- 1Department of Chemistry, Maseno University, Maseno, Kenya
- 2Department of Chemistry, University of Malawi, Zomba, Malawi
- 3Department of Physical Sciences, Kaimosi Friends University, Kaimosi, Kenya
There has been an increase in the production of food waste materials worldwide due to rapid population growth. The ineffective and sometimes unscientific and ad hoc disposal of these food waste materials has led to environmental pollution. Studies have reported the occurrence of heavy metals in water resources poses serious health threats to the environment and human health. Heavy metals are documented to be recalcitrant to conventional water treatment facilities since they are non-biodegradable. The use of food waste-based adsorbents provides an alternative solution for the adsorption of heavy metals in water resources, with concomitant benefit of valorization of otherwise waste materials. Therefore, this study examined the applications of food waste-based adsorbents for the removal of heavy metal ions. The study adopted a literature-based approach which involved reviewing published papers from selected science databases. The results indicate that these bioadsorbents have great removal efficiencies for different heavy metals with, rice husks and sugarcane bagasse demonstrating special sorption properties, especially for chromium and lead metal ions, respectively. The adsorption data were mostly best described by the Langmuir and Freundlich isotherm models, suggesting a monolayer coverage with similar sites and a heterogeneous surface, respectively. Further, the kinetic studies indicated that the adsorption processes largely followed a pseudo second-order model, showing chemisorption-mediated rate-limiting steps. However, regardless of these encouraging results attained, the use of food waste-based adsorbents has limitations such as variation in the composition and the structure. This leads to inconsistencies in adsorption efficiencies, regenerations and reuse, and reduced removal capacities. There is also the possibility of leaching of heavy metals from the adsorbents which may in-turn cause secondary pollution. Sustainability investigations such as life cycle assessment, cost-benefit analysis, pilot-scale studies and optimization studies present areas for future research.
1 Introduction
Climate change and environmental degradation that has become a threat to the existence of life has sparked increased research in technologies, processes and the birth of academic disciplines that may be described with words such as “sustainable development”, “green chemistry”, “bioenergy”, “zero emissions” and “eco-friendly products”. There has been also development of policies and legislations related with environmental protection (Shen et al., 2023). The technologies, products and processes must be seen not only to be environmentally friendly but must also be demonstrated to be cost effective. Only then can they be described as ‘sustainable’ especially when the waste generated possess economic value (Tonini et al., 2018). These concerns inevitably affect the agricultural and food sectors where huge amounts of waste, in the range of billions of kilograms are generated annually.
The food industry generates billions of kilograms of food wastes annually (Amicarelli et al., 2021; Amicarelli and Bux, 2021). Unfortunately, food is highly susceptible to microbial spoilage, a critical limitation for their reuse besides legal restrictions and logistical issues related to the costs of collection and storage (Kohli et al., 2024). As a result, food wastes are mostly used as animal feed, incineration materials or disposed to sometimes already mountainous landfill (Girotto et al., 2015). However, while in landfills the wastes produce secondary wastes that require further treatment, such as fugitive air emissions (unpleasant odors) and especially leachate containing toxic organic compounds and heavy metals which contaminate ground water (Lu et al., 2015; Tang, 2020).
In terms of value, it is noteworthy that food wastes generally contain high amounts of cellulose, lignin and proteins that can be harnessed as renewable natural resources for a plethora of low-cost, environmentally friendly and sustainable materials (Figure 1). Examples of such inexpensive materials are low cost adsorbents.
Adsorption is universally recognized as an efficient and cost effective method for organic and inorganic water contaminants sequestration in wastewater treatment regimes. Adsorption as a technique possesses the inherent advantages of flexibility in design besides high removal efficiency of various pollutants and possibility of adsorbent recovery, regeneration by desorption process and reuse for numerous cycles (Nyairo et al., 2017). Activated carbon (AC) is the most widely used adsorbent in the removal of heavy metals from wastewater. The large micropore and mesopore volumes and high surface area of activated carbons imbue them with high adsorption capabilities (Sánchez-Ponce et al., 2022). However, due to the recent depletion of source materials for commercial coal-based AC, the capital costs for AC has been on an exponential rise. Consequently, there has been increased research in search of alternative, abundant and inexpensive carbonaceous materials as precursors for development of activated carbons.
Food wastes possess the dual advantage of availability (since they are generated in large scales), being inexpensive and thus can be potential precursor materials for development of low-cost sorbents. In the recent years, there has been an increase in scientific publications reporting the potentiality of food wastes as adsorbents or precursors for the development of as low-cost adsorbents for removal of various pollutants from wastewater (Neolaka et al., 2023). This paper discusses the recent developments and application of food wastes derived adsorbents, namely,; bone chars, biosorbents, and chitin and chitosan derivatives, for removal of heavy metals from wastewater. The reuse of otherwise low value food wastes in water treatment is particularly important for the food industry.
2 Bone chars
Activated carbon has traditionally been the most widely used adsorbent in wastewater treatment. However, activated carbon is relatively expensive compared to other adsorbents. In recent years, many low-cost sorbents including agricultural waste and byproducts have also been tested in batch and fixed bed sorption systems by a number of authors. The ability of bone char to adsorb various water pollutants has been demonstrated in the recent past (Medellín-Castillo et al., 2020; Piccirillo, 2023; Rahman, 2024).
The application of bone char in large scale can be dated back to the 19th century in the sugar industry. It was used for decolorization and ash removal (Barrett et al., 1951). In later years, its application was extended to defluoridation of drinking water, but due to problems associated with cost and effect of the adsorbent on the taste of the so-treated water, its application was discontinued. However, in the recent past carbonization of bones to obtain char through thermal treatment of bones via pyrolysis has been adopted. In order to reduce the cost of production, the pyrolysis process focuses on energy recovery and at the same time the high temperatures (above 400°C) helps to completely eliminate organic matter from the char (C. K. Rojas-Mayorga et al., 2015). Although production and use of bone char in large scale is practiced in many countries, primarily for decolorization of sugar, there exists a paucity of data in the scientific literature on usage of bone char as an adsorbent relative to the other types of adsorbents. However, this trend seems to be taking a different trajectory in the recent past championed by McKay and co-workers (Cheung et al., 2001; Cheung et al. 2002; Choy and McKay, 2005; Ko et al., 2004; Piccirillo et al., 2017).
2.1 Preparation of bone chars
The production of bone chars follows several important steps that considerably determines the physicochemical characteristics of the resulting char and its subsequent applications in adsorption processes. The preparation process starts with pretreatment, which involves cleaning of the bones with distilled water followed by the drying process that eliminates both moisture and organic matter in order to promote the efficiency of carbonization process. Thereafter, pyrolysis is conducted at controlled temperatures and finally, possible post-treatment modifications are conducted (Nigri et al., 2017). The uniqueness of the resulting char is determined by the characteristics of the each bone since the bone char is mostly derived through the carbonization of animal bones of different sources such as cattle, goat, chickens, fish, rabbit and pigs among others (Thompson and Kearns, 2021).
The pyrolysis process involves thermal decomposition of organic matter in a deficient supply of oxygen gas, and is regarded as the most significant during bone char production. Further, the operating temperature and pyrolysis duration can greatly affect the structure, composition and adsorption efficiencies of the derived bone chars. Hence, researchers have indicated the pyrolysis optimal temperature to range between 400°C and 600°C. Studies have demonstrated that temperatures above the optimal range cause degradation of the hydroxyapatite structure on the bone char which is suitable for ions removal (C. K. Rojas-Mayorga et al., 2013). Post treatment of the derived bone chars such as washing with acid has indicated to promote the adsorption characteristics by increasing the surface area and functional groups (C. K. Rojas-Mayorga et al., 2015). The bone chars and their derivatives are made up of carbon and calcium phosphate which poses great surface porosity leading to high surface area for removal of chemical pollutants. A composite of bone char-zinc oxide investigated for the removal of hexavalent chromium showed that the composite mainly comprised of calcium hydroxyapatite, (Ca5(PO4)3OH) and had a specific surface area 28.09 m2g-1 suggesting its suitability for use in water treatment (Ranjbar et al., 2018). The Scanning electron microscope (SEM) and Fourier-transform spectroscopy (FTIR) analytical instruments are among the tools used for characterization of bone chars to determine physicochemical properties such as morphology and functional groups (Nigri et al., 2017; Ranjbar et al., 2018). These analyses are significant as they provide insight on understanding on the removal of Cr(VI), Cd(II), Ni(II), and Pb(II) ions from water using the bone char and the associated adsorption mechanisms between bone chars and various chemical contaminants in water.
2.2 Application of bone chars in water treatment
As aforementioned, the earliest uses of bone char was in sugar decolorization (Barrett et al., 1951). However, there exists a substantial amount of scientific literature describing the use of bone chars in sequestration of various water contaminants. For example, bone chars have been used for removal of heavy metal ions, fluoride and dyes from aqueous solutions and removal of phosphorous and chemical oxygen demand (COD) from a real secondary effluent (W. Xie et al., 2004).
The next section discusses the use of bone chars for removal of heavy metals from aqueous solutions.
2.3 Adsorption of heavy metals onto bone chars
Bone chars obtained by calcination of cattle bones at 450°C were examined for the removal of Cr(VI) from contaminated water and the char demonstrated a capability of reducing the levels of the metal ions from aqueous solutions to acceptable limits. Results showed 100% uptake of Cr(VI) ions at pH 1, initial Cr(VI) concentration of 10 mgL-1, and adsorbent dosage of 2 g for 2 h contact time. The char attained a maximum adsorption capacity of 4.8 mgg-1 at an initial Cr(VI) concentration of 800 mgL-1 after 8 h. The adsorption data conformed to the Langmuir isotherm model and the kinetic studies followed pseudo-second order kinetic model (Hyder et al., 2015). A similar study, investigating the removal of Cd(II), Ni(II), and Pb(II) ions from water using the bone chars also reported an uptake of the metal ion in the range 60%–92%, indicating the effectiveness of the prepared chars to decontaminate polluted water. Variability in the uptake of these metals was attributed to the difference in their physical properties of which ionic radius was dominant. Ion exchange between the Ca2+ ions from the hydroxyapatite of the bone char and the divalent ions played an important role in the removal of the heavy metal ions from aqueous media (Mendoza-Castillo et al., 2015). Again, another separate study reported that Cd(II), Zn(II) and Ni(II) ions were successfully eliminated from aqueous solution through the use of bone chars. Optimized conditions of the pyrolysis process enhanced the adsorption capability of the char by 143%. In the unary system, the study reported adsorption capacity ranging from 68.3 to 119.4 mg/g with the removal of the metals following the order Cd(II) > Zn(II) > Ni(II). However, antagonistic adsorption was observed in both the binary and tertiary aquatic systems. Uptake of the metal ions by the char was through ions exchange of Ca2+ ions from the hydroxyapatite with the cations (C. Rojas-Mayorga et al., 2016). Another investigation was carried out on bone char obtained from invasive fish species for the adsorption for Cd(II) and fluoride ions in single and binary aqueous systems. The study revealed that the uptake of both ions was pH dependent, with the uptake of Cd(II) increasing with increase in solution pH but the contrary applied for the adsorption of fluoride ions. In the binary system it was established that the presence of fluoride ions did not interfere with Cd(II) sorption but Cd(II) had an antagonistic effect on the adsorption of fluoride ions. Physisorption and ion exchange were the proposed mechanisms for the uptake of the metals (Medellín-Castillo et al., 2020).
