- 1Center of Materials and Nanotechnologies, Faculty of Chemical Technology, University of Pardubice, Pardubice, Czechia
- 2Chair and Department of Pharmaceutical Chemistry, Poznan University of Medical Sciences, Poznań, Poland
- 3Central European Institute of Technology, Brno University of Technology, Brno, Czechia
Herein, TiO2 single-tube (TiO2 ST-NT) powders with and without magnetite Fe3O4 nanoparticles (TiO2 ST-NT@Fe3O4NPs) are presented for the first time as excellent photocatalysts for the degradation of one of the most popular non-steroidal anti-inflammatory drugs (NSAIDs), naproxen (NPX). The TiO2 ST-NT powders were synthesized by anodization followed by etching of the double wall, bending, sonication, ultra-centrifugation, and finally annealing at 600°C. A part of the obtained TiO2 ST-NT powders was decorated with Fe3O4 nanoparticles using a simple one-step decoration process. The best photocatalytic performance of TiO2 ST-NT and TiO2 ST-NT@Fe3O4NPs powders was obtained under the white light (6.2 × 10−4 s-1) and the blue light (2.7 × 10−4 s-1), respectively. During NPX photodegradation using TiO2 ST-NT powders, three main NPX transformation products (P1, P2, and P3) were detected. Upon excitation with the blue light illumination, TiO2 ST-NT@ Fe3O4NPs powders exhibited higher performance (∼80%) than TiO2 ST-NT powders (∼23%) within 1 h, resulting in an approximately three times increased photocatalytic rate constant. Moreover, under simulated sunlight conditions, TiO2 ST-NT powders demonstrated remarkable activity, achieving a 94% NPX degradation within 1 h. TiO2 ST-NT and TiO2 ST-NT@Fe3O4NPs powders represent excellent photocatalysts for NPX degradation.
1 Introduction
In recent years, efforts have been carried out to improve the elimination of pharmaceutical compounds found in various water sources worldwide, including water inlets and outlets, streams, rivers, and groundwater. Pharmaceutical pollutants, persistent in aquatic environments, can have harmful effects on the human health, including kidney problems, skin diseases, and poisoning (Margot et al., 2015; Petrie et al., 2015). Non-steroidal anti-inflammatory drugs (NSAIDs) have gained particular attention as an often-detected class of pollutants because of their stability and resistance. NSAIDs discharge to the environment can originate from hospitals, pharmaceutical industry effluents, and domestic wastewater, which results from their frequent use, over-the-counter accessibility, and high excretion rates (Memmert et al., 2013; Simon and Evan Prince, 2017).
Many solutions have been proposed to reduce or eliminate organic pollutants in wastewater and improve its quality. Advanced oxidation processes (AOPs) have emerged as a viable option for treating a wide range of emerging contaminants, including pharmaceuticals (Stasinakis, 2008; Miklos et al., 2018; Anjali and Shanthakumar, 2019). The efficacy of AOPs is determined by the generation of reactive oxygen species, primarily hydroxyl radicals (OH•). Due to their non-selective nature, OH• can degrade various organic compounds present in water and wastewater, forming carbon dioxide, water, and mineral acids (Legrini et al., 1993). The most used AOPs include ozone-based processes (Abromaitis et al., 2022), Fenton reaction (Im et al., 2015a), advanced oxidation with persulfate (Ge et al., 2021), and photocatalysis (Ye et al., 2018). Among these, the photocatalytic process using a semiconductor material has been extensively researched for the elimination, degradation, and mineralization of organic compounds in aqueous systems (Li and Li, 2001; Choi et al., 2007).
One of the most widely investigated semiconductors is TiO2, a very efficient photocatalyst used for the degradation of pharmaceutical contaminants in water (Mills et al., 1993; Mills and Le Hunte, 1997; Hashimoto et al., 2005; Ni et al., 2007). The crystallinity of TiO2 plays a crucial role in photocatalysis (Tanaka et al., 1991; Li et al., 2012). For instance, the anatase phase exhibits superior activity when exposed to UV illumination, while the presence of mixed anatase-rutile crystalline phases has been associated with photoactivity at longer wavelengths in the visible light region (Collins-Martinez et al., 2007; Solís-Casados et al., 2017).
The application of TiO2 in the photocatalytic oxidative remediation of pharmaceutically polluted water has already been reported (Molinari et al., 2006; Coleman et al., 2007). Different shapes of TiO2 such as nanoparticles (Kanakaraju et al., 2015), nanofibers (Doh et al., 2008), and nanotubes (Albu et al., 2007; Macak et al., 2007; García-Valverde et al., 2014) can be used as a photocatalyst. The most popular ways to synthesize TiO2-based nanomaterial include sol-gel (Akpan and Hameed, 2010), solsolvothermal (Zhou et al., 2010), hydrothermal (García-Valverde et al., 2014; Kasuga et al., 1999), and anodization (Macak et al., 2008). TiO2 nanotube (TiO2 NT) layers obtained via anodization have been particularly useful for the degradation of pharmaceuticals (Im et al., 2015a; Qian et al., 2021).
Among the NSAIDs, naproxen (NPX), which is widely prescribed for skeleton-muscle pain or inflammatory rheumatic disorders, is one of the most frequently detected pharmaceutical residues in water bodies (Todd and Clissold, 1990; Stovitz and Johnson, 2003). TiO2 nanoparticles and TiO2 nanotube (TiO2 NT) layers have been reported in the elimination of NPX from various wastewater matrices (Im et al., 2015a; Kanakaraju et al., 2015). Different shapes of TiO2 unmodified (Méndez-Arriaga et al., 2008b, 2008a; Jallouli et al., 2016a; Kanakaraju et al., 2016a) and modified for instance with Ce (Hao et al., 2023), MoS2 (Sheydaei et al., 2022), and Cu-S (Amini et al., 2020) in the removal of different drugs show a positive impact on the degradation rates as shown in Supplementary Tables S1, S2. However, only one publication focused on TiO2 NT layers as a catalyst using AOPs to determine their effectiveness in degrading NPX under ultrasonic radiation. In that study was demonstrated a remarkable 96.0% improvement in NPX degradation efficiency when TiO2 NT layers were present, as opposed to their absence (Im et al., 2015).
To further enhance the photocatalytic performance of TiO2-based nanomaterials and also to extend the light absorption in the visible spectral region, Fe3O4 nanoparticles (NPs) were incorporated into TiO2-based nanomaterials (Liu et al., 2016; Bi et al., 2019; Yilmaz et al., 2020). Apart from the more favorable light absorption, this enhancement can be attributed to the efficient separation of h+ and e−, which occurs by reducing iron ions into Fe2+ or Fe0 (Li et al., 2017). Additionally, whenever a magnetic field can be applied to the material or system used, this modification facilitates and accelerates the separation of the liquid phase from the solid photocatalyst, using an external magnet, without the need for centrifugation or filtration. Recently, it was shown that TiO2 single-tube (TiO2 ST-NT) powders, derived from TiO2 NT layers, present an excellent photocatalytic performance under UV light using methylene blue as a model dye (Beketova et al., 2020). The TiO2 ST-NT powders were synthesized by anodization, followed by an etching process, and modification with Fe3O4 (magnetite) NPs, resulting in TiO2 nanotube powders modified with Fe3O4 NPs (TiO2 ST-NT@Fe3O4NPs) as a guidable photocatalyst (Beketova et al., 2020). The effect of the annealing temperature of TiO2 ST-NT and TiO2 ST-NT@Fe3O4NPs powders on the photocatalytic performance was evaluated, revealing that the best results were obtained after annealing at 600°C (Sepúlveda et al., 2023).
