- 1Key Laboratory of Integrated Regulation and Resources Development, College of the Environment, Hohai University, Nanjing, China
- 2Anhui Academy of Ecological and Environmental Sciences, Key Laboratory of Wastewater Treatment Technology in Anhui Province, Hefei, China
The concentrations, and removal rates of five phthalate esters (PAEs) (dimethyl phthalate (DMP), diethyl phthalate (DEP), dibutyl phthalate (DBP), diisobutyl phthalate (DiBP) and di (2-ethylhexyl) phthalate (DEHP)) in the five representative sewage treatment plants of Chaohu catchment were investigated by season in this study. Our results showed that five PAEs were ubiquitous contaminants in the five sewage treatment plants. Thereinto, the contents of DiBP were significantly higher than that of the other four kinds of PAEs, and the total concentrations of PAEs in the influents in summer (17.51–33.93 μg L−1) were significantly higher than those in winter (10.14–24.08 μg L−1). Different PAEs showed different removal efficiencies in sewage treatment plants, and the orders of individual removal rates was as follows: DMP > DEP > DBP > DiBP > DEHP. The total concentration of PAEs in the effluent was relatively low in Chaohu catchment. The biochemical treatment section of the sewage treatment plant contributed the most to the removal of total PAEs, while the pretreatment and advanced treatment sections contributed less. In addition, environmental temperature had an obvious effect on the biochemical treatment process. Appropriate combined treatment processes could efficiently improve the removal effect of PAEs in sewage treatment plants.
1 Introduction
Phthalates (PAEs) are widely used as important additives in the plastics industry, and they are synthetic organic compounds (Gani and Kazmi, 2016a). PAEs have been used as plasticizers and additives for decades in the plastics production industry (Zhu et al., 2018), In addition, they have a wide range of applications and are widely used in other industries, such as cosmetics, flooring, pharmaceutical products and medical devices, among others (Tian et al., 2018). The extensive use of PAEs not only changes product performance, but also improves human life and living conditions (Gao and Wen, 2016).
However, Since PAEs as additives do not chemically bind to the substrate of materials such as plastics, they are easily released from the substrate and then into the environmental media, such as water (Latini, 2005; Reyes-Contreras et al., 2011; Paluselli et al., 2018; He et al., 2019), soil (Wang et al., 2017; Wang et al., 2022), sediment (Kang et al., 2016), air (Lu and Zhu, 2021), and so on. PAEs have estrogenic activity, which will interfere with the normal endocrine function of organisms after entering the body (Yirun et al., 2021; Barbagallo et al., 2022). Besides, PAEs can easily bind to hemoglobin molecules in human body, which may influence erythrocytic function (Tan et al., 2017). China is a major PAE consumer, accounting for more than 25% of the global PAE consumption (Lu and Zhu, 2021). PAEs were ubiquitous in water environment, and dimethyl phthalate (DMP), diethyl phthalate (DEP), dibutyl phthalate (DBP) and di-(2-ethylhexyl) phthalate (DEHP) were the most frequently detected (Ding et al., 2019). The results showed that PAEs concentrations were high in groundwater in the United States, and the highest concentrations of DEP and DBP were 147 and 50 μg L-1, respectively (Gani et al., 2017). PAEs are reproductively toxic to aquatic organisms in aquatic environments, which may also pose a potential threat to human health (Wang et al., 2015). Hence, PAEs has been regarded as one of the priority risk control pollutants in many countries. It is of great significance to study effective removal methods and reduce the discharge of PAEs into the water environment (Yang et al., 2015).
The main sources of PAEs in sewage could mainly include the industrial production of PVC products, the escape of various PVC products in daily life, the use of various detergents and disinfectants, the use of personal care products, the sedimentation of PAEs in the atmosphere and the dissolution of PAEs in municipal water supply and drainage pipes (Gani et al., 2019). The activated sludge process and improved methods are mainly used for domestic sewage treatment in China and foreign countries (Chen et al., 2020). The traditional activated sludge process has a certain removal effect on the PAEs in sewage through the adsorption and degradation of aerobic microorganisms, but the overall effect is not obvious (Balabanič and Krivograd Klemenčič, 2011; Xu, 2017).
In this work, five PAEs (DMP, DEP, DBP, DiBP, and DEHP) were investigated, in five typical sewage treatment plants with different processes in the Chaohu catchment in different seasons, and the concentration distribution of these PAEs in the influent/effluent and each section of the sewage treatment plant was grasped. The removal rules of PAEs in different processes and treatment technologies were analyzed to provide technical support for improving the removal efficiency of PAEs in the sewage of the Chaohu catchment.
