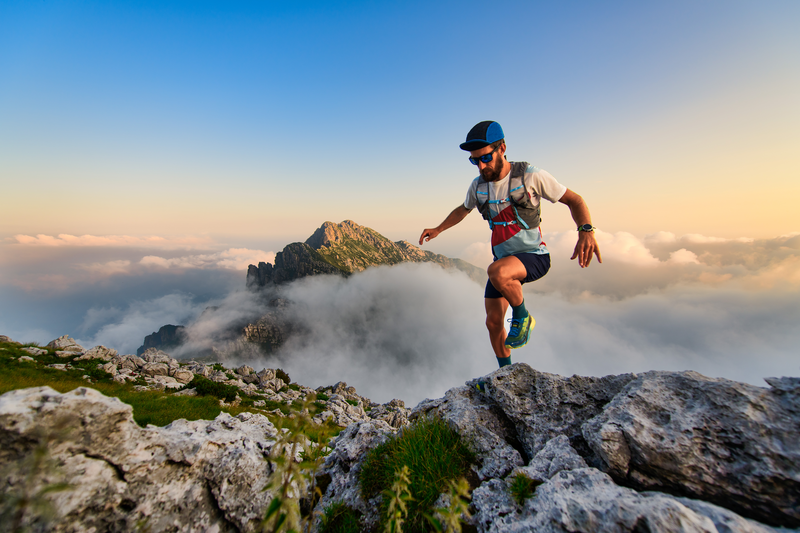
94% of researchers rate our articles as excellent or good
Learn more about the work of our research integrity team to safeguard the quality of each article we publish.
Find out more
MINI REVIEW article
Front. Environ. Chem. , 18 January 2024
Sec. Environmental Analytical Methods
Volume 5 - 2024 | https://doi.org/10.3389/fenvc.2024.1339628
This article is part of the Research Topic Advanced Characterization of Dissolved Organic Matter in Natural Aquatic Environments and Water/Wastewater Treatment Processes View all 5 articles
The alpine area has become a sensitive indicator and amplifier of global climate change and human activities because of its unique geographical and climatic conditions. Being an essential part of biochemical cycling, dissolved organic matter (DOM) could effectively help understand the process, structure, and function of alpine aquatic ecosystems. Due to the low content and sampling difficulties, the analysis of DOM in alpine water demands high sensitivity with low sample volume, which has not been comprehensively reviewed. This review summarizes the DOM sampling, pretreatment, and analysis methods involving the characterization of concentration, spectroscopy, and molecular structure. Overall, conventional parameters are the basis of advanced characterization methods. Spectroscopic tests can reveal the optical properties of DOM in response to lights from ultraviolet to infrared wavelengths, to distinguish the chemical composition. Molecular structure characterizations can provide microscopic information such as functional groups, element ratios, and molecular weights. The combination of multiple methods can depict DOM composition from multiple perspectives. In sum, optimized sampling and pretreatment, high-sensitivity molecular characterization, and method integration are crucial for effectively analyzing DOM components in alpine waters. These perspectives help to standardize the DOM characterization process and to understand the correlation between DOM composition and its properties, as well as the migration and transformation of DOM.
Dissolved organic matter (DOM) is a complex mixture of aromatic and aliphatic hydrocarbon structures that have attached various functional groups (Leenheer and Croué, 2003). In practice, it generally refers to organic components in water that can pass through 0.22–0.7 μm pore-size membranes (Chen et al., 2019; Li YT. et al., 2021; Sun et al., 2021). In natural waters, DOM generally contains proteins, amino acids, polysaccharides, lipids, lignins, humus, other trace substances, and unknown substances (Singer et al., 2012; He C. et al., 2022).
DOM is the major reactant and product in biogeochemical processes, which is proven to be important in global carbon cycling and climate change (Singer et al., 2012; Mu et al., 2016). The special geographical conditions in alpine regions, i.e., high altitude, low temperature, and remote geographical location, lead to slow biological processes (Zhou et al., 2019) and weak anthropogenic activities, resulting in low DOM content in alpine water. For example, dissolved organic carbon concentrations in alpine water samples (elevation >4,000 m) from the Qinghai-Tibet Plateau were commonly less than 1 mg L-1 (Chen et al., 2019; Li YT. et al., 2021; Niu et al., 2022). Alpine water includes alpine runoff, snow/ice, rainwater, reservoir water, alpine lake, and spring/underground water (Huss and Hock, 2018; Li et al., 2020; Zabłocka et al., 2020; Chen et al., 2021; Guo et al., 2022; Zhou et al., 2023). Moreover, due to the low road network density and common altitude stress, researchers generally need to walk a long way to sample in valleys or on the glacier, which leads to a long sampling period and largely restricts the volume and amount of water samples (Mostofa et al., 2009; Gao et al., 2019; Li Z. et al., 2021). Meanwhile, the variation of DOM during this long sampling period may strongly affect the analysis of DOM concentration and composition. Although pretreatments of water samples such as filtration, low-temperature storage, or acidification (pH < 2) might help maintain the stability of DOM (He J. et al., 2022), there are still uncertainties. For instance, water samples in the transect survey were generally collected in chronological order; differences in the period of low-temperature storage may lead to variances in DOM content and components. On the other hand, the acidification process may also cause a DOM reaction and affect analysis results (Du et al., 2021). In summary, 1) an appropriate sampling protocol (sampling point and quantity settings), 2) a simple but effective pretreatment protocol, and 3) high sensitivity with low sample volume are crucial for DOM analysis in alpine regions. These are also a few principles for subsequent screening of DOM research methods suitable for alpine water.
