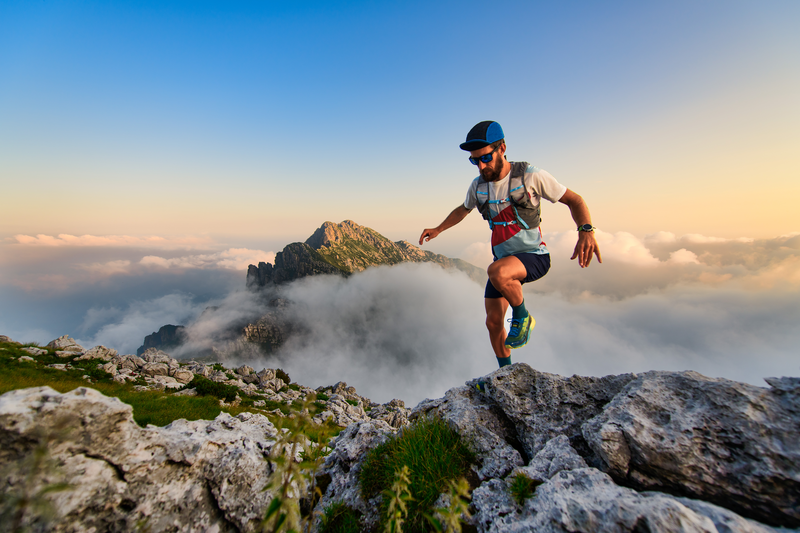
94% of researchers rate our articles as excellent or good
Learn more about the work of our research integrity team to safeguard the quality of each article we publish.
Find out more
ORIGINAL RESEARCH article
Front. Environ. Chem. , 26 February 2024
Sec. Inorganic Pollutants
Volume 5 - 2024 | https://doi.org/10.3389/fenvc.2024.1332967
Despite the growing prevalence of Bismuth (Bi), very little research has been carried to assess its potential toxic effects on aquatic organisms. This study aimed to address this gap by investigating the interactive effects of Bi exposure and elevated temperature on freshwater snails of the genus Lymnaea, specifically on their fatty acid (FA) profiles, oxidative stress markers (superoxide dismutase (SOD), catalase (CAT), glutathione peroxidase (GPx), glutathione S-transferase (GST)) and lipid peroxidation (malondialdehyde (MDA) content). Bismuth exposure was introduced through two distinct routes: i) food via Bi-exposed biofilm (grown under 2 μM Bi), and ii) water (2 μM Bi). Exposed snails were maintained at two temperatures, 19°C and 25°C, over a duration of 14 days. Bismuth bioaccumulation occurred in Bi-exposed biofilm concurrently with a pronounced increase in polyunsaturated fatty acids (PUFA), likely as a protective mechanism to preserve cell structure and integrity. Bismuth bioaccumulation also occurred in snails with their FA composition largely reflecting the composition of their dietary source highlighting the direct link between diet and snail FA profiles. Additionally, the antioxidant enzymes studied exhibited diverse responses under Bi exposure and thermal stress, suggesting the induction of oxidative stress in snails. SOD activity increased at 25°C, suggesting a thermal stress. CAT activity remained high under all conditions, unaffected by temperature or Bi exposure. GPx levels increased in snails fed with Bi-laden biofilm, particularly at 19°C. GST activity showed great variability with a significant three-way interaction. The observed elevation in MDA levels among Bi-exposed snails suggested a potential deficiency in their antioxidant enzyme systems, leading to an increased susceptibility to lipid peroxidation. This research highlights the complex interaction between Bi contamination, temperature, and the physiological responses of aquatic organisms, and reveals the need for future research into the environmental impact of Bi in aquatic ecosystems. We further highlight the importance of food for Bi transfer to higher consumers and the importance of considering dietborne exposures in ecotoxicological studies.
- PUFA increased in biofilm exposed to Bi
- Dietary exposure represents the dominant pathway for Bi bioaccumulation in snails
- Bi exposure altered FA composition in snails
- There was an interactive effect of temperature and Bi on snail SFA and PUFA
- Oxidative response and lipid damage occurred in Bi-exposed snails
The use of bismuth (Bi) has recently increased in various industrial applications (e.g., drug manufacturing, cosmetics, hair dye formulations, hunting shots, plumbing fixtures, fishing sinkers, etc.) because it is considered as a non-toxic alternative to lead (Pb) (Fahey and Tsuji, 2006; Wang et al., 2019; Deady et al., 2022). The increased use of Bi has led to increased concentrations of this critical metal in soils and in aquatic ecosystems (Amneklev et al., 2015; Amneklev et al., 2016). Despite the fact that the presence of Bi in natural environments may represent a threat to living organisms, to our knowledge, no studies have focused on the toxic effects of this metal on aquatic organisms.
In addition to stresses caused by contaminants, such as Bi, aquatic ecosystems also face the consequences of climate change, including increasing water temperatures (Staudinger et al., 2021). Global climate models predict a median global warming in air temperature of 2.8°C (2.1°C–3.4°C) degrees by the turn of the next century, and consequently an increase in water temperature in marine and freshwater ecosystems (IPCC, 2023). Temperature stress is expected to increase metabolic rates and oxygen consumption, leading to an increase in reactive oxygen species (ROS) production that can result in oxidant stress to aquatic organisms (Lushchak, 2011). Temperature also affects how living organisms regulate their fatty acid (FA) composition to maintain optimal membrane fluidity (homeoviscous adaptation) and certain cellular functions (Hazel, 1995). Therefore, in addition to its direct impact on biota, thermal stress may also occur concomitantly with other stressors such as metal exposure, and result in complex multi-stressor effects (e.g., additive, synergistic, and antagonistic) on aquatic organisms (Jackson et al., 2016).
Among biological endpoints used to assess environmental stresses, FA profiles and enzyme activity may be used as biomarkers. For example, it has been shown that metal toxicity can directly affect aquatic organisms by altering their metabolic functions, shifting membrane structural and physicochemical properties (i.e., membrane lipid peroxidation), inducing oxidative stress, and damaging cells and tissues (Le Saux et al., 2020). Metals can also alter overall lipid composition and a wide range of aquatic organisms has been used to study the response of FA composition to metal exposure. Indeed, changes in the proportions of saturated FA (SFA), monounsaturated FA (MUFA) and polyunsaturated FA (PUFA), as well as lipid peroxidation have been observed in metal-exposed biofilm (Fadhlaoui et al., 2020), invertebrates and molluscs (Fokina et al., 2013; Silva et al., 2017), and fish (Fadhlaoui and Couture, 2016; Fadhlaoui et al., 2018).
Although aquatic organisms are often exposed to multiple stressors in the environment, most studies focus on a single stressor. In addition, most toxicity experiments evaluate waterborne exposure and exclude the trophic exposure pathway via ingestion of contaminated food, which may therefore underestimate the potential toxicity of a contaminant (Baines and Fisher, 2008; Iheanacho and Odo, 2020a; Iheanacho and Odo, 2020b; Bonnet, 2020). Indeed, the trophic route can also contribute significantly to toxic effects in consumers (Croteau et al., 2007; Ng et al., 2011). Investigating the response of biota to a contaminant exposure through both waterborne and dietary pathways in addition to other stressors therefore more closely resembles realistic exposure conditions encountered in aquatic ecosystems.
In this study, we evaluated individual and interactive effects of Bi and temperature on an aquatic snail consumer of the genus Lymnaea. We considered the effect of a Bi exposure via water as well as via ingestion of Bi-exposed biofilm. Our specific objectives were: 1) to investigate if the trophic route (dietborne) also contributes significantly to Bi bioaccumulation in snails, 2) to assess the trophic linkages between biofilm (diet) and snails (consumer) in terms of FA composition, and 3) to determine the interactive effects of an increased temperature and Bi exposure on snail FA composition, oxidative stress (superoxide dismutase (SOD), catalase (CAT), glutathione peroxidase (GPx) and glutathione S-transferase (GST)) and on lipid peroxidation (malondialdehyde (MDA)).