Wilson and co-workers (2003) investigated the adsorption efficiency and mechanisms of Cu(II) and Zn(II) onto bone charcoal. The detection of calcium ions in the bulk solution with concomitant decrease in the concentration of Cu(II) ions indicated that ion-exchange was the most significant mechanism for the removal of Cu(II) from the solution. This hypothesis was further strengthened by the approximately 1:1 mol ratio of Ca:Cu reported, corresponding to the suggested adsorption mechanism by ion exchange. The study also revealed that Zn(II) adsorption mechanism was predominantly controlled by chemisorption rather than ion exchange as depicted by the relatively low Ca:Zn mol ratios (∼0.2:1). The authors did not report the precise mechanisms for Zn(II) adsorption. Bone char had a higher adsorption capacity for Cu(II) than Zn(II) but it is noteworthy that the adsorption capacities for both metal ions were relatively higher compared to other adsorbents (Wilson et al., 2003).
Cheung et al. (2001) investigated the sorption kinetics for the removal of Cd(II) from effluents using bone char in a batch reactor. The time-dependent sorption data was fitted to four sorption kinetic models, namely,; the pseudo-first order, the Ritchie second order, the modified second order and the Elovich equations in order to determine the best-fit equation for the adsorption of Cd(II) ions onto bone char. Based on the sum of the errors squared (SSE), the Elovich equation best described the sorption of Cd(II) onto bone char among the four kinetic models. Conformity to the Elovich model is consistent with heterogeneous nature of bone char surface since it contains various types of binding sites. The sorption capacity of bone char for Cd(II) ions was found to be 0.57 mmol g-1 at an equilibrium solution concentration of 3.0 mmol dm-3. In a separate study, (Cheung, Chan, et al., 2001), reported the sorption of Cu(II) and Zn(II) onto bone char under batch equilibration studies. The work demonstrated that the metal ions were removed from the aqueous media by calcium hydroxyapatite, which is the main component in bone char, by means of adsorption and ion exchange mechanisms. X-ray desorption patterns depicted a reduction in the degrees of the crystalline of hydroxyapatite lattice in the bone char below pH 4, an indicator that at pH five and above, the bone char is suitable for the sorption of the metal ions from effluent to minimize the loss of the sorbent. Additionally, the experimental data from the adsorption isotherms and batch kinetics studies were fitted using the Langmuir equation and a film-pore diffusion mass transport model, respectively. The work demonstrated the possibility of applying bone char as an alternative adsorbent for water treatment. Later on, Choy and McKay (2005) reported the adsorption rates of Cd(II), Cu(II) and Zn(II) ions in single component systems onto bone char using the Crank diffusion model. The effects of initial metal ion concentration and bone char dosage were also reported. Noteworthy, these values are comparable to other adsorbents reported in literature. The solution of the Crank diffusion model indicated that the surface diffusion coefficient was a variable function of initial metal ion concentration. The bone char had considerably high adsorption capacities of 0.477, 0.709 and 0.505 mmol g-1 for Cd(II), Cu(II) and Zn(II), respectively. These capacities are notably higher than most other literature values for other materials. Finally, (Ko et al. (2004) investigated the ability of bone char to adsorb copper (II), zinc (II) and cadmium (II) ions from synthetic wastewater in single-component and binary equilibrium systems. The single-component equilibrium data were analyzed using the Langmuir and the Sips equilibrium isotherm equations. Here, the Sips isotherm gave a better fit of the experimental data than the Langmuir isotherm based on the sum of squares errors (SSE) analysis. The Cu–Zn, Cu–Cd and Cd–Zn binary equilibrium experimental data were examined by incorporating the Langmuir and the Sips isotherm equations into the ideal adsorbed solution theory (IAST) in an attempt to correlate the experimental sorption equilibrium capacity data in multicomponent systems. The calculations showed that the sorption of metal ions for multicomponent systems could be predicted reasonably well from the IAS theory with the Langmuir equation or the Sips equation for metal ions. The modeling assumed no interaction between the adsorbing ions and this may affect the accuracy of the calculations. These studies unequivocally indicate the potential of bone chars as low cost adsorbents for metal ions’ removal from wastewaters.
Bone char exhibits high adsorption capacity for Cd(II), Zn(II), Cu(II) ions without modification. Studies also show that the uptake of metal ions by bone char in through ion exchange between Ca2+ ions from the hydroxyapatite of the bone char. The adsorption data for the heavy metal ions on bone char fit better with Sips adsorption isotherm model than Langmuir model.
3 Biosorbents
Biosorption is a process involving the physicochemical uptake of a sorbate from a solution by living or non-living biomass cells of plants (S. Xie, 2024). The search for low-cost and readily available adsorbents to remove heavy metals ions from wastewater has been a main research focus. The solid residue from agricultural practice and food waste has been found to provide an abundant and cheap precursor material for biosorption (Neolaka et al., 2023). As a result, numerous food and agricultural waste materials have been studied as biosorbents for the removal of heavy metal ions from wastewater because they are produced in large quantities. They include: rice husks (Okoro et al., 2022), wheat bran (Ogata et al., 2013), sugarcane bagasse (Yogeshwaran and Priya, 2021), potato peels (Osman et al., 2019), egg shells (Kim et al., 2019), citrus peels (Husoon et al., 2013) among others.
The biosorbents may be used either in their natural form or chemically modified form. The chemical modification of biosorbents makes them acquire reasonable surface areas and hence enhance their adsorption efficiencies (Hegazi, 2013). The pretreatment methods that have been applied include the use of activating agents such as basic solutions (sodium carbonate, sodium hydroxide, calcium hydroxide), acidic solutions (hydrochloric acid, sulphuric acid, nitric acid, tartaric acid, citric acid), organic compounds (methanol, ethylenediamine, epichlorohydrin) and oxidizing agents such as hydrogen peroxide (Neolaka et al., 2023).
3.1 Rice husks
Rice husks, a product of rice milling process comprises of lignocellulosic components (shown in Figure 2) such as, cellulose, hemicellulose and lignin which contains several functional groups such as hydroxyl, amine and carbonyl. These functional groups are recognized for the effective and efficient removal of heavy metals from aqueous solution (Fan et al., 2016). However, effectiveness in adsorption is influenced by several factors such as, pH, contact time, adsorbent dosage, initial heavy metal concentration among others (Okoro et al., 2022). The adsorption characteristics of biochar derived from rice husks were investigated for the uptake of Pb(II) and Cu(II) ions from contaminated water under optimal conditions. The sorption of Pb(II) and Cu(II) ions by the biochar obtained at a pyrolysis temperature of 500°C equilibrated at 60 min using the optimum dosage of 0.250 g and optimum initial concentration of 2 mg/L. The FTIR spectra indicated the presence of O-H, -C-H, C=C, C=O, N-O, and -COO which participated in heavy metal ions adsorption (Ndekei et al., 2021). Similarly, ZiCl2 activated carbon prepared using rice husk (RH-AC) was synthesized through an innovative route of microwave and electric dual-mode heating method and tested for the adsorption of Cr(VI) ions. The RH-AC, with a high specific surface area of 1719.32 m2/g and a total pore volume of 1.05 cm3/g showed a maximum adsorption capacity for Cr(VI) of 56.82 mgg-1 at pH 3 with an equilibrium time of 90 min. The adsorption data correlated well with Langmuir adsorption and second order pseudo kinetic model. The authors suggested that the adsorption process was mostly physical and governed by surface functional groups. However, the thermodynamic studies reported low values of Gibb’s free energy of (−5.92to −8.46 kJ mol-1) indicate weak interactions raising questions on the stability of the adsorbed Cr(VI) and the regenerability potential of the adsorbent changing environmental conditions (Zhang et al., 2023).
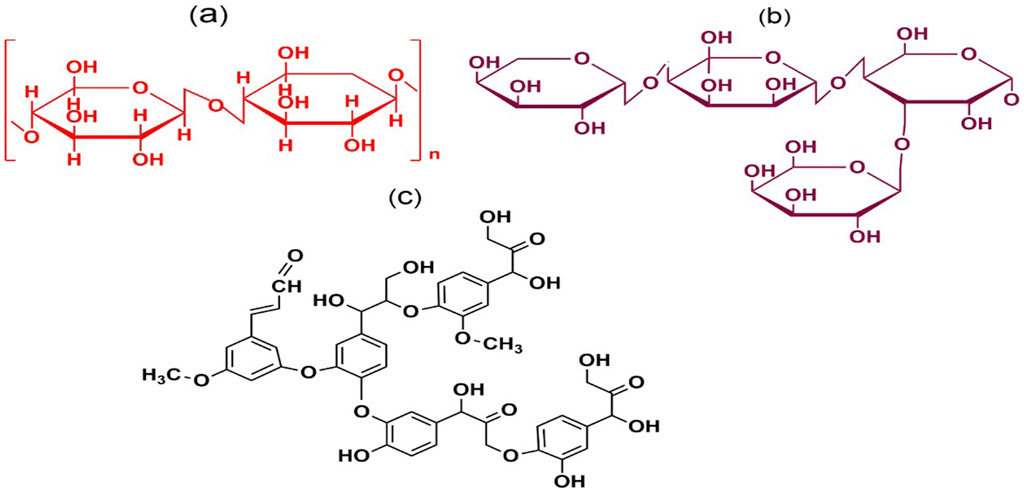
Figure 2. Chemical structures of lignocellulose components: Cellulose (A), Hemicellulose (B) and Lignin (C).
In a separate study investigators examined the efficacy of rice husks both carbonized rice husks (CRH) and activated rice husks (ARH) for the uptake of Pb(II), Cd(II), Cu(II), Zn(II) ions from industrial wastewater. The registered adsorption efficiencies for the divalent ions of Pb, Cd, Cu, Zn for CRH were 54.3%, 8.24%, 51.4% and 56.7%, respectively while those for a similar dosage of ARH were 74.04%, 43.4%, 70.08% and 77.2%, respectively (Nhapi, 2011). The study was conducted at pH 7, which is a neutral pH but industrial wastewater is often at varying pH levels thus the performance of CRH and ARH under acidic and alkaline conditions were not explored. This limits the applicability of the adsorbents in real situations involving treatment industrial wastewater where pH fluctuations commonly occur. However, according to the findings the adsorbent is can be applied in the treatment of natural water systems which are usually at neutral pH.
The reduction efficiency of rice husks is reported to be varied due to the alterations and modifications done on the rice husks (Rahman et al., 2020). For instance, results of rice husks treated with sodium carbonate solution and subsequently tested for the uptake of Cu(II) ions indicated that the activation with the carbonate significantly increased sorption of Cu(II) ions from 90% to 97% (Kumar et al., 2014). Another related study examining the capacity of rice husks for the removal of copper (II) ions through the fixed bed column indicated that the removal efficiency of expanded rice husks was better than that of raw rice husks (Luo et al., 2011). Similarly, the adsorption and recovery study of Zn(II), Cu(II) and Cd(II) metal ions onto and from rice husks suggested that the removal affinities of rice husks can be improved if the surface chemistry is modified or treated (Yanaka, 2021). Generally, studies indicate that the modification of rice husks enhances its adsorption performance.