2 Material and methods
2.1 Reagents and materials
DMP, DBP, DEP, DiBP, DEHP, 3,4,5,6-deuterated DBP, 3,4,5,6-deuterated DnOP and 3,4,5,6-deuterated DEP were all purchased from AccuStandard Inc., NewHaven, Connecticut, USA. 3,4,5,6-deuterated DnOP and 3,4,5,6-deuterated DEP were selected as internal standards (IS), and 3,4,5,6-deuterated DBP was selected as surrogate standard (SS). High-performance liquid chromatography (HPLC) grade methanol, dichloromethane (DCM), n-hexane (HEX), and pesticide grade acetone (ACE) were all purchased fromThermo Fisher Scientific, Waltham, Massachusetts, United States. Other reagents used in this study were all purchased from Sinopharm Chemical Reagent Co., Ltd., Shanghai. Glasswarewas cleaned in an ultrasonic cleaner (FRQ-1054ST, Hangzhou Farant Ultrasonic Technology Co., LTD., China) and then dried. C18 solid phase extraction (SPE) columns (6 mL, 500 mg) were purchased from Supelco Co., Bellefonte, Pennsylvania, United States. All chemical reagents were of analytical grade and used without further purification. All the chemical reagents used were excellent or analytical pure. Ultra-pure water was used throughout the experiment.
2.2 Sample collection and pretreatment
Five representative sewage treatment plants in the basin were selected, including Tangxihe Sewage Treatment Plant (TX), Zipeng Sewage Treatment Plant (ZP), Industrial Sewage Treatment Plant of Circular Economy Park (XH), Wangxiaoying Domestic Sewage Treatment Plant (WXY) and Beilaoxu Sewage Treatment Plant in Binhu (BH). Water samples were collected once a month with a volume of 1 L per time from July to September (summer) and October to December (winter) in 2020–2021. The detailed information was included in Table 1.
PAEs were extracted from water samples based on the method developed by He et al. (2019). The collected water samples were filtered through a 0.45 μm fibre membrane within 24 h. One liter of filtered water was infunded in a brown glass bottle for PAE analysis. 200 ng of SS was added into the prepared water. Water sample extraction and elution were performed in a fully automatic solid-phase extraction instrument (Activation: the SPE column was activated with 12 mL of a mixture of HEX and DCM(1:1), 6 mL of methanol, and 6 mL of ultrapure water. Extraction: the water sample containing SS was passed through the SPE column. Elution: the target compounds were eluted with 18 mL of a mixture of HEX and DCM (1:1) and repeated three times). The eluent was dehydrated with anhydrous sodium sulfate and concentrated to approximately 1 mL by quantitative concentrator (Buchi), and stored in Teflon lined screw-cap glass vials until analyzed.
2.3 Sample analysis
PAEs were identified and quantified by gas chromatograph mass spectrometer (GCMS TRACE1300) (Thermo Fisher, United States). DB-5MS (30 m × 0.25 mm × 0.25 μm) capillary column was used as the chromatographic column. The initial column temperature was maintained for 1 min at 60°C, rising to 220°C at 20°C/min, then to 250°C at 5°C/min for 1 min, and finally to 280°C at 200°C/min for 7 min. The sample volume was 1 µL with a splitless inlet, the injector port temperature was 280 °C, and high purity He (>99.99%) was used as carrier gas at a 1 mL/min flow rate. EI was the ion source with a temperature of 300°C and electron energy of 70 eV.
2.4 Quality assurance and quality control
For sample collection, three parallel samples with procedural blanks were processed for each quarterly sampling site, each sample was measured 3 times, and the data obtained were all mean values. The SS was added to the samples tomonitor the accuracy and precision of the pretreatment and analysis process. The method recoveries for water of the studied PAEs ranged from 73.5% to 101.3%. The instrument detection limits (IDLs) ranged from 0.1 to 0.5 n g L−1.
2.5 Removal rate calculation
The following equation was used to calculate the Removal rate of PAEs in the effluent of each section.
The ŋ values were the removal rate of PAEs, these values were expressed in %. Ci and Co values were the concentrations of these PAEs in the influent and effluent of sewage treatment plants, respectively, these values were expressed in μg·L−1.
3 Results and discussion
3.1 Concentration distribution of PAEs in the influent
DMP, DEP, DBP, DiBP and DEHP were all detected in five sewage treatment plants in the Chaohu catchment. The concentrations of these PAEs in the influent of sewage treatment plants in summer and winter are shown in Figure 1.
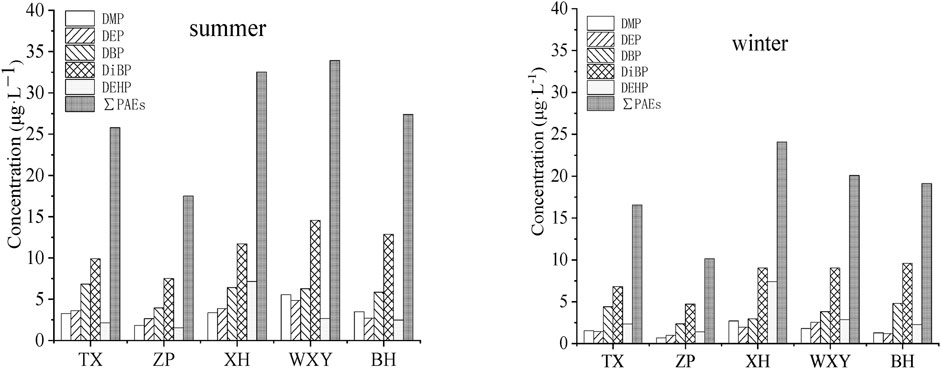
FIGURE 1. Percentage distribution of PAEs in the influent of sewage treatment plants in summer and winter (μg·L−1).
