- Chair of Urban Water Systems Engineering, Technical University of Munich, Garching, Germany
Metal roofs have always been in the focus of stormwater runoff contamination. However, other roofing materials are also suspected of releasing metals and other inorganic substances with stormwater runoff. Hence, this review focuses on the impact of commonly used non-metal roofs - vegetated and non-vegetated - on stormwater runoff quality. Results from 42 studies were compiled and assessed to gain an overview of substances in runoff from nine roofing types with a special focus on green roofs. Concentrations of 27 substances including nutrients, heavy metals, and other inorganic substances were compared. Results show that the nine roof types that were assessed can be a potential source for inorganic substances in the runoff. Threshold values for groundwater protection are exceeded especially for PO43-, Cu, Pb, and Zn for some roofing materials. As the concentrations vary strongly for different parameters, no roofing material can be identified as clearly superior or inferior to the others. Gravel roofs act as a sink for NH4+ and can retain some heavy metals. Elevated heavy metal concentrations were found in runoff from wood shingle roofs treated with preservative chemicals and in runoff from most roofing types usually due to the used gutter materials. Green roof runoff shows increased concentrations of Ptot, PO43-, Na, K, Ca, and Mg. The concentrations depend strongly on the green roof age, the growth substrate, and applied fertilizers. In addition to the roofing materials, external factors dependent on the location (rural or urban site) influence the runoff quality. Runoff from the analyzed roofs must be seen as a diffuse source of environmental pollution and requires appropriate treatment before it is released into the environment or used for further applications. Overall, there are only a few studies on the topic so it is not possible to make statistically significant statements. More serious in-depth studies are urgently needed.
Introduction
Due to the increasing population, more and more formerly green land surfaces are being sealed, especially in growing cities (EU European Commission’s Directorate-General Environment, 2012). Thereby, stormwater’s exposure to contaminants originating from the impermeable surface materials increases. One type of impermeable surface is roofs, which can be constructed using a wide variety of materials. Until now, metal roofs have always been a strong focus as a source of contamination of stormwater runoff and thus also of water bodies (e.g., Gromaire et al., 2002; Hedberg et al., 2014; Galster and Helmreich, 2022). However, non-metal roofing materials are also suspected of releasing pollutants in stormwater runoff (e.g., Förster, 1996; Müller et al., 2020). Studies analyzing pollution in roof runoff especially came up when countries started to infiltrate stormwater runoff into the soil and respectively groundwater in the 1970s and 1980s (Lye, 2009). In these early studies, particularly runoff from non-vegetated roofs was monitored. In 2021, De Buyck et al. (2021) reviewed literature about pollutants in roof runoff from different metal and non-vegetated roofs as well as atmospheric characteristics. However, the review does not focus on green roof runoff. In the 2000s studies analyzing the runoff from green roofs appeared more frequently. Reviews like Berndtsson (2010), Li and Babcock (2014), and Vijayaraghavan (2016) summarized findings on green roof runoff. On the one hand, green roofs are of special interest as they fulfill a retention function in water-sensitive cities, support evapotranspiration, and thus reduce runoff and contribute to a more natural stormwater management. On the other hand, green roofs have an impact on the runoff quality. The recent review of Müller et al. (2020) named green roofs as a potential source of pollutants.
However, no review could be found that combines the available literature data on the runoff quality from non-metal roofs. Therefore, this review aims to close this gap by directly comparing concentrations of individual parameters of the runoff of non-metal roofs, which are non-vegetated, and green roofs with a focus on inorganic substances. In addition, it is analyzed whether the substances in the runoff can negatively affect groundwater quality by comparing runoff values with threshold values for groundwater and consider background concentrations due to atmospheric pollution of rainwater, if available.
Organic substances (e.g., Mecoprop, which is used to protect against root penetration), are also found in some roof runoffs, particularly in flat gravel roofs, bitumen roofs, and green roofs (e.g., Bucheli et al., 1998; Vialle et al., 2013). However, organic substances are not the subject of this review, as it requires separate consideration.
Materials and methods
Selected roofing types and data evaluation
The following non-metal roofing types have been considered: wood shingle roof, flat gravel roof, concrete tile roof, concrete flat roof, asbestos concrete roof, asphalt shingle roof, bitumen roof, clay tile roof, and green roof. Detailed findings from 42 scientific studies were analyzed. All considered studies sampled runoff from at least one relevant roof either in full-scale or pilot-scale design. Publications were only included in the review if values for the relevant parameters were gained from two or more natural rain events–no investigations from simulated rain events were considered. Results of each investigation were only considered once if released in different articles.
In Results and discussion Section, studies analyzing runoff from the same roof materials are compiled and results for the different parameters are compared. The main aspects of the respective studies are described and possible analogies or dissents are highlighted. The following information about the examined publications were considered: the location of the study site and the surroundings (urban, suburban, rural), the scale of the roof (full-scale or pilot-scale), the number of analyzed storm events, whether a first flush division was conducted or not and the tested parameters (see Supplementary Tables S1–S9 in Supplementary Material). Parameters analyzed in the respective study but not relevant for this review are not listed in Results and discussion Section.
To compare results of different studies from the same roof types, median concentrations found in the runoff are displayed and compared amongst each other and with the respective threshold values. If no median value is given the mean value was extracted from the publication as a replacement. The values were either taken from text or tables or deduced visually from figures from the respective studies.
The values always represent concentrations in runoff from complete rain events. If “first flush” and “after first flush” values are given they have not been compared to results from a complete runoff analysis. The first flush is the first stage of stormwater runoff that typically contains a larger number of pollutants than the later runoff (Gupta & Saul, 1996; Lee et al., 2002). In case one study monitored two or more roofs of the respective roofing material, results from every study site are listed. However, only parameters are displayed which have been analyzed at least in two independent publications.
The values represent concentrations in runoff without distinguishing the contribution of atmospheric deposition or leaching from roofing materials. The focus is set on which pollutant concentration can occur in runoff from the analyzed roof types. Differences might occur not only through leaching out of different materials but also due to varying influences of surface characteristics on atmospheric deposition. If the information is given in the respective study, influences of used gutters, pipes, or preservative chemicals on the roofs are mentioned. It is, however, beyond the scope of this study to consider further factors that might influence runoff quality like the roof’s orientation, antecedent dry weather period, wind characteristics, and others as there are only a few data.
Analyzed parameters and threshold values
For comparison of the runoff quality, results of the following 27 parameters were analyzed in detail: nutrients like nitrate (NO3−), nitrite (NO2−), ammonia (NH4+), sulphate (SO42-), phosphate (PO43-), total phosphorous (Ptot), total nitrogen (Ntot), other anions like chloride (Cl−) and fluoride (F−), the heavy metals cadmium (Cd), chrome (Cr), copper (Cu), iron (Fe), lead (Pb) and zinc (Zn) as well as other metals like arsenic (As), aluminum (Al), manganese (Mn), sodium (Na), potassium (K), magnesium (Mg) and calcium (Ca). Other chemical and physical parameters like pH value (pH), electrical conductivity (EC), and total suspended solids (TSS) were also analyzed.
Some studies measured concentrations of NO3−, NO2−, NH4+, and PO43-, others NO3-N, NO2-N, NH4-N, or PO4-P. To make the results consistent and comparable all values were converted with the respective factors to the ion-concentration. Total concentrations of heavy metals are used when values for dissolved, particulate, and total concentrations were given in the reviewed study. In the supplementary information all considered studies, the location, scale (full-scale or pilot-scale), a number of analyzed rain events, information about the first flush analysis, and the analyzed parameters are listed in Supplementary Tables S1–S9.