Biofilm was grown on plexiglass slides submerged in six microcosms filled with 40 L of dechlorinated tap water inoculated with a composite biofilm sample (20 rocks) collected from a nearby stream (Cap Rouge River, Quebec City). The microcosms were maintained at room temperature (19°C) and water circulation was maintained using aquarium pumps. After 1 month of biofilm colonization, three aquaria (40 L) were randomly selected as control biofilm and the other three aquaria were contaminated with Bi using a Bi stock solution (1,000 ± 4 μg/mL, Plasma CAL, SCP Science) to obtain a final concentration of 2 µM Bi. Exposure duration was 21 days and water samples were collected twice per week to verify Bi concentrations. At the end of the experiment, biofilm samples were collected to determine the concentration of Bi bioaccumulated. The remaining biofilm was centrifuged to produce pellets and stored at −80°C for later use as snail food.
Prior to the start of the experiment, snails (weight = 188 ± 28 mg; n = 288) were acclimatized (7 days) to laboratory conditions in a 30-L aquarium filled with dechlorinated tap water that was aerated using an air stone. During the acclimation period, snails were fed with commercial flakes (Nutrafin Max). After acclimation, 12 snails were placed into individual 1.5 L plastic microcosms (n = 24) equipped with air pumps and bubblers, filled with 1 L of dechlorinated tap water and covered with plastic lids. The microcosms were then randomly divided into two temperature treatment groups: one group of 12 microcosms maintained at ambient temperature (19°C), and a second group of 12 microcosms placed in a water bath where the temperature was raised gradually at a rate of 1°C/day to reach 25°C. During the exposure period (14 days), snails from both temperature conditions were fed daily either with control or Bi-exposed biofilm. This experimental design resulted in a set of four conditions (Figure 1):
- Control: water control + food control
- Food Bi: water control + Bi-containing food
- Water Bi: water with added Bi + food control
- Water Bi x Food Bi: Bi-containing water and food
Over the duration of the experiment, water was partially renewed twice per week and water samples were taken to ensure that a concentration of 2 μM Bi was maintained. Temperature as well as mortality (inactive snails for 24 h or empty shells) were verified daily. At the end of the exposure period, a 24-h fasting period was carried out to eliminate the residual food present in the gut and consequently reduce potential non-tissue sources that may influence our assessment of Bi bioavailability. Soft tissues were separated from the shell, immediately frozen in liquid nitrogen, and stored at −80°C.
Snail soft tissues were homogenised in ice-cold homogenisation buffer (pH 7.5; 20 mM 4-(2-hydroxyethyl)-1-piperazineethanesulfonic acid (HEPES); 1 mM ethylenediaminetetraacetic acid (EDTA); 0.1% Triton X-100, Sigma Aldrich Canada) using an Ultra Turrax T25 homogenizer (Janke and Kunkel, IKA-labortechnik, Staufen, Germany). Antioxidant enzymes SOD, CAT and GPx were analyzed in the homogenate using assay kits 706002, 707002 and 703102, respectively (Cayman Chemical Company Inc., Ann Arbor, Michigan United States). Superoxide dismutase activity was determined indirectly by the quantification of the superoxide generated. Catalase activity was determined by following formaldehyde formation. Glutathione peroxidase was also determined indirectly by measuring nicotinamide adenine dinucleotide phosphate (NADP+) concentration. Glutathione S-transferase was measured according to Habig et al. (1974) using 1-chloro-2,4-dinitrobenzene (CDNB) as substrate. Enzyme activities were expressed in term of protein concentration determined according to Bradford, (1976), using bovine serum albumin as standard.
Lipid content in biofilm and snails (soft tissues) was extracted according to Folch et al. (1957). Briefly, the extraction was performed by adding 21 mL of a mixture of chloroform/methanol (1 V/2 V, 0.01% butylated hydroxytoluene (BHT)) to 100 mg of biofilm pellets (after centrifugation) or snail tissues. The chloroform/methanol mixture was adjusted when the weight of the samples was below 100 mg. An additional step of sonication was necessary for biofilm samples to ensure algal cell disruption. After homogenisation, NaCl was added and the mixture was centrifuged to separate polar and non-polar phases. The upper phase (polar) was then esterified (Boron trifluoride (BF3), 4% methanol) at 75°C to obtain fatty acid methyl esters (FAME) detectable with gas chromatography-flame ionization detector (GC-FID, Adgilent Technologies 7890B GC System). A more detailed protocol for FA extraction in biofilm and snails can be found in Fadhlaoui et al. (2020) and in Fadhlaoui and Lavoie, (2021), respectively. FA composition was described as individual FA and summarized in groups based on double bonds which included MUFA with one double bond, PUFA with two or more double bonds and SFA without double bonds; n-3 and n-6 fatty acids are a class of PUFA for which the first double bond is located respectively on the third and sixth carbon, and U:S is the ratio of unsaturated:saturated FA.
Endogenous lipid peroxidation in snail samples was assessed according to the thiobarbituric acid reactive substances (TBARS) method by measuring malondialdehyde (MDA) concentrations. A detailed protocol is available in the assay kit (10009055) from Cayman Chemical Company (Ann Arbor, Michigan United States).
Snail soft tissues and centrifuged biofilm samples were lyophilised and digested in concentrated trace metal grade nitric acid (HNO3) for 48 h followed by concentrated hydrogen peroxide for 24 h. The final sample was diluted with Milli-Q water to reach a final HNO3 concentration of 10% according to the detailed protocol protocol presented in Fadhlaoui et al. (2020). Bismuth concentrations were determined using inductively coupled plasma-mass spectrometry (ICP-MS, Thermo XSeries2). A multi-element calibration standard (C00-061-401, Plasma CAL, SCP Science) was used as an internal control to validate measurements.
A three-way analysis of variance (ANOVA) was used to assess the effect of the three factors (temperature, contamination via food and contamination via water) and their interaction on the biological descriptors (FA profiles, antioxidant enzymes and MDA concentration) as well as on Bi bioaccumulation in snails. A one-way analysis of variance was used to evaluate differences in Bi bioaccumulation in biofilm. Analyses of variance were performed in R (version 4.0.3) using the stats and car packages (Fox and Weisberg, 2019). A principal component analysis (PCA) was conducted to further explore patterns in fatty acid distributions within biofilm and snails as function of temperature and Bi contamination via food and/or water. To facilitate interpretation, the ordination was performed using the most abundant FA in the biofilm and in snails as listed in Supplementary Tables S1–S3. Treatment effects within PCA distributions were evaluated using a one-way permutation multivariate analysis of variance (PERMANOVA) for biofilm and a three-way PERMANOVA for snails. Statistical analyses were performed in R (version 4.0.3) using the vegan package (Oksanen et al., 2020; R Core Team, 2020). Significance was evaluated conservatively from the perspective of the aquatic environment at p < 0.1 to limit the probability of type II error. Data were expressed as mean ± standard deviation of the mean (n = 9 for biofilm samples and n = 6 for snail samples).
Bismuth content was negligible in control biofilm (<0.003 µg Bi/mg dw; n = 5) as opposed to those exposed to Bi for 3 weeks (11.28 ± 0.48 µg Bi/mg dw; n = 5). Earlier studies have also suggested that biofilm could be used as a biological indicator of anthropogenic metal contamination (Hill et al., 2000; Behra et al., 2002). Later laboratory and field studies highlighted positive correlations between biofilm metal content and aqueous metal exposure concentrations (Morin et al., 2008; Thi et al., 2010; Lavoie et al., 2012; Leguay et al., 2016; Fadhlaoui et al., 2020).
Exposure to Bi was found to alter the FA composition of biofilm (Figure 2). The two principal components of the PCA including 27 biofilm FA explained 79.2% of the total variation (PC1 = 57.4%; PC2 = 21.8%), where Bi exposed biofilm was oppositely loaded on both PC axes compared to control biofilm. A one-way PERMANOVA indicated that Bi exposure explained 43.7% (R2 = 0.437) of the variation in Euclidian distances among multivariate biofilm FA profiles. Fatty acid loadings indicated that exposed biofilm was associated with greater abundances of C22:6n3, C18:2n6, C16:1n7, C14:0 and C14:1n5, and lower abundances of C16:0, C17:0, C18:0, and C18:1n9 compared to control biofilm (Figure 2). Bismuth also altered FA composition when individual FA were grouped based on their saturation levels: SFA, MUFA and PUFA (Supplementary Table S1). Bi exposure resulted in a significant increase in biofilm unsaturated FA (F1,4 = 43.9, p = 0.003), particularly PUFAs (F1,4 = 9.6, p = 0.036), including n-3 (F1,4 = 126.9, p < 0.001) and n-6 FAs (F1,4 = 16.3, p = 0.016) which affected the n-3:n-6 ratio (F1,4 = 12.0, p = 0.023). No differences were observed in MUFA, and the proportion of n-9 FA declined in the presence of Bi. Likewise, there was a significant decrease in SFA resulting in a significant increase in the U:S FA ratio when biofilm was exposed to Bi.