3.2 Sugarcane bagasse and fly ash
Sugarcane bagasse and fly ash (BFA) is byproduct of sugar industry produced during the burning of sugarcane bagasse in boilers. Studies on BFA has revealed that it contains high content of silica which enhances its adsorption properties. A recent study synthesized varying zeolites from sugarcane bagasse fly ash modified with iron (III) oxide hydroxide. The resulting derived mesoporous adsorbents (powder and beads) were subsequently used for the removal of Pb(II) ions from aqueous solution. The results obtained from all the adsorbents indicated excellent removal efficiencies of Pb(II) ions from wastewater of higher than 82%. The high removal efficiency was attributed to the addition of iron (III) oxide hydroxide which helped to increase the specific surface area and reduce the pore size of the BFA. Electrostatic attraction between the negatively charged surface of the adsorbents and the Pb(II) ions played a central role in the uptake of Pb(II) ions. The adsorption patterns and mechanisms of the adsorbent were best explained by Langmuir isotherm and pseudo-second-order kinetic models. The study also indicated that the prepared adsorbent could be used for the remediation of wastewater polluted with heavy metals, however it does not provide an understanding of the regeneration potential of the adsorbent which could be important for applicability in the industrial setting (Praipipat et al., 2023). A related study examined the use of fly ash treated with alkali for the removal of Cu(II) ions from aqueous solution. The findings obtained from the study demonstrated that the NaOH treated fly ash reduced Cu(II) ions attaining maximum of 90% removal, with Langmuir calculated maximum adsorption capacities for Cu (II) ions of 30.21 mgg-1 and 16.89 mgg-1 for the fly ash that was activated by NaOH and KOH, respectively. The kinetic data agreed well with pseudo second order and the suggested adsorption mechanism of electrostatic attraction between the adsorbent and Cu (II) ions (Darmayanti et al., 2018). The study provides insight into the strategy of optimization with regards to alkali-activation of fly ash and sustainable water treatment technology. Competitive, desorption and pH variability studies were not reported, however, this could offer a better understanding in the applicability of this material in real world situations. Another fly ash prepared through microwave-incinerated sugarcane bagasse was tested for the adsorption of Zn(II) ions from aqueous solutions. The results indicated that at the optimum conditions of pH 6, and equilibrium time of 180 min the removal efficiency was 96.3%. The equilibrium data was well described by Freundlich as well as the Langmuir model with a correlation coefficient of 0.997 and 0.984, respectively (Salihi et al., 2014). The study indicated that the prepared ash could be utilized to reduce the levels of Zn(II) ions in wastewater however, for broader applicability the incorporation of real environmental situations, detailed material characterization and kinetic modelling could make the study more feasible. Pilot projects which aimed at determining the adsorption of heavy metals from wastewater using zeolitic materials obtained from sugarcane bagasse fly ash were conducted. The hydrothermally and electrically synthesized zeolites were tested for both batch and column adsorption of Pb(II) and Cd(II) ions from aqueous media with the column method showing higher adsorption efficiency compared to batch method. The results from the study indicated that the levels of Pb(II) and Cd(II) ions were reduced up to 90%. Langmuir adsorption model best described the adsorption data while the kinetic modelling followed pseudo-second-order and intra-particle diffusion model indicating that film diffusion was the rate determining reaction in the adsorption process (Shah et al., 2013). Incorporating column studies provided insight in the practical approach for these adsorbent materials. Again, carbonized fly ash followed by chemical activation was tested for the removal of Cr(VI) and Cu (II) ions from textile industry wastewater. At pH 6, contact time of 60 min using an adsorbent dose of 1 g and a concentration of 50 mg/L of metal ions, the reported maximum adsorption of Cu(II) and Cr(VI) ions of 0.9994 mg/g and 0.9325 mgg-1, respectively. The study indicated that the presence of silica and alumina in the BFA and activation with KOH greatly improved the performance of the BFA (Mulyatun, 2018). The kinetic studies and adsorption patterns were however not provided in this study. In addition the competitive studies which could have provided insight on the performance of the adsorbent in the binary system was lacking.
3.3 Wheat bran
Researchers have reportedly used wheat bran as a low cost adsorbent for the remediation of water containing heavy metals. Several studies have indicated that wheat bran, pristine or treated, can be used to reduce the levels of heavy metals such as, chromium, lead, copper, zinc, cadmium among others, in wastewater. For instance, a study was conducted by Ogata et al. (2014) to examine the effectiveness of untreated wheat bran for the removal Pb(II) and Cd(II) ions from aqueous solution. The Langmuir calculated maximum adsorption capacities were reported to be 0.667 and 1.667 mgg-1 for Cd(II) and lead (II) ions, respectively. The authors linked the adsorption process to the existence of several functional groups within the bran which significantly provided binding sites of metal ions. The adsorption data of the metal ions on the bran was described by both Langmuir and Freundlich adsorption isotherm models. The thermodynamic studies indicated that the reaction was spontaneous and that there was increased disorder in the solid-liquid interphase Furthermore, the adsorbent’s regenerability tests indicated that it could reused up to four cycles (Ogata et al., 2014). However the study reported a recovery rate of approximately 100% for Cd(II) and 56% for lead (II), the lower rate for lead (II) indicates that the optimization of desorption of lead (II) should be investigated. A similar study compared the sorption properties of untreated wheat bran (WB) and modified wheat bran (M-WB) treated with tartaric acid for the removal of Cr (VI) metal ions. The results indicated maximum removal of 51.0% and 90.0% for WB and M-WB, respectively. The adsorption data fitted best onto Freundlich isotherm model with the adsorption capacity of 4.53 mgg-1 for WB and 5.28 mgg-1 for M-WB obtained at pH two and 2.2, respectively (Kaya et al., 2014).
3.4 Fruit and vegetable waste
Osman et al. (2019) synthesized a high-surface -area activated carbon (AC) and hydrophilic carbon nanotubes (MWCNTs) derived from potato peels waste for the adsorption of Pb(II) ions. The acid and alkali co-activated AC showed a better adsorption capacity for the divalent metal ion compared to MWCNTs. The AC adsorbent attained a removal efficiency of 84% for Pb(II) ions within 1 h indicating fast adsorption kinetics. The adsorption capacities for Pb(II) ions by these adsorbents AC and MWCNTs based on the initial concentration of Pb(II) ions were 9.3 and 5.7 mgg-1, respectively (Osman et al., 2019). The adsorption process however was not analyzed using the Langmuir, Freundlich or kinetic studies which could have provided an understanding on the adsorption mechanism of these adsorbents. Fruit peels have also shown remarkable adsorption capacities for the heavy metal ions, as demonstrated by Husoon et al. (2013). Three different forms of peels (fresh, dried and powdered) were tested along with the effect of pH and adsorption efficiency for the removal Pb(II) and Cu(II) ions from industrial wastewater. The study concluded that the lemon peels were more effective than the orange peels with the powdered form of lemon and orange peels outperforming the fresh and dried pieces of these peels. The study reported biosorption removal by the lemon peel powder as 72.5% and 71.3% for Pb(II) and Cu(II) ions, respectively and the orange peel powder as 56.7% and 34.5% for Pb(II) and Cu(II) ions, respectively. These results were obtained at optimum sorption solution pH five and at a temperature of 40°C (Husoon et al., 2013). The authors did not apply the adsorption isotherm models or kinetics hence there is limited understanding of adsorption mechanism of these adsorbents.
Soybeans hulls modified with different concentrations of citric acid were compared to unmodified hulls in the adsorption of Cu(II) ions. The study showed that the citric acid introduced more carboxyl groups onto the adsorbent’s surface resulting to increased uptake of Cu(II) ions which was reported to be between 0.68 and 2.44 mmolg-1 better than that of untreated soybeans which was 0.39 mmolg-1. In addition, it was observed that the hulls’ negative charge increased as the concentrations of citric acid increased. Furthermore, the study demonstrated that pretreatment of the hulls with NaOH before citric acid treatment resulted to hulls with faster adsorption kinetics albeit with lower adsorption capacity than those not treated with NaOH (Marshall et al., 1999).
3.5 Coffee husks
Several studies have examined the efficiency of coffee husks for the removal of heavy metals from water and registered excellent results. Some of the reported investigations are presented and discussed herein. A study assessed the biosorption of Cr(VI) ions from aqueous solution using coffee husks and the result obtained indicated positive reduction of Cr(VI) ions from the simulated solution. The generated adsorption data were found to conform to both Langmuir and Freundlich isotherm models with Langmuir adsorption capacity of 44.95 mgg-1. Desorption studies indicated that the coffee husks had 60% removal efficiency. Also, the FTIR spectra revealed that the adsorption of Cr(VI) ions was enabled by the hydroxyl and carboxyl functional groups present on the husks’ surface (Ahalya et al., 2010). On the other hand, a maximum adsorption of 87% for Cr(VI) ions from aqueous solution by natural coffee husks was observed at optimum conditions. A maximum adsorption capacity of Cr (VI) onto the mesoporous biosorbent of 0.660 mgg-1 was recorded at pH 2 after 60 min using 40 mg L-1 of Cr (VI) solution with no addition of KNO3. The kinetic data followed the pseudo-second-order model (Alhogbi, 2023). The adsorbent also revealed recyclability potential of up to three adsorption-desorption cycles, although this dropped drastically thereafter raising concerns on the stability of material. In addition, the reported adsorption capacity of 0.660 mgg-1 is relatively low compared to other bio-sorbents such as activated carbon. Surface modification through activation could improve the pore structure of material hence its adsorption capability. Similarly, the adsorption capability for Ni (II) ions by nitric acid activated coffee husks carbonized at 400°C for 30 min was seen to be better than those that were not acid activated. The study indicated that acid activation increased the adsorbent’s capacity for Ni (II) ions by tenfold. The reported maximum adsorption capacities were 21.14 mg g-1 and 1.97 mgg-1 for the acid treated and untreated activated carbon, respectively. The data obtained from the adsorption processes fitted well onto Langmuir isotherm model indicating that the adsorption process was homogeneous surface (Tien, 2018).
In another independent study, coffee husks were used for the removal of Zn(II) and Ni(II) metal ions from the wastewater. The results obtained from this study revealed that the husks are capable of reducing the concentrations of the studied metal ions from wastewater. The batch adsorption studies recorded Langmuir adsorption capacity of the husks for Zn2+ and Ni2+ ions as 12.97 and 11.11 mgg-1, respectively. The experimental data followed pseudo-second-order kinetic model suggesting that chemisorption was the rate determining step in the adsorption process (Alhogbi and Al-Enazi, 2018). A similar study tested the ability of activated carbon derived from coffee husk for the adsorption of the Cr (VI) from electroplating wastewater. The adsorption process optimization revealed 99.65% maximum Cr(VI) adsorption efficiency at 120 min contact time, 40 mgL-1 initial concentration, 150 rpm agitation speed, pH 7.0, and 20 gL-1 adsorbent dosages. The adsorption data fitted well onto Freundlich isotherm model and pseudo-second-order kinetic model. Hydroxyl groups, carboxyl groups and other functional groups on the structure of the adsorbent as confirmed by FTIR facilitated the uptake of Cr(VI) ions from the wastewater (Aragaw et al., 2022).
The extensive list of biosorbents including rice husks, wheat bran, fruit waste, coffee husks, sugarcane bagasse, among others show good sorption capacities for Cd(II), Cu(II), Pb(II), Zn(II), Ni(II) and Cr(VI). The biosorption of heavy metal ions from aqueous solutions is therefore a promising method for sequestering heavy metal ions from wastewater. Generally, these studies indicate that these biosorbents, especially in modified form, can be effectively applied for the removal of heavy metal ions from wastewater thus achieving waste valorization strategy. Surface modification enhances adsorption of rice husks. Rice husks activated by sodium carbonate increases sorption of Cu(II) from 90% to 97%, similarly ARH exhibited an adsorption efficiency of 74% for Pb (II) ions compared to CRH (54%). The high silica content, lignin and functional groups (carboxyl and hydroxyl groups) facilitated the uptake of metal ions by rice husks. The adsorption data of the metal ions on the rice husks-based adsorbents was described by Langmuir adsorption isotherm models. The rate determining reaction for the rice husks based sorbents was pseudo-second-order reaction indicating chemisorption adsorption process.
