- 1School of Resource and Safety Engineering, Wuhan Institute of Technology, Wuhan, China
- 2Centre for Technology in Water and Wastewater, School of Civil and Environmental Engineering, University of Technology Sydney, Sydney, NSW, Australia
- 3School of Civil and Environmental Engineering, The University of New South Wales, Sydney, NSW, Australia
This review comprehensively explores the synthesis and diverse applications of the Al trimesate-based metal-organic framework, MIL-96 (Al). It begins with an introduction to the structure and properties of MIL-96 (Al), followed by an in-depth discussion of various synthesis strategies, including hydrothermal, microwave irradiation, electrochemical, mechanochemical, and sonochemical methods. The paper then delves into environmental remediation applications, highlighting MIL-96 (Al)’s effectiveness in fluoride and heavy metal removal, as well as in the elimination of volatile organic compounds (VOCs) and CO2. The review further examines the role of MIL-96 (Al) in catalysis and its emerging significance in battery technology, showcasing its versatility and potential in sustainable energy solutions. Finally, the paper concludes with perspectives on future research directions, emphasizing the ongoing development and optimization of MIL-96 (Al) for environmental and energy-related applications.
1 Introduction
As technology has advanced, the production of novel materials, including Metal-Organic Frameworks (MOFs), has escalated (Ebrahimi Farshchi et al., 2023). Developed initially in the 1990s (Liao et al., 2013), early MOF research primarily focused on experimenting with various metal salt and linker combinations. This exploration aimed to create MOFs with distinct crystalline structures, high surface areas, and large pore volumes (Qian et al., 2023). Over time, the focus expanded to include diverse synthesis routes and a broad spectrum of applications (Chen et al., 2023; Guo et al., 2023). MOFs are synthesized using several methods, with the traditional solvothermal method being one of the earliest (Liao et al., 2013; Ahmad et al., 2018; Fiaz et al., 2020). While effective, solvothermal synthesis is time-consuming, prompting researchers to investigate alternative, more efficient methods. These include electrochemical synthesis, mech microwave-assisted synthesis, mechanochemical and sonochemical approaches (Varsha and Nageswaran, 2020; Głowniak et al., 2021a; Głowniak et al., 2021b). These innovative techniques have proved advantageous in synthesizing MOFs more rapidly while maintaining or even enhancing their performance. The versatility of MOFs is evident in the extensive research into their potential applications. MOFs have been found capable of drug delivery, gas separation and storage, catalysis, sensing, and energy storage. Each application utilizes the unique properties of MOFs, such as their customizable pore structures and surface areas, to achieve specific functional goals. The continued exploration and development of MOFs and their synthesis methods promise further advancements in various fields, from medicine to environmental science and energy technology (Mahmoodi et al., 2017; Mahmoodi et al., 2018; Zhang et al., 2022).
MIL-96(Al) boasts remarkable porosity, high thermal stability, and selective absorption capabilities for various pollutants. These exceptional traits render it a robust and versatile material for the removal of a variety of pollutants, from fluoride ions in drinking water to harmful greenhouse gases in the atmosphere. Its potential in mitigating heavy metals and VOCs further underlines its comprehensive applications in the field of environmental cleanup (Qiu et al., 2015; Mehdinia et al., 2018; Wang et al., 2019; Zhou et al., 2019; Mahmoodi et al., 2020). MIL-96 (Al)’s potential in catalysis and battery technology is rooted in its unique structural and chemical characteristics. For catalysis, the high surface area of MIL-96 (Al), combined with its porous framework, offers numerous active sites for chemical reactions. This structure facilitates the absorption and interaction of reactants, thereby enhancing the efficiency and selectivity of various catalytic processes. Additionally, the stability of MIL-96 (Al) under different chemical environments makes it suitable for a wide range of catalytic applications. In battery technology, MIL-96 (Al)’s high porosity and large surface area are key assets. These properties enable better electrode material utilization and improved ion transport, which are crucial for battery performance. The structural stability of MIL-96 (Al) is also beneficial, contributing to the longevity and cycle stability of batteries. These features make MIL-96 (Al) a promising candidate for developing advanced battery materials, particularly for applications requiring high capacity and durability. However, to the best of our knowledge, there has been no exploration of reviews related to the synthesis and application of MIL-96 (Al).
The objective of this review paper is to provide a comprehensive overview of the synthesis, applications, and potential advancements of MIL-96 (Al). The review aims to critically examine various synthesis strategies, including hydrothermal, microwave irradiation, electrochemical, mechanochemical, and sonochemical methods, and their impact on the properties of MIL-96 (Al). Additionally, the paper seeks to explore the significant roles of MIL-96 (Al) in environmental remediation, catalysis, and battery technology, highlighting its versatility and potential for innovation in these fields. The ultimate goal is to offer insights into the future directions and challenges in the development and application of MIL-96 (Al), underscoring its importance in both environmental and technological domains.
2 Synthetic strategies
The synthesis of MIL-96 (Al) typically adheres to the foundational procedures, although it often undergoes modifications in various synthetic parameters, including adjustments in reaction time, temperature, pH, the incorporation of additives, and the utilization of support materials, among others (García Márquez et al., 2012; Wang et al., 2019). Among the diverse synthetic techniques available, hydrothermal methods are particularly favoured due to their inherent advantages and procedural simplicity (Fang et al., 2020; Mahmoudi et al., 2020; Zhang et al., 2022). Nevertheless, as time has progressed, several advanced synthetic methodologies to produce MIL-96 (Al) have also emerged. Each of these approaches possesses its distinct advantages and limitations. However, the development of a synthesis protocol that combines ease of execution, environmental friendliness, maintenance of crystallinity, and scalability is of paramount importance (Mahmoudi et al., 2020; Tomar and Singh, 2021; Keshta et al., 2023). Within the scope of this comprehensive review, the fabrication of MIL-96 (Al) is meticulously examined through two primary methods: the hydrothermal process and microwave synthesis. Each of these methods is presented alongside its respective morphology, as briefly outlined in Table 1.
2.1 Hydrothermal reactions
Hydrothermal synthesis is a frequently employed method to produce MIL-96 (Al) and typically occurs within a Teflon-lined autoclave, precisely controlled at a specific temperature. This approach is favoured over alternative synthetic techniques for several compelling reasons, including its feasibility, simplicity, ability to yield high crystallinity, and cost-effectiveness in comparison to other methods (Ndlwana et al., 2021; Steenhaut et al., 2021; Zheng et al., 2022). The synthesis of MIL-96 relies on two primary precursors: the aluminium source (Al3+), which acts as the metal cluster, and 1,3,5-benzenetricarboxylic acid (H3BTC), serving as the organic linker within the secondary building unit of MIL-96 (Al). When water and organic solvents are used as media, the reactions are classified into hydrothermal reactions and solvent hydrothermal reactions, respectively.
Hydrothermal synthesis of MIL-96 (Al) takes place at elevated temperature (>140°C) and pressure (>1 atm). This specific condition accelerates the growth of MIL-96 (Al) crystals from initially water-insoluble components (Nadimpalli et al., 2018). Consequently, most studies have reported synthesis temperatures ranging from 140°C to 220°C, using a Teflon-lined autoclave, with synthesis times spanning from 24 h to 48 h. The molar ratio of Al3+: H3BTC can be adjusted within a range of 1–12, allowing precise control of MIL-96 (Al)’s morphology. Furthermore, changes in the morphology of MIL-96 (Al) can also be achieved by substituting some of the Al(NO3)3 with alternative aluminium sources like red mud and aluminium isopropoxide. For instance, an increase in aluminium isopropoxide content led to a gradual decline in crystallinity and particle size of the products. As the amount of aluminium isopropoxide continued to rise, it began to influence the morphology, impacting the growth of different crystal facets (Liu et al., 2015). Moreover, the partial replacement of H3BTC with trimethyl 1,3,5-benzenetricarboxylate (Me3BTC) can also be employed to modulate crystal growth. With an increasing percentage of Me3BTC, there is a corresponding rise in the relative ratio of the (102) crystal face, coupled with a decrease in the relative ratios of the (200) and (100) planes, as compared to bulk MIL-96 (Al). The addition ratio of Me3btc in the dual ligand does not alter the fundamental structure of MIL-96 (Al) products but does influence the orientation of crystal growth in MIL-96 (Al). The underlying mechanism for controlling MIL-96 (Al) morphology using dual metal and dual organic sources lies in the competitive coordination of isopropanol, generated during the hydrolysis of aluminium isopropoxide, with BTC. This coordination inhibits the nucleation and growth process of crystals. Additionally, Me3BTC’s effect is derived from its slow hydrolysis rate, which limits the release of BTC and subsequently restrains crystal growth.
The input parameters, including reaction time, temperature, molar ratio, and solvent choice, can exert a significant influence on the structure and properties of MIL-96 (Al). These effects manifest in characteristics such as morphology, surface area, crystallinity, particle size, and pore size. It is advisable to conduct further investigations into these input parameters to systematically tailor the structure of the desired materials.
2.2 Microwave irradiation reactions
A magnetron is commonly used to convert high-voltage current into high-frequency radiation. This radiation, when interacting with substances like water (H2O) due to their dielectric properties, results in the rapid rotation of molecules and the release of heat through molecular collisions. Microwave heating has gained widespread use in various fields over the past few decades, including applications such as biomass pyrolysis (Foong et al., 2020), fuel recovery (Yek et al., 2021), biochar production (Nguyen et al., 2021), dry reforming syngas production (Pham et al., 2020), oil extraction (Taheri-Shakib and Kantzas, 2021), environmental chemistry (Xia et al., 2022), and nanomaterial synthesis (Wojnarowicz et al., 2020). Regarding the synthesis of MOFs, microwave heating offers distinct advantages, including high production yields, short reaction durations, and rapid kinetics during crystal nucleation and growth (Zornoza et al., 2011). Additionally, the use of continuous flow microwave heating allows for the separate execution of nucleation and growth steps, significantly improving reagent conversion and nucleation kinetics, all achieved under mild pressure conditions. As a result, this technique minimizes the release of residues, secondary products, or by-products into the environment, making it an environmentally friendly and efficient approach for MOF synthesis.
