- Man-Technology-Environment Research Centre (MTM), Örebro University, Örebro, Sweden
Perfluorooctane sulfonic acid (PFOS) has been produced in large quantities for the use in various applications. As a consequence, PFOS is ubiquitous in the environment. Managing transportation of PFOS requires a clear understanding of PFOS mobilization in soils and their interactions with different soil components. The current study investigated a pH-dependent sorption of PFOS isomers onto soil and the effect of dissolved humic substances and Na2SO4. Sorption experiments of PFOS isomers was conducted on top and subsoils to assess their capacity to retain PFOS. Topsoil and subsoil samples were sampled from two areas in Kvarntorp, Kumla, Sweden. Sorption experiments were performed by shaking a mixture of soil and soil solutions spiked with PFOS isomers. One way ANOVA showed that linear PFOS (L-PFOS) and branched PFOS (Br-PFOS) isomers showed different sorption behavior onto soils. Calculated logarithmic partition coefficients revealed that L-PFOS is readily sorbed onto soils sampled at area 1) at very low pH (<4.5) whereas it was the least sorbed onto soils collected at area 2) under the studied pH range. Electrostatic interactions governed the sorption of PFOS isomers during acidic conditions whereas other mechanism controlled the sorption during neutral to alkaline conditions. The presence of humic acid enhanced the sorption of all PFOS isomers whereas fulvic acid inhibited their sorption onto soil. Sorption results revealed that the topsoil had a high capacity to sorb all PFOS isomers compared to the subsoil from the same area. For soils collected from an area covered by young oak trees, a high sorption on the topsoil was attributed to a high organic content [measured as loss on ignition (LOI)] and cation exchange capacity (CEC). However, there was no correlation between sorption capacity and LOI or CEC content for soil collected from another area covered by spruce forest. This suggested that the sorption on latter soils was controlled by other physicochemical properties.
Introduction
As a result of using materials containing per- and polyfluoroalkyl substances (PFAS), soil contaminated by these compounds is reported globally (Nakayama et al., 2019; Kurwadkar et al., 2021). The highest PFAS contaminations are reported at sites contaminated by point sources such as the use of aqueous film forming foams (AFFF) for firefighting (Langberg et al., 2021; Nickerson et al., 2021). AFFF mainly contained PFOS that was manufactured via electrochemical fluorination (ECF). The ECF produced a mixture of linear (L-PFOS) and branched (Br-PFOS) isomers with up to 70% and 30% proportion respectively. Although PFOS was banned from production and use in AFFF, these chemicals are persistent in the environment. Hekster et al. (2002) showed that the estimated half-life of PFOS in the environment is 41 years, and this time can be even longer. This implies that PFOS still exists in soil if no remediation was undertaken. PFAS existing in soil may be mobilized and lead to the contamination of natural waters. Consequently, several aquatic systems such as rivers and groundwaters are reported to contain high concentration of PFOS isomers (Schulz et al., 2020; Ali et al., 2021; Nickerson et al., 2021). Numerous adverse effects associated with inhaling of PFOS from contaminated water are reported (Andersson et al., 2019; Zeng et al., 2019; Zeeshan et al., 2021). Toxicological studies have shown that high exposure to PFOS may cause various health effects including alteration to immune system, liver and kidney diseases, adverse reproduction and developmental effects and cancer (Fenton et al., 2021). To be able to halt these adverse effects caused by PFOS, it is very crucial to fully understand the behavior of PFOS isomers in different materials through which human life could be exposed to these chemicals.
Managing bioavailability of PFAS in soil requires a clear understanding of PFAS mobilization and their interactions with different soil components. The authors acknowledge previous efforts done to help understand the effect of different parameters such as increased PFAS sorption as pH decreases (Campos-Pereira et al., 2020; Gagliano et al., 2020; Lu et al., 2016; Uwayezu et al., 2019, Uwayezu et al., 2022), increasing PFAS sorption in presence of dissolved organic carbon (Higgins and Luthy, 2006; Uwayezu et al., 2019; Gagliano et al., 2020), enhancement of PFAS sorption in the presence of divalent metal cations (Wang et al., 2012), decreased sorption in the presence of anions (Uwayezu et al., 2019; Schulz et al., 2020) and the contribution of soil minerals (Milinovic et al., 2015; Li et al., 2018; Liu et al., 2020; Nguyen et al., 2020).
Though several studies reported different distribution behavior of PFOS in soils, available literature do not provide a clear understanding about the behavior of PFOS isomers in soils. PFAS isomers exhibits difference in distribution behavior as a result of their physicochemical properties such as hydrophobicity and water solubility (Gao et al., 2019). Kärrman et al. (2011) reported different distribution of PFAS isomers in soil from a training facility that used an AFFF. Schulz et al. (2020) also mentioned the difference in distribution of PFOS isomers between soil and water. However, soils contain dissolved natural organic matters and other inorganic substances, which could affect the soil capacity governing the mobility of PFOS isomers. Our previous studies have shown that dissolved humic substances and sulfate may affect the distribution of PFOS isomers to goethite and hydrargillite (Uwayezu et al., 2019, Uwayezu et al., 2022). Currently, it is not fully understood how substances present in soils affect the transport of PFOS isomers in soils. Thus, a systematic investigation of the sorption of PFOS isomers to soil would add more knowledge about the mobility and bioavailability of PFOS in the environment.
This study was conducted to 1) investigate the distribution of L-PFOS and Br-PFOS isomers of PFOS between soils and water 2) to evaluate the effect of soil depth on the distribution of PFOS isomers in soil and 3) to understand the pH-dependent sorption of PFOS isomers in soils and the effect of humic substances and sulfate ions. We hypothesize that the PFOS isomers might exhibit different sorption pattern due to their physical properties whereas presence of humic substances might enhance the capacity of a soil via hydrophobic mechanisms.
Materials and Methods
Materials
Potassium salt of PFOS containing linear PFOS and branched PFOS isomers was obtained from Sigma Aldrich. Sodium hydroxide (NaOH) and hydrochloric acid (HCl) were also obtained from VWR (>99%). Fulvic acid (Siikajoki) with an average molecular weight around 1 400 Da and an acid capacity of 5.15 meq g−1 was extracted from humic substances according to Pettersson et al. (1997). A humic acid (Aldrich soil humic acid) (technical grade) with a molecular weight of around 3,400 Da was obtained from Sigma-Aldrich (Chemie GmbH, Germany). Ammonium acetate (> 99%) was purchased from Fluka (Steinheim, Germany). Organic solvents such as LC-grade methanol (99.9%) and acetonitrile (>99.9%) were bought from Fisher Scientific (Leicestershire, United Kingdom) and in Fisher Scientific, United States, respectively.
