- 1School of Culture, History and Language, ANU College of Asia and the Pacific, Australian National University, Canberra, ACT, Australia
- 2ARC Centre of Excellence for Australian Biodiversity and Heritage, Australian National University, Canberra, ACT, Australia
- 3Monash Indigenous Studies Centre, Monash University, Clayton, VIC, Australia
- 4ARC Centre of Excellence for Australian Biodiversity and Heritage, Monash University, Clayton, VIC, Australia
- 5GunaiKurnai Land and Waters Aboriginal Corporation, Kalimna West, VIC, Australia
- 6Radiocarbon Dating Laboratory, University of Waikato, Hamilton, New Zealand
- 7ARC Centre of Excellence for Australian Biodiversity and Heritage, James Cook University, Cairns, QLD, Australia
- 8Laboratoire EDYTEM, Université Savoie Mont Blanc, Le Bourget du Lac Cedex, France
- 9School of Geography, Earth and Atmospheric Sciences, The University of Melbourne, Parkville, VIC, Australia
In southeastern Australia, GunaiKurnai caves are known by current Aboriginal Elders and from nineteenth century ethnographic documents as special places used by mulla-mullung (“clever men” and “clever women”) for the practice of magic and medicine. Pollen analysis conducted on sediments from one such cave, Cloggs Cave, reveals an unusually well-preserved and well-stratified pollen sequence extending back >25,000 years, with much of the pollen introduced into the cave by people carrying flowering plants. High concentrations of pollen, rare for limestone cave settings, were recovered, including pollen clumps of individual taxa representing deposition of in situ flowering material. These taxa are dominated by plants known through GunaiKurnai knowledge and ethnography to have special cultural uses and that match the plants known to have been used by mulla-mullung, and some edible plants. These include taxa such as Banksia spp., Pimelea spp. (rice flower), and Plantago spp. (plantain) and the plant families of Asteraceae (daisy) and Poaceae (grass). The largely anthropogenic pollen assemblage also signals the presence of plants from cooler and drier climates dominated by more open vegetation during the Last Glacial Maximum than that observed around Cloggs Cave in recent times. The Early Holocene pollen then reflects a warmer and wetter climate that supported the expansion of woodland elements. Together, the pollen record of Cloggs Cave provides remarkable insights into two articulating histories: the cultural practices of the GunaiKurnai Old Ancestors in a special, secluded cave; and the environmental history of Country.
1 Introduction
How does pollen enter deep cave cavities so sheltered from the elements as to have barely felt the faintest whisper of wind? If not carried by the wind, what other processes may be involved in transporting pollen grains from living plants to cave sediments? Did water carry the tiny particles into a site? Or perhaps there were other means—people, cave-dwelling marsupials or insects, for instance—each selecting which plants were collected, transported, and deposited (e.g., Hunt and Fiacconi, 2018). Can pollen in cave sediments have been brought in on the fur or in the scats of flower-eating animals? And if the buried pollen came from people carrying flowers or flowering plants in ancient times, are such pollen records a type of archaeological assemblage open to archaeological interpretation? What can such pollen records tell us about how people used caves in the past, and what the environment was like outside?
With such questions in mind, we—a multidisciplinary team of GunaiKurnai Elders and knowledge-keepers and university-based archaeologists, palynologists, biogeographers, and geomorphologists—set out to interpret the pollen record of Cloggs Cave in GunaiKurnai Country (East Gippsland, SE Australia; Figure 1). Following initial archaeological excavations by Josephine Flood in 1971–1972 (Flood, 1973, 1980), new excavations were requested in 2017 by the GunaiKurnai Land and Waters Aboriginal Corporation, representing the site's Aboriginal Traditional Owners (Figure 2). The aims of the new research were to reveal fresh archaeological details of how the cave was used by the Old Ancestors,1 in light of cultural knowledge held by past and present GunaiKurnai Elders and recorded in nineteenth century ethnographic texts (e.g., Howitt, 1887), along with recent methodological developments. The GunaiKurnai Land and Waters Aboriginal Corporation was also interested in finding out about how the environment around the cave had changed through the millennia, both as a result of climate change and through the activities of the Old Ancestors, such as cultural burning. Many of the archaeological and geomorphological results of this new research have already been published (e.g., David et al., 2021a,b; Delannoy et al., 2020; McDowell et al., 2022; Mialanes et al., 2022; Mullett et al., 2021; Stephenson et al., 2020). The oldest definitive archaeological evidence of the Old Ancestors in the cave are flaked stone artifacts from excavation square R31 excavation unit (XU) 56, dated between 25,640 and 48,470 cal BP in mixed sediments disturbed by massive rockfall. The oldest definite stone artifacts in the well-stratified layers with good chronostratigraphic resolution come from XU45 in stratigraphic unit (SU) 4V. They date to sometime between 24,760–25,740 cal BP and 25,600–27,390 cal BP (these two age ranges are the uncertainty ranges of the start and end of the time envelope that contains the earliest definite artifacts; Mialanes et al., 2022; for the Bayesian modeling of the square R31 radiocarbon and nearby OSL ages, presented at 95.4% probability throughout this paper, see David et al., 2021a: Supplementary Table 3). Cultural activities in the excavated part of the cave continued largely unabated (at an archaeological timescale) until no later than c. 1,460 cal BP. A feature of the archaeological deposit inside the cave is a dense sequence of stratified, in situ burning events dating from c. 4,400 cal BP to 1,460 cal BP. Here superimposed ash layers had accumulated around a standing stone 28.1 cm high erected c. 2,000 cal BP, gradually burying it in the process (David et al., 2021b). Contemporaneous with the standing stone and deposited 30–40 cm away is a small, hand-sized portable grindstone that had been used for the preparation of Bogong moths (Agrotis infusa) and for the crushing and grinding of crystalline minerals (probably calcite, analyses in progress; David et al., 2021b; Stephenson et al., 2020). One hundred and fifty-eight broken soda-straws (thin stalactite growths) were found in the excavations, especially concentrated in the horizon with the standing stone. A well-formed quartz crystal and a small number of stone artifacts, mostly of quartz, were also found in the excavations (Mialanes et al., 2022). Eight meters from the excavations, on the floor of an alcove deep in the back of the cave, there is a stone arrangement; the alcove's floor is strewn with what also appears to be crushed calcite. Overhead in the alcove's entrance, stalactites had been broken in antiquity. Uranium–thorium (U–Th) dating of soda-straws growing over the stumps of the broken stalactites indicate that the stalactites were first broken by 23,230 ± 300 years ago, with the most recent age of 120 ± 30 years ago on soda-straw re-growths indicating that some of the stalactites could have been broken as recently as the nineteenth century CE (David et al., 2021b, p. 16; Delannoy et al., 2020, p. 19). While these U–Th ages on the proximal ends of the new soda-straws growing over the stumps of the original stalactites may approximate the time of breakage of the original stalactites, the U–Th ages should be read as minimum ages for the breaking of the stalactites, because what is dated is the start of the post-breakage regrowth, not the time of breakage itself. Elsewhere in the cave, in excavation square R31, two ritual installations incorporating well-preserved trimmed Casuarina sticks smeared with animal or human fat directly dated to c. 12,000 and 11,000 cal BP (David et al., 2024).
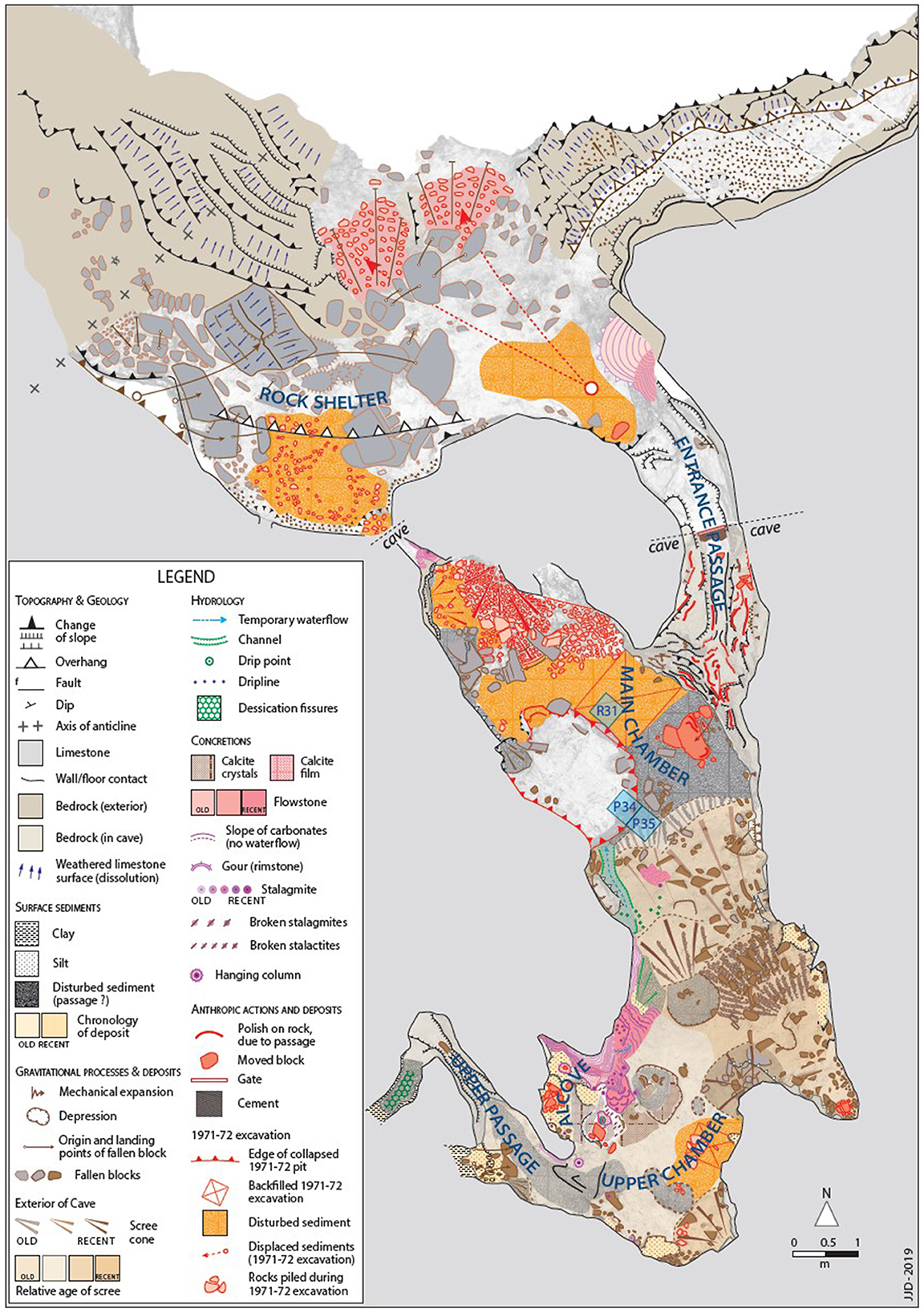
Figure 2. Geomorphological map of Cloggs Cave, GunaiKurnai Country. Squares A–TT were excavated by Flood (1980) in 1971–1972, those in green (P34, P35, and R31) by the GKLaWAC–David team in 2019–2020 (e.g., David et al., 2021b).
These archaeological findings align well with nineteenth century and current GunaiKurnai knowledge of caves. In GunaiKurnai culture, caves were the dens of nargun, treacherous and at times dangerous beings partly made of stone (Howitt, 1876, 1971). Caves were also used by learned individuals—GunaiKurnai doctors or “clever men” and “clever women,” in the twentieth century anthropological literature also called individuals of “high degree” who possessed heightened levels of knowledge over sacred matters, magic, healing and ritual practices (Elkin, 1945). GunaiKurnai call these special individuals mulla-mullung (David et al., 2021b; for both women and men as mulla-mullung holders of special powers, see e.g., Howitt, 1887, p. 34–35; Smyth, 1878, p. 474).
2 The Cloggs Cave pollen: the conundrum and the opportunity
Cloggs Cave is a well-protected cave set quite deep into a limestone ridge. Preservation conditions inside the cave are exceptional, so much so that plant material in the deposits, including leaves, date back to the Late Pleistocene (see also Flood, 1974, p. 180–181). There is also a remarkable abundance of pollen in the excavated sediments (see below). Yet the cave is a poor pollen trap. Today, the only entryway into the cavity is via a 5 m-long and curving, 1.55 m-tall, narrow 0.85 m-wide keyhole duct (the Entrance Passage) that restricts the flow of wind from the environment outside into the still air of the chambers within. In addition, since very little, indirect sunlight penetrates the interior, plant life does not grow and thus none of the pollen is local to the site. We are left with both a conundrum and an opportunity: how did the pollen enter Cloggs Cave? This question is key to interpreting what the pollen relates to: the general environment outside, or cultural activities within.
First, the conundrum: in the absence of significant direct inputs from the wind (the primary way pollen usually disperses across the landscape), what—or who—caused the pollen to enter the cave, and, in some horizons, in great abundance? Is this, in fact, an anthropogenic pollen record? Second, the opportunity: the excellent preservation conditions, the exquisitely intact and deep sediments, and a cornucopia of GunaiKurnai plant knowledge combine as an opportunity to investigate pollen sources not usually well-represented in palynology: the flowers or flowering plants selectively harvested from the local landscape and carried into the cave by people in the past.
This paper interrogates the composition and structure of the pollen from the sediments of two excavation squares in Cloggs Cave, P35 and R31. We ask three major questions of the pollen:
1. What was the environment outside Cloggs Cave like in the past?
2. How have abundant possum scats in the sediments affected the pollen in the sediments, and how can the pollen in those scats further reveal details of local palaeoenvironments?
3. Does pollen in the cave sediments reveal details of flowers or flowering plants carried into the cave by the Old Ancestors deep in the past?
