- Division of Biomedical Sciences, School of Medicine, University of California, Riverside, Riverside, CA, United States
Introduction: Military personnel and local civilians at various deployment locations are plagued with serious health conditions. Evidence points to burn pit emissions as the cause of these pathologies; however, similar diseases are also caused by environmental exposures, smoking, genetic predispositions, or other comorbidities. Burn pits, which are large smoldering piles of refuse ignited with jet or diesel fuel, contain human and medical waste as well as paint, plastics, ammunition, and other materials—each of which can be attributed to health concerns in other industrial settings. Here we compare various pathologies attributable to toxic aerosol exposures and discuss distinct pathologies that may be linked to burn pit exposures.
Objectives: We performed a literature review where we provide information on toxic exposures that may pose relevance to burn pit exposure and furthermore, highlight what is already known about burn pit exposures and what steps need to be taken to diagnose and correlate certain respiratory pathologies to chronic exposure from overseas burn pits.
Data sources: We conducted searches through PubMed and Google Scholar to determine where gaps in our knowledge of burn pit exposure lie. Thorough review on jet-fuel properties and particulate matter were performed as supporting evidence of potential toxins from burn pit emissions.
Results: To date, studies on burn pit emissions consist mainly of systematic reviews and discussions to address the problem, with very few acute-exposure studies and little to no chronic-exposure studies. We found that symptoms range from respiratory pathologies to neurological deficits, but treatment has been limited as medical facilities, such as the Veterans Administration (VA), require proof that a condition is service-connected.
Conclusions: To determine the effects of burn pit exposure on humans, chronic exposure to mimicked burn pit emissions is necessary to draw definitive conclusions between phenotypic differences in pathologies linked to exposure. By determining phenotypic differences, conclusions can be made about the pathologic origins, potentially leading to future diagnoses and treatments for veterans and affected civilians.
1 Introduction
1.1 Introduction to military burn pits
Military burn pits were utilized through various war and support operations throughout the Middle East and Southeast Asia. At 297 total documented locations, burn pits were distributed across Iraq (n = 152), Afghanistan (n = 99), Kuwait (n = 14), Somalia (n = 4), and other deployment sites (n = 28), annotated in Table 1 (2). These pits were mainly used to eliminate refuse; however, due to their proximity to operational sites and living quarters, the emissions from burn pit combustions likely exposed military personnel and local civilians to many potentially toxic chemicals. Refuse in burn pits included human waste, medical waste, various plastics, fabric, wood, munitions, metal, paint, and other potentially carcinogenic materials that aided in combustion (3). Consequently, the burning of various wastes released particulate matter (PM) with numerous harmful toxins including PM2.5., PM10, dioxins, furans, lead, mercury, volatile organic compounds, carbon monoxide, hexachlorobenzene, polycyclic aromatic hydrocarbons and nitrogen dioxide (4, 5), which is commonly associated with negative respiratory health effects. However, pollutant type and level of toxicity will differ depending on the combustion mechanism, such as flaming or smoldering.
To ignite burn pits, chemicals such as diesel fuel and JP-8 jet fuel were used as accelerants. JP-8 jet fuel contains low levels of the harmful chemical benzene and was formerly considered non-toxic (6). However, the levels of benzene in jet-fuel were determined as carcinogenic in 2002 by The American Conference of Governmental Industrial Hygienists (ACGIH) due to kerosene-based jet fuel exposure resulting in increased carcinogenic states in animals (7). In 1996, the National Research Council (NRC) initially reported that lower levels of benzene and other kerosene-based fuels would not cause harm if exposures were under 500 mg/m3. They found that the average aerosol exposure for aircraft mechanics in the Air Force was 350 mg/m3, below the threshold considered harmful at that time (8). However, once the ACGIH determined carcinogenicity, the NRC was prompted to conduct a study published in 2003 where JP-8 jet fuel was analyzed and found to contain various exposure levels of more than 200 aromatic hydrocarbons including naphthalene and benzene. Studies by different NRC subcommittees were conducted, including effects of JP-8 jet fuel on the respiratory and nervous systems. Findings included development of multiple lung pathologies and neurological problems. Symptoms such as headache, nausea, fatigue, dizziness, vomiting, irritability, gait problems, and attention and memory deficits were noted. Interestingly, older studies on long-term occupational exposure to jet fuel had previously indicated the same symptomatic findings (7, 9–12). Importantly, environmental air samples collected from various deployment sites from 2005 to 2012 showed evidence of toxic materials in the air, detecting 23 different volatile compounds including acrolein and benzene. Moreover, various amounts of PM were measured, with increasing levels at sites where there was less vegetation and more industrialization (13).
Given the likely exposure of military personnel to hazardous partial and complete combustion products of the material listed, it would not be surprising if many of the symptoms and pathologies have direct links to specific toxic pathologic mechanisms. Unfortunately, these adverse effects have not been actively studied in the exposed population due to the fact that exposure to burn pits was not considered a cause for concern until after documented burn pits were decommissioned. Because of this, there have been no detailed or comprehensive epidemiological studies regarding the health impacts from these exposures. Despite this, it is important to understand the impacts of emissions related to burn pit exposures so further research can be conducted to link health effects of burn pit exposure, especially chronic exposures, such as exposing rodent models in an aerosolized chamber, similar to previous environmental chamber studies conducted by Biddle et al. (14).
Because of deficiencies in literature, this review is intended to provide a general overview of toxic aerosols that are likely present in burn pit emissions that may have a negative health-related impact on personnel stationed overseas. The information provided is a worthy focus and can lead to future epidemiological and lab-based studies. Currently, veterans registered with the Airborne Hazards and Open Burn Pit Registry (AHOBPR) attribute a plethora of respiratory health conditions to burn pit exposure, so we must understand its components to provide a framework for future studies to identify consequences of these exposures, specifically to distinguish them from other more common causes of chronic lung disease and provide service-connection to disabilities attributable to burn pit emission exposure.
1.2 What is known about volatile aromatic hydrocarbons?
Considering that acrolein was found in environmental air sampling at various deployment locations, it is important to understand its impacts and association with burn pits. Acrolein is formed when certain materials are incinerated, such as organic matter from trash or fuel combustion, released as vehicle exhaust (13). When organic matter combustion is incomplete, it forms a substance known as creosote. Creosote is a natural source that contains various hydrocarbons and is found in materials such as coal and crude oil (15). Other volatile aromatic hydrocarbons (VAHs), such as benzene, xylene, toluene, ethylbenzene, and styrene, are also found in the atmosphere as by-products of increasing industrialization (16). Commonly measured in parts per million (ppm), each VAH has inconsistent reports of potentially hazardous concentrations that are deemed safe for environmental exposure (Table 2). The inconsistencies exist because of discrepancies on exposure type and setting, such as indoor vs. outdoor exposure, and short term vs. chronic exposure.