The raw water used to perform sorption experiment was collected from Lake Vättern (south of Stora Hammarsundet). Physicochemical properties of Lake Vättern water is provided in our previous publications (Uwayezu et al., 2019, Uwayezu et al., 2022). Four different soils were sampled in Kvarntorp, Kumla, Sweden. The first two soils were collected at an area covered by young oak trees; the first soil being collected at the surface after removing soil covers (topsoil) and the second soil at 25 cm depth (subsoil) using a shovel. These two soils are referred to as topsoil (1) and subsoil (1). The other two soils referred to as topsoil (2) and subsoil (2) were sampled at another area covered with spruce forest, one soil at the surface and one 25 cm below the surface.
Batch Tests
Spiked solutions used in sorption experiments were prepared following procedures published in our recent works (Uwayezu et al., 2019, Uwayezu et al., 2022). Briefly, 3 mg of PFOS salt containing linear and branched isomers was dissolved in 25 L of Lake Vättern water and mixed using a stirrer to ensure complete dissolution. The soils were dried at 60°C for 3 days and homogenized using a mortar. Sieves with a screen of 0.5 mm was used to obtain the fine particles from the soils that were being used during the batch experiments.
Sorption experiments were carried out in 50 ml polypropylene tubes containing 0.25 g soil. Before each batch test, solution chemistry was modified by separate additions of humic acid (HA), fulvic acid (FA) and Na2SO4. A final concentration of 20 mg/L FA or HA solutions were used whereas final concentration of Na2SO4 was 100 or 1,000 mg/L after addition. pH was adjusted to between 2.5 and 11 using either HCl or NaOH. The rationale behind the selection of levels of added chemicals was discussed in details in our previous studies (Uwayezu et al., 2019, Uwayezu et al., 2022). Previous studies have shown that sorption of PFOS to soil particles reached equilibrium in 24 h (Milinovic et al., 2015; Campos Pereira et al., 2018). Therefore, suspensions containing PFOS isomers, soils and added chemicals were shaken for 24 h. During each series of sorption experiment a positive control (i.e., sorption experiment with PFOS spiked water and without adsorbent) and a negative control [i.e., sorption experiment with only MilliQ water (18.2 MΩ)] were used to make sure that there was no PFOS loss or contamination. After shaking, suspensions were centrifuged at 5,432 g for 15 min, and filtered through 0.45 μm syringe filters. The supernatants were stored for PFAS and other chemical analysis. For each batch of tests, a procedural blank (MilliQ water) and a quality control (PFOS spiked solution) were conducted in parallel, but no electrolyte and sorbent were included.
Perfluorooctane Sulfonic Acid Quantification and Other Measurements
Concentration of PFOS isomers in the supernatants was measured using an ultra-high-performance liquid chromatography coupled to tandem mass spectrometry (UPLC-MS/MS). The used UPLC-MS/MS was Waters Acquity Ultra-performance Liquid chromatograph coupled to a triple quadrupole mass spectrometers XEVO-TQS (UPLC/MS/MS, Waters Corp, Milford, MA, United States) equipped with a 100 mm × 2.1 mm C18 BEH column (1.7-μm particle size). Chromatographic separation conditions were adopted from our previous studies (Uwayezu et al., 2019, Uwayezu et al., 2022). The L-PFOS was detected as an individual isomer whereas Br-PFOS isomers were quantified in two groups namely 3/4/5-PFOS and 6/2-PFOS.
Equilibrium pH and electrical conductivity were measured using a 744 PH Meter Ω Metrohm and a sensIONTM + EC7 respectively. An ICP-MS Agilent 7500 was used for the measurement of metal concentrations in the supernatants. Briefly, 1 ml aliquot of each solution was filtered using a 0.2 μm Millipore nylon filter and diluted with 9 ml of 1% HNO3. Diluted solutions were then spiked with rhodium (103Rh) internal standard before aspiration into the ICP-MS.
Loss on ignition (LOI) was performed by weighing in 5 g of each soil sample in crucibles and employing 550°C for 1 h in a muffle furnace. A solution of ammonium acetate (1 mol L−1) at pH 6.95 was used to determine the cation exchange capacity (CEC) capacity of the soil samples (Burt, 2011). CEC was determined by measuring the amount of extractable calcium, magnesium, sodium and potassium.
Data Analysis
The amount of PFOS isomers sorbed to soils was determined by subtracting the amount of PFOS isomers in the supernatant at equilibrium from the initially spiked PFOS amount. It was assumed that PFOS lost from the solutions was adsorbed to the soil in suspension. A partition coefficient of PFOS between the aqueous phase and the soil was calculated using Eq. 1. All determined partitioning coefficients were transformed into their logarithmic form.
The analysis of variance (one way ANOVA) computed using Minitab 19 was used to evaluate the statistical difference in sorption capacity of different soils and different PFOS isomers. In this approach, duplicate sorption tests were carried out on four unmodified soils. The averages of partition coefficients (Kd) were determined to elaborate the difference in sorption behavior at 95% confidence interval.
The evaluation of sorption behavior of PFOS isomers as a function of pH and added chemicals was performed using scatter charts plotted using Microsoft Excel program.
Results and Discussion
Soil Characterization
The measured physicochemical properties of the four soil samples are presented in Table 1. In general, the soils collected from area (1) were higher in magnitude of all measured parameters. To estimate soil organic carbon (SOC), the LOI was measured. The LOI may be used to determine the SOC when a rough estimate is needed (Jensen et al., 2018). The current study showed that topsoil (1) and subsoil (1) had 9% and 6% LOI respectively. The LOI was the same (3%) for topsoil (2) and subsoil (2). Following the expression (SOC = 0.39*LOI-0.28) found by Jensen et al. (2018), the estimated SOC values are given in Table 1. The EC was the same for soils (1) and was relatively higher for subsoil (2) compared to topsoil (2). On the other hand, CEC was higher for subsoil (1) compared to topsoil (1) and was the same for soils (2).

TABLE 1. Physicochemical characteristics of the used soils. EC, electrical conductivity; LOI, loss on ignition, CEC (cation exchange capacity) and pH.
Soils collected at area (2) were characterized by equal LOI content (3%) and CEC (0.03 meq/100 g) and were both more acidic than the soils sampled at area (1).
Despite that elemental composition analysis was not performed on the soils, the measurement of metal concentration in soil leachates revealed that there was a higher leaching of metals such as Al, Ba, Ca, Fe, Mg and Mn from topsoil (1) compared to subsoil (1) and from subsoil (2) compared to topsoil (2). The extent of dissolved metals in solutions are considered to be an indicator of metal oxide content in soil (Schwertmann, 1991; Lainer et al., 2008). As such, the aluminum and iron minerals were slightly higher in topsoil (1) compared to subsoil (1) and in subsoil (2) compared to topsoil (2).