Up to November 2023, using alpine (plateau, alpine, or highland), water quality, and dissolved organic matter as keywords, ∼500 articles were found in the Web of Science (WOS) database. Further screening found that ∼70 papers focused on the DOM analysis and characterization methods for alpine water, among which most papers (∼2/3) focused on the Tibetan Plateau (e.g., Hayakawa et al., 2004; Li et al., 2020; Du et al., 2022). Other study areas include Europe (Laurion et al., 2000; Mladenov et al., 2008; Singer et al., 2012), North America (Belzile et al., 2002; Morris and Hargreaves, 2003; Miller et al., 2009), and Antarctica (Guo et al., 2022). Using the same keywords to search in Chinese on China National Knowledge Infrastructure, 11 papers were retrieved (all on the Qinghai-Tibet Plateau). Overall, many methods, such as the element analysis, ultraviolet-visible (UV-vis) spectroscopy, fluorescence spectroscopy, and high-resolution mass spectrometry, have been applied to investigate the DOM composition in alpine water. This paper comprehensively reviews the advantages and disadvantages of these various methods.
Total organic carbon (TOC) is a basic index indicating the total amount of organic matter in water by carbon content, while chemical oxygen demand (COD) measures reducing matter in water samples by chemical method. Generally, the TOC is measured by a TOC analyzer. After removal of inorganic carbon by acidification (pH < 2), the organic carbon remained in the sample is converted to CO2 via catalytic combustion and quantified by a non-dispersive infrared detector (Mu et al., 2016). COD measures the oxygen equivalent consumed by oxidizing organic matter to CO2 with a strong oxidizing agent (potassium permanganate or dichromate) under acidic conditions. The average oxidation state of carbohydrate carbon in DOM is about 0. The average oxidation state of carbon in proteins and humic acids usually ranges from 0 to −2 (Stumm and Morgan, 1996). The average oxidation state calculated by TOC and COD can obtain information such as the occurrence and valence of organic matter in water samples (Eq. 1), which can be used in the study of DOM component migration and transformation in alpine water environment and regional carbon cycle (Stumm and Morgan, 1996; Mu et al., 2016; Rodriguez-Cardona et al., 2020).
Total organic nitrogen (TON) is a basic index indicating the total amount of organic matter in water by nitrogen content, which is usually measured using a TON analyzer (combustion oxidation-chemiluminescence method). Total phosphorus (TP) is the sum of various chemical forms of granular and dissolved phosphorus in water, including orthophosphate, condensed phosphate, pyrophosphate, metaphosphate, and organic group combined phosphate. The water sample is usually dissolved with potassium persulfate or nitro-perchloric acid as an oxidant and then tested by spectrophotometry. N and P in water are derived from microbial metabolism, soil dissolution, anthropogenic sources, etc. (Qu et al., 2018). These processes are closely related to the formation and release of aquatic organic matter. The retention time of different molecular weight components in size-exclusion chromatography is different. Refining the molecular weight-related C and N composition by liquid chromatography combined with organic carbon and organic nitrogen detector (LC-OCD-OND) can potentially help explore the changing trend of DOM (Huber et al., 2011).
Figure 1 illustrates the principle and classification of spectral methods, ranging from ultraviolet and visible to infrared analysis. The UV-vis spectrum can detect the conjugated double bond structure, such as benzene ring and amide, generating n or π electron excitation. Different components in DOM have various molar absorption coefficients, and the absorbance follows Lambert-Beer’s law. On the peptide bond backbone of protein-like components, the carbonyl has π→π* transition at 190 nm and the peptide bond has n→π* transition at 210–220 nm. On the peptide side chain, the phenylalanine benzene ring, tyrosine phenolic group, and tryptophan indole group exhibit π→π* transitions at 255–270 nm, 270–285 nm, and 285–305 nm, respectively. The nucleic acids absorb light at around 260 nm due to π→π* transition of the bases (Rodger, 2013). Humic substances exhibit a broad spectrum of absorption from UV to visible range with relatively high absorbance at 250–300 nm, which could be attributed to the presence of multiple aromatic groups and complex inter-chromophore interactions (Del Vecchio and Blough, 2004).
Empirical secondary parameters (ratios of absorbance at different wavelengths) such as molecular weight index A365/A250, humification index A400/A300, hydrophobicity index A250/A204, aromaticity index A210/A254, and nonpolar index A220/A254 can be obtained by calculating absorbance data (Peuravuori and Pihlaja, 1997; Erlandsson et al., 2012; Al-Juboori et al., 2016). The larger these indices, the stronger their corresponding properties. In addition, the absorbance ratio can be obtained by dividing the absorbance by the TOC concentration, such as the specific UV absorbance at 254 nm (SUV254) (Weishaar et al., 2003), representing aromaticity. Compared with the total amount analysis method, the UV-vis spectroscopy has the advantages of fast detection speed (consuming a few to 10 minutes), low detection limit (mg L-1 level), and small sample volume (a few to 10 mL), which can meet the needs of DOM characterization in alpine water (Yan et al., 2016; Song et al., 2019). In addition, the sensitivity of DOM detection in alpine water can be improved by sample concentration or by using appropriate cuvettes to enlarge optical path difference. The UV-vis spectral analysis based on characteristic absorbance and secondary parameters can offer a quick glance at the composition and content of DOM in alpine water, and the detailed properties of DOM components can be cross-examined by other methods.
