- 1Kweichow Moutai Winery (Group) Health Wine Co., Ltd, Renhuai, China
- 2Shaanxi Provincial Land Engineering Construction Group, Key Laboratory of Degraded and Unused Land Consolidation Engineering, Ministry of Natural Resources, Xi’an, China
- 3Shool of Resource and Environmental Engineering, Wuhan University of Science and Technology, Wuhan, China
- 4China Construction Third Engineering Bureau Second Construction Engineering Co., Ltd, Wuhan, China
Hydrogen peroxide (H2O2) is gaining recognition as an eco-friendly and highly effective algicide for combating cyanobacterial blooms. This study investigates the algicidal potential of H2O2 catalyzed by both inorganic and organic iron. Our findings indicate that inorganic iron (FeSO4) shows minimal catalytic activity on H2O2 under varying light conditions. In contrast, organic iron, specifically the combination of H2O2, EDTANaFe, and light irradiation, demonstrates significant algicidal effects. The optimal dosages were identified as 10 mg/L for H2O2 and 3 mg/L for Fe3+.The limited efficacy of inorganic iron stems from the transformation of Fe2+ to Fe3+ ions via the Fenton reaction. Under neutral conditions, Fe3+ ions precipitate as large-sized goethite, which adheres to the extracellular polymeric substances (EPS) of cyanobacterial cells, thereby hindering H2O2 catalysis and hydroxyl radical (·OH) formation in natural waters. Conversely, the combination of light radiation and organic iron salts greatly enhances the algicidal efficiency of H2O2. This synergy accelerates H2O2 decomposition and facilitates the production of a substantial amount of OH radicals by altering the Gibbs free energy. Thus, bright and sunny conditions, particularly in the afternoon, are crucial for effectively combating cyanobacterial blooms using Fenton-like reagents. The methodology presented in this study offers a viable solution to global cyanobacteria bloom issues and elucidates the mechanisms driving its efficacy.
1 Introduction
Eutrophication remains a pressing global issue, significantly impacting major lakes and rivers, particularly in closed or semi-closed hydrological systems (Suresh et al., 2023). This phenomenon not only disrupts aquatic ecosystems but also poses serious threats to drinking water sources and aquaculture, primarily through the proliferation of cyanobacterial blooms. These blooms can severely diminish water quality and biodiversity, underscoring the urgent need for effective management strategies. Various methods have been developed to combat cyanobacterial blooms (Shao et al., 2013; Zeng et al., 2023; Matthijs et al., 2012), each offering unique benefits and drawbacks. Among these approaches, the use of hydrogen peroxide (H2O2) has emerged as a particularly promising solution for controlling algae (Barrington and Ghadouani, 2008). Its effectiveness in reducing cyanobacterial growth, coupled with advantages over traditional methods, makes it a compelling option for water treatment processes. The ongoing challenge of eutrophication highlights the critical importance of innovative water management techniques. As such, exploring and refining H2O2-based algae control methods could play a vital role in protecting water resources and preserving aquatic ecosystems worldwide (Santos et al., 2021). This introduction sets the stage for a deeper examination of hydrogen peroxide’s potential, as well as the broader implications of its use in combating eutrophication and safeguarding aquatic environments.
Hydrogen peroxide generates highly reactive hydroxyl radicals (·OH), which are effective in damaging cyanobacteria due to their strong oxidizing properties. One of the key advantages of using H2O2 is its eco-friendliness; upon decomposition, it breaks down into water and oxygen (O2), minimizing environmental pollution. Studies indicate that low concentrations of H2O2, specifically below 50 mg/L, can effectively inhibit cyanobacterial growth (Weenink et al., 2015; Randhawa et al., 2013). However, there is a pressing need for further research to identify even lower concentrations that can satisfy engineering requirements without compromising efficacy. Additionally, a more comprehensive understanding of the reaction mechanisms at these low concentrations is essential for optimizing H2O2 use in practical applications. This knowledge will enhance the effectiveness of H2O2 as a sustainable solution for managing cyanobacterial blooms in aquatic systems. Reducing the use of H2O2 while lowering the associated costs of controlling bloom-forming cyanobacteria is essential for sustainable water management. This initiative involves not only refining the H2O2 application method but also optimizing its use to achieve maximum efficacy at minimal concentrations, thereby minimizing environmental impact. Factors such as light radiation and catalysts like iron salts can significantly enhance the rapid generation of reactive OH, increasing the algicidal effectiveness of H2O2.
Research suggests that the presence of light or small amounts of iron can boost the toxicity of H2O2, allowing for a reduced dosage while maintaining algicidal efficiency (Randhawa et al., 2013; Bokare and Choi, 2014). In advanced wastewater treatment, H2O2 catalyzed by iron salts, specifically FeSO4 (Fenton reagent) and EDTANaFe (Fenton-like reagent), is commonly employed due to their strong oxidizing properties, which can effectively degrade persistent organic pollutants (POPs) such as benzene derivatives (Ziembowicz and Kida, 2022). Despite their known efficacy in other applications, there is a notable gap in research regarding the use of trace amounts of Fenton or Fenton-like reagents specifically for controlling cyanobacterial blooms. This study aims to fill that gap by investigating the algicidal effects of H2O2 when catalyzed by various iron salts and elucidating the underlying mechanisms of their algicidal activity. Understanding these interactions will be helpful in developing more effective and environmentally sustainable strategies for controlling cyanobacterial blooms.
The Fv/Fm measurement is a standard technique used to assess the photosynthetic efficiency of algae, especially in terms of the maximum quantum yield of photosystem II (PSII). It provides insight into the health and photosynthetic potential of algal cells. A higher Fv/Fm ratio generally indicates healthy and functional algae, while a lower value may suggest stress, damage, or compromised viability (Tan et al., 2019). Research suggests that the Fv/Fm ratio is highly sensitive to changes in environmental conditions such as nutrient stress, light availability, and chemical treatments like hydrogen peroxide. Algal viability is closely tied to photosynthetic efficiency, so a significant drop in Fv/Fm would typically be associated with reduced viability or even cell death.
Objective: This study aims to investigate the algicidal effects of hydrogen peroxide (H₂O₂) catalyzed by iron salts, specifically FeSO₄ and EDTANaFe, for controlling cyanobacterial blooms. The objective is to identify how these catalysts influence the generation of reactive oxygen species (ROS) and elucidate the underlying mechanisms of their effectiveness at low concentrations.
Scope: This study is limited to investigating the catalytic effects of iron salts, particularly FeSO₄ and EDTANaFe, in the H₂O₂-based degradation of cyanobacteria under controlled laboratory conditions. The study focuses on understanding the reaction mechanisms at low concentrations of H₂O₂ (below 10 mg/L) and the potential enhancement of algicidal efficiency through the presence of light and iron catalysts. The study does not address the use of H₂O₂ in larger-scale field applications or explore other types of catalysts beyond iron salts.