Application of microwave heating in the fabrication of MIL-96 (Al) has been previously documented. For instance, (Qiu et al., 2015) conducted a study in which they employed hydrothermal synthesis to produce MIL-96 (Al) using microwave irradiation. In this study, deionized water served as the environmentally friendly solvent, and microwave irradiation was carried out at a power level of 150 W. The researchers successfully obtained the final products after subjecting the synthesis to a temperature of 190°C for a duration of 30 min. Moreover, they provided evidence that the synthesis of a pure phase of MIL-96 (Al) using the conventional hydrothermal method posed challenges due to the phase conversion involving MIL-100, MIL-110, and MIL-96 (Al). MIL-100 appeared as the initial phase, and with extended reaction time, both MIL-100 and MIL-110 underwent conversion into MIL-96 (Al). This transformation arises because MIL-100 is the phase controlled by kinetics, whereas MIL-96 (Al) is controlled by thermodynamics. The synthesis process involves dynamic cyclic processes of crystallization, hydrolysis, and recrystallization. The recovery of precursor species from MIL-100, followed by successive crystallization, leads to the conversion of MIL-100 into MIL-96 (Al) (Khan et al., 2012; Lin et al., 2013). To mitigate these issues, Me3BTC was introduced as a ligand to slow down the hydrolysis of BTC3− and the crystallization process, facilitating the achievement of a pure phase of Al-BTC. Remarkably, their work enabled the synthesis of a pure phase of Al-MIL-96 within just 30 min, a stark contrast to the conventional hydrothermal method, which requires over 24 h. The resulting samples exhibited octadecahedral morphologies with dimensions of 600 nm in width and 800 nm in length, and the morphologies could be precisely controlled by altering synthesis concentrations.
While the synthesis of MIL-96 (Al) through microwave heating offers accelerated results compared to conventional hydrothermal methods, it is essential to consider certain key factors related to safety and reproducibility. For large-scale production of MIL-96 (Al), a well-equipped system, potentially requiring high-cost safety shields, may be necessary to ensure safe and efficient operations. Additionally, it is crucial to note that the morphology and purity of the synthesized MIL-96 (Al) can be significantly influenced by the input conditions, emphasizing the importance of strict control over these parameters to achieve desired results consistently.
2.3 Other methods
In addition to the aforementioned synthetic techniques, a variety of alternative methodologies, including electrochemical, mechanical, and ultrasonic approaches, are viable for the large-scale fabrication of MOFs materials. Nevertheless, the application of these techniques has been confined to other MOFs and is yet to be documented for MIL-96(Al). Prospective synthesis endeavours could explore the integration of these methods into the production process of MIL-96(Al), potentially facilitating the material’s mass production, curtailing the duration of synthesis, diminishing associated costs, and augmenting output.
2.3.1 Electrochemical methods
Electrochemistry, the study of the conversion between chemical and electrical energies, merges principles of electricity and chemistry to explore changes induced by electrical currents. For example, an electrodeposition mechanism is presented in Figure 1 with a sketch and SEM pictures. It can be divided in four phases: nucleation, growth of islands, intergrowth and detachment. At the beginning, copper(II) ions are released in solution where the linker is present; when the critical concentration of reagents is reached, nuclei form in solution and on the surface (Phase I: initial nucleation); these nuclei grow to micrometric-sized crystals on the surface next to and on top of each other (Phase II: growth of islands); new crystals keep on nucleating (progressive nucleation) and grow forming an intergrown layer (Phase III: intergrowth). Lastly, parts of the MOF layer lose contact with the substrate and are released into the solution, aided by the internal stress in the MOF layer and the undercut of the crystals (Phase IV: detachment).
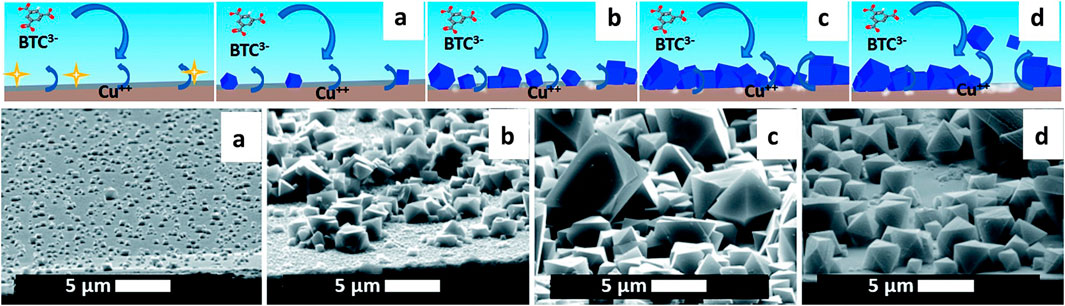
FIGURE 1. Proposed mechanism of MOF anodic electrodeposition and SEM pictures, taken at a 75° angle with the normal, of the four phases: (I) initial nucleation (A), (II) growth of islands (B), (III) intergrowth (C) (III) and detachment (D). Copper-coated wafer substrate, 2 V vs. counter electrode, after 10 s, 10 min, 60 min and 125 min.
Since this initial innovation, electrochemical synthesis of MOFs has garnered significant interest due to several benefits. It facilitates continuous synthesis, operates under milder conditions than traditional solvothermal or microwave methods, and significantly reduces reaction time—transforming processes that once took hours or days into ones that take minutes or hours. Additionally, electrochemical synthesis allows precise control over MOF formation through the manipulation of current and voltage. This method also enables the creation of uniform thin films or coatings (Li et al., 2016). Building on these advancements, the electrochemical approach holds promise for synthesizing materials like MIL-96 (Al).
2.3.2 Mechanochemical methods
Mechanosynthesis, initially a mainstay in metallurgy and mineral processing, has seen a significant expansion into various chemistry fields such as catalysis, inorganic chemistry, and pharmaceutical synthesis in recent decades (Kaupp, 2003; Pichon et al., 2006; Friščić, 2012). This synthesis method relies on initiating chemical reactions by milling or grinding solids, either without solvents or using minimal solvent quantities (Boldyrev, 1993; Stolle et al., 2011). This technique replaces conventional solvothermal MOF reactors with simpler tools like a mortar and pestle or, in more mechanized setups, automated ball mills. The mechanical milling process is not only energy-intensive but also ensures batch-to-batch reproducibility.
Mechanosynthesis typically involves grinding solid precursors in a ball miller without solvents (Neat Grinding, NG), enabling the use of insoluble metal sources—safer and more eco-friendly than conventional MOF synthesis solvents. Hydrated reactants, releasing water during the reaction, enhance NG efficiency. Liquid-Assisted Grinding (LAG) introduces small solvent amounts to improve the synthesis process, enhancing reaction rates and product crystallinity, and extending the range of materials that can be mechanochemically synthesized. Ion- and Liquid-Assisted Grinding (ILAG) combines both liquid and salt, dissolving solid reagents for a homogeneous reaction mixture and intensified substrate reactivity, enhancing efficiency, selectivity, and product quality.
(Liu et al., 2019) developed a novel synthesis strategy for creating various lanthanide (Ln)-containing MOFs. This method involves a short, 5-minute NG of lanthanide nitrate hydrates (Ln (NO3)3nH2O, where Ln can be Europium, Erbium, Dysprosium, Yttrium, or Thulium) with H3BTC, followed by a 24 h solvent-free thermal treatment at 160°C. The NG process initially produces a mix of amorphous metal complexes and crystal seeds of MIL-78. These seeds then undergo growth during the thermal heating phase, driven by strong chelating interactions. The resulting Ln-MOFs exhibit high crystallinity and a unique morphology. They form accordion-like microrods, which consist of numerous ultrathin nanosheets vertically aligned on the surface of the Ln-MOF crystals. This hierarchical structure is significant as it endows the materials with special room-temperature properties, making them suitable for use as ratiometric luminescent thermometers. The Ln-MOFs' ability to indicate temperature changes through variations in luminescence makes them valuable in the field of temperature sensing.
A key advantage of this approach is the rapid and efficient synthesis of MOFs, often achieving quantitative yields. It allows the use of MOF precursors with low solubility, such as oxides, hydroxides, and carbonates. However, scaling up mechanosynthesis poses a challenge; it remains essentially a batch processing technique with limited production rates. Additionally, while the synthesis itself may be ‘solvent-free', subsequent purification steps may require solvents. Despite these limitations, mechanosynthesis stands out as an environmentally friendly process for MOF production, potentially reducing manufacturing costs substantially. The application and underlying mechanism of mechanosynthesis for synthesizing MIL-96 (Al) remains an area needing more clarity. There is a growing recognition in the scientific community that a detailed and systematic investigation is essential to fully understand and harness this method for MIL-96 (Al) production, particularly if scaling up the process for larger-scale industrial applications is to be achieved.
2.3.3 Sonochemical methods
Sonochemistry, leveraging ultrasound power, has emerged as a rapidly evolving field in chemistry. It offers several advantages over traditional energy sources such as electrical heating or microwaves, including simplicity, reduced reaction times, and enhanced energy efficiency. Over the years, its application in organic synthesis has expanded considerably due to these benefits, enabling a wide range of organic reactions to be conducted with high yields and shorter reaction times (Vaitsis et al., 2019).
The ongoing evolution in the field of MOFs has been remarkable, with innovations in the choice of metals, custom linkers, synthesis methods, post-synthetic modifications, and specific morphological tailoring for increased application efficiency. The integration of ultrasound in MOF research has significantly contributed to this growth, particularly by enabling the synthesis of smaller particle sizes under milder conditions compared to traditional methods, which often require high temperatures and lengthy reaction times (Vaitsis et al., 2019).