FIGURE 2. Principal component analysis (PCA) of FA composition in control biofilm (Water Cont.) and exposed biofilm (Water Bi), (A) bivariate plots of individual FA in biofilm for PC1 versus PC2 and (B) the position of each FA. PCA loadings for only the 10 most influential FA are shown.
The observed FA modifications in the presence of Bi were not consistent with previous findings using nickel which showed that PUFA decreased and SFA increased with increasing nickel concentrations (Fadhlaoui et al., 2020). Similarly, Sun et al. (2015) reported a decrease in PUFA and an increase in SFA (16:0 and 18:0) as well as in MUFA (16:1 and 18:1) in Nannochloropsis sp. when exposed to arsenic, which is also in contradiction with the observations from our study. Algae can adapt their metabolism to cope with stressors such as metal contamination, and the contradictory responses observed may mean that these responses are metal specific.
Under stressed conditions, FA proportions may be modified leading to an alteration in membrane structure. Functionally, PUFA confer flexibility and fluidity to cellular membranes, while SFA provide rigidity and reduce fluidity (Hazel, 1995). In addition, many PUFA polar lipids such as phospholipids are crucial in the photosynthesis process, where changes in FA from this groups may affect the photosynthetic rate (White et al., 2019). The increase in PUFA under Bi-exposed biofilm may thus represent a response to maintain an optimal photosynthetic efficiency. According to Rocha et al. (2020), Selenastrum gracile (Chlorophyceae) increases its photosynthetic capacity under cadmium exposure by increasing PUFA. Cell membrane SFA and MUFA are also integral in a cell’s capacity to cope with contaminant accumulation where higher SFA and MUFA result in a more rigid membrane limiting metal accumulation (de Carvalho and Caramujo, 2018). In our study, the observed increase in Bi accumulation in exposed biofilm is likely related to the increase in PUFA and associated decrease in SFA. This change in FA groups suggests a trade-off in the conformation of cell membrane structure related to an acclimation strategy of photosynthetic efficiency versus membrane fluidity (Rocha et al., 2016). Based on the knowledge that metals can change membrane structure, and in light of the observed modifications in lipid composition under Bi exposure, we suggest that biofilm may use PUFA production as a defense strategy against Bi contamination to maintain photosynthetic activities. Finally, the increase in PUFA production in response to Bi exposure may also be attributed to an adaptive response aimed at countering lipid peroxidation. This was previously proposed by Rocha et al. (2016) in Selenastrum gracile under copper exposure, suggesting a common strategy employed by algae to cope with lipid peroxidation that may occur under oxidative conditions.
Bismuth bioaccumulated in snails under both exposure pathways (Figure 3). The results from the three-way ANOVA showed that no significant interaction was observed among the three experimental factors (temperature, Bi in food and Bi in water). No significant difference was observed between Bi content at 19°C and 25°C, suggesting that temperature does not seem to affect the uptake of Bi either via water or via the trophic route. Water and dietary Bi exposure together showed the highest bioaccumulation in snails, but snails fed with Bi-laden biofilm accumulated more Bi compared to snails exposed to Bi via water only (Figure 3), indicating the relative importance of a dietary exposure in snails. At 19°C, the snail Bi content from the Water Bi x Food Bi treatment was approximately four times higher than the sum of the measured Bi in snails exposed separately to Bi from food or water (Figure 3). Previous studies have demonstrated that the assimilation of metals from a contaminated diet is correlated to ingestion rate and gut passage time. Generally, the assimilation of metals increases with prolonged gut passage time and at a lower ingestion rates (Croteau et al., 2007; Guo et al., 2015). In our study, the observed increase of Bi in snails exposed to the water x food treatment may thus be related to a modification of feeding ratio and to a decrease in ingestion rate. Wang and Ke. (2002) made a similar observation for two marine gastropods exposed to cadmium and zinc. Likewise, Bonnet (2020) found higher bioaccumulation of cadmium in snails exposed through their diet compared to water only. Similar to other metals, both exposure pathways need to be considered for Bi.
FIGURE 3. Snail bismuth content (Lymnaea sp., dry wt) at 19°C and 25°C (n = 6). (1) Control: water control + food control; (2) Food Bi: water control + Bi-containing food; (3) Water Bi: water with added Bi + food control and (4) Water Bi x Food Bi: Bi-containing water and food.
Bismuth was found to alter the FA composition of snails, but effects were conditional on temperature and the exposure pathway. A PCA explained 51.6% of the total variation on two principal components (PC1 = 34.6%; PC2 = 17.0%) (Figure 4). Based on a three-way PERMANOVA, temperature (R2 = 0.103) explained the most variation in Euclidian distances among multivariate FA profiles. Variable FA loadings indicated that snails in the high temperature treatment had greater proportions of C18:3n6, C22:1n9, and C22:2n6 and lower proportion of C16:1n7 compared to those in the low temperature treatment.
FIGURE 4. Principal component analysis (PCA) of FA composition in snails (Lymneae sp.) exposed to one of six treatment combinations of temperature (19°C: light grey, 25°C: dark grey), water Bi contamination (control: circle, Bi: square), and food contamination (control: no overlay, Bi: ‘X’ overlay). (A) Bivariate plots of individual FA in snails for PC1 versus PC2 and (B) the position of each FA. PCA loadings for only the 10 most influential FA are shown.
Differences in FA composition were explained by Bi in the water (R2 = 0.076), but this was conditional on the temperature treatment (R2 = 0.092) where Bi had little effect on snails in the high temperature treatment compared to the low temperature treatment. Dietborne Bi also influenced FA composition but its effects were more nuanced through an interaction with water exposure (R2 = 0.066). Snails fed Bi-laden biofilm had increasingly different FA profiles when exposed to aqueous Bi compared to control. FA profiles that had greater proportions of C18:2n6 and C20:4n6 and lower proportions of C18:0 and C16:0 were largely associated with exposure to Bi.
The FA composition of snails exposed to Bi contaminated food was similar to the FA composition of their food (Bi-laden biofilm), characterized by a low proportion of SFA and a high proportion of PUFA (Figure 5). Several laboratory and field studies have shown that herbivore FA composition is mainly affected by diet FA composition (Goedkoop et al., 2007; Lau et al., 2013; Chaguaceda et al., 2020). For example, Caers et al. (1999) demonstrated that the bivalve Ruditapes philippinarum feeding on a diet rich in 22:6n3 had an increased content of this FA in its tissues. Likewise, Albentosa et al. (1994) and Albentosa et al. (1996) concluded that the decrease in 22:6n3 and 20:5n3 in Ruditapes philippinarum and in Venerupis pullastra fed different microalgal diets (of low PUFA content) was related to a lack of these FA in their diet.
FIGURE 5. Proportions of saturated fatty acid (SFA) (A), polyunsaturated fatty acid (PUFA) (B), monounsaturated fatty acid (MUFA) (C), and ratio Saturated: Unsaturated fatty acid (U:S) (D) in snails under different exposure conditions: (1) Control: water control + food control; (2) Food Bi: water control + Bi-containing food; (3) Water Bi: water with added Bi + food control and (4) Water Bi x Food Bi: Bi-containing water and food.