To the best of our knowledge, this study is the first to report on the photocatalytic performance of TiO2 ST-NT and TiO2 ST-NT@Fe3O4NPs powders for the degradation of NPX under visible light of different wavelengths. The aim of this study was to i) obtain hybrid photocatalytic materials based on TiO2 ST-NT powders and Fe3O4 NPs, and ii) assess their photocatalytic activity. The TiO2 ST-NT and TiO2 ST-NT@Fe3O4NPs powders annealed at 600°C were tested for the photocatalytic degradation of NPX using visible light: the blue light (λmax = 425 nm), the green light (λmax = 525 nm), and the white light. The kinetics of degradation and the possible NPX degradation products were also discussed.
2 Materials and methods
2.1 Synthesis of TiO2 ST-NT powders
To obtain TiO2 single-tube (TiO2 ST-NT) powders, the following procedure was employed, as described in our previous work (Sepúlveda et al., 2023). In brief, TiO2 nanotube (TiO2 NT) layers were synthesized on Ti foils (127 µm thick, Sigma-Aldrich) in ethylene glycol (Anhydrous, 99.8% CAS-No: 107-21-1, Sigma-Aldrich) -based electrolyte containing 10% water and 0.15 M NH4F (ACS reagent, purity ≥98.0%, CAS-No: 12125-01-8, Sigma-Aldrich) at 100 V for 4 h using a high-voltage potentiostat (PGU-200 V, IPS Elektroniklabor GmbH) (Das et al., 2017). Afterward, the TiO2 NT layers were etched to remove the inner wall of the tubes (Motola et al., 2018). This was achieved by treating the TiO2 NT layers with piranha solution (H2SO4 (Sulfuric acid 96% A.G., CAS-No: 7664-93-9, Penta): H2O2 (Hydrogen peroxide 30% A.G., CAS-No: 7722-84-1, Penta) = 3:1) for 16 min at 70°C. Afterwards, the foils were bent to facilitate the removal of TiO2 NT layers from Ti foils (Beketova et al., 2020).
To transform the TiO2 NT layers into TiO2 ST-NT powders, the TiO2 NT layers were sonicated in isopropanol (98.8% A.G., CAS-No: 67-63-0, Penta) using an ultrasonic bath (FB11203, Fisherbrand) for 5 h at 37 kHz and 100% power. Subsequently, ultra-centrifugation (Optima MAX-XP, Beckman Coulter) at 25,000 g force for 10 min at 25°C was performed to separate the TiO2 ST-NT powders from the isopropanol. The obtained powders were then annealed at 600°C in a muffle oven with a sweep rate of 2.1°C min-1 (Sepúlveda et al., 2023).
Fe3O4 (magnetite) NPs were prepared using an oleic acid process approach, as described in our previous works (Beketova et al., 2020; Sepúlveda et al., 2023). As a final step, the TiO2 ST-NT powders were decorated with Fe3O4 NPs using a simple one-step decoration process, resulting in TiO2 ST-NT@Fe3O4NPs powders (Sepúlveda et al., 2023).
2.2 Material characterization
The morphological characterization was performed using a Transmission Electron Microscope Titan Themis 60–300 (Thermo Fisher Scientific) operated at 300 kV equipped with a high-angle annular dark field detector for scanning transmission electron microscopy (HAADF-STEM) and Super-X detector for STEM energy dispersive X-ray (EDX) spectroscopy. The chemical surface composition of TiO2 ST-NT and TiO2 ST-NT@Fe3O4NPs powders was analysed through X-ray photoelectron spectroscopy (XPS) using an ESCA2SR instrument from Scienta-Omicron. The measurements were conducted with a monochromatic Al Kα X-ray source operating at 250 W. To ensure accuracy, a binding energy scale correction was applied using the C 1s adventitious carbon peak at 284.8 eV. The data analysis was carried out using the CasaXPS program, developed by Casa Software Ltd.
2.3 Photocatalytic performance
To select the suitable light sources, an absorption spectrum NPX, published in the literature was considered (Arany et al., 2013; Jallouli et al., 2016; Yu et al., 2019). Naproxen photocatalytic degradation tests under the illumination of the blue light (λmax = 425 nm, power = 10 W, irradiance = 20 mW/cm2) or the green light (λmax = 525 nm, power = 10 W, irradiance = 5 mW/cm2) were carried out in a reactor consisting of three glass vials placed either on magnetic stirrers (when TiO2 ST-NT powders were used) or on an orbital shaker (when TiO2 ST-NT@Fe3O4NPs powders were used) (Krakowiak et al., 2022; Musial et al., 2022). The tests under the illumination of the white light were conducted in a PhotoCube™ reactor (ThalesNano Inc., Hungary) equipped with four glass vials and four LED panels (32 W each).
During all the experiments, each vial contained 10 mg of the photocatalytic material and 10 mL of 20 mg/L naproxen water solution. The working solution was diluted from a stock solution prepared beforehand by dissolving 100.0 mg of naproxen (PHR 1040, CAS-No: 22204-53-1, Sigma-Aldrich) in 10.0 mL of acetonitrile (HPLC-grade, CAS-No: 75-05-8, Sigma-Aldrich). After adding the photocatalytic material, the mixture was sonicated for 5 min and stirred for 30 min in the dark to reach the adsorption-desorption equilibrium. Then, the light source (blue, green, or white) was turned on. The mixtures were illuminated for 3 h and constantly stirred. Samples of 0.75 mL were taken at the following time points: 0, 15, 30, 60, 120, and 180 min. Collected samples were centrifuged at 10,000 rpm for 20 min. Prior to the HPLC analyses, the samples were additionally filtered through 0.2 µm PTFE syringe filters.
NPX concentrations were measured using an HPLC instrument equipped with a diode array detector (DAD) (Agilent 1,220 Infinity LC System, PerkinElmer, United States), operating at a wavelength of 231 nm, and a Phenomenex Kinetex C18 column (100 × 4.6 mm, 2.6 μm). The flow rate was 1.0 mL/min, and the injected sample volume was 10 μL. The mobile phase consisted of acetonitrile and 1% acetic acid (HPLC-grade, 99.5%–99.9%, CAS-No: 64-19-7, POCH Avantor Poland), 50:50 (v/v).
3 Results and discussion
The X-ray diffraction (XRD) patterns, extensive scanning electron microscopy (SEM) evidence, specific surface areas, high-resolution scanning transmission electron microscopy (HRTEM-STEM-EDX) elemental maps exhibiting the distribution of Ti, Fe, and O, and pore size distributions of TiO2 ST-NT and TiO2 ST-NT@Fe3O4NPs powders annealed at 600°C have been reported in our previous work (Sepúlveda et al., 2023). To provide a new insight into the materials used, Figure 1 shows TEM images of TiO2 ST-NT and TiO2 ST-NT@Fe3O4NPs powders, both annealed at 600°C. As shown in Figure 1B, the surface of TiO2 ST-NT@Fe3O4NPs powders exhibits an uniform distribution of Fe3O4 NPs on the surface of TiO2 ST-NT powders.
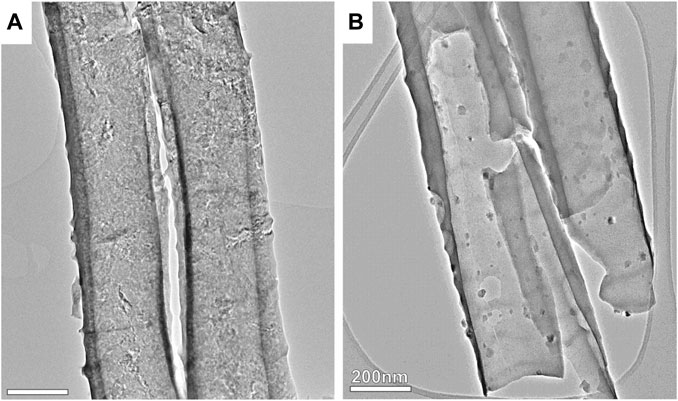
FIGURE 1. Illustrative TEM images of the (A) TiO2 ST-NT and (B) TiO2 ST-NT@Fe3O4NPs powders. The scale bar corresponds to the distance of 200 nm.