All the investigated PAEs were detected in the influent of each sewage treatment plant. The contents of DiBP were significantly higher than that of the other 4 kinds of PAEs. The DiBP concentrations ranged from 6.82 to 14.56 μg L−1, and the ranges of the other 4 kinds of PAEs were 0.67–5.56 μg L−1 (DMP), 0.98–4.85 μg L−1 (DEP), 2.36–6.84 μg L−1 (DBP) and 1.42–7.17 μg L−1 (DEHP), respectively.
Among the five wastewater treatment plants, the XH is an industrial sewage treatment plant, where DEHP concentrations in the influents (7.17–7.40 μg L−1) were significantly higher than those of the other 4 plants (1.42–2.67 μg L−1). This might be because there are multiple chemical enterprises in the water collection range of the plant, and DEHP is currently the most widely used PAEs in the production process of the chemical industry, which is used as a plasticizer in the PVC production process to improve the flexibility of materials. By comparison, it was found that DiBP showed a high concentration in both domestic sewage and industrial sewage, which was similar to the results of Wu et al. (2019) in Shanghai. This might be due to the increasing use of DiBP as a substitute for DEHP and DBP because of the increasingly strict regulation in recent years (Çifci et al., 2013). Although, at present, there are few researches on the pollution level of DiBP in sewage treatment plants in China, DIBP and DBP were the most abundant PAEs in Lake Chaohu, and DIBP should attract more attention in the future (He et al., 2019). The high concentration of DiBP in the influent indicates that DiBP might have become one of the primary PAEs pollutants in the investigated area.
Through analysis of PAEs content in the influent of all sewage treatment plants in different seasons (summer and winter), the results showed that the total concentration of PAEs in the influent in summer (17.51–33.93 μg L−1) was significantly higher than that in winter (10.14–24.08 μg L−1). Compared with DBP, DiBP and DEHP, the influent concentration of DMP and DEP decreased significantly in winter. This difference might be because the higher environmental temperature in summer, the concentrations of these PAEs in the influent of sewage treatment plants had great influence on temperature.
3.2 Concentration distribution of PAEs in the effluent of each section
The concentrations of PAEs in the effluent of each section of the five sewage treatment plants in summer and winter are presented in Figure 2.
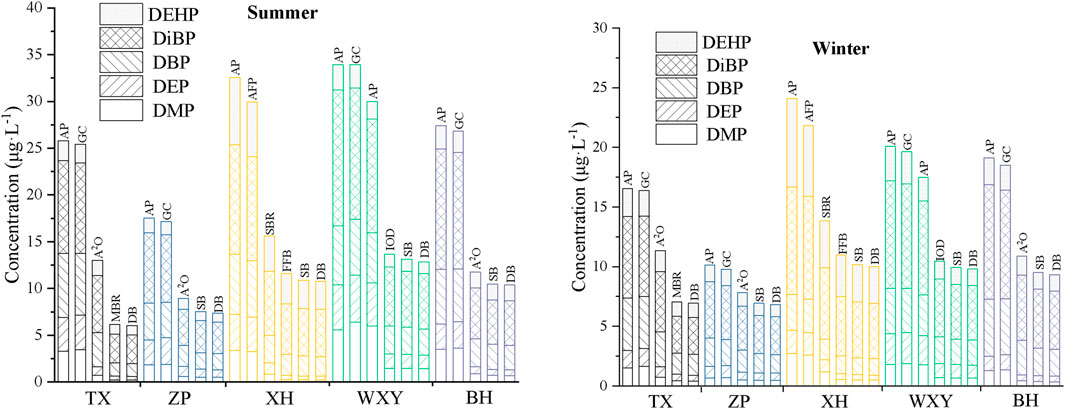
FIGURE 2. Concentration of PAEs in the effluent of each section of the sewage treatment plants in different seasons (μg·L−1) (AP: adjust the pool, GC: grit chamber, DB: drain basin, SB: settling basin, AFP: air flotation pool, FFB: Fenton fluidized bed, IOD: Improved oxidation ditch).
The concentrations of DBP, DiBP, DEHP, DMP, and DEP showed a gradual decline in the effluent of each section of the investigated treatment plants, indicating that each section had a certain removal efficiency on PAEs (Figure 2). DiBP was still present in the highest concentrations, with 3.04–5.92 μg L−1. In addition, the concentrations of DBP and DEHP were 1.38–2.79 μg L−1 and 0.96–3.10 μg L−1, respectively, while those of DMP and DEP were between 0.20–1.43 μg L−1 and 0.38–1.45 μg L−1, respectively. These values were significantly lower than the other 3 kinds of PAEs. Although the concentrations of PAEs in the effluent have met the existing discharge requirements (there are no relevant discharge standards for DiBP and DMP), which does not mean that it will not cause a hazard to the aquatic ecological environment (Akinola et al., 2021; Zhang et al., 2021).