3 Ways that pollen gets deposited in caves
Deposition of pollen in caves results from a complex interplay of factors. Those factors are different and much less understood than the depositional mechanisms governing pollen accumulation in other sedimentary environments, such as lakes, swamps and marine settings (Carrión et al., 2022; Hunt and Fiacconi, 2018; McGarry and Caseldine, 2004). The main distinguishing feature of cave pollen deposition is the variety of transport pathways responsible for the accumulation of pollen. Similar to open-air catchment basins, air-fall—wind blowing pollen into a cave—is an important contributor (Hunt and Fiacconi, 2018), yet how pollen accumulates will vary spatially depending on how enclosed or open the cave is, distance from the entrance, the configuration of internal walls and installations, and internal slope factors (e.g., Rowe et al., 2020). Pollen from plants growing in or near cave entrances may be over-represented in pollen assemblages, and streams and floods can also deposit pollen into cave sediments (Hunt and Fiacconi, 2018; McGarry and Caseldine, 2004). People using and/or discarding plants and flowering material in caves may be another important factor (Hunt and Fiacconi, 2018; Hunt et al., 2016) that would result in spatially variable pollen distributions indicative of activity areas. Animals (e.g., bees, birds, and bats) can also transport considerable quantities of pollen into caves, such as on their coats, plumage or via their scats (Basumatary and Tripathi, 2021; Hunt and Fiacconi, 2018; Hunt et al., 2016), especially among flower-eating fauna. In Australia, small marsupials such as rodents, bushrats, native mice, sugar gliders, possums and dasyurids have been recorded as pollination vectors (Bradshaw, 2014). From the original excavations at Cloggs Cave in 1971–1972, Hope (1973; Flood, 1974, p. 180) had concluded that most, and possibly all, of the faunal remains in the cave deposits had been introduced by owls and other natural animal deaths rather than through human occupation, emphasizing that food consumption by the Old Ancestors had not been a major activity. Similarly, the consumption of animal foods was not evident from the subsequent excavations undertaken in 2019–2020. McDowell et al.'s (2022) detailed analysis of the animal bones from square R31 concluded that many of the bones were deposited by Masked Owls (Tyto novaehollandiae) and/or Sooty Owls (Tyto tenebricosa) whose hunting range covered a number of habitats surrounding the cave. Masked Owls have the larger hunting range, spanning 2.5–2.8 km (McDowell et al., 2022, p. 1,640; Young et al., 2020).
Large numbers of possum scats were also observed in the sediments during the excavations, indicating that live possums repeatedly frequented the cave, living in small individual hollows inside the cave. As herbivores they could, therefore, have introduced pollen through their scats and from their coats (see below).
Cave sediments can also experience chemical diagenesis and complex reworking, erosion, redeposition and mixing of strata (Goldberg and Mandel, 2008). In contrast to the often relatively continuous accumulation of sediments in lakes and swamps (Bennett and Willis, 2001), sedimentation in caves is often episodic and can incorporate redeposited sediments from, and mix sediments through, for example, roof collapse (Hunt and Fiacconi, 2018; Rowe et al., 2020). The complex entrance, floor, wall and ceiling configuration of cave environments means that a number of geomorphic, animal, and human factors influence when, and what, pollen is introduced into caves, and where within a cavity pollen will be deposited. The implication is that the resultant pollen record may not necessarily be sequential or continuous (Rowe et al., 2020). Fortunately for the case of Cloggs Cave, the fine-scale and high-resolution chronostratigraphy and geomorphological reconstructions, discussed further below, enable an accurate and precise chronological and spatial resolution of its pollen sequences (David et al., 2021a,b; Delannoy et al., 2020).
3.1 Possum scats
One small animal species that repeatedly occupied Cloggs Cave and whose scats have been well-preserved in high numbers in the stratified deposits is the Common Brushtail Possum (Trichosurus vulpecula). Common Ringtail Possums (Pseudocheirus peregrinus) are also present in the region and are thus also discussed here, despite no definitive signs of their scats in the deposit.
3.1.1 Herbivory of the Common Brushtail Possum (Trichosurus vulpecula)
Common Brushtail Possums live a predominantly solitary lifestyle, making their dens in natural cavities like tree hollows and caves. Their home range averages 5.4 ha for males and 2.4 ha for females, meaning that the pollen in their scats and from their coats will preserve a signal of some of the vegetation within that range (McKay and Winter, 1989).
Common Brushtail Possums can survive almost anywhere in Australia, but prefer dry Eucalyptus spp. forests and woodlands. This species of possum is a quintessential example of a generalist herbivore, feeding on a wide range of plants (Fitzgerald, 1984; Kerle, 1984). With a head-and-body length of 35–55 cm, a tail length of 25–40 cm and body mass of 1.2–4.5 kg, they are larger than Common Ringtail Possums.
Common Brushtail Possum scats are quite variable in size but are typically 3 cm long, 1.0–1.2 cm wide and cylindrical with a distinctive nipple-like pinch-point at one end. Their scats are found in clumps, strings and singles. The possum scats analyzed in this paper have these characteristics and are from this species. Unlike the Common Ringtail Possum, the Common Brushtail Possum's liver cannot metabolize the abundant toxins in Eucalyptus spp. leaves. Consequently, they need to consume a more varied diet of leaves, flowers and fruits, supplemented with bird eggs, baby birds, and some insects. They can determine the nutrient quality of Eucalyptus spp. leaves and only eat those with the highest nutrient contents (Marsh et al., 2003). They also consume some shrubs (mainly Acacia spp.) and herbs. Seasonal flowers and fruits are very important food items and can comprise up to half their diet when in season. They are the likely source of the pollen in their scats.
3.1.2 Herbivory of the Common Ringtail Possum (Pseudocheirus peregrinus)
The Common Ringtail Possum can be found in tall open and closed forests, woodlands, rainforests, and eucalypt forests. Common Ringtail Possums construct dreys, spherical nests made of sticks and leaves. They too use tree hollows, but rarely nest in caves (Lindenmayer et al., 2008). Weighing up to 1 kg, they are smaller than the Common Brushtail Possum, with a head-and-body length of 30–35 cm and a prehensile tail of about the same length.
The Common Ringtail Possum predominately eats Eucalyptus spp. leaves, but they also eat fruits, flowers and leaves of other native trees. Common Ringtail Possum scats are typically 2 cm long and 0.5 cm wide and cylindrical with rounded ends and a granular surface. While the intact possum scats in Cloggs Cave have the characteristics of scats from the Common Brushtail Possum, it is possible that some of the more fragmented scats (not analyzed in this paper) whose characteristic features have disintegrated came from Common Ringtail Possums. Elsewhere, each Common Ringtail Possum pellet consists of 61–98% Eucalyptus spp. leaves. The remainder consist of leaves from several species of shrub and flowers, and flower buds of Eucalyptus spp. (Pahl, 1987).
4 Cloggs Cave
Cloggs Cave is a limestone karst cave whose entrance is perched 72.3 m above sea level (a.s.l.), in the southern foothills of Victoria's Great Dividing Range, SE Australia. It abuts the southern floodplain of the Buchan River, between the township of Buchan and the Buchan River's confluence with Murrindal Creek, c. 4 km northwest of where the Buchan River joins the Snowy River (Delannoy et al., 2020). Today the cave is largely surrounded by farmland. Pre-European settlement vegetation along the Buchan River valley bottom, from the township of Buchan east to the Snowy River, consisted of Riparian Scrubs or Swampy Scrubs and Woodlands with moist soils, tall eucalypts (e.g., Eucalyptus globoidea, E. viminalis), wattles (e.g., Acacia dealbata, A. melanoxylon), ferns (e.g., Calochlaena dubia, Cyathea australis), and grasses (e.g., Carex breviculmis, Microlaena stipoides var. stipoides) [Ecological Vegetation Class (EVC) 18 of the Victorian government's bioregion categories]; and Dry Forest with tall open forest (e.g., Eucalyptus globoidea, E. viminalis), acacias (e.g., Acacia melanoxylon, A. mearnsii), rich in lilies, grasses and sedges (e.g., Themeda triandra, Poa australis, Carex spp.), and many native plant foods including apple berry (Billardiera scandens) and pale-fruit ballart (Exocarpos strictus; EVC 47). On the valley sides and crests surrounding Cloggs Cave, the vegetation was a typical Lower Slopes or Hills Woodlands (sub-group: Grassy), an open woodland with tall eucalypts (e.g., Eucalyptus melliodora, E. viminalis) and a diverse ground layer of herbs (e.g., Hypericum gramineum) and grasses (e.g., Poa labillardierei), including an important native cereal, kangaroo grass (Themeda triandra), still growing abundantly on the valley sides (EVC 175; Department of Energy, Environment and Climate Action, 2023; see also Victoria State Government Department of Sustainability Environment, 2004).
The entrance to Cloggs Cave is found halfway down a vertical escarpment overlooking the Buchan River floodplain 17 m below (Delannoy et al., 2020, Figure 2). The base of the Middle Devonian Buchan Group limestone formation extends to the Buchan River's lower terrace and abuts a convex arc of an ancient meander of the Buchan River. The location of Cloggs Cave against this palaeo-meander indicates that the larger cave network must once have extended further toward the valley. The cliff-face has retreated in response to the river's gradual undercutting, which removed the cliff's (and the cave's) outer (northern) palaeo-walls (Delannoy et al., 2020).
Today Cloggs Cave is entered through an 8 m-high, vertical open cavity (the “Porch”) that gives way to the Entrance Passage (0.85 m-wide × 1.55 m-high; Figure 2). Beneath the Porch is a talus slope consisting of a mixture of soft sediments and rocks of varied sizes. The talus slope extends to the alluvial terrace below. Immediately to the west of the Porch is the modestly sized Rockshelter (2.9 m-high × 3.2 m-wide × 2.5 m-deep).
The presence of ancient stalagmitic formations in the Porch confirms that Cloggs Cave was once part of a larger cave network that extended into the now-open Buchan Valley. At the cave-end of the Entrance Passage, inside the cave, a 1.2 m-deep drop onto a soft sediment floor marks the opening of the Main Chamber (5 m-long × 3.5 m-wide × 5 m-high), which itself intersects a Lower and Upper Gallery. The Upper Gallery then leads to the Upper Chamber onto the Upper Passage (0.6 m high). The cave terminates 18 m from the front of the Porch (Delannoy et al., 2020; Figure 2).
The pollen analyses reported here come from sediment samples collected during the 2019–2020 archaeological excavations. These archaeological investigations focused on three areas positioned against the still-open footprint of Flood's 2 × 2 m excavation pit of 1971–1972, in the Main Chamber. Square P35, 50 × 50 cm in size, was positioned against the southeast wall of Flood's excavation pit to target the layers of interleaved ashy remains of old fires exposed in the upper section of the pit and to investigate the older deposits beneath. Square P34, also a 50 × 50 cm excavation square, was set against P35 and began at the level of its base to find out what lay in the deeper levels exposed by the original 1971–1972 pit (Figures 2, 3). Square R31, of the same size, was positioned against the northeast wall of the open pit, to investigate its long and finely stratified cultural sequence and to resolve long-standing chronological questions about the use of the cave by the Old Ancestors and now-extinct megafauna before the Last Glacial Maximum (LGM; for results, see David et al., 2021a).
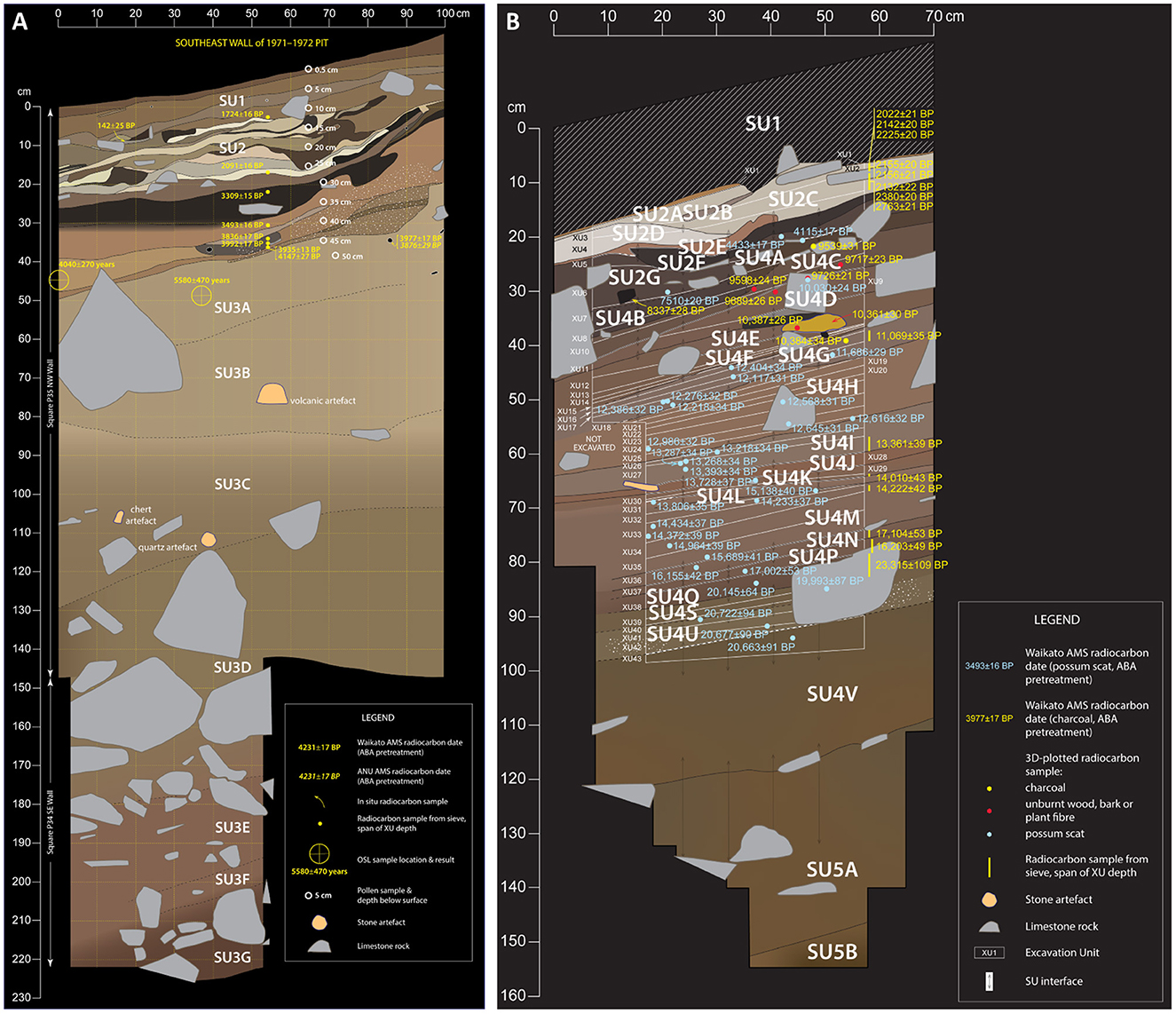
Figure 3. Cloggs Cave stratigraphic profiles showing the location of pollen sediment samples and their chronostratigraphic contexts. (A) Squares P34–P35. (B) Square R31, with XUs deeper than XU43 not shown as they were not analyzed for pollen due to sediment mixing, as described in-text.
Our palynological study focuses on pollen samples collected from the upper levels of P35 and most of the sequence of R31. The lower levels of P35 and all of P34 are mixed sediments that rapidly collapsed into a localized sinkhole that formed c. 6,000 cal BP during a phase of heightened rainfall (Delannoy et al., 2020). This lower part of P35 and P34 were thus not analyzed for pollen and do not feature in this paper. The upper P35 samples were taken directly from the cleaned southeast wall of Flood's partly collapsed 1971–1972 pit. They were collected by pushing 1.5 cm-diameter phials into the wall at 5 cm depth intervals (with the depth measurements taken at the mid-point of each phial), along a vertical sequence from the present surface down to 50 cm depth. The pollen samples came from 10 to 18 cm southwest of the northwestern edge of square P35; their collection location is contiguous with the P35 chronostratigraphy (Figure 3).