The surface elemental composition of the TiO2 ST-NT and TiO2 ST-NT@Fe3O4NPs powders before the photocatalytic measurements was obtained by XPS analysis and it is shown in Supplementary Table S3. The TiO2 ST-NT@Fe3O4NPs powders show a significantly increased amount of C compared to the TiO2 ST-NT powders due to the Fe3O4 NPs modification using an oleic acid process approach. The high-resolution Ti 2p, O 1s, and C 1s XPS spectra of TiO2 ST-NT powders are shown in Supplementary Figure S1. The Ti 2p spectrum exhibits its spin-orbit splitting doublet Ti 2p3/2 and Ti 2p1/2 fitted using six components that correspond to three different chemical species. The first doublet (red line) was assigned to Ti4+ from the TiO2 (peaks centered at ∼458.7 and 464.4 eV) (Hoyos et al., 2017; Sopha et al., 2020). The second doublet (blue line) is related to Ti3+ in the TiO2 lattice (peaks located at 457.5 and 463.2 eV) (Hoyos et al., 2017; Sopha et al., 2023). The third doublet (olive line) corresponds to non-stoichiometric TiOx (peaks at 459.9 and 465.6 eV) (Sopha et al., 2020). The O 1s spectrum reveals the presence of four chemical states. The red peak was assigned to O2- or TiO2 at ∼529.9 eV (Hoyos et al., 2017; Sopha et al., 2020). The blue peak is related to –OH at ∼530.9 eV (Wu et al., 2017; Sopha et al., 2020), the olive peak corresponds to C-O species at 532.0 eV (Rouxhet and Genet, 2011) and the orange peak evidences the presence of (C=O)-OH at ∼533.1 eV (Rouxhet and Genet, 2011). The fitting of the C 1s spectrum was carried out with three different chemical states, adventitious carbon at ∼284.8 eV, C-O species at ∼286.4 eV and (C=O)-OH at ∼288.8 eV (Rouxhet and Genet, 2011).
Supplementary Figure S2 displays the Ti 2p, Fe 2p, O 1s, and C 1s XPS spectra of the TiO2 ST-NT@Fe3O4NPs powders before the photocatalytic measurements. The fitting of the Ti 2p spectrum revealed one doublet suggesting the presence of one titanium oxidation state, Ti4+ (peaks located at ∼458.6 and ∼464.3 eV). In the case of the Fe 2p spectra its corresponding spin-orbit splitting Fe 2p3/2 and Fe 2p1/2 is evidenced. The peak fitting suggests the presence of two different oxidation states, in line with the expected states of the Fe3O4 NPs. The oxidation states for iron were Fe2+ (peaks at ∼710.3 and ∼723.9 eV), and Fe3+ (peaks at ∼712.3 and ∼725.9 eV) (Tang et al., 2018; Ai et al., 2019). O 1s and C 1s spectra reveal the same chemical species as the TiO2 ST-NT powders, with the difference in the intensity and a small shifting in the binding energy ∼0.4 eV of –OH, C-O and (C=O)-OH species in the O 1s signal.
The photocatalytic performance of the powders was assessed in a series of NPX degradation tests, carried out using light sources of different main wavelengths. Experiments were conducted using either LED lamps emitting either blue (λmax = 425 nm) or green (λmax = 525 nm) light or using a solar simulator emitting white light. NPX degradation was observed in each experiment. Moreover, three main NPX transformation products were detected and monitored throughout the experiments: P1, P2, and P3 (marked according to their detection order, Supplementary Figure S3).
Figure 2A shows the photocatalytic performance of TiO2 ST-NT powders activated using the blue light illumination. A 60% reduction of NPX initial concentration was noted within 3 h. At the same time, the concentration of all three transformation products, P1, P2, and P3, increased gradually. In the case of TiO2 ST-NT@Fe3O4NPs powders, 92% of NPX was degraded within 3 h (Figure 2B). As for the NPX transformation products, only P1 and P3 appeared – P2 was not formed. At the end of the experiment, the levels of both P1 and P3 were higher than when unmodified ST-NT powder was used. Therefore, modification with Fe3O4 NPs enhanced the photocatalytic activity of the TiO2 ST-NT powders under the blue light illumination.
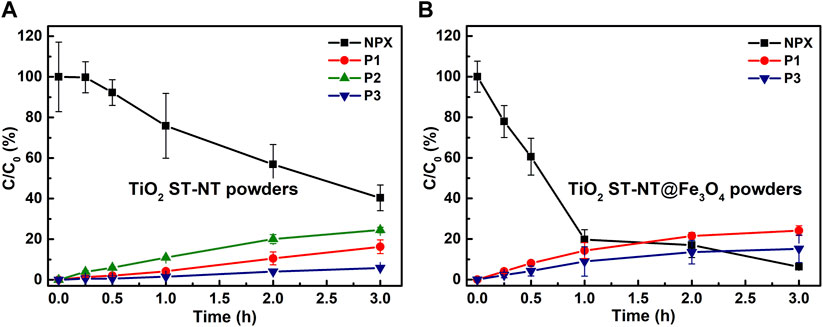
FIGURE 2. Changes in the concentration of NPX and its transformation products (P1, P2, and P3) during the blue light illumination (wavelength ≈425 nm) of the water suspension containing; (A) TiO2 ST-NT powders and (B) TiO2 ST-NT@Fe3O4NPs powders as a photocatalyst.
Upon excitation of the powders with the green light illumination, only 20% NPX degradation was noted in both experiments – when either TiO2 ST-NT or TiO2 ST-NT@ Fe3O4NPs powders were used. In both tests, the NPX transformation products were detected at a very low level (∼1%). Figure 3A shows that all three transformation products appeared when TiO2 ST-NT powders were used, whereas when TiO2 ST-NT@ Fe3O4NPs powders were used, P2 was not detected (Figure 3B). Noteworthy, P2 was not found during both experiments using Fe3O4-modified materials, even if these photocatalysts were more active than bare TiO2 ST-NTs. There are two possible explanations for this result. First, P2 could be adsorbed on the surface of TiO2 ST-NT@Fe3O4NPs and removed via filtration prior to the HPLC analysis. Adsorption of various pharmaceuticals on iron oxides has already been described (Olusegun et al., 2023). Therefore, it is likely that in the present work, such a phenomenon exists between iron oxides and transformation products of pharmaceuticals, too. Alternatively, when Fe3O4-decorated powders were used, P2 could be degraded via photo-Fenton reactions typical for iron oxide-based materials, and thus it was not detected during the analysis (Punjabi et al., 2018; Olusegun et al., 2023). Two photodegradation mechanisms should be considered for iron oxides – regular excitation of the semiconducting materials and photo-dissolution of iron oxides – and P2 could be more susceptible to the latter, which led to its quick elimination from the system. It is also worth noting that the blue and green LED lamps had the same power. Consequently, the irradiance values differed because they depended on the irradiation wavelength. Although most studies on photocatalytic degradation provide little information on the light source used and this detail is rarely pointed out, it should be considered that differences in irradiance values can affect the photocatalytic degradation rates (Eskandarian et al., 2016; Zaveri et al., 2018).
