- 1Southwest Florida Research and Education Center, University of Florida, Immokalee, FL, United States
- 2Horticultural Sciences Department, University of Florida, Gainesville, FL, United States
- 3Citrus Research and Education Center, University of Florida, Lake Alfred, FL, United States
Chemical weed control using herbicide glyphosate to manage emerged weeds is an important production practice in Florida citrus. Despite the extensive use of glyphosate in citrus orchards, very limited information is available on its environmental fate and behavior in Florida soils that are predominantly sandy in nature. Hence, the study’s objective was to understand the adsorption-desorption, dissipation dynamics, and vertical movement or leaching of glyphosate in sandy soils in citrus orchards. Laboratory, field, and greenhouse experiments were conducted at Southwest Florida Research and Education Center in Immokalee, Florida. The adsorption-desorption behavior of glyphosate in the soils from three major citrus production areas in Florida was studied utilizing a batch equilibrium method. The dissipation of glyphosate was tracked in the field following its application at the rate of 4.20 kg ae ha−1. Soil leaching columns in greenhouse conditions were used to study the vertical movement of glyphosate. The results suggest that glyphosate has a relatively lower range of adsorption or binding (Kads = 14.28–30.88) in the tested soil types. The field dissipation half-life (DT50) of glyphosate from surface soil was found to be ∼26 days. Glyphosate moved vertically or leached into the soil profile, up to 40 cm in the soil column, when analyzed 40 days after herbicide application. The primary degradation product of glyphosate, i.e., aminomethyl phosphonic acid (AMPA), was also detected up to the depth of 30 cm below the soil surface, indicating the presence of microbial metabolism of glyphosate in the soil.
Introduction
Ever since the recognition of its phytotoxic activities in 1970s, glyphosate has been regarded as a relatively safe herbicide in the environment because it is quickly inactivated in the soil by adsorption and degradation (Quinn et al., 1988; Cuhra et al., 2016). However, prior reports have also indicated that improper application practices and excessive use of this herbicide may potentially contribute to its non-target movement and consequent effects in aquatic and terrestrial environments (Borggaard and Gimsing, 2008; Hanke et al., 2010; Cederlund and Cheng, 2017). Besides the direct sprays, glyphosate’s exposure routes in the environment may also include decaying plant residues and exudation from roots of sprayed plants (Neumann et al., 2006; Mamy et al., 2016), which is especially the case for genetically modified, glyphosate-tolerate crops with a high level of accumulated glyphosate (Bøhn et al., 2014). Furthermore, the adoption of agriculture crops with genetically introduced tolerance to glyphosate indirectly increases the risk of its excessive and non-judicious use of this herbicide in crop production, thereby raising more environmental and crop safety implications worldwide (Kanissery et al., 2019). Among these environmental concerns related to glyphosate, its persistence and movement within the soil profile and its effects on non-target components of the crop ecosystem have received the most attention and are deemed one of the most important factors to be addressed by scientific research.
The major pathway for glyphosate’s dissipation from the soil is microbial-mediated transformation or biodegradation (Sprankle et al., 1975; Torstensson, 1985; Gimsing et al., 2004b; Okada et al., 2019). Glyphosate may also be transported to groundwater, surface water, and water-sediment by processes like surface runoff, erosion, drift, and leaching (Newton et al., 1994; Ellis and Griffin, 2002; Shushkova et al., 2010; Bott et al., 2011; Lupi et al., 2015). Major factors that affect glyphosate’s persistence and movement in the soils include soil pH, soil type, mineralogy, texture, organic matter content, soil nutrient status, and surface vegetation cover (De Jonge et al., 2001; Laitinen et al., 2006; Mamy et al., 2016). Glyphosate-soil interaction occurs by processes such as adsorption and desorption; while both processes play important roles in determining the availability, persistence and movement of the herbicide in soil, relatively fewer studies have been conducted to evaluate the movement and environment fate in a noncontrolled environment (Borggaard and Gimsing, 2008; Aparicio et al., 2013). Understanding the topics of glyphosate-soil interactions will allow more informed usage of glyphosate as an effective, crop- and environmentally safe weed management tool.
Florida’s citrus-producing areas have soils with unique properties, characterized by very low organic matter content, sandy soil texture, low water holding capacity, etc. For instance, Immokalee and Fort Pierce, the major citrus production areas in southwest and southeast Florida, respectively, have poorly drained sandy soils with high water tables, whereas Lake Alfred, in the central production area, is known for its extremely well-drained sandy soils with low water holding capacity (Obreza and Collins, 2008; Obreza and Morgan, 2008). Although glyphosate is an extensively used herbicide in citrus weed management in the state (USDA, 2018), scarce information is available on the herbicide’s fate and behavior in these soils. Hence, it is imperative to understand how glyphosate binds, persists and moves in Florida’s soils to improve its utilization in citrus weed management. To address this need, we evaluated the adsorption and desorption pattern of glyphosate in soils from three major citrus-producing areas (southwest, southeast, and central) of Florida in a laboratory study. Moreover, field and soil column studies were conducted in southwest Florida soil to characterize glyphosate dissipation and movement.
Materials and Methods
Adsorption-Desorption Study
Soil Collection
The soils used for this study were collected from citrus orchards in three major citrus-producing areas with similar sandy soil types in Florida: southwest (Location: Immokalee; latitude: 26°28′7″N, longitude: 81°26°26′22″W), southeast (Location: Fort Pierce; latitude: 28°26°6′7″N, longitude: 81°26°41′35″W) and central (Location: Lake Alfred; latitude: 2726°26′7″N, longitude: 80°26°25′25″W). Soils without a history of glyphosate application in the past 2 years were chosen in each sampling location. Soils were collected from the top layer (15 cm depth) at five different sampling points in a transect fashion, and each of these three locations is treated as a spatial replicate. Following the collection, a composite sample for each location was prepared by mixing all the subsamples and was stored at -20 oC until the experiment. The soil samples were air-dried, sieved through a 2-mm mesh before use in experiments. The relevant physico-chemical properties of the soils are provided in Table 1 (Analysis by Waters Agricultural Laboratories, Camilla, GA).