To assess the level of pollution and the influence on the aquatic environment this study considers the insignificance threshold values (GFS), which are defined as concentrations at which no relevant ecotoxic effects occur even with an increase in the content of the respective substance (LAWA, 2016). These no-effect levels represent evaluation criteria for changes in groundwater quality in Germany; for concentrations below these levels, no adverse modification of groundwater exists (LAWA, 2016). The following GFS threshold values are used for evaluation in the study: SO42-: 250 mg/L, Cl−: 250 mg/L, F−: 900 mg./L, Cd: 0.3 μg/L, Cr: 3.4 μg/L, Cu: 5.4 μg/L, Pb: 1.2 μg/L, Zn: 60 μg/L, As: 3.2 μg/L.
To evaluate the concentrations of NO3−, NO2−, NH4+, and PO43- for which no GFS values exist, the threshold values of the German Groundwater Ordinance (GrwO, 2010) were used: NO3−: 50 mg/L, NO2−: 0.5 mg/L, NH4+: 0.5 mg/L, PO43-: 0.5 mg/L. Additionally, in the GrwO the following concentrations are relevant for the study: SO42-: 250 mg/L, Cl−: 250 mg/L, Cd: 0.5 μg/L, Pb: 10 μg/L, As: 10 μg/L.
Results and discussion
About 16% of the reviewed studies were published between 1989 and 1999, approximately 23% in the years from 2000 to 2010, and over 60% in the last 10 years. Regarding the location of the analyzed roofs, about 50% of the study sites were in Europe, 23% in Asia, 19% in North America, 6% in Africa, and 2% in Australia. About half of the studies analyzed the runoff with respect to the potential pollution of the receiving ground or surface water bodies. Nearly the same share of the studies evaluated the runoff quality regarding the suitability for rainwater harvesting with potable and non-potable applications. Therefore, the focus was not on the evaluation of environmental impacts.
Wood shingle roofs
Only four studies investigated the runoff data from sloped wood shingle roofs (Supplementary Table S1). Chang & Crowley (1993) compared data from wood shingle roofs with clay tile roofs, asphalt shingle roofs, and rock and tar roofs over a six-month period. Of these, wood shingle roof runoff showed the highest concentrations of most analyzed parameters, and the concentrations of Zn, Pb, and NH4+ were clearly higher than rainwater without contact with the roofing material and Zn and Pb exceeded the GFS thresholds. A second study by Chang et al. (2004) aimed to revisit the study from 1993 eliminating its design shortages and focusing on the eight parameters mentioned in Supplementary Table S1. Eight pilot-scale roofs were built using the four-roofing materials wood, shingles, asphalt shingles, aluminum, and galvanized iron. Runoff from wood shingle roofs showed the lowest pH values and the highest concentrations for all other parameters, particularly for Zn and EC. Reasons for the high Zn concentrations are the impregnation with Zn-containing chemicals that have a preservative function and leach out over time. Additionally, on the wood’s rough surface water and particles get trapped and thus mosses and plants can grow. Due to the decomposition of the roofing material, ions were released. As a result, the pH value dropped and other parameters like EC and Zn concentrations increased (Chang et al., 2004). The median concentration of Zn in the second study (9 717 μg/L) was even higher than the values in the first study (2 549 μg/L). Both studies far exceed the GFS threshold of 60 μg/L. As the investigated wood shingle roof in the first study was over 20 years old compared to the newly built pilot-scale roofs in the second study, Chang et al. (2004) concluded that the chemicals for impregnation wash out over the years. Chang & Crowley (1993) and Chang et al. (2004) give further reasons for the high Zn concentrations: the galvanized gutters in combination with the acidic runoff, elevated dry deposition, and relatively high median concentrations in pure rainwater due to several industrial influences in the area. Overall, the Zn runoff concentrations of wood shingle roofs with impregnation are comparable to Zn roof runoff concentrations. For example, in a field study, Schriewer et al. (2008) found a median of 5 600 μg/L (n = 38 rain events) in the runoff of a zinc roof. Therefore, the runoff of an impregnated wood shingle roof must be treated prior to discharge into groundwater, as is the case for Zn roof runoff (Galster & Helmreich, 2022).
The study by Winters et al. (2015) also confirms that impregnation can lead to high metal concentrations in the runoff. The data were collected in two rounds of ten rain events respectively. Round 2 corresponded roughly to one year of exposure. Two types of wood shingle roofs were monitored: wood shingles treated with chromated copper arsenate and untreated wood shingles. The concentrations are calculated on the basis of all samples from the first and second rounds. In the runoff of the treated wood shingle roof the median concentrations of As and Cu were 139 μg/L and 835 μg/L, respectively. Both runoff values exceeded the GFS thresholds, caused by the treatment with copper arsenate. In contrast, the runoff of untreated wood shingle roofs had maximum concentrations of As and Zn of 1 μg/L each. The median Cu concentration in the runoff of wood shingle roofs treated with chromated copper arsenate is in the range of copper roof runoff concentrations (Galster and Helmreich, 2022). Median Zn concentrations were elevated for treated compared to untreated wood shingle roof runoff and compared to the control slope runoff of frosted glass, but under the GFS value (see Table 1). Comparing the two rounds of sampling, the elevated levels of Cu and As found in the runoff from treated wood shingle roof panels were considerably lower in round 2 (Winters et al., 2015). However, it can be assumed that the effectiveness of the impregnation decreases as the concentration of As and Cu decreases.
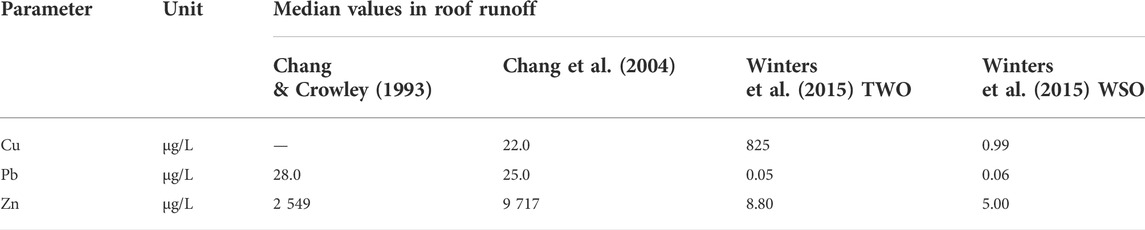
TABLE 1. Heavy metal concentrations in WSR runoff (TWO: Wood shingles treated with chromated copper arsenate; WOS: untreated wood shingles).
The heavy metal concentrations for the three studied WSRs without first flush separation are displayed in Table 1.
In addition to metals, anions were also found in the runoff from wood shingle roofs. Lee et al. (2012) monitored the runoff of a wood shingle roof as one of four pilot-scale roofs in South Korea. Before building the roofs, the materials were stored in an outdoor environment for one year. On the wood shingle roof, the greatest number of lichens and mosses were found. This resulted in comparatively high concentrations of NO3− and SO42- in the respective runoff, but which were below the GFS thresholds.
In summary, the water quality parameters in runoff from wood shingle roofs exceeded the respective GFS and GrwO values for metals in the studies where the roof was impregnated. The runoff quality of wood shingle roofs seems to be strongly dependent on the chemicals used for the preservation, the age, and the used guttering materials. It can be concluded that if metal-containing preservatives are applied on wood shingle roofs, treatment of the runoff is imperative before discharge into groundwater. Nevertheless, the number of studies on WRSs is limited. More data for a statistical analysis would be desirable.