Fluorescence excitation-emission matrix (FEEM) detects the fluorophoric portion of DOM by exciting the electrons through n→π or π→π* transition, which then experience vibrational relaxation or internal conversion and emit fluorescence through π*→n or π*→π transition back to the ground state. Substances with high fluorescence intensity and fluorescence quantum yield often have structures of conjugated π bonds, rigid planes, heterocycles, and/or electron donating groups (-OH or -CN group). Substances with asymmetric structures, such as tryptophan/tyrosine in proteins and phenols in humus, have distinctive fluorescence properties (Peuravuori and Pihlaja, 1997; Mostofa et al., 2009). The quenching effect of halogen and metal ions in water samples may reduce the fluorescence intensity, and the pH and temperature during the detection may also change the properties of organic matter and affect the fluorescence intensity.
In the FEEM test, fluorescence signals can be scanned in the excitation wavelength (Ex) range of 200–650 nm and the emission wavelength (Em) range of 200–800 nm. For DOM identification, the occurrence of fluorescence in different excitation/emission (Ex/Em) wavelength positions indicates the occurrence of different DOM components (Supplementary Table SA1). At a given wavelength position the fluorescence intensity indicates the relative abundance of the corresponding DOM component. Protein-like substances usually exhibit fluorescence at a relatively low Em (<380 nm), with aromatic proteins having a lower Ex (<250 nm) than microbial byproduct-related proteins (250–360 nm) (Chen et al., 2003; Yu et al., 2020). Humus-like substances usually have fluorescence at a medium Em (380–520 nm), with the fulvic acid-like and humic acid-like subset excited at low and medium Ex (<250 nm and 250–420 nm), respectively (Chen et al., 2003; Mladenov et al., 2007). In contrast, the fluorescence of biological pigments (such as chlorophyll a, phycocyanin and phycoerythrin) occurs in notably high Em (>550 nm) regions with varied Ex from 400 to 630 nm (Nebbioso and Piccolo, 2013).
Rich information can be obtained from FEEM by different data analysis methods, including fluorescence regional integration (FRI), parallel factor analysis (PARAFAC), and two-dimensional correlation spectroscopic analysis (2DCOS). FRI divides the scanning matrix into five wavelength regions and calculates the contribution rate of fluorescence intensity in each wavelength region to the total fluorescence, which can be used to distinguish humic acid-like and protein-like components in alpine waters (Li YT. et al., 2021). The derived parameters of FRI include the hydrophobicity parameter, humification parameter, wavelength parameter, and Stokes shift parameter related to fluorescence energy (Xiao et al., 2020). PARAFAC can extract principal components from complex DOM and trace their spatiotemporal variations accordingly (García et al., 2020). The 2DCOS can spread the spectral changes caused by external disturbances to two dimensions, and explore the sensitivity relationship between spectral signal changes and external factors such as altitude, location, time, and meteorological conditions (Noda and Ozaki, 2004; Chen et al., 2019; Li YT. et al., 2021). FEEM has the advantages of high sensitivity (μg L-1 level), small sample amount (less than 2–5 mL), fast measurement speed (consuming a few to 10 minutes), and rich information. The characteristics of microsample but high sensitivity can well fit the needs of alpine water analysis. Therefore, FEEM has been widely used in exploring DOM components in alpine waters.
Fourier transform infrared (FT-IR) spectroscopy detects specific structures with dipole moments because their molecular vibrations and rotations are related to infrared light absorption. By analyzing the absorption wavenumber of the spectrum, information of chemical bonds (functional groups) corresponding to different molecules can be obtained. For example, characteristic wavenumbers useful for DOM analysis include 3,200–3,600 cm-1 (hydrogen bonds), 2,850–2,960 cm-1 (-CH), 1,690–1760 cm-1 (carbonyls), 1,600–1,690/1,480–1,575/1,260–1,300 cm-1 (amide I/II/III in proteins), ∼1,100 cm-1 (C-O in polysaccharides), and ∼1,030 cm-1 (C-O-C in polysaccharides or humus) (Krimm and Bandekar, 1986; Pretsch et al., 2011; Xiao et al., 2022). FT-IR is fast and non-destructive to the sample. FT-IR can obtain the molecular structure of DOM, but the characteristic peaks overlapping caused by the coexistence of multiple functional group absorption peaks often appear in the analysis of DOM samples. This method has not been frequently used for characterization of DOM in alpine waters.
Charged ions with different charge-mass ratios can be detected by mass spectrometry (MS). The inorganic salts in alpine water samples greatly challenge the analysis of ultra-low DOM concentration (Pan et al., 2023). Generally, the desalting, separation, and concentration of DOM components are prerequisites for DOM characterization. DOM can usually be extracted by ultrafiltration, freeze-drying, reverse osmosis combined with electrodialysis, or solid phase extraction methods (Kim et al., 2022). Fourier transform ion cyclotron resonance (FT-ICR) MS can deeply analyze the chemical structure of DOM in natural environments and water treatment processes with ultra-high mass resolution (the m/Δm ratio reaching 104–106) and sub-ppb level mass accuracy. It is suitable for accurately analyzing molecular weights and chemical compositions of various structural fragments in the complex DOM macromolecules in alpine water (He C. et al., 2022).
