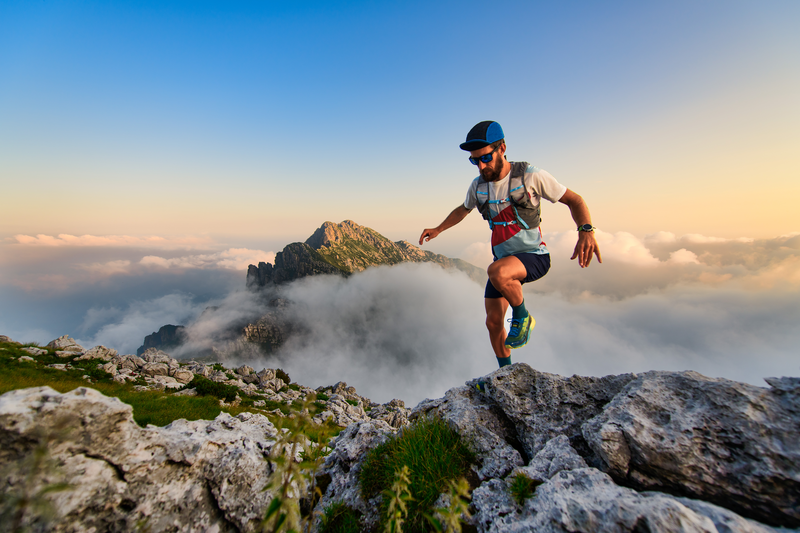
94% of researchers rate our articles as excellent or good
Learn more about the work of our research integrity team to safeguard the quality of each article we publish.
Find out more
ORIGINAL RESEARCH article
Front. Environ. Econ. , 17 March 2023
Sec. Ecological Economics
Volume 2 - 2023 | https://doi.org/10.3389/frevc.2023.1073089
This article is part of the Research Topic Insights in Ecological Economics: 2022 View all 5 articles
Since the early 1990s, the coastal Semarang City in Indonesia has been undergoing rapid industrialization and population expansion. To meet growing water demand, groundwater is abstracted with an ever-increasing number of abstraction wells. This has led to lower groundwater tables in the largely unconsolidated substrate and this, in turn, causes land subsidence in the area. This has led to significant direct and indirect economic damage. In the context of limited public resources, this study aims to analyze the economic rationale of alternative (public) investment strategies to reduce subsidence impact in the Semarang-Demak region. With Cost-Benefit Analysis (CBA), three alternative strategies to replace groundwater abstraction wells are analyzed: (1) Installment of a piped clean water supply system with 1,000 L/s capacity, (2) a new 2 km2 coastal freshwater reservoir in West Semarang, (3) installment of a piped clean water supply system with 2,000 L/s capacity. All strategies have a positive (>1) benefit-cost ratio, which indicates that there is an economic rationale for investment to mitigate subsidence in Semarang and Demak. Under a low water demand scenario, the best strategy is strategy 1; under a high water demand scenario, the best strategy is strategy 3. As strategy 3 gives the highest economic benefit in the worst-case scenario, this is the most robust strategy.
Land subsidence is defined as the gradual settling or sudden sinking of the Earth's surface (Galloway et al., 2000). It may be caused by a range of natural or anthropogenic triggers but is often related to the removal of underground solid or fluid resources like gas or water. This potentially destructive hazard often occurs over large areas and can have very significant direct and indirect economic impacts. The reduction in elevation typically increases susceptibility to flooding or even permanent loss of land, and the process often leads to damage to buildings and infrastructure and changes in natural features of the landscape. Although global damage reports are lacking, estimates amount to billions of dollars annually around the globe (Kok and Costa, 2021). According to Herrera-García et al. (2021), 1.2 billion people, 19% of the global population, live in an area with potential subsidence. The economic assets exposed to subsidence make up 12% of the global gross domestic product (US$8.17 trillion). As much as 86% of the population exposed to subsidence lives in Asia, particularly China (421 million), Japan (81 million), India (41 million), Indonesia (39 million), and Bangladesh (18 million). Potential subsidence areas are concentrated in and near densely urbanized and irrigated areas with high water stress and high groundwater demand, often overlying large depleted aquifer systems (Herrera-García et al., 2021).
Although the subsidence hazard itself has been increasingly studied by the scientific community in the past decades (UNESCO, 2020), there is still a knowledge gap in decision support on dealing with subsidence. Such studies can be very valuable in supporting policymakers to deal with the problem: as anthropogenic drivers and impacts of subsidence are often very localized; subsidence is a relatively manageable environmental problem. In this context, cost-benefit analysis (CBA) is a well-established economic tool used to support the evaluation of different strategies and support the rationale for investment or changes in policy in a variety of fields, including natural hazard management (Gamper et al., 2006). Yet, despite the potential added value of CBA in mitigation strategies of subsidence, to date, the number of studies that address this topic is very limited (Kok and Costa, 2021). In the previous decade, there have been some publications related to the economic impact of (mitigating) subsidence: particularly in the Netherlands in Europe, (Born et al., 2016; Kok and Hommes-Slag, 2020; Willemsen et al., 2020) and China and Thailand in Asia (Shin et al., 1997; Qi-yan et al., 2008; Lixin et al., 2010; Liu et al., 2012; Hu et al., 2013; Zeitoun and Wakshal, 2013).