2 Materials and methods
2.1 Algae acquisition and culture
The bloom-forming cyanobacteria were obtained from the Freshwater Algae Culture Collection at the Institute of Hydrobiology, Chinese Academy of Sciences (Supplementary Table S1). The test organisms were cultured in sterilized BG11 medium at 25°C under continuous fluorescent white light (50 μmol m⁻2s⁻1) with a light/dark cycle of 12 h/12 h (Lee et al., 2015). The BG11 medium is commonly used for the large-scale cultivation and test of cyanobacteria, and the experimental results are generally consistent with those observed in real water (Pandey et al., 2023). The cultures were manually agitated every 8 h. The growth state of the organisms was monitored every 2 days by measuring the optical density of cells at 685 nm (OD685) using a spectrophotometer (UV-2800, Unico, Shanghai, China). The concentration of cyanobacteria was consistently maintained at an OD685 of 0.8 in all subsequent experiments. The cultures were harvested for experiments during the exponential growth phase (Randhawa et al., 2013). The pH value was set at 7.4 and changed with time (Supplementary Figure S1).
2.2 Batch algicidal experiment
Microcystis Aeruginosa (MA), separated from the culture medium through a centrifugation process (4,000 rpm, 10 min), was diluted to OD685 = 0.8 using newly prepared BG11 medium (without FeSO4). A suitable volume of FeSO4 and H2O2 was added to the algae solutions, with the H2O2 concentration set at 10 mg/L and Fe2+ concentrations of 0, 1, 2.5, 5, and 10 mg/L, respectively (Experiment started). Fv/Fm, a performance index of biological activity (Li et al., 2010), was measured at 0, 0.5, 1, 2, 4, 6, and 8 h after adding H2O2 and FeSO4. All samples were incubated at 25°C under dark conditions and continuous fluorescent white light (50, 150, and 300 μmol m-2s-1). Each experiment was conducted in triplicate.
MA and Microcystis Panniformisin (MP) was collected from culture through centrifuge process respectively, and diluted to OD685 = 0.8 by new-prepared BG11 (without FeSO4). EDTANaFe and H2O2 were added to the algae solutions, so that the H2O2 concentration was 10 mg/L, and the Fe3+ concentration was 0, 1, 2.5, 5, 10, and 20 mg/L respectively. Fv/Fm was tested at 1.5 h, 4 h, 24 h or 4 days after adding the reagents. All the samples were incubated at 25°C, under dark and continuous fluorescent white light (50 μmol m-2s-1) conditions. Each experiment had three duplicates.
Eight kinds of common bloom cyanobacteria were separately collected from the culture through a centrifugation process and diluted to OD685 = 0.8 using newly prepared BG11 medium (without FeSO4). EDTANaFe and H2O2 were then added to every algae solution, resulting in an H2O2 concentration of 10 m/L and Fe3+ concentrations of 3 mg/L, respectively. Fv/Fm was measured at 24 h after adding the reagents, and photographs were taken for each type of algae after 4 days. All samples were incubated at 25°C under continuous fluorescent white light (50 μmol m-2s-1). Each experiment was conducted in triplicate.
2.3 Iron distribution analysis and the particle size testing
Algae MA and MP were collected from culture through a centrifugation process and diluted to OD685 = 0.8 using newly prepared BG11 medium (without FeSO4). The samples were divided into two groups: group 1, which was treated with H2O2 and FeSO4 (H2O2 = 10 mg/L, Fe2+ = 3 mg/L), and group 2, which was treated with H2O2 and EDTANaFe (H2O2 = 10 mg/L, Fe3+ = 3 mg/L). The samples were then incubated at 25°C under continuous fluorescent white light (50 μmol m-2s-1). Each group was manually agitated every 15 min, and after 2 hours, the samples were centrifuged (4,000 rpm) to obtain supernatant and algal body fractions.
The supernatant was filtered using a 0.45 µm microfiltration membrane, and then the concentrations of Fe2+, Fe3+ and total iron were measured (Fortune and Mellon, 1938). The algae body was washed with 0.01M PBS and dried for 24 h under vacuum freeze conditions and then analyzed by SEM-EDS. Meanwhile, FeSO4 and EDTANaFe were separately added into a 0.01 M PBS solution (pH = 7.4) to achieve total iron concentrations of 5, 50, 500, and 2000 m/L. The solution was treated at 25°C under continuous fluorescent white light (50 μmol m-2s-1). The particle size of the solution was measured using a Malvern Particle Size Analyzer (ZS90).
2.4 Hydrogen peroxide degradation kinetics and hydroxyl radical yield test
The concentration of H2O2 was measured using the four-channel free radical analyzer TRB4100 (WPI). The TBR4100 free radical detector can convert the chemical signals produced by H2O2 on the detection probe into electrical signals and record and analyze them in real time (Young et al., 2011). Detailed procedures see Supporting Information (Supplementary Section S1). The yield of OH was determined using the rapid capture method with methylene blue (MB). Fenton regent (H2O2 was 10 mg/L, inorganic iron of FeSO4 was 3 mg/L) and Fenton-like regent (H2O2 was 10 mg/L, organic iron of EDTANaFe was 3 mg/L) were added in methylene blue (MB, 60 μM) solution respectively under magnetic stirring condition. The reactions proceeded under dark and light (50 μmol m-2s-1) condition. After 5 min of reaction, the absorbance value (OD660) was immediately measured to calculated the corresponding concentration of MB. Subsequently, the MB concentration was substituted into Equation 1 to calculate the amount of OH within 5 min, which was used to represent the yield of hydroxyl radical.
Ck: Indicates the MB concentration without adding H2O2 group.
C5min: Represents the MB concentration after treatment with the corresponding H2O2 dose for 5 min.
3 Results and discussion
3.1 Algicidal effect of H2O2 catalyzed by inorganic iron
The algicidal effect of H2O2 catalyzed by FeSO4 was evaluated using chlorophyll fluorescence (Fv/Fm), as shown in Figure 1. Significant differences were observed between dark and light conditions, with Fv/Fm values being lower under light (Figures 1A,B). Furthermore, as light intensity increased, the reduction in Fv/Fm became more pronounced (Figures 1A–D). When the light intensity is set at 50 μmol m-2s-1, the Fv/Fm values for the experimental groups with ferrous iron doses of 5 and 10 mg/L are above the death line (Figure 1B). The phenomenon shows that the addition of inorganic ferrous salts not only fails to enhance the algicidal effect of H2O2 but actually reduces its toxicity against algae. Fv/Fm is an important parameter for assessing photosynthetic performance and is highly sensitive. When photosynthetic organisms like cyanobacteria experience external stress, Fv/Fm responds immediately (Baker, 2008). If the Fv/Fm value drops below 0.1, it is highly likely that recovery to a normal state is difficult, and it can be considered a criterion for the death of cyanobacteria (Zhu et al., 2020). When the light intensity is increased to 150 μmol m-2s-1, all experimental groups have Fv/Fm values below the death line. At a light intensity of 300 μmol m-2s-1, all experimental groups show almost complete failure in Fv/Fm. This suggests that light significantly enhances the algicidal effect of Fenton reagents. These results are consistent with findings by Mikula, and Jia, who reported that light radiation stimulates the rapid production of OH by H2O2, thereby enhancing the algicidal effect (Mikula et al., 2012; Jia et al., 2018).