Recent studies have shown that in some environments, such as urbanized areas and near petroleum and crude oil industries, levels of hazardous pollutants may go above what is considered safe for exposed humans and animals, resulting in respiratory symptoms such as coughing, wheezing, asthma, and dyspnea (19); and neurological concerns such as fatigue, nausea, confusion, memory problems, and dizziness (20). Detection of increased concentrations of ethylbenzene, toluene, styrene, and xylene in the atmosphere have all been linked to these health concerns (16, 20), all of which are potentially found in burn pit emissions. Other VAHs are known to cause further pathological concerns. For example, benzene causes leukemia and hematotoxicity (16, 17, 21, 22) at low exposure levels (>1 ppm) due to its ability to affect metabolic properties of progenitor cells, leading to a decrease in total blood cell count (21).
1.3 Tumorigenicity and other lung pathologies from aromatic hydrocarbons
A multiple cohort study conducted by The Working Group (comprised of 27 scientists from 13 different countries) followed adults who had evidence of benzene exposure in their daily environment or occupational conditions. Many people from different cohorts developed some form of lung cancer from benzene exposure (17). An increased prevalence of lung adenoma was also found, which coincides with findings from a study involving mouse models where whole-body benzene exposure resulted in 42 out of 118 (36%) mice developing lung adenoma (23). Furthermore, multiple other aromatic hydrocarbons were found to be the cause of both malignant and non-malignant lung diseases (17), and many of these pollutants have been known to adhere to allergens and change the body's allergic response to the affected allergen, resulting in an increased inflammatory status in the lungs (15).
A study by Ramage et al. exposed mice to low burning properties and incomplete combustion, then examined bronchoalveolar lavage fluid (BALF) samples, where carbonaceous properties and varying degrees of lung inflammation were present (24). These observations became more clinically relevant when it was reported that rural African women developed cases of Hut Lung, or pneumoconiosis, due to constant exposure of a low functioning wood burner where the wood was soaked in some form of petroleum (25). Other exposures to poorly ventilated and low-burning solid fuel, such as fuel-soaked wood or coal burning, are further linked to other respiratory diseases, such as Chronic Obstructive Pulmonary Disease (COPD) and diffuse anthracosis (26). COPD in forms of emphysema and chronic bronchitis are also associated with solid fuel smoke exposure, similar to conditions found at open air burn pits. Studies involving COPD, lung carcinogenicity, fibrosis, and other lung diseases have substantial evidence of associations with exposure to aromatic hydrocarbons, resulting in the various aforementioned pathologies (15).
1.4 Lung damage from particulate matter and smoke
Due to incomplete combustion, burn pits are known to release different size fractions of PM (i.e., PM2.5, PM2.5−10, and PM10) into the air. In environmental settings, acute exposure to PM can result in increased cases of hospital admissions, where patients complain of symptoms such as coughing, wheezing, and difficulty breathing (27). These symptoms are consistent with both short and long-term effects of PM in the lungs, along with impaired lung function. Furthermore, adults over the age of 65 are susceptible to the development of cardiovascular disease and respiratory symptom-related hospital admission upon short-term exposure to PM, and those above age 75 have a significantly increased risk of death upon initial PM exposure (27). Multiple studies on humans exposed to the difference size fractions of PM showed that, regardless of race, ethnicity, sex, genetic factors, and comorbidities, respiratory and cardiovascular diseases are most prevalent after short-term PM exposure (27). In terms of respiratory distress, asthma and COPD are the most common forms of pulmonary disease derived from PM exposure, demonstrated in multiple laboratory tests and research methodologies, including measurements of BALF protein concentrations and increased eosinophil counts within the respiratory tract (27, 28).
Aerosolized PM has several potential origins, including particulate formation from volatile organic components, environmental dust from soil, and asphalt and brake dust in urban environments. PM produced from combustion presents an important subset with a variety of specific impacts. For example, studies on exposure to smoke in humans and animals show a multitude of pulmonary pathologies. To date, COPD is by far the most prevalent consequence of smoke exposure from cigarette, wildfire, wood, or other biomass smoke, such as household-originated smoke (29). However, tobacco smoke can induce COPD that presents rather differently from wood or other biomass smoke-induced COPD (Figure 1). The pathophysiology of non-cigarette smoke related COPD remains poorly understudied, even though 25%–40% of COPD diagnoses do not originate from cigarette/tobacco smoke exposure (29). The evidence of varied symptoms between COPD pathologies is important to note when thinking about burn pit emission exposure because the changed components and resulting VAH and PAH emissions within the smoke could indicate a changed pathophysiology in potential COPD and other lung pathology diagnoses from overseas burn pit smoke exposure.