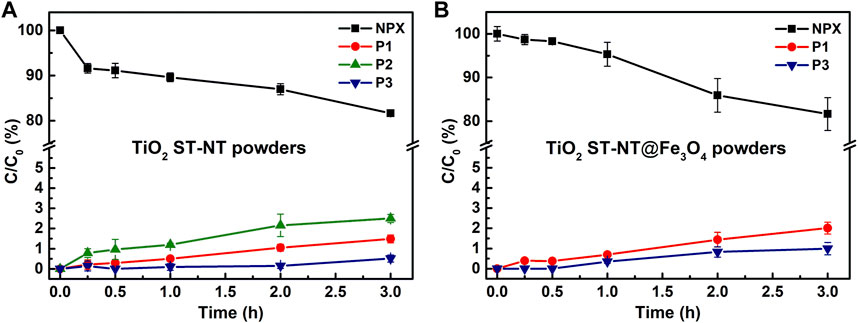
FIGURE 3. Changes in the concentration of NPX and its transformation products (P1, P2, and P3) during the green light illumination (wavelength ≈525 nm) of the water suspension containing; (A) TiO2 ST-NT and (B) TiO2 ST-NT@Fe3O4NPs powders.
Among several works on the photocatalytic degradation of pharmaceutical contaminants using TiO2 combined with Fe-oxides (Amini et al., 2020; Pena-Velasco et al., 2021; Krakowiak et al., 2022) only a few reports show efficient removal rates under the visible light illumination (Sheydaei et al., 2022; Hao et al., 2023). High efficiency is often achieved when other processes, such as ozonation or photoelectrocatalysis, are additionally applied (Amini et al., 2020; Sheydaei et al., 2022). In the present study, we determined the influence of the visible irradiation of a given main wavelength, emitted from a low-power lamp on the TiO2 ST-NT@Fe3O4NPs and noted comparable results. This indicates that the composites display high activity upon excitation with the visible light illumination. Recent studies on photocatalytic removal of commonly used medicines using TiO2-iron oxide materials and visible light are listed in Supplementary Table S1.
Furthermore, the activity of TiO2 ST-NT powders upon simulated sunlight excitation was assessed. In this case, PhotoCube™ was used – a reactor equipped with four LED panels emitting white light. 90% of NPX was degraded within 1 h, and a complete removal occurred within 2 h. Figure 4 shows that all three transformation products were detected during the photocatalytic experiment. Noteworthy, at the end of the experiment, not only NPX but also P2 were completely degraded.
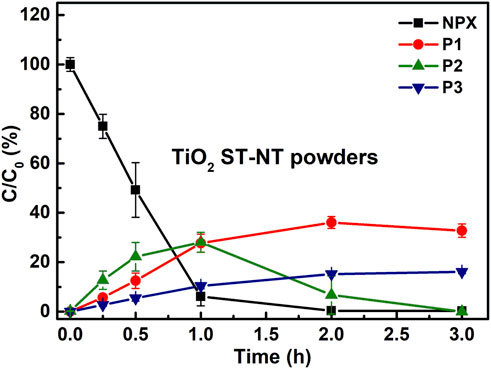
FIGURE 4. Changes in the concentration of NPX and its transformation products (P1, P2, and P3) during the white light illumination of the water suspension containing TiO2 ST-NT powders.
NPX degradation under the white light (i.e., using simulated sunlight) occurred faster than under the other light sources, as compared in Table 1 (photocatalytic rate constants) and plotted in Supplementary Figure S3 (degradation curves). It is important to bear in mind that the tests conducted under the blue light and the white light (Figures 2–4) cannot be directly compared due to the different experimental setups and power of the illumination sources (Musial et al., 2023). Nevertheless, these results confirmed the high activity of unmodified TiO2 ST-NT powder upon the white light illumination, comparable or higher to NPX degradation efficiencies shown in other photocatalytic studies, listed in Supplementary Table S2 (Uheida et al., 2019; Eslami et al., 2020; Kowalkińska et al., 2023). For instance, Eslami et al. achieved complete NPX removal within 2 h, using polycarbonate coated with N- and S-doped TiO2, but employed a high-power (350 W) Xe lamp (Eslami et al., 2020). Similar results under comparable conditions (Xe lamp of 125 W) – complete degradation within 1.5 h – were reported by Uheida et al. (2019), using TiO2 nanoparticles immobilized on polyacrylonitrile/multiwall carbon nanotubes composite nanofiber. However, in this study, only irradiation of a wavelength higher than 420 nm was used, whereas in the present work simulated sunlight conditions also include the participation of the UV-range irradiation in the solar light spectrum. Experiments using TiO2 ST-NT@Fe3O4NPs powders and white light were not conducted due to technical obstacles, because the built-in magnetic stirring precluded using TiO2 ST-NT@Fe3O4NPs powders without an unwanted influence on the degradation rates.

TABLE 1. NPX degradation reaction rate constants k (s-1) under the blue light or the white light illumination using TiO2 ST-NT and TiO2 ST-NT@Fe3O4NPs powders as photocatalysts. r is the correlation coefficient.
Previous studies have demonstrated that the two main NPX degradation routes are decarboxylation and hydroxylation (demethylation) as one can see in Figure 5 (Kanakaraju et al., 2016; Changanaqui et al., 2020; Jung et al., 2020). Noteworthy, these two routes can occur simultaneously or successively (Méndez-Arriaga et al., 2008b; Fan et al., 2019; Kowalkińska et al., 2023). Another route, dimerization, that leads to the formation of more complex compounds, was also described (Kowalkińska et al., 2023). Interestingly, the dimer formation was reported to depend on the facets exposition of the fluorinated TiO2. The results of our analyses match previous reports and considerations. During high-performance liquid chromatography with photodiode-array detection (HPLC-DAD) analyses, it was noted that P1 was eluted earlier than NPX and both P2 and P3 later than NPX. This suggests that P1, is a more polar compound than NPX and is a product of NPX hydroxylation, whereas P2 and P3 are products of its decarboxylation. Furthermore, during the NPX degradation experiment using TiO2 ST-NT powders and simulated sunlight, P2 was completely removed at the end of the experiment. This proves that not only the main tested contaminant but also its transformation products are susceptible to photocatalytic degradation using TiO2 nanotube layers (Jallouli et al., 2016a).
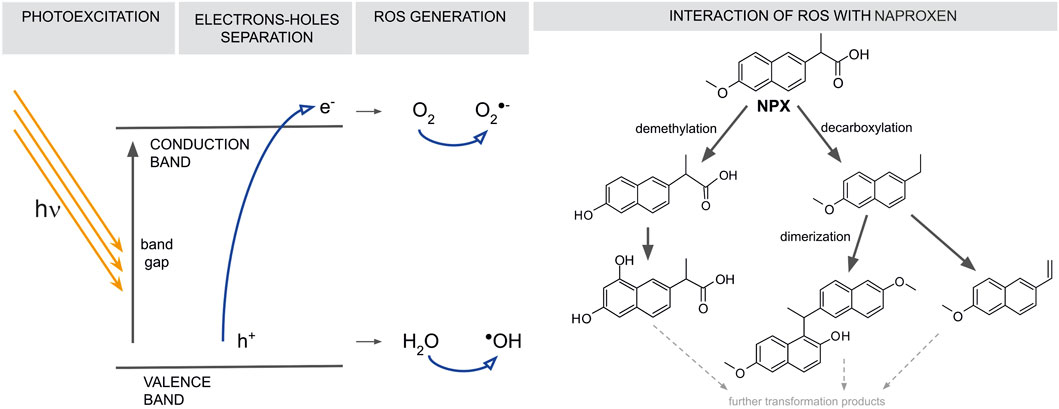
FIGURE 5. Semiconducting material photoexcitation mechanism. Upon an absorption of light of energy higher than the material’s band gap, an electron (e−) is excited to the conduction band, leaving a hole (h+). These species further react with either oxygen or water, leading to the generation of reactive oxygen species (ROS). ROS attacks organic molecules–here, NPX (Kanakaraju et al., 2016a; Changanaqui et al., 2020; Jung et al., 2020).