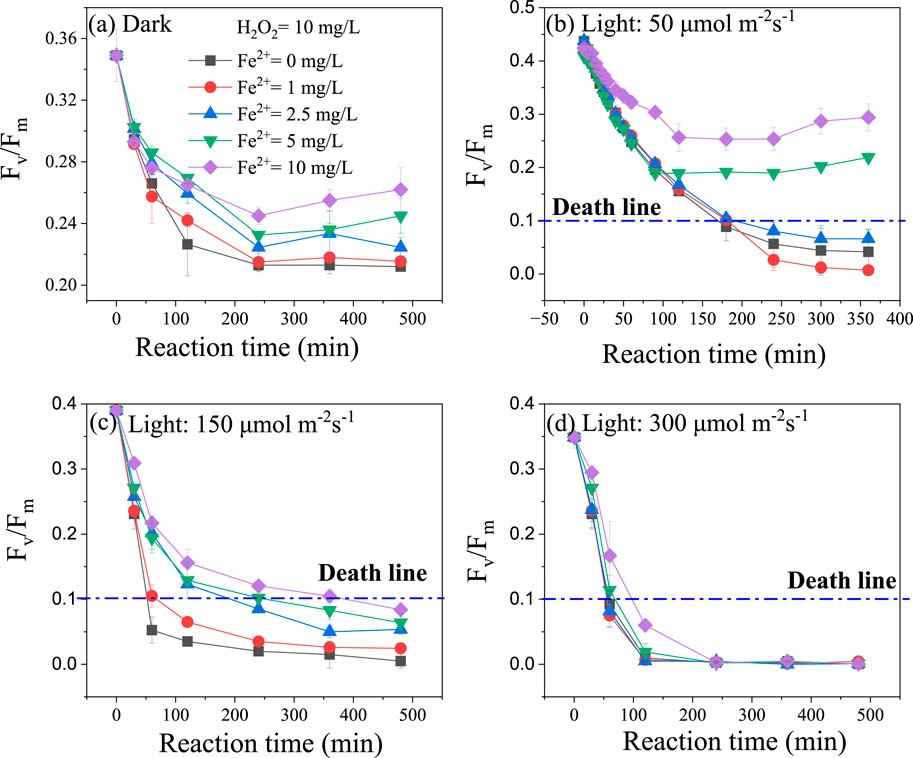
Figure 1. Effect of inorganic iron (Fe2+) concentration on Fv/Fm variation in microcystis aeruginosa under 0 (A), 50 (B), 150 (C), and 300 μmol·m−2s−1 of light intensity (D). Experiment condition: OD685 = 0.8; H2O2 = 10 mg/L; Fe2+= 0∼10 mg/L; Temperature= 25°C.
The phenomenon where the addition of a ferrous catalyst actually inhibited the oxidative performance of H2O2 contradicts traditional understanding (Huang et al., 2016). As a catalyst for H2O2, FeSO4 played an adverse role from Figures 1A–D. Traditional Fenton reagents (H2O2+FeSO4) require an acidic environment (pH < 4) for optimal reaction, as excessively high pH values (pH > 6) tend to suppress the formation of OH (Hermosilla et al., 2009). The progression of the Fenton reaction depended on the existence morphology of iron, as illustrated in Figure 2. Figure 2 illustrated the distribution of iron complexes with pH using the MINTEQ model (Le Calve et al., 1997). When the pH value was above 4, Fe3+ precipitated completely, primarily forming goethite (FeOOH), which adsorbed onto the extracellular polymeric substances (EPS) on the surface of Microcystis Aeruginosa. This hindered the further advancement of the Fenton reaction. Additionally, iron salts serve as nutrient substances required for the growth of algae (Chen et al., 2011). A small amount of Fe2+ could actually enhance biological activity. Consequently, traditional Fenton reagents cannot be effectively employed to control water blooms in natural lakes.
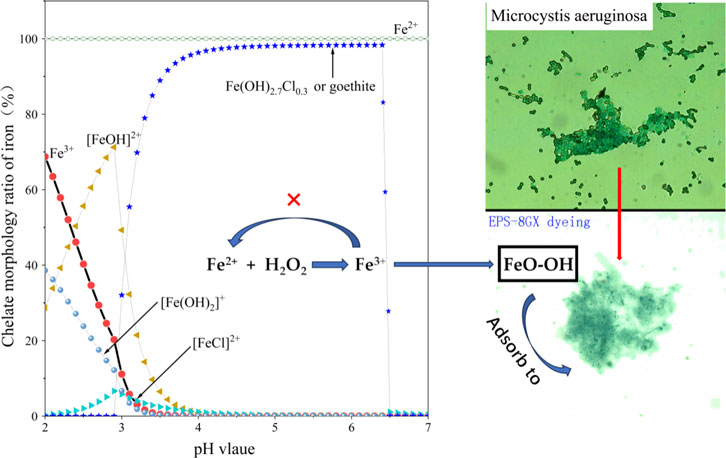
Figure 2. Chelate morphology ratio of iron and its oxide adsorbed onto EPS (fitted by MINTEQ model).
3.2 Algicidal effect of H2O2 catalyzed by organic iron
To prevent deactivation caused by inorganic iron precipitation under natural conditions, organically complexed iron was selected for subsequent experiments. Organic iron chelators, such as EDTA or DTPA, have been found to enhance the Fenton reaction and broaden the effective pH range (Zhang and Zhou, 2019; Clarizia et al., 2017), therefore, we used EDTANaFe as the representative organic iron compound for the experiments. Figures 3A,C illustrate that the Fv/Fm ratios measured at 1.5, 4, and 24 h, as well as 4 days, fluctuated with increasing concentrations of organic Fe3+. Regardless of the ferrous ion concentration, the Fv/Fm values are generally above 0.2 (MA: Fv/Fm > 0.2; MP: Fv/Fm > 0.3). This indicates that the algicidal effect of H2O2 catalyzed by EDTANaFe is ineffective under dark conditions.