Three-way analyses of variance revealed complicated interactive responses of FA groups (i.e., SFA, MUFA, PUFA, and U:S ratio) to temperature and Bi exposure (Figure 5). Bismuth in the Food Bi treatment and in the Water Bi x Food Bi treatment appeared to increase the U:S ratio under low temperatures. The increase in this ratio was driven by a corresponding decrease in SFA in these two Bi treatments. The decrease in SFA was matched by an increase in MUFA and PUFA with food and water Bi exposures, respectively. However, the increase in MUFA was stronger in the high temperature treatment and the increase in PUFA was stronger in the low temperature treatment. The observed changes in overall FA composition and the U:S ratio were related to an increase in 18:2n6, 20:2n6 and 20:4n6, and a drastic decrease in 18:0, respectively.
Interactive effects of temperature and Bi exposure were observed in all analyses of variation in snail FA composition (Figures 5A–D). Temperature is known to be an important factor modulating FA cellular composition in aquatic organisms. According to previous studies, an increase in temperature is usually accompanied with a decrease in unsaturation index, i.e., in PUFA and in long chain PUFA (Hazel, 1995). However, in our study, we generally observed a marked increase in unsaturation and higher proportions of C20 PUFA at our highest temperature. It seems that snails may possess a high homeostatic capacity to minimize temperature increase effects and to maintain elevated PUFA content, essential for different functions. At 25°C, the increase in PUFA was related to an increase in 20:4n6 (ARA) and in 20:5n3 (EPA) (Supplementary Tables S2, S3). Crockett (2008) reported that FA with greater unsaturation degrees such as EPA and ARA are more prone to lipid peroxidation and typically decrease with environmental perturbations such as thermal stress and metal contamination. However, in this study, ARA and EPA exhibited an increase in Bi-exposed snails when compared to the control condition (Supplementary Tables S2, S3). The increase we observed in EPA and ARA may thus suggest that these FA play a role in a compensatory adjustment during lipid metabolism in the presence of Bi. This response may be considered as a defense mechanism to protect membranes against damages that can be caused by stressors, such as elevated temperature and metals. It seems that stressful conditions triggered a regulatory mechanism to produce PUFA to maintain functions and structures requiring specific FA (e.g., 20:5n3 and 20:4n6 for membrane structure and fluidity). An induction of elongation/desaturation may be considered as a mechanism to compensate the loss of FA due to lipid peroxidation. Previous studies have shown that changes in lipid composition is a protective strategy to maintain membrane fluidity and related functions such as activities of membrane-bound enzymes, pumps and receptors as well as an inflammatory response (Hazel and Landrey, 1988; Lee, 2004; Hulbert and Else, 2005; Bruno et al., 2007). A similar response, with an increase in certain PUFA, was observed in the sea snail Gibbula umbilicalis exposed to cadmium, nickel and mercury (Silva et al., 2017) as well as in blue mussels Mytilus edulis exposed to cadmium and copper (Fokina et al., 2013). The present study demonstrates that temperature, Bi (via water and diet) and the nutritional quality of the biofilm (in terms of FA composition) affected snails FA profiles differently.
Fatty acids are important biomolecules transferred through aquatic and terrestrial food webs (Torres-Ruiz et al., 2007; Guo et al., 2017; Ohler et al., 2023). Consequently, the FA compositions of living organisms are inherently connected (Guo et al., 2016). For example, highly unsaturated fatty acids (HUFA), such as eicosapentanoic acid (EPA, 20:3n5) and docosahesanoic acid (DHA, 22:6n3), are crucial for several metabolic functions (i.e., growth and reproduction) and the physical structure (i.e., membrane structure) of consumers (Hazel, 1995; Tocher et al., 2003). Through dietary pathways, primary consumers (e.g., aquatic grazers) therefore link lower and upper trophic levels and play a critical role in FA composition within food webs (Brett et al., 2006; Torres-Ruiz et al., 2010; Kelly and Scheibling, 2012; Galloway et al., 2015; Guo et al., 2022).
Stressors affecting the composition of the FA transferred can have an important ecological impact by affecting the overall health and productivity of aquatic and terrestrial ecosystems. To investigate the trophic transfer of FA, we compared FA composition in snails fed with the control biofilm and snails fed with Bi-laden biofilm. We focused our assessment solely on the FA showing the highest proportions in snails (Supplementary Tables S2, S3) as well as on FA that were also observed in the biofilm (Supplementary Table S1). The mean increase in 16:1n7 from 4% to 10% in the biofilm exposed to Bi did not lead to an increase in the proportion of this FA in snails fed with this biofilm (Supplementary Table S1). This observation is comparable to Delaporte et al. (2005) who suggested that snails may modulate the incorporation of certain FA when dietary intake surpasses animals’ requirement.
The PUFA 20:4n6 and 20:5n3 were significantly higher than 22:6n3 in snails. Docosahexaenoic acid (DHA, 22:6n3) is known as a crucial FA for different functions such as development and reproduction. However, it seems that snails in our study required only a low proportion of 22:6n3 for metabolic functions as observed in the sea snail Gibula umbilicalis (Silva et al., 2017). The proportion of 20:5n3 was generally maintained in groups fed on control and Bi-exposed biofilm at 19 and at 25°C, in contrast with ARA and 20:2n6 which greatly increased in snails exposed to Bi through their diet at 19°C and decreased at 25°C (Supplementary Tables S2, S3). According to previous studies, dietary nutritional quality can affect tissue FA composition in different mollusks (Delaporte et al., 2005; Pirini et al., 2007). The results from this study also showed an effect of diet FA composition on grazers, although this response seemed to be strongly affected by temperature (Supplementary Tables S2, S3). At 19°C, snails fed on the Bi-exposed biofilm (rich in PUFA) were rich in 20:4n6 compared to those fed on the control biofilm, suggesting that their FA composition largely reflects their diet composition, as shown in previous studies (Torres-Ruiz et al., 2010; Guo et al., 2016). At 25°C, the significant decrease in 20:4n6 proportions in snails exposed to dietborne Bi compared to snails fed on the control biofilm may be related to an impairment of the FA incorporation process at higher temperatures. This decrease may also be related to a decrease in the desaturation process in response to a decrease in the availability of the precursor 18:2n6 (Supplementary Table S3).
Apart from CAT, enzyme activities in snails varied among treatments, particularly with temperature (Figure 6). A significant fixed effect of temperature was observed for SOD activity where the high temperature treatment resulted in snails with higher SOD activities compared to the low temperature treatment (Figure 6A). An increase in temperature is known to increase physiological processes like metabolic rates and oxygen consumption (Schulte, 2015). Higher temperatures are also prone to induce the overproduction of ROS, thereby increasing the risk of oxidative stress (Lushchak, 2011). SOD is known to sequester ROS, converting it to H2O2, a less harmful molecule that can be offset by other antioxidant enzymes such as CAT, GST and GPx (Iheanacho et al., 2020c). The increased SOD activity observed in snails at 25°C is indicative of a thermal stress response to reduce oxidative damage. A similar SOD response was observed in the soft-shell clam Mya arenaria (Greco et al., 2011), and in the bivalve Perna viridis (Verlecar et al., 2007) as a result of temperature changes. The enzymes CAT and SOD are the first line of defense and act interdependently to remove ROS; the H2O2 produced by SOD is reduced by CAT to water and molecular oxygen (Halliwell, 2006). In our study we observed an elevated CAT activity relative to the other enzyme activities in all conditions with no significant effect of temperature or Bi exposure regime (Figure 6C). Troschinski et al. (2014) also found high invariant CAT activity in Mediterranean snails (Xeropicta derbentina) among temperature treatments. High CAT activity (compared to the other enzymes) and lack of differences among treatments, particularly temperature, suggests that this enzyme is being produced for permanent protection and to maximize the elimination of H2O2 (Troschinski et al., 2014).
FIGURE 6. Effects of temperature and Bi [in water and food] on (A) SOD, (B) GPx (C) CAT and (D) GST activities in snails Lymneae sp. at 19°C and 25°C (n = 6 for each enzyme) under different exposure conditions: (1) Control: water control + food control; (2) Food Bi: water control + Bi-containing food; (3) Water Bi: water with added Bi + food control and (4) Water Bi x Food Bi: Bi-containing water and food.