Prolonged irradiation of the photocatalytic mixture may lead to the complete elimination of the drug transformation products. This is an important observation, as recent research on the toxicity of pharmaceutical pollutants focuses not only on the parent compound but also on the transformation products (Maculewicz et al., 2022). In vitro assays using bioluminescent bacteria (Allivibrio fischeri), showed that the transformation products displayed higher potential toxicity than the parent compound, but the toxicity of the whole post-degradation mixture was not considered as a major concern (Cazzaniga et al., 2020). In addition, phototransformation products of lower molecular weight were found to be more active towards A. fisheri. However, not all the NPX transformation products displayed higher toxicity than the parent compound, which was a proof of the stereostructure-activity relationship (DellaGreca et al., 2003). Other bioassays performed on algae, rotifers, and microcrustaceans proved that phototransformation products displayed higher acute and chronic toxicity than NPX. However, no genotoxic or mutagenic effects were found (Isidori et al., 2005). Moreover, mixtures of NPX and its transformation products proved to be more toxic toward southern toads (Anaxyrus terrestris) than NPX alone (Cory et al., 2019). In this regard the complete degradation of pharmaceuticals along with their transformation products during water treatment is necessary. Toxicity assessment of the wastewater effluents should be considered in the design of AOPs-based water remediation systems. All in all, as highly effective photocatalytic materials, TiO2 ST-NTs powders hold great promise for successful implementation into wastewater treatment procedures.
4 Conclusion
TiO2 ST-NT and TiO2 ST-NT@Fe3O4NPs powders annealed at 600°C were used as catalysts for the degradation of naproxen (NPX) using the blue, green, or white light, for the first time. The photocatalytic performance of TiO2 ST-NT and TiO2 ST-NT@ Fe3O4NPs powders examined under the blue light showed a 60% and 92% reduction in NPX initial concentration within 3 h, respectively. This clearly indicates that the incorporation of Fe3O4 NPs enhanced the photocatalytic activity of TiO2 ST-NT powders under the blue light. Under the green light, both TiO2 ST-NT and TiO2 ST-NT@Fe3O44NPs powders only exhibited a 20% NPX degradation. Complete removal of NPX and one of its transformation products were observed in the experiment conducted using TiO2 ST-NT powders and white light. Remarkably, 94% of NPX degraded within only 1 h. These findings demonstrate the immense potential of TiO2 ST-NT powders for successful implementation in wastewater treatment.
Data availability statement
The original contributions presented in the study are included in the article/Supplementary Material, further inquiries can be directed to the corresponding author.
Author contributions
MS: Conceptualization, Writing–review and editing, Investigation, Methodology, Validation, Writing–original draft. JoM: Conceptualization, Investigation, Methodology, Writing–original draft, Writing–review and editing. IS: Investigation, Writing–review and editing. PC: Investigation, Writing–review and editing. JR-P: Investigation, Writing–review and editing, Visualization. HS: Writing–review and editing, Methodology, Supervision. BS: Methodology, Supervision, Writing–review and editing, Funding acquisition. JaM: Funding acquisition, Supervision, Writing–review and editing, Conceptualization, Project administration, Resources.
Funding
The author(s) declare financial support was received for the research, authorship, and/or publication of this article. The authors were supported by the Ministry of Education, Youth and Sports of the Czech Republic (projects LM2023037 and LM2023051).
Acknowledgments
The authors acknowledge the Ministry of Education, Youth and Sports of the Czech Republic for supporting CEMNAT (nr. LM2023037) and Czech Nano Lab (nr. LM2023051) infrastructures. The authors thank MSc Aleksandra Wójta for her help in conducting the photocatalytic experiments and Dr. Dariusz T. Mlynarczyk for his support and fruitful discussions.
Conflict of interest
The authors declare that the research was conducted in the absence of any commercial or financial relationships that could be construed as a potential conflict of interest.
The author(s) declared that they were an editorial board member of Frontiers, at the time of submission. This had no impact on the peer review process and the final decision.
Publisher’s note
All claims expressed in this article are solely those of the authors and do not necessarily represent those of their affiliated organizations, or those of the publisher, the editors and the reviewers. Any product that may be evaluated in this article, or claim that may be made by its manufacturer, is not guaranteed or endorsed by the publisher.
Supplementary material
The Supplementary Material for this article can be found online at: https://www.frontiersin.org/articles/10.3389/fenvc.2024.1373320/full#supplementary-material
Abbreviations
AOPs, advanced oxidation processes; EDX, energy dispersive X-ray Analysis; HPLC-DAD, high-performance liquid chromatography with photodiode-array detection; HRTEM, high-resolution scanning transmission electron microscopy; NSAIDs, non-steroidal anti-inflammatory drugs; NPs, nanoparticles; NPX, naproxen; P1, P2, and P3, transformation products; SEM, scanning electron microscopy; STEM, scanning transmission electron microscopy; TiO2 NT, TiO2 nanotube; TiO2 ST-NT, TiO2 single-tube nanotube powders; TiO2ST-NT@Fe3O4NPs, TiO2 single-tube nanotube powders decorated with Fe3O4 nanoparticles; XPS, X-ray photoelectron spectroscopy; XRD, X-ray powder diffraction.
References
Abromaitis, V., Svaikauskaite, J., Sulciute, A., Sinkeviciute, D., Zmuidzinaviciene, N., Misevicius, S., et al. (2022). Ozone-enhanced TiO2 nanotube arrays for the removal of COVID-19 aided antibiotic ciprofloxacin from water: process implications and toxicological evaluation. J. Environ. Manage 318, 115515. doi:10.1016/j.jenvman.2022.115515
Ai, Q., Yuan, Z., Huang, R., Yang, C., Jiang, G., Xiong, J., et al. (2019). One-pot co-precipitation synthesis of Fe3O4 nanoparticles embedded in 3D carbonaceous matrix as anode for lithium ion batteries. J. Mat. Sci. 54, 4212–4224. doi:10.1007/s10853-018-3141-3
Akpan, U. G., and Hameed, B. H. (2010). The advancements in sol–gel method of doped-TiO2 photocatalysts. Appl. Catal. A Gen. 375, 1–11. doi:10.1016/j.apcata.2009.12.023
Albu, S. P., Ghicov, A., Macak, J. M., Hahn, R., and Schmuki, P. (2007). Self-organized, free-standing TiO2 nanotube membrane for flow-through photocatalytic applications. Nano Lett. 7, 1286–1289. doi:10.1021/nl070264k