Previous studies have shown that the total concentrations of PAEs in the effluent of sewage treatment plants in China were mainly found in the range of 1–10 μg L−1 (Xu, 2017; Wu et al., 2019). The total concentrations of PAEs in the effluents of sewage treatment plants in the Chaohu catchment are similar to that in Jinan, Xi’an, Handan and other cities (Xu, 2017), but lower than that in Qingdao, Shanghai and Harbin, showing a relatively low level (Wu et al., 2019). However, in developed countries such as the United States, France and Australia (Dargnat et al., 2009; Clara et al., 2010), the total concentration of PAEs in the effluent of sewage treatment plants does not exceed 10 μg L−1. In the investigation on five kinds of PAEs in the sewage treatment plants in Australian, the total concentration of PAEs was only 0.71 μg L−1 (Tan et al., 2017), which was significantly lower than the levels of PAEs in the effluent of sewage treatment plants in China. However, in developing countries such as India, South Africa and Nigeria (Gani and Kazmi, 2016b; Salaudeen et al., 2018), the concentrations of PAEs in the effluents mainly ranged from 10 μg L−1 to 100 μg L−1. In particular, Olujimi et al. (2017) reported the levels of seven PAEs in the sewage treatment plants of Nigeria reaching 56.10–129.08 μg L−1, which were significantly higher than those in the other countries in the world.
3.3 Removal efficiency of PAEs in the pretreatment section
The pretreatment structures mainly include grit chamber (TX, ZP, BH and WXY) and air flotation pool (XH), the removal efficiency of PAEs in the pretreatment section of each sewage treatment plant is shown in Figure 3.
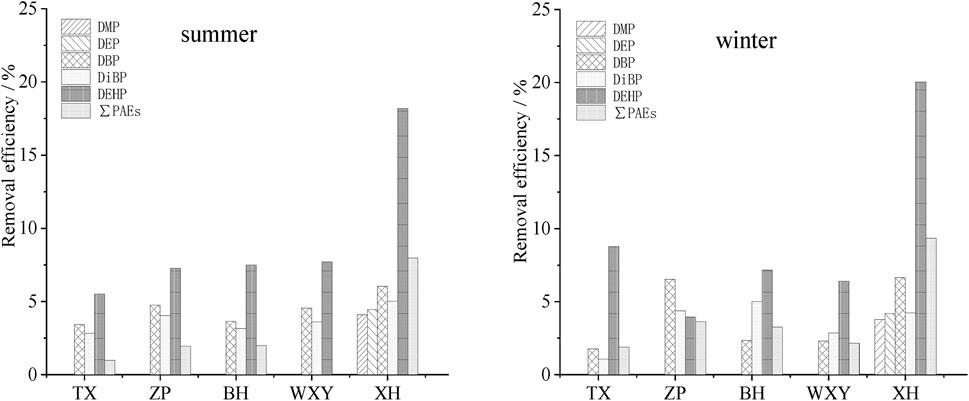
FIGURE 3. Removal efficiency of PAEs in the pretreatment section of five sewage treatment plants in summer and winter.
The removal rates of PAEs in the grit chamber of different sewage plants are different (Figure 3), while DMP and DEP were not removed in all the sewage plants except for XH. The total removal rate of PAEs was between 0.02% and 3.63%, and the removal effect was not pronounced. Even in some sewage treatment plants (TX, ZP, BH), the contents of DMP and DEP in the effluent of the grit chamber did not decrease but increased. This is mainly because DMP and DEP are characterized by low logKow (1.47 and 2.38, respectively), high water solubility (4,000 mg L−1 and 1,000 mg L−1, respectively) and short alkyl chain length (1 and 2, respectively). In the sedimentation process, DMP and DEP attached to suspended sediment were easy to fall off in the water, leading to the phenomenon that the contents of DMP and DEP increased instead of decreasing.
The air flotation section of XH could remove most suspended sediment and parts of organic matter in sewage. The removal effect of PAEs was slightly better than that of the grit chamber, especially for DEHP, the removal rates 18.09%–20.04%, were higher than those of the other PAEs.
By comparing the results of different seasons, it was found that there was little seasonal difference, which might be because the pretreatment process was mainly physical process, and the removal of PAEs in this section was less affected by water temperature.
3.4 Removal effect of PAEs in the biochemical treatment section
3.4.1 Removal effect of PAEs by A2O and its combined treatment process
The biochemical section of TX adopted an A2O+MBR combined process, and the ZP and BH adopted A2O+ settling basin process, and the PAEs removal rates in the biochemical section of the three plants shown in Figure 4.
The A2O biological reactor tank and its combined section had higher removal rates for DMP, DEP, DBP, and DiBP (Figure 4), among which the removal effect for DEP and DMP was the most significant, with the highest removal rates up to 93.2% and 89.6%, respectively (summer, TX). The removal rates were followed by DBP and DiBP, with 78.4% and 68.3%, respectively (summer, TX). The removal rate of DEHP was relatively lower, with a maximum of 48.7% (summer, TX). The efficiency of biochemical treatment in TX was the highestamong the three plants, with the removal rates of total PAE of 76.1% in summer and 57% in winter.