Despite their similar role as a pro-oxidant, the activities of GST and GPx in snails responded differently to temperature and to Bi treatments (Figures 6B, D). GPx increased in snails fed with Bi exposed biofilm and was more prevalent at 19°C, not differing among any other treatment levels. In contrast, there was a high variability in GST activity with a significant three-way interaction term among treatments. Given this variability, the response of GST to Bi exposure was difficult to generalize, but there appears to be a consistent positive effect of temperature on enzyme activity. Nevertheless, as both enzymes are involved in the elimination of hydroperoxides produced under oxidative stress, their induction suggests increased oxidative damage (Iheanacho et al., 2021). The observed increase in GPx activity in snails exposed to dietborne Bi may be in response to Bi toxicity and the continuous production of O2-. Similar responses were observed in laboratory exposures of bivalves to mercury (Verlecar et al., 2008) and at a field site contaminated with copper, cadmium and lead (Torres et al., 2002). However, GPx activity is often more pronounced in the breakdown of H2O2 at lower concentrations, above which CAT operates to eliminate H2O2 (Molavian et al., 2015; Kurutas, 2016). Given the uniformly elevated CAT activities providing permanent protection, it is not surprising that we did not observe a strong GPx response.
Significant treatment interactions confound the interpretation of the main effects of temperature and Bi on GST activity in snails. This enzyme plays an important role in the detoxification of contaminants (metals, organic pollutants, etc.), and the response of this enzyme to contaminant exposure and natural stressors has been investigated in several organisms (Hellou et al., 2012). Yet, previous studies have shown contradictory responses of this enzyme to metal exposure. For example, an increase in GST activity was observed in a freshwater mussel (Lasmigona costata) over a gradient of metal exposure (e.g., cadmium, nickel, zinc, lead) in a contaminated ecosystem (Machado et al., 2014), while a decrease in GST was observed in a freshwater gastropod (Theba pisana) exposed to copper (Radwan et al., 2010). In our study, both up and downregulation of GST was observed in snails exposed to Bi, thus limiting our understanding. However, GST activity was always higher for the snails maintained at high temperature (Figure 6D). Temperature driven increases in GST may be related to the corresponding increase in SOD activity and the transformation ROS to H2O2 and its subsequent elimination by GST. Thus, the increase in GST activity is probably related to an induction of the elimination metabolism of lipid hydroperoxides formed by the temperature-induced peroxidation of PUFA in cellular membranes.
Lipid peroxidation is a complex chain reaction mediated by ROS leading to the peroxidation of PUFA in cell membranes (Halliwell and Gutteridge, 1984) and where MDA is a final product used as a marker of oxidative stress. In the present study, we observed an effect of temperature as well as an effect of Bi in the water on lipid peroxidation where the highest MDA concentrations were observed in snails maintained at 25°C and in those exposed to aqueous Bi (Figure 7). These results are surprising due to the higher bioaccumulation of Bi in snails exposed via their diet. Several studies showed increased MDA concentrations in molluscs (bivalves) when exposed to metals: Mytillus galloprovincialis exposed to cadmium, mercury and copper (Kournoutou et al., 2020), Ruditapess decussatus exposed to cadmium (Romeo and Gnassiabarelli, 1995) and Perna perna exposed to cadmium and copper (Khati et al., 2012). There appears to be some differences in MDA concentrations between snails exposed through their diet at low temperatures, but the temperature-food interaction term was not significant, thus these results remain equivocal. Similar to previous studies, thermal stress was observed to significantly increase MDA concentrations (Verlecar et al., 2007; Falfushynska et al., 2014). For example, an MDA increase was suggested to result from a decrease in ROS removal capacity due to the decrease in SOD and GPx activities in the ark shell (Scapharca subcrenata) at 32°C compared to those maintained at 17°C and 27°C (Zhao et al., 2016). Verlecar et al. (2007) also observed oxidative damages and lipid peroxidation in Perna viridis with increased temperatures. This response was also observed in feshwater mussels Anodonta anatina sampled in polluted sites which had elevated temperatures (Falfushynska et al., 2014).
FIGURE 7. Malondialdehyde (MDA) concentration in snails (Lymnaea sp.) exposed to Bi (food and water) at 19°C and 25°C (n = 6) under different exposure conditions: (1) Control: water control + food control; (2) Food Bi: water control + Bi-containing food; (3) Water Bi: water with added Bi + food control and (4) Water Bi x Food Bi: Bi-containing water and food.
Despite our observations of significant increases in SOD and GST activities in snails exposed at a high temperature, MDA concentrations were elevated suggesting that the antioxidant capacity of these enzymes were insufficient to remove excess ROS and prevent lipid peroxidation. In our study, no interaction effects of Bi and temperature on MDA were observed. However the observed MDA increase seems to be a consequence of the presence of Bi that may result in a higher sensitivity of snails to temperature-induced oxidative stress. A similar response was observed in the eastern oyster (Crassostrea virginica) exposed to thermal stress and cadmium (Lannig et al., 2006). The clear response of MDA concentrations to thermal stress and Bi exposure further suggests that MDA concentrations may be considered as a more sensitive indicator of oxidative stress in snails compared to antioxidant enzymes activities.
Bismuth was long considered a “green” metal and used in various industrial application as a lead alternative to reduce environmental problems related to metal contamination. Few studies have investigated its toxic effects on aquatic organisms. To our knowledge, this is the first study to examine Bi effects on primary producers (biofilm) and primary consumers (aquatic snails). In addition, Bi effects on lipid composition in biofilm and snails has never been investigated. Despite the fact that the interpretation of certain responses and interactions between factors were not always straightforward, our experimental design mimicked real scenarios involving different biotic and abiotic interactions, and our results highlighted that interactions between stressors enhanced their individual impacts in certain cases.
In our study, we highlighted 1) an increase of PUFA in Bi-exposed biofilm related to Bi bioaccumulation and considered this response as a protective strategy to preserve cell structure and integrity, 2) that FA composition of snails fed control and contaminated biofilm reflected their diet composition, 3) that the four antioxidant enzymes studied responded differently suggesting an induction of oxidative stress under Bi exposure and thermal stress, and 4) an increase in MDA in Bi-exposed snails suggesting a deficiency of antioxidant enzymes to prevent lipid peroxidation. The present study provides novel information about Bi toxicity (via water and food) and temperature stress on lipid composition, antioxidant capacity and lipid peroxidation. Finally, we demonstrated the importance of food as source of Bi to higher consumers. Therefore, the assessment of toxicological effects of metals based only on aqueous exposure seems not to be sufficient in aquatic ecosystems and dietborne exposures should also be considered.
The original contributions presented in the study are included in the article/Supplementary Material, further inquiries can be directed to the corresponding author.
The manuscript presents research on animals that do not require ethical approval for their study.
MF: Conceptualization, Writing–review and editing, Formal Analysis, Investigation, Methodology, Writing–original draft. NP: Formal Analysis, Methodology, Writing–review and editing. IL: Writing–review and editing, Conceptualization, Project administration, Supervision, Validation. CF: Funding acquisition, Project administration, Supervision, Validation, Writing–review and editing, Conceptualization.
The author(s) declare that financial support was received for the research, authorship, and/or publication of this article. This work was supported by a Natural Sciences and Engineering Research Council of Canada (NSERC) Engage grant EGP 542547 - 19 in collaboration with AGAT Laboratories.
The authors would like to thank Virginie Bérubé and Pierre-Yves Robidoux (AGAT Laboratories) for helpful discussions and support in the elaboration of this project, as well as Kim Racine at the Institut national de la recherche scientifique, Centre Eau Terre Environnement for Bi analyses.
The authors declare that the research was conducted in the absence of any commercial or financial relationships that could be construed as a potential conflict of interest.
All claims expressed in this article are solely those of the authors and do not necessarily represent those of their affiliated organizations, or those of the publisher, the editors and the reviewers. Any product that may be evaluated in this article, or claim that may be made by its manufacturer, is not guaranteed or endorsed by the publisher.