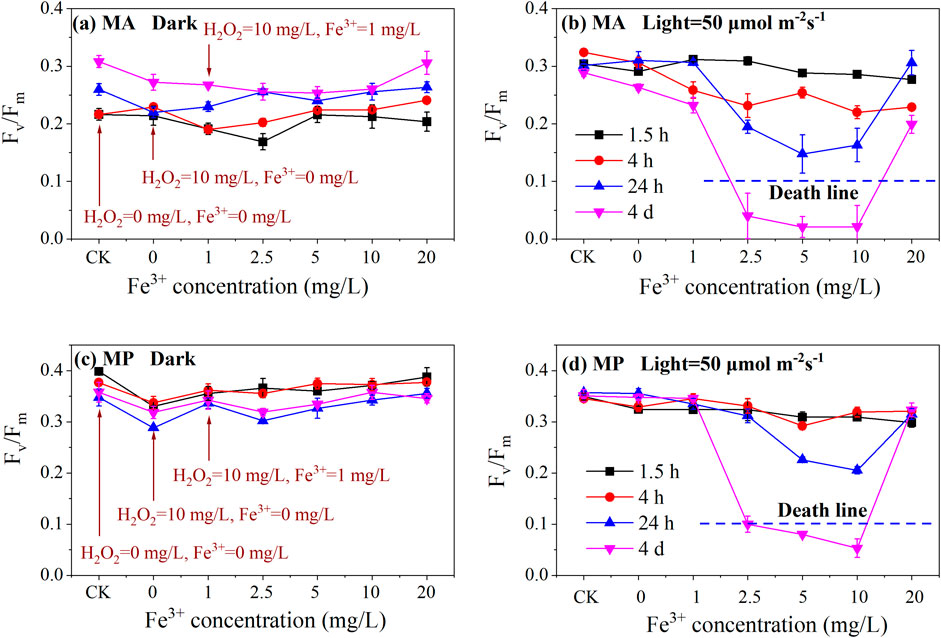
Figure 3. Effect of organic iron (EDTANaFe, Fe3+) concentration on Fv/Fm variation under 0 (A) and 50 (B) μmol·mm−2s−1 of light intensity for MA, 0 (C) and 50 (D) μmol·mm−2s−1 of light intensity for MP. Experiment condition: OD685 = 0.8; H2O2 = 10 mg/L; Fe3+ = 0∼20 mg/L; T = 25℃; MA = microcystis aeruginosa; MP = microcystis panniformisin.
In contrast, the results under light conditions (as depicted in Figures 2B,D) were markedly different from those in the dark. The Fv/Fm values of the experimental group were significantly lower than those of the control group (H2O2 =10 mg/L, Fe3+ = 0 mg/L), regardless of whether the algae were Microcystis Aeruginosa (MA) or Microcystis Panniformis (MP). This suggests that the addition of organic iron (EDTANaFe) was effective in killing cyanobacteria under light conditions.
Figures 2B,D also demonstrated that Fv/Fm reached its lowest point (below death line) when the organic iron (Fe3+) ranged from 2.5 to 10 mg/L. This indicates that the Fenton-like reagents caused lethal damage to the algae MA and MP under light conditions. Both insufficient (<2.5 mg/L) and excessive (>10 mg/L) concentrations of organic Fe3+ were not conducive to effectively eliminating the algae. In general, the parameters of H2O2 = 10 mg/L and organic Fe3+=2.5–10 mg/L were found to be suitable for controlling water bloom. The molar ratio of H2O2 to Fe(II/III) in the photo-Fenton process affects the efficiency of ferric ion (Liu et al., 2018).
Low Fe3+ concentrations catalyze H2O2 decomposition to generate hydroxyl radicals, with OH concentration increasing as Fe3+ levels rise. However, an excess of Fe3+ can consume a significant amount of OH, leading to the ineffective decomposition of H2O2. Furthermore, Figures 2B,D showed that the Fv/Fm ratio gradually decreased over time (from 1.5 h to 4 days), indicating that the algicidal effect took several days to manifest. This suggests that the appearance of algicidal effects lagged by a few days.
Light radiation played a crucial role in the eradication of bloom-forming cyanobacteria using Fenton-like reagent, enhancing the toxicity of H2O2 (Sukenik and Kaplan, 2021). The use of Fenton-like reagents in the presence of light was markedly effective in killing algae because light radiation also served as a catalyst, promoting the production of OH. Additionally, light can increase the transformation efficiency between Fe2+ and Fe3+, thereby allowing the Fenton reaction to proceed to completion (Mao et al., 2024).
Based on the aforementioned findings, eight primary bloom-forming cyanobacteria were selected for treatment, and the results are depicted in Figure 4. After treatment with Fenton-like reagents (H2O2 = 10 mg/L; organic Fe3+=3 m/L), all eight selected cyanobacterial strains exhibited various reductions in Fv/Fm, indicating the varying efficacy of Fenton-like reagents in controlling different cyanobacterial species. With the exception of strain E, the other seven strains suffered fatal damage, leading to the subsequent death of the majority of cyanobacteria in the following days. These results confirmed that the combination of H2O2, organic iron, and light radiation exerted a significant algicidal effect. It is noteworthy that the elimination of cyanobacteria cells may lead to the rise of algae membrane permeability (Supplementary Figure S2) and the release of cyanotoxins (Schneider and Bláha, 2020), posing significant ecological and health risks to the aquatic environment.
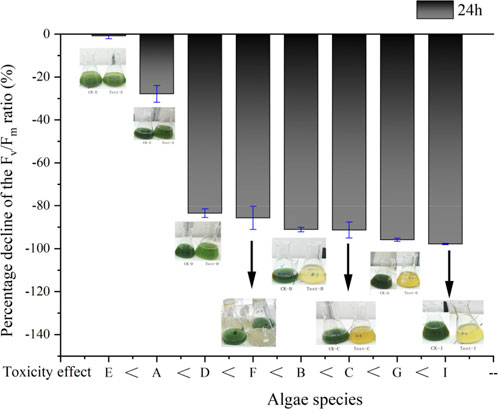
Figure 4. Percent decline of Fv/Fm after adding Fenton-like reagent (test result after 24 h). Experiment condition: OD685 = 0.8; H2O2 = 10 m/L; Fe3+ = 3 mg/L; Temperature = 25°C; Light intensity = 50 μmol m-2s-1; A (M. aeruginosa); B (M. flos-aquae); C (M. wesenbergii); D (M. viridis); E (M. ichthyoblabe); F (M. panniformis); G (A. flos-aquae); I (A. cylindrical).