Amini, Z., Givianrad, M. H., Husain, S. W., Azar, P. A., and Saber-Tehrani, M. (2020). Cu-S codoping TiO2/SiO2 and TiO2/SiO2/Fe3O4 core-shell nanocomposites as a novel purple LED illumination active photocatalyst for degradation of diclofenac: the effect of different scavenger agents and optimization. Chem. Eng. Commun. 207, 1536–1553. doi:10.1080/00986445.2019.1660652
Anjali, R., and Shanthakumar, S. (2019). Insights on the current status of occurrence and removal of antibiotics in wastewater by advanced oxidation processes. J. Environ. Manage 246, 51–62. doi:10.1016/j.jenvman.2019.05.090
Arany, E., Szabó, R. K., Apáti, L., Alapi, T., Ilisz, I., Mazellier, P., et al. (2013). Degradation of naproxen by UV, VUV photolysis and their combination. J. Hazard Mater 262, 151–157. doi:10.1016/j.jhazmat.2013.08.003
Beketova, D., Motola, M., Sopha, H., Michalicka, J., Cicmancova, V., Dvorak, F., et al. (2020). One-step decoration of TiO2 nanotubes with Fe3O4 nanoparticles: synthesis and photocatalytic and magnetic properties. ACS Appl. Nano Mat. 3, 1553–1563. doi:10.1021/acsanm.9b02337
Bi, J., Huang, X., Wang, J., Wang, T., Wu, H., Yang, J., et al. (2019). Oil-phase cyclic magnetic adsorption to synthesize Fe3O4@ C@ TiO2-nanotube composites for simultaneous removal of Pb (II) and Rhodamine B. Chem. Eng. J. 366, 50–61. doi:10.1016/j.cej.2019.02.017
Cazzaniga, N., Varga, Z., Nicol, E., and Bouchonnet, S. (2020). UV-visible photodegradation of naproxen in water–Structural elucidation of photoproducts and potential toxicity. Eur. J. Mass Spectrom. 26, 400–408. doi:10.1177/1469066720973412
Changanaqui, K., Alarcon, H., Brillas, E., and Sires, I. (2020). Blue LED light-driven photoelectrocatalytic removal of naproxen from water: kinetics and primary by-products. J. Electroanal. Chem. 867, 114192. doi:10.1016/j.jelechem.2020.114192
Choi, H., Stathatos, E., and Dionysiou, D. D. (2007). Photocatalytic TiO2 films and membranes for the development of efficient wastewater treatment and reuse systems. Desalination 202, 199–206. doi:10.1016/j.desal.2005.12.055
Coleman, H. M., Vimonses, V., Leslie, G., and Amal, R. (2007). Removal of contaminants of concern in water using advanced oxidation techniques. Water Sci. Technol. 55, 301–306. doi:10.2166/wst.2007.421
Collins-Martinez, V., López Ortiz, A., and Aguilar Elguézabal, A. (2007). Influence of the anatase/rutile ratio on the TiO2 photocatalytic activity for the photodegradation of light hydrocarbons. Int. J. Chem. React. Eng. 5, 1–11. doi:10.2202/1542-6580.1613
Cory, W. C., Welch, A. M., Ramirez, J. N., and Rein, L. C. (2019). Naproxen and its phototransformation products: persistence and ecotoxicity to toad tadpoles (Anaxyrus terrestris), individually and in mixtures. Environ. Toxicol. Chem. 38, 2008–2019. doi:10.1002/etc.4514
Das, S., Sopha, H., Krbal, M., Zazpe, R., Podzemna, V., Prikryl, J., et al. (2017). Electrochemical infilling of CuInSe2 within TiO2 nanotube layers and subsequent photoelectrochemical studies. ChemElectroChem 4, 495–499. doi:10.1002/celc.201600763
DellaGreca, M., Brigante, M., Isidori, M., Nardelli, A., Previtera, L., Rubino, M., et al. (2003). Phototransformation and ecotoxicity of the drug Naproxen-Na. Environ. Chem. Lett. 1, 237–241. doi:10.1007/s10311-003-0045-4
Doh, S. J., Kim, C., Lee, S. G., Lee, S. J., and Kim, H. (2008). Development of photocatalytic TiO2 nanofibers by electrospinning and its application to degradation of dye pollutants. J. Hazard Mat. 154, 118–127. doi:10.1016/j.jhazmat.2007.09.118
Eskandarian, M. R., Choi, H., Fazli, M., and Rasoulifard, M. H. (2016). Effect of UV-LED wavelengths on direct photolytic and TiO2 photocatalytic degradation of emerging contaminants in water. Chem. Eng. J. 300, 414–422. doi:10.1016/j.cej.2016.05.049
Eslami, A., Amini, M. M., Asadi, A., Safari, A. A., and Daglioglu, N. (2020). Photocatalytic degradation of ibuprofen and naproxen in water over NS-TiO2 coating on polycarbonate: process modeling and intermediates identification. Inorg. Chem. Commun. 115, 107888. doi:10.1016/j.inoche.2020.107888
Fan, G., Zhan, J., Luo, J., Zhang, J., Chen, Z., and You, Y. (2019). Photocatalytic degradation of naproxen by a H2O2-modified titanate nanomaterial under visible light irradiation. Catal. Sci. Technol. 9, 4614–4628. doi:10.1039/C9CY00965E
García-Valverde, M. T., Lucena, R., Galán-Cano, F., Cárdenas, S., and Valcárcel, M. (2014). Carbon coated titanium dioxide nanotubes: synthesis, characterization and potential application as sorbents in dispersive micro solid phase extraction. J. Chromatogr. A 1343, 26–32. doi:10.1016/j.chroma.2014.03.062
Ge, M., Hu, Z., Wei, J., He, Q., and He, Z. (2021). Recent advances in persulfate-assisted TiO2-based photocatalysis for wastewater treatment: performances, mechanism and perspectives. J. Alloys Compd. 888, 161625. doi:10.1016/j.jallcom.2021.161625
Hao, X., Tian, J., Zhao, Y., Jing, T., Zheng, Y., and Lu, Z. (2023). Photocatalytic degradation of tetracycline over Ce-doped TiO2@ SiO2@ Fe3O4 magnetic material. New J. Chem. 47, 5939–5945. doi:10.1039/D3NJ00110E
Hashimoto, K., Irie, H., and Fujishima, A. (2005). TiO2 photocatalysis: a historical overview and future prospects. Jpn. J. Appl. Phys. 44, 8269. doi:10.1143/JJAP.44.8269
Hoyos, L. J., Rivera, D. F., Gualdrón-Reyes, A. F., Ospina, R., Rodríguez-Pereira, J., Ropero-Vega, J. L., et al. (2017). Influence of immersion cycles during n–β–Bi2O3 sensitization on the photoelectrochemical behaviour of N–F–codoped TiO2 nanotubes. Appl. Surf. Sci. 423, 917–926. doi:10.1016/j.apsusc.2017.06.209
Im, J.-K., Yoon, J., Her, N., Han, J., Zoh, K.-D., and Yoon, Y. (2015a). Sonocatalytic-TiO2 nanotube, Fenton, and CCl4 reactions for enhanced oxidation, and their applications to acetaminophen and naproxen degradation. Sep. Purif. Technol. 141, 1–9. doi:10.1016/j.seppur.2014.11.021
Isidori, M., Lavorgna, M., Nardelli, A., Parrella, A., Previtera, L., and Rubino, M. (2005). Ecotoxicity of naproxen and its phototransformation products. Sci. total Environ. 348, 93–101. doi:10.1016/j.scitotenv.2004.12.068
Jallouli, N., Elghniji, K., Hentati, O., Ribeiro, A. R., Silva, A. M. T., and Ksibi, M. (2016a). UV and solar photo-degradation of naproxen: TiO2 catalyst effect, reaction kinetics, products identification and toxicity assessment. J. Hazard Mat. 304, 329–336. doi:10.1016/j.jhazmat.2015.10.045
Jung, S.-C., Bang, H.-J., Lee, H., Kim, H., Ha, H.-H., Yu, Y. H., et al. (2020). Degradation behaviors of naproxen by a hybrid TiO2 photocatalyst system with process components. Sci. Total Environ. 708, 135216. doi:10.1016/j.scitotenv.2019.135216
Kanakaraju, D., Motti, C. A., Glass, B. D., and Oelgemöller, M. (2015). TiO2 photocatalysis of naproxen: effect of the water matrix, anions and diclofenac on degradation rates. Chemosphere 139, 579–588. doi:10.1016/j.chemosphere.2015.07.070