Other soil properties such as texture and zero point of charge (pHpzc) were not measured in the current study. However, based on previous studies, we believed that the present soils were of glacial till type, with the pHpzc in the range 7.0 ± 2.5 (Olsson, 1999; Al-Hamdan and Reddy, 2006).
Distribution of Perfluorooctane Sulfonic Acid Isomers on Soils
The PFOS isomeric profile in the spiked solutions was 78.8% L-PFOS, 8.6% 3/4/5-PFOS and 10.6% 6/2-PFOS based on 19-F NMR analysis provided in the certificate of analysis, which was similar to PFOS manufactured using ECF process. The concentration of PFOS isomers in solutions that were in contact with unmodified soils (no added chemicals) showed that the proportion of linear and branched PFOS slightly changed (i.e., 84% L-PFOS, 6% 3/4/5-PFOS and 11% 6/2-PFOS). This was probably due to the L-PFOS and Br-PFOS which exhibited different sorption behavior on the investigated soils. The distribution behavior of PFOS isomers on the four studied soils is shown in Figure 1. The results showed that the logarithmic values of partition coefficients of PFOS isomers varied from log Kd = 1 to log Kd = 2.
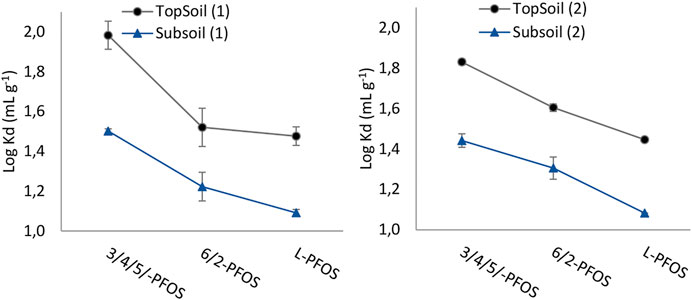
FIGURE 1. Logarithmic partitioning coefficients for PFOS isomers on soils. The dots represent the minimum and maximum values (n = 2). The pH in solutions were 5.5 ± 0.4.
In general, the vertical spread of PFOS isomers in soils showed that the sorption of all PFOS isomers were higher onto the topsoils compared to the soils sampled at 25 cm below the ground. A drop in log Kd from topsoil to subsoil (1) was 1.5 to 1.1, 1.5 to 1.4, and 2.0 to 1.5 for L-PFOS, 6/2-PFOS and 3/4/5-PFOS, respectively. The corresponding variation in partition coefficient in soils collected at area (2) was 1.4 to 1.1, 1.6 to 1.4, and 1.8 to 1.3 for L-PFOS, 6/2-PFOS and 3/4/5-PFOS, respectively.
The soils collected from the area covered with young oak trees (area 1) had different composition with respect to LOI, EC and CEC (Table 1). For these soils, there was an observable correlation between the partitioning coefficients of PFOS isomers and soil content in SOC or CEC. For instance, the average sorption ability of topsoil (1) (high SOC and low CEC) was about 1.4 (log Kd) higher that the sorption capacity of subsoil (1). The observed PFOS partitioning behavior regarding CEC was expected as the CEC measures soil ability to exchange cations. Given that PFOS are anionic during the studied pH values, the soil ability to hold PFOS is inversely proportional to the CEC (Li et al., 2018).
The soils sampled from the area covered with spruce forest did not display any differences in SOC and CEC. Even though the topsoil (2) and subsoil (2) were equivalent in SOC and CEC composition, they displayed differences in sorption capacity of PFOS isomers. The current behavior may indicate that the sorption may be controlled by other factors besides electrostatic and hydrophobic mechanisms. It was demonstrated that not only the organic matter of soil adequately explains the partitioning of PFOS on soil but also other physicochemical properties of soil have influence on the sorption behavior (Milinovic et al., 2015). Previous studies have shown a positive correlation between the distribution of PFOS and soil mineral content (Hellsing et al., 2016; Li et al., 2019; Liu et al., 2020). In the current study, metal concentration in the solutions that were in contact with the soils was measured as indicators of Fe and Al mineral content of the soils (Table 2). However, these indicators could not help to understand the observed sorption pattern. The sorption of PFOS isomers was higher onto topsoil (2) which showed lower levels of Fe and Al in solution. The lack of consistency in the PFOS isomers distribution onto soils (2) could indicate that the soils contained significant amounts of other components not measured in our study and which may have affected the sorption.
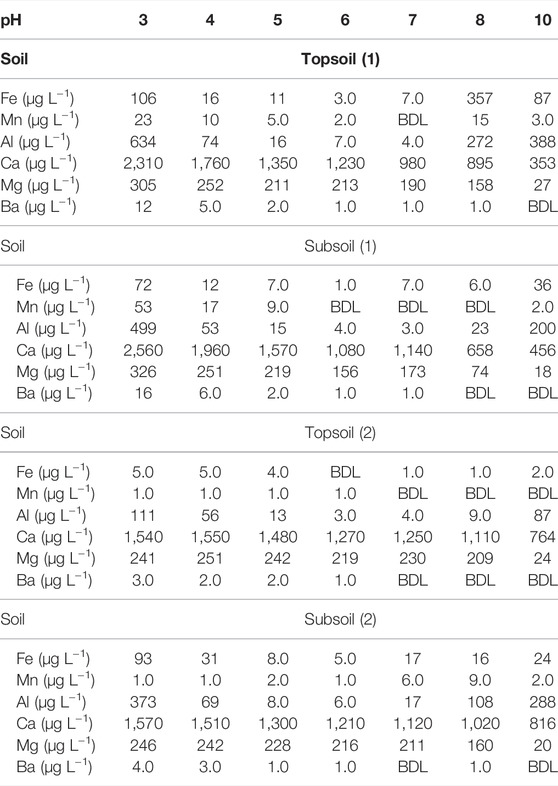
TABLE 2. Metal concentration in solutions that were in contact with the four studied soils at different pH. BDL, below detection limit.
Moreover, comparing soils (1) and soils (2), the order of their sorption capacity toward the sorption of PFOS was topsoil (1) > topsoil (2) > subsoil (2) and subsoil (1). Obviously, the relatively higher sorption capacity of topsoil (1) could be associated to the higher SOC (or LOI) content. The lower capacity of subsoil (1) in comparison to other soils was probably due to its higher CEC.