In Indonesia, there has been no substantial analysis of the economic consequences and mitigation strategies to date, although subsidence in the island of Java is known to occur in several big cities, including Jakarta, Semarang, and Bandung (Abidin et al., 2013; Chaussard et al., 2013; Kahn et al., 2019). Moreover, according to the global land subsidence map by Herrera-García et al. (2021), several cities on the island of Sumatra, Kalimantan, and Papua are also at risk of subsidence. This study focuses on the Semarang-Demak area: an exploratory analysis by Mahya et al. (2021) estimates the extent of the damage related to subsidence in Semarang and Demak at respectively at least 79 trillion IDR present value (circa US$ 5.5 billion) and 39 trillion IDR present value (circa US$ 2.7 billion) until 2040, that was based on the total cost of loss of land, increase risk of coastal flood, increase cost of road maintenance, and building damage cost. This study aims to address the knowledge gap around a cost-benefit analysis of subsidence mitigation strategies in general, and specifically in Indonesia by analyzing the costs and benefits of various mitigation strategies for subsidence in the case of Semarang-Demak, Indonesia. The cost of subsidence was analyzed based on the analysis by Mahya et al. (2021) and in addition, the loss of coastal ecosystem was also calculated to better capture the total cost of subsidence. In this study, the study site is introduced (section 2) and the methodology used to assess the costs and benefits of distinct mitigation strategies is described (section 3). Then the results are presented (section 4) and it is closed with a discussion (section 5) and conclusion (section 6).
The study area includes the Semarang city (population in 2019 1.8 million) and nearby Demak (population in 2019 is 1.1 million), located on the northern coast of Central Java. The area has been experiencing land subsidence since the 1980s (Marfai and King, 2007). According to Putranto and Rüde (2016), the overexploitation of groundwater is the main cause of the decrease in the groundwater head in the lowland area. The subsurface of Demak and the northern part of Semarang consists of a thick layer of Alluvium deposits consisting of unconsolidated clays, surrounded by Quaternary volcanic rocks and Tertiary sedimentary rocks (Thanden et al., 1996). The areas with (thick) unconsolidated clays are the most susceptible to compaction and subsidence (Chaussard et al., 2013). Subsidence develops mostly in the industrial area in Semarang, correlated to industrial groundwater abstraction. According to Mahya et al. (2021), based on Ellipsis data for Semarang Central Bureau of Statistics (2020c) and DSInSAR data from Yuwono et al. (2019) for Demak, the land subsidence rates in the area vary between 0–2 to >10 cm/year (Figure 1). Ellipsis subsidence rate was derived from Sentinel-1A bi-monthly observation data that was taken from April 2016 to October 2019 and processed with inSAR (Interferometric synthetic aperture radar) technique (Ellipsis, 2020). Demak subsidence data was derived using DinSAR (Interferometric synthetic aperture radar) techniques and GNSS (Global Navigation Satellite System) technology to predict the rate of land subsidence in the coastal area of Demak (Yuwono et al., 2019).
Figure 1. Subsidence rate in the study area based on observed data. The study area is depicted with solid black line. The area with no subsidence rate information is assumed to be not impacted by subsidence and is not considered in CBA analysis. Source: Mahya et al. (2021) modified from Ellipsis (2020) and Yuwono et al. (2019).
The study site is located in Semarang Demak groundwater basin with 16.5 million m3/year deep groundwater potential from the confined aquifer (Development Planning Agency Semarang, 2013). Groundwater is intensively used as it is relatively simple, constant, and easily accessible. According to Beek et al. (2019) groundwater only requires a few treatment steps and does not need a distribution network, while other water sources such as surface water requires big water storage, and seawater is associated with high cost, as well as ecological threat due to its brine discharge. Moreover, although since 2018, there have been efforts to increase clean water provision in Semarang, including the construction of reservoirs in Manyaran and Kampung Ndesel Gunungpati, still, due to the low capacity for clean piped water supply, groundwater abstraction has become common practice in this region, especially in the industrial sector (Valentino, 2013).
Since 1995, Semarang's population has grown from 1.23 million to more than 1.8 million in 2019 (Semarang Central Bureau of Statistics, 2020a); since 2010, the industry has grown from 313 companies in 2010 to 446 in 2019 (Semarang Central Bureau of Statistics, 2020b). This trend of population growth and industrialization is expected to continue: the drinking water company providing clean piped water, PDAM (Perusahaan Daerah Air Minum; local drinking water company) does not have the capacity to cover the expected increase in water demand. In 2017, the capacity of clean piped water supply was 29.6% of Demak and 65.2% of Semarang water demand (Agency for Improvement of Drinking Water Supply System, 2018). With the expected further increase in water demand in the (near) future, the rate of groundwater abstraction and consequent subsidence is expected to increase as well.
In this study, the economic feasibility of alternative strategies to deal with the land subsidence issue in Semarang and Demak is assessed, using a cost benefit analysis following the framework described by Romijn and Renes (2013): the methodology is illustrated in Figure 2. It is begun with an analysis of water demand considering different population and economic development scenarios in Semarang and Demak (step 1) and calculate the corresponding expected deep groundwater abstraction for each scenario (step 2) (section 3.1). Then, the potential alternative strategies are described in section 3.2. Next, the physical effects of alternative strategies are estimated, by assessing their impacts on groundwater abstraction and corresponding subsidence rate in the area (steps 3). In section 3.3 (step 4), the corresponding economic effects are assessed, focusing on loss of land, increased coastal flood risk, damage to roads and buildings, and decreased coastal tourism. After reviewing investment costs for each alternative strategy (step 5), the economic loss and investment costs are analyzed in a cost-benefit analysis (step 6) and then the robustness of results is reviewed in a sensitivity analysis related to the discount rate and the correlation between groundwater abstraction and subsidence rate (step 7; section 3.4).
To assess the extent to which alternative strategies can reduce groundwater abstraction and corresponding subsidence rates, the expected water demand and groundwater abstraction in the study area were first analyzed. Although the impacts of subsidence occur in both Semarang and Demak, the main cause of subsidence in this region is attributed to overexploitation of groundwater in Semarang, largely due to industrial deep groundwater abstraction (Popang and Tirta, 2020)—groundwater abstraction for domestic use mainly comes from shallow groundwater abstraction which is not expected to have a significant influence on subsidence (Letitre and Kooi, 2018). Therefore, the analysis is limited to industrial water demand in Semarang. Two scenarios were developed: low and high water demand.