The R31 pollen samples were collected as unsieved bulk sediment samples from each excavation unit (XU) between XU1 (at the top) and XU43 at the SU4U–SU4V interface. The XUs (and thus bulk sediment samples) averaged 2.3 cm thick and followed the stratigraphy. The deeper and older sediments of XU44–XU49 (SU4V) have minor age reversals caused by small amounts of rockfall; those of XU50–XU56 (SU4V–SU5A interface and SU5A) have major age reversals due to massive rockfall. The XU44–XU56 sediments were thus not analyzed for pollen. The rockfall came from the ceiling and did not include the roof of the cave, which remains sealed overhead.
The pHs of the samples are all in the neutral to slightly alkaline range between 7 and 8, which is conducive to good pollen preservation (Bryant and Holloway, 1983).
4.1 Chronostratigraphy
4.1.1 Square P35
The thin XUs of square P35 and its offset P34 yielded a 227.7 cm-deep stratigraphic profile consisting of three main layers (stratigraphic units, “SUs”). P35 represents the upper 125.7 cm of this profile, the adjacent P34 the underlying part (Figures 2, 3A).
The lowermost 170 cm of SU3 (encompassing SU3A to SU3G) are in the infilled sinkhole that formed in the floor of the cave c. 6,000 cal BP. Soon after its formation, the sinkhole rapidly infilled with soft sediments and rocks that cascaded in from immediately upslope (David et al., 2021a; Delannoy et al., 2020). The upper levels of the largely infilled sinkhole subsequently formed a shallow depression, which began to fill more slowly between c. 6,000–4,400 cal BP (SU3A).
Overlying SU3 is SU2, a c. 40 cm-thick band of 76 finely stratified, mostly ash-rich layers and lenses from numerous fires lit by the Old Ancestors across this part of the cave (the ashy layers encompass SU2A to SU2BU; see David et al., 2021b). This dense sequence of ash layers accumulated between c. 4,400 cal BP and 1,600 cal BP, particularly in the last 400 years of that period (David et al., 2021b, p. 7). The topmost unit, SU1, comprises three thin sandy loam layers and lenses (SU1A–SU1C; c. 10 cm in total thickness) that formed in recent times [bottle glass fragments and spent matchsticks dating to the late nineteenth or twentieth century were the only cultural items found in SU1 and its mixed interface (at c. 10 cm depth) with SU2].
From bottom to top, the 11 pollen samples span the upper part of SU3A, the depression that slowly filled between c. 6,000 and 4,400 cal BP (as described above); the entirety of the culturally rich SU2 ash layers dating from c. 4,400 to 1,600 cal BP; and SU1 and its interface with the upper few centimeters of SU2 (deposited in the past c. 150 years).
4.1.2 Square R31
Square R31 was excavated to 153 cm depth (Figures 2, 3B). However, the apparently culturally sterile upper 20 cm (SU1) had been removed by Flood in 1971–1972. Flood then protected the underlying sediments by laying thick plastic sheeting overlain with imported soft sediments. Upon removal of those overlying sediments and plastic sheeting in 2020, the underlying sediments were found to have remained intact. This part of the Main Chamber was unaffected by the localized sinkhole that had destroyed the pre-6,000 cal BP stratigraphy in P34 and P35. In contrast, R31 possesses a finely stratified sequence with excellent chronostratigraphic integrity down to XU43, as described above (Figure 3B). Sixty-nine radiocarbon ages on single pieces of charcoal, wood, bark and possum scats signal a long and very well-dated sequence for this square. Optically stimulated luminescence (OSL) ages for the deeper and older sediments 1.4 m to the southeast, where unexcavated SU5 deposits were not affected by roof-fall, are in excellent agreement with the calibrated radiocarbon age sequence (David et al., 2021a).
Sediments in the lowermost stratigraphic unit, SU5, contain the bones and teeth of extinct megafauna. The calibrated radiocarbon and OSL ages from the 2019–2020 excavations indicate that SU5 was actively accumulating until sometime between 43,230 and 46,580 cal BP (David et al., 2021a, p. 10); underlying undated deposits signal the presence of even older unexcavated sediments. Overlying SU5 is SU4, a unit containing 22 distinct layers each averaging 1–2 cm in thickness. Most of SU4 has excellent chronostratigraphic resolution, but rockfall from the ceiling resulted in some mixing of sediments and corresponding reversals in radiocarbon ages in SU4V, the lower sub-layer of SU4 that lies immediately above SU5 (David et al., 2021a). These age reversals occur in XU44–XU50. As noted above, for this reason the pollen analysis was only undertaken down to XU43 at the SU4U–SU4V interface (dated to 24,760–25,740 cal BP).
Some of the upper SU4 sub-layers are small ashy lenses from fireplaces built by the Old Ancestors as far back as the Pleistocene–Holocene boundary. Above SU4, SU2 is a c. 15 cm-thick deposit consisting of seven ash lenses from anthropic fires (SU3, the infilled sinkhole, is entirely absent in R31) beginning sometime between 4,860 and 7,290 cal BP (uncertainty range) and ending between 1,460 and 2,020 cal BP (uncertainty range). Most of SU2′s burnt micro-layers date between 1,460 and 3,360 cal BP (SU2A–SU2D; David et al., 2021a: Supplementary Table 3).
Forty-three pollen samples from R31 constitute the pollen sequence analyzed in this paper. Together they represent a long and continuous pollen record beginning at a time of extreme environmental conditions [the LGM, defined globally as spanning 26,500–19,000 cal BP (Clark et al., 2009) and locally as spanning 28,000 ± 2,800 cal BP to 17,700 ± 2,200 cal BP (Cadd et al., 2021)] and continuing to the Late Holocene. But it is the rarity of this record—(1) the insulated cave environment inhibiting the deposition of wind-blown pollen; (2) a lack of plant life and thus of locally produced and deposited pollen within the cave; (3) archaeological evidence of ritual items indicating the special use of the cave; (4) specialized GunaiKurnai botanical knowledge and practices; combined with (5) the movement into the cave of flower-eating, cave-dwelling fauna—that makes Cloggs Cave's pollen record particularly important for understanding the story of the site and its surrounding landscape.
5 The Cloggs Cave pollen: methods
Bulk sediment samples for pollen analysis were collected by the excavation team in 2019 (P35: n = 11) and 2020 (R31: n = 43) and analyzed at the palaeoecology laboratories in the Department of Archaeology and Natural History, Australian National University. To assess potential taphonomic pathways of pollen into the cave (e.g., cave-dwelling marsupials), Common Brushtail Possum (Trichosurus vulpecula) scats excavated from P35 (n = 4) and R31 (n = 18) were also analyzed. In R31, the individual, well-preserved possum scats came from the same XUs as the bulk sediment samples analyzed for pollen.
Cave environments around the world typically report low to variable recovery rates of pollen (Hunt and Fiacconi, 2018). To enhance recovery, we processed 6 cm3 from each sediment sample, double that of typical sediment volumes used in depositional settings such as lakes and swamps. Chemical preparation followed standard techniques to disperse the sediment and remove humic acids, calcium carbonates, bulk organics, cellulose and silicates, as well as to render pollen wall ornamentation more visible (Na4P2O7, KOH, HCl acetolysis, heavy liquid; see Bennett and Willis, 2001). A Lycopodium clavatum exotic spike was added to each sample determine relative pollen and micro-charcoal concentrations (9,666 spores per sample). In some samples presenting as barren (R31: XU1–XU7), an additional treatment with bleach (NaClO) was used to discolor the humic and carbonized organic fragments that may have obscured pollen visibility. All samples were mounted onto glass slides using glycerol and were analyzed at 400× magnification using a Zeiss Axio Imager.Z2m microscope. Taxonomic identifications were made using reference pollen taxa held in the Australasian Pollen and Spore Atlas (APSA) at the Australian National University (Haberle et al., 2021). Where possible, a minimum target of 300 pollen and spore grains were counted per sample.
Microscopic analysis revealed unusually high numbers of “clumps,” or aggregates, of single-taxon pollen. To obtain taxonomic and frequency data from the clumps, an additional analysis, separate to the pollen counts, was undertaken by scanning entire pollen slides to count the clumped pollen. The clumps were identified by taxa where possible and plotted as concentrations per 1 cm3 of sediment sample volume using the Lycopodium values.
We used C2 software (Juggins, 2007) to generate pollen diagrams and to perform a Principal Component Analysis (PCA) to explore taxonomic relationships between samples. Only taxa with frequency values of ≥2% of the total pollen and spore sum in at least one sample were included in the PCA analysis. The statistical distribution of samples in the resulting PCA bi-plot were then correlated with their Bayesian-modeled (calibrated) radiocarbon ages to distinguish chronological zones.
6 Results
Overall, the sedimentary pollen from P35 and R31 is well-preserved, although 11 samples were barren or had low pollen contents (P35: n = 4; R31: n = 7). These 11 samples are all from the ash layers of SU2 (Figure 4).
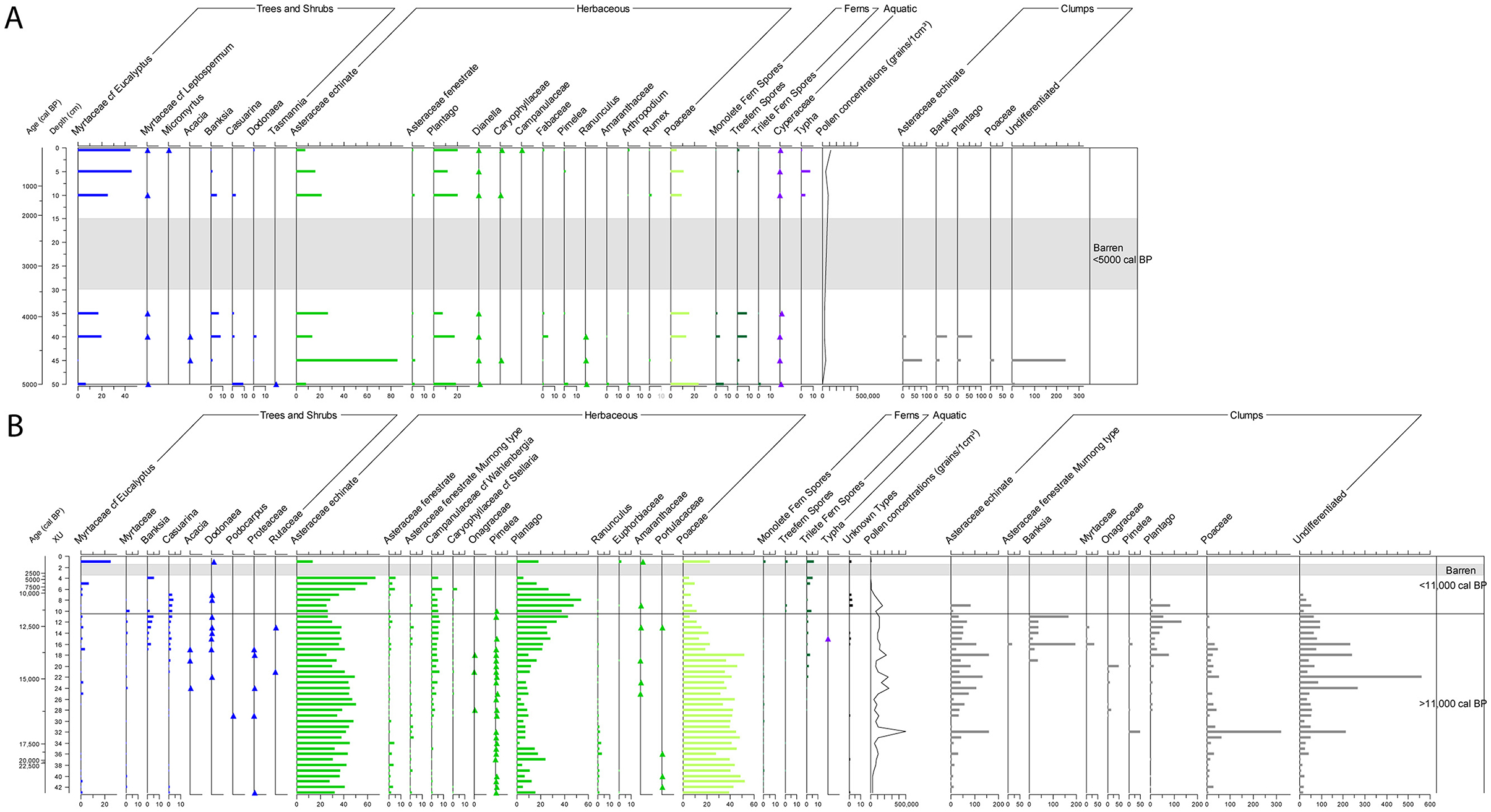
Figure 4. Percentage sediment-pollen diagrams for the two excavation squares. (A) Square P35. (B) Square R31. In both pollen diagrams, the dominant taxa (contributing ≥2% of the total pollen and spore sum in at least one sample) are shown. ▴ = presence of culturally important taxa contributing <2% of total pollen and spore sum. Clumped pollen was counted separately to the normal pollen counts. They are plotted at the end of the diagrams as concentrations per 1 cm3 sediment volume.
In general, there is a cohesiveness to the pollen signatures of P35 and R31, as demonstrated by the consistency of their dominant taxa. Across the two excavation squares, 71 pollen and four spore types were identified, although most are present only in trace amounts (<2% of the total pollen sum in any sample). Dominant taxa (≥2% of the total pollen and spore sum in any one sample) include 27 types representing 20 plant families, with a clear predominance of Asteraceae (daisies; 9–88% of total pollen in any sample) and Poaceae (grasses; 2–53% in any sample) (Figures 4, 5). There are nevertheless major differences in the pollen composition of the two assemblages, despite the two squares being located only 1.65 m apart (see also Supplementary material 1).