3.3 Catalytic mechanism of iron when killing the algae using H2O2
To explain why organic iron catalyzes H2O2 to kill cyanobacteria more effectively than inorganic iron. Two hours after the addition of the Fenton reagents, iron concentration was measured, as shown in Figure 5A. Regardless of whether in the MA solution or the MP solution, Fe3+ remained the predominant species after 2 h of reaction in both groups (inorganic iron and organic iron). The total iron concentration in Group 1 (inorganic iron, FeSO4) was significantly lower than that in Group 2 (organic iron, EDTANaFe). Figure 5A also indicated that only about 10% of Fe(II) remained in solution for Group 1, whereas 35% of Fe(II) was still present in the solution for Group 2. These findings suggest that the majority of the iron salt in Group 1 might have precipitated onto the surface of the algae cells, while the iron salt in Group 2 remained in a dissolved state. This aligns with several studies that have explored the interplay between Fe(II) and Fe(III) (Fujii et al., 2010; Wang et al., 2017).
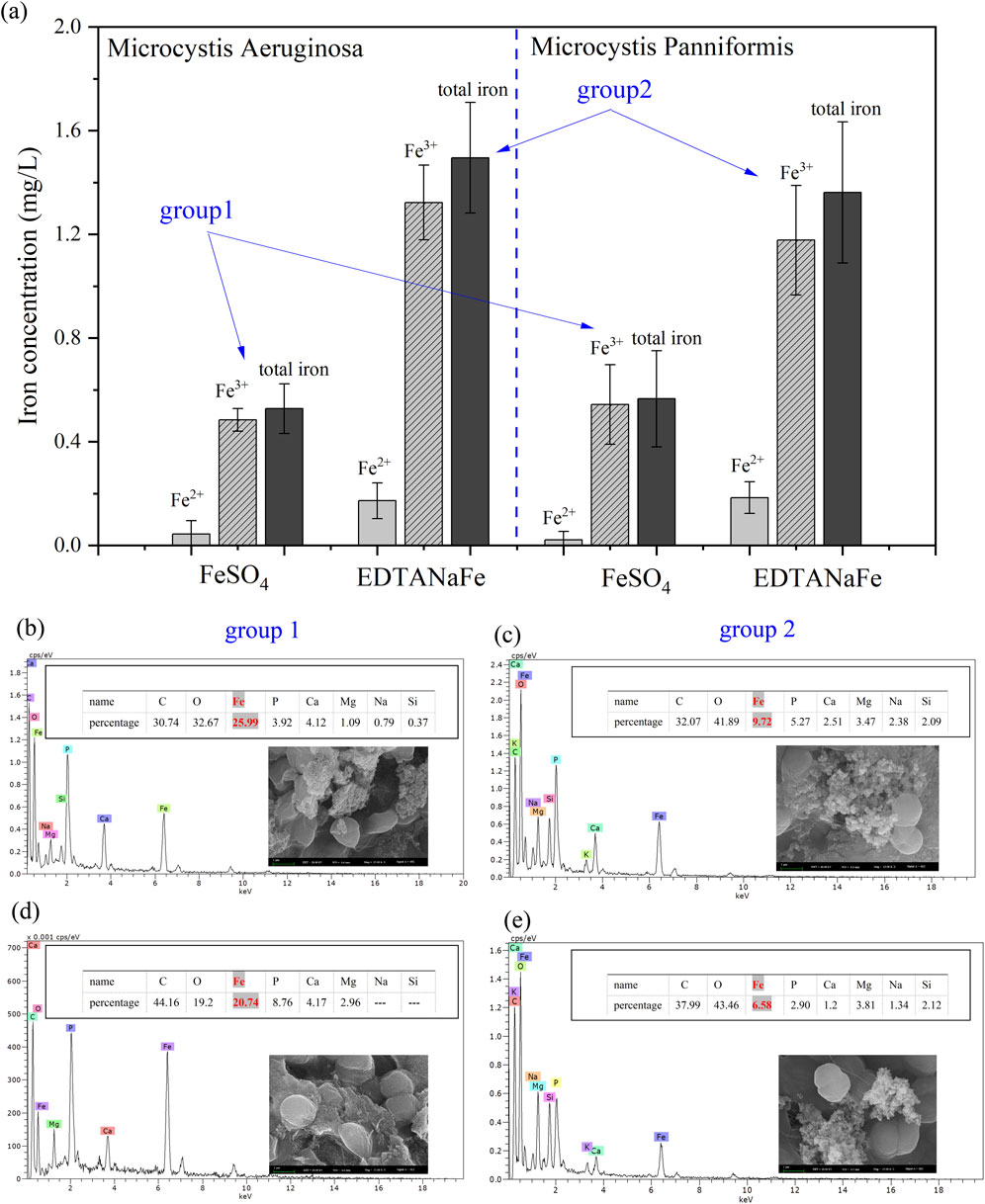
Figure 5. Iron concentration in algae solution (A); Percentage of iron on the surface of algae for group MA+FeSO4 (B), group MA+EDTANaFe (C), group MP+FeSO4 (D), group MP+EDTANaFe (E).
To investigate the distribution of iron on the surface of algae cells, SEM images of the two sample groups were obtained. Figures 5B–E show complex compounds surrounding the cell surfaces, composed of EPS. Figures 5B,D reveal that the percentage of iron on the surface of MA and MP (inorganic iron, group 1) was 25.99% and 20.74%, respectively, exceeding the percentage of iron (9.72% and 6.58%) in group 2 (organic iron). This indicates a greater amount of iron deposition and adsorption on the surface of cyanobacterial cells in group 1. This difference can be attributed to the tendency of FeSO4 to form larger particles (goethite) that are more readily adsorbed by the EPS on the cell surface, as demonstrated in Figure 2, while organic iron remained dissolved throughout the entire duration. These findings suggest that the physical characteristics of iron compounds, influenced by their chemical form, significantly affect their interaction with algal cells (Mishra et al., 2011).
Figure 6 further illustrates the particle sizes in the FeSO4 and EDTANaFe solutions, revealing that the particle size in the FeSO4 solution was significantly larger than that in EDTANaFe solution at the same iron concentration. In both solutions, particle size increased progressively with rising iron concentrations. Iron in chelated states, such as in the EDTANaFe solution, remained dissolved, whereas the inorganic iron in the FeSO4 solution existed in particulate form. This difference explains why chelating agents like EDTANaFe are crucial for maintaining catalytic properties during Fenton-like processes (Wang et al., 2017).
Iron oxides, such as goethite, are more readily adsorbed by EPS surrounding the surface of algal cells (as shown in Figure 2). Daoyong Zhang’s research suggested that EPS from biofilms were important organic ligands for metal complexation and can significantly affect their chemical forms, mobility, bioavailability, and ecotoxicity (Zhang et al., 2010). As a result, there was a reduction in the catalytic components available in the solution. Conversely, the Fe3+ ions in the EDTANaFe solution were relatively difficult to adsorb onto the surface of the cells. This difference in adsorption behavior affected the availability of iron for catalytic reactions in the water, potentially impacting the efficacy of algae treatments involving these iron forms (Li et al., 2016). Therefore, we may confidently conclude that: Fe2+ in the FeSO4 solution was oxidized to Fe3+ and subsequently precipitated as sediment (goethite), whereas the Fe3+ in the EDTANaFe solution remained in a chelated state, preserving its catalytic activity.