In scenario 1, industrial water demand in Semarang is assumed to be correlated with domestic water demand, which in turn, is related to population growth. To predict population growth in the study area, the population data from the Semarang Central Bureau of Statistics (2020a) and Indonesian population growth rate projections from the Indonesia National Population Family Planning Agency (2015) were used. The domestic water demand was estimated by multiplying the total population and average water use per capita in Semarang city, 142 liter/day/ person (Development Planning Agency Semarang, 2013). To derive expected industrial water demand, a distribution ratio of 17:83 for industry and domestic water demand (Development Planning Agency Semarang, 2013) was used. Using this approach, the population is estimated to grow from 1.87 million in 2022 and reach its peak to 2.18 million in 2062 and then decline to 1.92 million in 2122 with total water demand of 116.6, 136.5, and 120.2 million m3/year for 2022, 2062, and 2122 respectively. The total groundwater abstraction under Business as Usual (BaU) in this scenario from 2022 to 2122 is 830,210,606 m3.
In scenario 2, 5% industrial growth is applied. This is based on the average economic growth in Indonesia (Indonesia Business Council for Sustainable Development, 2015). This industrial growth is until 2062 when Indonesia's population is set to reach its peak (Indonesia National Population Family Planning Agency, 2015). After this period, it is assumed that industrial water demand will remain constant. Expected deep groundwater abstraction is calculated by multiplying the number of industries that use deep groundwater, by their water use. At present (2019) there are 243 medium and 203 big industries in Semarang (Semarang Central Bureau of Statistics, 2020b). Medium industries are assumed to use 49,500 liter/unit/day, while big industries are assumed to use 99,500 liter/unit/day (Santikayasa et al., 2017). Using this approach, total water demand is expected to be 110.4, 209.1, and 195.7 million m3/year for 2022, 2062, and 2122 respectively. The total groundwater abstraction under BaU in this scenario from 2022 to 2122 is 6,088,233,556 m3.
There are two developments that are expected to provide 13.9 million m3/year of piped water for industries in Semarang since 2022, in an effort to reduce groundwater abstraction: (1) construction of a clean water supply system in West Semarang and (2) construction of a 2.5 km2 freshwater reservoir near the coast in East Semarang. The clean water supply system in West Semarang will have a capacity of 1,000 L/s, corresponding to 31.6 million m3/year (Development Planning Agency Semarang, 2013; Ministry of Public Work Indonesia, 2019) of which 6.3 million m3/year will be used for industrial use (Salam, 2018). The coastal reservoir in East Semarang can produce approximately 7.6 million m3/year of water that is allocated for industrial use (One Architecture and Urbanism et al., 2019).
For the purpose of this study, it is assumed that the amount of abstracted groundwater is equal to industrial water demand (estimated in scenario 1, and 2) minus the amount of clean water supplied by the West Semarang piped water system and the East Semarang coastal reservoir. The registered historical groundwater abstraction rate is not used to project the groundwater abstraction rate, as this data does not take into account the unregistered abstraction that is still common in the study area (Beek et al., 2019).
The effects of alternative strategies will be compared to development under BaU to analyze their impact. BaU includes the developments of the West Semarang clean water supply system and East Semarang freshwater reservoir along the coast. Three alternative strategies to reduce groundwater abstraction and consequently reduce land subsidence were analyzed: all strategies follow the rationale of increasing the capacity of clean water supply by means other than groundwater abstraction. Table 1 summarizes the alternative strategies.
Jatibarang dam is located in Semarang city with a total storage capacity of 20.4 million m3 (Ministry of Public Work Indonesia, 2012). The water from this dam can be used to provide additional clean water, but this would require the construction of a water supply distribution system. In strategy 1, a new clean water distribution system consisting of an intake facility, water treatment plant, and water distribution system is developed, with 1,000 L/s (31.5 million m3/year) capacity to distribute the clean water from the Jatibarang dam. 70% of the distributed water is assumed to be distributed for domestic use and 30% for industrial use.
Another strategy proposed in this study is building a new coastal reservoir in the industrial area to specifically meet industrial water demand in West Semarang. Required investments include the construction of a 2 km2 water reservoir, water treatment facility, retention pond, and water distribution network. 100% of the water produced would be for industrial use. MLA+ (2019) describe the characteristics and potential of such a reservoir: the authors assume that water produced in the coastal reservoir is directly proportional to its reservoir size (km2) and will be able to produce 6.08 million m3/year.
Similar to strategy 1, strategy 3 includes the construction of a new clean water supply system to distribute the surface water from the Jatibarang dam, but with a larger capacity: a capacity of 2,000 L/s or 63 million m3/year. 60% of the produced water is assumed to be distributed for domestic use and 40% of the water is assumed to be distributed for industrial use.
After calculating the expected groundwater extraction rate for each strategy and scenario, the expected corresponding subsidence rate is assessed. To do so, the following correlations between groundwater abstraction, groundwater drop, and land subsidence based on Suripin (2005) is used:
Where S is subsidence and Su is groundwater drop. By calculating S in Eq 1 with a range of values for Su (Su takes a value from 1 to 20, representing groundwater drop by 1 to 20 meters), the following relationship between S and ΔSu is derived:
By calculating the difference of S with Eq 2 with a range of values of Su (1 to 20), an equation for ΔS as a function of ΔSu is derived:
By calculating ΔS and ΔSu with Eq 3, an equation between ΔS in percent and ΔSu in percent is derived:
As groundwater drop is directly proportional to groundwater abstraction (Abidin et al., 2010; Chen et al., 2010, 2016; Gong et al., 2018), it is assumed that the correlation between groundwater abstraction and land subsidence will follow Eq 4 (“exponential correlation assumption”). In the sensitivity analysis (section 3.4) an assumption of a linear correlation between groundwater abstraction and subsidence is also tested. For more information, (see Supplementary material).