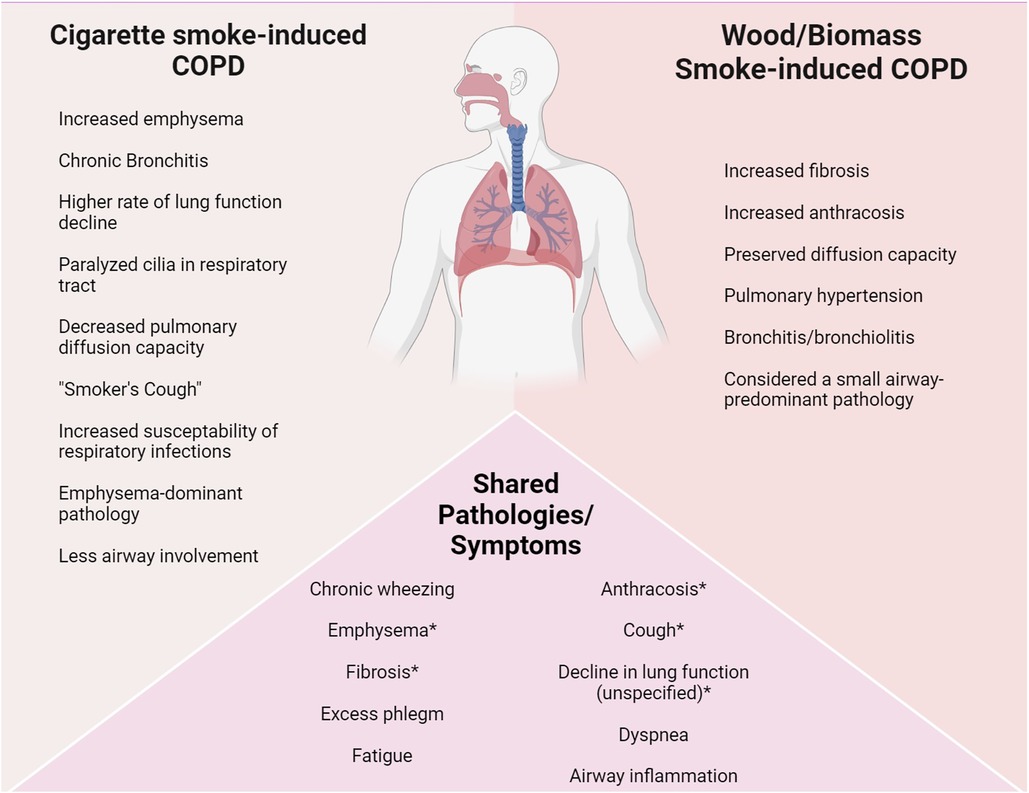
Figure 1 Phenotypic differences in (chronic exposure) COPD diagnoses. COPD is a common diagnosis among cigarette smokers, with high association of emphysema. Biomass/woodsmoke induced COPD exhibits different symptoms and pathologies, mainly being considered a small-airway disease (30). Despite their phenotypic differences, both fall under the umbrella term of COPD. Pathobiology and phenotype changes between COPD diagnoses and other respiratory pathology diagnosis is important because it highlights a direct cause-and-effect relationship, showcasing a link between chronic hazardous exposures and their resulting diseases. Variation in diseases can be linked to components of the initial chronic exposure. *These symptoms are observed differently in both pathologies (e.g., cigarette smoke-induced COPD exhibits quicker lung function decline compared to biomass smoke-induced, whereas biomass smoke-induced exhibits more fibrotic lesions in the lungs).
1.5 Neurological effects of smoke exposures
Burn pit victims experience more than just respiratory effects from smoke and PM exposure. Upon both acute and chronic exposure, many individuals experience attention and memory deficits, concentration and vision problems, and headaches. Furthermore, Iraqi citizens that have been chronically exposed to burn pit smoke and emissions have reported increased instances of chronic headaches and even brain cancer (1). However, neurological effects from burn pit smoke and emissions exposure are still greatly underrepresented in the literature, whereas a multitude of studies exist that encompass derived neuropathology from other smoke-related and PM exposures. While pathophysiology can change depending on what is burnt and where smoke derived, such as a difference in wood species burnt and the initial combustion properties (29), humans and animals alike are prone to toxicological effects causing neuro-deficits because of smoke and woodsmoke properties which contain carbon monoxide, PM, and hydrogen cyanide (31).
1.6 Industrial exposures, negative health outcomes, and burn pit relevance
Studies on volatile compounds and their health impacts suggest that increased exposure leads to pathologies such as leukemia, chronic respiratory and neurological disorders, birth defects, cardiovascular disease and different cancers, annotated in Table 3 (36–38). Chronic lung pathology, including lung cancer, has an increased prevalence in communities where harmful emissions, such as nitrogen dioxide (NO2), aldehydes, and diesel exhaust particles (DEPs), are found in greater atmospheric concentrations and in those who work with different types of emissions, such as diesel mechanics (39). This may provide some relevance to burn pit exposures due to the possibility that diesel fuel, being readily available at deployment locations, may have been used as an attempted accelerant as opposed to JP-8 jet fuel. While diesel fuel is not considered flammable, it is combustible and because of its incomplete combustion, it's possible that NO2, aldehydes, and DEPs may be associated with burn pit emission exposures. Furthermore, a change in chronic respiratory disease prevalence exists in different regions around the world and demonstrates a correlation between a pathology and its origin of exposure (40). Whether it be from tobacco smoke, wildfire smoke, hazardous environmental conditions, or burn pit emission exposure, we can speculate that burn pit-derived pathologies will likely have phenotypic differences from their other-origin derived counterparts.
2 Methods
2.1 Literature review
To further understand the correlation between respiratory disease, neurological deficits, and burn pit emission exposure, we searched the literature from 8/1/2023 through 5/8/2024 for specific topics relevant to burn pit exposures. Initially, we used “burn pits” as the keyword search and over 200,000 results returned. From here, we refined our search to specify overseas burn pits, their components, relevant exposure types, potential health concerns, and relevant study types. With this, a methodological approach was used to survey the literature with the following strategies:
1. Searches based on burn pit components (n = 27,955 results returned)
a. Key terms used: “burn pit components”; “what’s in burn pits”; “type of trash found in burn pits”
2. Searches based on emission properties (n = 15,621 results returned)
a. Key terms used: “burn pit emissions”; “exposure to burn pits”; “what’s in burn pit emissions”; “accelerant used for burn pits”
3. Searches based on VAH exposure in different settings (n = 40,219 results returned)
a. Key terms used: “JP-8 jet fuel exposure”; “benzene exposure”; other hydrocarbon “…exposure”
4. Searches based on particulate matter (PM) exposure (n = 21,840 results returned)
a. Key terms used: “particulate matter in burn pits”; “particulate matter size fraction”; “particulate matter exposures”
5. Searches based on chronic diseases from VAH and PM exposures (n = 3,250 results returned)
a. Key terms used: “health problems from particulate matter exposure”; “acute vs. chronic exposure to particulate matter”; “health problems from benzene exposure”; “toxic effects from VAH exposure in industrial settings”; “toxic effects from VAH exposure in occupational settings”
6. Searches based on previous studies on burn pit exposure (n = 18,900 results returned)
a. Key terms used: “what is known about overseas burn pits”; “burn pit exposure experiments”
7. Searches based on pathologies from burn pit exposure (n = 18,606 results returned)
a. Key terms used: “respiratory pathologies from burn pit exposure”; “burn pit registry”; “health concerns from overseas burn pit exposure”; “neurological deficits from burn pit exposure”
All searches were conducted via PubMed and Google Scholar. Furthermore, sources from heavily cited articles were analyzed for more information. Multiple reviews of the AHOBPR were analyzed which provided us with further evidence suggesting a direct correlation to increased instances of pulmonary and other diseases prevalent in veterans exposed to burn pits. Additionally, a systematic approach was implemented that refined a multiple literature review analysis to include experimental studies of burn pit emissions in both acute and chronic exposure conditions. Despite various search efforts, few experimental studies were found, although many reviews addressing the concerns of burn pit emission exposure were accessible and analyzed. Overall, despite a lack of research conducted on the topic at hand, a thorough review of current burn pit emission exposure indicated potential findings between chronic exposure and development of various respiratory pathologies. The number of articles we ultimately used for this review regarding burn pit emission exposure was n = 10 for experimental studies, n = 3 for clinical or case studies, and n = 10 for review papers.