The presence of high silica and alumina content in sugarcane bagasse and fly ash (BFA) greatly contributes to its adsorption capability. In addition, modification of the sugarcane bagasse with the iron (III) hydroxide exhibited excellent adsorption efficiency (>82%) for Pb(II). Similarly alkali activation of BFA enhances the adsorption efficiency of BFA. However, while the adsorption efficiency was >90% in some studies the adsorption capacity for the heavy metal ions was relatively low. This implies that the adsorbent may not be applicable for treatment of industrial wastewater or mine effluents. The adsorption patterns for BFA mostly followed Langmuir adsorption isotherm indicating that the adsorption was mainly monolayer. Pseudo-second-order reaction rate was the rate determining describing the adsorption of heavy metal ions onto BFA indicating that the adsorption process was chemical in nature. While studies on the variation on adsorption conditions such as pH, adsorbent dosage and initial metal ion concentration were provided, most studies did not report on competitive studies and reusability of the biosobents which could provide an understanding on the scalability of the materials for wastewater treatment.
The hydroxyl and carboxyl groups on the lignocellulosic structure of the wheat bran play a central role for the uptake of metal ions from aqueous media. However, wheat bran exhibited moderate adsorption capacity and the studies indicate that without modification the adsorption capability of bran is limited. The adsorption data for the uptake of the metal ions on wheat bran could be described by both Langmuir and Freundlich adsorption isotherm models indicating that the adsorption process is either on homogenous or homogenous surfaces. The studies also indicate that reusability of wheat bran is possible despite there being a lower rate of uptake for Pb(II) ions after desorption. Similarly, coffee husks are rich with hydroxyl and carboxyl groups which provide binding sites for heavy metal ions. Studies also indicate a reasonable adsorption capacity for Cr(VI) ions by the coffee husks. However, coffee husks exhibited low stability towards multiple adsorption-desorption cycles. The experimental data for adsorption of heavy metal ions by coffee husks followed pseudo-second-order kinetic model suggesting that chemisorption was the rate determining step in the adsorption process. The adsorption data fitted well with both Langmuir and Freundlich adsorption isotherm models suggestion that the adsorption can either be monolayer or multilayer.
Studies indicated that chemical treatment enhanced adsorption efficiency of fruits and vegetables for heavy metal ions. Cellulose, pectin and lignin components in fruits and vegetables provide diverse binding sites for the heavy metals. Lemon peels, particularly, the powdered form exhibited a good adsorption capability for Pb (II) and Cu(II) indicating that they are suitable for wastewater treatment.
3.6 Biosorption mechanisms
The removal dynamics of metal ions from aqueous media onto agricultural and food waste-based adsorbents is governed by metal biosorption (S. Xie, 2024). Metal biosorption is a relatively complex process influenced by multiple factors. Mechanisms involved in the biosorption process include chemisorption, complexation, adsorption–complexation on the surface and pores, ion exchange, microprecipitation, chelation, heavy metal hydroxide condensation onto the biosurface. In addition, the adsorbate and adsorbent interaction can be via surface adsorption through physical forces, entrapment in inter- and intrafibrillar capillaries, and spaces of the structural polysaccharides network as a result of the concentration gradient and diffusion through cell wall and membrane (Basso et al., 2002; Sud et al., 2008). Among the functional groups present in food waste molecules are acetamido groups, carbonyl, phenolic, structural polysaccharides, amido, amino, sulphydryl, carboxyl groups, alcohols and esters. These groups have the affinity for metal complexation. The interactions between various functional groups and their complexation with heavy metals during biosorption process can be diagnosed using spectroscopic techniques. The adsorption mechanisms and affinities of the biosorbents for metal ions can be metal-specific or non-selective (Aziz et al., 2023; Vardhan et al., 2019).
4 Chitin and chitosan
Chitin is the world’s second most abundant naturally occurring polysaccharide. Much of it is disposed of as waste from sea food crustacean, mainly in shrimps, prawns, crabs, and lobsters. It occurs as a significant structural component of the shells/exoskeletons of crustaceans, the cuticles of insects, and the cell walls of fungi and yeast. It consists of N-acetyl glucosamine units linked by β (1→4) bonds. Chitin carries one linear amino group per glucose ring, thus making electron pairs available for coordination (Sofiane and Sofia, 2015).
Chitosan is a partially N-deacetylated product of chitin, deacetylation process produces a chain of amino groups along the chitosan structure and is an important natural biopolymer due to its biocompatibility and biodegradability, with broad applications in wastewater treatment, chemical, biomedical and pharmaceutical applications, agriculture and biotechnology. Its high nitrogen atom contents and hydroxyl groups allows uptake of several metal ions through various mechanisms such as chelation, electrostatic attraction or ion-exchange (Sofiane and Sofia, 2015). Its chelating properties are attributed to the amino and hydroxyl groups that can act as chelation sites for different targets such as metals. The high adsorption potential of chitosan for heavy metals can be attributed to; (i) high hydrophilicity associated with the large number of hydroxyl groups of glucose units, (ii) large number of functional groups, (iii) high chemical reactivity of these groups, and, (iv) flexible structure of the polymer chain (Bai et al., 2022).
However, chitosan has some demerits such as poor mechanical strength, limited surface area, low water resistance, high solubility in acidic media, high tendency towards agglomeration and incomplete regeneration after adsorption (Eltaweil et al., 2021). To overcome these setbacks, several modifications including amination, carboxymethylation, and the formation of hybrid adsorbents through crosslinking other materials have been conducted in order to enhance the adsorptive capabilities and selectivity of chitosan towards the removal of from wastewater (Marrakchi et al., 2020).
The difference between chitin and chitosan lies in the degree of deacetylation, usually defined as the number of glucosamine groups to the total number of N-acetylglucosamine and glucosamine groups (Figure 3). This parameter is the most important description of chitosan and chitin properties. The quality and characteristics of chitosan products, such as purity, viscosity, molecular weight, polymorphic structure and the degree of deacetylation may differ due to many factors in the production process, thereby affecting the nature of the final product (Radomski et al., 2014).
Due to its widespread abundance, its chemical and physical versatility, and the problems of its disposal as a waste material, a wide range of value-added applications of chitin and chitosan is being initiated, investigated and developed. One of the significant developments in the new range of applications is the study of the ability of chitosan, as a potentially major environmental treatment material, to remove metal ions from industrial wastewaters and leachates (Gerente et al., 2007). Due to its large abundance, literature is replete with studies of chitin and chitosan as adsorbents for metal ions removal from water.
4.1 Removal of heavy metals onto chitin and chitosan
Sofia and Sofiane (2015) investigated the usefulness of chitin and chitosan produced from shrimp carapaces for the removal of Zn2+, Cd2+ and Cu2+. The kinetic data and equilibrium sorption isotherms were measured in batch conditions. The influences of different experimental parameters such as contact time, initial concentration of the metal, chitin mass, initial pH of solution and temperature on the kinetics of the metal removal were studied. The process was found to follow pseudo second-order kinetics. Results also indicated that the Langmuir model gave a better fit to the experimental data than the Freundlich equation. The biosorption of the metals studied by chitin and the chitosan varied in the order: Cu (ІІ) > Cd (ІІ) > Zn (ІІ) and the quantities biosorbed in equilibrium by the chitosan were higher than that of chitin (Sofiane and Sofia, 2015).
Abdel-Mohdy et al. (2005) carried out a study on radiation grafting of diethylaminoethyl methacrylate (DEAEMA) on chitosan to impart its ion exchange properties for the sorption of metal ions from wastewater. The effect of experimental conditions such as monomer concentration and the radiation dose on grafting were studied. On using chitosan, grafted chitosan and chitosan composites of silica and titania in removing some metal cations, a high up-take capacity for Cu2+ and lower uptake capacities for Zn2+ and Co2+ metal ions in both unary and multicomponent systems was observed. The high selectivity for Cu2+ions was attributed to the fact that Cu2+ cations form more stable complexes that the Zn2+ and Co2+ cations. The study also indicated that the adsorptive capability of chitosan increased with increase in the monomer concentration. Similarly adsorptive capacity of the composite increased as the oxide content increased but only up to 50% (Abdel-Mohdy et al., 2005). Ráheľ et al. (2008) investigated the removal of copper metal ions from aqueous solutions by plasma made chitosan filter. When used for the water decontamination in its natural flake form, chitosan suffers from gelling and associated hydrodynamic flow problems. Moreover the specific binding sites are not readily available for sorption in the natural form. The use of filter fabric was favored by its large surface area, excellent control on flow transfer characteristics and the low-cost. Chitosan coated samples were immersed into 100 mL Cu2+ solutions with the concentration ranging from 0.01 M to 2–10−4 M. The adsorption capacity of chitosan coated on fabric was found to be at least 40% higher than its natural flake form. The adsorption behavior of chitosan filter was also well described by the Langmuir adsorption isotherm. The theoretical adsorption capacity of chitosan Cu2+ determined at pH 5.5 from the Langmuir isotherm and it was found to be 313 ± 5 mgg−1 (Ráheľ et al., 2008).
Equilibrium isotherms and the adsorption kinetics of the heavy metals copper, zinc, mercury, and arsenic on chitosan produced from shrimp shells at a laboratory level onto chitosan were studied experimentally by Benavente (2008). The results showed that the adsorption capacity depended strongly on pH and on the species of metallic ions in the solution. The optimum pH value for the metallic cation adsorption was between 4 and 6, whereas for arsenic adsorption it was about 3. It was also found that the Langmuir equation described very well the experimental adsorption data for each metallic ion. The adsorption capacity for the metals on chitosan was found to follow the sequence Hg > Cu > Zn > As. The analysis of kinetic models showed that the pseudo-second-order adsorption mechanism was predominant, and the overall rate of the metallic ion adsorption process was therefore controlled by adsorption reactions and not by mass transfer for the range of particle sizes examined in the study. The ability of chitosan to remove arsenic from natural water, and copper and zinc from mining waste water was verified (Benavente, 2008). Muhaemin and coworkers was carried a study to determine the chelating ability of crab shell particles and extracted acetamido groups (chitin and chitosan) from portunus sp to lead (Pb2+). The crab shell was powdered, sieved, and added with lead (II) in various pH values. The result showed that the removal efficiency of lead with crab shell depended on pH value, but it was less sensitive than that of the control without crab shell. Biosorption of chitin and chitosan showed that both materials adsorb with the best capacity at pH 4.0. Chitosan had a higher sorption than chitin for all treatments. The removal efficiency in the solution of 0.5 g crab shell was about 22%. It indicated that the acetamido group (chitin and chitosan) acts as a non-specific chelating agent and establishes weak hydrogen bonds with lead (II) ions in the solution (Muhaemin, 2005).
(Edokpayi et al., 2015) studied the synthesis and characterization of biopolymeric chitosan derived from land snail shells and its potential for Pb2+ removal from aqueous solution. The experiments were conducted in the range of 1–50 mg/L initial Pb2+ concentrations at 298 K. The effects of pH, adsorbent dosage and contact time on the adsorptive property of the adsorbent were investigated and optimized. The Langmuir model and pseudo second order kinetic model suitably described the adsorption and kinetics of the process, respectively. From the results obtained, it was concluded that synthesized biopolymers from land snail shells had the potential for an average of 99% removal of Pb2+ from aqueous solutions (Edokpayi et al., 2015).