The specific interactions of chelates are relatively complex. However, for the Fe-EDTA chelate system, Mingqiong Wang (Wang and Xu, 2012) have detailed how EDTA interacts specifically with iron ions during the degradation of organic matter (as Equations 2, 3). The presence of EDTA acts like a crab, competing with other anions to capture iron ions. This competition alters its precipitation equilibrium state, as defined by the equilibrium constant Log K (Kontoghiorghes, 2020). Thus, EDTA ensures that iron remains in a hydrated ionic state, facilitating the continuous cycling of ferric (Fe³⁺) and ferrous (Fe2⁺) ions. This cycling process consistently generates reactive oxygen species (ROS) such as hydroxyl radicals (˙OH) and hydroperoxyl radicals (HO₂˙).
3.4 H2O2 decomposition kinetics and radical yield analysis
To elucidate the reasons why inorganic iron catalyzes the ineffectiveness of H2O2 in eliminating cyanobacteria in natural water bodies while organic iron catalyzes its effectiveness, we measured the variations of H2O2 concentration under six different conditions and monitored the corresponding yields of hydroxyl radicals, as depicted in Figure 7.
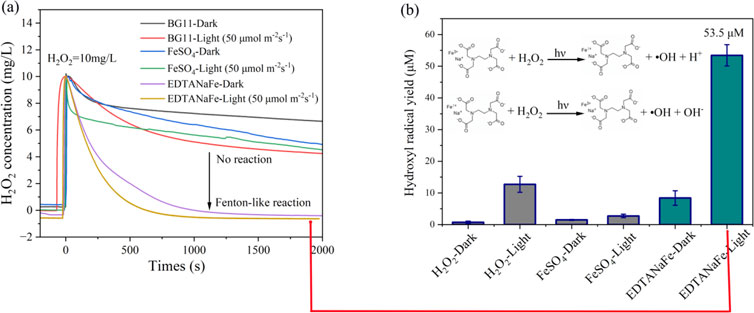
Figure 7. (A) H2O2 decomposition kinetics in various conditions; (B) yields of hydroxyl radical within initial 5 minutes.
As shown in Figure 7A, in BG11 culture medium (with only H2O2 present), illumination (50 μmol m-2s-1) significantly enhanced the degradation rate of H2O2 and increased the production yield of hydroxyl radicals (Figure 7B). The addition of inorganic iron (FeSO4) did not noticeably alter the trend of H2O2 degradation (Figure 7A). The addition of FeSO4 also did not significantly increase the production of free radicals hydroxyl radicals (Figure 7B). Overall, it was difficult for H2O2 catalyzed by inorganic ferrous iron to produce hydroxyl radicals under neutral conditions (Wang et al., 2012).
The addition of organic iron (EDTANaFe) significantly accelerated the degradation rate of H2O2 under both dark and illuminated conditions, achieving complete degradation of 10 mg/L H2O2 within half an hour (Figure 7A). However, Figure 7B indicated that although H2O2 degraded rapidly, the production yield of hydroxyl radicals was low under dark conditions, suggesting that H2O2 was predominantly undergoing ineffective decomposition. Conversely, under illuminated conditions, the degradation of H2O2 produced a significant amount of OH (53.5 μM within 5 min), indicating effective decomposition. This is also why EDTANaFe exhibits significant algicidal effects only under light conditions. The main reason for the effective degradation of H2O2 might be that, under light radiation, the catalytic reaction activation energy (Ea) for the process generating free radicals is lower compared to that in the absence of light radiation (Fındık, 2022). This change in energy states influences the production of free radicals, affecting the overall algicidal effect. Consequently, it can be affirmed that in natural water bodies, achieving optimal cyanobacteria eradication with H2O2 (10 mg/L) necessitates the addition of a small amount of organic iron (EDTANaFe, 3 mg/L) under illuminated conditions.
4 Conclusion
This study investigated the effectiveness of inorganic and organic iron-catalyzed H2O2 in eliminating cyanobacteria under different light conditions. The results indicated that light radiation significantly enhanced the algicidal effect of H2O2 by increasing the H2O2 decomposition rate and the production yield of hydroxyl radicals. The addition of inorganic iron negatively impacted H2O2 algicidal activity, regardless of light or dark conditions. Under dark conditions, the addition of organic iron (EDTANaFe) did not significantly enhance H2O2 algicidal activity. However, under light conditions, it significantly improved the effectiveness of H2O2 in killing cyanobacteria. The optimal dosage of the Fenton-like reagent was H2O2 at 10 mg/L and Fe3+ at 3 mg/L, which could eradicate most common cyanobacteria (OD685 = 0.8). The significantly lower efficacy of inorganic iron in catalyzing H2O2 to eradicate cyanobacteria, compared to organic iron, was attributed to the rapid conversion of Fe3+ ions (formed through the Fenton reaction by inorganic ferrous) to goethite (FeOOH) under neutral conditions, which rapidly adsorbed onto the EPS of cyanobacterial cells. This blocked the continuous progression of the Fenton reaction. In contrast, organic iron in EDTANaFe remained in a homogeneous dissolved state, ensuring the continuous progression of the Fenton-like reaction and the generation of hydroxyl radicals. Experimental results from hydrogen peroxide degradation kinetics confirmed that the addition of inorganic iron did not significantly increase the degradation rate of H2O2, while the addition of organic iron significantly enhanced its degradation. However, significant hydroxyl radical generation only occurred under light conditions in the Fenton-like system. This synergy accelerates H₂O₂ decomposition and facilitates the production of a substantial amount of ·OH radicals under light conditions, possibly due to the reduction of catalytic reaction activation energy (Ea) as suggested by thermodynamic principles and supported by observed experimental trends. This explained why only under light conditions could organic iron catalyze H2O2 to kill cyanobacteria with high efficiency.
Data availability statement
The original contributions presented in the study are included in the article/Supplementary Material, further inquiries can be directed to the corresponding author.
Author contributions
YL: Investigation, Data curation, Software, Writing–original draft. ZN: Formal Analysis, Investigation, Methodology, Writing–review and editing. JL: Project administration, Writing–original draft, Conceptualization, Resources, Visualization. SL: Investigation, Methodology, Project administration, Writing–original draft. HL: Data curation, Formal Analysis, Funding acquisition, Writing–review and editing. LL: Software, Writing–original draft, Resources, Supervision, Writing–review and editing.