2.2 Inclusion and exclusion criteria for burn pit studies
Despite the number of results returned from each search, many searches returned duplicate publications, repetitive information and/or studies, or non-relevant studies such as non-military related burn pit exposures and VAH or PAH exposures that have no significant relevance to burn pit emissions. Both review and research articles were considered for inclusion; however, many of the research articles returned were variations of meta-analyses regarding disease prevalence of various pathologies from VA health records, such as medical reports provided by the AHOBPR. Upon further investigation of returned results, we found that there were not many relevant research or experimental studies published on military burn pit exposures. Due to the low volume of experimental burn pit related studies, all found peer-reviewed sources directly linked to burn pit exposures were used. Sources that were not peer-reviewed, forthcoming, in press, or abstract only were excluded.
2.3 Inconsistencies and bias in literature
Bias existed in toxic exposure studies where different levels of hazardous exposures were highlighted. Because of this, inconsistencies were annotated (found in Table 2) and hazardous levels listed in the table came from sources that either involved burn pit emission exposure or provided relevance to burn pits due to similar properties of exposure types and burn pit exposures. However, other sources that were not used may report different toxic exposure levels for PM and polycyclic or aromatic hydrocarbons.
Currently, there are no existing human-based studies on active burn pit exposure because research efforts to date have been focusing on assessing burn pit exposures retrospectively, and there currently exists no epidemiological data on active human exposures. Because of this, we had to rely on findings from rodent-based active exposure studies, where only acute exposure findings were highlighted.
3 Results
3.1 Potential lung diseases from burn pits
To date, potential lung pathologies have been identified from burn pit exposure but lack experimental data regarding chronic exposure and detrimental respiratory outcomes. Furthermore, a plethora of systematic reviews and possible clinical approaches have been conducted. Many clinical findings and meta-analyses suggest that while lung disease is prevalent, it is heterogenous, meaning that burn pit exposure may predispose personnel to a variety of lung pathologies, such as small airway disease, obstructive lung disease, sarcoidosis, and other deployment-related respiratory diseases (DRRDs) (41–43).
A study done by Liu et al. surveyed 4,343 burn pit registry participants between 2012 and 2015 where an elevated prevalence of emphysema, chronic bronchitis, and COPD were diagnosed in service members who did not have these diseases prior to deployment. Those who were directly exposed to the burn pits for longer time periods each day also had significantly increased prevalence of symptoms linked to emphysema, chronic bronchitis, COPD, and a higher risk of hypertension (4). All information gathered came from personnel who were stationed within two miles of an active burn pit.
Active-duty personnel self-reported different forms of respiratory distress during and after deployment. The Armed Forces Health Surveillance Center (AFHSC) performed a study where they determined that personnel who deployed overseas to locations that had burn pits were more likely to develop respiratory illness than those who never deployed (4). This study jump-started when a symposium was held in 2012 to discuss potential health detriments to burn pit exposure. After initial assessment, the VA created a registry for veterans who reported burn pit exposure in Iraq or Afghanistan (4). The registry, titled Airborne Hazards and Open Burn Pit Registry, is for active-duty military personnel, veterans, national guard, and Coast Guard. Although it was initially available for those who served in Afghanistan, Djibouti, or Iraq on or after September 11, 2001, it has since been modified to include those who served in Southwest Asia on or after August 2, 1990 (3). One of the most common concerns that veterans report on this registry is impairment of respiratory function and shockingly, only about 10% of the personnel that requested a physical examination by the VA have obtained one (1).
Impairment of respiratory function is a broad explanation of some of the symptoms associated with burn pit exposure. Dyspnea is one of the most reported symptoms in exposure-related pathologies. Constrictive bronchiolitis (44), sinonasal disease (45), other lung diseases (46), and lung fibrosis (47) have all been linked to burn pit emission exposure and all of which are commonly referred to as DRRDs. This spectrum of symptoms is related to a broad range of lung diseases, not limited to COPD, asthma, lung cancer, and chronic rhinosinusitis (48).
3.2 Neurological and other health concerns from chronic burn pit emission exposure
Aside from developing chronic respiratory pathologies, many personnel exposed to burn pits for long periods of time have developed other concerning health conditions, such as neurological deficits, cardiovascular disease, and hemorrhagic or ischemic strokes (7, 41). Studies suggest that those with higher instances of cardiovascular disease are potentially predisposed to increased frequencies of ischemic stroke (41). Because a higher probability of stroke is associated with increased cardiovascular concerns, this may suggest a correlation between various neurological and cardiac symptoms and diagnoses. Furthermore, while headaches, abnormal gait, vertigo, irritability, vision problems, and attention and memory deficits have been noted in the exposed veteran population (7, 9–12, 20), many of the underpinning mechanisms of neurological diseases from burn pit exposure are still unknown.
Studies suggest that pathological outcomes from burn pit related exposures are not only present in the exposed veteran population, but also in citizens that lived near burn pits while they were in use. To elaborate, Iraqi citizens were chronically exposed to burn pits near Joint Base Balad—many of which were exposed over ten years. Consequently, these citizens have experienced an increased prevalence of brain cancer (1). Furthermore, mothers who were exposed to the smoke during pregnancy had a significantly higher chance of delivering children with congenital abnormalities. Some of these conditions include congenital heart defects from those exposed to chromium-contaminated drinking water during pregnancy and in-utero development of clubfoot—a malformation that occurs due to a mutation along the neuromuscular unit (49). There also exists an increased prevalence of mental health concerns among those on the AHOBPR but unfortunately, the effects of which are highly underrepresented in veteran-related research and medicine (50). Despite research divulging the potential implications of burn pit exposure on neurological and other health concerns, most studies indicate the importance of needing more research to link many of these negative health outcomes to chronic burn pit emission exposure.