Flat gravel roofs
Five relevant publications were found that monitored inorganic substances in runoff from flat gravel roofs in Europe and North America (Supplementary Table S2). Quek & Förster (1993) collected data on a flat gravel roof in comparison with four other roofing materials (clay tile, asbestos concrete, tar felt, and zinc) during two rain events for the first 3 mm of runoff. The runoff from the flat gravel roof showed the lowest substance concentrations due to its storage function and filtering through the gravel stone layer (Quek & Förster, 1993). This tendency was also described by Zobrist et al. (2000), who reported that most of the heavy metals and phosphorus are retained by the flat gravel roof material. NH4+ values are low as it gets oxidized to NO3− on the gravel roof during the retention period (Zobrist et al., 2000). The same effect was monitored by Farreny et al. (2011) who found the lowest concentrations of NH4+ in the flat gravel roof runoff due to the nitrification process. Farreny et al. (2011) compared runoff from a flat gravel roof with a clay tile roof, metal sheets, and polycarbonate plastic roof. They demonstrated that the flat gravel roof runoff contains the highest EC levels. The reasons are probably the accumulation of particles and colonization trough plants promoted by the low slope (Farreny et al., 2011).
Malcolm et al. (2014) analyzed runoff from the different pilot and full-scale flat gravel roofs and green roofs in Virginia. Concentrations of Ntot and Ptot were lower in the runoff from the flat gravel roofs compared to green roofs (Malcolm et al., 2014).
Apart from Cu in runoff analyzed by Zobrist et al. (2000) which exceeded the GFS value, all other parameters were below the threshold values of the GFS and GrwO. However, no further explanation for the high Cu concentrations is given in Zobrist et al. (2000) and the guttering system of the monitored roof was made of polyvinyl chloride (PVC).
Overall, runoff from flat gravel roofs shows low NH4+ concentrations because of nitrification occurring on the roof. Additionally, due to their structure, FGRs can act as a sorption filter. However, more studies are required for more specific statements.
Concrete tile roofs
Seven studies published between 1989 and 2020 analyzed stormwater runoff from sloped concrete tile roofs (Supplementary Table S3), of which six analyzed the first flush concentrations. Yaziz et al. (1989) compared the first five liters of runoff from a concrete tile roof with a galvanized iron roof as the first flush. The concrete tile roof runoff showed higher values for TSS and EC than the iron roof, which probably relates to the rougher surface facilitating the accumulation of pollutants from the atmosphere. Runoff pH values were higher than in the rainwater without contact with the roofing material for both roofs. Pb concentration in concrete tile runoff was higher than the GFS threshold (Table 2), probably as a result of elevated concentrations in the rainwater (mean: 200 μg/L) caused by a major highway close to the study site (Yaziz et al., 1989). A study from Texas analyzed runoff from a pilot-scale concrete tile roof in comparison to four other roof types at pilot-scale or full-scale. The concrete tile runoff showed the highest pH values of all other pilot-scale roofs (Mendez et al., 2010). Lee et al. (2012), Nosrati (2017) and Zdeb et al. (2020) confirmed the finding of elevated pH values in runoffs of concrete tile roofs. Concrete tile runoff has the highest pH values with an average of 7.2 in the first flush and after the first flush. This is caused by alkaline ingredients which react with the rainwater (Lee et al., 2012). With an increasing pH value, the acidity of the runoff is lowered, which may cause public health risks as some bacteria hazardous to health to grow best in neutral conditions (Zdeb et al., 2020).
Winters et al. (2015) found higher Pb concentrations in concrete tile roof runoff than in runoff from other roof types and a glass control roof. Thomas & Greene (1993) monitored runoff after the first flush from three concrete tile roofs and three galvanized iron roofs each in a rural, urban, and industrial environment. The pH and EC values were higher and Zn values lower in concrete tile roof runoff compared to the metal roofs. Pb and Zn concentrations were highest in runoff in the industrial area, followed by the urban environment. Thomas & Greene (1993) explain high Zn values in roof runoff, which exceeded the GFS value, with an extensive Zn use in the industrial area. Lee et al. (2012) propose that atmospheric deposition might be the reason for elevated Cu concentrations in concrete tile roof runoff. Gutters for the pilot-scale concrete tile roof in Mendez et al. (2010) were made of aluminum. No further explanation for the elevated Zn value is given. NO3− concentrations showed the highest levels in rural roof runoffs (Thomas & Greene, 1993). Nonetheless, this concentration is well below the GrwO threshold value.
Most investigations differentiated between first-flush analysis and an analysis of the collected runoff after the first flush. The values collected after the first flush are presented in Table 3.
In summary, runoff values of Zn and Cu exceed the thresholds in some studies (see Table 2). While elevated levels of heavy metals seem to be caused by atmospheric pollution, the increased pH value in runoff is a result of alkaline compounds in the concrete.
Concrete flat roofs
Six studies from China and Greece provide information about runoff from concrete flat roofs (Supplementary Table S4). Stormwater runoff from three concrete flat roofs was analyzed in comparison to other roofing types by Melidis et al. (2007). The pure rainwater without contact with the roofing material had pH values between 6.7 and 7.9, caused by dust and particles from rock erosion due to strong winds from nearby mountains and increased by contact with the roofing material. Due to the elevated pH value, the runoff has low corrosive properties. This results in low runoff concentrations of heavy metals from gutters and piping material (Melidis et al., 2007). Gikas & Tsihrintzis (2012) analyzed concrete flat roofs and clay tile roofs at a pilot scale in the same areas as Melidis et al. (2007). One concrete flat roof was in a rural and one in a suburban region. Higher values of NH4+ and Ptot, found in the concrete flat roof and clay tile roof runoff in the rural areas, may be caused by bird and animal excrements and plants on the respective roofs. For both parameters, values in the first flush runoff were higher than in the storage tank (Gikas & Tsihrintzis, 2012) in which the rest of the runoff was collected.
Zhang et al. (2014) observed two pilot-scale concrete flat roofs and compared these with bitumen roofs, green roofs, and clay tile roofs. Remarkably higher levels of mean pH (7.6) and TSS (120 mg/L) were found in runoff from the concrete flat roofs. Qin et al. (2015) analyzed runoff from a flat cement mortar roof. In the study, this roof is categorized as a concrete flat roof due to similar properties of the material and the flat slope. The pH from the runoff with a median value of 7.8 was clearly higher than in the associated rainwater where the median pH value was 5.0. A clear upwards trend can also be seen for dissolved Mg, Ca, Na, K, Cl−, F−, SO42- and NO3−. The NH4+ concentration found by Qin et al. (2015) exceeded the GrwO threshold. Nevertheless, the NH4+ concentration in the concrete flat roof runoff was lower compared to the analyzed rainwater showing that the NH4+ concentration is not from the roofing material. Furthermore, Qin et al. (2015) showed that there is a positive correlation between NOx and PM10 (Particulate matter with 10 µm) in the atmosphere with measured NH4+ and NO3− in the wet deposition.
Gikas & Tsihrintzis (2017) compared runoff from a concrete flat roof and a clay tile roof in a village in Greece close to the main highway with a high traffic load. Concentration differences were found for NH4+ and PO43- which were higher in the clay tile roof runoff while Ca values were higher in the concrete flat roof runoff. As the roof was approximately 30 years old, the increased Ca value was assigned to the erosion processes of the concrete. Liu et al. (2020) gathered water quality parameters from two green roofs and a concrete flat roof. Compared to rainwater, the pH value increased in runoff from all three roofs. Values of the study sites described previously in which no first flush division was conducted are summarized in Table 4. Zhang et al. (2014) evaluated mean and median values; here the mean values of the study are used as the other three studies analyzed the mean concentrations.