In order to evaluate the difference or similarity in sorption behavior between L-PFOS and Br-PFOS isomers, one way ANOVA using Tukey comparison was used to test the difference of means. The ANOVA outputs showed that there is a statistical difference in means of logarithmic partition coefficients between the two groups of Br-PFOS isomers (i.e., 6/2-PFOS and 3/4/5/-PFOS) or Br-PFOS and L-PFOS (except for a few cases (Supplementary Table S1). The results in Figure 1 indicate that the group of 3/4/5-PFOS isomers has the highest sorption whereas the L-PFOS was the least sorbed. The order in sorption was 3/4/5-PFOS > 6/2-PFOS > L-PFOS. It is known that PFOS isomers exhibit differences in hydrophobicity properties that lead to variation in their distribution behavior (Schulz et al., 2020). The current PFOS distribution behavior reveals that the Br-PFOS isomers are more readily sorbed onto soil compared to the linear PFOS, which is consistent with their distribution onto goethite or hydrargillite sorbents during the same pH ranges (Uwayezu et al., 2019, Uwayezu et al., 2022). Notably, the current distribution of PFOS isomers was dissimilar to observations from a previous study, which reported that the Br-PFOS are likely to be enriched in the water phase (Kärrman et al., 2011). As the pH in the study performed by Kärrman et al. (2011) was not measured, it can be speculated that difference in soil pH could lead to differences in the distribution of L-PFOS and Br-PFOS isomers. In our previous studies, the sorption of L-PFOS dominated at very acidic conditions (pH < 4.5) (Uwayezu et al., 2019, Uwayezu et al., 2022).
The partition coefficients of PFOS isomers as a function of pH revealed that coefficients for L-PFOS were only slightly higher than those for Br-PFOS during acidic conditions (pH < 4.5) on the soil collected from area (1). At pH > 4.5, the coefficients for Br-PFOS became slightly higher (Figure 2). This indicates that acidic conditions disfavored the leaching of linear PFOS whereas neutral to alkaline conditions favored sorption of Br-PFOS isomers on this type of soil. Soil organic matter gets more protonated at low pH, which enhances the sorption via electrostatic and hydrophobic mechanisms (Nguyen et al., 2020). Thus, we can speculate that one likely reason for the observed higher sorption of L-PFOS during acidic conditions was stronger hydrophobic interactions caused by higher hydrophobicity character of L-FOS. L-PFOS was the least sorbed onto soil (2) under all investigated pH. This is the opposite distribution behavior compared to soils (1) and that previously reported onto hydrargillite/goethite (Uwayezu et al., 2019, Uwayezu et al., 2022). The reason for this is not known; however, if the observed behavior of L-PFOS onto soils (1) (higher sorption at pH < 4.5) was caused by the high SOC content, one of the reasons that could explain the distribution of L-PFOS onto soils (2) could be the low SOC content.
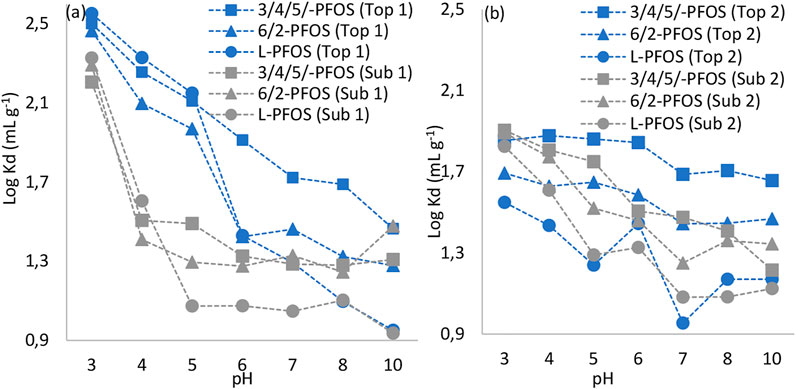
FIGURE 2. Logarithmic sorption coefficients of PFOS isomers onto soils as a function of pH: (A) sub and top soils taken at the area (1) and (B) sub and top soils taken at the area (2).
In summary, the current PFOS isomeric profile on the four investigated soils is consistent with several other studies, which showed that structural isomers of PFOS display different distribution on soil and other environmental matrices (Kärrman et al., 2011; Gao et al., 2019; Schulz et al., 2020).
Effect of pH and Chemical Additives on the Sorption of Linear Perfluorooctane Sulfonic Acid Isomers
The sorption behavior of L-PFOS as a function of pH and in the presence of humic substances or sulfate is plotted in Figure 3. It is noteworthy that the soils collected from area (1) which display differences in measured physicochemical properties are the only one taken into consideration during the paragraph presented here. The analysis of partitioning coefficients showed that the sorption of L-PFOS on the two soils (1) was high during acidic conditions and become less during neutral to alkaline conditions (Figures 3A,B). To easily illustrate the trending, averaged partitioning coefficient were plotted versus acidic [pH 2.5–5.5], neutral [pH 6.0–7.0] and alkaline conditions [pH 7.0–10.5] (Supplementary Figure S1). pH excluded in the intervals were not measured in any of the performed tests. The current sorption behavior of L-PFOS as a function of pH is consistent with the behavior of other PFAS compounds (Du et al., 2014; Nguyen et al., 2020). Based on the study by Al-Hamdan and Reddy, (2006), it is estimated that the pHpzc of the current soils is in the 7.0 ± 2.5 range. Thus, at pH < 7.0 ± 2.5, the soils bear positive charges which attract L-PFOS carrying negative charges. A decrease in sorption during an increase in pH is attributed to a decrease in net positive charges on the soil surface. Though the soil surface is expected to be negatively charged at pH > 7.0 ± 2.5, sorption of L-PFOS was still observed. This is probably due to other mechanisms such as hydrophobicity, ligand exchange, or a metal bridging effect which control the sorption of PFAS in the absence of electrostatic interactions (Milinovic et al., 2015; Wei et al., 2017).
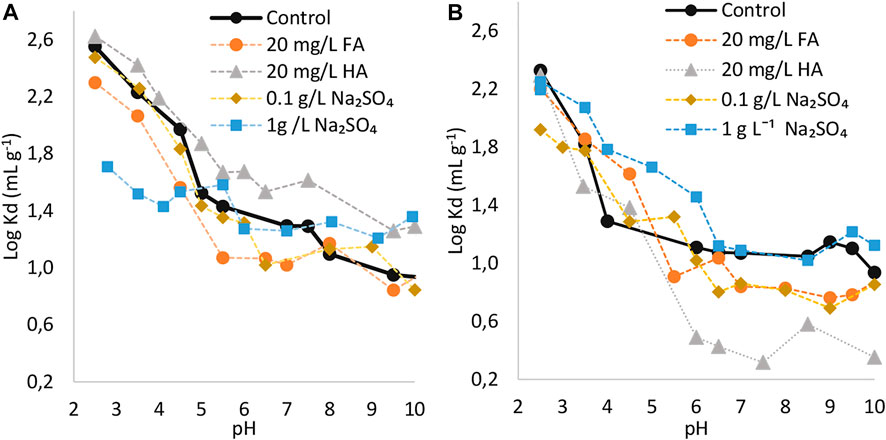
FIGURE 3. Logarithmic sorption coefficients of L-PFOS on (A) topsoil (1) and (B) subsoil (1) as a function of pH and effect of dissolved humic substances or sodium sulfate.