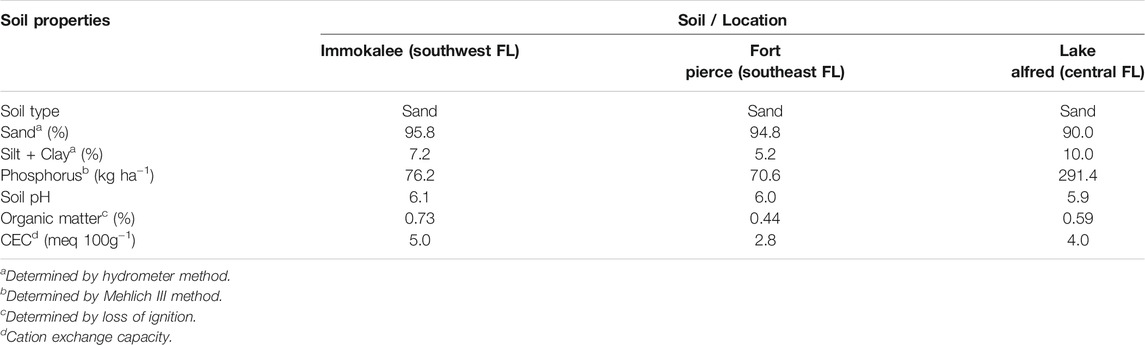
TABLE 1. Selected physical and chemical properties of the citrus production soils (top 15 cm) used in this study.
Chemicals and Reagents
The analytical standard (99.99% purity) of glyphosate in water (1,000 mg L−1) was procured from Restek (Bellefonte, PA, United States). Five different concentrations (5, 10, 25, 50, and 100 mg L−1) of glyphosate were prepared in 0.1 M (M) potassium chloride (KCl) solution in water (Gimsing and Borggaard, 2001). All the chemicals, reagents and solvents used in this experiment were of analytical grade and obtained from Fisher Scientific (Pittsburgh, PA).
Experimental Protocol
The methodology for determining adsorption isotherms was established according to the adsorption/desorption batch equilibrium study in the environmental protection agency’s test guidelines (USEPA, 2008). A preliminary study based on these guidelines was carried out to determine the optimum soil/solution ratio for this experiment and is provided in the supplemental information (Supplementary Table S1; Supplementary Figure S1). As suggested by the preliminary study (1:5 soil/solution ratio), 4 g of air-dried soil were weighed and equilibrated with 20 ml of glyphosate test concentrations (5, 10, 25, 50, and 100 mg L−1), prepared in 0.1 M KCl solution. The soil was shaken with the glyphosate solutions in 40 ml Teflon centrifuge tubes with a threaded cap on a horizontal shaker (200 rpm) for 24 h (Accinelli et al., 2005). The likelihood of significant degradation of glyphosate during this contact time (24 h) with soil during the shaking is assessed to be negligible previously (Bergström et al., 2011). The study was conducted in duplicate for each soil type and each concentration in a semi-dark room with a controlled temperature of 25 ± 2 oC. At the end of shaking time, following the equilibration of soil and glyphosate solution, the resultant soil suspension in the tubes was centrifuged at 6,000 rpm for 15 min. Subsequently, after centrifugation, two 1-ml aliquots were taken from the supernatant of each sample. The glyphosate concentration in each aliquot of aqueous soil solution was determined by high-performance liquid chromatography-mass spectrometry (HPLC-MS) at Southwest Florida Research and Education Center (SWFREC), Immokalee, FL. The HPLC-MS conditions for analysis of glyphosate in an aqueous solution are provided as supplemental information (Supplementary Table S2). The limit of detection for glyphosate in the analysis was 500 μg L−1. Two blanks (treatments without glyphosate) and controls (treatment without soil) for each concentration and soil type were also included in the study for calibration and background correction process. Background glyphosate content was not detected in any test soils (Supplementary Table S3), and significant adsorption of glyphosate on the tubes was not observed (Supplementary Table S4). The amount of herbicide adsorbed to the soil was determined by calculating the difference between herbicide concentration in the aqueous soil solution at equilibrium and initial concentration in the shaking solution.
Following the adsorption experiment, the desorption of glyphosate from soils was determined. The supernatant from the adsorption study was removed, and an equal amount (20 ml) of fresh 0.1 M KCl solution was added again to each Teflon tube. Soil aggregates were dispersed by vibration, and the contents of all the tubes were homogenized on a horizontal table shaker for 24 h at 200 rpm. Following re-equilibration, the Teflon tubes were centrifuged (15 min, 6,000 rpm), and 1 ml aliquots of the supernatant were pipetted into plastic vials in duplicates. The supernatants were then analyzed for glyphosate concentration by HPLC-MS. The desorbed amounts were calculated by analyzing the amount of herbicide in the aqueous solution following equilibration.
Field Dissipation Study
Experimental Design and Treatments
The dissipation of glyphosate was determined concurrently from a field experiment evaluating glyphosate effects on young citrus trees in a newly established citrus orchard located at SWFREC, Immokalee, Florida. The experiment had a randomized complete block design (RCBD) with four replications. Each replication (experimental plot) consisted of five young trees. One tree was left as a buffer between experimental plots. The experimental site did not have any prior application history of glyphosate-based herbicide products. For this study, herbicide was applied once during the fall season of 2018. The treatments consisted of glyphosate applied at 4.20 kg ae ha−1, which is within the recommended range of glyphosate rates in citrus (University of Florida, 2020) and an untreated control consisting of water spray.
Herbicide Application and Orchard Management
The herbicide spray solutions were made by mixing the appropriate amount of glyphosate product (Roundup custom™, 53.8% glyphosate in the form of its isopropylamine salt, EPA registration number 524–343) in distilled water according to manufacturer recommendation. The carrier volume selected was 280 L ha−1. Herbicide solution was applied using a handheld backpack sprayer to the tree rows, i.e., roughly one square meter around each tree. Weed pressure was minimal around trees during the application. For nutrition, irrigation, disease, and pest management, recommended orchard management practices were followed throughout the study (University of Florida, 2020). The citrus trees received homogenous irrigation with the micro-sprinklers twice a week for 2 h each day. The daily rainfall data were obtained from the Florida automated weather network (FAWN) weather station at SWFREC.
Soil Sampling and Analysis
The soil was sampled from the top 15 cm depth at pre-determined intervals (0, 7, 14, 28, 42, 56, 70, and 90 days after treatment) beginning from the first day after herbicide application. The soil samples were immediately stored at -20oC after sampling until analysis of the soil samples for glyphosate. For assessing the field dissipation kinetics, soil samples from the experimental plots collected at different time points were analyzed for herbicide content. To reduce the analytical costs, a total of two composite samples for each time point were formed by combining the soil samples from four replications before the analysis (USEPA, 1995). The analysis of glyphosate content in the soil samples was performed using HPLC-MS/MS in a commercial laboratory (Waters Agricultural Laboratories, Camilla, GA). Glyphosate was extracted from the soil samples, and analysis was conducted utilizing methods described in Gorica et al., 2016. The method detection limit for glyphosate was 50 μg kg−1 soil.