Table 2 presents an overview of the cost and effects evaluated in this study, a short description, and the approach and data used to estimate the monetary value. Estimation of investment costs of the alternative strategies includes construction as well as operation and maintenance (O&M) costs. The benefits of the alternative strategies assessed in this study are twofold: (1) the provision of clean water and (2) reducing the impact of subsidence. In relation to the latter, five major benefits related to subsidence impacts are quantified: reduced loss of land, reduced coastal flood risk, reduced damage to roads and buildings, and protection of coastal ecotourism. These impacts are considered the most significant impacts in the area: according to Lixin et al. (2010) the damage due to increased flood risk, land loss, and damage to roads and buildings is estimated to be 60–80% of the total damage of subsidence in Metropolitan area of Tianjin, China. Nonetheless, the extent of damage resulting from subsidence is much broader, including among other things damage to other infrastructures such as bridges, railroads, train stations, communications, power and energy, and water infrastructure, increased pluvial and fluvial flood risk, reduced attractiveness of business climate due to increased flood risk and damage to infrastructure (or conversely, higher maintenance costs/ taxes), lower agricultural yields and lower quality of life due to (stress in relation to) lower infrastructure service levels; increased flooding; changes in landscape quality (Kok and Costa, 2021; Mahya et al., 2021). These losses however were not calculated in this study due to the lack of data on economic loss in other infrastructures, increased pluvial and fluvial flood risk, and reduced attractiveness of business climate. And even though the method to quantify the loss of agricultural value in relation to increasing flooding has been used in another study by calculating the willingness to pay (WTP) for flood insurance (Hossain et al., 2022) and by implementing the relationship between net crop income and climate variables (Hossain et al., 2018), the agricultural loss is not calculated in this study due to the unavailability of WTP data and the crop income data.
The time horizon used in this study lies between 2022–2122, and a discount rate of 10% is used as it is commonly used by the Asian Development Bank in economic analysis in Asia (Asian Development Bank, 2017). For the purpose of the analysis, the assumption that all strategies will come into effect immediately in 2022 is applied. Further, the assumption that the subsidence rate under business as usual corresponds to the current subsidence rate is also applied (Figure 1).
To test the robustness of the results a sensitivity analysis on two aspects is conducted: (1) the correlation between groundwater abstraction and land subsidence rate and (2) the discount rate. The relation between groundwater abstraction and land subsidence, estimated using an exponential correlation relationship (eq4) is uncertain: land subsidence is a complex geological process that also depends on other factors beyond groundwater abstraction, such as natural compaction and loading. In the sensitivity analysis, the sensitivity of the outcome if a linear correlation is used is tested, i.e., a reduction of X% of groundwater abstraction due to an alternative strategy will reduce the subsidence rate also by X%. Thus, the linear correlation assumption follows Eq 5.
The sensitivity of results to the discount rate is tested as discount rates in the literature vary strongly. Therefore, the initial 10% to an alternative discount rate of 2.4% is compared, based on Bloomberg (2021).
Due to limited available data, this study did not analyze the full range of economic effects related to subsidence and was based on several (coarse) assumptions, e.g. the correlation between groundwater abstraction and subsidence and the monetization of effects. Results should therefore be regarded as exploratory. The unaddressed effects in this study cause underestimation of the result: these include reduced (avoided) damage/ loss to railways, telecommunication network, energy network, drinking water network, sewage or water management/ drainage, public/private green space, reduced attractiveness of business climate due to damage to the building and increased risk of flooding, lower productivity of agriculture due to salinity and drainage problems, decreased quality of the living environment and higher health problems, business interruption due to increased restoration/ maintenance works as well as traffic delays caused by the inundation of roads, and increase in fluvial and pluvial flood risk.
Aside from excluded benefits, limited data available also cause this study to apply several assumptions in the analysis. This study assumes that the subsidence rate will remain constant in the future under BaU unless an alternative strategy to reduce or stop deep groundwater abstraction is implemented. In reality, economic development may lead to an increase in the subsidence rate due to increased water demand and consequent deep groundwater abstraction. This also cause the underestimation of the expected subsidence impact. On the other hand, land subsidence can also reduce the attractiveness of the business climate which then can lead to avoided deep groundwater demand by the industry as well as a reduction in subsidence rate. This can cause an overestimation of the expected subsidence impact.
All strategies are assumed to be applied and will come into effect immediately in 2022. It may lead to an overestimation of the benefits of reducing land subsidence due to a lack of knowledge of how long each strategy will take to show the desired result. This study also disregards any small-scale adaptation measures already in place, e.g. dikes, which to some degree can reduce the economic impact of land subsidence.
The results of the analysis are presented in Table 3. This table represents the investment cost and effects relative to BaU. The benefit of the alternative strategies in avoiding loss of land is the most significant, followed by the provision of clean water, avoided damage to roads and buildings, as well as avoided increased coastal flood risk. The smallest economic impact is the protection of the value of the coastal ecosystem for tourism.
Table 3. Cost-benefit analysis of strategy 1, 2, and 3 under Scenario 1 and 2 with base analysis (exponential groundwater-land subsidence correlation assumption and 10% discount rate) in 2022–2122 in billion IDR (present value).
Compared with the investment costs, all strategies have an economic rationale (benefit-cost ratio (BCR) >1) under both the low and high-water demand scenarios. Strategy 1 has the highest benefit-cost ratio under low water demand (51.4), while strategy 3 has the highest benefit-cost ratio under the high water demand scenario (13.4). Benefit-cost ratios are lower under the high water demand scenario as the relative impact of the new clean water supply on the higher water demand (and consequent higher abstraction and subsidence) is lower. Accounting for the fact that many potential benefits—e.g. reduced damage to other infrastructure, reduced pluvial and fluvial flood risk, more attractive business climate, higher agricultural yields, and higher quality of life—the positive economic impact of all strategies can be expected to be even higher.
Table 4 shows that all strategies also show a positive benefit-cost ratio value (>1) under both water demand scenarios when a linear groundwater-land subsidence correlation is used. The difference between linear and exponential assumption is relatively small, which means that the CBA of the strategies is not very sensitive to assumptions regarding the correlation between deep groundwater abstraction-subsidence rates. As expected, a lower discount rate leads to significantly higher BCRs: as benefits of dealing with subsidence problems will continue far in the future, the chosen discount rate highly influences the result.