The effect of pH on the sorption of PFOS varies among added analytes (Du et al., 2014). Humic substances, which are abundant substances of soil organic matter influence the transport of PFAS in soils (Campos Pereira et al., 2018). In the present study, the presence of HA and FA has affected the sorption capacity of L-PFOS to topsoil (Figures 3A,B). HA enhanced the sorption of L-PFOS on topsoil. The observed results were expected since HA has larger molecular weight (3,400 Da) compared to FA (average molecular weight around 1,400 Da). The current sorption behavior of L-PFOS onto soil and in the presence of HA was consistent with its sorption behavior reported onto goethite and hydrargillite under similar conditions (Uwayezu et al., 2019, Uwayezu et al., 2022). The observed sorption enhancement in the presence of HA during acidic conditions could be linked to added hydrophic interactions besides existing electrostatic interactions.
Nevertheless, the presence of HA enhanced the sorption of L-PFOS during neutral to alkaline conditions. As previous discussed in our previous study (Uwayezu et al., 2019), the presence of divalent metal cations (e.g., Ca2+ or Mg2+) leaching from the soil (or initially present in Lake Vättern water) could induce the sorption of HA on soil and ultimately enhance the sorption of L-PFOS via hydrophobic mechanisms. Note that the presence of HA reduced the sorption of L-PFOS on subsoil for unknown reasons. On the other hand, the presence of FA reduced the sorption in the entire pH range. One possible explanation is that the FA has a low molecular weight and thus might have competed with the sorption of L-PFOS on soil. The current behavior of L-PFOS distribution on soil in the presence of FA was similar to the distribution on hydrargillite (Uwayezu et al., 2022).
Sorption of L-PFOS was influenced by addition of Na2SO4. The presence of Na2SO4 increased the ionic strength of the solution (Supplementary Table S2). It was demonstrated that changes in ionic strength affects the sorbent properties by reducing effective charges on the surface (Cai et al., 2022). In the current study the presence of Na2SO4 in the solutions decreased the sorption of L-PFOS at about pH < 5. This could be explained by decreased positive charges during acidic conditions which ultimately decreased the electrostatic attraction between the anionic L-PFOS and a positively charged surface. At increased pH (>5), an increased sorption was observed in 1 g L−1 Na2SO4 solutions. This could be due to a reduction in negative charges on the soil surface, which ultimately reduces a repulsion between the surface and PFOS molecules. Alternatively, similar to the PFOS sorption behavior in the presence of sulfate salts observed in previous studies (Zhang et al., 2011; Uwayezu et al., 2019, Uwayezu et al., 2022), the current decreased sorption of L-PFOS at pH < 5 could be due to a competition for binding sites on soil between L-PFOS and SO42− during acidic conditions. However, as observed for the sorption of L-PFOS on subsoil and in the presence of humic substances, the sorption behavior of L-PFOS on subsoil and in the presence of Na2SO4 was dissimilar to the sorption behavior on the topsoil and other studies for unclear reasons. The present results showed that the presence of SO42− (i.e., 1 g L−1 Na2SO4 solution) decreased the sorption of L-PFOS during acidic conditions (pH < 5) by 0.8 order of magnitude. A such inhibition of PFOS sorption by the presence of SO42− was also found in sorption studies carried out onto goethite and hydrargillite (Uwayezu et al., 2019, Uwayezu et al., 2022).
To better describe the vertical mobility of L-PFOS from the topsoil to the soil at 25 cm below the surface, ratios of logarithmic coefficient between the two soils were determined (Table 3). A ratio higher than one unit (>1) implied that L-PFOS was less mobile in the topsoil.
Based upon the determined ratios, the L-PFOS was more retained in the topsoil during nearly all environmental pH conditions. When any chemical additive was made, the L-PFOS isomer was always higher than unit and was the highest in the presence of humic acid.
Effects of pH and Chemical Additives on the Sorption of Branched Perfluorooctane Sulfonic Acid Isomers
As observed from the sorption behavior of L-PFOS, the sorption of Br-PFOS was higher during acidic conditions and decreased after shifting experimental conditions from acidic to alkaline. The curves for logarithmic coefficients of 6/2-PFOS isomers as a function of pH are plotted in Figures 4A,B. Similarly, in Figure 5 the sorption logarithmic coefficients of 3/4/5-PFOS as function of pH are plotted. Average values of logarithmic coefficients for 6/2-PFOS and 3/4/5-PFOS isomers in in acidic, neutral and alkaline conditions are shown in Supplementary Figure S1.
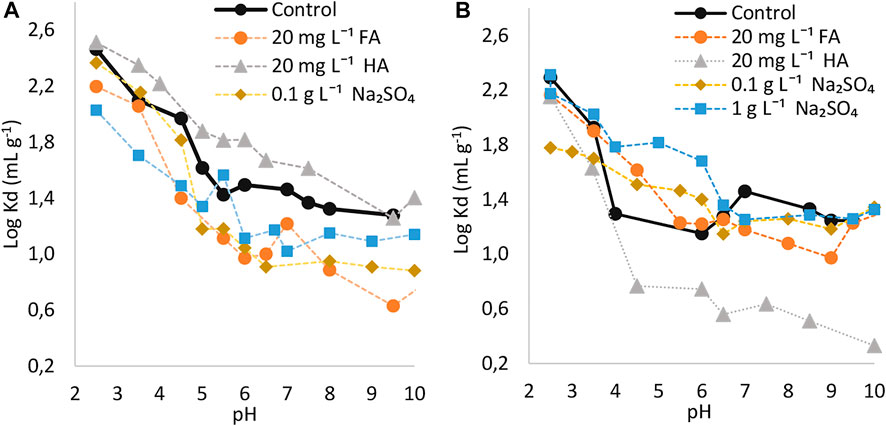
FIGURE 4. Logarithmic sorption coefficients of 6/2-PFOS on (A) topsoil (1) and (B) subsoil (1) as a function of pH and effect of dissolved humic substances or sodium sulfate. Control without adding any additives.