The full procedure for FT-ICR MS analysis is illustrated in Supplementary Figure SA1. Electrospray ionization can produce ions of various valence states and has high selectivity for polar acidic functional groups; however, it has seldom been used to study DOM (Persson et al., 2005). The FT-ICR MS results can be presented with a van Krevelen diagram to show the differences in DOM components from different sources, and the evolution of specific structures in DOM in different environmental processes can be found through molecular comparison (Pan et al., 2023). Each test requires an equivalent of 100–200 μg TOC mass. FT-ICR MS has high resolution and can provide a huge amount of information; however, its preprocessing is complex and the test is relatively expensive. Moreover, ionization of the complex DOM is often variable, likely to produce unrepeatable results. Quantitative analysis of DOM at molecular level based on FT-ICR MS is a significant but challenging task in future alpine water research.
Nuclear magnetic resonance (NMR) is a physical phenomenon of nuclear absorption and re-emission of electromagnetic radiation in the magnetic field. Before testing, the sample should be pretreated to ensure it does not contain paramagnetic substances and insoluble impurities to eliminate the resolution error (Emery et al., 2001; Hertkorn et al., 2016). NMR is highly selective and provides detailed picture information of the molecular structure of solid and liquid phase samples to distinguish the different connections of functional groups within the DOM molecule (Mao and Schmidt-Rohr, 2003). Measurement of the 1H spectrum in conventional continuous wave methods requires more than 50 mg of samples. When testing the 13C spectrum, measuring the Fourier transform (FT) spectrum is necessary. The FT 13C spectrum requires 50–100 mg samples and the FT 1H spectrum requires 1–10 mg samples (Simpson et al., 2011). NMR can distinguish multiple atoms in a single molecule or collections of molecules of the same type. However, since DOM bears complex compositions, there could be considerable overlap of signals in the NMR spectrum. NMR has been rarely used in the alpine water DOM analysis.
Chromatographic separation can achieve selective enrichment through the difference of charged properties. Combining chromatographic separation with optical detector and mass spectrometry can effectively characterize the DOM components in alpine water (Sandron et al., 2015; Maurischat et al., 2022). LC-OCD-OND requires no complex pretreatment, while DOM samples generally need to be filtered through a 0.22 µm mixed cellulose ester membrane. An LC-OCD-OND test often requires more than 50 mL of a sample with a TOC sample concentration of less than 5 mg L-1. LC-OCD-OND can be applied to characterize natural organic matter to indicate the properties and changes of substances through different molecular weights (Huber et al., 2011). Currently, the coupling of chromatography and detector based on molecular weight and hydrophobicity has been widely used in non-alpine water analysis, showing great potential in the DOM analyses of alpine water.
Disentangling the DOM composition and structure, such as microscopic morphology, molecular weight distribution, and chemical bond composition, is essential for understanding its reaction processes and mechanisms and feedback to environmental change. Various DOM characterization methods have been applied to the alpine water quality assessment and the evaluation of anthropogenic effects on DOM-induced biogeochemical cycling (Zhang et al., 2007; Mu et al., 2016; Ma et al., 2018; Wang et al., 2018; Zhou et al., 2019; Li et al., 2020; Du et al., 2022). Different characterization methods have their own advantages and disadvantages for the case of alpine water analysis (Table 1). Basic analysis (TOC, COD, TN, and TP) can obtain the information of DOM based on total concentration, which is the basis of advanced characterization methods. Spectral testing requires a small water volume and can reveal the optical properties of DOM in response to light from ultraviolet to infrared wavelengths to distinguish its chemical composition. In addition, further analysis of the spectral data can obtain more information on the properties and composition of DOM. Such advantages ensure its great potential in both in-situ monitoring and large spatial-scale DOM research (e.g., regional studies in the Tibetan Plateau or a large river basin), particularly in rural alpine areas. However, the spectral characterization methods (UV-vis and FEEM) could not achieve full quantification of the molecular composition, and thus, we need the molecular structure characterization to depict the microscopic information, such as functional groups, element ratios, and molecular weights. Nevertheless, the molecular structure characterization requires a large amount of water and is generally achievable in long-term observational studies with a fixed location or small spatial-scale studies (e.g., a small watershed or a lake).
TABLE 1. Molecular characterization methods of dissolved organic matter and their application in alpine waters.
The DOM components of alpine water are complex; therefore, using a single method is inadequate and may produce inaccurate results. The comprehensive use of spectroscopy, chromatography, and high-resolution mass spectrometry provides technical support for disentangling DOM’s intricate environmental behaviors, such as adsorption, migration, binding, complexation, and photoinduction. In summary, future research should pay attention to the following issues.
1. Optimized sampling and pretreatment. Some structural characterization methods (e.g., NMR and MS) often require complex DOM extraction and separation processes. Many steps, such as sample collection, transport, storage and pretreatment, will lead to disturbance. Obtaining uncontaminated DOM samples with high repeatability is a challenge. Using portable instruments or simple equipment for on-site testing and optimizing the pretreatment method can improve the sample’s representativeness.
2. High-sensitivity molecular characterization. The concentration of DOM in alpine water is often low. Adequate concentration of samples and choosing appropriate experimental conditions, such as appropriate cuvettes or light sources in spectral analysis, can improve the sensitivity of analysis.