By comparing the results obtained in summer and winter, the A2O biological reaction tank and its combined process in the three sewage treatment plants all showed higher removal rates of PAEs in summer than in winter. This might be because the influent temperature in winter would reduce the activity of microorganisms in the reaction chamber, leading to a decrease in the population and the enzyme activity of microorganisms degrading PAEs, resulting in a lower removal rate and higher effluent concentration of PAEs in the sewage (Gao et al., 2014). In addition, no matter in what season, the removal effect of PAEs by the A2O biological reaction tank of BH was better than that of ZP, which might be because the influent concentration of PAEs in BH was higher than that of ZP, the hydraulic retention time of BH (16 h) was longer than that of ZP (13 h), and the effect of external temperature was lower due to indoor operation.
3.4.2 Removal efficiency of PAEs by anaerobic + improved oxidation ditch combined process
In the biochemical treatment section of WXY, the anaerobic + improved oxidation ditch + settling basin combined process was adopted. The PAEs removal rates of PAEs in this process is detailed in Figure 5.
The improved oxidation ditch can remove DMP, DEP, DBP and DiBP efficiently (Figure 5), especially for DEP and DMP, with the removal rates of 76.9% and 70.3%, respectively (summer). The removeal efficiencies were followed by DBP and DiBP, while the removal rate of DEHP was relatively lower, with the maximum of only 47.8% (summer). This result was roughly similar to the situation of the A2O biological reaction tank and its combined section. By comparing different seasons, it was found that the total PAEs removal rate in the improved oxidation ditch was higher in summer (60.7%) than in winter (49.5%), indicating that the process was also affected by external temperature.
3.4.3 Removal effect of PAEs by SBR+ Fenton fluidized bed combined process
In the biochemical treatment section of XH, the SBR+ Fenton fluidized bed + settling basin combined process was adopted. As shown in Figure 6, in summer, the SBR reactor and Fenton fluidized bed’s combined process had higher removal rates on DMP, DEP, DBP and DiBP, with 92.1%, 89.0%, 64.8%, and 54.3%, respectively. In winter, the removal rates were slightly decreased, reaching 80.0%, 76.5%, 49.5%, and 45.9%, respectively. The total PAEs removal rate was 63.6% in summer and 53.4% in winter, exhibiting an obvious seasonal difference.
3.4.4 Differences in biochemical treatment segments
The removal rate of total PAEs in the biochemical treatment section was noticeable. PAEs removal in the biochemical section is performed mainly in two ways, one is the degradation of PAEs by microorganisms, the other is the adsorption of activated sludge, and the biodegradability of PAEs will gradually decrease with the increase of its logKow. It is difficult to obtain a satisfactory removal effect through biodegradation for PAEs with a logKow greater than 4 (Meng et al., 2014). Therefore, DEHP with a logKow of 7.5 might be removed mainly by adsorption into activated sludge in this process (Zhao et al., 2017), and microbial degradation is relatively limited.
Dealing with the A2O treatment process adopted by sewage treatment plants in other areas in China, the removal effect of the same kind of PAEs was significantly different. For example, the removal rate of DEHP was only 37% in the study on the Harbin Sewage Treatment Plant (Gao et al., 2014). However, the removal rate of DEHP by this process was as high as 90% in the study on the Qingdao Sewage Treatment Plant (Wu et al., 2019). Xu (2017) discovered in the study of Xi ' Sewage Treatment Plant that the removal rate of DBP by this process could reach 68%–94%, consistent with the research results of this paper. Dargnat et al. (2009) found in the study of a French sewage treatment plant using the oxidation ditch process that the total removal rate of 3 kinds of PAEs (DMP, DEP and DEHP) reached 84%. Gani and Kazmi (2016a) studied 3 wastewater treatment plants in India (traditional activated sludge process, SBR process and UASB process) and concluded that the removal rate of total PAEs in the 3 sewage treatment plants was in the range of 74%–83%. While Salaudeen et al (2018). Studied 3 sewage treatment plants in South Africa (traditional activated sludge process and oxidation ditch process) and the removal rate of total PAEs reached 92%, which was similar to the results in this paper. In conclusion, there are many reasons that the same process has similar or different removal efficiency, such as the location of the sewage treatment plant, sewage composition, and sampling season. However, how these factors affect the removal efficiency remain to be further studied.
By comparing the removal rates of total PAEs by three combined processes in the biochemical section, it was found that the combined treatment process of A2O+MBR and SBR+ Fenton fluidized bed reaction system could efficiently improve the removal of PAEs by sewage treatment plants.
3.5 Removal effect of PAEs in the advanced process section
Different advanced treatment methods were adopted in the investigated sewage treatment plants. TX used contact disinfection basin, ZP used efficient settling basin + active sand filter + ultraviolet disinfection canal, XH used tertiary sedimentation tank + denitrification filter + rotating filter + disinfection basin, and WXY used denitrification filter + disinfection basin and BH used efficient settling basin + denitrification settling basin + ultraviolet disinfection canal. Water samples were all collected at the disinfection end. The removal rates of PAEs in this section are shown in Figure 7.