Radomski et al. (2014) carried out a study on the application of chitosan and its modified derivatives for removal of heavy metal ions from industrial wastes. Application of chitosan/aspartic acid copolymer cross-linked with ethylene glycol moderated the sorption processes in comparison with pure chitosan. The rate of removal of lead and mercury was found to be above 80%, while the elimination of zinc and iron ions from the solution was negligible as compared with unmodified chitosan (Radomski et al., 2014). A composite of chitosan-g-poly (acrylic acid-co-acrylamide) was investigated for the adsorption of heavy metal cations: Cu(II), Cd(II), Ni(II), and Pb(II) from aqueous solution. The effect of experimental parameters, such as pH, treatment time, temperature, adsorbent dose and initial metal ion concentration on the removal of metal ions were also studied. The optimum pH corresponding to the maximum adsorption of Cu(II), Cd(II), Ni(II), and Pb(II) removal was pH 5-6 and the metal ions were adsorbed onto the adsorbents very rapidly within the first 120 min. This low-cost adsorbent was seen to be effective for the removal of metal ions from aqueous solutions and showed the following order of % extraction: Cu2+>Cd2+>Ni2+>Pb2+ (Khairkar and Raut, 2014).
Hadi (2013) carried out a research on the synthesis of chitosan from fish shells and its use in Cu(II) ions removal. Synthesis of chitosan involved four main stages: preconditioning, demineralization, deprotenisation and deacetylation. Adsorption of copper ions by chitosan was investigated under different conditions. The amount of copper adsorbed after adding 50 mg of chitosan at an operating temperature of 30°C and pH of 5.7 was above 85% (Hadi, 2013).
A modified form of chitosan beads, namely, protonated chitosan beads (PCB), carboxylated chitosan beads (CCB) and grafted chitosan beads (GCB) were investigated for the removal of Cr(VI) ions from aqueous solution. Batch adsorption studies were conducted to optimize various equilibrating conditions such as the contact time and pH. The beads were observed to possess enhanced Cr(VI) sorption capacities of 3,239, 3,647 and 4,057 mg/kg, respectively than the raw chitosan beads (CB) which possess the sorption capacity of 1,298 mg/kg with a minimum contact time of 10 min. The adsorption characteristics of the adsorbents was described by the Freundlich and Langmuir isotherm models and the interaction between the Cr(VI) metal ions and the modified beads were governed by electrostatic attraction and complexation (Kousalya et al., 2010). Modified chitosan obtained by demineralization, deproteinization, decolorization and deacetylation of waste shrimp shell was studied for the removal of chromium, arsenic, nickel, and cobalt heavy metal ions from wastewater. The results indicated that the highest adsorption of obtained of arsenic, nickel, and cobalt, was 98.50, 74.50, and 47.82%, respectively, at neutral pH, whereas the highest attained adsorption of chromium was 97.40% at pH 3. The calculated maximum adsorption capacities of the chitosan for chromium, arsenic, nickel, and cobalt ions were reported as 15.92, 20.37, 7.00, and 6.27 mgg-1, respectively (Rahman et al., 2023).
Unagolla and Adikary (2015) investigated the adsorption characteristics of Cd(II) and Pb(II) heavy metals ions onto locally synthesized chitosan biopolymer. Kinetic studies were conducted for batch systems using different pH values of initial metal ion solution and two different degrees of deacetylation (DD) values of chitosan. Simplified models such as pseudo-first-order, pseudo-second-order, and intra-particle diffusion equations were used to determine the rate controlling step. A strong dependence of the adsorption capacity on pH and DD was observed and the sorption capacity was reported to increase as pH and DD values increased. Significantly high rate of adsorption was observed in the chitosan which has a higher DD value. According to the study, chitosan is a good adsorbent in the removal of heavy metals from wastewater (Unagolla and Adikary, 2015).
The adsorption of Cr6+ and Pb2+ from simulated wastewater onto chitosan grafted cocoa husk char was determined by Aderonke et al. (2014). The study optimized metal ion adsorbent dosage, agitation time and adsorbent particle size for the removal of the metals from simulated wastewater and the removal efficiencies were calculated. The equilibrium experimental data results of Pb2+ fitted well to Langmuir and Freundlich adsorption isotherm models. The study concluded that cocoa husks, an agricultural waste material, has potential as an adsorbent to remove toxic heavy metal like chromium and lead from industrial wastewater. Modification of this waste with chitosan also enhances its performance in the removal of the metal ions (Aderonke et al., 2014).
Sobhanardakani et al. (2014) studied the efficiency of chitosan for the removal of Pb(II), Fe (II) and Cu(II) ions from aqueous solutions. The results showed that the adsorption of Pb (II), Fe (II) and Cu(II) ions on chitosan strongly depended on pH. The removal process followed the Langmuir isotherm and maximum adsorption capacity for the adsorption of Pb(II), Fe(II) and Cu(II) ions by the chitosan were 55.5, 71.4 and 59 mgg−1, respectively, under equilibrium conditions of 25ºC ± 1°C. The adsorption process was found to be well described by the pseudo second-order rate model (Sobhanardakani et al., 2014).
Mohanty (2015) studied the removal of hexavalent chromium using chitosan prepared from shrimp and crab shells. The study concluded that as the dose of the adsorbent was increased keeping the time and speed of the shaker (rpm) constant, more percentage of Cr6+was removed. For the case of crab chitosan, 80.91% maximum removal of Cr6+ was obtained whereas 73.89% maximum removal was observed for the case of shrimp chitosan. The study also concluded that keeping the amount of adsorbent and speed of the shaker (rpm) constant, with increased time of contact, the concentration of Cr6+ solution decreased. But after a lapse of about 5–6 h, no further change in concentration was observed. The percentage of removal of Cr6+ for the case of crab chitosan and shrimp chitosan were 64.29% and 80.11%, respectively when studying the effect of contact time on the adsorption process. The obtained results showed that the readily available chitosan, was an economical adsorbent and was found suitable for removing Pb(II), Fe(II) and Cu(II) ions from aqueous solutions (Mohanty, 2015).
Three chitosan derivatives cross-linked and grafted with amido- or carboxyl groups using acrylamide and acrylic acid were prepared and investigated for the adsorption of Cu(II) and Cr(IV) ions. The acrylamide grafted chitosan adsorbent attained a maximum adsorption of 318 mgg-1 for Cu(II) at pH 6 while the acrylic acid chitosan derivative presented a highest adsorption 935 mgg-1 for Cr(IV) ions at pH 3. The kinetic studies followed the diffusion-reaction model (DIFRE) which indicates that there were several interactions between the metals ions and the adsorbent, namely,; diffusion of the ions through the polymer, mass transfer of the ions from the bulk solution, and chelation or electrostatic attraction on the amino groups of the polymer. Regeneration studies indicated that the adsorbent retains its adsorption efficiency after four adsorption-desorption cycles (Kyzas et al., 2009). Chitin was studied for the removal of Cd(II) ions from aqueous solutions through batch experiments. Among the adsorption parameters examined, initial concentration of cadmium ions, particle size and adsorbent dosage were found to play a central role in the removal of the Cd(II)ions. The maximum adsorption capacity for the chitin for Cd(II) ions was 114 mgg-1. The adsorption data followed Langmuir adsorption isotherm model and kinetic data was fitted well with pseudo-second-order kinetic model (Benguella and Benaissa, 2002).
Dried fungi biomass (Rhizopus oryzae) was compared to commercially available chitosan and chitosan cross-linked with benzoquinone for the adsorption of copper, zinc, arsenic and chromium ions from simulated water. The uptake of the heavy metals by chitosan was found to be dependent on the initial concentration. At pH 4, adsorbent/solution ratio (1 g/L), and initial metal ion concentration of 400 mg/L the highest uptake values were reported to be 137, 108, 58, and 124 mgg-1, for copper, zinc, arsenic and chromium ions, respectively. However, the adsorptive capacity of the chitosan for the metal ions decreased in copper/zinc and gold mine simulated effluents. At the same time, both the R. aryzae and benzoquinone cross-linked chitosan were reported to be stable at low pH compared to chitosan albeit with lower adsorption capacity (Mcafee et al., 2001).
An investigation on the adsorptive characteristics of chitosan coated with polyvinyl chloride (PVC) for the uptake of Cu(II) and Ni(II) ions from aqueous solution revealed Langmuir maximum adsorption capacity of the adsorbent was 87.9 and 120.5 mgg-1 for Cu(II) and Ni(II) ions, respectively (Popuri et al., 2009). Optimization of Cr(VI) adsorption using Response Surface Methodology by chitosan flakes against adsorption conditions (pH, adsorbent dose, initial metal ion concentration) were conducted by (Aydın and Aksoy, 2009). The maximum adsorption capacity achieved by the flakes for the Cr(VI) was 22.09 mgg-1 at pH 3, initial concentration of 30 mg/L and adsorbent dosage of 13 g/L. The adsorption data followed Langmuir adsorption isotherm. The kinetic studies fitted well with pseudo-second-order and intra-particle diffusion models. Alumina coated chitosan composite was studied for both batch and column adsorption of Cr(VI) from synthetic water and wastewater sourced from a chrome plating plant. The highest adsorption capacity attained by the composite as calculated from the Langmuir model 153.85 mgg-1, which was according the authors the remarkably great compared to those reported in literature. Furthermore, the study indicated that the adsorbent was reusable and could be regenerated using sodium hydroxide solution (Boddu et al., 2003).
In order to prevent the solubility of chitosan in acidic medium Rojas and coworkers synthesized a cross-linked chitosan. The chitosan attained a maximum uptake for Cr(VI) ions at pH 4 of 215 mgg-1. This was attributed to the Cr(VI) ions interaction with the highly protonated active sites of chitosan at low pH. The adsorption followed both Langmuir and Freundlich adsorption isotherm models (Rojas et al., 2005). In another study, amination of chitosan was reported to remarkably enhance its adsorptive capability for Ni(II) and Cr(VI) ions. The adsorbent attained an adsorption capacity of 30.2 and 28.7 mgg-1 for Ni(II) and Cr(VI) ions, respectively at an initial concentration of 1,000 mg/L. The authors indicated that aminated chitosan was suitable for the removal of both cations and anions from aqueous solutions (Yan et al., 2007). Table 1 is a list of chitin and chitosan based adsorbents and their adsorption capacities for various metal ions.
The modified chitosan showed many advantages over flaked or powdered chitosan, this is because modification results to enhanced higher internal surface area, and reduced solubility in low-pH solutions, thus proving their suitability over a broad pH range. Chitosan exhibited exceptional adsorption capacities for the heavy metals (Cu(II) ions 313 mgg-1) and was effective in the adsorption of multiple heavy metal ions (anion and cations). However, pristine chitosan is soluble in acidic media it therefore requires crosslinking to attain chemical stability. Metal ions uptake by chitosan and chitosan composites was mainly aided by the high amino and hydroxyl groups of the chitosan skeleton and functionalized or grafted carboxyl groups. The adsorption data mainly followed Langmuir and Freundlich adsorption isotherm models. The adsorption process was found to be well described by the pseudo second-order rate model an indication that the reaction involves the chemical interaction of the adsorbent and the metal ions.
5 Limitations and future prospects associated with food waste-based adsorbents
The utilization of the food waste-based adsorbents present a new chapter towards remediation of wastewater polluted with heavy metals since they are available at low cost and their usage provides a prospect to resolve environmental issues associated with their disposal. There are several limitations that hinder the application of food waste-based adsorbents in the removal of heavy metals ions from water and wastewater despite their numerous benefits. Various researchers have reported limitations associated with usage of food waste-based adsorbents for remediation of wastewater polluted with heavy metal ions and some of these limitations are presented and discussed.