The Supplementary Material for this article can be found online at: https://www.frontiersin.org/articles/10.3389/fenvc.2024.1332967/full#supplementary-material
Albentosa, M., Labarta, U., Fernandez-Reiriz, M. J., and Perez Camacho, A. (1996). Fatty acid composition of Ruditapes decussatus spat fed on different microalgae diets. Comp. Biochem. Physiol. A Mol. Integr. Physiol. 113, 113–119. doi:10.1016/0300-9629(95)02041-1
Albentosa, M., Labarta, U., Perezcamacho, A., Fernandez-Reiriz, M. J., and Beiras, R. (1994). Fatty-acid composition of Venerupis pullastra spat fed on different microalgae diets. Comp. Biochem. Physiol. A Mol. Integr. Physiol. 108, 639–648. doi:10.1016/0300-9629(94)90350-6
Amneklev, J., Augustsson, A., Sörme, L., and Bergbäck, B. (2016). Bismuth and silver in cosmetic products: a source of environmental and resource concern? J. Ind. Ecol. 20 (1), 99–106. doi:10.1111/jiec.12251
Amneklev, J., Sörme, L., Augustsson, A., and Bergbäck, B. (2015). The increase in bismuth consumption as reflected in sewage sludge. Water Air Soil Poll. 226 (4), 92. doi:10.1007/s11270-015-2374-x
Baines, S. B., and Fisher, N. S. (2008). Modeling the effect of temperature on bioaccumulation of metals by a marine bioindicator organism, Mytilus edulis. Environ. Sci. Technol. 42, 3277–3282. doi:10.1021/es702336q
Behra, R., Landwehrjohann, R., Vogal, L., Wagner, B., and Sigg, L. (2002). Copper and zinc content of periphyton from two rivers as a function of dissolved metal concentration. Aquat. Sci. 64, 300–306. doi:10.1007/s00027-002-8074-9
Bonnet, M. (2020). Évaluation des effets d’une contamination au cadmium sur la composition en acides gras d’un biofilm et de ses répercussions sur un consommateur primaire (Physa sp.). MSc thesis. Université du Québec. Avaliable at: https://espace.inrs.ca/id/eprint/11371/.112p.
Bradford, M. M. (1976). A rapid and sensitive method for the quantitation of microgram quantities of protein utilizing the principle of protein-dye binding. Anal. Biochem. 72, 248–254. doi:10.1006/abio.1976.9999
Brett, M. T., Muller-Navarra, D. C., Ballantyne, A. P., Ravet, J. L., and Goldman, C. R. (2006). Daphnia fatty acid composition reflects that of their diet. Limnol. Oceanogr. 51, 2428–2437. doi:10.4319/lo.2006.51.5.2428
Bruno, M. J., Koeppe, R. E., and Andersen, O. S. (2007). Docosahexaenoic acid alters bilayer elastic properties. Proc. Natl. Acad. Sci. USA. 104, 9638–9643. doi:10.1073/pnas.0701015104
Caers, M., Coutteau, P., and Sorgeloos, P. (1999). Dietary impact of algal and artificial diets, fed at different feeding rations, on the growth and fatty acid composition of Tapes philippinarum (L.) spat. Aquac 170, 307–322. doi:10.1016/S0044-8486(98)00410-4
Chaguaceda, F., Eklov, P., and Scharnweber, K. (2020). Regulation of fatty acid composition related to ontogenetic changes and niche differentiation of a common aquatic consumer. Oecol 193, 325–336. doi:10.1007/s00442-020-04668-y
Crockett, E. L. (2008). The cold but not hard fats in ectotherms: consequences of lipid restructuring on susceptibility of biological membranes to peroxidation, a review. J. Comp. Physiol. B 178, 795–809. doi:10.1007/S00360-008-0275-7
Croteau, M. N., Luoma, S. N., and Pellet, B. (2007). Determining metal assimilation efficiency in aquatic invertebrates using enriched stable metal isotope tracers. Aquat. Toxicol. 83, 116–125. doi:10.1016/j.aquatox.2007.03.016
Deady, E., Moon, C., Moore, K., Goodenough, K. M., and Shail, R. K. (2022). Bismuth: economic geology and value chains. Ore. Geol. Rev. 143, 104722. doi:10.1016/j.oregeorev.2022.104722
de Carvalho, C. C. C. R., and Caramujo, M. J. (2018). The various roles of fatty acids. Mol 23, 2583. doi:10.3390/molecules23102583
Delaporte, M., Soudant, P., Moal, J., Kraffe, E., Marty, Y., and Samain, J. F. (2005). Incorporation and modification of dietary fatty acids in gill polar lipids by two bivalve species Crassostrea gigas and Ruditapes philippinarum. Comp. Biochem. Physiol. A Mol. Integr. Physiol. 140, 460–470. doi:10.1016/j.cbpb.2005.02.009
Fadhlaoui, M., and Couture, P. (2016). Combined effects of temperature and metal exposure on the fatty acid composition of cell membranes, antioxidant enzyme activities and lipid peroxidation in yellow perch (Perca flavescens). Aquat. Toxicol. 180, 45–55. doi:10.1016/j.aquatox.2016.09.005
Fadhlaoui, M., Laderriere, V., Lavoie, I., and Fortin, C. (2020). Influence of temperature and nickel on algal biofilm fatty acid composition. Environ. Toxicol. Chem. 39, 1566–1577. doi:10.1002/etc.4741
Fadhlaoui, M., and Lavoie, I. (2021). Effects of temperature and glyphosate on fatty acid composition, antioxidant capacity, and lipid peroxidation in the gastropod Lymneae sp. Water 13, 1039. doi:10.3390/w13081039
Fadhlaoui, M., Pierron, F., and Couture, P. (2018). Temperature and metal exposure affect membrane fatty acid composition and transcription of desaturases and elongases in Fathead minnow muscle and brain. Ecotoxicol. Environ. Saf. 148, 632–643. doi:10.1016/j.ecoenv.2017.10.040
Fahey, N. S. C., and Tsuji, L. J. S. (2006). Is there a need to re-examine the approval of bismuth shotshell as a non-toxic alternative to lead based on the precautionary principle? J. Environ. Monit. 8, 1190–1194. doi:10.1039/b612603k
Falfushynska, H., Gnatyshyna, L., Yurchak, I., Ivanina, A., Stoliar, O., and Sokolova, I. (2014). Habitat pollution and thermal regime modify molecular stress responses to elevated temperature in freshwater mussels (Anodonta anatina: unionidae). Sci. Total Environ. 500, 339–350. doi:10.1016/j.scitotenv.2014.08.112
Fokina, N. N., Ruokolainen, T. R., Nemova, N. N., and Bakhmet, I. N. (2013). Changes of blue mussels Mytilus edulis L. lipid composition under cadmium and copper toxic effect. Biol. Trace Elem. Res. 154, 217–225. doi:10.1007/s12011-013-9727-3
Folch, J., Lees, M., and Sloane Stanley, G. H. (1957). A simple method for the isolation and purification of total lipids from animal tissues. J. Biol. Chem. 226, 497–509. doi:10.1016/S0021-9258(18)64849-5
Fox, J., and Weisberg, S. (2019). An R companion to applied aegression. California: SAGE Publications, Inc.