Soil Column Leaching Study
Column Preparation
Soil leaching columns (130 cm in length, 10 cm in diameter) made of polyvinyl chloride (PVC) pipes were set up in the greenhouse at SWFREC. The columns were filled with pre-collected soil from the incremental depths (up to 120 cm) from a site (without prior glyphosate application history) adjacent to a citrus orchard in Immokalee to mimic the soil depth profile of the orchard (Figure 1A). The column was fitted with a PVC cap at the bottom, equipped with a nylon screen and Whatman no. 4 filter paper to collect the leachate, if any. The columns were secured upright in a specially prepared wooden stand (Figures 1B,C), and each column was watered to field capacity and allowed to drain for 24 h.
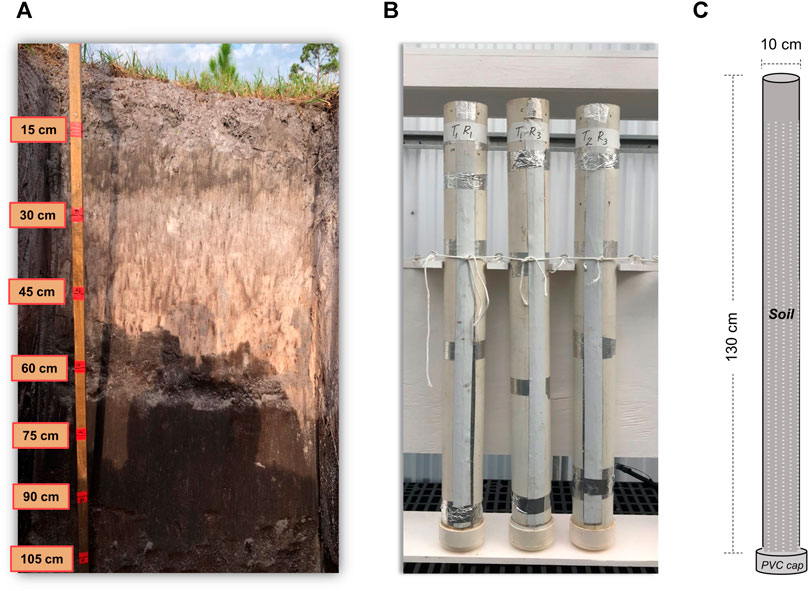
FIGURE 1. (A) Picture of soil profile used for packing the columns (B) Picture of soil leaching columns used in the experiment (C) Diagram of a soil leaching column.
Experimental Design and Treatments
The experiment was set up in a completely randomized design (CRD) with three replications. The treatments consisted of glyphosate application (4.20 kg ae ha−1) and untreated control (water spray). Control was included for background correction purposes. Glyphosate (Roundup Custom™) was mixed thoroughly in deionized water (carrier volume = 280 L ha−1) to prepare the herbicide solutions and applied with a handheld CO2 backpack sprayer to the soil surface in the soil column. The frequency and volume of water being added to a citrus orchard per unit area through irrigation were calculated and applied to the column regularly (two times a week) to simulate field irrigation. The soil columns were kept intact until sampling (for 40 days).
Sample Collection and Analysis
After 40 days, the PVC cap at the end of the soil column was removed, and the columns were split longitudinally by removing the duct tape from one side. The soil in the columns was collected in an interval of 10 cm depths for the column’s entire length. The collected soil samples were analyzed for parent herbicide, glyphosate, and its primary metabolite aminomethylphosphonic acid (AMPA) content using HPLC-MS/MS (Gorica et al., 2016) in a commercial laboratory (Waters Agricultural Laboratories, Camilla, GA). The method detection limit for glyphosate and AMPA were 50 and 120 μg kg−1 soil, respectively.
Data Analysis
The adsorption and desorption isotherms were fitted using the transformed Freundlich isotherm equation: log Cs = log Kf + 1 /n log Ce, where “Cs” is the concentration of glyphosate adsorbed in the soil (mg kg−1), Kf is the equilibrium constant of Freundlich reflecting the binding affinity of the soil for the herbicide, ‘Ce” is the concentration of glyphosate in the solution (mg L−1) at equilibrium, and 1/n is the degree of linearity of the isotherm. The K (intercept) and 1/n (slope) were calculated using regression analysis in the adsorption and desorption isotherms.
The field dissipation data of glyphosate in soils were fitted into the first-order kinetics model Ct = C0 e-kt where ‘C0” is the initial concentration (µg kg−1 soil) of the herbicide in the soil, “Ct” is the herbicide concentration (µg kg−1 soil) detected in the soil at time t, and k is the first-order rate constant. Computer Assisted Kinetic Evaluation (CAKE) (version 3.3; Tessella, Newton, MA) software was utilized to model the dissipation kinetics and estimate the first-order dissipation parameters.
PROC GLM statement in SAS (version 9.4; SAS Institute, Cary, NC) software was used for the analysis of variance (ANOVA), and mean separation was achieved using Tukey’s HSD test at p ≤ 0.05.
Results and Discussion
Adsorption-Desorption Study
For the range of herbicide concentrations (5–100 mg L−1) and soils, adsorption data from the current experiment were well-fitted into the Freundlich isotherm model (R2 > 0.91). Figure 2 demonstrates the adsorption isotherm plots, and Table 2 shows the calculated Freundlich adsorption coefficient (Kads), slope (1/nads), and the corresponding goodness of fit (R2) for glyphosate adsorption in various soil types tested. Among the different soils, the slope (1/nads) values ranged from 0.27 to 0.43, and the Freundlich adsorption coefficient (Kads) values ranged from 14.3 to 30.9. A lower Kads value indicates a low adsorption affinity of the soil to the herbicide. The soil from Lake Alfred location showed a significantly higher (p ≤ 0.05) adsorption coefficient than the soils from Immokalee and Fort Pierce. The adsorption coefficients for Immokalee and Fort Pierce soil were found to be similar (Table 2).