Food waste-based adsorbents are reported to possess different sorption capacities at different operating conditions for different metal ions. Research has indicated that the sorption capacity of banana peels, watermelon peels and grape waste revealed varying sorption for multi-element solutions on dependence of operating parameters such as, solution pH and the adsorbent dosage (Massimi et al., 2018). This can adversely compromise the wastewater treatment process, the fact that the effectiveness of the specific food waste-based adsorbent may not be dependable across the various environmental conditions or the kinds of metal ions present. Again, the existence of competing of metal ions in the wastewater can greatly decrease the adsorption efficiencies of the food waste materials are reported by other investigators (Shafiq et al., 2018).
Furthermore, in the physical and chemical properties of the food waste materials is another limitation which can also affect their adsorption performance. The adsorption efficacies of the adsorbent is greatly influenced by the structure and surface area. Several studies have indicated that surface modification of the food waste-based adsorbent materials with various chemical reagents such as, acids and bases through activation can significantly increase the performance of the adsorbent materials. This suggests that the characteristic properties of raw food waste materials generally inhibit their adsorption capacities (Belete, 2017). Further, the occurrence of organic matter and contaminants in the food waste materials can affect adsorption process, resulting in the lower removal efficiencies of the heavy metals from wastewater (Sulyman et al., 2017).
Regeneration and reusability of food waste-based materials adsorbent is also indicated to be another limitation. Multiple studies have reported that the adsorption abilities of food waste materials loses affinities after numerous usage, which raises worries on sustainability of food waste-based adsorbents (Samra et al., 2014; Coltre et al., 2020). Moreover, during the regeneration studies adsorbed metal ion are capable of undergoing leaching resulting to secondary pollution thereby counteracting the privileges of using food waste-based adsorbents as eco-friendly option (Chai et al., 2021; Jean Claude et al., 2022). Again the disposal of used food waste-based adsorbents can result into environmental issues especially if not appropriately handled (Singh et al., 2019).
Economic factors further affect the adsorption process of food waste-based adsorbents. Despite the fact that food waste adsorbent materials are considered low cost compared with conventional adsorbents materials, the cost connected with their collection, processing and treatment of food waste materials are excessive (Sen, 2023). Again, food waste materials require pre-treatment to increase their adsorption capacities, this may result into higher operational costs thus limiting their large scale application (Ooishi et al., 2011). This implies that the application of the food waste-base adsorbents require appropriate balancing of the costs involved against the privileges of reduced environmental impact.
Finally, there’s also need to observe regulatory and safety concerns when considering the food waste-based adsorbents for the heavy metal removal. The presence of pathogens and hazardous chemicals present in food waste materials raises their safety concerns in water treatment applications (Zheng et al., 2010; Shafiq et al., 2018). Additionally, regulatory bodies may not accommodate the use of food waste-based adsorbents for the removal of heavy metals, suggesting further research and authentication to ensure compliance with health and safety standards (Belete, 2017; Singh et al., 2019). The existence of regulatory uncertainty can prevent the implementation of food waste-based adsorbents in commercial applications, regardless of their potential advantages.
Despite the afore mentioned limitations, the food waste based-adsorbents are considered to be cost effective and they poses low negative impact on the environment and humans compared to other adsorbents such as metal oxides. Thermal treatments of food waste materials through processes such as pyrolysis and hydrothermal carbonization have been employed in the preparation of these adsorbents to ensure complete elimination of pathogens. In addition, the integration of energy recovery systems during thermal treatment mitigates against high energy consumption thus cutting down on the production costs. Some countries have instituted regulations governing the use of biomass based adsorbents for wastewater treatment. For instance, the European Biochar Certificate provides a framework that addresses aspects of sustainable biochar production such as the selection of feedstock, pyrolysis procedures, environmental impacts and certification standards.
6 Conclusion
The present study aimed at examining the application of food waste-based adsorbents for the remediation of water resources polluted with heavy metal ions. Food waste materials are discarded without further use leading to environmental and ecosystem concerns. These adsorbent materials are indicated to possess great surface area, excellent chemical composition and functional groups that have proved to be suitable for the removal of heavy metal ions. Food waste derived adsorbents exhibit several advantages including, abundance and availability, cost-effectiveness, environmental sustainability and efficiency in heavy metal removal. Adsorbents such as chitosan, rice husks and sugarcane bagasse have exceptionally high sorption capacities for heavy metal ions. The most improved by chemical modification are rice husks, wheat bran and coffee husks. However, the best overall in efficiency and selectivity is chitosan and chitosan composites. Bone char offer natural adsorption without modification but the cost of production limits its availability.
The drawbacks of food waste-based adsorbents include low selectivity relative to activated carbons, high cost of chemical treatment or modification requirements to enhance their porosity structures, surface areas and affinities for heavy metal ions, regeneration and reusability and low relative efficiency in multicomponent systems. Further, the disposal of the used adsorbents can lead to serious environmental pollution if not inappropriately managed that might over surpass the use of food waste adsorbents as ecofriendly. Finally, there is also other possibility that if food adsorbent materials contain pathogens and hazardous chemical substance can raise safety concerns when used in water treatment but thermal treatment of food waste materials is a promising pathway for eliminating pathogens in the preparation of food waste-based adsorbents. Life-cycle assessment (LCA) studies are required for a comprehensive understanding on the potential of food wastes as adsorbents for heavy metal removal from water.
Author contributions
WN: Writing – original draft, Writing – review and editing. JN: Writing – original draft, Writing – review and editing. VS: Writing – original draft.
Funding
The author(s) declare that no financial support was received for the research and/or publication of this article.
Conflict of interest
The authors declare that the research was conducted in the absence of any commercial or financial relationships that could be construed as a potential conflict of interest.
Generative AI statement
The author(s) declare that no Generative AI was used in the creation of this manuscript.
Publisher’s note
All claims expressed in this article are solely those of the authors and do not necessarily represent those of their affiliated organizations, or those of the publisher, the editors and the reviewers. Any product that may be evaluated in this article, or claim that may be made by its manufacturer, is not guaranteed or endorsed by the publisher.
References
Abdel-Mohdy, F., EL-Sawy, S., and Ibrahim, M. (2005). Heavy metal removal by chitosan and chitosan composite.
Aderonke, A. O., Abimbola, B. A., Ifeanyi, E., Omotayo, S. A., Oluwagbemiga, S. A., and Oladotun, W. M. (2014). Adsorption of heavy metal ions onto chitosan grafted cocoa husk char. Afr. J. Pure Appl. Chem. 8 (10), 147–161. doi:10.5897/AJPAC2014.0591
Ahalya, N., Kanamadi, R. D., and Ramachandra, T. V. (2010). Removal of hexavalent chromium using coffee husk. Int. J. Environ. Pollut. 43 (1/2/3), 106. doi:10.1504/IJEP.2010.035917
Alhogbi, B. G. (2023). Applicability of natural coffee husk as a mesoporous adsorbent for removal of chromium (VI) from aquatic environments. Mod. Appl. Sci. 17 (2), 37. doi:10.5539/mas.v17n2p37
Alhogbi, B. G., and Al-Enazi, Z. F. (2018). Retention profile of Zn2+ and Ni2+ ions from wastewater onto coffee husk: kinetics and thermodynamic study. J. Encapsulation Adsorpt. Sci. 08 (01), 1–17. doi:10.4236/jeas.2018.81001
Amicarelli, V., Lagioia, G., and Bux, C. (2021). Global warming potential of food waste through the life cycle assessment: an analytical review. Environ. Impact Assess. Rev. 91, 106677. doi:10.1016/j.eiar.2021.106677
Amicarelli, V., and Bux, C. (2021). Food waste measurement toward a fair, healthy and environmental-friendly food system: a critical review. Br. Food J. 123, 2907–2935. doi:10.1108/bfj-07-2020-0658
Aragaw, T., Leta, S., Alayu, E., and Mekonnen, A. (2022). Chromium removal from electroplating wastewater using activated coffee husk carbon. Adsorpt. Sci. and Technol. 2022, 7646593. doi:10.1155/2022/7646593
Aydın, Y. A., and Aksoy, N. D. (2009). Adsorption of chromium on chitosan: optimization, kinetics and thermodynamics. Chem. Eng. J. 151 (1–3), 188–194. doi:10.1016/j.cej.2009.02.010
Aziz, K. H. H., Mustafa, F. S., Omer, K. M., Hama, S., Hamarawf, R. F., and Rahman, K. O. (2023). Heavy metal pollution in the aquatic environment: efficient and low-cost removal approaches to eliminate their toxicity: a review. RSC Adv. 13, 17595–17610. doi:10.1039/d3ra00723e
Bai, L., Liu, L., Esquivel, M., Tardy, B. L., Huan, S., Niu, X., et al. (2022). Nanochitin: chemistry, structure, assembly, and applications. Chem. Rev. 122 (13), 11604–11674. doi:10.1021/acs.chemrev.2c00125
Barrett, E. B., Brown, J. M., and Oleck, S. M. (1951). Some granular carbonaceous adsorbents for sugar refining—a study of bone char replacements based on hydroxyapatite. Industrial and Eng. Chem. 43 (3), 639–654. doi:10.1021/ie50495a026
Basso, M. C., Cerrella, E. G., and Cukierman, A. L. (2002). Lignocellulosic materials as potential biosorbents of trace toxic metals from wastewater. Industrial and Eng. Chem. Res. 41 (15), 3580–3585. doi:10.1021/ie020023h
Belete, G. (2017). A review on elimination of heavy metals from wastewater using agricultural wastes as adsorbents. Sci. J. Anal. Chem. 5, 72–75. doi:10.11648/j.sjac.20170505.12
Benavente, M. (2008). “Adsorption of Metallic ions onto chitosan: Equilibrium and kinetic studies. Kemiteknik, Chemical and Engineering and Technology,” in Kungliga tekniska högskolan.