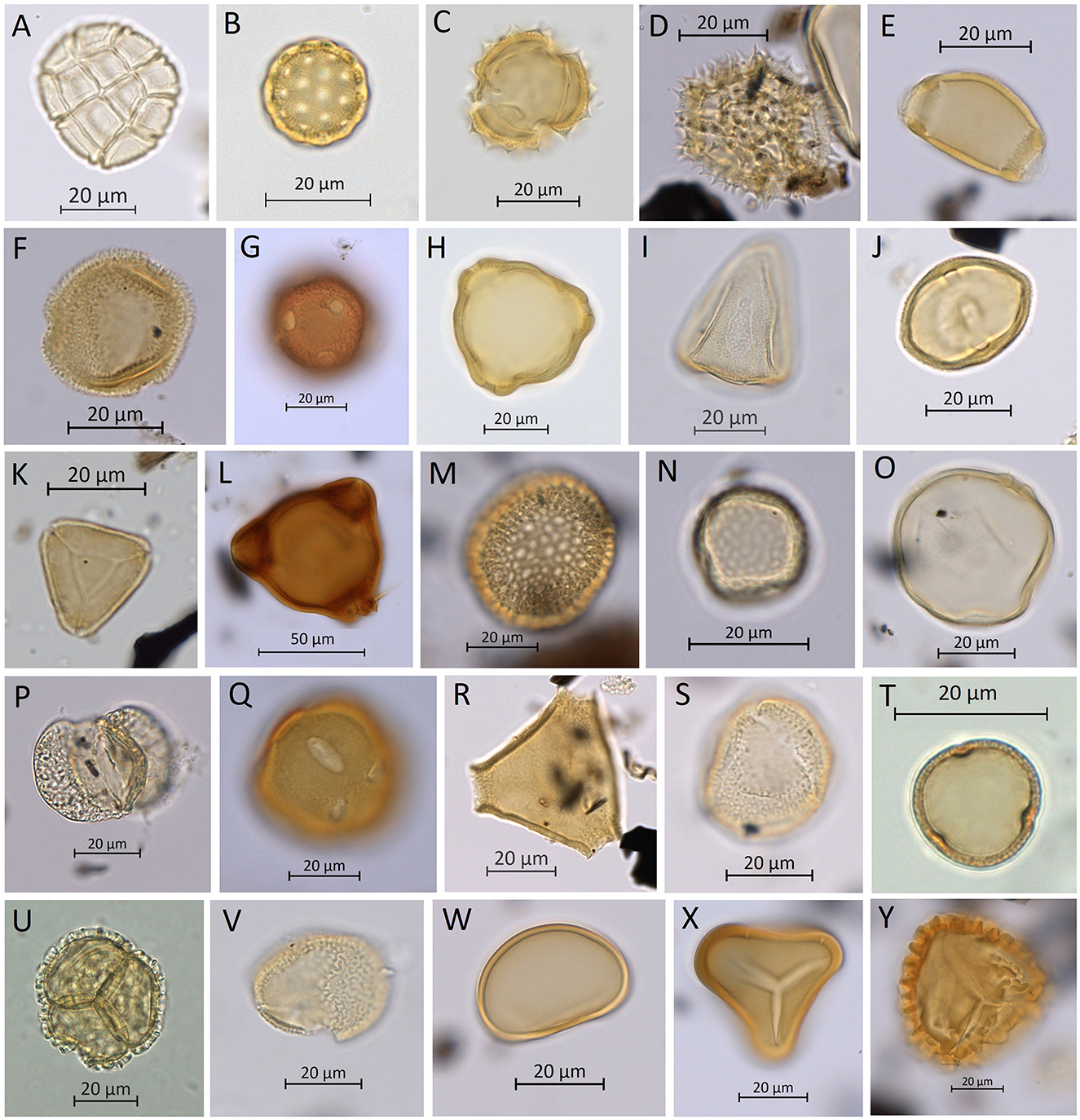
Figure 5. Commonly occurring pollen and spore types present in the Cloggs Cave samples. (A) Acacia spp. (wattle). (B) Amaranthaceae (saltbush). (C) Asteraceae—echinate-type (daisy). (D) Asteraceae—fenestrate-type (Microseris spp.—murnong-type, yam daisy). (E) Banksia spp. (F) Campanulaceae cf. Wahlenbergia spp. (bluebell). (G) Caryophyllaceae cf. Stellaria spp. (chickweed). (H) Casuarina spp. (she-oak). (I) Cyperaceae (sedge). (J) Dodonaea spp. (hopbush). (K) Myrtaceae cf. Eucalyptus spp. (gum tree). (L) Onagraceae (evening primrose). (M) Pimelea spp. (rice flowers). (N) Plantago spp. (plantain). (O) Poaceae (grass). (P) Podocarpus spp. (plum pine). (Q) Portulacaceae (purslane). (R) Proteaceae. (S) Ranunculus spp. (buttercup). (T) Rumex spp. (sorrel/dock). (U) Tasmannia spp. (mountain pepper). (V) Typha spp. (bulrush/cattail/reed). (W) Monolete fern spores. (X) Tree-fern spores. (Y) Trilete fern spores (micrographs are imaged from the Cloggs Cave samples).
6.1 The P35 pollen sequence
The 11 pollen samples from P35 are from sediments that accumulated over the past 5,000 cal BP. The top two samples (0.5 and 5 cm depth) are both in SU1 and date to ≤ 1,600 cal BP. They are the only samples from SU1 (SU1 being absent from the R31 sequence). The seven samples (10–40 cm depth) below this are associated with the stratified ash layers of SU2. Four of the SU2 samples (15, 20, 25, and 30 cm depth) are barren (Figure 4A). The two samples further below (35 and 40 cm depth) are in the lower part of SU2 and pre-date the dense sequence of burnt micro-layers (Figure 3). The two lowermost samples (45 and 50 cm depth) are from the upper part of SU3A.
Based on the geomorphology and chronostratigraphy, the samples from 45 and 50 cm depth came from the mixed infilled depression that is the top of SU3A, and thus likely include sediment redeposited from upslope (David et al., 2021a; Delannoy et al., 2020). Palynologically, this is particularly evident in the 45 cm-depth sample. With a dominance of Asteraceae (daisy) and lack of woody elements, its pollen content is different to that of the other samples in the P35 assemblage (Figure 4A). The 50 cm (Figure 6A) sample also derives from the top of SU3A, but the coherence of its pollen composition to other samples further up in the P35 pollen sequence suggests less incorporation of redeposited sediments (Figure 4A).
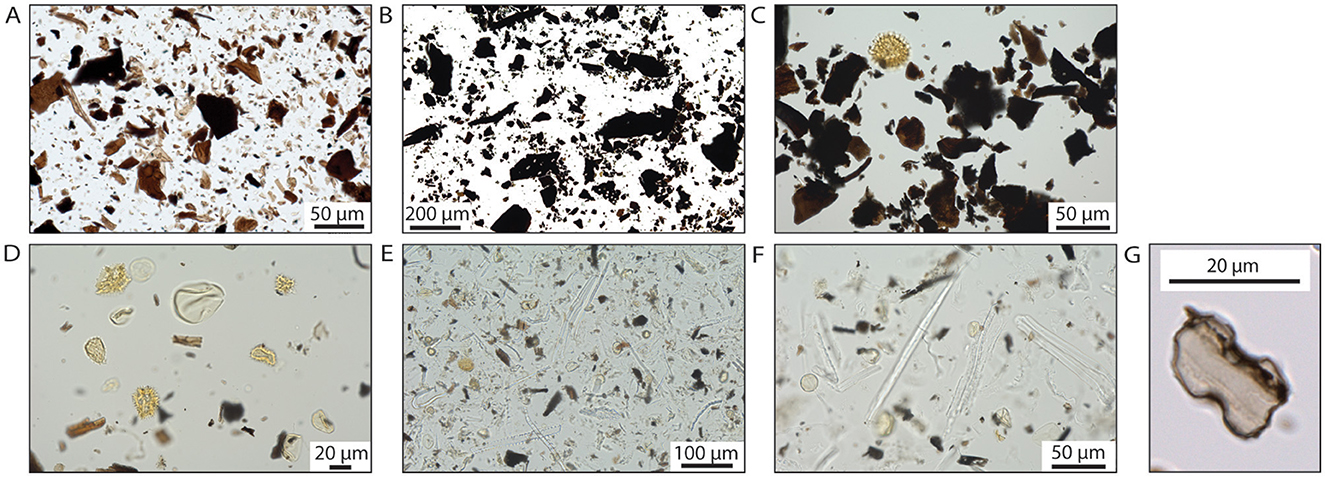
Figure 6. Differences in sediment composition within the P35 and R31 pollen assemblages. (A) P35 samples (depicted here is 50 cm depth) containing organic matter and charcoal on the pollen slides. (B, C) Uppermost samples of R31 (XU1–XU7; depicted here is XU3) have very low pollen contents and an abundance of fine charcoal and humified organic fragments. The exotic pollen marker Lycopodium can be seen in C, but few other pollen grains are present in these XUs. (D) At lower depths of R31 (XU8–XU43), the pollen samples exhibit high concentrations of pollen and relatively good pollen preservation. Here we show a typical pollen micrograph from XU13 with its abundant pollen. (E, F) Some samples in the lower levels of R31 (micrographs depicted here are from XU22) show high abundances of silica bodies including phytoliths and sponge spicules, even following treatment with HF (which removes silica). (G) Bilobate short cell phytolith from panicoid grasses, blackened from fire, from XU23.
The 35 cm and 40 cm samples are dominated by woodland elements (Myrtaceae, Banksia spp., Casuarina spp.) and herbaceous plants (mainly Asteraceae, Plantago spp. and Poaceae; Figure 4A). While the 15–30 cm samples are barren, the three uppermost samples (0, 5, and 10 cm depth) show a similar pollen composition to the 35 and 40 cm samples, namely the dominance of the Myrtaceae family as well as herbaceous elements (Asteraceae, Plantago spp. and Poaceae; Figure 4A).
6.2 The R31 pollen sequence
Only 10 of the 43 samples from R31 are younger than 11,000 cal BP. Of these, seven are barren or otherwise exhibit low pollen contents (XU1–XU7; Figures 4B, 6C). These uppermost R31 samples are mainly from SU2 (XU1–XU6), the ash deposits, and thus have very low pollen content, being dominated by burnt particles (“charcoal”) and humified organic fragments (Figures 4B, 6B, C). XU1–XU4 (SU2A to the SU2F–SU2G interface) date to <5,000 cal BP, and thus overlap in time with the P35 samples collected from 0.5 to 50 cm depth (SU1–top of SU3A). From XU5 down, the R31 pollen record relates to a period older than that covered by the P35 pollen sequence. Most of these XUs contain high concentrations of well-preserved pollen (Figures 4B, 6D). In addition to pollen, sponge spicules and phytoliths (silica bodies formed in plant cells, largely among the herbaceous taxa) were also observed in large abundance on the pollen slides in some of the lower levels (e.g., XU22–XU23; Figures 6E, F), including grass phytoliths blackened by fire (Figure 6G).
Most of the pollen assemblage from R31 is older than 11,000 cal BP (XU8–XU43, spanning the SU4B–SU4C interface down to the SU4U–SU4V interface; see Figure 3). It is largely composed of herbaceous taxa (Asteraceae and Poaceae pollen), with very little input from woody taxa until after c. 13,000–14,000 cal BP (XU15–XU17, from the SU4F–SU4G interface and SU4G; Figure 4B). After 12,000 cal BP (XU14, at the base of SU4F), woody elements such as Eucalyptus spp., Casuarina spp. and Banksia spp. increase, with the uppermost XU1 sample overlapping in composition with the upper samples of P35 (Figure 4B). Leading up to and after 11,000 cal BP (XU11), there is a shift from Poaceae (grass) to Plantago spp. (plantain) pollen, reverting back to a dominance of grass after 7,000 cal BP (XU5; Figure 4B). Ferns are found throughout the assemblage, with tree-ferns and ground-ferns generally increasing in abundance through time.
6.3 Principal Component Analysis
A Principal Component Analysis (PCA) was performed on the P35 and R31 pollen results to explore chronostratigraphic patterns in the pollen taxa. The PCA results show clear differences in the pollen composition of sets of samples from distinct chronostratigraphic horizons (Figure 7). PCA Axis 1 reflects woodiness, while PCA Axis 2 is driven by grass and other herbaceous taxa.
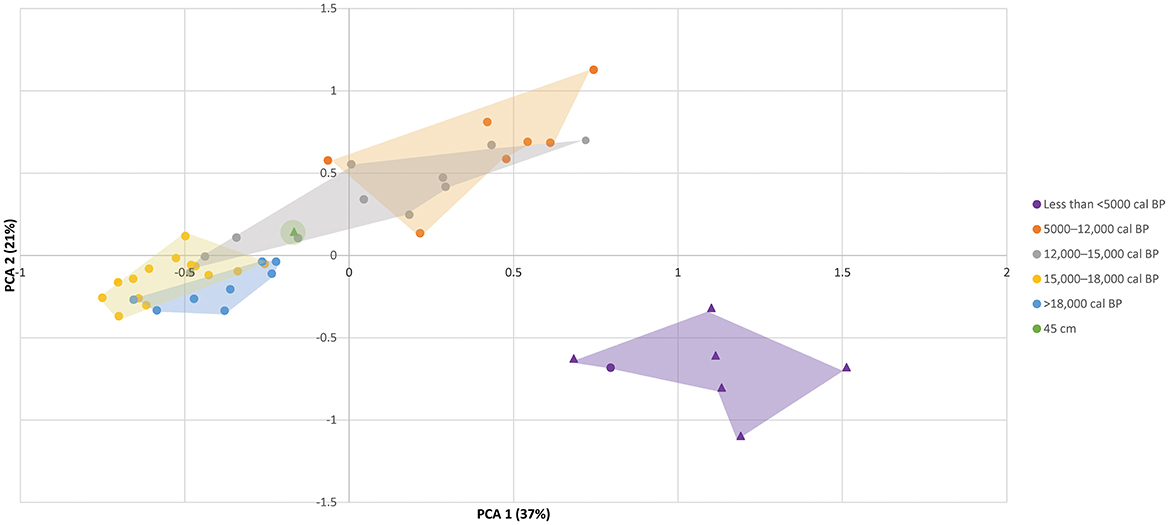
Figure 7. PCA bi-plot of the P35 and R31 sedimentary pollen, showing clusters which relate to chronostratigraphic zones in the pollen record. A total of 58% variation in the samples is explained by PCA Axis 1 (37%) and PCA Axis 2 (21%). The P35 samples are depicted as triangles, those from R31 as circles. The different colors of the samples and clusters indicate the temporal windows defined in the legend.
The samples from P35 are statistically different from most of those from R31. The major difference is that the P35 samples are mainly from woody taxa and ferns. From R31, only the XU1 sample, dated to c. 2,000 cal BP, sits within the P35 cluster (Figure 7). All of these samples date to within the past 5,000 years. In contrast, the R31 samples with pollen (other than XU1) all cluster together but signal a progressive shift from a grass-dominated assemblage to increasing woody contributions over time. The P35 sample taken from 45 cm depth plots within the R31 samples, probably indicating that it contains intermixed sediments given that it came from the upper part of the SU3A infilled sinkhole depression.
6.4 Clumped pollen
Both the P35 and R31 sediment samples contain large quantities of pollen that had not undergone dehiscence—the critical moment in the dispersal of pollen when individual pollen grains separate from their parent plant and are then caught up by the wind and spread across the landscape. Instead, pollen grains from individual taxa were still aggregated (clumped), indicating that they were never released and transported by wind. This indicates that the pollen clumps were almost certainly introduced into Cloggs Cave while still attached to their parent plants or flowers.