An illustrative example of sonochemistry’s impact is Haque et al.'s kinetic study comparing three synthesis methods: conventional, microwave, and ultrasound (Haque et al., 2010). They used MIL-53 (Fe) for this study due to its feasibility of synthesis under mild conditions. The findings showed that conventional synthesis took 1.5–3 days at 70°C–80°C, microwave synthesis took 1.5–2.5 h at 60°C–70°C, while ultrasound synthesis only required 0.5–1 h at 50°C–70°C. These results underscore the ultrasound method’s potential in reducing reaction times and enhancing productivity.
However, the application of ultrasound methods in the synthesis of MIL-96 (Al) remains unexplored. Given the demonstrated benefits of sonochemistry in other MOF syntheses, its potential to facilitate and possibly scale up MIL-96 (Al) synthesis warrants further investigation. This exploration is essential to determine whether ultrasound can offer similar advantages in terms of reaction efficiency, time reduction, and perhaps even product quality for MIL-96 (Al) as it has for other MOFs.
3 Applications of MIL-96(Al)
3.1 Environmental remediation
MIL-96(Al) exhibits exceptional proficiency in the removal of pollutants like fluoride ions and heavy metals from aqueous solutions and gaseous pollutants. Its high surface area, adjustable pore size, and strong Lewis acid sites enable efficient fluoride binding, making it a promising candidate for mitigating excess pollutants (Jiang et al., 2021; Lawrence et al., 2022; Zou et al., 2022). Additionally, the material excels in the removal of diverse pollutants due to its tailored structure, capturing and immobilizing heavy metals effectively (Xu et al., 2021; Zhao et al., 2021; Shamim et al., 2022; Lin et al., 2023). MIL-96(Al)’s selectivity, recyclability, and sustainability further enhance its suitability as a valuable tool for addressing critical environmental and public health challenges (Mokhtari-Shourijeh et al., 2020; Chen et al., 2022a; Chen et al., 2022b; Duan et al., 2022; Chu et al., 2023).
3.1.1 Removal of fluoride
Excessive fluoride concentrations in potable water can have detrimental implications for human health. The utilization of aluminium-based materials for fluoride adsorption is gaining prominence due to their widespread availability, cost-effectiveness, and importantly, their enhanced affinity towards fluoride, as explicated by the Hard-Soft-Acid-Base theory. Consequently, the highly water-stable, structurally adaptable MOF, MIL-96 (Al), was synthesized and subsequently modified through the introduction of metal ions dopants to facilitate efficacious fluoride removal from aqueous environments. The adsorption behaviours for each variant of MIL-96 (Al) are systematically elucidated in Table 2.
Four distinct variants of MIL-96 (Al) were synthesized to facilitate the removal of fluoride from aqueous solutions. This approach was initially proposed by Zhang et al., who employed MIL-96 (Al) as an adsorbent to reduce fluoride content to less than 1.5 mg L-1. Their research validated both the feasibility and effectiveness of this approach. The adsorption process conformed closely to both the pseudo-second-order model and the Langmuir model, suggesting a predominance of chemisorption and monolayer adsorption (Mahmoodi and Mokhtari-Shourijeh, 2016; Rahimi Aqdam et al., 2021). The synthesized MIL-96 (Al) demonstrated remarkable adsorption performance across a broad pH range, and notably, exhibited substantial resistance to interference from competing ions such as chloride (Cl−), nitrate (NO3−), sulfate (SO42-), bicarbonate (HCO3−) and phosphate (PO43-). This can be largely attributed to the unique size-selective properties of the micropores of MIL-96 (Al). The maximum adsorption capacity was quantified at 31.69 mg g-1, and it was projected to retain approximately 68.63% of its original capacity after 5 cycles. The principal mechanism of adsorption is hypothesized to involve ion exchange between F and Al-OH. Yang et al. sought to introduce Ce into the interior structure of MIL-96 (Al) for improving the applicability of Ce-based adsorbents over a wider range of pH value. In their study, the synthesized Ce- MIL-96 (Al) adsorbent showed an adsorption capacity of 38.65 mg g-1 and it was supposed to maintain its original capacity of 70% after 9 cycles. It should be noted that the adsorption behavior is similar to the MIL-96 (Al) supposed by Zhang et al. The key divergence is that the adsorption process of Ce- MIL-96 (Al) toward fluoride is exothermic instead of endothermic and authors also believed the adsorption mechanism is attributable to the ion exchange of F and Ce-OH rather than Al-OH.
Contrary to the pencil-like MIL-96 (Al) powders synthesized by Zhang et al. and Yang et al., Wang et al. altered the molar ratio of the metal source to organic source from 2.75:1 to 7:1, resulting in the synthesis of rice grain-like MIL-96 (Al) nanoparticles. Remarkably, this adsorbent demonstrated superior fluoride adsorption capacity, reaching 42.19 mg g-1, significantly surpassing conventional activated aluminum materials. Moreover, the nanoparticle adsorbent exhibited a noteworthy regeneration capability, recovering 61.8% of its initial adsorption capacity following seven cycles. To address environmental concerns stemming from the accumulation of red mud, and in an effort to curb costs, Wang et al. further synthesized a MIL-96 (Al) variant using red mud as metal salts, designated as MIL-96 (Al) (RM), to supplant Al(NO3)39H2O. The morphology of MIL-96 (Al) (RM) diverged significantly from the original MIL-96 (Al), presenting a rougher appearance with several small particles adhering to its surface. This is primarily attributed to the coexistence of multiple metallic elements within the red mud, including Al, zirconium (Zr), and titanium (Ti). Each of these elements is postulated to induce distinct crystal growth behaviors, thereby influencing adsorption performance. Remarkably, MIL-96 (Al) (RM) surpassed the original MIL-96 (Al) in adsorption capacity, achieving a maximum adsorption capacity of 82.65 mg g-1 under equivalent conditions. Nonetheless, its regeneration capacity diminished somewhat, restoring only 50% of the adsorption capacity after seven cycles.
In summary, it is evident that although MIL-96 (Al) demonstrates outstanding adsorption performance in fluoride ion removal, there is still room for improvement in terms of its cyclic stability. MIL-96 (Al) exhibits commendable structural stability in acidic solutions, but during the desorption process using sodium hydroxide, it is susceptible to a certain degree of structural damage, resulting in decreased efficiency in terms of cyclic utilization. Future research endeavors should prioritize enhancing its stability, considering the varying binding affinity between different crystal facets and fluoride ions. Additionally, it is important to recognize that different crystal facets exhibit varying degrees of stability, which may manifest differently during the adsorption-desorption processes.
3.1.2 Removal of heavy metals
In addition to the removal of fluoride anions, MIL-96 (Al) also possesses promising capabilities for efficient heavy metal extraction from aqueous solutions, a potential attributable to several key advantages. Firstly, the abundant presence of weak Lewis acid sites facilitates strong coordination bonding with water molecules, hydroxyl groups, or other hard base groups present within the aqueous medium. Secondly, the propensity for proton release within the structure of MIL-96 (Al) fosters the generation of negative charges, consequently augmenting the material’s affinity for cationic ions.
Considering these inherent advantages of MIL-96 (Al), a magnetically recyclable Fe3O4/MIL-96 (Al) nanoparticle (Mehdinia et al., 2018) has been successfully synthesized and utilized for highly efficient removal of Pb(II) ions from aqueous solutions. It has been postulated that the H3BTC ligand exhibits a tendency to coordinate with Fe(III) sites on the surface of Fe3O4 nanoparticles. Following a hydrothermal reaction, MIL-96 (Al) crystals can proliferate in the vicinity of Fe3O4 nanoparticles. The results of adsorption have indicated that the Fe3O4/MIL-96 (Al) nanoparticle exhibits a considerable adsorption capacity towards Pb2+ ions, exceeding 301.5 mg g-1. This enhanced capacity is primarily attributed to the ability of positively charged hydrated Pb2+ ions to interact with the anionic structures of MIL-96 (Al) via electrostatic interactions. Furthermore, water molecules, which represent Lewis acid sites on the MIL-96 (Al) surface, can react with Pb2+ ions in accordance with the hard and soft acid base theory. The adsorption process of the adsorbate-adsorbent system can be more comprehensively elucidated through the utilization of the Temkin model, and this process is exothermic and spontaneous. This model suggests that the adsorption phenomenon primarily relies on electrostatic interactions between positively and negatively charged species, aligning with the ion exchange adsorption mechanism.
Owing to its unique capabilities, MIL-96 (Al) can serve a dual role, not only as a primary body for heavy metal removal from aqueous solutions, but also as a supporting material for the growth of functional groups due to its extensive specific surface area and exceptional water stability. In a study conducted by (Zhou et al., 2019), an attempt was made to embellish the surface of MIL-96 (Al) with α-MnO2 to catalyze a synergetic removal effect on Hg0. The MIL-96 (Al) nanoparticles with regular-shaped forms were synthesized and α-MnO2 particles dispersed and adhered to the surface of MIL-96 (Al). The resulting composite materials displayed a highly promising performance in the removal of mercury. The outcomes of the study underscore that MIL-96 (Al) can effectively amplify the availability of active sites for mercury binding, a phenomenon attributed to the augmentation of its specific surface area. The loading of MnO2 in varying quantities exhibited a negligible impact on the crystal structure of MIL-96, with MnO2 nanoparticles primarily serving the Hg0 adsorption process. The oxidation of Hg0 to HgO and the concurrent reduction of Mn4+ to Mn3+ were identified as critical factors influencing removal efficiency. Moreover, the presence of O2 and NO gases was found to promote mercury removal positively, whereas the presence of SO2 resulted in a converse effect.