3.3 Limited information about burn pit exposure and rodent studies
For ethical reasons, active-exposure burn pit studies on human subjects would be difficult to achieve in any setting, but detailed longitudinal studies are going to be especially difficult today, since health problems from emission exposure did not draw attention until after documented burn pits were decommissioned by the US military. Thus, clinical studies on military personnel exposed to burn pits will mainly be possible only after a significant lag between active exposure to manifestation of symptoms. Presently, studies addressing chronic illnesses from burn pit exposure have only been conducted in one of four ways: determining the types of pollutants likely present in the smoke from burn pits; sampling air, water, or soil from areas around burn pits and determining the amount of each pollutant present; exposing rodent models to burn pit-like emissions; and reviews or analyses of symptoms reported by those who are on the burn pit registry (6).
While there have not been many studies to date, evidence of decreased respiratory function in animal models exposed to burn pit emissions has been reported. Research performed by Vance et al. involved exposing mice to smoke that was either flaming or smoldering for one-hour periods on two consecutive days. The smoke was generated from burning either plywood, cardboard, mixed plastics, or a combination of all three. Reduced breathing frequency and reduced minute volume were noted in mice after exposure as opposed to their baseline lung function (51).
In a 2018 study performed by the Naval Medical Research Unit Dayton (52), rats were used and separated into three different cohorts, each of the three cohorts consisting of four groups. Cohorts were separated by time (i.e., euthanized four days after exposure, euthanized 32 days after exposure, and euthanized 90 days after exposure) and groups were separated by exposure condition. The only significant finding was an increase of TNF-α (Tumor Necrosis Factor alpha) levels in BALF (52) after exposure. The pro- and anti-inflammatory profiles studied showed some potential for future studies though refinement of the exposure conditions will be needed.
Further studies were conducted on rat models with exposure to both sand and burn pit emissions. Nuclear Magnetic Resonance (NMR)-based metabolomic analysis was performed on urine collected from the rats at different time points to determine any increase in metabolites after sand or burn pit emission exposure. Results indicated that there was an increase in metabolites during exposure; however, the data could not rule out stress-based factors causing the increase in metabolites. While there might be an effect from exposure alone, quantifying it against stress-induced responses has proven difficult (53).
Mouse models were exposed to different forms of emissions that mimicked burn pits to determine the amount of PM they were inhaling. To do this, smoke was created from both flaming and smoldering conditions, where cardboard, plywood, plastic, diesel, and a mixture of all were burned. PM was generated from each combustion with the largest PM size equating to 2.5 µm, derived from plastic combustion. The results indicated that overall, mice had impaired lung function when exposed to the emissions from burnt plastic, with a more severe response compared to other exposures (54). Other mouse model studies have shown that previous airway diseases increase the likelihood of further respiratory system damage with smoke exposure (55). Unfortunately, these studies were all limited by the inability to expose rodents for long periods of time, resulting in only subtle findings from acute exposures and a lack of evidence of chronic health concerns from burn pit emission exposure.
This brief overview of animal studies on burn pit-related exposures illustrates some of the limitations of the methodologies used to date. It is important to note that we must clearly distinguish between studies on chronic lung diseases (e.g., COPD) vs. diseases caused by chronic exposure. Animal studies tend to be limited to relatively brief or acute exposures, which do not replicate the real-world exposures of humans to environmental aerosols. Toxic effects from an acute high dose exposure will be quite different from the effects of chronic exposure at lower doses. Moreover, these studies were not able to provide evidence linking exposures to other diseases including lung cancer. Without specific details of the burn pit, what was burned, type of exposure and how long each person was exposed, it is difficult to draw definitive conclusions.
4 Discussion
4.1 Unanswered questions
Chronic health concerns from burn pit exposure are greatly underappreciated in our veteran population. Multiple systematic reviews have been conducted on veterans registered for the Airborne Hazards and Open Burn Pit Registry, providing evidence that there is a direct correlation between burn pit emission exposure and various chronic respiratory diseases. Recreations of burn pits have been attempted a few times in chamber-exposed rodent studies, but studies involving continuous burning and prolonged exposure to both specific mixtures and individual components of burn pits remain greatly lacking. Furthermore, rodent studies have some limitations. For starters, rodents have notable sex differences and species/strain difference in response to air pollution-induced stress. Sex plays an important role in epidemiology and mechanistic pathophysiological responses as female mice tend to have higher sensitivity to aerosolized environmental exposures (56). Rodents are also obligate nasal breathers and have a different rate of breathing and orientation because they are four-pawed. The mouse lung structure also differs anatomically and physiologically from humans via the total lung capacity, lobe orientation, parenchyma, alveoli, and blood-gas barrier thickness (57). However, the mouse genome is adequately similar to the human genome with a ∼99% similarity (57) and the anatomical differences may not necessarily affect the deposition of aerosols in the lungs. Pre-clinical rodent-based studies are a necessary approach for many health-related studies as human experimentation is often unethical and unachievable due to invasiveness of some experimental procedures. If we can recreate burn pits in environmental chambers and have a controlled, long-term exposure on mice, we can potentially better our understanding of what pathologies, especially chronic lung pathologies, may be a direct result of exposure.
Experimental exposure studies may reveal a range of lung pathologies, so comparisons with respiratory health concerns reported by veteran populations will help us deduce potential cause-and-effect relationships from burn pit exposures. However, the various pathologies may not be distinct and can overlap with each other and with other cause-and-effect relationships, such as lung disease caused by cigarette smoke or asthma caused by pollutant exposure from living next to a major highway. We can address these concerns by comparing what we do know vs. what we do not know. Pathogenic mechanisms can change depending on what caused it. For example, the way COPD presents is very different between COPD caused by tobacco smoke vs. COPD caused by wildfire, wood, or biomass smoke exposure (29). Accordingly, by exposing animal models to chronic burn-pit simulated exposures, we can study the resulting lung pathologies and compare their phenotypes to lung pathologies reported by exposed active-duty personnel and veterans. With the knowledge of phenotypic differences among various lung pathologies and understanding the smoke conditions and involved aromatic hydrocarbons, we can potentially link different chronic respiratory pathogenic mechanisms to specific pollutants or smoke conditions. Thus, studies like these can help provide more effective diagnosis and treatment for exposed personnel by targeting specific underlying mechanisms and pathologies.