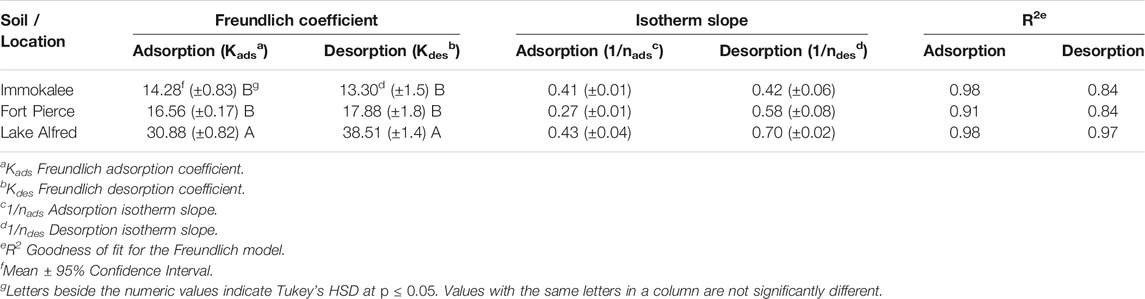
TABLE 2. Adsorption and desorption (Freundlich model) parameters of glyphosate in Florida’s citrus production soils.
Desorption isotherms for glyphosate in all the soils also fitted into the Freundlich model (R2 > 0.84) (Table 2). Freundlich desorption coefficient (Kdes) values of glyphosate were considerably lower in the Immokalee and Fort Pierce soils than the Lake Alfred soil. A lower Kdes value indicates lesser retention of herbicide on the soil surface, and consequently, higher release into the soil solution.
The adsorption parameter, Kads, observed in the current study, agrees with the previous observation from similar soil types, where glyphosate had a Kads of 34.5 in sandy loam soil (Al-Rajab et al., 2008). The results obtained from the adsorption-desorption study are in the lower range of Kads and 1/n values obtained for glyphosate from different soil types and crop production systems. There is a large variation in the literature values, with Kads ranging from 0.6 to 5.0 x 105 and 1/n ranging from 0.26 to 1.26 (Vereecken, 2005). This variability in glyphosate adsorption is attributed to soils’ physical and chemical heterogeneity (Al-Rajab et al., 2008). The lower adsorption values of glyphosate obtained from the present study indicate lower soil adsorption affinity of this herbicide in all three citrus-producing locations. Clay and organic matter content of soils tend to affect glyphosate adsorption positively (Vereecken, 2005). The citrus growing soils in Florida are predominantly sandy (>90% sand) and low in organic matter (Obreza and Collins, 2008) and could be a plausible reason behind relatively lower adsorption and higher desorption of glyphosate in these soil types. Soil organic matter (SOM) seems to play a questionable role in soil adsorption of glyphosate. On the one hand, investigations have shown that soil sorption of glyphosate is positively correlated with SOM content (Piccolo et al., 1996). However, there are reports that it sometimes negatively impacts glyphosate adsorption to soil (Gimsing et al., 2004a).
Also, it has to be noted that adsorption was comparatively higher in soil from Lake Alfred compared to the Immokalee and Fort Pierce soils. Lake Alfred soil has more silt and clay, and phosphorus (P) than the other two soils tested in this study (Table 1). Although most herbicides, due to their non-polar functional groups, are adsorbed in soils primarily by SOM, glyphosate, a small molecule with three polar functional groups (carboxyl, amino and phosphonate groups), is strongly adsorbed by soil minerals (Borgaard and Elberling, 2007). Hence, clay minerals like iron (Fe) and aluminum (Al) oxides in the soil can provide binding or adsorption sites to the glyphosate (Gimsing and Borggaard 2002; Kanissery et al., 2015). Likewise, sand particles coated with Fe and Al sesquioxides (also known as ‘coated sands’) have been found to improve P adsorption and retention in Florida soil (Obreza and Collins, 2008). It is well known that glyphosate and phosphate are structurally analogous and share similar adsorption mechanisms in soil (Gerritse et al., 1996; Gimsing and Borggaard 2001; Wang et al., 2005). The active phosphonate end group in the glyphosate molecule allows it to form inner-sphere complexes on Fe and Al oxide surfaces (Gimsing and Borggaard 2002; Sheals et al., 2002). Hence, significantly higher amounts of phosphorus observed in the Lake Alfred soil (Table 1) could be an indication of better availability of adsorption sites in that soil, owing to the presence of coated sands, and could be a probable reason behind the higher adsorption of glyphosate in this particular soil.
The value of slope (1/n) < 0.5 in the adsorption model (Table 2) indicates a strong non-linear relationship between the amount of herbicide adsorbed onto the soil surface and its concentration in the aqueous solution. The result indicates that less glyphosate will be adsorbed in the soil as the glyphosate concentration in the solution increases, possibly because most of the adsorption sites are bound to glyphosate, and fewer sites are available for adsorption. This observation suggests a high potential for glyphosate leaching when higher concentrations of this herbicide are present in the soil profile.
Soils with a lower adsorption affinity showed greater desorption, as evident by the Kdes value (Table 2). Higher desorption or release of glyphosate from Immokalee and Fort Pierce soils suggests that the herbicide’s mobility for leaching losses or its availability for biodegradation and plant uptake in these soils will be higher. The relatively lower desorption in the Lake Alfred soil suggests that glyphosate will be less mobile and more bound to this soil than the other two citrus production soils tested. Generally, glyphosate is non-bioavailable and inactive when bound or adsorbed to soils (Sorensen et al., 2006).
Field Dissipation Study
The dissipation parameters of glyphosate obtained from the field study conducted in Immokalee, FL, are presented in Table 3. The dissipation of glyphosate from the surface soil (top 15 cm) over time (Figure 3) adequately fitted into a first-order kinetic model with an R2 value of 0.88. The first-order rate constant (k) value was 0.026 days−1. The dissipation half-life (DT50: time for dissipating 50% of initially applied herbicide from the soil) of glyphosate from the surface soil was 25.9 days. The time for dissipating 90% of initial herbicide (DT90) was 86 days. The cumulative amount of precipitation received in the experimental site during the dissipation study duration is shown in the supplemental materials (Supplementary Figure S2).
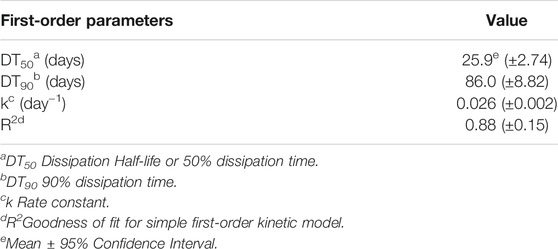
TABLE 3. First-order field dissipation parameters of glyphosate in a citrus orchard (Location: Immokalee, FL).