Clumps of pollen were recorded separately and are plotted at the end of the pollen diagrams, standardized to concentrations per 1 cm3 sediment volume using Lycopodium values (Figure 4). All taxa represented by the clumps—Asteraceae, Banksia spp., Plantago spp., Pimelea spp., Poaceae, Onagraceae, and Myrtaceae (Figures 8A–E)—have important Aboriginal cultural uses as recorded in ethnographic sources from SE Australia and as known by current GunaiKurnai Elders (see below and Supplementary material 2). Clumps of single-taxon pollen in the sediment samples are found throughout the P35 and R31 sequences, with changes in frequency and taxa generally also reflecting the counts of individual (non-clumped) pollen grains through time (Figure 4). For example, while clumps of Asteraceae are identified throughout the R31 sequence (XU9–XU42), clumps of Poaceae are frequent in lower depths (XU17–XU43), giving way in the upper depths (XU11–XU19) to clumps of Plantago spp. and Banksia spp., both taxa also becoming more prevalent in the single pollen grain counts at this time (Figure 4). This shift reflects a change from a more open to woody landscape over time, but the continued incidence of single-taxon pollen clumps across these periods of environmental change indicates the continued deposition of pollen in Cloggs Cave through transport mechanisms other than wind.
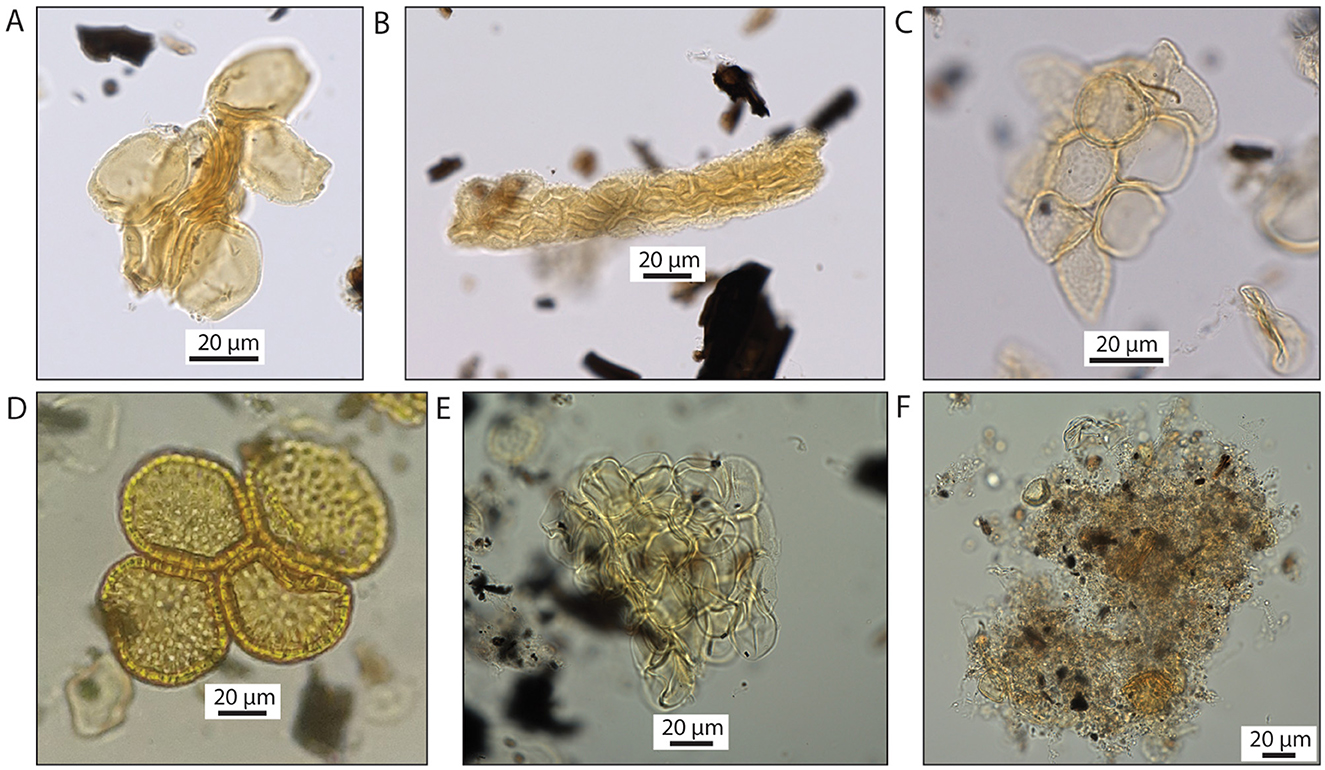
Figure 8. Examples of pollen clumps at Cloggs Cave. (A–E) Pollen clumps containing a single type of pollen, observed only in the sediment (not in the possum scat) samples. The taxa observed in clumps have all been recorded to be culturally important plants used by Aboriginal peoples in SE Australia, including GunaiKurnai (see Supplementary material 2). (A) Banskia spp. (B) Asteraceae (echinate-type). (C) Plantago spp. (D) Pimelea spp. (E) Poaceae. (F) Example of clumps observed in the possum scats. In contrast to (A–E), pollen clumps in the possum scats show dark, amorphous, and heterogenous micro-masses containing discrete grains of pollen of different taxa as well as charcoal, organic matter and other impurities. This composition indicates fecal material.
Single-taxon clumps of pollen were not detected in the possum scat samples. Any pollen bundles in these samples are a mixture of pollen from multiple taxa as well as organic matter and impurities within an amorphous, humified, heterogenous organic matrix of fecal material (Figure 8F; see below). The single-taxon pollen clumps detected in the sediments are thus interpreted not to have originated from disintegrated possum scats, although possums as vectors in others cannot be completely ruled out.
6.5 Possum scats
The possum scats from P35 and R31 are dominated by pollen of Asteraceae, Poaceae, Myrtaceae, Plantago spp., Casuarina spp., and fern spores (Figure 9). This suite of taxa broadly reflects the dominant taxa in the sedimentary pollen, and thus the vegetation composition and landscape surrounding the cave. The possum scats signal a relatively open landscape between c. 22,000 and 10,000 cal BP, with the pollen dominated by Poaceae and Asteraceae (R31; Figure 9B). Increasing woodiness of the landscape occurred through time, beginning 15,000 cal BP (XU24 in R31) and especially after 11,000 cal BP (XU10 onwards in R31 and in all the P35 scat samples), as evident by an increase in Myrtaceae, Casuarina spp., Fabaceae and other woody taxa (Figure 9). Plants with known cultural uses that are present in the sediments are absent or negligible in the scat samples (compare Figures 4, 9). For example, murnong-type Asteraceae, Onagraceae, and Pimelea spp. pollen are absent, while only one grain of Banksia spp. pollen was detected in a single scat (square R31 XU10) of the 22 analyzed, whereas Banksia spp. pollen is abundant in the sediment samples at this level. At the same time, the possum scat samples include pollen that does not have recorded cultural uses (such as Euphorbiaceae, Fabaceae, and Hibbertia spp.; Figure 9).
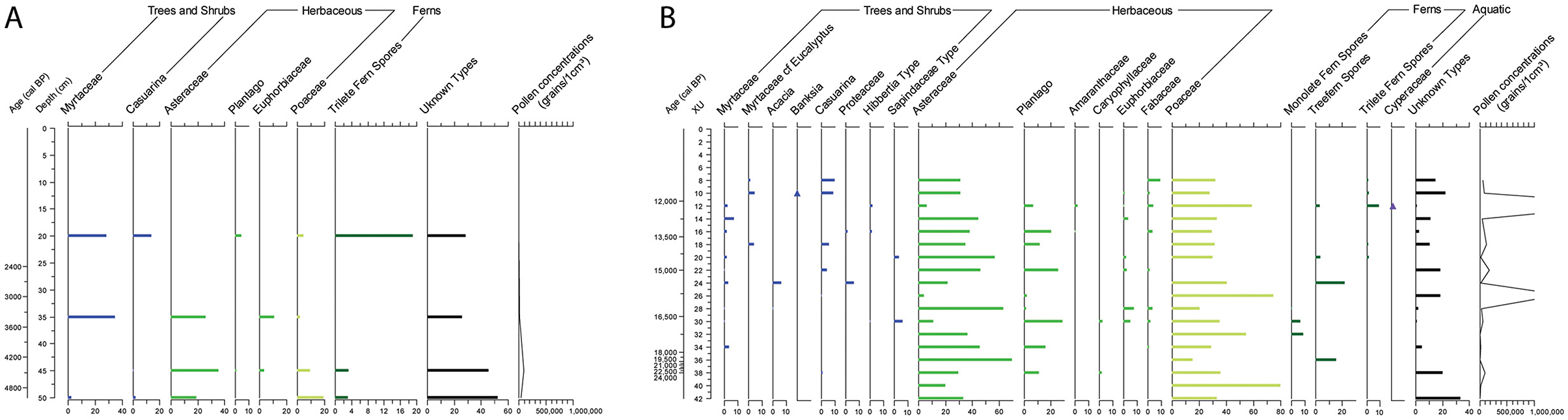
Figure 9. Percentage pollen diagrams of pollen extracted from possum scats. (A) Possum scats from P35. (B) Possum scats from R31. Dominant taxa (accounting for ≥2% of the total pollen and spore sum in at least one sample) are plotted. ▴ = presence of culturally important taxa contributing <2% of total pollen and spores. The pollen concentration scales are capped at 1 million grains per 1 cm3 scat sample, with two R31 scat samples exceeding this value (XU12: 3,093,120 grains/cm3 scat; XU26: 1,493,280 grains/cm3 scat).
7 Discussion
Cloggs Cave's pollen record is unusual for an Australian setting due to its abundant and well-preserved pollen sequence that extends back at least 25,000 years, and which appears to have a major contribution from human plant use. The selection of plants by the Old Ancestors also has an environmental correlate, indicating elements of vegetation growing near the cave that were available for cultural selection. Therefore, both the air-fall component of the pollen signature, and anthropogenic selection of flowering plants carried into the cave, provide insights into palaeoenvironmental conditions and environmental change during the LGM and on into the warmer and wetter post-glacial period (especially the Early and Mid-Holocene beginning 11,700 cal BP).
7.1 Geomorphological context of the pollen sequence
Pollen concentrations decline markedly after c. 5,000 cal BP and remain very low to the present (Figure 4). The start of this decrease coincides with the formation of a sinkhole in the Main Chamber, in the area of P34 and P35 (Delannoy et al., 2020). Around 6,000 cal BP, the Main Chamber's sediments (Figure 3) received an influx of drip-water causing a localized sinkhole to form, almost simultaneously infilling with torrential sediment collapse from upslope. The top of the sinkhole remained a depression until it was more gradually infilled under very low topographic energy, a process associated with the closure of a second, lower palaeoentrance into the cave (see below; Delannoy et al., 2020).
Cloggs Cave once had two entrances, one on top of the other; the two were separated by a thin horizontal rock platform (the floor of the current Entrance Passage; Figure 10). Today only the upper entrance remains, the lower one being closed off by accumulated fill. From the Porch immediately outside the cave (see Figure 2), the top of the lower entrance's ceiling is still visible today. Its upper 36 cm remain free of sediment, so that its ceiling remains exposed. The rest of the lower (palaeo) entrance is clogged with fine sediments and limestone blocks that fell from the Porch's walls and ceiling, as revealed by Josephine Flood's (1980) 1971–1972 Porch excavations in squares W–X–Y–V, positioned outside the cave against the lower entrance. Although that fill has not been well-dated—a single radiocarbon age of 1,110 ± 70 BP (798–1,176 cal BP, ANU-1183) was obtained on combined comminuted charcoal from the upper part of the fill in square W spit 5 (Flood, 1973, Figures 39–40)—inside the cave the stratigraphy of R31 (Figure 3), excavated in 2020, reveals important details. Here SU4U–SU4A are oriented relatively flat, indicating more a process of clogging than of evacuation of deposits toward the outside through an open entrance acting as a conduit. That conduit has been completely clogged since 6,000 cal BP, as indicated by the slope of the SU3–SU2–SU1 deposits that all date to 6,000 cal BP or younger in P34–P35 and R31 (Delannoy et al., 2020). These upper stratigraphic units largely cover an ancient stalagmite that grew from a lower floor level next to the lower entrance in the Main Chamber. Only the top of the stalagmite is visible today (Figure 11).
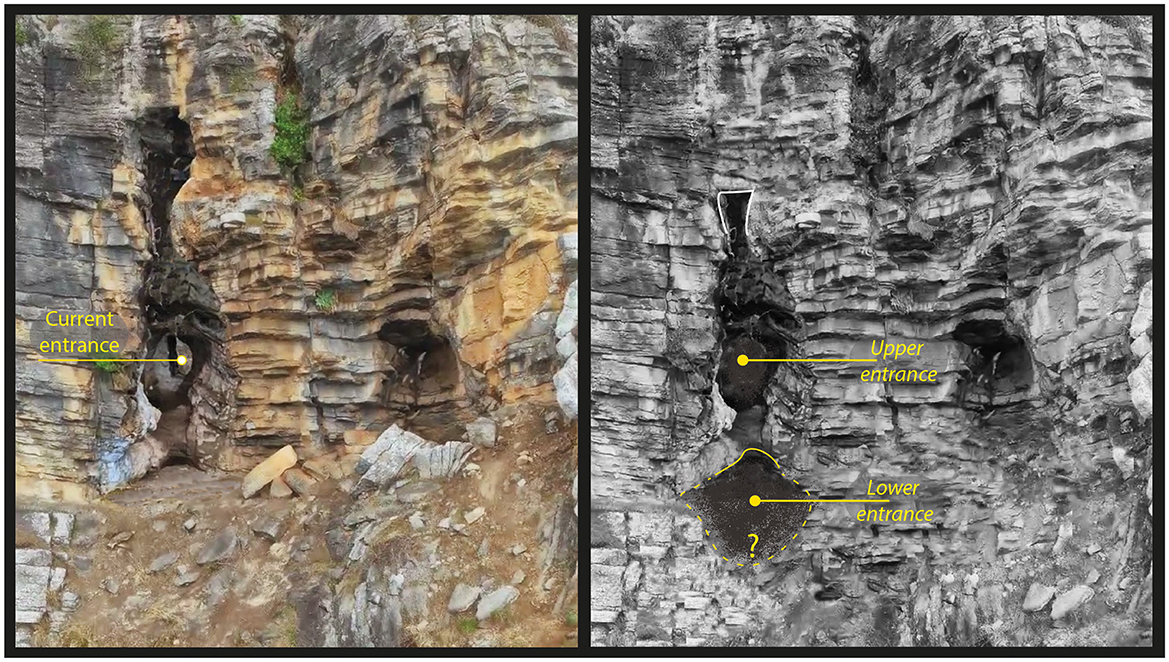
Figure 10. Locations of Cloggs Cave's current and former entrances. The white line (in right-hand image) above the upper entrance shows the ceiling section from which the blocks in Josephine Flood's 1971–1972 Porch excavations originated. The right-hand image is a reconstruction (figure by Jean-Jacques Delannoy).
