793–801. doi: 10.2166/wst.2009.211
Vergara, W., Deeb, A., Leino, I., Kitoh, A., and Escobar, M. (2011). Assessment of the Impacts of Climate Change on Mountain Hydrology Development of a Methodology through a Case Study in the Andes of Peru. Washington, DC: A World Bank Case Study.
Weingartner, R., Schädler, B., Reynard, E., Bonriposi, M., Graefe, O., Herweg, K., et al. (2014). MontanAqua: Wasserbewirtschaftung in Zeiten von Knappheit und globalem Wandel. Wasserbewirtschaftungsoptionen für die Region Crans-Montana-Sierre im Wallis. Forschungsbericht des Nationalen Forschungsprogramms NFP 61 (Bern).
Whitfield, P. H., Moore, R. D., Shook, K., Spence, C., Boon, R., Woods, R., et al. (2013). “Summary and synthesis of workshop breakout group discussions,” in Putting Prediction in Ungauges Basins into Practice, eds J. M. Pomeroy, P. H. Whitfield, and C. Spence (Ottawa, ON: Canadian Water Resources Association), 271–304.
Keywords: global change, water consumption, floods, water scarcity, water quality, ski resorts, lakes, stakeholder knowledge
Citation: de Jong C (2015) Challenges for mountain hydrology in the third millennium. Front. Environ. Sci. 3:38. doi: 10.3389/fenvs.2015.00038
Received: 07 February 2015; Accepted: 25 April 2015;
Published: 20 May 2015.
Edited by:
Rob Swart, Alterra, NetherlandsReviewed by:
Nathaniel K. Newlands, Agriculture and Agri-Food Canada, CanadaCopyright © 2015 de Jong. This is an open-access article distributed under the terms of the Creative Commons Attribution License (CC BY). The use, distribution or reproduction in other forums is permitted, provided the original author(s) or licensor are credited and that the original publication in this journal is cited, in accordance with accepted academic practice. No use, distribution or reproduction is permitted which does not comply with these terms.
*Correspondence: Carmen de Jong, Mountain Centre, Université Savoie Mont Blanc, 5, Boulevard de la Mer Caspienne, 73776 Le Bourget-du-Lac, France,ZGVqb25nLmNhcm1lbkBuZXVmLmZy
Disclaimer: All claims expressed in this article are solely those of the authors and do not necessarily represent those of their affiliated organizations, or those of the publisher, the editors and the reviewers. Any product that may be evaluated in this article or claim that may be made by its manufacturer is not guaranteed or endorsed by the publisher.
Research integrity at Frontiers
Learn more about the work of our research integrity team to safeguard the quality of each article we publish.