Under all circumstances, the benefits of the strategies outweigh the costs and are economically feasible. Results from the initial analysis remain robust: under scenario 1, strategy 1 is the preferred strategy, and under scenario 2 strategy 3. In this context, the most robust, “safe” strategy would be to invest in strategy 3 under “worst case” scenario 2. However, it may be advisable to develop an adaptive pathway in implementation: begin with implementing strategy 1, and if water demand seems to follow the pathway sketched in scenario 2, initiate further investments in scaling up piped water supply (to strategy 3) or even including strategy 2 (the coastal reservoir). Although strategy 2 does not show the highest benefit-cost ratio under any scenarios, it does have a positive CBR and may still be a part of a combined strategy.
Economic analysis in the field of land subsidence is very limited. A study by Dinar et al. (2021), developing a land subsidence extent index (Figure 3), shows the comparison of land subsidence impact around the world. It is known from this study that the study area in Semarang and Demak, Indonesia, has the highest subsidence impact index, hence, stressing the importance of analyzing the economic rationale of public investment strategies to reduce subsidence impact in this region.
Figure 3. Global impact extent of land subsidence. Source: Dinar et al. (2021).
This study addressed a knowledge gap in the economic analysis of subsidence impact as the first study analyzed the costs and benefits of land subsidence mitigation strategies in Indonesia. The result of this study is valuable in giving an indication of the economic impact of subsidence, as well as in supporting public authorities in the decision-making process on how to deal with the subsidence issue. The most notable impacts of land subsidence in Semarang and Demak are damage to buildings and infrastructure (direct effect) and increasing coastal flood risk and even permanent land loss (indirect effect). Although many different impacts of land subsidence were not addressed in this study, these impacts may also be very significant, e.g. obstruction of economic activities and decreased quality of life. Even though the impact of subsidence in the study area is very significant, it has only recently received public attention in the past few years: this can likely be attributed to the fact that land subsidence is often not seen as an urgent problem due to its slow onset and invisibility of subsidence as a hazard (Lixin et al., 2010). Currently, the public authorities of Indonesia, Central Java, and Semarang are developing a land subsidence roadmap to adapt to and mitigate land subsidence in Semarang specifically and in Indonesia in general: this study demonstrates a methodology that may be of use in the further development of this roadmap, and the results of this study offer valuable insights for the development of subsidence mitigation strategies in Semarang and Demak.
This study shows consistent results, both in the main analysis and sensitivity analysis, that show that strategy 1—a piped water supply system with 1,000 L/s capacity—has the highest benefit-cost ratio in a low water demand scenario (scenario 1) and strategy 3—a piped water supply system with 2,000 L/s capacity—in a high water demand scenario (scenario 2). Both strategies have positive BCRs under all scenarios: the most robust solution, i.e. the solution that is best under the worst-case (high water demand) scenario is strategy 3.
The alternative strategies proposed in this study require a significant investment. This type of project—water supply infrastructure—is usually funded by the government. From the public perspective, providing clean water for domestic use may have a higher political urgency than providing clean water for industry: this may act as a disincentive to prioritize industrial water supply, even though this is the main cause of overexploitation of deep groundwater as well as land subsidence. This study may serve as background to demonstrate the wider benefit for society at large if the industrial water supply is prioritized.
The result of this study shows that loss of land has the highest socio-economic impact caused by land subsidence. As the proposed alternative strategies take time to be implemented and come into effect, short-term adaptation actions may be considered in addition to the strategies proposed in this study to minimize the impact of land subsidence, e.g. building (higher) dikes to protect the land against flooding and pumping stations to drain the land. Continuous monitoring of groundwater abstraction, subsidence rate, as well as the implemented measures is also recommended. Moreover, if the high water demand scenario (scenario 2) turns out to be real in the future, the alternative strategies analyzed in this study cannot meet water demand: additional measures will be needed. Based on a study by Jianbing et al. (2010) in Beijing China, applying urban rainwater harvesting gives an average benefit cost ratio of 2 with one of the benefits included in the analysis being a decrease in land subsidence. Aquifer recharge may also be an attractive strategy in the region to mitigate subsidence as implemented in Las Vegas Valley, Nevada (Donovan et al., 2002). A study case in Gouda the Netherlands shows another potential mitigation strategy, i.e. managing subsidence by reducing the consequences of subsidence rather than preventing subsidence altogether e.g. strengthening building structure to cope with subsidence damage, which has economic rationale with 1.48 BCR (Kok and Hommes-Slag, 2020). The approach and results of this study are mostly relevant to other cities and/or countries where land subsidence is related to overexploitation of deep groundwater, e.g. in Jakarta and Bangkok.
The subsidence rates for the study area (shown in Figure 1) which form the basis of our economic impact analysis are based on satellite data of a very limited time series—at the time of writing, no more elaborate data was available. The resulting estimation of subsidence rates is highly uncertain (as also discussed by Yuwono et al., 2019). Furthermore, due to limited available exposure data and lack of dose-effect relationships, this study did not analyze the full range of economic effects related to subsidence.
Furthermore, the study would greatly benefit from improved analysis of the subsidence hazard based on more extensive time series of observational data. This study has shown that the development of water demand over time has a large impact on the economic rationale of various strategies. A more elaborate assessment of water demand (including the relation between groundwater abstraction and subsidence rate) is needed. Furthermore, it would be valuable to analyze the economic impacts not yet quantified in this study to enable more a comprehensive analysis. To avoid under or overestimation of the result, study related to effect of applied small scale adaptation measure, how long the alternative strategies effect the subsidence, and how the economic development influences the subsidence rate under BaU would be valuable. The results of this study demonstrate there is likely a clear rationale for taking action in reducing the negative impacts of subsidence: more CBA research in other susceptible regions would be valuable.