3.1.3 Removal of VOCs
VOCs present a considerable hazard to human health and contribute significantly to environmental issues such as global warming, ozone layer depletion, and the formation of photochemical smog (Ramos et al., 2010; Yamamoto et al., 2010; Dou et al., 2011). MOFs, characterized by their customizability and extensive surface area, have garnered substantial interest across various applications. Specifically, the MIL-n series of MOFs, renowned for their superior hydrothermal stability and Lewis acid sites, demonstrate particular efficacy in the selective adsorption of sulfur- and nitrogen-bearing VOCs (Chen et al., 2012; Qiu et al., 2015; Sun et al., 2015).
(Qiu et al., 2015) attempted the synthesis of MIL-96 (Al) particles utilizing a microwave reactor, a method demonstrated to significantly diminish the required reaction time. The employed molar ratio of the metal source to the organic source was 12:1. It was shown that these synthesized MIL-96 (Al) particles exhibited superior adsorption capacity for pyridine. The adsorption process adhered closely to the pseudo-second-order kinetics model and Langmuir model, indicating that the process is largely governed by typical monolayer chemisorption. Additionally, thermodynamic calculations portrayed the adsorption process as spontaneous and exothermic. However, the adsorption capacity retained approximately 40% of its initial value after four cycles, primarily attributed to acid washing, which impairs the structural integrity of MIL-96 (Al). The adsorption mechanism for MIL-96 (Al) is attributed to its weak Lewis acid sites, which serve as effective adsorption sites. When a molecule, characterized by a lone pair of electrons and high polarity, comes into contact with MIL-96, it occupies the vacant sites created by the activation of Al-MIL-96’s pores. This process occurs as the weaker bonds in the material are broken due to heating.
3.1.4 Removal of carbon dioxide
The Paris Agreement mandates significant reductions in carbon emissions to limit global warming to well below 2°C above pre-industrial levels, with an aspirational target of 1.5°C. According to Article 4.1, the goal is to rapidly reduce emissions after reaching a global peak as early as possible, aiming for a balance between human-caused emissions and natural absorption by the latter half of the century, effectively reaching net-zero emissions. The Intergovernmental Panel on Climate Change (IPCC) emphasizes the need for net global CO2 emissions to drop to zero by approximately 2050 for a 1.5°C limit and by 2070 for a 2°C limit. This net-zero concept has become a practical focal point for countries, including major economies like the UK, Canada, France, China, and Japan, who have aligned their emissions reduction targets with the IPCC’s 1.5°C guideline (Iyer et al., 2021).
Achieving net-zero emissions necessitates rapid decarbonization across energy, industrial, and land sectors, coupled with extensive implementation of carbon dioxide removal (CDR) strategies (Clarke et al., 2009; Weyant and Kriegler, 2014). Discussed CDR approaches range from terrestrial methods like afforestation, reforestation, enhancing coastal blue carbon, and soil carbon enrichment through biochar, to subsurface techniques such as sequestering atmospheric CO2 in geological formations using bioenergy with carbon capture and storage (BECCS) and direct air capture (DAC) (Sohi, 2012; Wilberforce et al., 2021).
MOFs, extensively studied for their ability to capture greenhouse gases from gaseous mixtures, have emerged as a promising solution. The industrial application of adsorption techniques for the sequestration of these gases is a practical approach widely recognized for its effectiveness. Conventional porous materials, such as activated carbon, zeolites, and metal oxides, have been reliable adsorbents in curbing greenhouse gas emissions (Pellerano et al., 2009; Hernandez et al., 2015). However, recently, MOFs have captivated scientific interest, propelled by their tremendous potential across a range of industrial applications, notably including greenhouse gas storage and separation (Stock and Biswas, 2012). While MOFs do exhibit limited thermal and chemical stability when compared to their conventional counterparts, they possess larger surface areas, tunable pore sizes and volumes, and easy functionalization. These features render them attractive alternative adsorbents, ideally suited for deployment across various environmental fields. Their unique characteristics underscore the potential of MOFs to revolutionize our approach to combatting global warming and achieving broader environmental sustainability.
MIL-96 (Al) has recently received significant attention due to its remarkable chemical stability and strong CO2 adsorption capacity. In an intriguing study (Abid et al., 2020), embarked on an endeavor to synthesize pure MIL-96 (Al) and MIL-96 (Al) modified through secondary metal coordination with calcium (Ca). The authors employed a hydrothermal reaction, maintaining a molar ratio of 3.33:1 between the metal and organic sources. Calcium coordination was achieved by introducing varying quantities of CaCO3 into the reaction mixture. Their research revealed that the use of Ca2+ as a secondary metal in the single pot synthesis induced substantial morphological changes in the resultant MOF, significantly influencing the crystal anisotropy. Notably, the secondary metal, Ca2+, did not supplant the primary metal, Al3+, but instead likely coordinated with an active site of the Al3+ metal center. In terms of CO2 adsorption, the parent sample demonstrated a capacity of 8.09 mmol g-1. However, this capacity noticeably enhanced when MIL-96 (Al) was modified with Ca2+ coordination. Even a small amount of Ca2+ coordination was found to augment the BET surface area, pore volume, and micropore content of MIL-96 (Al), thereby proving advantageous for CO2 adsorption.
(Azhar et al., 2018) synthesized three variants of MIL-96 (Al), using water, methanol, and ethanol as solvents. They maintained a molar ratio of 5:1 between the metal and organic source. Interestingly, all three samples exhibited similar XRD peak characteristics, though the intensities varied, potentially due to differences in crystal size. Hexagonal crystal shapes were consistent across all samples, but crystal lengths differed. All three versions of MIL-96 (Al) showed high adsorption capacity for both CO2 and N2, albeit with less selectivity. This research highlights the role of solvent choice in influencing the characteristics and performance of synthesized MIL-96 (Al).
In addition to its adsorption capacity, the stability of MOFs under high humidity conditions serves as another crucial evaluation criterion (Qian et al., 2017). In the case of MIL-96 (Al), it demonstrates robust stability while maintaining a notable adsorption rate within a relative humidity range spanning from 3% (resulting in an adsorption of 1.6 mmol g−1) to 10% (1.1 mmol g−1) (Benoit et al., 2018). Of particular significance is the consistent adsorption performance of MIL-96 even under the latter condition. This noteworthy behavior can be attributed to the specific adsorption mechanisms involved: H2O is initially absorbed within cavities formed by H2O–Al (Trimer), terminal-OH-Al, and terminal-H2O-Al, whereas CO2 is adsorbed within a separate, independent cavity. This segregation of absorption sites ensures the stable capture of CO2 even in the presence of high humidity levels.
(Sakai et al., 2023) introduced a novel one-pot synthesis method for MIL-96 (Al) monoliths. The method involves hydrothermal treatment of an α-Al2O3 monolith in a solution containing H3BTC and HNO3, without adding a metal source. The effects of synthesis temperature and HNO3 concentration on the crystallization of MIL-96 (Al) were examined. The study found that HNO3 enhances the dissolution of α-Al2O3 and the growth of MIL-96. The crystallization required a synthesis temperature above 453 K. The synthesized MIL-96 (Al) monoliths showed comparable CO2 adsorption capacity to that of well-grown MIL-96 (Al) powdery crystals and demonstrated good stability, retaining adsorption properties even after 2 months of storage under atmospheric conditions.
(Andrés et al., 2018) details the fabrication and characterization of ultrathin films of MIL-96(Al) nanoparticles. It optimized MOF dispersions in chloroform to create dense, high-quality monolayer films without nanoparticle agglomeration. These films could be deposited on various solid substrates. The MIL-96(Al) films are of interest for CO2 capture due to their interaction with CO2 molecules, facilitated by Al3+ Lewis centers and hydroxyl groups. A comparative study using different film fabrication procedures revealed that CO2 uptake depends significantly on the method and storage conditions. Notably, the CO2 adsorption capacity of Langmuir-Blodgett (LB) films increased by 30% after a simple water immersion treatment. The study also confirmed the stability of LB MOF monolayers across multiple CO2 adsorption/desorption cycles.
MIL-96 (Al) offers several advantages for CO2 capture, making it a promising candidate for environmental applications. Its key strengths lie in its effective CO2 adsorption capability, as demonstrated in studies where MIL-96 (Al) monoliths and ultrathin films showed comparable CO2 uptake to well-grown MIL-96 (Al) powdery crystals. This attribute is crucial for efficient CO2 capture in various industrial processes. Moreover, MIL-96 (Al) exhibits remarkable stability, maintaining its adsorption properties even after prolonged storage periods, ensuring long-term usability. The versatility in its fabrication, evidenced by the development of ultrathin films, expands its applicability across different CO2 capture settings, potentially enabling integration into diverse systems and technologies. However, MIL-96 (Al) also faces some challenges. The synthesis process is intricate, requiring careful control of environmental conditions such as temperature and the concentration of nitric acid, which influence the crystallization and growth of MIL-96 (Al). This complexity can pose difficulties in scaling up production for commercial applications.
3.2 Catalysis
MIL-96 (Al) is an effective catalyst due to its distinct structural characteristics, including large surface areas, adjustable pore sizes, and a stable framework that can support and disperse various metal nanoparticles. This adaptability is exemplified in its application in different catalytic processes:
For example, Wen et al. demonstrated that bimetallic Ni-Pt nanoparticles, grown on MIL-96 using a simple liquid impregnation method, exhibit enhanced catalytic activity for hydrogen generation from hydrazine at room temperature. Ni64Pt36/MIL-96 (Al) showed the highest catalytic activity among tested catalysts, with a turnover frequency of 114.3 h-1 and 100% hydrogen selectivity. This high performance is attributed to the synergistic effect between the MIL-96 support and NiPt nanoparticles (Wen et al., 2015).