No explanation is given for the elevated SO42- value in the study by Zhang et al. (2014), but the concentration was far below the GFS threshold. High concentrations of Zn were found in runoff from two roofs by Melidis et al. (2007). The reasons were mostly the erosion of drainage pipe material, which was iron and zinc alloy (Melidis et al., 2007).
In summary, for concrete flat roofs, most parameters do not exceed the thresholds. Elevated concentrations of Zn may come from the guttering system. However, the data situation is also very limited for concrete flat roofs.
Asbestos concrete roofs
Six publications, summarized in Supplementary Table S5, give information about asbestos concrete roof runoff. Quek & Förster (1993) found pH values to be highest in runoff from the asbestos concrete roof, caused by CaCO3 dissolution. Due to the higher pH values, more heavy metals are present in the particulate phase. Additionally, the high roughness of the material enables particles to stay on the roof. Thus, in comparison with the other sloped roofs in Quek & Förster’s study, the asbestos concrete roof showed the lowest runoff pollution.
Nevertheless, in all studies, the heavy metal concentrations in asbestos concrete roof runoff are very high and almost all exceed the GFS values. Ayenimo et al. (2006) found an extremely high concentration of Pb (1 430 μg/L) in the runoff which exceeds the GFS value by a factor of 1,000. A possible source of Pb can be the joints of asbestos concrete roofs which are sometimes made of Pb. In addition, the primary pollution of the rainwater by atmospheric pollution is relevant in this study. However, in this study, the rainwater without contact with the roofing material already showed high mean values for Pb (530 μg/L) and also for many other parameters: PO43-: 13.7 mg/L, SO42-: 5.39 mg/L, Cd: 350 μg/L, Zn: 880 μg/L, Fe: 470 μg/L. This demonstrates that the primary contamination of rainwater must not be ignored when analyzing runoff data.
A study in Poland gathered information about roof runoff from asbestos concrete roofs, clay tile roofs, bitumen roofs, and zinc roofs. Apart from high Zn concentration in Zn roofs, results showed no clear differences between the roofing types (Tobiszewski et al., 2010). Akoto et al. (2011) analyzed stormwater runoff from asbestos concrete roofs, clay tile roofs, and metal sheet roofs. They collected no runoff in the first 15 min of the rain event. Runoff from asbestos concrete roofs showed higher Pb and Cd concentrations than from metal roofs (Akoto et al., 2011). A study in Nigeria found–analog to observations of the study of Quek & Förster (1993)—pH values to be highest in asbestos concrete roof runoff. Concentrations of TSS, PO43-, NO3−, Cd, Cu, Fe, and Pb are elevated in runoff from the asbestos concrete roof in the study by Chizoruo & Onyekachi (2016). Quality differences in runoff from asbestos concrete roofs, concrete tile roofs, and galvanized zinc roofs were observed by Wahyuningsih et al. (2020). NO2− concentration was highest in asbestos concrete roof runoff. Similarly, the mean Zn values in asbestos cement roof runoff sampled by Wahyuningsih et al. (2020) were high (240 μg/L) but did not exceed the GFS thresholds.
Table 5 displays values of only three studies as the others analyzed either the first flush volume, and the volume after the first flush, or gave only minimum and maximum values.
In summary, asbestos concrete roofs act as a source of most heavy metals and nutrients in runoff. Values of PO43-, Cd,, Pb and Zn in all studies exceed the thresholds. Nevertheless, here, too, more numerous samples and sampling sites would be desirable.
Asphalt shingle roofs
Five studies in the U.S. analyzed runoff from asphalt shingle, asphalt fiberglass shingle, asphalt composition shingle, or composition shingle roofs. All of these are summarized with the term asphalt shingle roofs and are listed in Supplementary Table S6.
The two studies by Chang & Crowley (1993) and Chang et al. (2004) that also investigated wood shingle roof runoff showed that for most parameters, asphalt shingle roof runoff had lower concentrations compared to wood shingle roof runoff and higher concentrations than runoff from the clay tile roof runoff in Chang & Crowley (1993). One conclusion of the study is that the roofing material overall seems to influence the runoff quality, especially on the pH, EC, and Zn values. Runoff from the asphalt shingle roof has lower Zn values than runoff from the wood shingle roof runoff (Chang et al., 2004), but was still over the GFS threshold value. Chang & Crowley (1993) and Chang et al. (2004) used galvanized gutters which could be one reason for the elevated Zn levels.
Mendez et al. (2010) analyzed runoff from two full-scale and one pilot-scale asphalt shingle roof and compared it to another roofing material. Runoff quality from the two full-scale asphalt shingle roofs of similar age differed because of other influencing factors like the geographical location and the amount of vegetation. The pilot-scale asphalt shingle roof runoff showed a high Cu concentration (median 339 μg/L in the first flush), which exceed the GFS thresholds. The pure rainwater was uncontaminated (median 0.98 μg/L in the first flush). The source was not clear, as no copper gutters or fittings were used on the pilot-scale asphalt shingle roof (Mendez et al., 2010). Winters et al. (2015) analyzed runoff from different asphalt shingle roofs with and without algae-resistant copper granules. Runoff from the asphalt shingle roof with the algae-resistant copper granules contains higher concentrations of Cu (median 30 μg/L) than the other asphalt shingle roof (median 2.1 μg/L) and runoff from a glass control panel (median 0.5 μg/L).
Toland et al. (2012) compared runoff from asphalt shingle roofs with other roofing materials (green roof, bitumen roof, and metal roof). Compared to the green roof runoff, Ptot, and Ntot concentrations were lower in the asphalt shingle roof runoff. Concentrations of NH4+, NO3−, and NO2− showed no clear difference (Toland et al., 2012). Buffam et al. (2016) monitored stormwater runoff from an asphalt shingle roof and rainwater over 22 months. Only the Zn concentration in asphalt shingle roof exceeded the GFS thresholds, and in rainwater itself the concentrations were low. No information about the gutter material is given in Buffam et al. (2016), therefore the origin of Zn in runoff cannot be assessed.
Table 6 summarizes the runoff data from the studies. Chang & Crowley (1993) and Chang et al. (2004) had no explanation for the elevated Pb values in the runoff. More studies with detailed information about the used gutter materials and preservation chemicals would be needed to make assumptions about the heavy metal concentrations in asphalt shingle roof runoff.
Bitumen roofs
Nine publications were found that analyzed runoff data from so-called bitumen, flat tar, and asphalt roofs, which are summarized here with the term bitumen roofs. All roofs had a gentle slope (<10°) or were completely flat. An overview is given in S.7.
Teemusk & Mander (2007) analyzed runoff from a bitumen roof and a green roof during two moderate and one heavy rain event as well as snowmelt. The value in Supplementary Table S7 is the calculated average of the three rain events. Regarding these rain events, NO3− and SO42- levels were always lower in the bitumen roof runoff than in the green runoff, and Ptot concentration was higher. The reasons are stated to be dust and other contaminants. However, due to the small number of events sampled, the available data are not sufficient to make reliable statements.
There is more data from Lamprea & Ruban (2008) who monitored the runoff of four roofing materials, namely bitumen roof and concrete tile roof (both with PVC gutter), slate and zinc sheets (both with galvanized gutters) over a period of five months in France (Lamprea & Ruban, 2008). No obvious concentration differences could be found for Cd, Cr, and Cu. Roofs with galvanized gutters showed higher Zn concentrations and those with PVC gutters elevated Pb values. The elevated Pb value in bitumen roof runoff in this study, which exceeded the GFS threshold, can be explained by the addition of Pb to the PVC for higher resistance.