The alternative strategies analyzed in this study only address the land subsidence problem by providing clean water from surface water in order to reduce (stop) the deep groundwater abstraction. Expanding the analysis to include other mitigation strategies would be very valuable, e.g. artificial aquifer recharge, land use management, and adaptation measures like dikes, houses on stilts, and lightweight infrastructure. Since this study only analyze the economic rational of the alternative strategy, further study related to technical and/ or social feasibility is highly recommended.
Economic and population growth in Semarang have caused increased water demand, largely met by increased groundwater abstraction which in turn has led to significant land subsidence in the area of Semarang and Demak. Public authorities have already taken some actions to address this issue by a clean water supply system in West Semarang and a freshwater reservoir along the coast in East Semarang. Despite these developments, the economic damage caused by the land subsidence in the study area is still expected to be very significant if no additional new policy or strategy is adopted. In this study, the economic rationale for taking further action by comparing three alternative strategies with business as usual (no additional measures taken) is analyzed. The three strategies include building (1) a new clean water supply system with 1,000 L/s capacity, (2) a 2 km2 coastal reservoir with a supply system in West Semarang, and (3) a new clean water supply system with 2,000 L/s capacity. All three strategies have a positive (>1) benefit-cost ratio: they all have an economic rationale for investment. The highest BCR is strategy 1 under a low water demand scenario and strategy 3 under a high water demand scenario. Thus, applying strategy 3; building a clean water supply system with a capacity of 2,000 L/s or 63 million m3/year to distribute the surface water from the Jatibarang dam is recommended as it is the most robust strategy; it gives the highest economic benefit of the public investment in the worst-case scenario to reduce subsidence impact in the Semarang-Demak region.
The original contributions presented in the study are included in the article/Supplementary material, further inquiries can be directed to the corresponding author.
BP: writing original draft. SK and BK: reviewing. MM: reviewing and editing. All authors contributed to the article and approved the submitted version.
This publication was funded by Deltares, Delft, The Netherlands.
The authors would like to thank Water Dialogues Project Partners; Deltares and Witteveen Bos, Mr. Bambang Triono (Financial Management Specialist), Mr. Helmi (Diponegoro University), and construction experts; Mr. Dhori, Mr. Muhtarom, Mr. Supriadi, and Mr. Tri for providing valuable feedback and insights.
The authors declare that the research was conducted in the absence of any commercial or financial relationships that could be construed as a potential conflict of interest.
All claims expressed in this article are solely those of the authors and do not necessarily represent those of their affiliated organizations, or those of the publisher, the editors and the reviewers. Any product that may be evaluated in this article, or claim that may be made by its manufacturer, is not guaranteed or endorsed by the publisher.
The Supplementary Material for this article can be found online at: https://www.frontiersin.org/articles/10.3389/frevc.2023.1073089/full#supplementary-material
Abidin, H. Z., Andreas, H., Gamal, M., Gumilar, I., Napitupulu, M., Fukuda, Y., et al. (2010). Land subsidence characteristics of the Jakarta basin (Indonesia) and its relation with groundwater extraction and sea level rise. In: Groundwater Response to Changing Climate. London: CRC Press. p. 113–130).
Abidin, H. Z., Andreas, H., Gumilar, I., Sidiq, T. P., Fukuda, Y. (2013). Land subsidence in coastal city of Semarang (Indonesia): characteristics, impacts and causes. Geomat. Nat. Hazards Risk. 4, 226–240. doi: 10.1080/19475705.2012.692336
Agency for Improvement of Drinking Water Supply System (2018). PDAM Performance 2018 - Region II. Jakarta: Ministry of Public Works and Public Housing of Republic Indonesia.
Asian Development Bank (2017). Guidelines for the Economic Analysis of Projects. Mandaluyong: Asian Development Bank.
Beek, V., Letitre, B., Hadiyanto, H., Sudarno, S. (2019). Alternatives to Groundwater Abstraction as a Measure to Stop Land Subsidence: A Case Study of Semarang, Indonesia. Semarang: E3S Web of Conferences. p. 125.
Bloomberg (2021). United States Rates and Bonds. Available online at: https://www.bloomberg.com/markets/rates-bonds/government-bonds/us (accesssed June 15, 2021).
Born, G. J., van den, Kragt, F., Henkens, D., Rijken, B., Bemmel, B., van, Sluis, S., et al. (2016). Dalende bodems, stijgende kosten. The Hague: Netherlands Environmental Assessment Agency.
Chaussard, E., Amelung, F., Abidin, H., Hong, S. H. (2013). Sinking cities in Indonesia: ALOS PALSAR detects rapid subsidence due to groundwater and gas abstraction. Rem. Sens. Environ. 128, 150–161. doi: 10.1016/j.rse.2012.10.015
Chen, C.-H., Wang, C.-H., Hsu, Y.-J., Yu, S.-B., Kuo, L.-C. (2010). Correlation between groundwater level and altitude variations in land subsidence area of the Choshuichi Alluvial Fan, Taiwan. Eng. Geol. 115, 122–131. doi: 10.1016/j.enggeo.2010.05.011
Chen, M., Tomás, R., Li, Z., Motagh, M., Li, T., Hu, L., et al. (2016). Imaging Land Subsidence Induced by Groundwater Extraction in Beijing (China) Using Satellite Radar Interferometry. Rem. Sens. 8, 468. doi: 10.3390/rs8060468
Development Planning Agency Semarang (2013). Penyusunan rencana induk sistem penyediaan air minum (RISPAM) Kota Semarang. Semarang: Development Planning Agency Semarang.
Dinar, A., Esteban, E., Calvo, E., Herrera, G., Teatini, P., Tomás, T., et al. (2021). We lose ground: Global assessment of land subsidence impact extent. Sci. Total Environ. 786, 147415. doi: 10.1016/j.scitotenv.2021.147415
Donovan, D. J. Katzer, T., Brothers, K., Cole, E., Johnson, M. (2002). Cost-benefit analysis of artificial recharge in Las Vegas Valley, Nevada. J. Water Res. Plan. Man. 128, 356–365. doi: 10.1061/(ASCE)0733-9496(2002)128:5(356)
Ellipsis (2020). Ellipsis-Earth. Available online at: https://insar.ellipsis-earth.com/ (accesssed May 20, 2020)
Galloway, D., Jones, D. R., Ingebritsen, S. E. (2000). Land subsidence in the United States. US Geological Survey Circular. Virginia: U.S. Geological Survey.