Galloway, A. W., Brett, M. T., Holtgrieve, G. W., Ward, E. J., Ballantyne, A. P., Burns, C. W., et al. (2015). A fatty acid based bayesian approach for inferring diet in aquatic consumers. PLOS One 10, e0129723. doi:10.1371/journal.pone.0129723
Goedkoop, W., Demandt, M., and Ahlgren, G. (2007). Interactions between food quantity and quality (long-chain polyunsaturated fatty acid concentrations) effects on growth and development of Chironomus riparius. Can. J. Fish. Aquat. Sci. 64, 425–436. doi:10.1139/f07-016
Greco, L., Pellerin, J., Capri, E., Garnerot, F., Louis, S., Fournier, M., et al. (2011). Physiological effects of temperature and a herbicide mixture on the soft-shell clam Mya arenaria (Mollusca, Bivalvia). Environ. Toxicol. Chem. 30, 132–141. doi:10.1002/etc.359
Guo, F., Bunn, S. E., Brett, M. T., and Kainz, M. J. (2017). Polyunsaturated fatty acids in stream food webs - high dissimilarity among producers and consumers. Freshw. Biol. 62, 1325–1334. doi:10.1111/fwb.12956
Guo, F., Ebm, N., Fry, B., Bunn, S. E., Brett, M. T., Ouyang, X., et al. (2022). Basal resources of river food webs largely affect the fatty acid composition of freshwater fish. Sci. Total Environ. 812, 152450. doi:10.1016/j.scitotenv.2021.152450
Guo, F., Kainz, M. J., Sheldon, F., and Bunn, S. E. (2016). Effects of light and nutrients on periphyton and the fatty acid composition and somatic growth of invertebrate grazers in subtropical streams. Oecol 181, 449–462. doi:10.1007/s00442-016-3573-x
Guo, Z. Q., et Guo, Z. Q., Zhang, W., Zhou, Y. Y., Gao, N., and Zhang, L. (2015). Feeding ratio and frequency affects cadmium bioaccumulation in black sea bream Acanthopagrus schlegeli. Aquac. Env. Interac. 7, 135–145. doi:10.3354/aei00140
Habig, W. H., Pabst, M. J., and Jakoby, W. B. (1974). Glutathione S-transferases. J. Biol. Chem. 249, 7130–7139. doi:10.1016/s0021-9258(19)42083-8
Halliwell, B. (2006). Reactive species and antioxidants. Redox biology is a fundamental theme of aerobic life. Plant Physiol. 141, 312–322. doi:10.1104/pp.106.077073
Halliwell, B., and Gutteridge, J. M. C. (1984). Oxygen toxicity, oxygen radicals, transition metals and disease. Biochem. J. 219, 1–14. doi:10.1042/bj2190001
Hazel, J. R. (1995). Thermal adaptation in biological-membranes - is homeoviscous adaptation the explanation. Annu. Rev. Physiol. 57, 19–42. doi:10.1146/Annurev.Ph.57.030195.000315
Hazel, J. R., and Landrey, S. R. (1988). Time course of thermal adaptation in plasma membranes of trout kidney. II. Molecular species composition. Am. J. Physiol. 255, 628–634. doi:10.1152/ajpregu.1988.255.4.R628
Hellou, J., Ross, N. W., and Moon, T. W. (2012). Glutathione, glutathione S-transferase, and glutathione conjugates, complementary markers of oxidative stress in aquatic biota. Environ. Sci. Pollut. Res. 19, 2007–2023. doi:10.1007/s11356-012-0909-x
Hill, W. R., Bednarek, A. T., and Larsen, I. L. (2000). Cadmium sorption and toxicity in autotrophic biofilms. Can. J. Fish. Aquat. Sci. 57, 530–537. doi:10.1139/cjfas-57-3-530
Hulbert, A. J., and Else, P. L. (2005). Membranes and the setting of energy demand. J. Exp. Biol. 208, 1593–1599. doi:10.1242/jeb.01482
Iheanacho, S. C., Adeolu, A., Nwose, R., Ekpenyong, J., Offu, P., Amadi-Eke, A., et al. (2021). Genotoxicity, oxidative stress and lysozyme induction in Clarias gariepinus chronically exposed to water-soluble fraction of burnt tire ash. Ecotoxicol 30, 1983–1996. doi:10.1007/s10646-021-02474-7
Iheanacho, S. C., and Odo, G. E. (2020a). Dietary exposure to polyvinyl chloride microparticles induced oxidative stress and hepatic damage in Clarias gariepinus (Burchell, 1822). Environ. Sci. Pollut. Res. 27, 21159–21173. doi:10.1007/s11356-020-08611-9
Iheanacho, S. C., and Odo, G. E. (2020b). Neurotoxicity, oxidative stress biomarkers and haematological responses in African catfish (Clarias gariepinus) exposed to polyvinyl chloride microparticles. Comp. Biochem. Phys. C-Toxicol. Pharmacol. 232, 108741. doi:10.1016/j.cbpc.2020.108741
Iheanacho, S. C., Odo, G. E., and Ezewudo, B. I. (2020c). Adulteration of aquafeed with melamine and melamine-formaldehyde chemicals; Ex situ study of impact on haematology and antioxidant systems in Clarias gariepinus. Aquac. Res. 52, 2078–2084. doi:10.1111/are.15059
IPCC (2023). “Climate change 2023,” in Synthesis report. Contribution of working groups I, II and III to the sixth assessment report of the intergovernmental panel on climate change. Editors H. Lee, and J. Romero (Geneva, Switzerland: IPCC), 35–115.
Jackson, M. C., Loewen, C. J. G., Vinebrooke, R. D., and Chimimba, C. T. (2016). Net effects of multiple stressors in freshwater ecosystems: a meta-analysis. Glob. Change Biol. 22, 180–189. doi:10.1111/gcb.13028
Kelly, J. R., and Scheibling, R. E. (2012). Fatty acids as dietary tracers in benthic food webs. Mar. Ecol. Prog. Ser. 446, 1–22. doi:10.3354/meps09559
Khati, W., Ouali, K., Mouneyrac, C., and Banaoui, A. (2012). Metallothioneins in aquatic invertebrates: their role in metal detoxification and their use in biomonitoring. Energy Procedia 18, 784–794. doi:10.1016/j.egypro.2012.05.094
Kournoutou, G. G., Giannopoulou, P. C., Sazakli, E., Leotsinidis, M., Kalpaxis, D. L., and Dinos, G. P. (2020). Oxidative damage of mussels living in seawater enriched with trace metals, from the viewpoint of proteins expression and modification. Toxics 8, 89. doi:10.3390/toxics8040089
Kurutas, E. B. (2016). The importance of antioxidants which play the role in cellular response against oxidative/nitrosative stress: current state. Nutr. J. 15, 71. doi:10.1186/s12937-016-0186-5
Lannig, G., Flores, J. F., and Sokolova, I. M. (2006). Temperature-dependent stress response in oysters, Crassostrea virginica: pollution reduces temperature tolerance in oysters. Aquat. Toxicol. 79, 278–287. doi:10.1016/j.aquatox.2006.06.017
Lau, D. C. P., Goedkoop, W., and Vrede, T. (2013). Cross-ecosystem differences in lipid composition and growth limitation of a benthic generalist consumer. Limnol. Oceanogr. 58, 1149–1164. doi:10.4319/lo.2013.58.4.1149
Lavoie, I., Lavoie, M., and Fortin, C. (2012). A mine of information: benthic algal communities as biomonitors of metal contamination from abandoned tailings. Sci. Total. Environ. 425, 231–241. doi:10.1016/j.scitotenv.2012.02.057
Lee, A. G. (2004). How lipids affect the activities of integral membrane proteins. Biochim. Biophys. Acta. 1666, 62–87. doi:10.1016/j.bbamem.2004.05.012
Leguay, S., Lavoie, I., Levy, J. L., and Fortin, C. (2016). Using biofilms for monitoring metal contamination in lotic ecosystems: the protective effects of hardness and pH on metal bioaccumulation. Environ. Toxicol. Chem. 35, 1489–1501. doi:10.1002/etc.3292
Le Saux, A., David, E., Betoulle, S., Bultelle, F., Rocher, B., Barjhoux, I., et al. (2020). New insights into cellular impacts of metals in aquatic animals. Environ 7, 46. doi:10.3390/environments7060046
Lushchak, V. I. (2011). Environmentally induced oxidative stress in aquatic animals. Aquat. Toxicol. 101, 13–30. doi:10.1016/j.aquatox.2010.10.006
Machado, A. A. D., Wood, C. M., Bianchini, A., and Gillis, P. L. (2014). Responses of biomarkers in wild freshwater mussels chronically exposed to complex contaminant mixtures. Ecotoxicol 23, 1345–1358. doi:10.1007/s10646-014-1277-8
Molavian, H., Tonekaboni, A. M., Kohandel, M., and Sivaloganathan, S. (2015). The synergetic coupling among the cellular antioxidants gutathione peroxidase/peroxiredoxin and other antioxidants and its effect on the concentration of H2O2. Sci. Rep. 5, 1–8. doi:10.1038/srep13620
Morin, S., Duong, T. T., Dabrin, A., Coynel, A., Herlory, O., Baudrimont, M., et al. (2008). Long-term survey of heavy-metal pollution, biofilm contamination and diatom community structure in the Riou Mort watershed, South-West France. Environ. Pollut. 151, 532–542. doi:10.1016/j.envpol.2007.04.023
Ng, T. Y. T., Pais, N. M., and Wood, C. M. (2011). Mechanisms of waterborne Cu toxicity to the pond snail Lymnaea stagnalis: physiology and Cu bioavailability. Ecotoxicol. Environ. Saf. 74, 1471–1479. doi:10.1016/j.ecoenv.2011.03.010
Ohler, K., Schreiner, V. C., Martin-Creuzburg, D., and Schäfer, R. B. (2023). Trophic transfer of polyunsaturated fatty acids across the aquatic-terrestrial interface: an experimental tritrophic food chain approach. Ecol. Evol. 13, 9927. doi:10.1002/ece3.9927
Oksanen, J., Blanchet, G., Friendly, R., Kindt, P., Legendre, D., McGlinn, P. R., et al. (2020). Vegan: community ecology package R package version 2. Avaliable at: https://github.com/vegandevs/vegan.