Similarly, (Lu et al., 2017) provide a detailed investigation into the enhanced catalytic capabilities of RuCo nanoparticles when supported on the MIL-96(Al) framework. They demonstrate that the Ru1Co1@MIL-96 catalyst significantly outperforms its monometallic counterparts in catalysing the hydrolysis of ammonia borane, a reaction crucial for hydrogen generation. Key findings include the uniform and effective distribution of RuCo nanoparticles on MIL-96(Al), leading to a synergistic effect between ruthenium and cobalt that greatly enhances catalytic activity. The catalyst not only shows a remarkably high turnover frequency but also maintains its structural integrity and performance over multiple cycles, highlighting its durability and potential for efficient hydrogen generation in practical applications. These findings underscore the importance of the structural and chemical properties of MIL-96(Al) in facilitating and optimizing the performance of supported metal nanoparticles in catalysis.
(Xue et al., 2018) demonstrate that MIL-96(Al), is exceptionally effective in dispersing platinum particles, leading to the creation of PtXMIL-96 (Al) catalysts with varied platinum contents. Their findings show that these catalysts, particularly Pt5MIL-96 (Al)/CP, achieve impressive NO removal efficiencies of up to 100% at 60 °C when used in a gas diffusion reactor for low-temperature H2-SCR. This study highlights the potential of MIL-96(Al) as a robust catalyst support, maintaining the structural integrity of both the platinum and the framework itself, indicating its suitability for efficient low-temperature catalytic applications.
MIL-96 (Al) stands out as a catalyst due to its unique combination of high surface area and porosity, which allows for efficient dispersion and accessibility of active catalytic sites. This structure, coupled with its thermal stability, makes it suitable for various catalytic reactions, especially those requiring the support of metal nanoparticles, enhancing both activity and selectivity. However, MIL-96 (Al) faces challenges in practical applications. Its stability might be compromised under certain chemical conditions or in high humidity environments. The complexity and potential costliness of its synthesis, particularly for tailored catalytic applications, pose additional hurdles. Furthermore, scalability and recovery of the catalyst in industrial settings remain challenging, potentially limiting its widespread adoption despite its notable advantages in laboratory-scale experiments.
3.3 Batteries
The MOFs materials with porous structure are attracting tremendous attention for function as host materials to anchor sulfur in Li-S batteries owing to their high theoretical capacity (1,675 mAh g-1) (Li et al., 2018; Baumann et al., 2019). More importantly, they have the potential to reduce the shuttle effect of lithium polysulfides (LPS, chemical formula: Li2Sx, 4≤ × ≤8) from the cathode to the anode with the aids of the Lewis acidic metal sites in MOFs, which is helpful in mitigating the irreversible loss of sulfur during the discharge process (Liang et al., 2019; Tian et al., 2019; Zhang et al., 2021; Geng et al., 2022a). It has been reported that the regulation of shape could affect the growth of planes and then change the adsorption capacity for LPS, implying that the planes with larger amounts of active sites are able to enhance the catalytic activity and cyclic stability (Wen et al., 2018; Bai et al., 2020). In this regard, Geng et al. synthesized MIL-96 with different shape and particle size and tried to reveal the relationship between shapes/sizes of an individual MOF and its cyclic performance for Li-S battery application.
The MIL-96 (Al) nanoparticles with hexagonal platelet crystals (HPC), hexagonal bipyramidal crystals (HBC), and hexagonal prismatic bipyramidal crystals (HPBC) were synthesized through adding different organic solvents including N,N-dimethylformamide (DMF) and tetrahydrofuran (THF) to affect the growth of the specific planes. The results demonstrate that the introduction of different polar regulators can dominate the growth of planes and affect the distribution of particles size, the primary exposed planes for HPC, HBC and HPBC are (002), (101), and (100), respectively. Among which, (101) plane has the largest density of Lewis acidic sites, followed by (100) and (002) planes, demonstrating that (101) plane exhibits the strongest adsorption ability. Moreover, a smaller particle size has a more superior ability to inhibit LPS diffusion because of the improved plane area.
The authors concluded that the sulfur-loading effect and the interaction with LPS demonstrate that a huge quantity of exposed (101) planes would enhance the adsorption ability and cyclic performance, especially mitigate the shuttle effect. Furthermore, particles with smaller size have a faster Li+/electron transfer rate and a higher cyclic stability.
Similarly, to inhibit the shuttle effect and mitigate the volume expansion, MIL-96 (Al) with HBC shape was synthesized and applied as the skeleton to prepare a core shell structure through coating poly pyrrole (PPy). The morphology of MIL-96 (Al) remained unchanged before and after the sulfur loading. A PPy shell with a thickness of 175 nm was then coated through an in situ oxidation polymerization process, which is beneficial for offsetting the volume expansion of the sulfur cathode during the electrochemical reaction process and the shell is able to physically confine LPS inside MIL-96 (Al)-S-PPy (Liu et al., 2019). Based on the experimental results it could be concluded that MIL-96 (Al)-S-PPy exhibited a strong chemical adsorption affinity towards S8 and Li2Sx as it can encapsulate S8 and Li2Sx into its large internal void space compared to that of MIL-96 (Al)-S. More importantly, a high specific capacity of 1233.8 mA h g-1 at 0.5 C could be realized regarding on the MIL-96 (AL)-S-PPy cathode (Wang et al., 2019).
MIL-96(Al) in lithium-sulfur batteries offers a blend of promising advantages and notable challenges. Its high porosity and surface area enhance energy density by accommodating more sulfur, while its good conductivity and chemical stability contribute to efficient and durable battery performance. Additionally, its ability to trap polysulfides addresses the common issue of capacity fading. However, the complexity and cost of its synthesis, potential for volume expansion during use, and limited scalability pose significant challenges. Furthermore, concerns about its thermal stability and the limited research on its long-term performance in lithium-sulfur batteries highlight the need for further exploration and optimization before it can be widely adopted in this application.
4 Future perspectives and conclusion
This thorough review systematically collates a range of synthesis techniques for generating MIL-96 (Al) crystals. It covers established methods such as hydrothermal and microwave reactions, and introduces emerging synthesis approaches like the electrochemical, mechanochemical, and sonochemical methods. These novel methods, already proven effective in synthesizing other MOFs, hold promise for scaling up the production of MIL-96 (Al) for industrial applications. The review emphasizes the importance of rational design in synthesis, highlighting the selection of metal and organic sources, the molar ratio, and the choice of solvent as key factors for precise control over the growth, shape, and facet development of MIL-96 (Al) crystals. Beyond synthesis strategies, the review extensively examines the applications of MIL-96 (Al) in environmental remediation, catalysis, and battery technology. MIL-96 (Al) demonstrates exceptional efficacy in removing pollutants like fluoride ions and heavy metals from water, as well as in capturing gaseous pollutants. Its application extends to catalysis and battery technologies. The material’s high surface area, tunable pore size, and robust Lewis acid sites position it as a versatile and promising candidate for a wide array of applications.
Nevertheless, the broader application of MIL-96(Al) does face certain challenges. Primarily, the process of scaling up the synthesis of this MOF to industrial levels poses substantial difficulty. Cost-effective, efficient, and reproducible large-scale synthesis is an area that requires more focused research and development. In addition, the longevity of MIL-96(Al) under different environmental conditions is yet another critical area of concern. Studies exploring its long-term stability, recyclability, and performance under diverse operational conditions are of paramount importance to ensure its reliability in sustained pollution control.
The prospects of MIL-96(Al) are, nonetheless, promising. Research endeavors should concentrate on fine-tuning synthesis methodologies for industrial scale-up and investigating novel strategies to enhance the material’s stability and recyclability. Moreover, a comprehensive assessment of its environmental impacts, stability, safety, and real-world efficiency should be undertaken. It is crucial to bridge the gap between laboratory-based research and real-world applications, underscoring the need for more in-depth, application-oriented studies that evaluate the performance of MIL-96(Al) in real settings.
Author contributions
HZ: Conceptualization, Formal Analysis, Investigation, Methodology, Writing–original draft, Writing–review and editing. QC: Investigation, Methodology, Writing–review and editing. ZC: Conceptualization, Formal Analysis, Investigation, Methodology, Writing–review and editing. B-JN: Conceptualization, Formal Analysis, Supervision, Writing–review and editing.
Funding
The author(s) declare that no financial support was received for the research, authorship, and/or publication of this article.
Conflict of interest
The authors declare that the research was conducted in the absence of any commercial or financial relationships that could be construed as a potential conflict of interest.
Publisher’s note
All claims expressed in this article are solely those of the authors and do not necessarily represent those of their affiliated organizations, or those of the publisher, the editors and the reviewers. Any product that may be evaluated in this article, or claim that may be made by its manufacturer, is not guaranteed or endorsed by the publisher.