Kanakaraju, D., Motti, C. A., Glass, B. D., and Oelgemöller, M. (2016a). Solar photolysis versus TiO2-mediated solar photocatalysis: a kinetic study of the degradation of naproxen and diclofenac in various water matrices. Environ. Sci. Pollut. Res. 23, 17437–17448. doi:10.1007/s11356-016-6906-8
Kasuga, T., Hiramatsu, M., Hoson, A., Sekino, T., and Niihara, K. (1999). Titania nanotubes prepared by chemical processing. Adv. Mater. 11, 1307–1311. doi:10.1002/(SICI)1521-4095(199910)11:15<1307::AID-ADMA1307>3.0.CO;2-H
Kowalkińska, M., Sikora, K., Łapiński, M., Karczewski, J., and Zielińska-Jurek, A. (2023). Non-toxic fluorine-doped TiO2 nanocrystals from TiOF2 for facet-dependent naproxen degradation. Catal. Today 413, 113959. doi:10.1016/j.cattod.2022.11.020
Krakowiak, R., Frankowski, R., Mylkie, K., Mlynarczyk, D. T., Ziegler-Borowska, M., Zgoła-Grześkowiak, A., et al. (2022). TiO2–Fe3O4 composite systems—preparation, physicochemical characterization, and an attempt to explain the limitations that arise in catalytic applications. Appl. Sci. 12, 8826. doi:10.3390/app12178826
Legrini, O., Oliveros, E., and Braun, A. M. (1993). Photochemical processes for water treatment. Chem. Rev. 93, 671–698. doi:10.1021/cr00018a003
Li, C.-J., Xu, G.-R., Zhang, B., and Gong, J. R. (2012). High selectivity in visible-light-driven partial photocatalytic oxidation of benzyl alcohol into benzaldehyde over single-crystalline rutile TiO2 nanorods. Appl. Catal. B 115, 201–208. doi:10.1016/j.apcatb.2011.12.003
Li, X. Z., and Li, F. B. (2001). Study of Au/Au3+-TiO2 photocatalysts toward visible photooxidation for water and wastewater treatment. Environ. Sci. Technol. 35, 2381–2387. doi:10.1021/es001752w
Li, Z.-J., Huang, Z.-W., Guo, W.-L., Wang, L., Zheng, L.-R., Chai, Z.-F., et al. (2017). Enhanced photocatalytic removal of uranium (VI) from aqueous solution by magnetic TiO2/Fe3O4 and its graphene composite. Environ. Sci. Technol. 51, 5666–5674. doi:10.1021/acs.est.6b05313
Liu, Y., Wan, J.-F., Liu, C.-T., and Li, Y.-B. (2016). Fabrication of magnetic Fe3O4/C/TiO2 composites with nanotube structure and enhanced photocatalytic activity. Mater. Sci. Technol. 32, 786–793. doi:10.1179/1743284715Y.0000000089
Macak, J. M., Hildebrand, H., Marten-Jahns, U., and Schmuki, P. (2008). Mechanistic aspects and growth of large diameter self-organized TiO2 nanotubes. J. Electroanal. Chem. 621, 254–266. doi:10.1016/j.jelechem.2008.01.005
Macak, J. M., Zlamal, M., Krysa, J., and Schmuki, P. (2007). Self-organized TiO2 nanotube layers as highly efficient photocatalysts. small 3, 300–304. doi:10.1002/smll.200600426
Maculewicz, J., Kowalska, D., Świacka, K., Toński, M., Stepnowski, P., Białk-Bielińska, A., et al. (2022). Transformation products of pharmaceuticals in the environment: their fate,(eco) toxicity and bioaccumulation potential. Sci. total Environ. 802, 149916. doi:10.1016/j.scitotenv.2021.149916
Margot, J., Rossi, L., Barry, D. A., and Holliger, C. (2015). A review of the fate of micropollutants in wastewater treatment plants. Wiley Interdiscip. Rev. Water 2, 457–487. doi:10.1002/wat2.1090
Memmert, U., Peither, A., Burri, R., Weber, K., Schmidt, T., Sumpter, J. P., et al. (2013). Diclofenac: new data on chronic toxicity and bioconcentration in fish. Environ. Toxicol. Chem. 32, 442–452. doi:10.1002/etc.2085
Méndez-Arriaga, F., Esplugas, S., and Giménez, J. (2008a). Photocatalytic degradation of non-steroidal anti-inflammatory drugs with TiO2 and simulated solar irradiation. Water Res. 42, 585–594. doi:10.1016/j.watres.2007.08.002
Méndez-Arriaga, F., Gimenez, J., and Esplugas, S. (2008b). Photolysis and TiO2 photocatalytic treatment of naproxen: degradation, mineralization, intermediates and toxicity. J. Adv. Oxid. Technol. 11, 435–444. doi:10.1515/jaots-2008-0302
Miklos, D. B., Remy, C., Jekel, M., Linden, K. G., Drewes, J. E., and Hübner, U. (2018). Evaluation of advanced oxidation processes for water and wastewater treatment–A critical review. Water Res. 139, 118–131. doi:10.1016/j.watres.2018.03.042
Mills, A., Davies, R. H., and Worsley, D. (1993). Water purification by semiconductor photocatalysis. Chem. Soc. Rev. 22, 417–425. doi:10.1039/CS9932200417
Mills, A., and Le Hunte, S. (1997). An overview of semiconductor photocatalysis. J. Photochem. Photobiol. A Chem. 108, 1–35. doi:10.1016/S1010-6030(97)00118-4
Molinari, R., Pirillo, F., Loddo, V., and Palmisano, L. (2006). Heterogeneous photocatalytic degradation of pharmaceuticals in water by using polycrystalline TiO2 and a nanofiltration membrane reactor. Catal. Today 118, 205–213. doi:10.1016/j.cattod.2005.11.091
Motola, M., Sopha, H., Krbal, M., Hromádko, L., Zmrhalová, Z. O., Plesch, G., et al. (2018). Comparison of photoelectrochemical performance of anodic single- and double-walled TiO2 nanotube layers. Electrochem. Commun. 97, 1–5. doi:10.1016/j.elecom.2018.09.015
Musial, J., Krakowiak, R., Frankowski, R., Spychala, M., Dlugaszewska, J., Dobosz, B., et al. (2022). Simple modification of titanium (IV) oxide for the preparation of a reusable photocatalyst. Mater. Sci. Eng. B 276, 115559. doi:10.1016/j.mseb.2021.115559
Musial, J., Mlynarczyk, D. T., and Stanisz, B. J. (2023). Photocatalytic degradation of sulfamethoxazole using TiO2-based materials–Perspectives for the development of a sustainable water treatment technology. Sci. Total Environ. 856, 159122. doi:10.1016/j.scitotenv.2022.159122
Ni, M., Leung, M. K. H., Leung, D. Y. C., and Sumathy, K. (2007). A review and recent developments in photocatalytic water-splitting using TiO2 for hydrogen production. Renew. Sustain. Energy Rev. 11, 401–425. doi:10.1016/j.rser.2005.01.009
Olusegun, S. J., Souza, T. G. F., Souza, G. de O., Osial, M., Mohallem, N. D. S., Ciminelli, V. S. T., et al. (2023). Iron-based materials for the adsorption and photocatalytic degradation of pharmaceutical drugs: a comprehensive review of the mechanism pathway. J. Water Process Eng. 51, 103457. doi:10.1016/j.jwpe.2022.103457
Pena-Velasco, G., Hinojosa-Reyes, L., Moran-Quintanilla, G. A., Hernandez-Ramirez, A., Villanueva-Rodriguez, M., and Guzman-Mar, J. L. (2021). Synthesis of heterostructured catalyst coupling MOF derived Fe2O3 with TiO2 for enhanced photocatalytic activity in anti-inflammatory drugs mixture degradation. Ceram. Int. 47 (17), 24632–24640. doi:10.1016/j.ceramint.2021.05.185
Petrie, B., Barden, R., and Kasprzyk-Hordern, B. (2015). A review on emerging contaminants in wastewaters and the environment: current knowledge, understudied areas and recommendations for future monitoring. Water Res. 72, 3–27. doi:10.1016/j.watres.2014.08.053
Punjabi, P. B., Ameta, R., Chohadia, A. K., and Jain, A. (2018). Fenton and photo-fenton processes. Rajasthan, India: Udaipur.