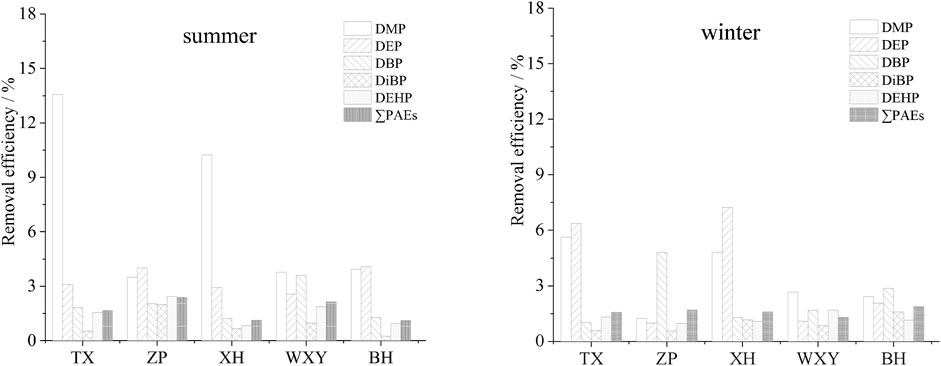
FIGURE 7. Removal efficiency of PAEs in the advanced treatment section of the sewage treatment plants.
The advanced treatment sections of TX and WXY had little effect on the removal of PAEs (Figure 7). In summer, the removal rates of total PAEs were only 1.7% and 2.1%, respectively. Although a denitrification filter was added in WXY, it failed to improve the removal efficiency. An efficient settling basin was added in the advanced treatment section in both ZP and BH. However, the removal rates of total PAEs were only 2.4% and 1.1% (both in summer). Compared with TX and WXY, the removal rates were not improved significantly. The advanced treatment section of XH was the most complex compared with other plants, but the removal rate of total PAEs was only 1.1%–1.6%, indicating that the removal of total PAEs in the advanced treatment section was negligible.
The removal rate of total PAEs in the advanced treatment section was not evident. Although many processes were used (XH), the total removal rate was not significantly improved.
4 Conclusion
The investigated five PAEs were all detected in the influent of sewage treatment plants in the Chaohu catchment, China, and DiBP was dominant PAEs. The total concentrations of PAEs in the influent in summer (17.51–33.93 μg L−1) were significantly higher than in winter (10.14–24.08 μg L−1). The order of removal rates of individual PAEs was DMP > DEP > DBP > DiBP > DEHP, and their concentrations in the effluent accord with the current relevant discharge requirements. The removal rate of total PAEs in the biochemical treatment section was noticeable, the pretreatment section and advanced treatment section contributed little, and the environmental temperature had a noticeable influence on the biochemical treatment process. The appropriate combined treatment processes (A2O+MBR and SBR+ Fenton fluidized bed reaction system) could improve the removal effect and stability of PAEs in the sewage treatment plant.
Data availability statement
The original contributions presented in the study are included in the article/supplementary material, further inquiries can be directed to the corresponding authors.
Author contributions
Conceptualization, XZ; Investigation, GL; Writing—Review and Editing, All authors contributed to the article and approved the submitted version.
Funding
This study was supported by the National Natural Science Foundation of China (Grant 52179063), and the key watershed environmental protection scientific research project of Anhui Province, China. (HKYKY05-2021).
Conflict of interest
The authors declare that the research was conducted in the absence of any commercial or financial relationships that could be construed as a potential conflict of interest.
Publisher’s note
All claims expressed in this article are solely those of the authors and do not necessarily represent those of their affiliated organizations, or those of the publisher, the editors and the reviewers. Any product that may be evaluated in this article, or claim that may be made by its manufacturer, is not guaranteed or endorsed by the publisher.
References
Balabanič, D., and Krivograd Klemenčič, A. K. (2011). Presence of phthalates, bisphenol A, and nonylphenol in paper mill wastewaters in Slovenia and efficiency of aerobic and combined aerobic-anaerobic biological wastewater treatment plants for their removal. Fresenius Environ. Bull. 20, 86–92.
Barbagallo, S., Baldauf, C., Orosco, E., and Roy, N. M. (2022). Di-butyl phthalate (DBP) induces defects during embryonic eye development in zebrafish. Ecotoxicology 31, 178–185. doi:10.1007/s10646-021-02468-5
Chen, H. G., Zhang, Z., Zhang, L. B., Tian, F., Tang, Z. Z., Cai, W. G., et al. (2020). Effects of di-n-butyl phthalate on gills-and liver-specific EROD activities and CYP1A levels in juvenile red snapper (Lutjanus argentimaculatus). Comp. Biochem. Physiology Part C Toxicol. Pharmacol. 232, 108757. doi:10.1016/j.cbpc.2020.108757
Çifci, D. İ., Kınacı, C., and Arikan, O. A. (2013). Occurrence of phthalates in sewage sludge from three wastewater treatment plants in Istanbul, Turkey, Clean–Soil. Clean. - Soil, Air, Water 41 (9), 851–855. doi:10.1002/clen.201200212
Clara, M., Windhofer, G., Hartl, W., Braun, K., Simon, M., Gans, O., et al. (2010). Occurrence of phthalates in surface runoff, untreated and treated wastewater and fate during wastewater treatment. Chemosphere 78 (9), 1078–1084. doi:10.1016/j.chemosphere.2009.12.052
Dargnat, C., Teil, M-J., Chevreuil, M., and Blanchard, M. (2009). Phthalate removal throughout wastewater treatment plant: Case study of marne aval station (France). Sci. Total Environ. 407 (4), 1235–1244. doi:10.1016/j.scitotenv.2008.10.027
Ding, M. Y., Kang, Q. Y., Zhang, S. Y., Zhao, F. R., Zhang, H. F., Yang, M., et al. (2019). National survey of phthalate metabolites in drinking source water of 23 cities in China. China Environ. Sci. 39, 4205–4211.