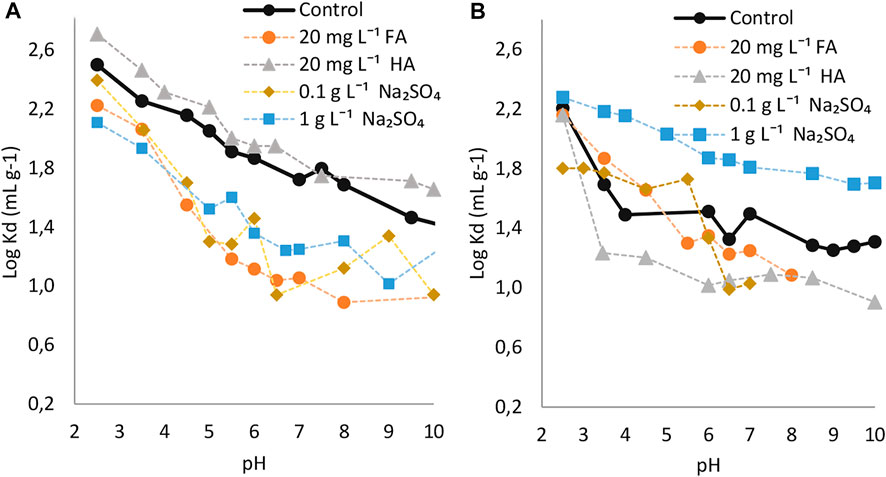
FIGURE 5. Logarithmic sorption coefficients of 3/4/5-PFOS on (A) topsoil (1) and (B) subsoil (1) as function of pH and effect of dissolved humic substances or sodium sulfate. Control without adding any additives.
In comparison with the L-PFOS, the Br-PFOS was sorbed to a lower degree during acidic conditions (Figures 2A,B). For instance, for tests carried out on topsoil (1), the respective averaged logarithmic coefficients were 2.29, 2.18 and 2.34 for 3/4/5-PFOS, 6/2-PFOS and L-PFOS in the pH range 2.5–4.5. On the other hand, at increased pH, the sorption of Br-PFOS became higher than that of L-PFOS (e.g., averaged log Kd were 1.89, 1.50 and 1.29 for 3/4/5-PFOS, 6/2-PFOS and L-PFOS respectively in the 5.0–7.0 pH range).
Similar to the sorption of L-PFOS or other PFAS compounds, the electrostatic interactions were the most important mechanisms through which the Br-PFOS were sorbed to the soils during acidic conditions (Uwayezu et al., 2019, Uwayezu et al., 2022). As mentioned in earlier paragraphs, other mechanisms resulting from the soil properties might have controlled the observed sorption of Br-PFOS during alkaline conditions. Besides electrostatic interactions, Br-PFOS could sorb to soils via hydrophobicity, ligand exchange, or metal bridging effect mechanisms, which as described in prior studies (Milinovic et al., 2015; Wei et al., 2017).
The presence of HA enhanced the sorption of both 3/4/5-PFOS and 6/2-PFOS groups of isomers on the topsoil (1) whereas the FA decreased the sorption. Regarding the sorption behavior on subsoil (1), the presence of both HA and FA decreased the sorption of Br-PFOS under the entire investigated pH range. In general, the presence of humic acids in the solutions containing PFOS and soil exhibited the same effect on the sorption of Br-PFOS as it was observed for L-PFOS. However, the magnitude in sorption enhancement was slightly higher for the L-PFOS. The average log Kd was increased by 1.08, 1.14 and 1.16 for 3/4/5-PFOS, 6/2-PFOS and L-PFOS respectively when 20 mg L−1 HA solutions containing PFOS isomers were used under natural pH of the current studied soils (approximately pH 4.5).
In contrast to the L-PFOS, which only sorbed less during acidic conditions when Na2SO4 was added in solutions, the sorption of two groups of identified Br-PFOS isomers was decreased under the entire range of investigated pH in the presence of Na2SO4. The reason for decreased sorption for Br-PFOS in neutral to alkaline conditions is not well known. The current behavior may indicate that the presence of Na2SO4 or increasing ionic strength disfavored the sorption of Br-PFOS, however, this phenomenon should be further investigated for better understanding.
To further investigate the mobility of Br-PFOS from the topsoil to subsoil and assess their transport to groundwater, ratios between log Kd were again determined (Table 4). The results showed that the ratios were higher than a unit (>1) when no substance was added in the solutions and the ratios for 3,4,5-PFOS were higher than those for the 6/2-PFOS group. This may again indicate that the Br-PFOS are more retained in the topsoil whereas the 3,4,5-PFOS group is more readily sorbed on topsoil than the 6/2-PFOS group. Here again, the presence of HA increased the ratio for the two groups of isomers in the entire investigated pH range.
Conclusion
The present study investigated the sorption behavior of PFOS isomers onto natural soils. The results indicated that L-PFOS and Br-PFOS display differences in sorption onto soils. The L-PFOS was readily sorbed at low pH whereas the Br-PFOS isomers sorbed more during nearly neutral and alkaline conditions. The soil content of LOI and CEC influenced the sorption of PFOS isomers onto soil collected from one area (1) while no correlation between sorption behavior and the same parameters for soil collected from another sampling area (2) was found. This suggested that besides hydrophobic interactions caused by LOI contents of soil, other physicochemical properties of soils influence the sorption behavior. The partition coefficients of PFOS isomers on soils varied among added chemicals. Our study showed that the presence of HA decreased the leaching of PFOS isomers from the topsoil (1) whereas, the FA increased it. In the presence of SO42, the sorption of PFOS isomers was decreased under the whole studied pH range.
A comparison of sorption capacity between the topsoil and subsoil showed that all investigated PFOS isomers were more retained on the topsoil than on the subsoil. Difference in soil properties was found to be one of the reasons of the difference in sorption capacity. Indeed, the results showed that the presence of HA enhances the sorption of PFOS isomers on the topsoils. The current study highlighted that the sorption of PFOS isomers onto the subsoil was inconsistent from that of PFOS isomers on the topsoil for unknown reasons. Thus, additional work is needed to further investigate the role of components of subsoils on the sorption of PFOS. Of particular importance would be to test the sorption/desorption kinetic of PFOS isomers onto all soil layers to explore the mechanisms and assess their transport to groundwater.
Data Availability Statement
The raw data supporting the conclusion of this article will be made available by the authors, without undue reservation.
Author Contributions
J-NU: Investigation, methodology, writing -manuscript, LY: Conceptualization, methodology, manuscript editing, MB: Conceptualization, methodology, manuscript editing.
Conflict of Interest
The authors declare that the research was conducted in the absence of any commercial or financial relationships that could be construed as a potential conflict of interest.