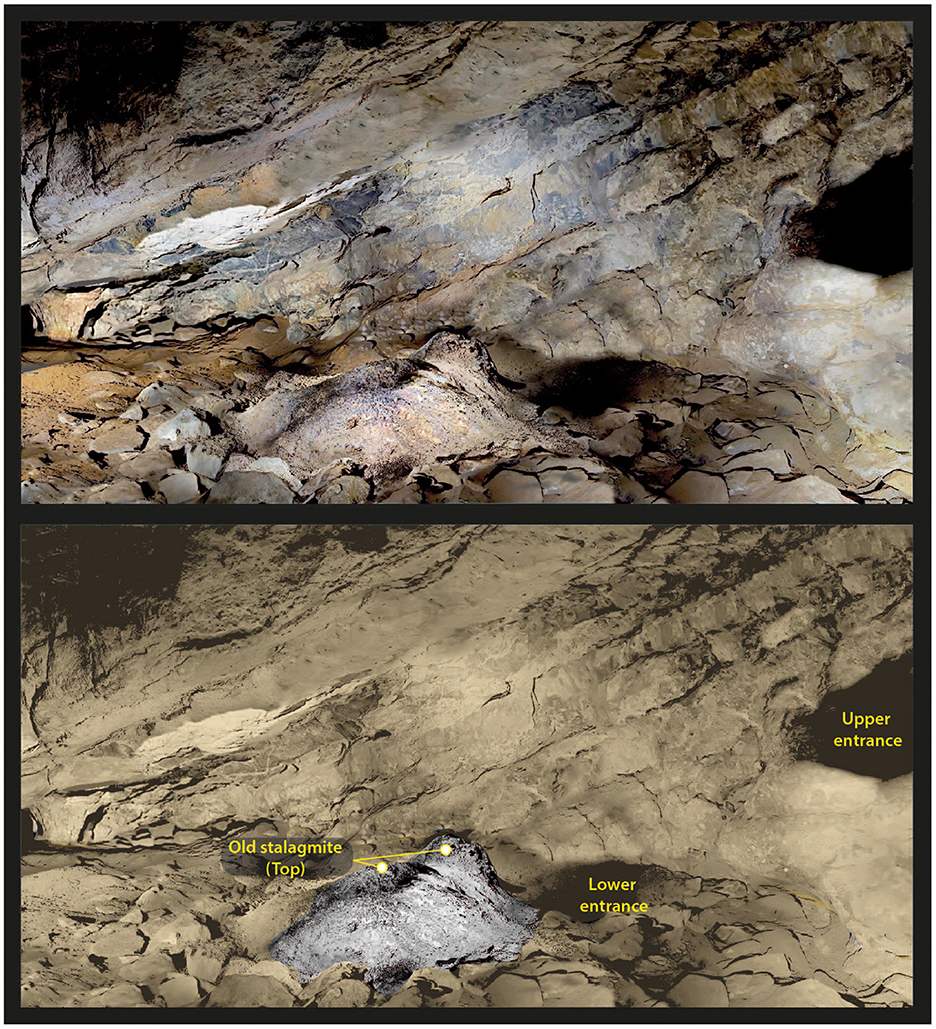
Figure 11. Location of the two entrances from inside the cavity (Main Chamber). Note the presence of an old stalagmite whose summit emerges from sediments accumulated since the closure of the lower entrance (image by Jean-Jacques Delannoy).
It is likely that the catastrophic event at the origin of the torrential flow into the then-newly formed sinkhole in the Main Chamber related to the climatic instabilities documented for southern Australia between 9,000 and 5,000 cal BP (Xia et al., 2001; Stanley and De Deckker, 2002; Gouramanis et al., 2013). Those climatic conditions also favored the fall of unstable blocks from the Porch's walls and ceiling. Such associations between gravitational rockfall/cliff collapse and heavy precipitation have been demonstrated many times both in Australia and elsewhere around the world (e.g., Gruner, 2008; Krautblatter and Moser, 2009). They are also consistent with Flood's (1980) description of the accumulated deposits in squares W–X–Y–V, at the base of the Porch excavations. Here the upper deposits are mainly composed of blocks, with fine sediments in-between. Toward the base of the excavations, fine sediments predominate. This dual deposit consisting of rockfall over finer sediments means that the rockfall from outside the cave was the cause of the lower entrance's closure. The lower entrance became sealed from the outside, causing the accumulating SU3–SU2–SU1 sediments in the Main Chamber to abut inside the cave.
Before 6,000 cal BP, the Main Chamber was thus connected with the external environment by both the upper and lower entrances. After the rockfall of c. 6,000 cal BP, only the upper entrance remained open (Figure 12). This change in entrance geometry not only reduced entry into the cave through a single entrance, but also altered air flows in and out of the cave. The existence of two entrances one immediately above the other had promoted the exchange of air between the exterior and interior of the cave, creating seasonal air currents. In winter, the lower entrance sucked in air from outside and the upper entrance pushed it out, while in summer the reverse happened. This phenomenon is called the “Chimney Effect,” caused by temperature differentials between inside and outside the cave: the temperature in the cave varies little through the course of the year, but that of the outside air varies seasonally. The cave thus works like a chimney: in winter cold air enters from below and warm air rises and escapes from above, while in summer the process is reversed (see e.g., Lewis, 1991; Lismonde, 2002; Wigley and Brown, 1976). The full northern exposure of the escarpment into which the cave opens contributes to the temperature differential between the exterior and interior of the cave, and thus to an accentuation of the Chimney Effect.
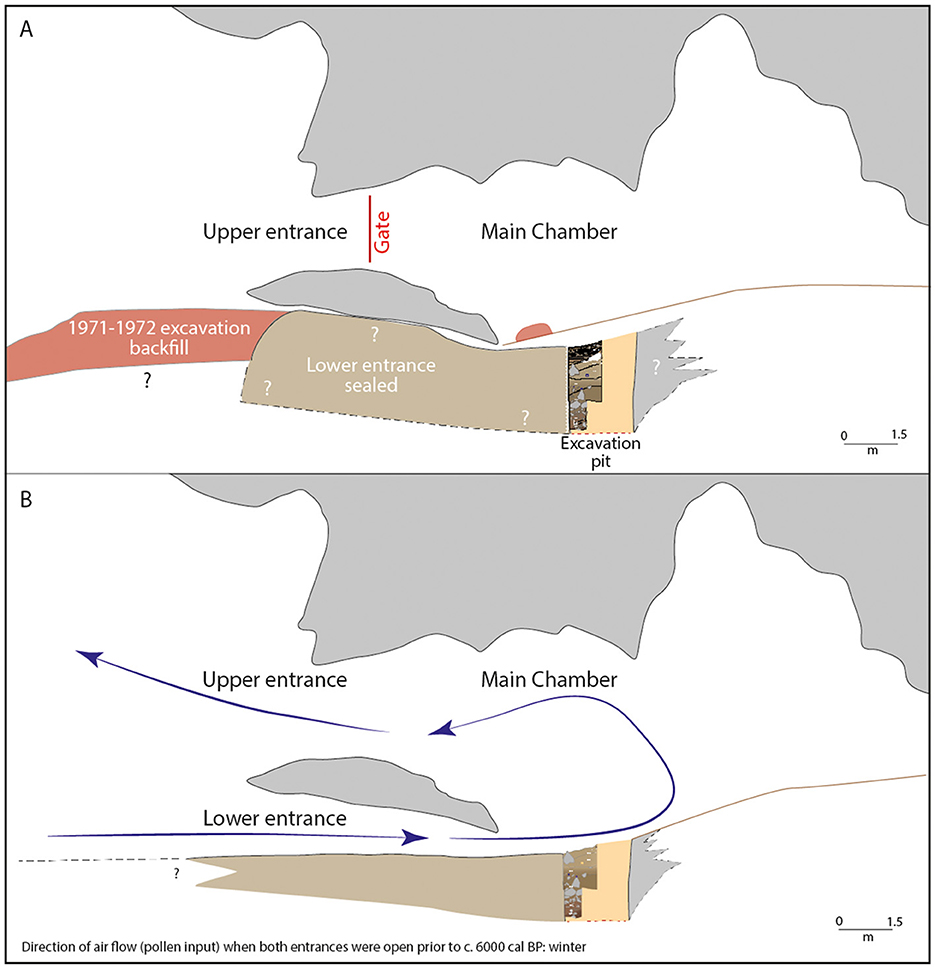
Figure 12. Profile view from the Porch to the Main Chamber, Cloggs Cave, showing the position of P34 and P35. (A) Current configuration with a single entrance. (B) Configuration before closure of the lower entrance c. 6,000 cal BP (with the infilled P34–P35 included, to show the location of the excavation square), showing winter air currents in and out of the cave through the two entrances (image by Jean-Jacques Delannoy).
These air currents would have carried pollen into the cave. The closure of the lower entrance changed the kinetics of the air currents and air flow in the cave. The inflow of pollen from outside became notably reduced, or even ceased altogether. These geomorphological observations (see Delannoy et al., 2020) are consistent with the reduction in total pollen in the cave sediments c. 5,000 cal BP (see Figure 4).
7.1.1 Predominant winds and the orientation of Cloggs Cave's palaeoentrances
The timing of deposition of the pollen in Cloggs Cave spans a period of major regional climate change, namely the transition from the cold LGM to the warmer Mid-Holocene. During the LGM, there is inferred to be widespread landscape instability across eastern Australia (Hill and Bowler, 1995; Thom et al., 1994). From c. 25,000 to 15,000 BP, winds were predominantly westerly south of 31°S (Thom et al., 1994), and this interpretation is supported in coastal Gippsland (Loch Sport) by luminescence ages for dunes indicative of active palaeowind directions of 75° to 91° between 23,000 ± 1,100 and 18,000 ± 900 years ago (Oliver et al., 2018). As the LGM dunes at Loch Sport are located a considerable distance from the Great Dividing Range, their orientation can be assumed to represent the regional wind patterns during the LGM. The current coastal wind-field for Gippsland also is dominated by winds from the west to southwest (O'Grady et al., 2015). Comparisons between LGM and Early Holocene wind records from the New England Tablelands indicate that there was little change in the dominant westerly wind flow during these two climatic periods (Shulmeister et al., 2016), and this appears to also apply to the Gippsland region. The cliffs in which Cloggs Cave is formed are exposed to a wind-field spanning 160°-278° (NNW–E). This orientation means they are protected from the dominant wind-field present on the coast during the LGM and Holocene. As the cave is found in a hilly terrain, modification of the regional wind-field is to be expected. Critically for this site, the relative similarity in regional winds between the LGM and Holocene, the cave entrance's orientation, and the river valley setting means that the wind-field at the entrance to Cloggs Cave remained virtually unchanged during the entire period of Aboriginal occupation. The cave entrance remained on the lee-side of predominant winds, minimizing pollen-bearing airflow into the cave from the outside.
7.2 Vegetation history and palaeoenvironments in Gunaikurnai Country
Proxy records of environmental change across temperate Australia reveal that the coldest and driest phase of the past 30,000 years centers on the LGM (for a synthesis, see Petherick et al., 2013 and references there-in). Records of palaeo-vegetation suggest an open landscape dominated by daisies and grasses during the LGM, with an expansion of forest elements with the onset of warmer and wetter conditions associated with the transition into the Holocene (11,700 cal BP). Caledonia Fen (Kershaw et al., 2007) is geographically the closest and best-resolved pollen record to Cloggs Cave, and although situated at 1,280 m a.s.l., has a similar pollen signature for the past 24,000 years, namely an open herbaceous landscape during the LGM and Late Glacial, followed by a forested landscape dominated by Eucalyptus spp. with the onset of the Holocene.
The differences between the pollen sequences of P35 and R31 (Figure 4), and highlighted in the PCA (Figure 7), are largely related to the different time brackets covered by their sediments. The R31 pollen sequence, mainly dating between 25,000 and 11,000 cal BP, indicates that until the Holocene the climate around Cloggs Cave was cooler and drier than present, and the environment supported a more open vegetation dominated by herbaceous taxa such as Asteraceae and Poaceae, a pattern reflected in both the sediment and possum scat pollen samples. This is also in keeping with the palaeo-vegetation records from elsewhere in the broader region (e.g., Dodson, 1974, 1975, 1977; Harle, 1997; Kershaw et al., 2007).
The increase in woody taxa and replacement of grass with more fleshy herbaceous taxa indicates that the climate gradually became warmer and wetter between c. 12,000 and 5,000 cal BP. This shift in vegetation structure is identified in detail in the R31 sediment pollen sequence, and is broadly supported by the R31 scat pollen samples that show the emergence of woody taxa through time. This transition is further delineated in the PCA of the pollen assemblages, where the main driver for variation along Axis 1 is the incidence of woody taxa relative to herbaceous taxa (Figure 7). The increase in ferns, requiring wet conditions, is consistent with the interpretation of a warmer and wetter climate after 12,000 cal BP. The time period after 5,000 cal BP is represented by the P35 sequence in which both sediment pollen and scat pollen assemblages demonstrate increasing tree cover. Low to no pollen in the stratified ash layers of SU2 precludes any detailed insights into the period between 5,000 and 1,460 cal BP.
There are only a handful of palaeoenvironmental records from SE Australia that capture the LGM and the Holocene, but all show a similar transition from an open herbaceous landscape to an environment with more woody elements over the past 12,000 years (e.g., Adeleye et al., 2023; Dodson, 1974, 1975, 1977; Harle, 1997; Kershaw et al., 2007). At Cloggs Cave, the pollen assemblage continues to be dominated by herbaceous taxa, suggesting taphonomic processes other than airfall.
7.3 Human plant use
As noted above, GunaiKurnai caves are known from nineteenth century ethnographic sources (e.g., Howitt, 1876, 1887) and present-day Elders to have been the abode of mulla-mullung (“clever men” and “clever women”). They are secluded places where secretive spiritual practices took place, including the performance of rituals for spells and healing (for details, see David et al., 2021b; Mialanes et al., 2022; Mullett et al., 2021; Pepper, 1980; Pepper and De Araugo, 1985). The pollen results presented here provide several lines of evidence that strongly suggest that a major source of pollen at Cloggs Cave was through the introduction and purposeful use of plant material by people. With the exception of four of the SU2 samples from P35, and the topmost seven samples from R31, all of which are barren or show low pollen contents, the high pollen concentrations of all other samples (Figure 4) are remarkable for a cave site, especially one with such a long and narrow, curving entrance, on the lee side of the prevailing winds.