To date, a small number of short-term exposures have been conducted, resulting in few significant findings. What is needed are more prolonged exposure studies where animal models can be exposed to controlled and continuous combustion of various burn pit combustion products. Analysis will also benefit from systems enabling comparisons between single component vs. multiple component combustion products, since chemical interactions are well documented in other situations such as urban pollutants (e.g., diesel exhaust). Resulting lung pathologies can be evaluated for inflammatory profiles, tissue changes, and neurological deficits. Combinations of combustible materials should be gathered and organized based on reports of waste used in burn pits overseas. JP-8 jet fuel, diesel fuel, and other accelerants could be compared based on reports from first-hand witnesses to burn pits. Previous environmental chamber exposure studies (14) have shown the feasibility of this approach; exposure chambers can be designed to release specific concentrations of individual combustion components allowing for focused studies on the resulting pathogenesis in the exposed animal models.
5 Conclusion
5.1 A need for chronic exposure research
Rodent based studies remain the most feasible approach to determining an initial understanding of underlying mechanisms of exposure-related disease. Despite differences in their orientation and breathing pattern, there remains enough rationale to use rodents for pre-clinical experimentation. Results derived from rodent-based chronic burn pit exposure studies may provide information regarding potentially derived pathologies from exposure and in turn, researchers can use this information to better determine disease development in exposed personnel. To date, there only exists speculation regarding the origin of chronic respiratory and other pathologies without any direct service-connected evidence of these conditions being the result of chronic burn pit exposure.
In sum, it is clear that more animal model-based chronic exposure studies are needed where burn pit emissions can be simulated. While animal models are not equivalent to human clinical studies, it would of course be impossible to contemplate human clinical studies on such toxic exposures. The complexity of the effects of toxic mixtures also requires carefully designed studies to integrate the interacting pathologies from combinations of components in the combustion products; this will allow us to identify differences in phenotypic mechanisms that underlie specific pathologies. In doing so, we can accurately match one or more phenotypic differences to burn pit emission exposure as distinct from other causes of chronic lung diseases, and in turn draw a more definitive conclusion regarding pathologic origin. Importantly, these results may also eventually provide rationale for conducting more focused clinical studies which will provide more accurate diagnoses and treatments for exposed personnel.
Author contributions
VP: Conceptualization, Data curation, Formal Analysis, Writing – original draft. DL: Conceptualization, Resources, Supervision, Writing – review & editing.
Funding
The author(s) declare financial support was received for the research, authorship, and/or publication of this article.
Acknowledgments
Research reported in this publication was supported by the National Institute On Minority Health And Health Disparities of the National Institutes of Health under Award Number U54MD013368. The content is solely the responsibility of the authors and does not necessarily represent the official views of the National Institutes of Health.
Conflict of interest
The authors declare that the research was conducted in the absence of any commercial or financial relationships that could be construed as a potential conflict of interest.
Publisher's note
All claims expressed in this article are solely those of the authors and do not necessarily represent those of their affiliated organizations, or those of the publisher, the editors and the reviewers. Any product that may be evaluated in this article, or claim that may be made by its manufacturer, is not guaranteed or endorsed by the publisher.
References
1. MacMahon J, Bruun D, Lein PJ. Military Burn Pits: A Toxic Legacy of War. UC Davis Veterinary Medicine (2023). Available online at: https://www.openaccessgovernment.org/military-burn-pits-a-toxic-legacy-of-war-verteran-health-conflict/149605/
2. Burn pit locations. VetsHQ. (2018). Available online at: https://www.vetshq.com/burn-pit-locations/ (retrieved August 25, 2023).
3. Ciminera P, Baird CP, Harkins D. (n.d.). Airborne Hazards and Open Burn Pit Registry Overview. Available online at: https://phc.amedd.army.mil/PHC%20Resource%20Library/AHOBPR%20Overview%207-25-14.pdf (retrieved August 25, 2023).
4. Liu J, Lezama N, Gasper J, Kawata J, Morley S, Helmer D, et al. Burn pit emissions exposure and respiratory and cardiovascular conditions among airborne hazards and open burn pit registry participants. J Occup Environ Med. (2016) 58(7):249–55. doi: 10.1097/jom.0000000000000776
5. Jones KA, Smith B, Granado NS, Boyko EJ, Gackstetter GD, Ryan MAK, et al. Newly reported lupus and rheumatoid arthritis in relation to deployment within proximity to a documented open-air burn pit in Iraq. J Occup Environ Med. (2012) 54(6):698–707. doi: 10.1097/jom.0b013e3182529799
6. American Cancer Society Medical and Editorial Content Team. Military Burn Pits and Cancer Risk. Atlanta, GA: American Cancer Society (2022). Available online at: https://www.cancer.org/content/dam/CRC/PDF/Public/9594.00.pdf
7. National Research Council. Toxicologic Assessment of Jet-Propulsion Fuel 8. Washington, DC: National Research Council (US) Subcommittee on Jet-Propulsion Fuel 8 (2003). doi: 10.17226/10578
8. The National Academies Press. Permissible exposure levels for selected military fuel vapors. Natl Acad Sci Engineering Med. (1996):1–8. doi: 10.17226/9133
9. Kendall RK, Smith E, Smith LB, Gibson RL. JP-8 Final Risk Assessment. (2001). Available online at: doi: 10.21236/ada443114 (retrieved August 17, 2023).