3. Methods integration. Methods such as spectroscopy and MS contain rich information detailing the composition and molecular structure information of DOM. In in-situ monitoring, we could build up the statistical relationship between spectroscopy and mass spectrometry analysis through methods such as machine learning. On this basis, it is possible to integrate high-frequency spectroscopy analysis and low-frequency mass spectrometry analysis via transfer learning and the relationship established by in-situ monitoring in large spatial-scale studies sharing similar environmental and hydrological conditions. Together with the improvement of portable spectral detection equipment, it would largely reduce the workload of field sampling and analysis in tracking DOM composition and changes in alpine waters, particularly in rural or glacial areas.
YZ: Investigation, Writing–original draft. JD: Methodology, Writing–review and editing. KX: Conceptualization, Supervision, Writing–review and editing, Funding acquisition.
The author(s) declare financial support was received for the research, authorship, and/or publication of this article. This research was funded by the Second Tibetan Plateau Scientific Expedition and Research Program (STEP) (No. 2019QZKK0304-02) and the Fundamental Research Funds for the Central Universities (No. E3EG9701X2).
The authors declare that the research was conducted in the absence of any commercial or financial relationships that could be construed as a potential conflict of interest.
All claims expressed in this article are solely those of the authors and do not necessarily represent those of their affiliated organizations, or those of the publisher, the editors and the reviewers. Any product that may be evaluated in this article, or claim that may be made by its manufacturer, is not guaranteed or endorsed by the publisher.
The Supplementary Material for this article can be found online at: https://www.frontiersin.org/articles/10.3389/fenvc.2024.1339628/full#supplementary-material
Al-Juboori, R. A., Yusaf, T., and Pittaway, P. A. (2016). Exploring the correlations between common UV measurements and chemical fractionation for natural waters. Desalin. Water Treat. 57 (35), 16324–16335. doi:10.1080/19443994.2015.1079805
Belzile, C., Gibson, J. A. E., and Vincent, W. F. (2002). Colored dissolved organic matter and dissolved organic carbon exclusion from lake ice: implications for irradiance transmission and carbon cycling. Limnol. Oceanogr. 47 (5), 1283–1293. doi:10.4319/lo.2002.47.5.1283
Chen, M., Li, C., Spencer, R. G. M., Maie, N., Hur, J., McKenna, A. M., et al. (2021). Climatic, land cover, and anthropogenic controls on dissolved organic matter quantity and quality from major alpine rivers across the Himalayan-Tibetan Plateau. Sci. Total Environ. 754, 142411. doi:10.1016/j.scitotenv.2020.142411
Chen, M., Li, C., Zeng, C., Zhang, F., Raymond, P. A., and Hur, J. (2019). Immobilization of relic anthropogenic dissolved organic matter from alpine rivers in the Himalayan-Tibetan Plateau in winter. Water Res. 160, 97–106. doi:10.1016/j.watres.2019.05.052
Chen, W., Westerhoff, P., Leenheer, J. A., and Booksh, K. (2003). Fluorescence excitation-emission matrix regional integration to quantify spectra for dissolved organic matter. Environ. Sci. Technol. 37 (24), 5701–5710. doi:10.1021/es034354c
Del Vecchio, R., and Blough, N. V. (2004). On the origin of the optical properties of humic substances. Environ. Sci. Technol. 38 (14), 3885–3891. doi:10.1021/es049912h
Du, Y., Chen, F., Xiao, K., Song, C. Q., He, H., Zhang, Q. Y., et al. (2021). Water residence time and temperature drive the dynamics of dissolved organic matter in alpine lakes in the Tibetan Plateau. Glob. Biogeochem. Cycles. 35 (11), e2020GB006908. doi:10.1029/2020gb006908
Du, Y., Luo, C., Chen, F., Zhang, Q., Zhou, Y., Jang, K.-S., et al. (2022). Water depth and transparency drive the quantity and quality of organic matter in sediments of alpine lakes on the Tibetan Plateau. Limnol. Oceanogr. 67 (9), 1959–1975. doi:10.1002/lno.12180
Emery, E. F., Junk, T., Ferrell, R. E., Hon, R. D., and Butler, L. G. (2001). Solid-state 2H MAS NMR studies of TNT absorption in soil and clays. Environ. Sci. Technol. 35 (14), 2973–2978. doi:10.1021/es9911566
Erlandsson, M., Futter, M. N., Kothawala, D. N., and Köhler, S. J. (2012). Variability in spectral absorbance metrics across boreal lake waters. J. Environ. Monit. 14 (10), 2643–2652. doi:10.1039/c2em30266g
Gao, T., Kang, S., Chen, R., Zhang, T., Zhang, T. J., Han, C. T., et al. (2019). Riverine dissolved organic carbon and its optical properties in a permafrost region of the Upper Heihe River basin in the Northern Tibetan Plateau. Sci. Total Environ. 686, 370–381. doi:10.1016/j.scitotenv.2019.05.478