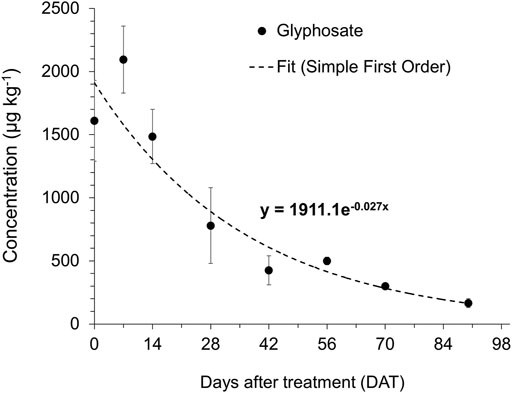
FIGURE 3. Field dissipation kinetics of glyphosate (4.20 kg ae ha−1) in a citrus orchard (Location: Immokalee, FL). Error bars, wherever visible, indicate 95% Confidence Interval. The regression equation for the simple first-order kinetic model (Ct = C0 e-kt) is shown.
The half-life of glyphosate applied in the field (∼26 days) was well within the range of half-lives in similar soil types reported previously (Okada et al., 2019); between 9 and 38 days. Glyphosate DT50 can vary from a few days to months and even years, depending upon several factors, including soil type, texture, organic matter content and other soil environmental conditions (Sprankle et al., 1975; Nomura and Hilton 1977; Carlisle and Trevors 1988). The glyphosate dissipation was well-fitted and described using first-order kinetics, showing rapid initial dissipation of glyphosate followed by a decreased glyphosate dissipation rate which are in agreement with previously conducted research (Mamy et al., 2005; Bergström et al., 2011; Ghafoor et al., 2011). Interestingly, the detected concentration of glyphosate peaked at 7 days after application and then began to decline. A similar observation was made by (Salazar López et al., 2016), where glyphosate concentrations reached a maximum in soil 10–18 days after application. Although the bulk of the applied glyphosate quickly dissipated from the soil surface in the current study, traces of herbicide could remain in the top 15 cm layer almost 3 months after the application, as noted from a prolonged DT90. Researchers have reported a DT90 of over 7 months for glyphosate in certain cases, particularly when the soils are rich in silt and clay content (Okada et al., 2019).
Dissipation of herbicides from the soil can occur through microbially or chemically mediated transformations, surface or subsurface runoff, and leaching. Physico-chemical processes like adsorption and leaching in the soil and microbial degradation are considered primary determinant factors for the glyphosate dissipation from the applied area (Laitinen, 2009). The relatively lower adsorption affinity of glyphosate to sandy soils in citrus orchards in Florida observed from the current study could be potentially implied as a reason behind the quick dissipation of glyphosate, owing to its enhanced mobility from the soil surface.
Soil Column Leaching Study
Figure 4 shows the vertical leaching of glyphosate at different depths in the soil columns. Glyphosate was detected up to 40 cm below the surface in the soil profile columns when they were opened and analyzed 40 days after the herbicide application. The primary degradation product of glyphosate, AMPA, was also detected up to the depth of 20–30 cm below the soil surface. About 35% of applied glyphosate was retained in the top 0–10 cm, and 28% of applied herbicide was found accumulated in the 10–20 cm soil layer, and ∼81% of the initially applied glyphosate was detected within a soil depth of 40 cm in the column. Glyphosate was not detected from the samples beyond 40 cm deep from the herbicide-treated columns or any depth samples in untreated control columns. Analysis of leachate for herbicide content was not performed as only an insignificant amount of leachate was collected at the column’s bottom cap. Also, leachate was observed to be turbid and brownish resulting from soil colloids.
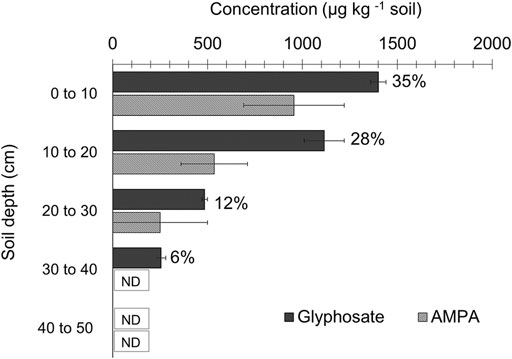
FIGURE 4. Vertical movement of glyphosate and AMPA in the soil leaching columns (40 Days after treatment–DAT). Error bars, wherever visible, indicate 95% Confidence Interval. Values near the glyphosate bars in the graph indicate glyphosate accumulation as the percentage of herbicide initially applied. ND: not detected. Data showed up to 50 cm depth in the soil columns; Glyphosate or AMPA was not detected at or below this depth in the columns.
Glyphosate has moved vertically up to 40 cm in the soil, as observed from soil columns. A similar trend was observed by Laitinen. (2009), who found glyphosate’s movement up to 35 cm in a soil profile, 38 days after the herbicide application. The loss of mass balance of glyphosate in the soil columns (recovery from various depths accounts for 81% of the initially applied amount) in the current study could be attributed to the herbicide’s microbial transformation. The primary metabolite AMPA was detected at various soil depths in the columns (Figure 4). Most citrus-producing soils in Florida, including the Immokalee soil used in this column study, have low soil organic matter (SOM) (Table 1). As SOM is closely linked to soil microorganisms, the potential for microbial transformation of herbicides is expected to be relatively low in these soils. However, the detection of AMPA in our soil columns, the most common product of glyphosate’s microbial metabolism (Dick and Quinn 1995), indicates the presence of microbial transformation as a contributing mechanism for glyphosate dissipation and loss from the citrus orchards.
Only 35% of the applied glyphosate stayed in the top 10 cm in the column, followed by relatively lower concentrations in the subsequent soil depths. The vertical movement of glyphosate through the soil profile could be associated with its hydrophilicity and high-water solubility. The soil type utilized for this column study (Immokalee soil) is notably very sandy (>90% sand) with low clay content characterized by lower herbicide retention. Therefore, this may have promoted glyphosate leaching from the surface soils, especially when coupled with simulated citrus tree irrigation in the soil columns. On the other hand, the soils from this location have poor drainage properties due to a subsurface organic hardpan (Obreza and Collins 2008) that possibly restricted the downward movement of the herbicide (>40 cm) into deeper soil layers. Comparable reports on relatively low leaching and mobility of glyphosate have been reported from a previous study conducted in sandy soils. Researchers attribute this to the strong adsorption of glyphosate to soils. Furthermore, degradation of glyphosate to its major metabolite, AMPA, was also observed in the studied soil (Zhou et al., 2010). High rates of glyphosate leaching will be expected in tropical sandy soils like citrus production soils in FL due to their low adsorption capacity and relatively high precipitation. However, field investigations and column studies have shown that physical properties, like porosity, compaction etc., greatly determine the mobility of glyphosate in these soils. For instance, Kjær et al., 2005 showed minimal leaching of glyphosate (or AMPA) in sandy soils and is attributed to the absence of macropores in these soils. Sandy soils in the tropics offer a wide range of such properties (Bruand et al., 2005) and must be considered while predicting the leaching and movement of glyphosate in such soils. The fact that the citrus tree root zone in these types of soils generally occurs within 0–45 cm (Leiva et al., 2015) entails further investigations into the retention of this herbicide in the citrus root zone for an extended period, thus enabling the uptake of its residues by roots (Cornish and Burgin, 2005; Tong et al., 2017).