Van Seters et al. (2009) collected runoff samples from a bitumen roof and compared them with green roof runoff. The concentrations of the investigated inorganic substances with the exception of Ptot were higher in bitumen roof runoff compared to green roof runoff, but mostly under the GFS or GrwO thresholds. Only Cu in bitumen roof runoff lay beyond the GFS threshold, which was not further explained. Tobiszewski et al. (2010) found no clear differences between runoff from bitumen roofs, concrete tile roofs, asbestos cement roofs, and zinc roofs. However, the study only lists the minimum and maximum values and thus the values cannot be directly compared to the mean and median values of the other studies.
For all parameters monitored by Toland et al. (2012) (NO2−, NH4+, Ntot, and Ptot), runoff from the bitumen roofs showed lower or similar concentrations compared to those from the asbestos shingle roofs, the green roofs, and the metal roof. The bitumen roof runoff in the study by Zhang et al. (2014) showed higher Zn concentrations than runoff from cement tile roofs and clay tile roofs. No obvious concentration difference between bitumen roof and concrete tile roof runoff was detected in the study by Nosrati (2017). Nawrot & Wojciechowska (2018) collected data from the copper roofs, clay tile roof, and bitumen roof runoffs during the first 15 min of rainfall. Runoff from the bitumen roof showed the greatest mean concentrations of Al (152 μg/L), Cd (0.60 μg/L), Pb (6.00 μg/L), and Zn (15,520 μg/L). The roughness and lower inclination angle compared to the other roofs are seen as reasons for the highest Pb and Al concentrations in bitumen roof runoff and no explanation for the other parameters are given (Nawrot & Wojciechowska, 2018). As the study of Nawrot & Wojciechowska (2018) analyzed only the first flush, the data are not listed in Table 7, which summarizes runoff concentrations of bitumen roofs.
Regarding Table 7, especially concentrations of heavy metals exceed the thresholds. High Pb values in Lamprea & Ruban (2008) are explained by the use of a PVC gutter. No explanation for the high concentrations of other heavy metals in bitumen roof runoff is given in this study and also in the studies of Van Seters et al. (2009) and Nawrot & Wojciechowska (2018).
As with the evaluation of the data from the roofing materials described previously, further investigations must be carried out for a scientific evaluation of bitumen roof runoff.
Clay tile roofs
Eighteen publications about runoff from clay and ceramic tile roofs, both referred to as clay tile roofs, were analyzed. Most of the studies were conducted in Europe and some in Asia, see Supplementary Table S8. Chang & Crowley (1993) found stormwater runoff from the clay tile roof to show the best quality when compared with wood shingle roof, asphalt shingle roof, and rock and tar roof runoff. Ca and pH values were higher which is attributed to acidic rain and weathering of the clay tiles containing Ca (Chang & Crowley, 1993). The same process for clay tile roofs was observed by Quek & Förster (1993). Wahyuningsih et al. (2020) found the highest pH values and the best overall quality in clay tile roof runoff compared to other roofing materials. Zdeb et al. (2020) also monitored an increase in pH value in clay tile roof runoff.
Regarding heavy metals, clay tile roof runoff from the study of Melidis et al. (2007) showed elevated concentrations of Zn and Fe which are probably caused by the metal gutters. The relatively high median Zn concentration of 620 μg/L in the runoff in the study of Chang & Crowley (1993) may also be caused by the galvanized gutters and high concentrations in the rainwater. Lamprea & Ruban (2008) explain high Pb values with Pb leaching from the PVC gutters. No explanation for the elevated Zn concentrations is given. However, runoff from the zinc sheets and slate roof showed 3 to 9 times higher Zn concentrations than from the clay tile roof. Zobrist et al. (2000) found elevated Cu values in the clay tile roof runoff that are probably caused by the copper gutters. Furthermore, the clay tile roof seems to act as a source for TSS, Mn, Fe, and Pb. However, the Pb values may also be elevated due to a highway close by in the study by Zobrist et al. (2000).
In the first flush study by Nawrot & Wojciechowska (2018), mean Zn and Cu concentrations in clay tile roof runoff (Zn: 1 470 μg/L, Cu: 20 μg/L) were far above the recommended GFS thresholds, which did not result from primary rainwater contamination. No information about the gutter material or other possible reasons is given in the study. As described in Concrete flat roofs Section, Gikas & Tsihrintzis (2017) found that NH4+, PO43-, and Ca2+ values showed differences in the roof runoff from concrete flat roofs and clay tile roofs. The main sources of elevated NH4+ and Ptot runoff concentrations in the rural area are lichens, birds, and rodents (Gikas & Tsihrintzis, 2012; Gikas & Tsihrintzis, 2017). In comparison, the NH4+ and Ptot values in runoff from the other clay tile roofs in a suburban and urban environment were lower. Hence, the local conditions may have a great impact on the quality of the runoff. Lee et al. (2012) compared four roof types with first the flush analysis in South Korea. PH values in clay tile roof runoff were higher than in the metal and wood shingle roof samples. This is in accordance with findings from the studies mentioned previously.
Ghazali & Sulaiman (2018) analyzed runoff from a clay tile roof and a galvanized roof in Malaysia. Fe and Zn concentrations were lower in the clay tile roof runoff, and EC and pH were marginally higher. The authors recommend the usage of runoff from clay tile roofs rather than from galvanized metal roofs for domestic purposes. Due to the low values of nearly all runoff quality parameters of the clay tile roof, Zhang et al. (2014) also consider the clay tile roof to be most suitable for rainwater harvesting (compared to a concrete flat roof, bitumen roof, and green roof). The reasons for this are better resistance to aging and weathering and no leaching of nutrients and organic pollutants (Zhang et al., 2014). In accordance with this, Mao et al. (2021) also claimed clay tile roofs are the best roofing material for rainwater harvesting. Within the scope of Mao’s study, stormwater runoff from sloped pilot-scale roofs (clay tile roof, galvanized metal, concrete, and asphalt) were monitored.
Ayenimo et al. (2006) reported that runoff from clay tile roofs shows better quality than from asbestos concrete roofs. Vialle et al. (2011) analyzed harvested roof runoff from a clay tile roof for one year. The values for most parameters correspond to the concentrations measured by other authors for clay tile roofs. This finding is also confirmed by Tobiszewski et al. (2010). Thresholds especially for Zn are exceeded in five studies. High Pb and Zn concentrations in Chang & Crowley (1993) and Melidis et al. (2007) may be explained by metal gutters. High Pb runoff concentrations may be caused by leaching out from the PVC gutter (Lamprea & Ruban, 2008). Wahyuningsih et al. (2020) reported mean Zn concentrations of 240 μg/L in rainwater without contact to the roofing material. Thus, the clay tile roof itself did not act as a source for Zn in that case. The same applies to Ayenimo et al. (2006) where the control samples of the rainwater showed mean concentrations of 880 μg/L.
The individual results of these studies are compared in Table 8.
Overall, it can be stated that high heavy metal concentrations in clay tile roof runoff often seem to be caused by guttering systems or already elevated values in the rainwater itself. Increased values of NH4+ and Ptot appear to depend on characteristics of the local conditions like animals or plants, whereas material properties of the clay tile roof lead to increased pH and Ca values. Several authors recommend clay tile roofs for rainwater harvesting applications in comparison to other roofing types.
Green roofs
The fourteen publications listed in Supplementary Table S9 analyzed complete runoff from green roofs. Green roofs can be divided into two categories: extensive and intensive. Extensive green roofs are built with thin substrate layers for vegetation like grasses or mosses and are planned to require no maintenance. In contrast, intensive green roofs contain a deep soil layer for bigger plants like bushes or trees and usually need maintenance (Berndtsson, 2010). In this review the term green roof is used for extensive green roofs.