Funding
The author(s) declare that financial support was received for the research, authorship, and/or publication of this article. This research was funded by Shaanxi Dijian Group internal project (DJNY 2024-32).
Conflict of interest
Authors YL and LL were employed by the Kweichow Moutai Winery (Group) Health Wine Co., Ltd. Authors ZN and JL were employed by the Shaanxi Provincial Land Engineering Construction Group. Author HL was employed by the China Construction Third Engineering Bureau Second Construction Engineering Co., Ltd.
The remaining author declares that the research was conducted in the absence of any commercial or financial relationships that could be construed as a potential conflict of interest.
Generative AI statement
The authors declare that no Generative AI was used in the creation of this manuscript.
Publisher’s note
All claims expressed in this article are solely those of the authors and do not necessarily represent those of their affiliated organizations, or those of the publisher, the editors and the reviewers. Any product that may be evaluated in this article, or claim that may be made by its manufacturer, is not guaranteed or endorsed by the publisher.
Supplementary material
The Supplementary Material for this article can be found online at: https://www.frontiersin.org/articles/10.3389/fenve.2024.1516999/full#supplementary-material
References
Baker, N. R. (2008). Chlorophyll fluorescence: a probe of photosynthesis in vivo. Annu. Rev. Plant Biol. 59 (1), 89–113. doi:10.1146/annurev.arplant.59.032607.092759
Barrington, D. J., and Ghadouani, A. (2008). Application of hydrogen peroxide for the removal of toxic cyanobacteria and other phytoplankton from wastewater. Environ. Sci. and Technol. 42 (23), 8916–8921. doi:10.1021/es801717y
Bokare, A. D., and Choi, W. (2014). Review of iron-free Fenton-like systems for activating H2O2 in advanced oxidation processes. J. Hazard. Mater. 275, 121–135. doi:10.1016/j.jhazmat.2014.04.054
Chen, M., Tang, H., Ma, H., Holland, T. C., Ng, K. S., and Salley, S. O. (2011). Effect of nutrients on growth and lipid accumulation in the green algae Dunaliella tertiolecta. Bioresour. Technol. 102 (2), 1649–1655. doi:10.1016/j.biortech.2010.09.062
Clarizia, L., Russo, D., Di Somma, I., Marotta, R., and Andreozzi, R. (2017). Homogeneous photo-Fenton processes at near neutral pH: a review. Appl. Catal. B Environ. 209, 358–371. doi:10.1016/j.apcatb.2017.03.011
Fındık, S. (2022). Decolorization of direct black 22 by photo Fenton like method using UV light and zeolite modified zinc ferrite: kinetics and thermodynamics. Acta Chim. Slov. 69, 552–563. doi:10.17344/acsi.2022.7431
Fortune, W. B., and Mellon, M. G. (1938). Determination of iron with o-phenanthroline: a spectrophotometric study. Industrial and Eng. Chem. Anal. Ed. 10 (2), 60–64. doi:10.1021/ac50118a004
Fujii, M., Rose, A. L., Omura, T., and Waite, T. D. (2010). Effect of Fe (II) and Fe (III) transformation kinetics on iron acquisition by a toxic strain of Microcystis aeruginosa. Environ. Sci. and Technol. 44 (6), 1980–1986. doi:10.1021/es901315a
Hermosilla, D., Cortijo, M., and Huang, C. P. (2009). Optimizing the treatment of landfill leachate by conventional Fenton and photo-Fenton processes. Sci. Total Environ. 407 (11), 3473–3481. doi:10.1016/j.scitotenv.2009.02.009
Huang, X., Hou, X., Zhao, J., and Zhang, L. (2016). Hematite facet confined ferrous ions as high efficient Fenton catalysts to degrade organic contaminants by lowering H2O2 decomposition energetic span. Appl. Catal. B Environ. 181, 127–137. doi:10.1016/j.apcatb.2015.06.061
Jia, P., Zhou, Y., Zhang, X., Zhang, Y., and Dai, R. (2018). Cyanobacterium removal and control of algal organic matter (AOM) release by UV/H2O2 pre-oxidation enhanced Fe (II) coagulation. Water Res. 131, 122–130. doi:10.1016/j.watres.2017.12.020
Kontoghiorghes, G. J. (2020). Advances on chelation and chelator metal complexes in medicine. Int. J. Mol. Sci. 21 (7), 2499. doi:10.3390/ijms21072499
Le Calve, S., Le Bras, G., and Mellouki, A. (1997). Temperature dependence for the rate coefficients of the reactions of the OH radical with a series of formates. J. Phys. Chem. A 101 (30), 5489–5493. doi:10.1021/jp970554x
Lee, C. S., Lee, S. A., Ko, S. R., Oh, H. M., and Ahn, C. Y. (2015). Effects of photoperiod on nutrient removal, biomass production, and algal-bacterial population dynamics in lab-scale photobioreactors treating municipal wastewater. Water Res. 68, 680–691. doi:10.1016/j.watres.2014.10.029
Li, L., Chen, X., Zhang, D., and Pan, X. (2010). Effects of insecticide acetamiprid on photosystem II (PSII) activity of Synechocystis sp.(FACHB-898). Pesticide Biochem. Physiology 98 (2), 300–304. doi:10.1016/j.pestbp.2010.06.022
Li, L., Song, W., Deng, C., Zhang, D., Al-Misned, F. A., Mortuza, M. G., et al. (2016). Effects of pH and salinity on adsorption of hypersaline photosynthetic microbial mat exopolymers to goethite: a study using a quartz crystal microbalance and fluorescence spectroscopy. Geomicrobiol. J. 33 (3-4), 332–337. doi:10.1080/01490451.2015.1052120
Liu, X., Zhou, Y., Zhang, J., Luo, L., Yang, Y., Huang, H., et al. (2018). Insight into electro-Fenton and photo-Fenton for the degradation of antibiotics: mechanism study and research gaps. Chem. Eng. J. 347, 379–397. doi:10.1016/j.cej.2018.04.142
Mao, H., Chen, F., Zhang, Q., Zhuang, L., Li, X., and Yao, C. (2024). Photogenerated and cocatalytic system cooperate to accelerate Fe3+/Fe2+ cycle in CNFe/FeS2/MoS2 composite with Z-scheme heterojunction constructed by interfacial chemical bond for advanced oxidation processes. J. Environ. Chem. Eng. 12 (1), 111708. doi:10.1016/j.jece.2023.111708
Matthijs, H. C., Visser, P. M., Reeze, B., Meeuse, J., Slot, P. C., Wijn, G., et al. (2012). Selective suppression of harmful cyanobacteria in an entire lake with hydrogen peroxide. Water Res. 46 (5), 1460–1472. doi:10.1016/j.watres.2011.11.016