Gamper, C. D., Thoeni, M., Weck-hannemann, H. (2006). A conceptual approach to the use of cost benefit and multi criteria analysis in natural hazard management. Nat. Hazards Earth Syst. Sci. 6, 293–302. doi: 10.5194/nhess-6-293-2006
Gong, H., Pan, Y., Zheng, L., Li, X., Zhu, L., Zhang, C., et al. (2018). Long-term groundwater storage changes and land subsidence development in the North China Plain (1971–2015). Hydrogeology 26, 1417–1427. doi: 10.1007/s10040-018-1768-4
Herrera-García, G., Ezquerro, P., Tomas, R., Béjar-Pizarro, M., López-Vinielles, J., Rossi, M., et al. (2021). Mapping the global threat of land subsidence. Science 371, 34–36. doi: 10.1126/science.abb8549
Hossain, M. S., Alam, G. M., Fahad, S., Sarker, T., Moniruzzaman, M., Rabbany, G. M. (2022). Smallholder farmers' willingness to pay for flood insurance as climate change adaptation strategy in northern Bangladesh. J Cleaner Prod. 338, 130584. doi: 10.1016/j.jclepro.2022.130584
Hossain, M. S., Qian, L., Arshad, M., Shahid, S., Fahad, S., Akhter, J. (2018). Climate change and crop farming in Bangladesh: an analysis of economic impacts. Int. J. Clim. Chan. Strat. Man. 11, 424–440. doi: 10.1108/IJCCSM-04-2018-0030
Hu, B., Zhou, J., Xu, S., Chen, Z., Wang, J., Wang, D., et al. (2013). Assessment of hazards and economic losses induced by land subsidence in Tianjin Binhai new area from 2011 to 2020 based on scenario analysis. Nat. Haz. 66, 873–886. doi: 10.1007/s11069-012-0530-9
Huizinga, J., de Moel, H., Szewczyk, W. (2017). Global Flood Depth-Damage Functions. Brussels: Joint Reserach Center.
Indonesia Business Council for Sustainable Development (2015). Visi Indonesia 2050 kontribusi sektor bisnis bagi Indonesia masa depan. Jakarta: Indonesia Business Council for Sustainable Development.
Indonesia National Population and Family Planning Agency (2015). Dinamika demografis Indonesia 1950 - 2100. Jakarta: Indonesia National Population and Family Planning Agency.
Japan International Cooperation Agency (2009). Preparatory Survey for Public-Private Partnership Infrastructure Project in the Republic of Indonesia. Jakarta: Japan International Cooperation Agency.
Jianbing, Z., Changming, L., Hongxing, Z. (2010). Cost–benefit analysis for urban rainwater harvesting in Beijing. Water Int. 35, 195–209. doi: 10.1080/02508061003667271
Kahn, M. E., Mohaddes, K., Ng, R. N. C., Pesaran, M. H., Raissi, M., Yang, J.-C. (2019). Long-Term Macroeconomic Effects of Climate Change: A Cross-Country Analysis. Washington, DC: International Monetary Fund.
Kok, S., Costa, A. L. (2021). Framework for economic cost assessment of land subsidence. Nat. Hazards 106, 1931–1949. doi: 10.1007/s11069-021-04520-3
Kok, S., Hommes-Slag, S. (2020). Cost-benefit analysis of urban subsidence mitigation strategies in Gouda, the Netherlands. Proc. Int. Assoc. Hydrol. Sci. 382, 761–766. doi: 10.5194/piahs-382-761-2020
Letitre, P., Kooi, H. (2018). National Capital Integrated Coastal Development Road Map Mitigation Land Subsidence. Jakarta: Deltares.
Liu, Y., Chen, Z., Wang, J., Hu, B., Ye, M., Xu, S. (2012). Large-scale natural disaster risk scenario analysis: a case study of Wenzhou City, China. Nat. Hazards 60, 1287–1298. doi: 10.1007/s11069-011-9909-2
Lixin, Y., Jie, W., Chuanqing, S., Guo, J., Yanxiang, J., Liu, B. (2010). Land subsidence disaster survey and its economic loss assessment in Tianjin, China. Nat. Hazards Rev. 11, 35–41. doi: 10.1061/(ASCE)1527-6988(2010)11:1(35)
Mahya, M., Kok, S., Lelij, A., van der, C, Pardo, M., de, L. (2021). Economic Assessment of Subsidence in Semarang and Demak, Indonesia. Jakarta: Deltares.
Marfai, M. A., King, L. (2007). Monitoring land subsidence in Semarang, Indonesia. Environ. Geol. 53, 651–659. doi: 10.1007/s00254-007-0680-3
Mehvar, S., Filatova, T., Syukri, I., Dastgheib, A., Ranasinghe, R. (2018). Developing a framework to quantify potential sea level rise-driven environmental losses: a case study in Semarang coastal area, Indonesia. Environ. Sci. Pol. 89, 216–230. doi: 10.1016/j.envsci.2018.06.019
Ministry of Public Work Indonesia (2012). Waduk Jatibarang Sebagai PSDA dan Pengendali Banjir. Jakarta: Ministry of Public Work Indonesia.
Ministry of Public Work Indonesia. (2019). Kerjasama Pemerintah dengan Badan Usaha dari sektor penyediaan air minum di Indonesia. Jakarta: Ministry of Public Work Indonesia.
MLA+ Fabrications, Deltares Witteveen Bos, UNDIP, Unissula, and IDN Liveable Cities.. (2019). Cascading Semarang Steps to Inclusive Growth. Jakarta: MLA+.