10. Knave B, Olson BA, Elofsson S, Gamberale F, Isaksson A, Mindus P, et al. Long-term exposure to jet fuel. II. A cross-sectional epidemiologic investigation on occupationally exposed industrial workers with special reference to the nervous system. Scand J Work Environ Health. (1978) 4(1):19–45. doi: 10.5271/sjweh.2725
11. Knave B, Persson HE, Goldberg JM, Westerholm P. Long-term exposure to jet fuel: an investigation on occupationally exposed workers with special reference to the nervous system. Scand J Work Environ Health. (1976) 2(3):152–64. doi: 10.5271/sjweh.2809
12. Porter HO. Aviators intoxicated by inhalation of JP-5 fuel vapors. Aviat Space Environ Med. (1990) 61(7):654–6. pubmed.ncbi.nlm.nih.gov.2386453
13. Blasch KW, Kolivosky JE, Heller JM. Environmental air sampling near burn pit and incinerator operations at Bagram Airfield, Afghanistan. J Occup Environ Med. (2016) 58(8):S38–43. doi: 10.1097/jom.0000000000000792
14. Biddle TA, Li Q, Maltz MR, Tandel PN, Chakraborty R, Yisrael K, et al. Salton sea aerosol exposure in mice induces a pulmonary response distinct from allergic inflammation. Sci Total Environ. (2021) 792:148450. doi: 10.1016/j.scitotenv.2021.148450
15. Connellan SJ. Lung diseases associated with hydrocarbon exposure. Respir Med. (2017) 126:46–51. doi: 10.1016/j.rmed.2017.03.021
16. Jeong S-Y, Moon YK, Wang J, Lee J-H. Exclusive detection of volatile aromatic hydrocarbons using bilayer oxide chemiresistors with catalytic overlayers. Nat Commun. (2023) 14(1):233. doi: 10.1038/s41467-023-35916-3
17. Loomis D, Guyton KZ, Grosse Y, El Ghissassi F, Bouvard V, Benbrahim-Tallaa L, et al. Carcinogenicity of benzene. Lancet Oncol. (2017) 18(12):1574–5. doi: 10.1016/s1470-2045(17)30832-x
18. Centers for Disease Control and Prevention. Benzene. Centers for Disease Control and Prevention (2015). Available online at: https://www.atsdr.cdc.gov/sites/toxzine/benzene_toxzine.html#:∼:text=Brief%20exposure%20(5%E2%80%9310%20minutes,ppm)%20can%20result%20in%20death
19. Chiurco J, Falvo MJ, Sotolongo AM, Arjomandi M, Krefft SD, Osterholzer JJ, et al. Multidimensional dyspnea assessment associated with forced oscillometry and pulmonary function testing in veterans in the airborne hazards and open burn pit registry. B105 Sick Job Uncovering Modern Occup Hazards. (2023) 207:A4369. doi: 10.1164/ajrccm-conference.2023.207.1_meetingabstracts.a4369
20. Correa SM, Arbilla G, Marques MRC, Oliveira KMPG. The impact of BTEX emissions from gas stations into the atmosphere. Atmos Pollut Res. (2012) 3(2):163–9. doi: 10.5094/apr.2012.016
21. Lan Q, Zhang L, Li G, Vermeulen R, Weinberg RS, Dosemeci M, et al. Hematotoxicity in workers exposed to low levels of benzene. Science. (2004) 306(5702):1774–6. doi: 10.1126/science.1102443
22. Verma DK, Johnson DM, McLean JD. Benzene and total hydrocarbon exposures in the upstream petroleum oil and gas industry. AIHAJ. (2000) 61(2):255–63. doi: 10.1080/15298660008984534
23. Farris GM, Everitt JI, Irons RD, Popp JA. Carcinogenicity of inhaled benzene in CBA mice. Toxicol Sci. (1993) 20(4):503–7. doi: 10.1093/toxsci/20.4.503
24. Ramage JE, Roggli VL, Bell DY, Piantadosi CA. Interstitial lung disease and domestic wood burning. Am Rev Respir Dis. (1988) 137(5):1229–32. doi: 10.1164/ajrccm/137.5.1229
25. Grobbelaar JP, Bateman ED. Hut lung: a domestically acquired pneumoconiosis of mixed aetiology in rural women. Thorax. (1991) 46(5):334–40. doi: 10.1136/thx.46.5.334
26. Gold JA, Jagirdar J, Hay JG, Addrizzo-Harris DJ, Naidich DP, Rom WN. Hut lung: a domestically acquired particulate lung disease. Medicine. (2000) 79(5):310–7. doi: 10.1097/00005792-200009000-00004
27. Sacks JD, Stanek LW, Luben TJ, Johns DO, Buckley BJ, Brown JS, et al. Particulate matter–induced health effects: who is susceptible? Environ Health Perspect. (2011) 119(4):446–54. doi: 10.1289/ehp.1002255
28. Morishita M, Keeler GJ, Wagner JG, Marsik FJ, Timm EJ, Dvonch JT, et al. Pulmonary retention of particulate matter is associated with airway inflammation in allergic rats exposed to air pollution in urban Detroit. Inhalation Toxicol. (2004) 16(10):663–74. doi: 10.1080/08958370490476550
29. Ortiz-Quintero B, Martínez-Espinosa I, Pérez-Padilla R. Mechanisms of lung damage and development of COPD due to household biomass-smoke exposure: inflammation, oxidative stress, MicroRNAs, and gene polymorphisms. Cells. (2022) 12(1):67. doi: 10.3390/cells12010067
30. Camp PG, Ramirez-Venegas A, Sansores RH, Alva LF, McDougall JE, Sin DD, et al. COPD Phenotypes in biomass smoke- versus tobacco smoke-exposed Mexican women. Eur Respir J. (2013) 43(3):725–34. doi: 10.1183/09031936.00206112
31. Sanderfoot OV, Bassing SB, Brusa JL, Emmet RL, Gillman SJ, Swift K, et al. A review of the effects of wildfire smoke on the health and behavior of Wildlife. Environ Res Lett. (2022) 16(12):123003. doi: 10.1088/1748-9326/ac30f6
32. Yost EE, Galizia A, Kapraun DF, Persad AS, Vulimiri SV, Angrish M, et al. Health effects of naphthalene exposure: a systematic evidence map and analysis of potential considerations for dose–response evaluation. Environ Health Perspect. (2021) 129(7):76002. doi: 10.1289/ehp7381
33. Centers for Disease Control and Prevention. Acrolein. Atlanta, GA: Centers for Disease Control and Prevention (2014). Available online at: https://wwwn.cdc.gov/TSP/MMG/MMGDetails.aspx?mmgid=552&toxid=102#bookmark02
34. Environmental Protection Agency. Basic Information About NO2. Washington, DC: EPA (2023). Available online at: https://www.epa.gov/no2-pollution/basic-information-about-no2
35. Environmental Protection Agency. Ethylbenzene. Washington, DC: EPA (2016). Available online at: https://www.epa.gov/sites/default/files/2016-09/documents/ethylbenzene.pdf
36. Wang C, Wang Y, Cheng P, Xu L, Dang F, Wang T, et al. In-situ generated Tio2/α-FE2O3 heterojunction arrays for batch manufacturing of conductometric acetone gas sensors. Sens Actuators, B. (2021) 340:129926. doi: 10.1016/j.snb.2021.129926