García, P. E., García, R. D., Soto Cárdenas, C., Gerea, M., Reissig, M., Pérez, G. L., et al. (2020). Fluorescence components of natural dissolved organic matter (DOM) from aquatic systems of an Andean Patagonian catchment: applying different data restriction criteria for PARAFAC modelling. Spectrochim. Acta A Mol. Biomol. Spectrosc. 229, 117957. doi:10.1016/j.saa.2019.117957
Guo, B., Li, W., Santibanez, P., Priscu, J. C., Liu, Y., and Liu, K. (2022). Organic matter distribution in the icy environments of Taylor Valley, Antarctica. Sci. Total Environ. 841, 156639. doi:10.1016/j.scitotenv.2022.156639
Hayakawa, K., Sakamoto, M., Kumagai, M., Jiao, C., Song, X., and Zhang, Z. (2004). Fluorescence spectroscopic characterization of dissolved organic matter in the waters of Lake Fuxian and adjacent rivers in Yunnan, China. Limnol 5 (3), 155–163. doi:10.1007/s10201-004-0130-9
He, C., He, D., Chen, C., and Shi, Q. (2022a). Application of Fourier transform ion cyclotron resonance mass spectrometry in molecular characterization of dissolved organic matter. Sci. China Earth Sci. 65 (12), 2219–2236. doi:10.1007/s11430-021-9954-0
He, J., Wu, X., Zhi, G., Yang, Y., Wu, L., Zhang, Y., et al. (2022b). Fluorescence characteristics of DOM and its influence on water quality of rivers and lakes in the Dianchi Lake basin. Ecol. Indic. 142, 109088. doi:10.1016/j.ecolind.2022.109088
Hertkorn, N., Harir, M., Cawley, K. M., Schmitt-Kopplin, P., and Jaffé, R. (2016). Molecular characterization of dissolved organic matter from subtropical wetlands: a comparative study through the analysis of optical properties, NMR and FTICR/MS. Biogeosciences 13 (8), 2257–2277. doi:10.5194/bg-13-2257-2016
Huber, S. A., Balz, A., Abert, M., and Pronk, W. (2011). Characterisation of aquatic humic and non-humic matter with size-exclusion chromatography-organic carbon detection-organic nitrogen detection (LC-OCD-OND). Water Res. 45 (2), 879–885. doi:10.1016/j.watres.2010.09.023
Huss, M., and Hock, R. (2018). Global-scale hydrological response to future glacier mass loss. Nat. Clim. Chang. 8 (2), 135–140. doi:10.1038/s41558-017-0049-x
Kim, S., Kim, D., Jung, M. J., and Kim, S. (2022). Analysis of environmental organic matters by ultrahigh-resolution mass spectrometry - a review on the development of analytical methods. Mass Spectrom. Rev. 41 (2), 352–369. doi:10.1002/mas.21684
Krimm, S., and Bandekar, J. (1986). Vibrational spectroscopy and conformation of peptides, polypeptides, and proteins. Adv. Protein Chem. 38, 181–364. doi:10.1016/S0065-3233(08)60528-8
Laurion, I., Ventura, M., Catalan, J., Psenner, R., and Sommaruga, R. (2000). Attenuation of ultraviolet radiation in mountain lakes: factors controlling the among- and within-lake variability. Limnol. Oceanogr. 45 (6), 1274–1288. doi:10.4319/lo.2000.45.6.1274
Leenheer, J. A., and Croué, J.-P. (2003). Peer reviewed: characterizing aquatic dissolved organic matter. Environ. Sci. Technol. 37 (1), 18A–26A. doi:10.1021/es032333c
Li, Y. T., Xiao, K., Du, J. Q., Han, B. J., Liu, Q., Niu, H. S., et al. (2021a). Spectroscopic fingerprints to track the fate of aquatic organic matter along an alpine headstream on the Tibetan Plateau. Sci. Total Environ. 792, 148376. doi:10.1016/j.scitotenv.2021.148376
Li, Z., Fu, Z., Zhang, Y., Guo, Y., Che, F., Guo, H., et al. (2021b). Temporal and spatial distribution and fluorescence spectra of dissolved organic matter in plateau lakes: a case study of Qinghai Lake. Water 13 (24), 3481. doi:10.3390/w13243481
Li, Z., Peng, H., Xie, B., Liu, C., Nie, X., Wang, D., et al. (2020). Dissolved organic matter in surface runoff in the Loess Plateau of China: the role of rainfall events and land-use. Hydrol. Process. 34 (6), 1446–1459. doi:10.1002/hyp.13660
Ma, X. L., Liu, G., Wu, X., Smoak, J. M., Ye, L., Xu, H., et al. (2018). Influence of land cover on riverine dissolved organic carbon concentrations and export in the Three Rivers Headwater Region of the Qinghai-Tibetan Plateau. Sci. Total Environ. 630, 314–322. doi:10.1016/j.scitotenv.2018.02.152
Mao, J. D., and Schmidt-Rohr, K. (2003). Recoupled long-range C-H dipolar dephasing in solid-state NMR, and its use for spectral selection of fused aromatic rings. J. Magn. Reson. 162, 217–227. doi:10.1016/S1090-7807(03)00012-0
Maurischat, P., Lehnert, L., Zerres, V. H. D., Tran, T. V., Kalbitz, K., Rinnan, A., et al. (2022). The glacial-terrestrial-fluvial pathway: a multiparametrical analysis of spatiotemporal dissolved organic matter variation in three catchments of Lake Nam Co, Tibetan Plateau. Sci. Total Environ. 838, 156542. doi:10.1016/j.scitotenv.2022.156542