Publisher’s Note
All claims expressed in this article are solely those of the authors and do not necessarily represent those of their affiliated organizations, or those of the publisher, the editors and the reviewers. Any product that may be evaluated in this article, or claim that may be made by its manufacturer, is not guaranteed or endorsed by the publisher.
Acknowledgments
The authors would like to acknowledge the Knowledge Foundation (KKS) for funding the project within the Enforce Research Project (20160019).
Supplementary Material
The Supplementary Material for this article can be found online at: https://www.frontiersin.org/articles/10.3389/fenvc.2022.905170/full#supplementary-material
References
Al-Hamdan, A. Z., and Reddy, K. R. (2006). Adsorption of Heavy Metals in Glacial till Soil. Geotech. Geol. Eng. 24, 1679–1693. doi:10.1007/s10706-005-5560-6
Ali, A. M., Langberg, H. A., Hale, S. E., Kallenborn, R., Hartz, W. F., Mortensen, Å.-K., et al. (2021). The Fate of Poly- and Perfluoroalkyl Substances in a Marine Food Web Influenced by Land-Based Sources in the Norwegian Arctic. Environ. Sci. Process. Impacts 23, 588–604. doi:10.1039/D0EM00510J
Andersson, E. M., Scott, K., Xu, Y., Li, Y., Olsson, D. S., Fletcher, T., et al. (2019). High Exposure to Perfluorinated Compounds in Drinking Water and Thyroid Disease. A Cohort Study from Ronneby, Sweden. Environ. Res. 176, 108540. doi:10.1016/j.envres.2019.108540
Cai, W., Navarro, D. A., Du, J., Ying, G., Yang, B., McLaughlin, M. J., et al. (2022). Increasing Ionic Strength and Valency of Cations Enhance Sorption through Hydrophobic Interactions of PFAS with Soil Surfaces. Sci. Total Environ. 817, 152975. doi:10.1016/j.scitotenv.2022.152975
Campos Pereira, H., Ullberg, M., Kleja, D. B., Gustafsson, J. P., and Ahrens, L. (2018). Sorption of Perfluoroalkyl Substances (PFASs) to an Organic Soil Horizon - Effect of Cation Composition and pH. Chemosphere 207, 183–191. doi:10.1016/j.chemosphere.2018.05.012
Campos-Pereira, H., Kleja, D. B., Sjöstedt, C., Ahrens, L., Klysubun, W., and Gustafsson, J. P. (2020). The Adsorption of Per- and Polyfluoroalkyl Substances (PFASs) onto Ferrihydrite Is Governed by Surface Charge. Environ. Sci. Technol. 54, 15722–15730. doi:10.1021/acs.est.0c01646
Du, Z., Deng, S., Bei, Y., Huang, Q., Wang, B., Huang, J., et al. (2014). Adsorption Behavior and Mechanism of Perfluorinated Compounds on Various Adsorbents-A Review. J. Hazard. Mater. 274, 443–454. doi:10.1016/j.jhazmat.2014.04.038
Fenton, S. E., Ducatman, A., Boobis, A., DeWitt, J. C., Lau, C., Ng, C., et al. (2021). Per‐ and Polyfluoroalkyl Substance Toxicity and Human Health Review: Current State of Knowledge and Strategies for Informing Future Research. Environ. Toxicol. Chem. 40, 606–630. doi:10.1002/etc.4890
Gagliano, E., Sgroi, M., Falciglia, P. P., Vagliasindi, F. G. A., and Roccaro, P. (2020). Removal of Poly- and Perfluoroalkyl Substances (PFAS) from Water by Adsorption: Role of PFAS Chain Length, Effect of Organic Matter and Challenges in Adsorbent Regeneration. Water Res. 171, 115381. doi:10.1016/j.watres.2019.115381
Gao, Y., Liang, Y., Gao, K., Wang, Y., Wang, C., Fu, J., et al. (2019). Levels, Spatial Distribution and Isomer Profiles of Perfluoroalkyl Acids in Soil, Groundwater and Tap Water Around a Manufactory in China. Chemosphere 227, 305–314. doi:10.1016/j.chemosphere.2019.04.027
Hekster, F. M., De Voogt, P., Pijnenburg, A. M. C. M., and Laane, R. W. P. M. (2002). Perfluoroalkylated Substances: Aquatic Environmental Assessment. Rapportnr.
Hellsing, M. S., Josefsson, S., Hughes, A. V., and Ahrens, L. (2016). Sorption of Perfluoroalkyl Substances to Two Types of Minerals. Chemosphere 159, 385–391. doi:10.1016/j.chemosphere.2016.06.016
Higgins, C. P., and Luthy, R. G. (2006). Sorption of Perfluorinated Surfactants on Sediments. Environ. Sci. Technol. 40, 7251–7256. doi:10.1021/es061000n
Jensen, J. L., Christensen, B. T., Schjønning, P., Watts, C. W., and Munkholm, L. J. (2018). Converting Loss-On-Ignition to Organic Carbon Content in Arable Topsoil: Pitfalls and Proposed Procedure. Eur. J. Soil Sci. 69, 604–612. doi:10.1111/ejss.12558
Kärrman, A., Elgh-Dalgren, K., Lafossas, C., and Møskeland, T. (2011). Environmental Levels and Distribution of Structural Isomers of Perfluoroalkyl Acids after Aqueous Fire-Fighting Foam (AFFF) Contamination. Environ. Chem. 8, 372–380. doi:10.1071/EN10145
Kurwadkar, S., Dane, J., Kanel, S. R., Nadagouda, M. N., Cawdrey, R. W., Ambade, B., et al. (2022). Per- and Polyfluoroalkyl Substances in Water and Wastewater: A Critical Review of Their Global Occurrence and Distribution. Sci. Total Environ. 809, 151003. doi:10.1016/j.scitotenv.2021.151003
Lainer, Y. A., Gorichev, I. G., Tuzhilin, A. S., and Gololobova, E. G. (2008). Dissolution Mechanism of Aluminum Hydroxides in Acid Media. Russ. Metall. 2008, 294–300. doi:10.1134/S0036029508040046
Langberg, H. A., Arp, H. P. H., Breedveld, G. D., Slinde, G. A., Høiseter, Å., Grønning, H. M., et al. (2021). Paper Product Production Identified as the Main Source of Per- and Polyfluoroalkyl Substances (PFAS) in a Norwegian Lake: Source and Historic Emission Tracking. Environ. Pollut. 273, 116259. doi:10.1016/j.envpol.2020.116259