Benguella, B., and Benaissa, H. (2002). Cadmium removal from aqueous solutions by chitin: kinetic and equilibrium studies. Water Res. 36 (10), 2463–2474. doi:10.1016/S0043-1354(01)00459-6
Boddu, V. M., Abburi, K., Talbott, J. L., and Smith, E. D. (2003). Removal of hexavalent chromium from wastewater using a new composite chitosan biosorbent. Environ. Sci. and Technol. 37 (19), 4449–4456. doi:10.1021/es021013a
Chai, W. S., Cheun, J. Y., Kumar, P. S., Mubashir, M., Majeed, Z., Banat, F., et al. (2021). A review on conventional and novel materials towards heavy metal adsorption in wastewater treatment application. J. Clean. Prod. 296, 126589. doi:10.1016/j.jclepro.2021.126589
Cheung, C. W., Chan, C. K., Porter, J. F., and McKay, G. (2001). Combined diffusion model for the sorption of cadmium, copper, and zinc ions onto bone char. Environ. Sci. and Technol. 35 (7), 1511–1522. doi:10.1021/es0012725
Cheung, C. W., Porter, J. F., and Mckay, G. (2001). Sorption kinetic analysis for the removal of cadmium ions from effluents using bone char. Water Res. 35 (3), 605–612. doi:10.1016/S0043-1354(00)00306-7
Cheung, C. W., Porter, J. F., and Mckay, G. (2002). Removal of Cu(II) and Zn(II) ions by sorption onto bone char using batch agitation. Langmuir 18, 650–656. doi:10.1021/la010706m
Choy, K. K. H., and McKay, G. (2005). Sorption of cadmium, copper, and zinc ions onto bone char using Crank diffusion model. Chemosphere 60 (8), 1141–1150. doi:10.1016/j.chemosphere.2004.12.041
Coltre, D. S. D. C., Cionek, C. A., Meneguin, J. G., Maeda, C. H., Braga, M. U. C., DE Araújo, A. C., et al. (2020). Study of dye desorption mechanism of bone char utilizing different regenerating agents. SN Appl. Sci. 2, 2150. doi:10.1007/s42452-020-03911-8
Darmayanti, L., Notodarmodjo, S., Damanhuri, E., and Mukti, R. R. (2018). Removal of copper (II) ions in aqueous solutions by sorption onto alkali activated fly ash. MATEC Web Conf. 147, 04007. doi:10.1051/matecconf/201814704007
Edokpayi, J., Odiyo, J., Popoola, E., Alayande, O., and Msagati, T. (2015). Synthesis and characterization of biopolymeric chitosan derived from land snail shells and its potential for Pb2+ removal from aqueous solution. Materials 8 (12), 8630–8640. doi:10.3390/ma8125482
Eltaweil, A. S., Omer, A. M., El-Aqapa, H. G., Gaber, N. M., Attia, N. F., El-Subruiti, G. M., et al. (2021). Chitosan based adsorbents for the removal of phosphate and nitrate: a critical review. Carbohydr. Polym. 274, 118671. doi:10.1016/j.carbpol.2021.118671
Fan, Y., Zhai, S., Liu, N., Lv, J., Lei, Z., and An, Q. (2016). Adsorption equilibrium, kinetics and mechanism of Pb(II) over carbon–silica composite biosorbent with designed surface oxygen groups. Res. Chem. Intermed. 42 (2), 869–891. doi:10.1007/s11164-015-2060-z
Gerente, C., Lee, V. K. C., Cloirec, P. L., and McKay, G. (2007). Application of chitosan for the removal of metals from wastewaters by adsorption—mechanisms and models review. Crit. Rev. Environ. Sci. Technol. 37 (1), 41–127. doi:10.1080/10643380600729089
Girotto, F., Alibardi, L., and Cossu, R. (2015). Food waste generation and industrial uses: a review. Waste Manag. 45, 32–41. doi:10.1016/j.wasman.2015.06.008
Hegazi, H. A. (2013). Removal of heavy metals from wastewater using agricultural and industrial wastes as adsorbents. HBRC J. 9 (3), 276–282. doi:10.1016/j.hbrcj.2013.08.004
Husoon, Z. A., Al-Azzawi, M. N. A., and Al-Hiyaly, S. (2013). Investigation biosorption potential of copper and lead from industrial WasteWater using orange and lemon peels. J. Al-Nahrain Univ. Sci., 16(2), 713–179. doi:10.22401/JNUS.16.2.27
Hyder, A. H. M. G., Begum, S. A., and Egiebor, N. O. (2015). Adsorption isotherm and kinetic studies of hexavalent chromium removal from aqueous solution onto bone char. J. Environ. Chem. Eng. 3 (2), 1329–1336. doi:10.1016/j.jece.2014.12.005
Jean Claude, N., Shanshan, L., Khan, J., Yifeng, W., Dongxu, H., and Xiangru, L. (2022). Waste tea residue adsorption coupled with electrocoagulation for improvement of copper and nickel ions removal from simulated wastewater. Sci. Rep. 12, 3519. doi:10.1038/s41598-022-07475-y
Kaya, K., Pehlivan, E., Schmidt, C., and Bahadir, M. (2014). Use of modified wheat bran for the removal of chromium(VI) from aqueous solutions. Food Chem. 158, 112–117. doi:10.1016/j.foodchem.2014.02.107
Khairkar, S., and Raut, A. (2014). Adsorption studies for the removal heavy metal by chitosan-G-poly acrylicacid-Co-acrylamide composite. Sci. J. Anal. Chem. 2 (6), 67. doi:10.11648/j.sjac.20140206.12
Kim, D., Hwang, S., Kim, Y., Jeong, C. H., Hong, Y. P., and Ryoo, K. S. (2019). Removal of heavy metals from water using chicken egg shell powder as a bio-adsorbent. Bull. Korean Chem. Soc. 40 (12), 1156–1161. doi:10.1002/bkcs.11884
Ko, D. C., Cheung, C. W., Choy, K. K., Porter, J. F., and Mckay, G. (2004). Sorption equilibria of metal ions on bone char. Chemosphere 54, 273–281. doi:10.1016/j.chemosphere.2003.08.004
Kohli, K., Prajapati, R., Shah, R., Das, M., and Sharma, B. K. (2024). Food waste: environmental impact and possible solutions. Sustain. Food Technol. 2, 70–80.
Kousalya, G. N., Rajiv Gandhi, M., and Meenakshi, S. (2010). Sorption of chromium(VI) using modified forms of chitosan beads. Int. J. Biol. Macromol. 47 (2), 308–315. doi:10.1016/j.ijbiomac.2010.03.010
Kumar, U., Kumar, B., and Pandey, K. M. (2014). SEM and EDAX study of pretreated rice husk for the sorption of Cu(II) from wastewater. Appl. Mech. Mater. 656, 17–22. doi:10.4028/www.scientific.net/AMM.656.17
Kyzas, G. Z., Kostoglou, M., and Lazaridis, N. K. (2009). Copper and chromium(VI) removal by chitosan derivatives—equilibrium and kinetic studies. Chem. Eng. J. 152 (2–3), 440–448. doi:10.1016/j.cej.2009.05.005
Lu, Y., Song, S., Wang, R., Liu, Z., Meng, J., Sweetman, A. J., et al. (2015). Impacts of soil and water pollution on food safety and health risks in China. Environ. Int. 77, 5–15. doi:10.1016/j.envint.2014.12.010
Luo, X., Deng, Z., Lin, X., and Zhang, C. (2011). Fixed-bed column study for Cu2+ removal from solution using expanding rice husk. J. Hazard. Mater. 187 (1–3), 182–189. doi:10.1016/j.jhazmat.2011.01.019
Marrakchi, F., Hameed, B. H., and Hummadi, E. H. (2020). Mesoporous biohybrid epichlorohydrin crosslinked chitosan/carbon–clay adsorbent for effective cationic and anionic dyes adsorption. Int. J. Biol. Macromol. 163, 1079–1086. doi:10.1016/j.ijbiomac.2020.07.032
Marshall, W. E., Wartelle, L. H., Boler, D. E., Johns, M. M., and Toles, C. A. (1999). Enhanced metal adsorption by soybean hulls modified with citric acid. Bioresour. Technol. 69 (3), 263–268. doi:10.1016/S0960-8524(98)00185-0
Massimi, L., Giuliano, A., Astolfi, M. L., Congedo, R., Masotti, A., and Canepari, S. (2018). Efficiency evaluation of food waste materials for the removal of metals and metalloids from complex multi-element solutions. Materials 11, 334. doi:10.3390/ma11030334
Mcafee, B., Gould, W., Nadeau, J., and Da Costa, A. (2001). BIOSORPTION OF METAL IONS USING CHITOSAN, CHITIN, AND BIOMASS OF RHIZOPUS ORYZAE. Sep. Sci. Technol. 36 (14), 3207–3222. doi:10.1081/SS-100107768
Medellín-Castillo, N. A., Cruz-Briano, S. A., Leyva-Ramos, R., Moreno-Piraján, J. C., Torres-Dosal, A., Giraldo-Gutiérrez, L., et al. (2020). Use of bone char prepared from an invasive species, pleco fish (Pterygoplichthys spp.), to remove fluoride and Cadmium(II) in water. J. Environ. Manag. 256, 109956. doi:10.1016/j.jenvman.2019.109956
Mendoza-Castillo, D. I., Bonilla-Petriciolet, A., and Jáuregui-Rincón, J. (2015). On the importance of surface chemistry and composition of Bone char for the sorption of heavy metals from aqueous solution. Desalination Water Treat. 54 (6), 1651–1662. doi:10.1080/19443994.2014.888684
Mohanty, S. R. (2015). Removal of hexavalent chromium using chitosan prepared from the shrimp and crab shells.
Muhaemin, M. (2005). Chelating ability of crab shell particles and extracted acetamido groups (chitin and chitosan) from Portunus sp to lead (Pb2+). J. Coast. Dev. 9, 1–7.
Mulyatun, M. (2018). Synthesis of low-cost adsorbent based on fly ash for heavy metal reduction of Cu and Cr in textile industrial liquid waste. J. Nat. Sci. Math. Res. 4 (2), 60–68. doi:10.21580/jnsmr.2018.4.2.11020
Ndekei, A., Gitita, M.-, Njomo, N., and Mbui, D. (2021). Synthesis and characterization of rice husk biochar and its application in the adsorption studies of lead and copper. Int. Res. J. Pure Appl. Chem., 36–50. doi:10.9734/irjpac/2021/v22i430402
Neolaka, Y. A. B., Riwu, A. A. P., Aigbe, U. O., Ukhurebor, K. E., Onyancha, R. B., Darmokoesoemo, H., et al. (2023). Potential of activated carbon from various sources as a low-cost adsorbent to remove heavy metals and synthetic dyes. Results Chem. 5, 100711. doi:10.1016/j.rechem.2022.100711
Nhapi, I., Banadda, N., Murenzi, R., Sekomo, C., and Wali, U. (2011). Removal of heavy metals from industrial wastewater using rice husks. Open Environ. Eng. J. 4, 170–180. doi:10.2174/1874829501104010170
Nigri, E. M., Bhatnagar, A., and Rocha, S. D. F. (2017). Thermal regeneration process of bone char used in the fluoride removal from aqueous solution. J. Clean. Prod. 142, 3558–3570. doi:10.1016/j.jclepro.2016.10.112
Nyairo, W. N., Eker, Y. R., Kowenje, C., Zor, E., Bingol, H., Tor, A., et al. (2017). Efficient removal of lead(II) ions from aqueous solutions using methyl-β-cyclodextrin modified graphene oxide. Water, Air, and Soil Pollut. 228 (11), 406. doi:10.1007/s11270-017-3589-9
Ogata, F., Kangawa, M., Iwata, Y., Ueda, A., Tanaka, Y., and Kawasaki, N. (2014). A study on the adsorption of heavy metals by using raw wheat bran bioadsorbent in aqueous solution phase. Chem. Pharm. Bull. 62 (3), 247–253. doi:10.1248/cpb.c13-00701
Ogata, F., Kangawa, M., Tominaga, H., Tanaka, Y., Ueda, A., Iwata, Y., et al. (2013). Study of adsorption mechanism of heavy metals onto waste biomass wheat bran. J. Oleo Sci. 62 (11), 949–953. doi:10.5650/jos.62.949
Okoro, H. K., Alao, S. M., Pandey, S., Jimoh, I., Basheeru, K. A., Caliphs, Z., et al. (2022). Recent potential application of rice husk as an eco-friendly adsorbent for removal of heavy metals. Appl. Water Sci. 12 (12), 259. doi:10.1007/s13201-022-01778-1
Ooishi, K., Ogino, K., Nishioka, H., and Muramatsu, Y. (2011). “Characterization and cadmium ion-removing property of adsorbents synthesized from inorganic wastes,” in IOP conference series: materials science and engineering. IOP Publishing.162020
Osman, A. I., Blewitt, J., Abu-Dahrieh, J. K., Farrell, C., Al-Muhtaseb, A. H., Harrison, J., et al. (2019). Production and characterisation of activated carbon and carbon nanotubes from potato peel waste and their application in heavy metal removal. Environ. Sci. Pollut. Res. 26 (36), 37228–37241. doi:10.1007/s11356-019-06594-w
Piccirillo, C. (2023). Preparation, characterisation and applications of bone char, a food waste-derived sustainable material: a review. J. Environ. Manag. 339, 117896. doi:10.1016/j.jenvman.2023.117896
Piccirillo, C., Moreira, I. S., Novais, R. M., Fernandes, A. J. S., Pullar, R. C., and Castro, P. M. L. (2017). Biphasic apatite-carbon materials derived from pyrolysed fish bones for effective adsorption of persistent pollutants and heavy metals. J. Environ. Chem. Eng. 5 (5), 4884–4894. doi:10.1016/j.jece.2017.09.010
Popuri, S. R., Vijaya, Y., Boddu, V. M., and Abburi, K. (2009). Adsorptive removal of copper and nickel ions from water using chitosan coated PVC beads. Bioresour. Technol. 100 (1), 194–199. doi:10.1016/j.biortech.2008.05.041
Praipipat, P., Ngamsurach, P., and Roopkhan, N. (2023). Zeolite A powder and beads from sugarcane bagasse fly ash modified with iron(III) oxide-hydroxide for lead adsorption. Sci. Rep. 13 (1), 1873. doi:10.1038/s41598-023-29055-4
Radomski, P., Piątkowski, M., Bogdał, D., and Jarosiński, A. (2014). The use of chitosan and its modified derivatives to remove trace amounts of heavy metals from industrial wastewater. Chemik 68, 39–46.