One Architecture and Urbanism Kota Kita, Deltares, Wetland International, Sherwood Design Engineers, Hysteria Grobak. (2019). One Resilient Semarang Volume II. Jakarta: One Architecture and Urbanism.
Putranto, T. T., Rüde, T. R. (2016). Hydrogeological model of an urban city in a coastal area, case study: Semarang, Indonesia. Indonesian J. Geosci. 3, 17–27. doi: 10.17014/ijog.3.1.17-27
Qi-yan, F., Gang-jun, L. I. U., Lei, M., Er-jiang, F. U., Hai-rong, Z., Ke-fei, Z. (2008). Land subsidence induced by groundwater abstraction and building damage level assessment — a case study of Datun, China. J. China Univ. Mining Technol. 18, 556–560. doi: 10.1016/S1006-1266(08)60293-X
Romijn, G., Renes, G. (2013). General Guidance for Cost-Benefit Analysis. The Hague: Netherlands Bureau for Economic Policy Analysis and Netherlands Environmental Assessment Agency.
Salam, N. (2018). Empat Investor Berminat Danai Proyek SPAM Semarang Barat. Available online at: https://semarang.merdeka.com/kabar-semarang/empat-investor-berminat-danai-proyek-spam-semarang-barat-180418z.html (accessed May 11, 2021).
Santikayasa, I., Agis, P., Maesaroh, S. (2017). Alokasi sumberdaya air berdasarkan kriteria ekonomi Menggunakan Aplikasi Aquarius (Studi kasus : SubDAS Ambang-Brantas, Indonesia). Agromet 31, 89–102. doi: 10.29244/j.agromet.31.2.89-102
Sarah, D., Satriyo, N. A., Mulyono, A. (2014). Kajian awal estimasi kerugian fisik akibat aamblesan tanah di Kota Semarang. Prosiding Pemaparan Hasil Penelitian Pusat Penelitian Geoteknologi Lipi Tahun 2014. Peran Penelitian Geoteknologi Untuk Menunjang Pembangunan Berkelanjutan Di Indonesia (Bandung: The Indonesian Institute of Sciences), 37–45.
Semarang Central Bureau of Statistics. (2020a). Jumlah penduduk menurut jenis kelamin. Available online at: https://semarangkota.bps.go.id/indicator/12/78/1/jumlah-penduduk-menurut-jenis-kelamin.html (accessed May 22, 2021).
Semarang Central Bureau of Statistics. (2020b). Profil industri besar dan sedang Kota Semarang 2019. Available online at: https://semarangkota.bps.go.id/publication/2020/11/30/fa72c8bfd80ee2f3639ba477/profil-industri-besar-dan-sedang-kota-semarang-2019.html (accessed May 22, 2021).
Semarang Central Bureau of Statistics. (2020c). Semarang Central Bureau of Statistics. Available online at: https://jateng.bps.go.id/subject/9/industri.html#subjekViewTab5 (accessed May 22, 2021).
Shin, E., Hufschmidt, M., Lee, Y., Nickum, J. E., Umetsu, C., Gregory, R. (1997). Valuating the Economic Impacts of Urban Environmental Problems: Asian Cities. Washington, DC: UNDP.
Suripin (2005). Contribution of groundwater abstraction to land subsidence at The North Coast of Semarang. Media Komunikasi Teknik Sipil 13, 27–36.
Thanden, R. E., Sumardirdja, H., Richards, P. W. (1996). Geological Map of Magelang and Semarang, Java. Bandung: Center of Geological Research and Development.
The World Bank (2006). Indonesia: Enabling Water Utilities to Serve the Urban Poor. Washington, DC:: The World Bank.
UNESCO (2020). International Land Subsidence Symposium Proceedings. Available online at: www.Landsubsidence-Unesco.Org (accessed July 2, 2021).
Valentino, D. (2013). Study of groundwater resource utilization supervision in industrial area of Semarang City. Jurnal Wilayah Dan Lingkungan 1, 265–274. doi: 10.14710/jwl.1.3.265-274
Willemsen, W., Kok, S., Kuik, O. (2020). The effect of land subsidence on real estate values. Proc. Int. Assoc. Hydrol. Sci. 382, 703–707. doi: 10.5194/piahs-382-703-2020
Yuwono, B. D., Subiyanto, S., Pratomo, A. S., Najib, N. (2019). “Time series of land subsidence rate on coastal Demak using GNSS CORS UDIP and DINSAR,” in E3S Web of Conferences, Vol. 94 (Bali: EDP Science), 04004. doi: 10.1051/e3sconf/20199404004
Keywords: cost-benefit analysis, economic assessment, land subsidence, mitigation, Semarang, subsidence damage
Citation: Pratiwi BA, Kok S, Kaiser BA and Mahya MJ (2023) Cost-benefit analysis of mitigating subsidence damage in Semarang and Demak, Indonesia. Front. Environ. Econ. 2:1073089. doi: 10.3389/frevc.2023.1073089
Received: 18 October 2022; Accepted: 27 February 2023;
Published: 17 March 2023.
Edited by:
Madhu Verma, World Resources Institute, United StatesReviewed by:
Md. Shakhawat Hossain, Northwest A&F University, ChinaCopyright © 2023 Pratiwi, Kok, Kaiser and Mahya. This is an open-access article distributed under the terms of the Creative Commons Attribution License (CC BY). The use, distribution or reproduction in other forums is permitted, provided the original author(s) and the copyright owner(s) are credited and that the original publication in this journal is cited, in accordance with accepted academic practice. No use, distribution or reproduction is permitted which does not comply with these terms.
*Correspondence: Berlian A. Pratiwi, YmVybGlhbnByYXRpd2kwN0BnbWFpbC5jb20=
Disclaimer: All claims expressed in this article are solely those of the authors and do not necessarily represent those of their affiliated organizations, or those of the publisher, the editors and the reviewers. Any product that may be evaluated in this article or claim that may be made by its manufacturer is not guaranteed or endorsed by the publisher.
Research integrity at Frontiers
Learn more about the work of our research integrity team to safeguard the quality of each article we publish.