References
Abid, H. R., Rada, Z. H., Li, Y., Mohammed, H. A., Wang, Y., Wang, S., et al. (2020). Boosting CO2 adsorption and selectivity in metal-organic frameworks of MIL-96(Al): via second metal Ca coordination. RSC Adv. 10, 8130–8139. doi:10.1039/d0ra00305k
Ahmad, N., Younus, H. A., Chughtai, A. H., Van Hecke, K., Khattak, Z. A. K., Gaoke, Z., et al. (2018). Synthesis of 2D MOF having potential for efficient dye adsorption and catalytic applications. Catal. Sci. Technol. 8, 4010–4017. doi:10.1039/C8CY00579F
Andrés, M. A., Benzaqui, M., Serre, C., Steunou, N., and Gascón, I. (2018). Fabrication of ultrathin MIL-96(Al) films and study of CO2 adsorption/desorption processes using quartz crystal microbalance. J. Colloid Interface Sci. 519, 88–96. doi:10.1016/j.jcis.2018.02.058
Azhar, M. R., Abid, H. R., Tade, M. O., Periasamy, V., Sun, H., and Wang, S. (2018). Cascade applications of robust MIL-96 metal organic frameworks in environmental remediation: proof of concept. Chem. Eng. J. 341, 262–271. doi:10.1016/j.cej.2018.02.030
Bai, X., Wang, S., Yan, X., Zhou, H., Zhan, J., Liu, S., et al. (2020). Regulation of cell uptake and cytotoxicity by nanoparticle core under the controlled shape, size, and surface chemistries. ACS Nano 14, 289–302. doi:10.1021/acsnano.9b04407
Baumann, A. E., Han, X., Butala, M. M., and Thoi, V. S. (2019). Lithium thiophosphate functionalized zirconium MOFs for Li–S batteries with enhanced rate capabilities. J. Am. Chem. Soc. 141, 17891–17899. doi:10.1021/jacs.9b09538
Benoit, V., Chanut, N., Pillai, R. S., Benzaqui, M., Beurroies, I., Devautour-Vinot, S., et al. (2018). A promising metal–organic framework (MOF), MIL-96(Al), for CO2 separation under humid conditions. J. Mat. Chem. A 6, 2081–2090. doi:10.1039/C7TA09696H
Boldyrev, V. (1993). Mechanochemistry and mechanical activation of solids. Solid State Ionics 63–65, 537–543. doi:10.1016/0167-2738(93)90157-X
Campagnol, N., Van Assche, T. R. C., Li, M., Stappers, L., Dincă, M., Denayer, J. F. M., et al. (2016). On the electrochemical deposition of metal-organic frameworks. J. Mat. Chem. A 4, 3914–3925. doi:10.1039/c5ta10782b
Chen, C., Zhang, M., Guan, Q., and Li, W. (2012). Kinetic and thermodynamic studies on the adsorption of xylenol orange onto MIL-101(Cr). Chem. Eng. J. 183, 60–67. doi:10.1016/j.cej.2011.12.021
Chen, Z., Han, N., Zheng, R., Ren, Z., Wei, W., and Ni, B.-J. (2023). Design of earth-abundant amorphous transition metal-based catalysts for electrooxidation of small molecules: advances and perspectives. SusMat 3, 290–319. doi:10.1002/sus2.131
Chen, Z., Wei, W., Chen, H., and Ni, B.-J. (2022a). Recent advances in waste-derived functional materials for wastewater remediation. Eco-Environment Health 1, 86–104. doi:10.1016/j.eehl.2022.05.001
Chen, Z., Wei, W., Ni, B. J., and Chen, H. (2022b). Plastic wastes derived carbon materials for green energy and sustainable environmental applications. Environ. Funct. Mater. 1, 34–48. doi:10.1016/j.efmat.2022.05.005
Chu, H., and Wang, C. C. (2023). MIL-100(Fe)-based functional materials for water decontamination: a state of the art review. Prog. Nat. Sci. Mater. Int. 33, 386–406. doi:10.1016/j.pnsc.2023.10.001
Clarke, L., Edmonds, J., Krey, V., Richels, R., Rose, S., and Tavoni, M. (2009). International climate policy architectures: overview of the EMF 22 international scenarios. Energy Econ. 31, S64–S81. doi:10.1016/j.eneco.2009.10.013
Dou, B., Li, J., Wang, Y., Wang, H., Ma, C., and Hao, Z. (2011). Adsorption and desorption performance of benzene over hierarchically structured carbon–silica aerogel composites. J. Hazard. Mater. 196, 194–200. doi:10.1016/j.jhazmat.2011.09.019
Duan, C., Liang, K., Zhang, Z., Li, J., Chen, T., Lv, D., et al. (2022). Recent advances in the synthesis of nanoscale hierarchically porous metal–organic frameworks. Nano Mater. Sci. 4, 351–365. doi:10.1016/j.nanoms.2021.12.003
Ebrahimi Farshchi, M., Madadian Bozorg, N., Ehsani, A., Aghdasinia, H., Chen, Z., Rostamnia, S., et al. (2023). Green valorization of PET waste into functionalized Cu-MOF tailored to catalytic reduction of 4-nitrophenol. J. Environ. Manag. 345, 118842. doi:10.1016/j.jenvman.2023.118842
Fang, Y., Yang, Z., Li, H., and Liu, X. (2020). MIL-100(Fe) and its derivatives: from synthesis to application for wastewater decontamination. Environ. Sci. Pollut. Res. 27, 4703–4724. doi:10.1007/s11356-019-07318-w
Fiaz, M., Athar, M., Rani, S., Najam-ul-Haq, M., and Farid, M. A. (2020). One pot solvothermal synthesis of Co3O4@UiO-66 and CuO@UiO-66 for improved current density towards hydrogen evolution reaction. Mater. Chem. Phys. 239, 122320. doi:10.1016/j.matchemphys.2019.122320
Foong, S. Y., Abdul Latiff, N. S., Liew, R. K., Yek, P. N. Y., and Lam, S. S. (2020). Production of biochar for potential catalytic and energy applications via microwave vacuum pyrolysis conversion of cassava stem. Mater. Sci. Energy Technol. 3, 728–733. doi:10.1016/j.mset.2020.08.002
Friščić, T. (2012). Supramolecular concepts and new techniques in mechanochemistry: cocrystals, cages, rotaxanes, open metal–organic frameworks. Chem. Soc. Rev. 41, 3493–3510. doi:10.1039/C2CS15332G
García Márquez, A., Demessence, A., Platero-Prats, A. E., Heurtaux, D., Horcajada, P., Serre, C., et al. (2012). Green microwave synthesis of MIL-100(Al, Cr, Fe) nanoparticles for thin-film elaboration. Eur. J. Inorg. Chem. 2012, 5165–5174. doi:10.1002/ejic.201200710
Geng, P., Du, M., Wu, C., Luo, T., Zhang, Y., and Pang, H. (2022b). PPy-constructed core–shell structures from MOFs for confining lithium polysulfides. Inorg. Chem. Front. 9, 2389–2394. doi:10.1039/D2QI00392A
Geng, P., Wang, L., Du, M., Bai, Y., Li, W., Liu, Y., et al. (2022a). MIL-96-Al for Li–S batteries: shape or size? Adv. Mater. 34, 2107836. doi:10.1002/adma.202107836
Głowniak, S., Szczęśniak, B., Choma, J., and Jaroniec, M. (2021b). Advances in microwave synthesis of nanoporous materials. Adv. Mater. 33, 2103477. doi:10.1002/adma.202103477
Głowniak, S., Szczęśniak, B., Choma, J., and Jaroniec, M. (2021a). Toward green synthesis of metal–organic frameworks. Mat. Today. 46, 109–124. doi:10.1016/j.mattod.2021.01.008
González-Santiago, B., García-Carrillo, A., Chávez-Guerrero, L., Poisot, M., Lemus-Santana, A. A., García-Sánchez, M. Á., et al. (2023). Enhancing the synthetic routes to prepare MIL-96(Al) and their efficiency for CO2 capture. Inorg. Chem. Commun. 155, 111095. doi:10.1016/j.inoche.2023.111095
Guo, F., Chen, Q., Liu, Z., Cheng, D., Han, N., and Chen, Z. (2023). Repurposing mining and metallurgical waste as electroactive materials for advanced energy applications: advances and perspectives. Catalysts 13, 1241. doi:10.3390/catal13091241
Haque, E., Khan, N. A., Park, J. H., and Jhung, S. H. (2010). Synthesis of a metal–organic framework material, iron terephthalate, by ultrasound, microwave, and conventional electric heating: a kinetic study. Chem. – A Eur. J. 16, 1046–1052. doi:10.1002/chem.200902382
Hernandez, M. A., Pestryakov, A., Portillo, R., Salgado, M. A., Rojas, F., Rubio, E., et al. (2015). CO2 sequestration by natural zeolite for greenhouse effect control. Procedia Chem. 15, 33–41. doi:10.1016/j.proche.2015.10.006
Iyer, G., Clarke, L., Edmonds, J., Fawcett, A., Fuhrman, J., McJeon, H., et al. (2021). The role of carbon dioxide removal in net-zero emissions pledges. Energy Clim. Change 2, 100043. doi:10.1016/j.egycc.2021.100043
Jiang, H., Cheng, H., Zang, C., Tan, J., Sun, B., and Bian, F. (2021). Photocatalytic aldehydes/alcohols/toluenes oxidative amidation over bifunctional Pd/MOFs: effect of Fe-O clusters and Lewis acid sites. J. Catal. 401, 279–287. doi:10.1016/j.jcat.2021.08.008
Kaupp, G. (2003). Solid-state molecular syntheses: complete reactions without auxiliaries based on the new solid-state mechanism. CrystEngComm 5, 117–133. doi:10.1039/B303432A
Keshta, B. E., Yu, H., and Wang, L. (2023). MIL series-based MOFs as effective adsorbents for removing hazardous organic pollutants from water. Sep. Purif. Technol. 322, 124301. doi:10.1016/j.seppur.2023.124301
Khan, N. A., Lee, J. S., Jeon, J., Jun, C.-H., and Jhung, S. H. (2012). Phase-selective synthesis and phase-conversion of porous aluminum-benzenetricarboxylates with microwave irradiation. Microporous Mesoporous Mater. 152, 235–239. doi:10.1016/j.micromeso.2011.11.025
Lawrence, A. S., Sivakumar, B., and Dhakshinamoorthy, A. (2022). Detecting Lewis acid sites in metal-organic frameworks by density functional theory. Mol. Catal. 517, 112042. doi:10.1016/j.mcat.2021.112042