Gani, K. M., Bux, F., and Kazmi, A. A. (2019). Diethylhexyl phthalate removal in full scale activated sludge plants: Effect of operational parameters. Chemosphere 234, 885–892. doi:10.1016/j.chemosphere.2019.06.130
Gani, K. M., and Kazmi, A. A. (2016b). Evaluation of three full scale sewage treatment plants for occurrence and removal efficacy of priority phthalates. J. Environ. Chem. Eng. 4 (3), 2628–2636. doi:10.1016/j.jece.2016.05.006
Gani, K. M., and Kazmi, A. A. (2016a). Phthalate contamination in aquatic environment:a critical review of the process factors that influence theirremoval in conventional and advanced wastewater treatment. Crit. Rev. Environ. Sci. Technol. 46, 1402–1439. doi:10.1080/10643389.2016.1245552
Gani, K. M., Tyagi, V. K., and Kazmi, A. A . (2017). Occurrence of phthalates in aquatic environment and their removal during wastewater treatment processes: A review. Environ. Sci. Pollut. Res. 24, 17267–17284. doi:10.1007/s11356-017-9182-3
Gao, D. W., Li, Z., Wen, Z. D., and Ren, N. (2014). Occurrence and fate of phthalate esters in full-scale domestic wastewater treatment plants and their impact on receiving waters along the Songhua River in China. Chemosphere 95, 24–32. doi:10.1016/j.chemosphere.2013.08.009
Gao, D. W., and Wen, Z. D. (2016). Phthalate esters in the environment: A critical review of their occurrence, biodegradation, and removal during wastewater treatment processes. Sci. Total Environ. 541, 986–1001. doi:10.1016/j.scitotenv.2015.09.148
He, Y., Wang, Q. M., He, W., and Xu, F. L. (2019). The occurrence, composition and partitioning of phthalate esters (PAEs) in the water-suspended particulate matter (SPM) system of Lake Chaohu, China. Sci. Total Environ. 661, 285–293. doi:10.1016/j.scitotenv.2019.01.161
Kang, L., Wang, Q. M., He, Q. S., He, W., Liu, W. X., Kong, X. Z., et al. (2016). Current status and historical variations of phthalate ester (PAE) contamination in the sediments from a large Chinese lake (Lake Chaohu). Environ. Sci. Pollut.Res. 23 (11), 10393–10405. doi:10.1007/s11356-015-5173-4
Latini, G. (2005). Monitoring phthalate exposure in humans. Clin. Chim. Acta 361 (1-2), 20–29. doi:10.1016/j.cccn.2005.05.003
Lu, H., and Zhu, Z. L. (2021). Pollution characteristics, sources, and health risk of atmosphericphthalate esters in a multi-function area of Hangzhou, China. Environ. Sci. Pollut. Res. 28, 8615–8625. doi:10.1007/s11356-020-11135-x
Meng, X. Z., Wang, Y., Xiang, N., Chen, L., Liu, Z. G., Wu, B., et al. (2014). Flow of sewage sludge-borne phthalate esters (PAEs) from human release to human intake: Implication for risk assessment of sludge applied to soil. Sci. Total Environ. 476-477, 242–249. doi:10.1016/j.scitotenv.2014.01.007
Ogunwole, G. A., Saliu, J. K., and Osuala, F. I. (2021). Seasonal occurrences and risk assessment of phthalate esters in sediment, water and biota of two sub-saharan rivers. Bull. Environ. Contam. Toxicol. 106, 832–838. doi:10.1007/s00128-021-03187-7
Olujimi, O. O., Aroyeun, O. A., Akinhanmi, T. F., and Arowolo, T. A. (2017). Occurrence, removal and health risk assessment of phthalate esters in the process streams of two different wastewater treatment plants in Lagos and Ogun States, Nigeria. Environ. Monit. Assess. 189 (7), 345–416. doi:10.1007/s10661-017-6028-x
Paluselli, A., Aminot, Y., Galgani, F., Net, S., and Sempere, R. (2018). Occurrence of phthalate acid esters (PAEs) in the northwestern mediterranean sea and the rhone river. Prog. Oceanogr. 163, 221–231. doi:10.1016/j.pocean.2017.06.002
Reyes-Contreras, C., Matamoros, V., Ruiz, I., Soto, M., and Bayona, J. M. (2011). Evaluation of PPCPs removal in a combined anaerobic digester-constructed wetland pilot plant treating urban wastewater. Chemosphere 84 (9), 1200–1207. doi:10.1016/j.chemosphere.2011.06.003
Salaudeen, T., Okoh, O., Agunbiade, F., and Okoh, A. (2018). Phthalates removal efficiency in different wastewater treatment technology in the Eastern Cape, South Africa. Environ. Monit. Assess. 190 (5), 299–314. doi:10.1007/s10661-018-6665-8