Ráheľ, J., Procházka, V., Zahoran, M., and Erben, D. (2008). Removal of copper metal ions from aqueous solutions by plasma made chitosan filter. Chem. Listy 102, s1432–s1435.
Rahman, A. (2024). Promising and environmentally friendly removal of copper, zinc, cadmium, and lead from wastewater using modified shrimp-based chitosan. Water 16 (1), 184. doi:10.3390/w16010184
Rahman, A., Haque, M. A., Ghosh, S., Shinu, P., Attimarad, M., and Kobayashi, G. (2023). Modified shrimp-based chitosan as an emerging adsorbent removing heavy metals chromium, nickel, arsenic, and cobalt from polluted water. Sustainability 15 (3), 2431. doi:10.3390/su15032431
Rahman, A., Seloma, A. S., and Ismail, I. I. (2020). Adsorption study of cadmium (II) and lead (II) on rice husk. Egypt. Acad. J. Biol. Sci. F. Toxicol. and Pest Control 12, 1–8. doi:10.21608/eajbsf.2020.105964
Ranjbar, N., Hashemi, S., Ramavandi, B., and Ravanipour, M. (2018). Chromium(VI) removal by bone char–ZnO composite: parameters optimization by response surface methodology and modeling. Environ. Prog. and Sustain. Energy 37 (5), 1684–1695. doi:10.1002/ep.12854
Rojas, G., Silva, J., Flores, J. A., Rodriguez, A., Ly, M., and Maldonado, H. (2005). Adsorption of chromium onto cross-linked chitosan. Sep. Purif. Technol. 44 (1), 31–36. doi:10.1016/j.seppur.2004.11.013
Rojas-Mayorga, C., Mendoza-Castillo, D., Bonilla-Petriciolet, A., and Silvestre-Albero, J. (2016). Tailoring the adsorption behavior of bone char for heavy metal removal from aqueous solution. Adsorpt. Sci. and Technol. 34 (6), 368–387. doi:10.1177/0263617416658891
Rojas-Mayorga, C. K., Bonilla-Petriciolet, A., Aguayo-Villarreal, I. A., Hernández-Montoya, V., Moreno-Virgen, M. R., Tovar-Gómez, R., et al. (2013). Optimization of pyrolysis conditions and adsorption properties of bone char for fluoride removal from water. J. Anal. Appl. Pyrolysis 104, 10–18. doi:10.1016/j.jaap.2013.09.018
Rojas-Mayorga, C. K., Bonilla-Petriciolet, A., Silvestre-Albero, J., Aguayo-Villarreal, I. A., and Mendoza-Castillo, D. I. (2015). Physico-chemical characterization of metal-doped bone chars and their adsorption behavior for water defluoridation. Appl. Surf. Sci. 355, 748–760. doi:10.1016/j.apsusc.2015.07.163
Salihi, I. U., Kutty, S. R. M., Isa, M. H., Aminu, N., and Ezerie, H. (2014). Microwave incinerated sugarcane bagasse ash (MISCBA) as a low cost adsorbent for the removal of zinc in aqueous solution. 1, 281–290. doi:10.2495/ESUS140241
Samra, S. E., Jeragh, B., EL-Nokrashy, A. M., and EL-Asmy, A. A. (2014). Biosorption of Pb2+ from natural water using date pits: a green chemistry approach. Mod. Chem. Appl. 2, 1–8.
Sánchez-Ponce, L., Díaz-de-Alba, M., Casanueva-Marenco, M. J., Gestoso-Rojas, J., Ortega-Iguña, M., Galindo-Riaño, M. D., et al. (2022). Potential use of low-cost agri-food waste as biosorbents for the removal of Cd(II), Co(II), Ni(II) and Pb(II) from aqueous solutions. Separations 9 (10), 309. doi:10.3390/separations9100309
Sen, T. K. (2023). Agricultural solid wastes based adsorbent materials in the remediation of heavy metal ions from water and wastewater by adsorption: a review. Molecules 28, 5575. doi:10.3390/molecules28145575
Shafiq, M., Alazba, A., and Amin, M. (2018). Removal of heavy metals from wastewater using date palm as a biosorbent: a comparative review. Sains Malays. 47, 35–49. doi:10.17576/jsm-2018-4701-05
Shah, B., Mistry, C., and Shah, A. (2013). Seizure modeling of Pb(II) and Cd(II) from aqueous solution by chemically modified sugarcane bagasse fly ash: isotherms, kinetics, and column study. Environ. Sci. Pollut. Res. 20 (4), 2193–2209. doi:10.1007/s11356-012-1029-3
Shen, G., Li, Z., Hong, T., Ru, X., Wang, K., Gu, Y., et al. (2023). The status of the global food waste mitigation policies: experience and inspiration for China. Environ. Dev. Sustain. 26 (4), 8329–8357. doi:10.1007/s10668-023-03132-0
Singh, S., Chaudhary, I. J., and Kumar, P. (2019). Utilization of low-cost agricultural waste for removal of toxic metals from environment: a review. Int. J. Sci. Res. Biol. Sci. 6, 56–61. doi:10.26438/ijsrbs/v6i4.5661
Sobhanardakani, S., Zandipak, R., Parvizimosaed, H., Javanshir Khoei, A., Moslemi, M., Tahergorabi, M., et al. (2014). Efficiency of chitosan for the removal of Pb (II), Fe (II) and Cu (II) ions from aqueous solutions. Iran. J. Toxicol. 8, 1145–1151.
Sofiane, B., and Sofia, K. S. (2015). Biosorption of heavy metals by chitin and the chitosan. Der Pharma Chem. 7, 54–63.
Sud, D., Mahajan, G., and Kaur, M. (2008). Agricultural waste material as potential adsorbent for sequestering heavy metal ions from aqueous solutions – a review. Bioresour. Technol. 99 (14), 6017–6027. doi:10.1016/j.biortech.2007.11.064
Sulyman, M., Namiesnik, J., and Gierak, A. (2017). Low-cost adsorbents derived from agricultural by-products/wastes for enhancing contaminant uptakes from wastewater: a review. Pol. J. Environ. Stud. 26, 479–510. doi:10.15244/pjoes/66769
Tang, Y.-T. (2020). “Accounting for the environmental impact of food waste on water resources and climate change,” in Food industry wastes (Elsevier), 305–329. doi:10.1016/B978-0-12-817121-9.00014-0
Thompson, M. O., and Kearns, J. P. (2021). Modeling and experimental approaches for determining fluoride diffusion kinetics in bone char sorbent and prediction of packed-bed groundwater defluoridator performance. Water Res. X 12, 100108. doi:10.1016/j.wroa.2021.100108
Tien, D. T. (2018). EXPERIMENTAL RESULTS OF ADSORPTION OF Ni (II) FROM WASTEWATER USING COFFEE HUSK BASED ACTIVATED CARBON. Vietnam J. Sci. Technol. 56 (2C), 126–132. doi:10.15625/2525-2518/56/2C/13039
Tonini, D., Albizzati, P. F., and Astrup, T. F. (2018). Environmental impacts of food waste: learnings and challenges from a case study on UK. Waste Manag. 76, 744–766. doi:10.1016/j.wasman.2018.03.032
Unagolla, J. M., and Adikary, S. U. (2015). Adsorption characteristics of cadmium and lead heavy metals into locally synthesized Chitosan Biopolymer. Trop. Agric. Res. 26 (2), 395. doi:10.4038/tar.v26i2.8102
Vardhan, K. H., Kumar, P. S., and Panda, R. C. (2019). A review on heavy metal pollution, toxicity and remedial measures: current trends and future perspectives. J. Mol. Liq. 290, 111197. doi:10.1016/j.molliq.2019.111197
Wilson, J. A., Pulford, I. D., and Thomas, S. (2003). Sorption of Cu and Zn by bone charcoal. Environ. Geochem. Health 25 (1), 51–56. doi:10.1023/A:1021288529358
Xie, S. (2024). Biosorption of heavy metal ions from contaminated wastewater: an eco-friendly approach. Green Chem. Lett. Rev. 17 (1), 2357213. doi:10.1080/17518253.2024.2357213
Xie, W., Wang, Q., Yao, J., Ma, H., Ohsumi, Y., and Ogawa, H. I. (2004). Study on advanced treatment of secondary effluent using fixed-bed filled with bone char. Water, Air, and Soil Pollut. 159 (1), 313–324. doi:10.1023/B:WATE.0000049181.63852.98
Yan, Z., Haijia, S., and Tianwei, T. (2007). Adsorption behaviors of the aminated chitosan adsorbent. Korean J. Chem. Eng. 24 (6), 1047–1052. doi:10.1007/s11814-007-0119-2
Yanaka, A., Shibata, K., Matsumoto, N., and Yoshida, H. (2021). STUDY ON ADSORPTION OF HEAVY METALS BY RICE HUSK AND EXTRACTION OF HEAVY METALS ADSORBED IN RICE HUSK. GEOMATE J. 20, 28–33. doi:10.21660/2021.79.6221
Yogeshwaran, V., and Priya, A. K. (2021). Experimental studies on the removal of heavy metal ion concentration using sugarcane bagasse in batch adsorption process. Desalination Water Treat. 224, 256–272. doi:10.5004/dwt.2021.27160
Zhang, X., Wu, S., Liu, Y., Wang, Z., Zhang, H., and Xiao, R. (2023). Removal of Cr(VI) from aqueous solution by Rice-husk-based activated carbon prepared by Dual-mode heating method. Carbon Resour. Convers. 6 (2), 76–84. doi:10.1016/j.crcon.2023.01.003
Keywords: food wastes, heavy metals, adsorption, wastewater, waste management
Citation: Nyairo W, Njewa JB and Shikuku VO (2025) Adsorption of heavy metals onto food wastes: a review. Front. Environ. Chem. 6:1526366. doi: 10.3389/fenvc.2025.1526366
Received: 11 November 2024; Accepted: 01 April 2025;
Published: 14 April 2025.
Edited by:
Qili Hu, Sichuan University of Science and Engineering, ChinaReviewed by:
Carmen Zaharia, Gheorghe Asachi Technical University of Iași, RomaniaEbenezer Dada, Ladoke Akintola University of Technology, Nigeria
Copyright © 2025 Nyairo, Njewa and Shikuku. This is an open-access article distributed under the terms of the Creative Commons Attribution License (CC BY). The use, distribution or reproduction in other forums is permitted, provided the original author(s) and the copyright owner(s) are credited and that the original publication in this journal is cited, in accordance with accepted academic practice. No use, distribution or reproduction is permitted which does not comply with these terms.
*Correspondence: Wilfrida Nyairo, d255YWlyb0BtYXNlbm8uYWMua2U=