Li, P., Ma, L., Wu, T., Ye, H., Zhou, J., Zhao, F., et al. (2018). Chemical immobilization and conversion of active polysulfides directly by copper current collector: a new approach to enabling stable room-temperature Li-S and Na-S batteries. Adv. Energy Mater. 8, 1800624. doi:10.1002/aenm.201800624
Li, W.-J., Tu, M., Cao, R., and Fischer, R. A. (2016). Metal–organic framework thin films: electrochemical fabrication techniques and corresponding applications & perspectives. J. Mat. Chem. A 4, 12356–12369. doi:10.1039/C6TA02118B
Liang, Z., Zhao, R., Qiu, T., Zou, R., and Xu, Q. (2019). Metal-organic framework-derived materials for electrochemical energy applications. EnergyChem 1, 100001. doi:10.1016/j.enchem.2019.100001
Liao, C., Zuo, Y., Zhang, W., Zhao, J., Tang, B., Tang, A., et al. (2013). Electrochemical performance of metal-organic framework synthesized by a solvothermal method for supercapacitors. Russ. J. Electrochem. 49, 983–986. doi:10.1134/S1023193512080113
Lin, G., Zeng, B., Li, J., Wang, Z., Wang, S., Hu, T., et al. (2023). A systematic review of metal organic frameworks materials for heavy metal removal: synthesis, applications and mechanism. Chem. Eng. J. 460, 141710. doi:10.1016/j.cej.2023.141710
Lin, Y., Kong, C., and Chen, L. (2013). Facile synthesis of aluminum-based metal–organic frameworks with different morphologies and structures through an OH−-Assisted method. Chem. Asian J. 8, 1873–1878. doi:10.1002/asia.201300135
Liu, D., Liu, Y., Dai, F., Zhao, J., Yang, K., and Liu, C. (2015). Size- and morphology-controllable synthesis of MIL-96 (Al) by hydrolysis and coordination modulation of dual aluminium source and ligand systems. Dalton Trans. 44, 16421–16429. doi:10.1039/C5DT02379C
Liu, J., Pei, L., Xia, Z., and Xu, Y. (2019a). Hierarchical accordion-like lanthanide-based metal–organic frameworks: solvent-free syntheses and ratiometric luminescence temperature-sensing properties. Cryst. Growth & Des. 19, 6586–6591. doi:10.1021/acs.cgd.9b01014
Liu, Y., Han, J., Fan, L., Li, Y., and Guo, R. (2019b). Pomegranate-like multicore–shell Mn3O4 encapsulated mesoporous N-doped carbon nanospheres with an internal void space for high-performance lithium-ion batteries. Chem. Commun. 55, 8064–8067. doi:10.1039/C9CC03727F
Loiseau, T., Lecroq, L., Volkringer, C., Marrot, J., Férey, G., Haouas, M., et al. (2006). MIL-96, a porous aluminum trimesate 3D structure constructed from a hexagonal network of 18-membered rings and μ3-oxo-centered trinuclear units. J. Am. Chem. Soc. 128, 10223–10230. doi:10.1021/ja0621086
Lu, D., Yu, G., Li, Y., Chen, M., Pan, Y., Zhou, L., et al. (2017). RuCo NPs supported on MIL-96(Al) as highly active catalysts for the hydrolysis of ammonia borane. J. Alloys Compd. 694, 662–671. doi:10.1016/j.jallcom.2016.10.055
Mahmoodi, N. M., Karimi, B., Mazarji, M., and Moghtaderi, H. (2018). Cadmium selenide quantum dot-zinc oxide composite: synthesis, characterization, dye removal ability with UV irradiation, and antibacterial activity as a safe and high-performance photocatalyst. J. Photochem. Photobiol. B Biol. 188, 19–27. doi:10.1016/j.jphotobiol.2018.08.023
Mahmoodi, N. M., Keshavarzi, S., and Ghezelbash, M. (2017). Synthesis of nanoparticle and modelling of its photocatalytic dye degradation ability from colored wastewater. J. Environ. Chem. Eng. 5, 3684–3689. doi:10.1016/j.jece.2017.07.010
Mahmoodi, N. M., and Mokhtari-Shourijeh, Z. (2016). Preparation of aminated nanoporous nanofiber by solvent casting/porogen leaching technique and dye adsorption modeling. J. Taiwan Inst. Chem. Eng. 65, 378–389. doi:10.1016/j.jtice.2016.05.042
Mahmoodi, N. M., Saffar-Dastgerdi, M. H., and Hayati, B. (2020). Environmentally friendly novel covalently immobilized enzyme bionanocomposite: from synthesis to the destruction of pollutant. Compos. Part B Eng. 184, 107666. doi:10.1016/j.compositesb.2019.107666
Mahmoudi, F., Amini, M. M., and Sillanpää, M. (2020). Hydrothermal synthesis of novel MIL-100(Fe)@SBA-15 composite material with high adsorption efficiency towards dye pollutants for wastewater remediation. J. Taiwan Inst. Chem. Eng. 116, 303–313. doi:10.1016/j.jtice.2020.11.025
Mehdinia, A., Jahedi Vaighan, D., and Jabbari, A. (2018). Cation exchange superparamagnetic Al-based metal organic framework (Fe3O4/MIL-96(Al)) for high efficient removal of Pb(II) from aqueous solutions. ACS Sustain. Chem. Eng. 6, 3176–3186. doi:10.1021/acssuschemeng.7b03301
Mokhtari-Shourijeh, Z., Langari, S., Montazerghaem, L., and Mahmoodi, N. M. (2020). Synthesis of porous aminated PAN/PVDF composite nanofibers by electrospinning: characterization and Direct Red 23 removal. J. Environ. Chem. Eng. 8, 103876. doi:10.1016/j.jece.2020.103876
Mueller, U., Schubert, M., Teich, F., Puetter, H., Schierle-Arndt, K., and Pastré, J. (2006). Metal–organic frameworks—prospective industrial applications. J. Mat. Chem. 16, 626–636. doi:10.1039/B511962F
Nadimpalli, N. K. V., Bandyopadhyaya, R., and Runkana, V. (2018). Thermodynamic analysis of hydrothermal synthesis of nanoparticles. Fluid Phase Equilibria 456, 33–45. doi:10.1016/j.fluid.2017.10.002
Ndlwana, L., Raleie, N., Dimpe, K. M., Ogutu, H. F., Oseghe, E. O., Motsa, M. M., et al. (2021). Sustainable hydrothermal and solvothermal synthesis of advanced carbon materials in multidimensional applications: a review. Mater. (Basel) 14, 5094. doi:10.3390/ma14175094
Nguyen, D. T. C., Le, H. T. N., Nguyen, T. T., Nguyen, T. T. T., Liew, R. K., Bach, L. G., et al. (2021). Engineering conversion of Asteraceae plants into biochars for exploring potential applications: a review. Sci. Total Environ. 797, 149195. doi:10.1016/j.scitotenv.2021.149195
Pellerano, M., Pré, P., Kacem, M., and Delebarre, A. (2009). CO2 capture by adsorption on activated carbons using pressure modulation. Energy Procedia 1, 647–653. doi:10.1016/j.egypro.2009.01.085
Pham, T. T. P., Ro, K. S., Chen, L., Mahajan, D., Siang, T. J., Ashik, U. P. M., et al. (2020). Microwave-assisted dry reforming of methane for syngas production: a review. Environ. Chem. Lett. 18, 1987–2019. doi:10.1007/s10311-020-01055-0
Pichon, A., Lazuen-Garay, A., and James, S. L. (2006). Solvent-free synthesis of a microporous metal–organic framework. CrystEngComm 8, 211–214. doi:10.1039/B513750K
Qian, J., Zhang, Y., Chen, Z., Yu, R., Ye, Y., Ma, R., et al. (2023). Sulfur-decorated Fe/C composite synthesized from MIL-88A(Fe) for peroxymonosulfate activation towards tetracycline degradation: multiple active sites and non-radical pathway dominated mechanism. J. Environ. Manag. 344, 118440. doi:10.1016/j.jenvman.2023.118440
Qian, X., Sun, F., Sun, J., Wu, H., Xiao, F., Wu, X., et al. (2017). Imparting surface hydrophobicity to metal–organic frameworks using a facile solution-immersion process to enhance water stability for CO2 capture. Nanoscale 9, 2003–2008. doi:10.1039/C6NR07801J
Qiu, M., Chen, C., and Li, W. (2015). Rapid controllable synthesis of Al-MIL-96 and its adsorption of nitrogenous VOCs. Catal. Today 258, 132–138. doi:10.1016/j.cattod.2015.04.017
Rahimi Aqdam, S., Otzen, D. E., Mahmoodi, N. M., and Morshedi, D. (2021). Adsorption of azo dyes by a novel bio-nanocomposite based on whey protein nanofibrils and nano-clay: equilibrium isotherm and kinetic modeling. J. Colloid Interface Sci. 602, 490–503. doi:10.1016/j.jcis.2021.05.174
Ramos, M. E., Bonelli, P. R., Cukierman, A. L., Ribeiro Carrott, M. M. L., and Carrott, P. J. M. (2010). Adsorption of volatile organic compounds onto activated carbon cloths derived from a novel regenerated cellulosic precursor. J. Hazard. Mater. 177, 175–182. doi:10.1016/j.jhazmat.2009.12.014
Sakai, M., Hori, H., Matsumoto, T., and Matsukata, M. (2023). One-pot synthesis method of MIL-96 monolith and its CO2 adsorption performance. ACS Appl. Mat. Interfaces. 15, 22395–22402. doi:10.1021/acsami.2c22955
Schäfer, P., Van Der Veen, M. A., and Domke, K. F. (2016). Unraveling a two-step oxidation mechanism in electrochemical Cu-MOF synthesis. Chem. Commun. 52, 4722–4725. doi:10.1039/c6cc00534a
Shamim, M. A., Zia, H., Zeeshan, M., Khan, M. Y., and Shahid, M. (2022). Metal organic frameworks (MOFs) as a cutting-edge tool for the selective detection and rapid removal of heavy metal ions from water: recent progress. J. Environ. Chem. Eng. 10, 106991. doi:10.1016/j.jece.2021.106991
Sohi, S. P. (2012). Carbon storage with benefits. Science 338, 1034–1035. doi:10.1126/science.1225987