Many of the dominant plant taxa identified in the P35 and R31 sediment samples have known cultural uses (Supplementary material 2), as evident from both nineteenth century ethnographic documents and current GunaiKurnai knowledge. These dominant pollen taxa (combination of single pollen counts and single-taxa pollen clumps) from Cloggs Cave include Asteraceae (daisies), Banksia spp., Casuarina spp. (she-oak), members of the Myrtaceae family including Eucalyptus spp. and Leptospermum spp., Onagraceae (evening primrose), Pimelea spp. (rice flowers), Plantago spp. (plantain), Poaceae (grass), and ferns (Figures 4, 5; Supplementary material 2).
The abundance of clumped single-taxon pollen in terms of deposition and taphonomy is unusual and suggests the purposeful transport of flowering material by the Old Ancestors as the primary source of much of the pollen in the P35 and R31 cave sediments (Figures 4, 8). Pollen is usually released from flowers as single grains for transport on air currents or by insect vectors to fulfill plant fertilization. The presence of single-taxon pollen clumps indicates that deposition onto the Cloggs Cave palaeo-floors happened before the natural detachment of the pollen grains or dehiscence, that is, while still on the flowers. Single taxon pollen clumps do occur naturally, especially in insect-pollinated plants (Martin et al., 2009). However, they are not aerodynamic enough to disperse by wind and so represent pollen transport over short distances from the parent plant (Cummings et al., 2007). Pollen clumps, often composed of a mix of taxa, can also result from the direct consumption of flowering material by people or animals, ultimately becoming a component of their waste (McDonough, 2019). Insect activity, such as bee nesting, can also produce pollen clumps (Hunt et al., 2023; see also Bottema, 1975). But once again these are usually a mix of taxa, and squares P35 and R31 at Cloggs Cave are not situated beneath walls that could support bee or wasp nests, nor are there any signs of bee or wasp nests in the excavated sediments at Cloggs Cave.
Clumps of pollen in sediments from archaeological sites have routinely been interpreted as the in situ remains of flowers, either from plants growing in or around the site, or from flowering material transported to the site (Cummings et al., 2007; Florenzano, 2019; Hunt et al., 2016; Mercuri, 2008; Weinstein-Evron, 1994). One of the most renown examples is Shanidar Cave in Iraqi Kurdistan, where pollen clumps from sediments adjacent to the skeleton of the Shanidar 4 Neanderthal has been interpreted in a variety of ways, initially as evidence of intentional placement of flowers and thus a purposeful burial (Leroi-Gourhan, 1975; Solecki, 1975). The most recent reinvestigation of the palynological evidence interprets these mixed-taxon pollen clumps as having accumulated through bee nesting activities, and do not reflect the actions of people (Hunt et al., 2023).
Cloggs Cave by contrast has numerous single-taxon pollen clumps, with all clumped taxa known to have Aboriginal cultural uses in various parts of SE Australia (Figures 4, 8). Banksia spp., Pimelea spp. (rice flower), Plantago spp. (plantain), Poaceae (grass), Myrtaceae (gum tree), Onagraceae (evening primrose), and various species of Asteraceae (daisy) are found in the pollen assemblage both as single grains and as indehiscent clumps. While Onagraceae, Plantago spp., Poaceae, and Banksia spp. provide sources of food (all) and materials (e.g., Poaceae for making baskets, and for burning to ash for a variety of purposes; Banksia spp. as fire-starters), the rest of the taxa are used in traditional medicine (e.g., Cumpston, 2020; De Angelis, 2005; Gott, 2008; Packer et al., 2012) in various parts of SE Australia. Cultural usage of the pollen species identified in the Cloggs Cave assemblage accord well with previous archaeological evidence and ethnohistoric knowledge of the site as a special place that was visited sporadically for magic, medicine and ritual rather than for everyday living activities and continued occupation (David et al., 2021a, b). Further details of the clumped pollen and other known useful plants represented by single pollen are discussed in the Supplementary material 2.
The clumped pollen is also associated with observations of high amounts of phytoliths including articulated phytoliths reflecting in situ deposition as well as phytoliths blackened from fire (Figure 6G). Moreover, clumps of single-taxon pollen were not detected in the possum scat samples (discussed below).
7.4 Small mammals and other vectors
The interpretation of plant use from the Cloggs Cave pollen record rests on the large quantities of pollen not normally seen in settings such as lakes and swamps, as well as the large concentration of single-taxon pollen clumps, suggesting the introduction of flowering material into the cave. Two alternative possibilities for the pollen clumps were considered. The first is that pollen clumps in the sequence are derived from fecal material deposited by animals (Florenzano, 2019). Our analyses show that clustering of pollen in the possum scats has a very different composition to the pure single-taxon pollen clumps in the sediments (compare Figures 8A–E with Figure 8F). The possum scat clusters contain heterogeneous mixtures of many different types of pollen as well as other organic matter (Figure 8F) that is largely consistent with descriptions of fecal material (Bronnimann et al., 2017a,b).
The second alternative possibility for the source of pollen clumps in the sediments is insect pollination and subsequent deposition of insects along with their pollen loads in the sediments (Davis and Buchmann, 1994; Grímsson et al., 2017). Unlike pollen deposited by insects, the pollen clumps at Cloggs Cave are common rather than isolated occurrences. Moreover, the Cloggs Cave single-taxon pollen clumps include taxa whose pollen is normally wind-dispersed (e.g., Plantago spp. and Poaceae), suggesting that insect pollination is not a factor.
We now turn to consider the role of small mammals such as possums in the accumulation of pollen at Cloggs Cave. A limitation of comparing the pollen accumulated in the sediments with that in the possum scats are the different timespans represented by the two types of samples. The pollen in individual possum scats represent a single night of foraging of a single possum. Thus, each possum scat's pollen represents a limited selection of plant taxa reflective of a single foraging event within a narrow time resolution—or “snapshot” of pollen accumulation. In contrast, the sediment samples typically contain tens and sometimes hundreds of years of pollen accumulation—or “time-averaged” assemblages. Due to the different temporal scales and taphonomic processes involved in the accumulation of pollen in the scats and sediments respectively, the two datasets are distinct and not directly comparable. Despite the sediment pollen's longer (time-averaged) time-frames, they nevertheless retain single-taxon pollen clusters from individual events of deposition, unlike the individual-event multi-taxa pollen clusters represented by the possum scats.
As possum scats give an indication of plant taxa which grew near the cave across short-term timescales, they can help to reconstruct vegetation and landscape structure in terms of openness of landscape. The pollen composition of the possum scats is chiefly composed of background vegetation elements also identified in the sedimentary pollen, such as Asteraceae, Myrtaceae, Plantago spp., Poaceae, Casuarina spp., and fern spores. These taxa comprise the dominant composition of the landscape, i.e., local vegetation that would have been readily available to both possums and people near the cave. Therefore, the scats broadly reflect trends in vegetation and landscape composition established by the sedimentary pollen. However, the scats do not show synchronicity with respect to specific plant compositions in the sedimentary pollen. Significantly, culturally important taxa that are uncommon in the landscape but which are found in the sediment pollen assemblages are absent from the possum scats, such as murnong-type Asteraceae, Onagraceae, and Pimelea spp. pollen, while only one grain of Banksia spp. pollen was found throughout the entire scat samples. At the same time, the possum scat samples include pollen that does not have recorded cultural uses (such as Euphorbiaceae, Fabaceae, and Hibbertia spp.). This suggests that cultural selection of plants, and not possums (through their scats and potentially also pollen on their coats), was the main driver of pollen accumulation in the sediments.
7.5 Other aspects of the story
This study sheds light on the deep antiquity of cultural activities and uses of Cloggs Cave by the Old Ancestors over many thousands of years. Archaeological investigations in the cave have revealed stratified ash layers (SU2) around a buried standing stone; a small, portable grindstone that had been used to crush crystalline minerals as well as to process Bogong moths; and the deposition of large numbers of soda-straw stalactite fragments and quartz crystals dated between c. 2,400 and 1,600 cal BP (David et al., 2021b; Mialanes et al., 2022; Stephenson et al., 2020). The majority of the pollen samples from this time period have low to no pollen content. However, the proliferation of well-preserved pollen in the earlier layers of the cave, including strong evidence for the cultural uses of particular plant taxa in layers with ritual installations including wooden artifacts whose Casuarina spp. wood was brought into the cave when green (for details, see David et al., 2024), adds significant information on the kinds of activities the Old Ancestors undertook in the cave during and after the LGM. The Casuarina spp. trimmed, green (recently living) wooden sticks that formed part of two ritual installations came from XU8–9 and XU11 in square R31, at precisely the same stratigraphic level (XU7–XU11, dated from 12,150 to 10,000 cal BP) as the peak of Casuarina spp. pollen in the sediments.
Low to no pollen in the finely stratified ash layers of SU2 in P35 and R31 is probably due to thermal destruction of pollen during use of the hearths. Pollen does not preserve when subjected to intense heating (Bryant and Holloway, 1983), and experimental studies on exposure of pollen to high temperatures indicate that complete deterioration and loss of pollen grains takes place at temperatures upward of 350°C (Ghosh et al., 2006; Pini et al., 2018; Sengupta, 1975), temperatures which hearths and open fires are capable of exceeding (David, 1990; Ward and Friesem, 2021). The ash layers of SU2 are associated with the standing stone of P35, dozens of broken soda-straws, and a portable grindstone used to process crystalline minerals (e.g., David et al., 2021b; Mialanes et al., 2022; Stephenson et al., 2020).
Earlier in the R31 sequence (e.g., XU22–XU26), the strong presence of phytoliths in the pollen slides (Figure 6E), some of which show burning (Figure 6G), indicates that grasses were introduced into the cave and subjected to burning. Phytoliths have different taphonomic pathways to pollen, being typically released into soils and sediments as a local in situ element of plant decay and not commonly transported on air currents (Piperno, 2006). Taken together, the evidence of pollen and phytoliths in the Cloggs Cave sediments indicates a large amount of plant material that must have accumulated in the cave through cultural deposition.
Unlike pollen grains, phytoliths can be discriminated to sub-families of Poaceae, and their identification also provides additional insights into particular plant parts (e.g., leaves/stems vs. inflorescences; Piperno, 2006). Micromorphology aids the observation of plant remains in their in situ sedimentary contexts, enabling the identification of how plants were used by people in the past, such as in the detection of bedding in Pleistocene deposits at Sibudu Cave, South Africa (Wadley et al., 2011), and the reconstruction of hearths and associated rake-out areas and site maintenance practices (e.g., Mallol et al., 2013; Whitau et al., 2018). Such micromorphological and phytolith analyses of the Cloggs Cave sediments are in progress.
8 Conclusion
The pollen assemblage from Cloggs Cave is an exceptional archaeological record in and of itself. It demonstrates evidence of the selection, transportation and use of a suite of plants (Asteraceae, Banksia spp., Casuarina spp., Myrtaceae, Onagraceae, Plantago spp., Pimelea spp., and Poaceae) throughout the sequence going back 25,000 years, with the selection of plants taking place during a range of climatic and environmental conditions, as evidenced especially by the pollen in the possum scats.
Ethnohistoric texts dating back to the nineteenth century, and current GunaiKurnai knowledge, testify to caves in GunaiKurnai Country being the abodes of “clever men” and “clever women” who undertook special activities in seclusion. All identified clumped single-taxon pollen—the flowering plants demonstrably brought into the cave by people—have known Aboriginal uses, and most are documented in nineteenth century GunaiKurnai ethnographic texts and/or through current GunaiKurnai knowledge. Of particular interest in this regard is the pollen from two taxa: Banksia spp. and Casuarina spp.. Banksia spp. is a plant genus whose spikes were commonly used as fire-starters (Smyth, 1878, viii, 132), and the nectar in their fresh flowers was drunk as a sweet secretion (Smyth, 1878, p. 213). Straight or straightened Casuarina spp. sticks were used by mulla-mullung to undertake powerful ritual fire incantations in secluded places (e.g., Howitt, 1887, p. 27–28, 33; 1904, p. 376; see also Smyth, 1878, p. 475). Two such ritual fireplaces and their associated Casuarina spp. sticks (which had been collected green in terminal Pleistocene and Early Holocene times) were excavated from the same layers as the Banksia spp. and Casuarina spp. pollen peaks in R31 (David et al., 2024).
While the main source of pollen accumulation in the cave appears to have been the Old Ancestors, climatic and paleoenvironmental conditions can also be gleaned from the pollen record, as the flowering plants brought into the cave probably grew nearby, and the possum scats reveal a demonstrably local environmental record. Together, Cloggs Cave's well-stratified pollen record represents people–plant relations over some 25,000 years of cultural history. The Cloggs Cave pollen—from all sources including through the manual introduction of flowers by the Old Ancestors, and pollen dispersal by air currents and animals—indicate a shift from the cooler and drier climate with an open landscape dominated by herbs and grasses at the peak of the Last Ice Age (the LGM), to the warmer and wetter climate of the Early Holocene that saw the expansion of woodland vegetation (e.g., Banksia spp., Eucalyptus spp.). Cloggs Cave's pollen record provides remarkable insights into two intimately connected histories: the cultural practices of the Old Ancestors and environmental conditions of GunaiKurnai Country across a thousand generations of ancestors.
Data availability statement
The original contributions presented in the study are included in the article/Supplementary material, further inquiries can be directed to the corresponding author.
Ethics statement
Ethical approval was not required for the study involving animals in accordance with the local legislation and institutional requirements because the animal samples were from ancient scats of possums excavated from an archaeological site.
Author contributions
EG: Conceptualization, Investigation, Methodology, Visualization, Writing – original draft. BD: Conceptualization, Funding acquisition, Resources, Visualization, Writing – original draft. JS: Conceptualization, Funding acquisition, Investigation, Methodology, Resources, Supervision, Visualization, Writing – original draft. JF: Writing – review & editing. RM: Conceptualization, Resources, Writing – review & editing. BK: Investigation, Writing – review & editing. CG: Investigation, Writing – review & editing. JA: Writing – review & editing. GunaiKurnai Land and Waters Aboriginal Corporation: Writing – review & editing. MM: Writing – review & editing. FP: Writing – review & editing. J-JD: Writing – review & editing. AR: Writing – review & editing. DK: Writing – review & editing.
Funding
The author(s) declare financial support was received for the research, authorship, and/or publication of this article. This work was undertaken through the Australian Research Council Center of Excellence for Australian Biodiversity and Heritage (CE170100015) and the Australian Research Council “Before and After the Last Ice Age: GunaiKurnai Archaeology Along the Snowy” Project (LP230100385). We thank the Australian Research Council and LP230100385 project partner Rock Art Australia for funding this project.