Tan, S. W., Wang, D. L., Chi, Z. X., Li, W. G., and Shan, Y. (2017). Study on the interaction between typical phthalic acid esters (PAEs) and human haemoglobin (hHb) by molecular docking. Environ. Toxicol. Pharmacol. 53, 206–211. doi:10.1016/j.etap.2017.06.008
Tian, M. P., Liu, L. P., Wang, H., Wang, X. F., Martin, F. L., Zhang, J., et al. (2018). Phthalates induce androgenic effects at exposure levels that can Be environmentally relevant in humans. Environ. Sci. Technol. Lett. 5, 232–236. doi:10.1021/acs.estlett.8b00138
Wang, B., Wang, H. X., Zhou, W., Chen, Y., Zhou, Y., and Jiang, Q. W. (2015). Urinary excretion of phthalate metabolites in school children of China: Implication for cumulative risk assessment of phthalate exposure. Environ. Sci. Technol. 49, 1120–1129. doi:10.1021/es504455a
Wang, F., Wang, Y., Xiang, L. L., Redmile-Gordon, M., Gu, C. G., Yang, X. L., et al. (2022). Perspectives on ecological risks of microplastics and phthalate acid esters in crop production systems. Soil Ecol. Lett. 4 (2), 97–108. doi:10.1007/s42832-021-0092-4
Wang, H., Liang, H., and Gao, D. W. (2017). Occurrence and risk assessment of phthalate esters (PAEs) in agricultural soils of the Sanjiang plain, Northeast China. Environ. Sci. Pollut.Res. 24 (24), 19723–19732. doi:10.1007/s11356-017-9646-5
Wu, J., Ma, T. J., Zhou, Z. F., Yu, N., He, Z., Li, B., et al. (2019). Occurrence and fate of phthalate esters in wastewater treatment plants in Qingdao, China. Hum. Ecol. Risk Assess. Int. J. 25 (6), 1547–1563. doi:10.1080/10807039.2018.1471341
Xu, L. Y. (2017). “Distribution and removal pathway of phthalic acid esters in urban sewage treatment plant,” MSc Thesis. (Xi 'an, China: Chang'an University).
Yang, J. L., Li, Y. X., Wang, Y., Ruan, J., Zhang, J., and Sun, C. J. (2015). Recent advances in analysis of phthalate esters in foods. TrAC Trends Anal. Chem. 72, 10–26. doi:10.1016/j.trac.2015.03.018
Yirun, A., Ozkemahli, G., Balci, A., Erkekoglu, P., ZeybekYersal, N. D. N., and Kocer-Gumusel, B. (2021). Neuroendocrine disruption by bisphenol A and/or di(2-ethylhexyl) phthalate after prenatal, early postnatal and lactational exposure. Environ. Sci. Pollut. Res. 28, 26961–26974. doi:10.1007/s11356-021-12408-9
Zhang, W. P., Li, X., Guo, C. S., and Xu, J. (2021). Spatial distribution, historical trend, and ecological risk assessment of phthalate esters in sediment from Taihu Lake, China. Environ. Sci. Pollut. Res. 28, 25207–25217. doi:10.1007/s11356-021-12421-y
Zhao, H., Wang, Q., Chen, Y., Tian, Q., and Zhao, G. (2017). Efficient removal of dimethyl phthalate with activated iron-doped carbon aerogel through an integrated adsorption and electro-Fenton oxidation process. Carbon 124, 111–122. doi:10.1016/j.carbon.2017.08.034
Keywords: phthalate esters (PAE), sewage treatment plant, removal rate, occurrence levels, chaohu catchment
Citation: Zheng X, Lu G, Liu J and Jiang R (2023) Phthalate esters in municipal sewage treatment plants: occurrence level, removal rate and optimum combination technology. Front. Environ. Eng. 2:1208689. doi: 10.3389/fenve.2023.1208689
Received: 24 April 2023; Accepted: 27 June 2023;
Published: 11 July 2023.
Edited by:
Claudia-Maria Simonescu, Politehnica University of Bucharest, RomaniaReviewed by:
Lijun Wang, Shaanxi Normal University, ChinaLei Zhou, East China University of Science and Technology, China
Mirjana Vojinović Miloradov, University of Novi Sad Faculty of Technical Sciences, Serbia
Copyright © 2023 Zheng, Lu, Liu and Jiang. This is an open-access article distributed under the terms of the Creative Commons Attribution License (CC BY). The use, distribution or reproduction in other forums is permitted, provided the original author(s) and the copyright owner(s) are credited and that the original publication in this journal is cited, in accordance with accepted academic practice. No use, distribution or reproduction is permitted which does not comply with these terms.
*Correspondence: Xiqiang Zheng, zxqlq0402@163.com; Guanghua Lu, ghlu@hhu.edu.cn