Miller, M. P., McKnight, D. M., Chapra, S. C., and Williams, M. W. (2009). A model of degradation and production of three pools of dissolved organic matter in an alpine lake. Limnol. Oceanogr. 54 (6), 2213–2227. doi:10.4319/lo.2009.54.6.2213
Mladenov, N., McKnight, D. M., Macko, S. A., Norris, M., Cory, R. M., and Ramberg, L. (2007). Chemical characterization of DOM in channels of a seasonal wetland. Aquat. Sci. 69 (4), 456–471. doi:10.1007/s00027-007-0905-2
Mladenov, N., Pulido-Villena, E., Morales-Baquero, R., Ortega-Retuerta, E., Sommaruga, R., and Reche, I. (2008). Spatiotemporal drivers of dissolved organic matter in high alpine lakes: role of Saharan dust inputs and bacterial activity. J. Geophys. Res. 113, G00D01. doi:10.1029/2008JG000699
Morris, D. P., and Hargreaves, B. R. (2003). The role of photochemical degradation of dissolved organic carbon in regulating the UV transparency of three lakes on the Pocono Plateau. Limnol. Oceanogr. 42 (2), 239–249. doi:10.4319/lo.1997.42.2.0239
Mostofa, K. M. G., Wu, F., Liu, C.-Q., Fang, W. L., Yuan, J., Ying, W. L., et al. (2009). Characterization of Nanming River (southwestern China) sewerage-impacted pollution using an excitation-emission matrix and PARAFAC. Limnol 11 (3), 217–231. doi:10.1007/s10201-009-0306-4
Mu, C., Zhang, T., Wu, Q., Peng, X., Zhang, P., Yang, Y., et al. (2016). Dissolved organic carbon, CO2, and CH4 concentrations and their stable isotope ratios in thermokarst lakes on the Qinghai-Tibetan Plateau. J. Limnol. 75 (2), 313–319. doi:10.4081/jlimnol.2016.1346
Nebbioso, A., and Piccolo, A. (2013). Molecular characterization of dissolved organic matter (DOM): a critical review. Anal. Bioanal. Chem. 405 (1), 109–124. doi:10.1007/s00216-012-6363-2
Niu, H., Kang, S., Sarangi, C., Zhang, G., Chen, M., Zhang, Y., et al. (2022). Source apportionment and elevational gradient of dissolved organic matter over the Tibetan plateau. Catena 216, 106372. doi:10.1016/j.catena.2022.106372
Noda, I., and Ozaki, Y. (2004). Two-dimensional correlation spectroscopy: applications in vibrational and optical spectroscopy. Chichester, England: John Wiley and Sons.
Pan, B., Liu, S., Wang, Y., Li, D., and Li, M. (2023). FT-ICR-MS combined with fluorescent spectroscopy reveals the driving mechanism of the spatial variation in molecular composition of DOM in 22 plateau lakes. Environ. Res. 232, 116272. doi:10.1016/j.envres.2023.116272
Persson, L., Bastviken, D., Alsberg, T., Tranvik, L., and Odham, G. (2005). Electrospray mass spectrometry to study lake water DOM and effects of microbial degradation. Intern. J. Environ. Anal. Chem. 85 (1), 15–27. doi:10.1080/0306731042000303198
Peuravuori, J., and Pihlaja, K. (1997). Molecular size distribution and spectroscopic properties of aquatic humic substances. Anal. Chim. Acta 337 (2), 133–149. doi:10.1016/S0003-2670(96)00412-6
Pretsch, E., Bülmann, P., and Badertscher, M. (2011). Structure determination of organic compounds: tables of spectral data. 4th ed. Berlin, Heidelberg: Springer.
Qu, B., Sillanpaa, M., Kang, S., Yan, F., Li, Z., Zhang, H., et al. (2018). Export of dissolved carbonaceous and nitrogenous substances in rivers of the “Water Tower of Asia”. J. Environ. Sci. 65, 53–61. doi:10.1016/j.jes.2017.04.001
Rodger, A. (2013). “UV absorbance spectroscopy of biological macromolecules,” in Encyclopedia of biophysics. G. Roberts, and A. Watts (Berlin, Heidelberg: Springer), 2714–2718. doi:10.1007/978-3-642-16712-6_780-1
Rodriguez-Cardona, B. M., Coble, A. A., Wymore, A. S., Kolosov, R., Podgorski, D. C., Zito, P., et al. (2020). Wildfires lead to decreased carbon and increased nitrogen concentrations in upland arctic streams. Sci. Rep. 10 (1), 8722. doi:10.1038/s41598-020-65520-0
Sandron, S., Rojas, A., Wilson, R., Davies, N. W., Haddad, P. R., Shellie, R. A., et al. (2015). Chromatographic methods for the isolation, separation and characterisation of dissolved organic matter. Environ. Sci. Process. Impacts 17 (9), 1531–1567. doi:10.1039/c5em00223k
Simpson, A. J., McNally, D. J., and Simpson, M. J. (2011). NMR spectroscopy in environmental research: from molecular interactions to global processes. Prog. Nucl. Magn. Reson. Spectrosc. 58 (3-4), 97–175. doi:10.1016/j.pnmrs.2010.09.001
Singer, G. A., Fasching, C., Wilhelm, L., Niggemann, J., Steier, P., Dittmar, T., et al. (2012). Biogeochemically diverse organic matter in Alpine glaciers and its downstream fate. Nat. Geosci. 5 (10), 710–714. doi:10.1038/ngeo1581
Song, K., Li, S., Wen, Z., Lyu, L., and Shang, Y. (2019). Characterization of chromophoric dissolved organic matter in lakes across the Tibet-Qinghai Plateau using spectroscopic analysis. J. Hydrol. 579, 124190. doi:10.1016/j.jhydrol.2019.124190
Stumm, W., and Morgan, J. J. (1996). Aquatic Chemistry: an introduction emphasizing chemical equilibria in natural waters. 2nd ed. New York: John Wiley and Sons.