Mikula, P., Zezulka, S., Jancula, D., and Marsalek, B. (2012). Metabolic activity and membrane integrity changes in Microcystis aeruginosa–new findings on hydrogen peroxide toxicity in cyanobacteria. Eur. J. Phycol. 47 (3), 195–206. doi:10.1080/09670262.2012.687144
Mishra, A., Kavita, K., and Jha, B. (2011). Characterization of extracellular polymeric substances produced by micro-algae Dunaliella salina. Carbohydr. Polym. 83 (2), 852–857. doi:10.1016/j.carbpol.2010.08.067
Pandey, S., Narayanan, I., Vinayagam, R., Selvaraj, R., Varadavenkatesan, T., and Pugazhendhi, A. (2023). A review on the effect of blue green 11 medium and its constituents on microalgal growth and lipid production. J. Environ. Chem. Eng. 11 (3), 109984. doi:10.1016/j.jece.2023.109984
Randhawa, V., Thakkar, M., and Wei, L. (2013). Effect of algal growth phase on Aureococcus anophagefferens susceptibility to hydrogen peroxide. Aquat. Toxicol. 142-143, 230–238. doi:10.1016/j.aquatox.2013.08.015
Santos, A. A., Guedes, D. O., Barros, M. U., Oliveira, S., Pacheco, A. B., Azevedo, S. M., et al. (2021). Effect of hydrogen peroxide on natural phytoplankton and bacterioplankton in a drinking water reservoir: mesocosm-scale study. Water Res. 197, 117069. doi:10.1016/j.watres.2021.117069
Schneider, M., and Bláha, L. (2020). Advanced oxidation processes for the removal of cyanobacterial toxins from drinking water. Environ. Sci. Eur. 32 (1), 94. doi:10.1186/s12302-020-00371-0
Shao, J., Li, R., Lepo, J. E., and Gu, J. D. (2013). Potential for control of harmful cyanobacterial blooms using biologically derived substances: problems and prospects. J. Environ. Manag. 125, 149–155. doi:10.1016/j.jenvman.2013.04.001
Sukenik, A., and Kaplan, A. (2021). Cyanobacterial harmful algal blooms in aquatic ecosystems: a comprehensive outlook on current and emerging mitigation and control approaches. Microorganisms 9 (7), 1472. doi:10.3390/microorganisms9071472
Suresh, K., Tang, T., Van Vliet, M. T., Bierkens, M. F., Strokal, M., Sorger-Domenigg, F., et al. (2023). Recent advancement in water quality indicators for eutrophication in global freshwater lakes. Environ. Res. Lett. 18 (6), 063004. doi:10.1088/1748-9326/acd071
Tan, L., Xu, W., He, X., and Wang, J. (2019). The feasibility of Fv/Fm on judging nutrient limitation of marine algae through indoor simulation and in situ experiment. Estuar. Coast. Shelf Sci. 229, 106411. doi:10.1016/j.ecss.2019.106411
Wang, J. L., and Xu, L. J. (2012). Advanced oxidation processes for wastewater treatment: formation of hydroxyl radical and application. Crit. Rev. Environ. Sci. Technol. 42 (3), 251–325. doi:10.1080/10643389.2010.507698
Wang, K., Garg, S., and Waite, T. D. (2017). Redox transformations of iron in the presence of exudate from the cyanobacterium microcystis aeruginosa under conditions typical of natural waters. Environ. Sci. and Technol. 51 (6), 3287–3297. doi:10.1021/acs.est.7b00396
Wang, M., Wang, N., Tang, H., Cao, M., She, Y., and Zhu, L. (2012). Surface modification of nano-Fe 3 O 4 with EDTA and its use in H 2 O 2 activation for removing organic pollutants. Catal. Sci. and Technol. 2 (1), 187–194. doi:10.1039/C1CY00260K
Weenink, E. F., Luimstra, V. M., Schuurmans, J. M., Van Herk, M. J., Visser, P. M., and Matthijs, H. C. (2015). Combatting cyanobacteria with hydrogen peroxide: a laboratory study on the consequences for phytoplankton community and diversity. Front. Microbiol. 6, 714. doi:10.3389/fmicb.2015.00714
Young, L. H., Chen, Q., and Weis, M. T. (2011). Direct Measurement of hydrogen peroxide (H2O2) or Nitric Oxide (NO) release: a powerful tool to assess real-time free radical production in biological models. Am. J. Biomed. Sci. 3 (1), 40–49. doi:10.5099/aj110100040
Zeng, G., Zhang, R., Liang, D., Wang, F., Han, Y., Luo, Y., et al. (2023). Comparison of the advantages and disadvantages of algae removal technology and its development status. Water 15 (6), 1104. doi:10.3390/w15061104
Zhang, D., Pan, X., Mostofa, K. M., Chen, X., Mu, G., Wu, F., et al. (2010). Complexation between Hg (II) and biofilm extracellular polymeric substances: an application of fluorescence spectroscopy. J. Hazard. Mater. 175 (1-3), 359–365. doi:10.1016/j.jhazmat.2009.10.011
Zhang, Y., and Zhou, M. (2019). A critical review of the application of chelating agents to enable Fenton and Fenton-like reactions at high pH values. J. Hazard. Mater. 362, 436–450. doi:10.1016/j.jhazmat.2018.09.035
Zhu, Q., Wu, L., Li, X., Li, G., Li, J., Li, C., et al. (2020). Effects of ambient temperature on the redistribution efficiency of nutrients by desert cyanobacteria-Scytonema javanicum. Sci. Total Environ. 737, 139733. doi:10.1016/j.scitotenv.2020.139733
Keywords: fenton-like regent, organic iron, free radical, cyanobacterial bloom, EDTANaFe
Citation: Lin Y, Niu Z, Li J, Li S, Li H and Li L (2025) Organic iron at ultralow doses catalyzes hydrogen peroxide to eliminate cyanobacterial blooms: a study on algicidal effects and mechanisms under natural conditions. Front. Environ. Eng. 3:1516999. doi: 10.3389/fenve.2024.1516999
Received: 25 October 2024; Accepted: 09 December 2024;
Published: 06 January 2025.
Edited by:
Babak Khorsandi, Amirkabir University of Technology, IranReviewed by:
Talis Juhna, Riga Technical University, LatviaBingdang Wu, Suzhou University of Science and Technology, China
Copyright © 2025 Lin, Niu, Li, Li, Li and Li. This is an open-access article distributed under the terms of the Creative Commons Attribution License (CC BY). The use, distribution or reproduction in other forums is permitted, provided the original author(s) and the copyright owner(s) are credited and that the original publication in this journal is cited, in accordance with accepted academic practice. No use, distribution or reproduction is permitted which does not comply with these terms.
*Correspondence: Lei Li, bGlsZWlwa3VAMTI2LmNvbQ==