Green roofs can act as a sink or as a source of inorganic substances. The intensity of the rain event and thus the runoff rate strongly influence the substance concentrations in green roof runoff (Teemusk & Mander (2007). For example, NO3− and PO43- concentrations were higher in the runoff of heavy rain events compared to low rain events.
Above all, phosphorus in form of dissolved PO43- and Ptot as well as nitrogen as Ntot is washed out of the substrate as proven in many studies (e.g., Berndtsson et al., 2006; Van Seters et al., 2009; Gregoire & Clausen, 2011; Liu et al., 2020). Hence, the age of the roof is one of the decisive factors in determining whether and how much phosphorus is emitted. Buffam et al. (2016) observed that especially in the beginning, green roofs act as a source of PO43-. Berndtsson et al. (2006) analyzed runoff from four green roofs. They stated that only the oldest green roof is not a substantial source of PO43- and Ptot. One reason for phosphorus in the runoff is the application of fertilizer. Toland et al. (2012) analyzed runoff from green roofs with and without compost-addition. Ptot, as well as Ntot values were higher when compost was added to a green roof. Berndtsson et al. (2009) monitored one green roof in Sweden and two intensive green roofs. The intensive and extensive green roofs showed different behaviors. High Ptot concentrations were found in the green roof runoff, while the intensive green roof released no Ptot and acted as a sink for Ntot. The reason for this might be higher nutrient requirements of the larger plants (Berndtsson et al., 2009). Seidl et al. (2013) also reported that green roofs acted as a source of PO43- and Ntot. However, after a dry period and for small rain events, a sink behavior of the green roofs for these parameters was observed (Seidl et al., 2013). Buffam et al. (2016), analyzed runoff from eight green roofs for three years during summer months only. Overall values showed that the older the roof, the better the water quality. This has especially been observed for TSS and NH4+ due to the increased stability of the substrate through the roots and a better adsorption of the positively charged NH4+ by the substrate. Ptot concentrations remained constant over the studied time. The type and thickness of substrate as well as the vegetation type had an influence on different parameters (Chai et al., 2018). Liu et al. (2020) reported that mean Ntot concentrations increased in runoff from the second of their monitored green roofs: 15.7 mg/L Ntot in green roof runoff compared to a mean concentration in rainwater of 2.18 mg/L. The high value is attributed to the used substrate as the Ntot content of the substrate decreased from 1.19 g/kg to 0.51 g/kg during the monitoring period (Liu et al., 2020). Malcolm et al. (2014) found levels of Ptot and Ntot being higher in green roof runoff than in flat gravel roof runoff. Values of this study are not listed in Table 9 as metal values were analyzed only in one rain event and no mean or median values of Ntot and Ptot can be given. Table 9 depicts clearly that especially values of PO43- are high and exceed the threshold value in most of the analyzed studies. However, no clear trend can be seen for Ntot. Some roofs show elevated Ntot levels, others had no influence or act as a sink for Ntot.
There were several studies which reported that green roofs could retain heavy metals like Cu, Pb, and Zn from rainwater (Gregoire & Clausen, 2011; Gnecco et al., 2013; Seidl et al., 2013). Some studies, however, showed elevated Cu or Zn concentrations in the green roof runoff (Gregoire & Clausen, 2011; Buffam et al., 2016). For these studies, the influences of gutters or other materials on the roofs cannot be excluded. However, no information about gutter material is provided which would explain the high Zn or Cu values.
Concentrations of SO42-, Ca, and Mg, which are ingredients of green roof substrates, were increased in the runoff (Teemusk & Mander, 2007). The values of Teemusk & Mander (2007) are not displayed in Table 9 as only samples from one roof were taken at two different outflows. Van Seters et al. (2009) found higher Ca and Mg values in green roof runoff compared to bitumen roof runoff. In accordance with this, runoff quality showed strong seasonal variations. Ca, K, Mg and Na concentrations increased during summer. The authors state that short-term observations might not adequately represent green roof runoff. These strong seasonal fluctuations have not been observed for the asphalt shingle roof runoff and precipitation quality (Buffam et al., 2016).
Zhang et al. (2014) compared runoff from conventional pilot-scale roofs with runoff from a pilot-scale green roof. Runoff from the green roof showed higher EC values, Cl−, SO42-, K, Na, Mg, and Cu concentrations compared to runoff from the other roofs. The authors state that green roof runoff is not suitable for rainwater harvesting (Zhang et al., 2014).
Overall, the applied substrate, application of fertilizer, the plants, the age, the season of the sampling, and other factors can have a strong influence on the runoff quality. Thus, these factors should be considered when comparing runoff from different green roofs. Data in Table 9 is exclusively from green roof runoffs without first flush separation.
Conclusion
As a result of this study, it can be stated that the studies which analyzed non-metal roof runoff are very limited and that no clear or statistically relevant statements can be made regarding pollutant emissions for most roofing materials. However, regardless of the number of studies of the different roofs, it can be clearly demonstrated that heavy metals are also an issue in runoff of non-metal roofs. In terms of concentration, depending on the metal they are usually 100 times lower compared to metal roof runoff, but they very often exceed thresholds, sometimes in considerable concentrations. In the case of the application of metal containing chemicals and preservatives, metal concentrations exceed thresholds up to a factor of 1,000 and are comparable to metal roof runoff. Additionally, strong concentration variations can occur due to the material of the guttering system and other construction materials.
In addition to heavy metals, some roofing materials also act as sources of nutrient emissions, for example, green roofs. Here, different substrate compositions and the roof age have an influence on the runoff quality. Different other factors in the roof’s surroundings also influence the runoff quality: on a large scale, pollutants in the air and rainwater from natural or anthropogenic sources, and on a small scale, plants and animals on the roof.
The runoff from non-metal roofs, whether vegetated or not, must also be considered as a potential source of soil and water contamination. Therefore, adequate treatment of runoff is required before entering the environment. Additionally, treatment is required before harvesting the runoff for domestic uses or even using it as a drinking water source. A treatment of at least the first flush could help reduce pollution with relatively little effort. However, it must be kept in mind that later runoff also contains contaminants. A detailed review of the first flush and later runoff qualities was not part of this study and should be investigated in further studies.
From a pollutant load standpoint, roofs that emit heavy metals in any way, be it through preservatives or increased amounts of metals through the guttering system, should be viewed critically. Particularly critical here are wood shingle roofs with preservatives. A ranking of the roofing materials examined cannot be made due to the limited data available on some roofing materials.
The overall conclusion is that there are only few studies on the topic which moreover present biases such as gutter materials and atmospheric depositions for example. More serious studies are urgently needed to make statistically evaluable statements. It is becoming apparent that some roofing materials emit water-relevant substances and therefore the runoffs must be treated to protect the aquatic environment from seeping into the groundwater.
Author contributions
Conceptualization, JD; methodology, JD; validation, JD, data curation, JD; writing—original draft preparation, JD; writing—review and editing, BH; visualization, JD and BH; supervision, BH. All authors have read and agreed to the version of the manuscript.
Funding
This work received no external funding but was supported by the German Research Foundation (DFG) and the Technical University of Munich (TUM) in the framework of the Open Access Supporting Program.
Acknowledgments
We would like to thank Daphne Keilmann-Gondhalekar for English proof-reading.
Conflict of interest
The authors declare that the research was conducted in the absence of any commercial or financial relationships that could be construed as a potential conflict of interest.
Publisher’s note
All claims expressed in this article are solely those of the authors and do not necessarily represent those of their affiliated organizations, or those of the publisher, the editors and the reviewers. Any product that may be evaluated in this article, or claim that may be made by its manufacturer, is not guaranteed or endorsed by the publisher.