Qian, X., Xu, L., Zhu, Y., Yu, H., and Niu, J. (2021). Removal of aqueous triclosan using TiO2 nanotube arrays reactive membrane by sequential adsorption and electrochemical degradation. Chem. Eng. J. 420, 127615. doi:10.1016/j.cej.2020.127615
Rouxhet, P. G., and Genet, M. J. (2011). XPS analysis of bio-organic systems. Surf. Interface Analysis 43, 1453–1470. doi:10.1002/sia.3831
Sepúlveda, M., Saldan, I., Mahnaz, A., Cicmancova, V., Michalicka, J., Hromadko, L., et al. (2023). Magnetically guidable single TiO2 nanotube photocatalyst: structure and photocatalytic properties. Ceram. Int. 49, 6764–6771. doi:10.1016/j.ceramint.2022.10.197
Sheydaei, M., Haseli, A., Ayoubi-Feiz, B., and Vatanpour, V. (2022). MoS2/N-TiO2/Ti mesh plate for visible-light photocatalytic ozonation of naproxen and industrial wastewater: comparative studies and artificial neural network modeling. Environ. Sci. Pollut. Res. 29, 22454–22468. doi:10.1007/s11356-021-17285-w
Simon, J. P., and Evan Prince, S. (2017). Natural remedies for non-steroidal anti-inflammatory drug-induced toxicity. J. Appl. Toxicol. 37, 71–83. doi:10.1002/jat.3391
Solís-Casados, D. A., Escobar-Alarcón, L., Gómez-Oliván, L. M., Haro-Poniatowski, E., and Klimova, T. (2017). Photodegradation of pharmaceutical drugs using Sn-modified TiO2 powders under visible light irradiation. Fuel 198, 3–10. doi:10.1016/j.fuel.2017.01.059
Sopha, H., Bacova, J., Baishya, K., Sepúlveda, M., Rodriguez-Pereira, J., Capek, J., et al. (2023). White and black anodic TiO2 nanotubes: comparison of biological effects in A549 and SH-SY5Y cells. Surf. Coat. Technol. 462, 129504. doi:10.1016/j.surfcoat.2023.129504
Sopha, H., Mirza, I., Turčičova, H., Pavlinak, D., Michalicka, J., Krbal, M., et al. (2020). Laser-induced crystallization of anodic TiO2 nanotube layers. RSC Adv. 10, 22137–22145. doi:10.1039/D0RA02929G
Stasinakis, A. S. (2008). Use of selected advanced oxidation processes (AOPs) for wastewater treatment–a mini review. Glob. NEST J. 10, 376–385. doi:10.30955/gnj.000598
Stovitz, S. D., and Johnson, R. J. (2003). NSAIDs and musculoskeletal treatment: what is the clinical evidence? Phys. Sportsmed. 31, 35–52. doi:10.3810/psm.2003.01.160
Tanaka, K., Capule, M. F. V., and Hisanaga, T. (1991). Effect of crystallinity of TiO2 on its photocatalytic action. Chem. Phys. Lett. 187, 73–76. doi:10.1016/0009-2614(91)90486-S
Tang, X. Q., Zhang, Y. D., Jiang, Z. W., Wang, D. M., Huang, C. Z., and Li, Y. F. (2018). Fe3O4 and metal–organic framework MIL-101 (Fe) composites catalyze luminol chemiluminescence for sensitively sensing hydrogen peroxide and glucose. Talanta 179, 43–50. doi:10.1016/j.talanta.2017.10.049
Todd, P. A., and Clissold, S. P. (1990). Naproxen A reappraisal of its pharmacology, and therapeutic use in rheumatic diseases and pain states. Drugs 40, 91–137. doi:10.2165/00003495-199040010-00006
Uheida, A., Mohamed, A., Belaqziz, M., and Nasser, W. S. (2019). Photocatalytic degradation of Ibuprofen, Naproxen, and Cetirizine using PAN-MWCNT nanofibers crosslinked TiO2-NH2 nanoparticles under visible light irradiation. Sep. Purif. Technol. 212, 110–118. doi:10.1016/j.seppur.2018.11.030
Wu, C.-Y., Tu, K.-J., Deng, J.-P., Lo, Y.-S., and Wu, C.-H. (2017). Markedly enhanced surface hydroxyl groups of TiO2 nanoparticles with superior water-dispersibility for photocatalysis. Materials 10, 566. doi:10.3390/ma10050566
Ye, Y., Feng, Y., Bruning, H., Yntema, D., and Rijnaarts, H. H. M. (2018). Photocatalytic degradation of metoprolol by TiO2 nanotube arrays and UV-LED: effects of catalyst properties, operational parameters, commonly present water constituents, and photo-induced reactive species. Appl. Catal. B 220, 171–181. doi:10.1016/j.apcatb.2017.08.040
Yilmaz, E., Salem, S., Sarp, G., Aydin, S., Sahin, K., Korkmaz, I., et al. (2020). TiO2 nanoparticles and C-Nanofibers modified magnetic Fe3O4 nanospheres (TiO2@ Fe3O4@ C–NF): a multifunctional hybrid material for magnetic solid-phase extraction of ibuprofen and photocatalytic degradation of drug molecules and azo dye. Talanta 213, 120813. doi:10.1016/j.talanta.2020.120813
Yu, H.-W., Park, M., Wu, S., Lopez, I. J., Ji, W., Scheideler, J., et al. (2019). Strategies for selecting indicator compounds to assess attenuation of emerging contaminants during UV advanced oxidation processes. Water Res. 166, 115030. doi:10.1016/j.watres.2019.115030
Zaveri, B. K., De Souza, N. G., Parenky, A. C., and Choi, H. (2018). LED-Based ultraviolet oxidation of pharmaceuticals: effects of wavelength and intensity, pH, and TiO2 loading. Water Environ. Res. 90, 790–799. doi:10.2175/106143017X15131012187926
Keywords: TiO2 single nanotube, Fe3O4 nanoparticles, photocatalysis, naproxen, water treatment
Citation: Sepúlveda M, Musiał J, Saldan I, Chennam PK, Rodriguez-Pereira J, Sopha H, Stanisz BJ and Macak JM (2024) Photocatalytic degradation of naproxen using TiO2 single nanotubes. Front. Environ. Chem. 5:1373320. doi: 10.3389/fenvc.2024.1373320
Received: 19 January 2024; Accepted: 27 February 2024;
Published: 13 March 2024.
Edited by:
Erick R. Bandala, DASCO Inc., United StatesReviewed by:
Eduardo Francisco Pino Lopez, University of Santiago, ChileMonica Cerro-Lopez, University of the Americas Puebla, Mexico
Copyright © 2024 Sepúlveda, Musiał, Saldan, Chennam, Rodriguez-Pereira, Sopha, Stanisz and Macak. This is an open-access article distributed under the terms of the Creative Commons Attribution License (CC BY). The use, distribution or reproduction in other forums is permitted, provided the original author(s) and the copyright owner(s) are credited and that the original publication in this journal is cited, in accordance with accepted academic practice. No use, distribution or reproduction is permitted which does not comply with these terms.
*Correspondence: Jan M. Macak, jan.macak@upce.cz; Beata J. Stanisz, bstanisz@ump.edu.pl
†These authors have contributed equally to this work