Conclusion
Glyphosate was found to have low adsorption or binding affinity to the soils from all three citrus-producing locations in Florida. Among the various production areas, soil from Lake Alfred in the central area had higher adsorption for glyphosate than the other two areas, Immokalee in the southwest and Fort Pierce in the southeast. Field dissipation of glyphosate from surface soils in citrus orchard was relatively fast, with a calculated DT50 of ∼26 days. The herbicide moved vertically from the surface soils up to 40 cm in soil profile columns within 40 days after herbicide application. The current study is among the first attempts to evaluate glyphosate’s interaction with FL sandy soils under citrus production to the best of our knowledge. The information generated in this study could be utilized to better understand glyphosate’s fate and behavior in citrus and other similar production systems in sandy soils. Additionally, these observations may be used to improve glyphosate’s crop-safe application while minimizing the non-target effects and other environmental implications. Due to the low adsorption of glyphosate observed in citrus soils of FL, the likelihood of its availability to reach unintended areas in citrus orchards through processes such as leaching and surface runoff is relatively high. Although found to dissipate relatively quickly from the topsoil following application, its potential to persist in the deeper layers of soils and consequent interaction with the citrus tree needs to be explored more thoroughly. Being the most widely used herbicide in citrus production in the region, findings from this study entail adopting glyphosate’s judicious utilization to enhance its use as a weed control tool in citrus production.
Data Availability Statement
The raw data supporting the conclusions of this article will be made available by the authors, without undue reservation.
Author Contributions
Conceptualization, RK and BG; Methodology, BG and RK; Investigation, BG; Resources, RK; Writing–Original Draft Preparation, BG; Writing–Review and Editing, RK, WL, OB, PD, and DK; Supervision, RK; Project Administration, RK; Funding Acquisition, RK.
Conflict of Interest
The authors declare that the research was conducted in the absence of any commercial or financial relationships that could be construed as a potential conflict of interest.
Publisher’s Note
All claims expressed in this article are solely those of the authors and do not necessarily represent those of their affiliated organizations, or those of the publisher, the editors and the reviewers. Any product that may be evaluated in this article, or claim that may be made by its manufacturer, is not guaranteed or endorsed by the publisher.
Acknowledgments
The authors thank the University of Florida and Southwest Florida Research and Education Center for assistance with the project.
Supplementary Material
The Supplementary Material for this article can be found online at: https://www.frontiersin.org/articles/10.3389/fenvc.2021.737391/full#supplementary-material
References
Accinelli, C., Koskinen, W. C., Seebinger, J. D., Vicari, A., and Sadowsky, M. J. (2005). Effects of Incorporated Corn Residues on Glyphosate Mineralization and Sorption in Soil. J. Agric. Food Chem. 53 (10), 4110–4117. doi:10.1021/jf050186r
Al-Rajab, A. J., Amellal, S., and Schiavon, M. (2008). Sorption and Leaching of 14C-Glyphosate in Agricultural Soils. Agron. Sustain. Dev. 28 (3), 419–428. doi:10.1051/agro:2008014
Aparicio, V. C., De Gerónimo, E., Marino, D., Primost, J., Carriquiriborde, P., and Costa, J. L. (2013). Environmental Fate of Glyphosate and Aminomethylphosphonic Acid in Surface Waters and Soil of Agricultural Basins. Chemosphere 93 (9), 1866–1873. doi:10.1016/j.chemosphere.2013.06.041
Bergström, L., Börjesson, E., and Stenström, J. (2011). Laboratory and Lysimeter Studies of Glyphosate and Aminomethylphosphonic Acid in a Sand and a clay Soil. J. Environ. Qual. 40 (1), 98–108. doi:10.2134/jeq2010.0179
Bøhn, T., Cuhra, M., Traavik, T., Sanden, M., Fagan, J., and Primicerio, R. (2014). Compositional Differences in Soybeans on the Market: Glyphosate Accumulates in Roundup Ready GM Soybeans. Food Chem. 153, 207–215. doi:10.1016/j.foodchem.2013.12.054
Borggaard, O. K., and Elberling, B. O. (2007). Pedological Biogeochemistry. Department of Natural Sciences, University of Copenhagen and Department of Geography and Geology, University of Copenhagen.
Borggaard, O. K., and Gimsing, A. L. (2008). Fate of Glyphosate in Soil and the Possibility of Leaching to Ground and Surface Waters: a Review. Pest Manag. Sci. 64 (4), 441–456. doi:10.1002/ps.1512
Bott, S., Tesfamariam, T., Kania, A., Eman, B., Aslan, N., Römheld, V., et al. (2011). Phytotoxicity of Glyphosate Soil Residues Re-mobilized by Phosphate Fertilization. Plant and soil. 342 (1), 249–263. doi:10.1007/s11104-010-0689-3
Bruand, A., Hartmann, C., and Lesturgez, G. (2005). “Physical Properties of Tropical sandy Soils: A Large Range of Behaviours,” in Management Of Tropical Sandy Soils For Sustainable Agriculture. A Holistic Approach for Sustainable Development of Problem Soils in the Tropics.
Carlisle, S. M., and Trevors, J. T. (1988). Glyphosate in the Environment. Water Air Soil Pollut. 39 (3), 409–420.
Cederlund, H. (2017). Effects of spray Drift of Glyphosate on Nontarget Terrestrial Plants-A Critical Review. Environ. Toxicol. Chem. 36 (11), 2879–2886. doi:10.1002/etc.3925
Cheng, H. H. (1990). “Pesticides in the Soil Environment—An Overview,” in Pesticides in the Soil Environment: Processes, Impacts and Modeling, Volume 2, 1–5.