Steenhaut, T., Filinchuk, Y., and Hermans, S. (2021). Aluminium-based MIL-100(Al) and MIL-101(Al) metal–organic frameworks, derivative materials and composites: synthesis, structure, properties and applications. J. Mat. Chem. A 9, 21483–21509. doi:10.1039/D1TA04444C
Stock, N., and Biswas, S. (2012). Synthesis of metal-organic frameworks (MOFs): routes to various MOF topologies, morphologies, and composites. Chem. Rev. 112, 933–969. doi:10.1021/cr200304e
Stolle, A., Szuppa, T., Leonhardt, S. E. S., and Ondruschka, B. (2011). Ball milling in organic synthesis: solutions and challenges. Chem. Soc. Rev. 40, 2317–2329. doi:10.1039/C0CS00195C
Sun, D., Ye, L., and Li, Z. (2015). Visible-light-assisted aerobic photocatalytic oxidation of amines to imines over NH2-MIL-125(Ti). Appl. Catal. B Environ. 164, 428–432. doi:10.1016/j.apcatb.2014.09.054
Taddei, M., Steitz, D. A., van Bokhoven, J. A., and Ranocchiari, M. (2016). Continuous-flow microwave synthesis of metal–organic frameworks: a highly efficient method for large-scale production. Chem. – A Eur. J. 22, 3245–3249. doi:10.1002/chem.201505139
Taheri-Shakib, J., and Kantzas, A. (2021). A comprehensive review of microwave application on the oil shale: prospects for shale oil production. Fuel 305, 121519. doi:10.1016/j.fuel.2021.121519
Tian, W., Xi, B., Feng, Z., Li, H., Feng, J., and Xiong, S. (2019). Sulfiphilic few-layered MoSe2 nanoflakes decorated rGO as a highly efficient sulfur host for lithium-sulfur batteries. Adv. Energy Mater. 9, 1901896. doi:10.1002/aenm.201901896
Tomar, S., and Singh, V. K. (2021). Review on synthesis and application of MIL-53. Mater. Today Proc. 43, 3291–3296. doi:10.1016/j.matpr.2021.02.179
Vaitsis, C., Sourkouni, G., and Argirusis, C. (2019). Metal organic frameworks (MOFs) and ultrasound: a review. Ultrason. Sonochemistry 52, 106–119. doi:10.1016/j.ultsonch.2018.11.004
Varsha, M. V., and Nageswaran, G. (2020). Review—direct electrochemical synthesis of metal organic frameworks. J. Electrochem. Soc. 167, 155527. doi:10.1149/1945-7111/abc6c6
Wang, C., Zhang, F., Yang, J., and Li, J. (2019b). Rapid and HF-free synthesis of MIL-100(Cr) via steam-assisted method. Mater. Lett. 252, 286–288. doi:10.1016/j.matlet.2019.06.003
Wang, X., Zhu, H., Sun, T., and Dai, H. (2020). Synthesis of a Matériaux Institut Lavoisier metal-organic framework 96 (MIL-96(RM)) using red mud and its application to defluorination of water. Mater. Today Commun. 25, 101401. doi:10.1016/j.mtcomm.2020.101401
Wang, X., Zhu, H., Sun, T., Liu, Y., Han, T., Lu, J., et al. (2019a). Synthesis and study of an efficient metal-organic framework adsorbent (MIL-96(Al)) for fluoride removal from water. J. Nanomater. 2019, 1–13. doi:10.1155/2019/3128179
Wen, L., Du, X., Su, J., Luo, W., Cai, P., and Cheng, G. (2015). Ni–Pt nanoparticles growing on metal organic frameworks (MIL-96) with enhanced catalytic activity for hydrogen generation from hydrazine at room temperature. Dalton Trans. 44, 6212–6218. doi:10.1039/C5DT00493D
Wen, Y., Zhang, J., Xu, Q., Wu, X.-T., and Zhu, Q.-L. (2018). Pore surface engineering of metal–organic frameworks for heterogeneous catalysis. Coord. Chem. Rev. 376, 248–276. doi:10.1016/j.ccr.2018.08.012
Weyant, J., and Kriegler, E. (2014). Preface and introduction to EMF 27. Clim. Change 123, 345–352. doi:10.1007/s10584-014-1102-7
Wilberforce, T., Olabi, A. G., Sayed, E. T., Elsaid, K., and Abdelkareem, M. A. (2021). Progress in carbon capture technologies. Sci. Total Environ. 761, 143203. doi:10.1016/j.scitotenv.2020.143203
Wojnarowicz, J., Chudoba, T., and Lojkowski, W. (2020). A review of microwave synthesis of zinc oxide nanomaterials: reactants, process parameters and morphologies. Nanomaterials 10, 1086. doi:10.3390/nano10061086
Xia, H., Li, C., Yang, G., Shi, Z., Jin, C., He, W., et al. (2022). A review of microwave-assisted advanced oxidation processes for wastewater treatment. Chemosphere 287, 131981. doi:10.1016/j.chemosphere.2021.131981
Xu, G.-R., An, Z.-H., Xu, K., Liu, Q., Das, R., and Zhao, H.-L. (2021). Metal organic framework (MOF)-based micro/nanoscaled materials for heavy metal ions removal: the cutting-edge study on designs, synthesis, and applications. Coord. Chem. Rev. 427, 213554. doi:10.1016/j.ccr.2020.213554
Xue, Y., Sun, W., Wang, Q., Cao, L., and Yang, J. (2018). Sparsely loaded Pt/MIL-96(Al) MOFs catalyst with enhanced activity for H2-SCR in a gas diffusion reactor under 80 °C. Chem. Eng. J. 335, 612–620. doi:10.1016/j.cej.2017.11.011
Yamamoto, T., Kataoka, S., and Ohmori, T. (2010). Characterization of carbon cryogel microspheres as adsorbents for VOC. J. Hazard. Mater. 177, 331–335. doi:10.1016/j.jhazmat.2009.12.036
Yang, X., Deng, S., Peng, F., and Luo, T. (2017). A new adsorbent of a Ce ion-implanted metal–organic framework (MIL-96) with high-efficiency Ce utilization for removing fluoride from water. Dalton Trans. 46, 1996–2006. doi:10.1039/C6DT03934K
Yek, P. N. Y., Chen, X., Peng, W., Liew, R. K., Cheng, C. K., Sonne, C., et al. (2021). Microwave co-torrefaction of waste oil and biomass pellets for simultaneous recovery of waste and co-firing fuel. Renew. Sustain. Energy Rev. 152, 111699. doi:10.1016/j.rser.2021.111699
Zhang, H., Hu, X., Li, T., Zhang, Y., Xu, H., Sun, Y., et al. (2022b). MIL series of metal organic frameworks (MOFs) as novel adsorbents for heavy metals in water: a review. J. Hazard. Mater. 429, 128271. doi:10.1016/j.jhazmat.2022.128271
Zhang, N., Yang, X., Yu, X., Jia, Y., Wang, J., Kong, L., et al. (2014). Al-1,3,5-benzenetricarboxylic metal–organic frameworks: a promising adsorbent for defluoridation of water with pH insensitivity and low aluminum residual. Chem. Eng. J. 252, 220–229. doi:10.1016/j.cej.2014.04.090
Zhang, W.-Y., Lu, Y., Li, Z., Wang, Y., Sun, X. W., Wang, Q., et al. (2022a). Incorporation of polyoxometalate-based acid–base pair into a sulfonated MIL-101 for achieving proton-conduction materials with high proton conductivity and high stability. Tungsten 4, 130–137. doi:10.1007/s42864-021-00123-4
Zhang, X., Li, G., Zhang, Y., Luo, D., Yu, A., Wang, X., et al. (2021). Amorphizing metal-organic framework towards multifunctional polysulfide barrier for high-performance lithium-sulfur batteries. Nano Energy 86, 106094. doi:10.1016/j.nanoen.2021.106094
Zhao, X., Yu, X., Wang, X., Lai, S., Sun, Y., and Yang, D. (2021). Recent advances in metal-organic frameworks for the removal of heavy metal oxoanions from water. Chem. Eng. J. 407, 127221. doi:10.1016/j.cej.2020.127221
Zheng, H., Hou, Y., Li, S., Ma, J., Nan, J., and Li, T. (2022). Recent advances in the application of metal organic frameworks using in advanced oxidation progresses for pollutants degradation. Chin. Chem. Lett. 33, 5013–5022. doi:10.1016/j.cclet.2022.01.048
Zhou, J., Cao, L., Wang, Q., Tariq, M., Xue, Y., Zhou, Z., et al. (2019). Enhanced Hg0 removal via α-MnO2 anchored to MIL-96(Al). Appl. Surf. Sci. 483, 252–259. doi:10.1016/j.apsusc.2019.03.261
Zornoza, B., Martinez-Joaristi, A., Serra-Crespo, P., Tellez, C., Coronas, J., Gascon, J., et al. (2011). Functionalized flexible MOFs as fillers in mixed matrix membranes for highly selective separation of CO2 from CH4 at elevated pressures. Chem. Commun. 47, 9522–9524. doi:10.1039/C1CC13431K
Keywords: metal organic framework, synthetic strategies, environmental remediation, catalysis, battery
Citation: Zheng H, Chen Q, Chen Z and Ni B-J (2024) Synthesis and application of Al trimesate-based metal-organic framework: a critical review. Front. Environ. Eng. 2:1329101. doi: 10.3389/fenve.2023.1329101
Received: 27 October 2023; Accepted: 06 December 2023;
Published: 08 January 2024.
Edited by:
Christos S. Akratos, Democritus University of Thrace, GreeceReviewed by:
Kathryn Ralphs, Queen’s University Belfast, United KingdomNiyaz Mohammad Mahmoodi, Institute for Color Science and Technology (ICST), Iran
Copyright © 2024 Zheng, Chen, Chen and Ni. This is an open-access article distributed under the terms of the Creative Commons Attribution License (CC BY). The use, distribution or reproduction in other forums is permitted, provided the original author(s) and the copyright owner(s) are credited and that the original publication in this journal is cited, in accordance with accepted academic practice. No use, distribution or reproduction is permitted which does not comply with these terms.
*Correspondence: Bing-Jie Ni, bingjieni@gmail.com