Pirini, M., Manuzzi, M. P., Pagliarani, A., Trombetti, F., Borgatti, A. R., and Ventrella, V. (2007). Changes in fatty acid composition of Mytilus galloprovincialis (Lmk) fed on microalgal and wheat germ diets. Biochem. Physiol. B Comp. Biochem. 147, 616–626. doi:10.1016/j.cbpb.2007.04.003
Radwan, M. A., El-Gendy, K. S., and Gad, A. F. (2010). Oxidative stress biomarkers in the digestive gland of Theba pisana exposed to heavy metals. Arch. Environ. Contam. Toxicol. 58, 828–835. doi:10.1007/s00244-009-9380-1
R Core Team (2020). R: a language and environment for statistical computing. Vienna, Austria: R Foundation for Statistical Computing.
Rocha, G. S., Parrish, C. C., and Espindola, E. L. G. (2020). Shifts in photosynthetic parameters and lipid production of the freshwater microalga Selenastrum gracile (Chlorophyceae) under cadmium exposure. J. Appl. Phycol. 32, 4047–4055. doi:10.1007/s10811-020-02255-5
Rocha, G. S., Parrish, C. C., Lombardi, A. T., and Melo, M. D. G. (2016). Copper affects biochemical and physiological responses of Selenastrum gracile (Reinsch). Ecotoxicol 25, 1468–1477. doi:10.1007/s10646-016-1698-7
Romeo, M., and Gnassiabarelli, M. (1995). Metal distribution in different tissues and in subcellular-fractions of the mediterranean clam Ruditapes decussatus treated with cadmium, copper, or zinc. Comp. Biochem. Phys. 111, 457–463. doi:10.1016/0742-8413(95)00060-7
Schulte, P. M. (2015). The effects of temperature on aerobic metabolism: towards a mechanistic understanding of the responses of ectotherms to a changing environment. J. Exp. Biol. 218, 1856–1866. doi:10.1242/jeb.118851
Silva, C. O., Simoes, T., Novais, S. C., Pimparel, I., Granada, L., Soares, A. M. V. M., et al. (2017). Fatty acid profile of the sea snail Gibbula umbilicalis as a biomarker for coastal metal pollution. Sci. Total Environ. 586, 542–550. doi:10.1016/j.scitotenv.2017.02.015
Staudinger, M. D., Lynch, A. J., Gaichas, S. K., Fox, M. G., Gibson-Reinemer, D., Langan, J. A., et al. (2021). How does climate change affect emergent properties of aquatic ecosystems? Fish 46, 423–441. doi:10.1002/fsh.10606
Sun, J., Cheng, J., Yang, Z. B., Li, K., Zhou, J. H., and Cen, K. F. (2015). Microstructures and functional groups of Nannochloropsis sp cells with arsenic adsorption and lipid accumulation. Bioresour. Technol. 194, 305–311. doi:10.1016/j.biortech.2015.07.041
Thi, T. D., Morin, S., Coste, M., Herlory, O., Feurtet-Mazel, A., and Boudou, A. (2010). Experimental toxicity and bioaccumulation of cadmium in freshwater periphytic diatoms in relation with biofilm maturity. Sci. Total. Environ. 408, 552–562. doi:10.1016/j.scitotenv.2009.10.015
Tocher, D. R., Agaba, M., Hastings, N., and Teale, A. J. (2003). “Biochemical and molecular studies of the polyunsaturated fatty acid desaturation pathway in fish,” in Big fish bang: proceedings of the 26th annual larval fish conference (Bergen, Norway: THE BIG FISH BANG), 211–227.
Torres, M. A., Testa, C. P., Gaspari, C., Masutti, M. B., Panitz, C. M. N., Curi-Pedrosa, R., et al. (2002). Oxidative stress in the mussel Mytella guyanensis from polluted mangroves on Santa Catarina Island, Brazil. Mar. Pollut. Bull. 44, 923–932. doi:10.1016/s0025-326x(02)00142-x
Torres-Ruiz, M., Wehr, J. D., and Perrone, A. A. (2007). Trophic relations in a stream food web: importance of fatty acids for macroinvertebrate consumers. J. N. Am. Benthol. Soc. 26, 509–522. doi:10.1899/06-070.1
Torres-Ruiz, M., Wehr, J. D., and Perrone, A. A. (2010). Are net-spinning caddisflies what they eat? An investigation using controlled diets and fatty acids. J. N. Am. Benthol. Soc. 29, 803–813. doi:10.1899/09-162.1
Troschinski, S., Dieterich, A., Krais, S., Triebskorn, R., and Kohler, H. R. (2014). Antioxidant defence and stress protein induction following heat stress in the mediterranean snail Xeropicta derbentina. J. Exp. Biol. 217, 4399–4405. doi:10.1242/jeb.113167
Verlecar, X. N., Jena, K. B., and Chainy, G. B. N. (2007). Biochemical markers of oxidative stress in Perna viridis exposed to mercury and temperature. Chem. Biol. Interact. 167, 219–226. doi:10.1016/j.cbi.2007.01.018
Verlecar, X. N., Jena, K. B., and Chainy, G. B. N. (2008). Modulation of antioxidant defences in digestive gland of Perna viridis (L), on mercury exposures. Chemosphere 71, 1977–1985. doi:10.1016/j.chemosphere.2007.12.014
Wang, R., Hongyan, L., and Hongzhe, S. (2019). Bismuth: environmental pollution and health effects. Encycl. Enviro. Health. 415–423. doi:10.1016/B978-0-12-409548-9.11870-6
White, D. A., Rooks, P. A., Kimmance, S., Tait, K., Jones, M., Tarran, G. A., et al. (2019). Modulation of polar lipid profiles in Chlorella sp. in response to nutrient limitation. Metabolites 9, 39. doi:10.3390/metabo9030039
Keywords: antioxidant enzymes, biofilm, bismuth, fatty acids, lipid peroxidation, snails
Citation: Fadhlaoui M, Pearce NJT, Lavoie I and Fortin C (2024) Interactive effects of bismuth exposure (water and diet) and temperature on snail fatty acid composition, antioxidant enzymes and lipid peroxidation. Front. Environ. Chem. 5:1332967. doi: 10.3389/fenvc.2024.1332967
Received: 03 November 2023; Accepted: 12 February 2024;
Published: 26 February 2024.
Edited by:
Željka Fiket, Ruđer Bošković Institute, CroatiaReviewed by:
Isabelle A. M. Worms, University of Geneva, SwitzerlandCopyright © 2024 Fadhlaoui, Pearce, Lavoie and Fortin. This is an open-access article distributed under the terms of the Creative Commons Attribution License (CC BY). The use, distribution or reproduction in other forums is permitted, provided the original author(s) and the copyright owner(s) are credited and that the original publication in this journal is cited, in accordance with accepted academic practice. No use, distribution or reproduction is permitted which does not comply with these terms.
*Correspondence: Mariem Fadhlaoui, bWFyaWVtLmVjb3RveEBnbWFpbC5jb20=
†These authors have contributed equally to this work
Disclaimer: All claims expressed in this article are solely those of the authors and do not necessarily represent those of their affiliated organizations, or those of the publisher, the editors and the reviewers. Any product that may be evaluated in this article or claim that may be made by its manufacturer is not guaranteed or endorsed by the publisher.
Research integrity at Frontiers
Learn more about the work of our research integrity team to safeguard the quality of each article we publish.