Cornish, P. S., and Burgin, S. (2005). Residual Effects of Glyphosate Herbicide in Ecological Restoration. Restor Ecol. 13 (4), 695–702. doi:10.1111/j.1526-100x.2005.00088.x
Cuhra, M., Bøhn, T., and Cuhra, P. (2016). Glyphosate: Too Much of a Good Thing? Front. Environ. Sci. 4, 28. doi:10.3389/fenvs.2016.00028
De Jonge, H., De Jonge, L. W., Jacobsen, O. H., Yamaguchi, T., and Moldrup, P. (2001). Glyphosate Sorption in Soils of Different pH and Phosphorus Content. Soil Sci. 166 (4), 230–238. doi:10.1097/00010694-200104000-00002
Dick, R. E., and Quinn, J. P. (1995). Glyphosate-degrading Isolates from Environmental Samples: Occurrence and Pathways of Degradation. Appl. Microbiol. Biotechnol. 43 (3), 545–550. doi:10.1007/bf00218464
Ellis, J. M., and Griffin, J. L. (2002). Soybean (Glycine max) and Cotton (Gossypium Hirsutum) Response to Simulated Drift of Glyphosate and Glufosinate1. Weed Tech. 16 (3), 580–586. doi:10.1614/0890-037x(2002)016[0580:sgmacg]2.0.co;2
Gerritse, R., Beltran, J., and Hernandez, F. (1996). Adsorption of Atrazine, Simazine, and Glyphosate in Soils of the Gnangara Mound, Western Australia. Soil Res. 34 (4), 599–607. doi:10.1071/sr9960599
Ghafoor, A., Jarvis, N. J., Thierfelder, T., and Stenström, J. (2011). Measurements and Modeling of Pesticide Persistence in Soil at the Catchment Scale. Sci. Total Environ. 409 (10), 1900–1908. doi:10.1016/j.scitotenv.2011.01.049
Gimsing, A. L., Borggaard, O. K., and Bang, M. (2004a). Influence of Soil Composition on Adsorption of Glyphosate and Phosphate by Contrasting Danish Surface Soils. Eur. J. Soil Sci. 55 (1), 183–191. doi:10.1046/j.1365-2389.2003.00585.x
Gimsing, A. L., and Borggaard, O. K. (2002). Competitive Adsorption and Desorption of Glyphosate and Phosphate on clay Silicates and Oxides. Clay miner. 37 (3), 509–515. doi:10.1180/0009855023730049
Gimsing, A. L., and Borggaard, O. K. (2001). Effect of KCl and CaCl2 as Background Electrolytes on the Competitive Adsorption of Glyphosate and Phosphate on Goethite. Clays. Clay Minerals. 49 (3), 270–275. doi:10.1346/ccmn.2001.0490310
Gimsing, A. L., Borggaard, O. K., Jacobsen, O. S., Aamand, J., and Sørensen, J. (2004c). Chemical and Microbiological Soil Characteristics Controlling Glyphosate Mineralisation in Danish Surface Soils. Appl. Soil Ecol. 27 (3), 233–242. doi:10.1016/j.apsoil.2004.05.007
Gimsing, A. L., Borggaard, O. K., and Sestoft, P. (2004b). Modeling the Kinetics of the Competitive Adsorption and Desorption of Glyphosate and Phosphate on Goethite and Gibbsite and in Soils. Environ. Sci. Technol. 38 (6), 1718–1722. doi:10.1021/es030572u
Gorica, V., Vojislava, B., Miroslav, A., Tijana, Z., and Đurović-Pejčev, R. (2016). “Polar Pesticide Analyses: Validation of Glyphosate Determination in Soil by LC-MS/MS,” in 22nd International Symposium on Analytical and Environmental Problems. Szeged, Hungary, 22.
Hanke, I., Wittmer, I., Bischofberger, S., Stamm, C., and Singer, H. (2010). Relevance of Urban Glyphosate Use for Surface Water Quality. Chemosphere 81 (3), 422–429. doi:10.1016/j.chemosphere.2010.06.067
Kanissery, R., Gairhe, B., Kadyampakeni, D., Batuman, O., and Alferez, F. (2019). Glyphosate: Its Environmental Persistence and Impact on Crop Health and Nutrition. Plants 8 (11), 499. doi:10.3390/plants8110499
Kanissery, R. G., Welsh, A., and Sims, G. K. (2015). Effect of Soil Aeration and Phosphate Addition on the Microbial Bioavailability of Carbon-14-Glyphosate. J. Environ. Qual. 44 (1), 137–144. doi:10.2134/jeq2014.08.0331
Kjaer, J., Olsen, P., Ullum, M., and Grant, R. (2005). Leaching of Glyphosate and Amino-Methylphosphonic Acid from Danish Agricultural Field Sites. J. Environ. Qual. 34 (2), 608–620. doi:10.2134/jeq2005.0608
Laitinen, P. (2009). Fate Of the Organophosphate Herbicide Glyphosate in Arable Soils and its Relationship to Soil Phosphorus Status. Doctoral Dissertation. MTT Agrifood Research Finland.
Laitinen, P., Siimes, K., Eronen, L., Rämö, S., Welling, L., Oinonen, S., et al. (2006). Fate of the Herbicides Glyphosate, Glufosinate-Ammonium, Phenmedipham, Ethofumesate and Metamitron in Two Finnish Arable Soils. Pest Manag. Sci. 62 (6), 473–491. doi:10.1002/ps.1186
Leiva, J. A., Nkedi-Kizza, P., Morgan, K. T., and Qureshi, J. A. (2015). Imidacloprid Sorption Kinetics, Equilibria, and Degradation in sandy Soils of Florida. J. Agric. Food Chem. 63 (20), 4915–4921. doi:10.1021/acs.jafc.5b00532
Lupi, L., Miglioranza, K. S. B., Aparicio, V. C., Marino, D., Bedmar, F., and Wunderlin, D. A. (2015). Occurrence of Glyphosate and AMPA in an Agricultural Watershed from the southeastern Region of Argentina. Sci. total Environ. 536, 687–694. doi:10.1016/j.scitotenv.2015.07.090
Mamy, L., Barriuso, E., and Gabrielle, B. (2005). Environmental Fate of Herbicides Trifluralin, Metazachlor, Metamitron and Sulcotrione Compared with that of Glyphosate, a Substitute Broad Spectrum Herbicide for Different Glyphosate-Resistant Crops. Pest Manag. Sci. 61 (9), 905–916. doi:10.1002/ps.1108
Mamy, L., Barriuso, E., and Gabrielle, B. (2016). Glyphosate Fate in Soils when Arriving in Plant Residues. Chemosphere 154, 425–433. doi:10.1016/j.chemosphere.2016.03.104
Neumann, G., Kohls, S., Landsberg, E., Stock-Oliveira Souza, K., Yamada, T., and Romheld, V. (2006). Relevance of Glyphosate Transfer to Non-target Plants via the Rhizosphere. J.Plant Diseases and Protection. ZEITSCHRIFT PFLANZENKRANKHEITEN PFLANZENSCHUTZ-SONDERHEFT- 20, 963.