Sun, Y., Clauson, K., Zhou, M., Sun, Z., Zheng, C., and Zheng, Y. (2021). Hillslopes in headwaters of Qinghai-Tibetan Plateau as hotspots for subsurface dissolved organic carbon processing during permafrost thaw. J. Geophys. Res. Biogeo. 126 (5), e2020JG006222. doi:10.1029/2020jg006222
Wang, Y. H., Spencer, R. G. M., Podgorski, D. C., Kellerman, A. M., Rashid, H., Zito, P., et al. (2018). Spatiotemporal transformation of dissolved organic matter along an alpine stream flow path on the Qinghai-Tibet Plateau: importance of source and permafrost degradation. Biogeosciences 15 (21), 6637–6648. doi:10.5194/bg-15-6637-2018
Weishaar, J. L., Aiken, G. R., Bergamaschi, B. A., Fram, M. S., Fujii, R., and Mopper, K. (2003). Evaluation of specific ultraviolet absorbance as an indicator of the chemical composition and reactivity of dissolved organic carbon. Environ. Sci. Technol. 37 (20), 4702–4708. doi:10.1021/es030360x
Xiao, K., Xu, Y. R., Cao, X. Y., Xu, H., and Li, Y. F. (2022). “Advanced characterization of membrane surface fouling,” in An L. 60 Years of the loeb-sourirajan membrane: principles, new materials, modelling, characterization, and applications. Editors H.-H. Tseng, W. J. Lau, and M. A. Al-Ghouti (Amsterdam: Elsevier), 499–532. doi:10.1016/B978-0-323-89977-2.00022-1
Xiao, K., Yu, J., Wang, S., Du, J., Tan, J., Xue, K., et al. (2020). Relationship between fluorescence excitation-emission matrix properties and the relative degree of DOM hydrophobicity in wastewater treatment effluents. Chemosphere 254, 126830. doi:10.1016/j.chemosphere.2020.126830
Yan, F., Kang, S., Li, C., Zhang, Y., Qin, X., Li, Y., et al. (2016). Concentration, sources and light absorption characteristics of dissolved organic carbon on a medium-sized valley glacier, northern Tibetan Plateau. Cryosphere 10 (6), 2611–2621. doi:10.5194/tc-10-2611-2016
Yu, J., Xiao, K., Xue, W., Shen, Y. X., Tan, J. H., Liang, S., et al. (2020). Excitation-emission matrix (EEM) fluorescence spectroscopy for characterization of organic matter in membrane bioreactors: principles, methods and applications. Front. Environ. Sci. Eng. 14 (2), 31. doi:10.1007/s11783-019-1210-8
Zabłocka, M., Kowalczuk, P., Meler, J., Peeken, I., Dragańska-Deja, K., and Winogradow, A. (2020). Compositional differences of fluorescent dissolved organic matter in Arctic Ocean spring sea ice and surface waters north of Svalbard. Mar. Chem. 227, 103893. doi:10.1016/j.marchem.2020.103893
Zhang, Y. L., Zhang, E. L., Liu, M. L., Wang, X., and Qiang, B. Q. (2007). Variation of chromophoric dissolved organic matter and possible attenuation depth of ultraviolet radiation in Yunnan Plateau lakes. Limnol 8 (3), 311–319. doi:10.1007/s10201-007-0219-z
Zhou, L., Zhou, Y., Zhang, Y., Wu, Y., Jang, K. S., Spencer, R. G. M., et al. (2023). Hydrological controls on dissolved organic matter composition throughout the aquatic continuum of the watershed of Selin Co, the largest lake on the Tibetan Plateau. Environ. Sci. Technol. 57 (11), 4668–4678. doi:10.1021/acs.est.2c08257
Zhou, Y., Zhou, L., He, X., Jang, K. S., Yao, X., Hu, Y., et al. (2019). Variability in dissolved organic matter composition and biolability across gradients of glacial coverage and distance from glacial terminus on the Tibetan Plateau. Environ. Sci. Technol. 53 (21), 12207–12217. doi:10.1021/acs.est.9b03348
Keywords: dissolved organic matter (DOM), alpine water, molecular characterization, analytical methods, spectroscopy
Citation: Zhang Y, Du J and Xiao K (2024) Methods for molecular characterization of dissolved organic matter in the alpine water environment: an overview. Front. Environ. Chem. 5:1339628. doi: 10.3389/fenvc.2024.1339628
Received: 16 November 2023; Accepted: 05 January 2024;
Published: 18 January 2024.
Edited by:
Maurice Millet, Université de Strasbourg, FranceReviewed by:
Ni Maofei, Guizhou Minzu University, ChinaCopyright © 2024 Zhang, Du and Xiao. This is an open-access article distributed under the terms of the Creative Commons Attribution License (CC BY). The use, distribution or reproduction in other forums is permitted, provided the original author(s) and the copyright owner(s) are credited and that the original publication in this journal is cited, in accordance with accepted academic practice. No use, distribution or reproduction is permitted which does not comply with these terms.
*Correspondence: Jianqing Du, anFkdUB1Y2FzLmFjLmNu; Kang Xiao, a3hpYW9AdWNhcy5hYy5jbg==
Disclaimer: All claims expressed in this article are solely those of the authors and do not necessarily represent those of their affiliated organizations, or those of the publisher, the editors and the reviewers. Any product that may be evaluated in this article or claim that may be made by its manufacturer is not guaranteed or endorsed by the publisher.
Research integrity at Frontiers
Learn more about the work of our research integrity team to safeguard the quality of each article we publish.