Supplementary material
The Supplementary Material for this article can be found online at: https://www.frontiersin.org/articles/10.3389/fenvc.2022.884021/full#supplementary-material
References
Akoto, O., Appiah, F., and Boadi, N. O. (2011). Physicochemical analysis of roof runoffs from the Obuasi area. Water Pract. Technol. 6, 2011003. doi:10.2166/WPT.2011.003
Ayenimo, J. G., Adekunle, A. S., Makinde, W. O., and Ogunlusi, G. O. (2006). Heavy metal fractionation in roof run off in Ile-Ife, Nigeria. Int. J. Environ. Sci. Technol. (Tehran). 3, 221–227. doi:10.1007/BF03325929
Berndtsson, J. C., Bengtsson, L., and Jinno, K. (2009). Runoff water quality from intensive and extensive vegetated roofs. Ecol. Eng. 35, 369–380. doi:10.1016/j.ecoleng.2008.09.020
Berndtsson, J. C., Emilsson, T., and Bengtsson, L. (2006). The influence of extensive vegetated roofs on runoff water quality. Sci. total Environ. 355, 48–63. doi:10.1016/j.scitotenv.2005.02.035
Berndtsson, J. C. (2010). Green roof performance towards management of runoff water quantity and quality: A review. Ecol. Eng. 36, 351–360. doi:10.1016/j.ecoleng.2009.12.014
Bucheli, T. D., Müller, S. R., Voegelin, A., and Schwarzenbach, R. P. (1998). Bituminous roof sealing membranes as major sources of the herbicide (R, S)-Mecoprop in roof runoff Waters: potential contamination of groundwater and surface waters. Environ. Sci. Technol. 32, 3465–3471. doi:10.1021/es980318f
Buffam, I., Mitchell, M. E., and Durtsche, R. D. (2016). Environmental drivers of seasonal variation in green roof runoff water quality. Ecol. Eng. 91, 506–514. doi:10.1016/j.ecoleng.2016.02.044
Chai, H., Tang, Y., Su, X., Wang, W., Lu, H., Shao, Z., et al. (2018). Annual variation patterns of the effluent water quality from a green roof and the overall impacts of its structure. Environ. Sci. Pollut. Res. 25, 30170–30179. doi:10.1007/s11356-018-3039-2
Chang, M., and Crowley, C. M. (1993). Preliminary observations on water quality of storm runoff from four selected residential roofs. J. Am. Water Resour. Assoc. 29, 777–783. doi:10.1111/j.1752-1688.1993.tb03237.x
Chang, M., McBroom, M. W., and Beasley, R. S. (2004). Roofing as a source of nonpoint water pollution. J. Environ. Manag. 73, 307–315. doi:10.1016/j.jenvman.2004.06.014
Chizoruo, I. F., and Onyekachi, I. B. (2016). Roof runoff water as source of pollution: A case study of some selected roofs in orlu metropolis, imo state, Nigeria. Int. Lett. Nat. Sci. 50, 53–61. doi:10.18052/www.scipress.com/ILNS.50.53
De Buyck, P. J., Van Hulle, S. W. H., Dumoulin, A., and Rousseau, D. P. L. (2021). Roof runoff contamination: A review on pollutant nature, material leaching and deposition. Rev. Environ. Sci. Biotechnol. 20, 549–606. doi:10.1007/s11157-021-09567-z
EU European Commission’s Directorate-General Environment (2012). Soil sealing, in-depth report. Sci. Environ. Policy. https://ec.europa.eu/environment/archives/soil/pdf/sealing/Soil%20Sealing%20In-depth%20Report%20March%20version_final.pdf.
Farreny, R., Morales-Pinzón, T., Guisasola, A., Tayà, C., Rieradevall, J., and Gabarrell, X. (2011). Roof selection for rainwater harvesting. Quantity and quality assessments in Spain. Water Res. 45, 3245–3254. doi:10.1016/j.watres.2011.03.036
Förster, J. (1996). Patterns of roof runoff contamination and their potential implications on practice and regulation of treatment and local infiltration. Water Sci. Technol. 33, 39–48. doi:10.2166/wst.1996.0079
Galster, S., and Helmreich, B. (2022). Copper and zinc as roofing materials – a review on the occurrence and mitigation measures of runoff pollution. Water 2022 14, 291. doi:10.3390/w14030291
Ghazali, S. N., and Sulaiman, F. R. (2018). Water quality of roof runoff in sub-urban Malaysia. Asian J. Agric. Biol. 6, 125–128.
Gikas, G. D., and Tsihrintzis, V. A. (2012). Assessment of water quality of first-flush roof runoff and harvested rainwater. J. Hydrology 466-467, 115–126. doi:10.1016/j.jhydrol.2012.08.020
Gikas, G. D., and Tsihrintzis, V. A. (2017). Effect of first-flush device, roofing material, and antecedent dry days on water quality of harvested rainwater. Environ. Sci. Pollut. Res. 24, 21997–22006. doi:10.1007/s11356-017-9868-6
Gnecco, I., Palla, A., Lanza, L. G., and La Barbera, P. (2013). The role of green roofs as a source/sink of pollutants in storm water outflows. Water Resour. manage. 27, 4715–4730. doi:10.1007/s11269-013-0414-0
Gregoire, B. G., and Clausen, J. C. (2011). Effect of a modular extensive green roof on stormwater runoff and water quality. Ecol. Eng. 37, 963–969. doi:10.1016/j.ecoleng.2011.02.004
Gromaire, M. C., Chebbo, G., and Constant, A. (2002). Impact of zinc roofing on urban runoff pollutant loads: The case of Paris. Water Sci. Technol. 45, 113–122. doi:10.2166/wst.2002.0123
GrwO (2010). GrwO: Grundwasserverordnung of november 9, 2010 (federal law gazette I S. 1513), which was last amended by article 1 of the ordinance of may 4, 2017. Federal Law Gazette 1, 1044.
Gupta, K., and Saul, A. J. (1996). Specific relationships for the first flush load in combined sewer flows. Water Res. 30 (5), 1244–1252. doi:10.1016/0043-1354(95)00282-0
Hedberg, Y. S., Hedberg, J. F., Herting, G., Goidanich, S., and Odnevall Wallinder, I. (2014). Critical review: Copper runoff from outdoor copper surfaces at atmospheric conditions. Environ. Sci. Technol. 48, 1372–1381. doi:10.1021/es404410s
Lamprea, K., and Ruban, V. Micro pollutants in atmospheric deposition, roof runoff and stormwater runoff of a suburban Catchment in Nantes, France. 11th International Conference on Urban Drainage. Edinburgh, Scotland, 2008.
LAWA (2016). Ableitung von Geringfügigkeitsschwellenwerten für das Grundwasser. Stuttgart, Germany: Working Group of the Federal States on Water. https://www.lawa.de/documents/geringfuegigkeits_bericht_seite_001-028_1552302313.pdf.