Newton, M., Horner, L. M., Cowell, J. E., White, D. E., and Cole, E. C. (1994). Dissipation of Glyphosate and Aminomethylphosphonic Acid in North American Forests. J. Agric. Food Chem. 42 (8), 1795–1802. doi:10.1021/jf00044a043
Nomura, N. S., and Hilton, H. W. (1977). The Adsorption and Degradation of Glyphosate in Five Hawaiian Sugarcane Soils*. Weed Res. 17 (2), 113–121. doi:10.1111/j.1365-3180.1977.tb00454.x
Obreza, T. T., and Collins, M. E. (2008). Common Soils Used for Citrus Production in Florida. Gainesville, FL: EDIS, University of Florida, Cooperative Extension Service.
Obreza, T. T., and Morgan, K. T. (2008). Nutrition of Florida Citrus Trees. Gainesville, FL: EDIS, University of Florida, Cooperative Extension Service.
Okada, E., Costa, J. L., and Bedmar, F. (2019). Glyphosate Dissipation in Different Soils under No-Till and Conventional Tillage. Pedosphere 29 (6), 773–783. doi:10.1016/s1002-0160(17)60430-2
Piccolo, A., Celano, G., and Conte, P. (1996). Adsorption of Glyphosate by Humic Substances†. J. Agric. Food Chem. 44 (8), 2442–2446. doi:10.1021/jf950620x
Quinn, J. P., Peden, J. M. M., and Dick, R. E. (1988). Glyphosate Tolerance and Utilization by the Microflora of Soils Treated with the Herbicide. Appl. Microbiol. Biotechnol. 29 (5), 511–516. doi:10.1007/bf00269078
Salazar López, N. J., Silveira Gramont, M. I., Zuno Floriano, F. G., Rodríguez Olibarría, G., Hengel, M., and Aldana Madrid, M. L. (2016). Dissipation of Glyphosate from grapevine Soils in Sonora, Mexico. Terra Latinoamericana. 34 (4), 385–391.
Sheals, J., Sjöberg, S., and Persson, P. (2002). Adsorption of Glyphosate on Goethite: Molecular Characterization of Surface Complexes. Environ. Sci. Technol. 36 (14), 3090–3095. doi:10.1021/es010295w
Shushkova, T., Ermakova, I., and Leontievsky, A. (2010). Glyphosate Bioavailability in Soil. Biodegradation 21 (3), 403–410. doi:10.1007/s10532-009-9310-y
Sørensen, S. R., Schultz, A., Jacobsen, O. S., and Aamand, J. (2006). Sorption, Desorption and Mineralisation of the Herbicides Glyphosate and MCPA in Samples from Two Danish Soil and Subsurface Profiles. Environ. Pollut. 141 (1), 184–194. doi:10.1016/j.envpol.2005.07.023
Sprankle, P., Meggitt, W. F., and Penner, D. (1975). Adsorption, Mobility, and Microbial Degradation of Glyphosate in the Soil. Weed Sci. 23, 229–234. doi:10.1017/s0043174500052929
Tong, M., Gao, W., Jiao, W., Zhou, J., Li, Y., He, L., et al. (2017). Uptake, Translocation, Metabolism, and Distribution of Glyphosate in Nontarget Tea Plant (Camellia Sinensis L.). J. Agric. Food Chem. 65 (35), 7638–7646. doi:10.1021/acs.jafc.7b02474
Torstensson, L. (1985). “Behavior of Glyphosate in Soils and its Degradation,” in Herbicide Glyphosate. Editors E. Grossbard, and D. Atkinson.
University of Florida (2020). Florida Citrus Production Guide. Gainesville, FL: EDIS, University of Florida, Cooperative Extension Service.
USDA (2018). National Agricultural Statistics Service. 2017 Fruit Chemical Use. Washington, DC: U.S.
USEPA (1995). EPA Observational Economy Series, Vol 1: Composite Sampling. EPA 230-R-95-005. Washington, DC: U.S. Government Printing Office.
USEPA (2008). Fate, Transport and Transformation Test Guidelines (OPPTS 835.1230 Adsorption/Desorption Batch Equilibrium). Washington, DC: US Government Printing Office.
Vereecken, H. (2005). Mobility and Leaching of Glyphosate: a Review. Pest Manag. Sci. 61 (12), 1139–1151. doi:10.1002/ps.1122
Wang, Y. J., Zhou, D. M., and Sun, R. J. (2005). Effects of Phosphate on the Adsorption of Glyphosate on Three Different Types of Chinese Soils. J. Environ. Sci. (China) 17 (5), 711–715.
Keywords: adsorption, desorption, dissipation, half-life, leaching
Citation: Gairhe B, Liu W, Batuman O, Dittmar P, Kadyampakeni D and Kanissery R (2021) Environmental Fate and Behavior of the Herbicide Glyphosate in Sandy Soils of Florida Under Citrus Production. Front. Environ. Chem. 2:737391. doi: 10.3389/fenvc.2021.737391
Received: 06 July 2021; Accepted: 06 September 2021;
Published: 21 September 2021.
Edited by:
Wei Chen, China University of Geosciences, ChinaReviewed by:
Junwu Xiong, China University of Geosciences, ChinaShizong Wang, Tsinghua University, China
Pei Pei Qi, Zhejiang Academy of Agricultural Sciences, China
Copyright © 2021 Gairhe, Liu, Batuman, Dittmar, Kadyampakeni and Kanissery. This is an open-access article distributed under the terms of the Creative Commons Attribution License (CC BY). The use, distribution or reproduction in other forums is permitted, provided the original author(s) and the copyright owner(s) are credited and that the original publication in this journal is cited, in accordance with accepted academic practice. No use, distribution or reproduction is permitted which does not comply with these terms.
*Correspondence: Ramdas Kanissery, rkanissery@ufl.edu