Li, F., Fang, X., Zhou, Z., Liao, X., Zou, J., Yuan, B., et al. (2019). Adsorption of Perfluorinated Acids onto Soils: Kinetics, Isotherms, and Influences of Soil Properties. Sci. Total Environ. 649, 504–514. doi:10.1016/j.scitotenv.2018.08.209
Li, Y., Oliver, D. P., and Kookana, R. S. (2018). A Critical Analysis of Published Data to Discern the Role of Soil and Sediment Properties in Determining Sorption of Per and Polyfluoroalkyl Substances (PFASs). Sci. Total Environ. 628-629, 110–120. doi:10.1016/j.scitotenv.2018.01.167
Liu, Y., Qi, F., Fang, C., Naidu, R., Duan, L., Dharmarajan, R., et al. (2020). The Effects of Soil Properties and Co-contaminants on Sorption of Perfluorooctane Sulfonate (PFOS) in Contrasting Soils. Environ. Technol. Innovation 19, 100965. doi:10.1016/j.eti.2020.100965
Lu, X., Deng, S., Wang, B., Huang, J., Wang, Y., and Yu, G. (2016). Adsorption Behavior and Mechanism of Perfluorooctane Sulfonate on Nanosized Inorganic Oxides. J. Colloid Interface Sci. 474, 199–205. doi:10.1016/j.jcis.2016.04.032
Milinovic, J., Lacorte, S., Vidal, M., and Rigol, A. (2015). Sorption Behaviour of Perfluoroalkyl Substances in Soils. Sci. Total Environ. 511, 63–71. doi:10.1016/j.scitotenv.2014.12.017
Nakayama, S. F., Yoshikane, M., Onoda, Y., Nishihama, Y., Iwai-Shimada, M., Takagi, M., et al. (2019). Worldwide Trends in Tracing Poly- and Perfluoroalkyl Substances (PFAS) in the Environment. TrAC Trends Anal. Chem. 121, 115410. doi:10.1016/j.trac.2019.02.011
Nguyen, T. M. H., Bräunig, J., Thompson, K., Thompson, J., Kabiri, S., Navarro, D. A., et al. (2020). Influences of Chemical Properties, Soil Properties, and Solution pH on Soil-Water Partitioning Coefficients of Per- and Polyfluoroalkyl Substances (PFASs). Environ. Sci. Technol. 54, 15883–15892. doi:10.1021/acs.est.0c05705
Nickerson, A., Rodowa, A. E., Adamson, D. T., Field, J. A., Kulkarni, P. R., Kornuc, J. J., et al. (2021). Spatial Trends of Anionic, Zwitterionic, and Cationic PFASs at an AFFF-Impacted Site. Environ. Sci. Technol. 55, 313–323. doi:10.1021/acs.est.0c04473
Olsson, M. (1999). “Soil Survey in Sweden,” in EUR18991-Soil Resources of Europe. Rep. No. 6. Editors P. Bullock, R. J. A. Jones, and L. Montanarella (Luxembourg: Office for Official Publications of the European Communities).
Pettersson, C., Allard, B., and Borén, H. (1997). River Discharge of Humic Substances and Humic-Bound Metals to the Gulf of Bothnia. Estuar. Coast. Shelf Sci. 44, 533–541. doi:10.1006/ecss.1996.0159
Schulz, K., Silva, M. R., and Klaper, R. (2020). Distribution and Effects of Branched versus Linear Isomers of PFOA, PFOS, and PFHxS: A Review of Recent Literature. Sci. Total Environ. 733, 139186. doi:10.1016/j.scitotenv.2020.139186
Schwertmann, U. (1991). Solubility and Dissolution of Iron Oxides. Plant Soil 130, 1–25. doi:10.1007/BF00011851
Uwayezu, J.-N., Yeung, L. W. Y., and Bäckström, M. (2022). Sorption of Perfluorooctane Sulfonate (PFOS) Including its Isomers on Hydrargillite as a Function of pH, Humic Substances and Na2SO4. J. Environ. Sci. 111, 263–272. doi:10.1016/j.jes.2021.03.041
Uwayezu, J.-N., Yeung, L. W. Y., and Bäckström, M. (2019). Sorption of PFOS Isomers on Goethite as a Function of pH, Dissolved Organic Matter (Humic and Fulvic Acid) and Sulfate. Chemosphere 233, 896–904. doi:10.1016/j.chemosphere.2019.05.252
Wang, F., Liu, C., and Shih, K. (2012). Adsorption Behavior of Perfluorooctanesulfonate (PFOS) and Perfluorooctanoate (PFOA) on Boehmite. Chemosphere 89, 1009–1014. doi:10.1016/j.chemosphere.2012.06.071
Wei, C., Song, X., Wang, Q., and Hu, Z. (2017). Sorption Kinetics, Isotherms and Mechanisms of PFOS on Soils with Different Physicochemical Properties. Ecotoxicol. Environ. Saf. 142, 40–50. doi:10.1016/j.ecoenv.2017.03.040
Zeeshan, M., Zhang, Y.-T., Yu, S., Huang, W.-Z., Zhou, Y., Vinothkumar, R., et al. (2021). Exposure to Isomers of Per- and Polyfluoroalkyl Substances Increases the Risk of Diabetes and Impairs Glucose-Homeostasis in Chinese Adults: Isomers of C8 Health Project. Chemosphere 278, 130486. doi:10.1016/j.chemosphere.2021.130486
Zeng, Z., Song, B., Xiao, R., Zeng, G., Gong, J., Chen, M., et al. (2019). Assessing the Human Health Risks of Perfluorooctane Sulfonate by In Vivo and In Vitro Studies. Environ. Int. 126, 598–610. doi:10.1016/j.envint.2019.03.002
Keywords: soil, perfluorooctane sulfonate isomers, sorption, humic substances, sodium sulfate
Citation: Uwayezu J-N, Yeung LWY and Bäckström M (2022) Sorption of Perfluorooctane Sulfonic Acid Including Its Isomers to Soils: Effects of pH, Natural Organic Matter and Na2SO4. Front. Environ. Chem. 3:905170. doi: 10.3389/fenvc.2022.905170
Received: 26 March 2022; Accepted: 16 May 2022;
Published: 01 June 2022.
Edited by:
Hongxia Xu, Nanjing University, ChinaCopyright © 2022 Uwayezu, Yeung and Bäckström. This is an open-access article distributed under the terms of the Creative Commons Attribution License (CC BY). The use, distribution or reproduction in other forums is permitted, provided the original author(s) and the copyright owner(s) are credited and that the original publication in this journal is cited, in accordance with accepted academic practice. No use, distribution or reproduction is permitted which does not comply with these terms.
*Correspondence: Mattias Bäckström, bWF0dGlhcy5iYWNrc3Ryb21Ab3J1LnNl