Lee, J. H., Bang, K. W., Ketchum, L. H., Choe, J. S., and Yu, M. J. (2002). First Flush analysis of urban storm runoff. Sci. Total Environ. 293, 163–175. doi:10.1016/S0048-9697(02)00006-2
Lee, J. Y., Bak, G., and Han, M. (2012). Quality of roof-harvested rainwater - comparison of different roofing materials. Environ. Pollut. 162, 422–429. doi:10.1016/j.envpol.2011.12.005
Li, Y., and Babcock, R. W. (2014). Green roofs against pollution and climate change. A review. Agron. Sustain. Dev. 34, 695–705. doi:10.1007/s13593-014-0230-9
Liu, R., Stanford, R. L., Deng, Y., Liu, D., Liu, Y., and Yu, S. L. (2020). The influence of extensive green roofs on rainwater runoff quality: A field-scale study in southwest China. Environ. Sci. Pollut. Res. 27, 12932–12941. doi:10.1007/s11356-019-06151-5
Lye, D. J. (2009). Rooftop runoff as a source of contamination: A review. Sci. Total Environ. 407, 5429–5434. doi:10.1016/j.scitotenv.2009.07.011
Malcolm, E. G., Reese, M. L., Schaus, M. H., Ozmon, I. M., and Tran, L. M. (2014). Measurements of nutrients and mercury in green roof and gravel roof runoff. Ecol. Eng. 73, 705–712. doi:10.1016/j.ecoleng.2014.09.030
Mao, J., Xia, B., Zhou, Y., Bi, F., Zhang, X., Zhang, W., et al. (2021). Effect of roof materials and weather patterns on the quality of harvested rainwater in Shanghai, China. J. Clean. Prod. 279, 123419. doi:10.1016/j.jclepro.2020.123419
Melidis, P., Akratos, C. S., Tsihrintzis, V. A., and Trikilidou, E. (2007). Characterization of rain and roof drainage water quality in xanthi, Greece. Environ. Monit. Assess. 127, 15–27. doi:10.1007/s10661-006-9254-1
Mendez, C. B., Afshar, B. R., Kinney, K., Barrett, M. E., and Kirisits, M. J. (2010). Effect of roof material on water quality for rainwater harvesting systems. Austin, TX: Texas Water Development Board. https://www.twdb.texas.gov/innovativewater/rainwater/projects/rainquality/SOW_Web.pdf.
Müller, A., Österlund, H., Marsalek, J., and Viklander, M. (2020). The pollution conveyed by urban runoff: A review of sources. Sci. Total Environ. 709, 136125. doi:10.1016/j.scitotenv.2019.136125
Nawrot, N., and Wojciechowska, E. (2018). Assessment of trace metals leaching during rainfall events from building rooftops with different types of coverage - case study. J. Ecol. Eng. 19, 45–51. doi:10.12911/22998993/85410
Nosrati, K. (2017). Identification of a water quality indicator for urban roof runoff. Sustain. Water Qual. Ecol. 9–10, 78–87. doi:10.1016/j.swaqe.2017.07.001
Qin, H. P., Tang, Q. L., Wang, L. Y., and Fu, G. (2015). The impact of atmospheric wet deposition on roof runoff quality in an urbanized area. Hydrology Res. 46 (6), 880–892. doi:10.2166/nh.2015.209
Quek, U., and Förster, J. (1993). Trace metals in roof runoff. Water Air Soil Pollut. 68, 373–389. doi:10.1007/BF00478464
Schriewer, A., Horn, H., and Helmreich, B. (2008). Time focused measurements of roof runoff quality. Corros. Sci. 50, 384–391. doi:10.1016/j.corsci.2007.08.011
Seidl, M., Gromaire, M. C., Saad, M., and De Gouvello, B. (2013). Time focused measurements of roof runoff quality. Environ. Pollut. 183, 195–203. doi:10.1016/j.envpol.2013.05.026
Teemusk, A., and Mander, Ü. (2007). Rainwater runoff quantity and quality performance from a greenroof: The effects of short-term events. Ecol. Eng. 30, 271–277. doi:10.1016/j.ecoleng.2007.01.009
Thomas, P. R., and Greene, G. R. (1993). Rainwater quality from different roof catchments. Water Sci. Technol. 28 (3-5), 291–299. doi:10.2166/wst.1993.0430
Tobiszewski, M., Polkowska, Ż., Konieczka, P., and Namieśnik, J. (2010). Roofing materials as pollution emitters – concentration changes during runoff. Pol. J. Environ. Stud. 19, 1019–1028. ISSN:
Toland, D. C., Haggard, B. E., and Boyer, M. E. (2012). Evaluation of nutrient concentrations in runoff water from green roofs, conventional roofs, and urban streams. Am. Soc. Agric. Biol. Eng. 55 (1), 99–106. doi:10.13031/2013.41258
Van Seters, T., Rocha, L., Smith, D., and MacMillan, G. (2009). Evaluation of green roofs for runoff retention, runoff quality, and leachability. Water Qual. Res. J. Can. 44, 33–47. doi:10.2166/wqrj.2009.005
Vialle, C., Sablayrolles, C., Lovera, M., Jacob, S., Huau, M. C., and Montrejaud-Vignoles, M. (2011). Monitoring of water quality from roof runoff: Interpretation using multivariate analysis. Water Res. 45, 3765–3775. doi:10.1016/j.watres.2011.04.029
Vialle, C., Sablayrolles, C., Silvestre, J., Monier, L., Jacob, S., Huau, M. C., et al. (2013). Pesticides in roof runoff: Study of a rural site and a suburban site. J. Environ. Manag. 120, 48–54. doi:10.1016/j.jenvman.2013.02.023
Vijayaraghavan, K. (2016). Green roofs: A critical review on the role of components, benefits, limitations and trends. Renew. Sustain. Energy Rev. 57, 740–752. doi:10.1016/j.rser.2015.12.119
Wahyuningsih, N. D., Suwartha, N., Hartono, D. M., and Pratama, M. A. Evaluating the effect of roof type variations on the quality of rainwater runoff for rainwater harvesting development. AIP Conference Proceedings, 04 May 2020; , 2230, 050004. doi:10.1063/5.0002816
Winters, N., Granuke, K., and McCall, M. (2015). Roofing materials assessment: Investigation of five metals in runoff from roofing materials. water Environ. Res. 87, 835–844. doi:10.2175/106143015X14362865226437
Yaziz, M. I., Gunting, H., Sapari, N., and Ghazali, A. W. (1989). Variations in rainwater quality from roof catchments. Water Res. 23, 761–765. doi:10.1016/0043-1354(89)90211-X
Zdeb, M., Zamorska, J., Papciak, D., and Slys, D. (2020). The quality of rainwater collected from roofs and the possibility of its economic use. Resources 9, 12. doi:10.3390/resources9020012
Zhang, Q., Wang, X., Hou, P., Wan, W., Li, R., Ren, Y., et al. (2014). Quality and seasonal variation of rainwater harvested from concrete, asphalt, ceramic tile and green roofs in Chongqing, China. J. Environ. Manag. 132, 178–187. doi:10.1016/j.jenvman.2013.11.009
Keywords: stormwater runoff, roofing material, green roof, roof runoff, pollutants, nutrients, heavy metals
Citation: Degenhart J and Helmreich B (2022) Review on inorganic pollutants in stormwater runoff of non-metal roofs. Front. Environ. Chem. 3:884021. doi: 10.3389/fenvc.2022.884021
Received: 25 February 2022; Accepted: 28 July 2022;
Published: 30 August 2022.
Edited by:
Elza Bontempi, University of Brescia, ItalyReviewed by:
Laure Wiest, UMR5280 Institut des Sciences Analytiques (ISA), FranceHaitham Elnakar, King Fahd University of Petroleum and Minerals, Saudi Arabia
Copyright © 2022 Degenhart and Helmreich. This is an open-access article distributed under the terms of the Creative Commons Attribution License (CC BY). The use, distribution or reproduction in other forums is permitted, provided the original author(s) and the copyright owner(s) are credited and that the original publication in this journal is cited, in accordance with accepted academic practice. No use, distribution or reproduction is permitted which does not comply with these terms.
*Correspondence: Brigitte Helmreich, Yi5oZWxtcmVpY2hAdHVtLmRl