37. Mazzatenta A, Pokorski M, Sartucci F, Domenici L, Di Giulio C. Volatile organic compounds (vocs) fingerprint of Alzheimer’s disease. Respir Physiol Neurobiol. (2015) 209:81–4. doi: 10.1016/j.resp.2014.10.001
38. Zhang K, Chang S, Fu Q, Sun X, Fan Y, Zhang M, et al. Occurrence and risk assessment of volatile organic compounds in multiple drinking water sources in the Yangtze river Delta region, China. Ecotoxicol Environ Saf. (2021) 225:112741. doi: 10.1016/j.ecoenv.2021.112741
39. Sydbom A, Blomberg A, Parnia S, Stenfors N, Sandström T, Dahlén S-E. Health effects of diesel exhaust emissions. Eur Respir J. (2001) 17(4):733–46. doi: 10.1183/09031936.01.17407330
40. Labaki WW, Han MK. Chronic respiratory diseases: a global view. Lancet Respir Med. (2020) 8(6):531–3. doi: 10.1016/s2213-2600(20)30157-0
41. Savitz DA, Woskie SR, Bello A, Gaither R, Gasper J, Jiang L, et al. Deployment to military bases with open burn pits and respiratory and cardiovascular disease. JAMA Network Open. (2024) 7(4):e247629. doi: 10.1001/jamanetworkopen.2024.7629
42. Zell-Baran LM, Krefft SD, Strand M, Rose CS. Longitudinal changes in lung function following post-9/11 military deployment in symptomatic veterans. Respir Med. (2024) 227:107638. doi: 10.1016/j.rmed.2024.107638
43. Patel D, Jaber J, Loso J, Perera H, Daouk S, Innabi A, et al. Sarcoidosis and burn pit exposure in military deployers to Iraq, Afghanistan, and Southwest Asia. Sarcoidosis Vasc Diffuse Lung Dis. (2023) 40(3):e2023035. doi: 10.36141/svdld.v40i3.13956
44. Fernandez JC, Ananthanarayanan V, Dilling DF. A case of constrictive bronchiolitis from burn-pit inhalational exposure in Iraq treated with tacrolimus. Am Thorac Soc J. (2017) 195:A5531. doi: 10.1164/ajrccm-conference.2017.195.1_MeetingAbstracts.A5531
45. Hill CJ, Meyer CD, McLean JE, Anderson DC, Hao Y, Lin F-C, et al. Burn pit exposure is associated with increased sinonasal disease. J Occup Environ Med. (2022) 64(8):629–34. doi: 10.1097/jom.0000000000002551
46. Teng J, Gao AY, Bowker W, Sher N, Singarajah C. Fire in the hole: giant bullous lung disease in a veteran with burn pit exposure and smoking. Chest. (2022) 162(4):A1974–5. doi: 10.1016/j.chest.2022.08.1626
47. Nunez ER, Rounds SI. Upper lobe cystic lung disease mimicking pulmonary langerhans cell histiocytosis in a veteran with burn pit exposure. Am J Respir Crit Care Med. (2018) B49(1):197. doi: 10.1164/ajrccm-conference.2018.197.1
48. Wang X, Doherty TA, James C. Military burn pit exposure and airway disease. Ann Allergy Asthma Immunol. (2023) 131(6):720–5. doi: 10.1016/j.anai.2023.06.012
49. Savabieasfahani M, Ahamadani F, Fadhel BT. Congenital anomalies in Baghdad children born near US military burn-pits: a case-control study showing tungsten and chromium association with increased odds of disease. Hyg Environ Health Adv. (2024) 9:100090. doi: 10.1016/j.heha.2024.100090
50. Hoisington AJ, Stearns-Yoder KA, Kovacs EJ, Postolache TT, Brenner LA. Airborne exposure to pollutants and mental health: a review with implications for United States veterans. Curr Environ Health Rep. (2024) 11(2):168–83. doi: 10.1007/s40572-024-00437-8
51. Vance SA, Kim YH, George IJ, Dye JA, Williams WC, Schladweiler MJ, et al. Contributions of particulate and gas phases of simulated burn pit smoke exposures to impairment of respiratory function. Inhalation Toxicol. (2023) 35(5–6):129–38. doi: 10.1080/08958378.2023.2169416
52. Rohan JG, Wu DX, Gargas NM, Wong BA. Measurement of Inflammatory Markers Resulting from Exposures to Southwest Asian Particulate Matter and Burn Pit Emissions in a Rat Model. Fort Belvoir, VA: Defense Technical Information Center (2021). Available online at: https://apps.dtic.mil/sti/pdfs/AD1147843.pdf
53. DelRaso NJ, Mauzy CA, Wong BA. Burn Pit Emission and Respirable Sand Exposures in Rats: NMR-Based Urinary Metabolomic Assessment. Fort Belvoir, VA: Defense Technical Information Center (2018). Available online at: https://apps.dtic.mil/sti/pdfs/AD1064148.pdf
54. Kim YH, Warren SH, Kooter I, Williams WC, George IJ, Vance SA, et al. Chemistry, lung toxicity and mutagenicity of burn pit smoke-related particulate matter. Part Fibre Toxicol. (2021) 18(1):45. doi: 10.1186/s12989-021-00435-w
55. Martin J, Monaghan-Benson E, Gomez JC, Kim Y, Gilmour MI, Doerschuk CM. The effect of a single exposure to simulated burn pit products in mice with healthy and inflamed airways. Am Thorac Soc. (2020) C58:201. doi: 10.1164/ajrccm-conference.2020.201.1_MeetingAbstracts.A5546
56. Li R, Sun Q, Lam SM, Chen R, Zhu J, Gu W, et al. Sex-dependent effects of ambient PM2.5 pollution on insulin sensitivity and hepatic lipid metabolism in mice. Part Fibre Toxicol. (2020) 17(1):14. doi: 10.1186/s12989-020-00343-5
Keywords: burn pit, emissions, veteran, lung pathology, environmental toxins, particulate matter, combustion, aerosolized exposure
Citation: Penuelas VL and Lo DD (2024) Burn pit exposure in military personnel and the potential resulting lung and neurological pathologies. Front. Environ. Health 3:1364812. doi: 10.3389/fenvh.2024.1364812
Received: 3 January 2024; Accepted: 23 May 2024;
Published: 21 June 2024.
Edited by:
Premkumari Kumarathasan, Environmental Health Sciences and Research Bureau, CanadaReviewed by:
Thomas Jackson, Research Triangle Park, United StatesKrishna Priya Syama, Environmental Health Science and Research Bureau, Canada
© 2024 Penuelas and Lo. This is an open-access article distributed under the terms of the Creative Commons Attribution License (CC BY). The use, distribution or reproduction in other forums is permitted, provided the original author(s) and the copyright owner(s) are credited and that the original publication in this journal is cited, in accordance with accepted academic practice. No use, distribution or reproduction is permitted which does not comply with these terms.
*Correspondence: David D. Lo, ZGF2aWQubG9AbWVkc2NoLnVjci5lZHU=