Acknowledgments
We thank the GunaiKurnai Elders Council, the Monash Indigenous Studies Center at Monash University, EDYTEM at the Université Savoie Mont Blanc, the Palaeoworks Lab at ANU, and Martin and Vicky Hanman in Buchan for research support.
Conflict of interest
JF and RM were employed by GunaiKurnai Land and Waters Aboriginal Corporation.
The remaining authors declare that the research was conducted in the absence of any commercial or financial relationships that could be construed as a potential conflict of interest.
Publisher's note
All claims expressed in this article are solely those of the authors and do not necessarily represent those of their affiliated organizations, or those of the publisher, the editors and the reviewers. Any product that may be evaluated in this article, or claim that may be made by its manufacturer, is not guaranteed or endorsed by the publisher.
Supplementary material
The Supplementary Material for this article can be found online at: https://www.frontiersin.org/articles/10.3389/fearc.2024.1488477/full#supplementary-material
Footnotes
1. ^The terms “Old Ancestors” and “Old People” are the respectful and culturally appropriate ways to refer to past generations of GunaiKurnai, as advised by the Elders today.
References
Adeleye, M. A., Haberle, S. G., Gallagher, R., Andrew, S. C., and Herbert, A. (2023). Changing plant functional diversity over the last 12,000 years provides perspectives for tracking future changes in vegetation communities. Nat. Ecol. Evol. 7, 224–235. doi: 10.1038/s41559-022-01943-4
Basumatary, S. K., and Tripathi, S. (2021). Is bat guano a potential pollen trap? a comparative assessment from conventional soil and moss substrates from Eraaning Cave of Meghalaya, India. Rev. Palaeobot. Palynol. 295:104539. doi: 10.1016/j.revpalbo.2021.104539
Bennett, K. D., and Willis, K. J. (2001). “Pollen,” in Tracking Environmental Change Using Lake Sediments. Volume 3: Terrestrial, Algal, and Siliceous Indicators, eds. J. P. Smol, H. J. B. Birks, and W. M. Last (New York, NY: Kluwer Academic Publishers), 5–32.
Bottema, S. (1975). The interpretation of pollen spectra from prehistoric settlements (with special attention of Liguliflorae). Palaeohistoria 1975, 17–35.
Bradshaw, D. (2014). “Pollination vectors: vertebrates,” in Plant Life on the Sandplains in Southwest Australia, a Global Biodiversity Hotspot, ed. H. Lambers (Perth, WA: UWA Publishing), 207–213.
Bronnimann, D., Ismail-Meyer, K., Rentzel, P., Pumpin, C., and Lenka, L. (2017a). “Excrements of herbivores,” in Archaeological Soil and Sediment Micromorphology, eds. C. Nicosia and G. Stoops (Hoboken, NJ: John Wiley and Sons), 55–66.
Bronnimann, D., Pumpin, C., Ismail-Meyer, K., Rentzel, P., and Égüez, N. (2017b). “Excrements of omnivores and carnivores,” in Archaeological Soil and Sediment Micromorphology, eds. C. Nicosia and G. Stoops (Hoboken, NJ: John Wiley and Sons), 67–81.
Bryant, V. M., and Holloway, R. G. (1983). “The role of palynology in archaeology,” in Advances in Archaeological Method and Theory, ed. M. B. Schiffer (New York, NY: Academic Press), 191–224.
Cadd, H., Petherick, L., Tyler, J., Herbert, A., Cohen, T. J., Sniderman, K., et al. (2021). A continental perspective on the timing of environmental change during the last glacial stage in Australia. Quat. Res. 102, 5–23. doi: 10.1017/qua.2021.16
Carrión, J. S., Ochando, J., Sniderman, K., and Festi, D. (2022). Palaeobotanical records from cave environments. Rev. Palaeobot. Palynol. 306:104759. doi: 10.1016/j.revpalbo.2022.104759
Clark, P., Dyke, A. S., Shakun, J. D., Carlson, A. E., Clark, J., Wohlfarth, B., et al. (2009). The last glacial maximum. Science 325, 710–714. doi: 10.1126/science.1172873
Cummings, L. S., Puseman, K., Yost, C., with Henderson, K., and Varney, R. A. (2007). Pollen, Phytolith, and Macrofloral Analysis of Samples From Feature 57 at the Scu-Leavy Site, Technical Report 07-72. Paleo Research Institute: Santa Clara, CA.
Cumpston, Z. (2020). Indigenous Plant Use: A Booklet on the Medicinal, Nutritional and Technological Use of Indigenous Plants. Clean Air and Urban Landscapes Hub, National Environmental Programme. Parkville, MD: University of Melbourne.
David, B. (1990). “How was this bone burnt?” in Problem Solving in Taphonomy: Archaeological and Palaeontological Studies from Europe, Africa and Oceania, eds. S. Solomon, I. Davidson and D. Watson (Tempus 2. St. Lucia: University of Queensland), 65–79.
David, B., Arnold, L. J., Delannoy, J.-J., Fresløv, J., Urwin, C., Petchey, F., et al. (2021a). Late survival of megafauna refuted for Cloggs Cave, SE Australia: implications for the Australian Late Pleistocene megafauna extinction debate. Quat. Sci. Rev. 253:106781. doi: 10.1016/j.quascirev.2020.106781
David, B., Fresløv, J., Mullett, R., GunaiKurnai, L., Waters Aboriginal Corporation, Delannoy, J.-J., et al. (2021b). 50 years and worlds apart: rethinking the Holocene occupation of Cloggs Cave (East Gippsland, SE Australia) five decades after its initial archaeological excavation and in light of GunaiKurnai world views. Austral. Archaeol. 87, 1–20. doi: 10.1080/03122417.2020.1859963
David, B., Mullett, R., Wright, N., Stephenson, B., Ash, J., Fresløv, J., et al. (2024). Archaeological evidence of an ethnographically documented Australian Aboriginal ritual dated to the last ice age. Nat. Hum. Behav. 24:1912. doi: 10.1038/s41562-024-01912-w
Davis, O. K., and Buchmann, S. L. (1994). “Insect sources of pollen clumps in archaeological sites in southestern USA: ground-nesting bees and mites,” in Aspects of Archaeological Palynology: Methodology and Applications, ed. O. K. Davis (Houston, TX: American Association of Stratigraphic Palynologists Foundation), 63–73.
De Angelis, D. (2005). Aboriginal Use Plants of the Greater Melbourne Area. Bundoora: La Trobe University.
Delannoy, J.-J., David, B., Fresløv, J., Mullett, R., GunaiKurnai, L., Waters Aboriginal Corporation, Green, H., et al. (2020). Geomorphological context and formation history of Cloggs Cave: what was the cave like when people inhabited it? J. Archaeol. Sci. 33:102461. doi: 10.1016/j.jasrep.2020.102461
Dodson, J. (1974). Vegetation history and water fluctuations at Lake Leake, southeastern South Australia. 1. 10,000 B.P. to present. Austral. J. Bot. 22, 719–741. doi: 10.1071/BT9740719
Dodson, J. (1977). Late quaternary palaeoecology of Wyrie Swamp, southeastern South Australia. Quat. Res. 8, 97–114. doi: 10.1016/0033-5894(77)90058-8
Dodson, J. R. (1975). Vegetation history and water fluctuations at lake leake, South-Eastern South Australia. II.* 50 000 B.P. To 10 000 B.P. Austral. J. Bot. 23, 815-831., 1977 doi: 10.1071/BT9750815
Fitzgerald, A. E. (1984). “Diet of the possum Trichosurus vulpecula in three Tasmanian forest types and its relevance to the diet of possums in New Zealand forests,” in Possums and Gliders, eds. A. P. Smith and I. D. Hume (Chipping Norton: Surrey Beatty & Sons), 137–143.
Flood, J. (1973). The Moth Hunters: Investigations Towards a Prehistory of the South-Eastern Highlands of Australia (Ph. D. thesis). Department of Prehistory, The Australian National University, Canberra, ACT, Australia.
Flood, J. (1974). Pleistocene man at Cloggs Cave: his tool kit and environment. Mankind 9, 175–188. doi: 10.1111/j.1835-9310.1974.tb01389.x
Flood, J. (1980). The Moth Hunters: Aboriginal Prehistory of the Australian Alps. Canberra, ACT: Australian Institute of Aboriginal Studies.
Florenzano, A. (2019). The history of pastoral activities in S Italy inferred from palynology: a long-term perspective to support biodiversity awareness. Sustainability 11:404. doi: 10.3390/su11020404
Ghosh, R., D'Rozario, A., and Bera, S. (2006). Can palynomorphs occur in burnt ancient potsherds? an experimental proof. J. Archaeol. Sci. 33, 1445–1451. doi: 10.1016/j.jas.2006.01.015
Goldberg, P., and Mandel, R. D. (2008). “Caves and rockshelters,” in Encyclopedia of Archaeology, ed. D. M. Pearsall (New York, NY: Academic Press), 966–974.
Gott, B. (2008). Indigenous use of plants in south-eastern Australia. Telopea 12, 215–226. doi: 10.7751/telopea20085811
Gouramanis, C., De Deckker, P., Switzer, A., and Wilkins, D. (2013). Cross-continent comparison of high-resolution Holocene climate records from southern Australia—deciphering the impacts of far-field teleconnections. Earth-Sci. Rev. 121, 55–72. doi: 10.1016/j.earscirev.2013.02.006
Grímsson, F., Zetter, R., Labandeira, C. C., Engel, M. S., and Wappler, T. (2017). Taxonomic description of in situ bee pollen from the middle Eocene of Germany. Grana 56, 37–70. doi: 10.1080/00173134.2015.1108997
Gruner, U. (2008). “Climatic and meteorological influences on rockfall and rockslides,” in Proceedings of the 11th Interpraevent Congress Protection of Populated Territories from Floods, Debris Flows, Mass Movements and Avalanches, Vol. 2 (Dornbirn), 147–158.
Haberle, S. G., Rowe, C., Proske, U., Stevenson, J., Hopf, F., and Hope, G. S. (2021). A new version of the online database for pollen and spores in the Asia-pacific region: the Australasian Pollen and Spore Atlas (APSA 2.0). Quat. Austral. 38, 27–31.
Harle, K. J. (1997). Late quaternary vegetation and climate change in southeastern Australia: palynological evidence from marine core E55-6. Palaeogeogr. Palaeoclimatol. Palaeoecol. 131, 465–483. doi: 10.1016/S0031-0182(97)00016-3
Hill, S. M., and Bowler, J. M. (1995). “Linear dunes at Wilson's Promontory and South-East Gippsland, Victoria: relict landforms from periods of past aridity,” in Proceedings of the Royal Society of Victoria, Vol. 107, 73–81.
Hope, J. (1973). Analysis of Bone From Cloggs Cave, Buchan, N. E. Victoria. In Flood, J., The Moth Hunters: Investigations Towards a Prehistory of the South-Eastern Highlands of Australia, Appendix XIV (Ph. D. thesis). Department of Prehistory, The Australian National University, Canberra, ACT, Australia.
Howitt, A. W. (1876). Notes on the Devonian rocks of north Gippsland. Progr. Rep. Geol. Surv. Vict. 3, 181–249.
Howitt, A. W. (1887). On Australian medicine men; or, doctors and wizards of some Australian tribes. J. Anthropol. Inst. Great Brit. Ir. 16, 23–59. doi: 10.2307/2841737
Howitt, M. (1971). Come Wind, Come Weather: A Biography of Alfred Howitt. Carlton: Melbourne University Press.
Hunt, C. O., and Fiacconi, M. (2018). Pollen taphonomy of cave sediments: what does the pollen record in caves tell us about external environments and how do we assess its reliability? Quat. Int. 485, 68–75. doi: 10.1016/j.quaint.2017.05.016
Hunt, C. O., Kealhofer, L., Premathilake, R., Rushworth, G., Gilbertson, D. D., Jones, S. E., et al. (2016). “Palynology, phytoliths and diatoms in and adjacent to the Great Cave: stratigraphic and taphonomic studies of Late Quaternary vegetation history,” in The Archaeology of the Niah Caves, Sarawak: Excavations 1954-2004. McDonald Monographs in Archaeology 2, eds. G. Barker and L. R. Farr (Cambridge: McDonald Institute), 149–176.
Hunt, C. O., Pomeroy, E., Reynolds, T., Tilby, E., and Barker, G. (2023). Shanidar et ses fleurs? reflections on the palynology of the Neanderthal 'Flower Burial' hypothesis. J. Archaeol. Sci. 2023:105822. doi: 10.1016/j.jas.2023.105822
Juggins, S. (2007). C2 Version 1.5: Software for Ecological and Palaeoecological Data Analysis and Visualisation (User Guide Version 1.5). Newcastle upon Tyne: Newcastle University.
Kerle, J. A. (1984). “Variation in the ecology of Trichosurus: its adaptive significance,” in Possums and Gliders, eds. A. P. Smith and I. D. Hume (Chipping Norton: Surrey Beatty & Sons), 115–128.
Kershaw, A. P., McKenzie, G. M., Porch, N., Roberts, R. G., Brown, J., Heijnis, H., and Orr, M. (2007). A high-resolution record of vegetation and climate through the last glacial cycle from Caledonia Fen, southeastern highlands of Australia. J. Quat. Sci. 22, 801–815. doi: 10.1002/jqs.1127
Krautblatter, M., and Moser, M. (2009). A nonlinear model coupling rockfall and rainfall intensity based on a four year measurement in a high Alpine rock wall (Reintal, German Alps). Nat. Hazard. Earth Syst. Sci. 9, 1425–1432. doi: 10.5194/nhess-9-1425-2009
Leroi-Gourhan, A. (1975). The flowers found with Shanidar IV, a Neanderthal Burial in Iraq. Science 190, 562–564. doi: 10.1126/science.190.4214.562
Lewis, W. C. (1991). Atmospheric pressure changes and cave airflow: a review. Natl. Speleol. Soc. Bullet. 53, 1–12.
Lindenmayer, D. B., MacGregor, C., Welsh, A., Donnelly, C. F., and Brown, D. (2008). The use of hollows and dreys by the common ringtail possum (Pseudocheirus peregrinus) in different vegetation types. Austral. J. Zool. 56, 1–11. doi: 10.1071/ZO08054
Lismonde, B. (2002). Climatologie du Monde Souterrain. Grenoble: Éditions du Comité Départementale de Spéléologie de l'Isère.
Mallol, C., Hernandez, C. M., Cabanes, D., Machado, J., Sistiaga, A., Perez, L., et al. (2013). Human actions performed on simple combustion structures: an experimental approach to the study of Middle Palaeolithic fire. Quat. Int. 315, 3–15. doi: 10.1016/j.quaint.2013.04.009
Marsh, K. J., Foley, W. J., Cowling, A., and Wallis, I. R. (2003). Differential susceptibility to Eucalyptus secondary compounds explains feeding by the common ringtail (Pseudocheirus peregrinus) and common brushtail possum (Trichosurus vulpecula). J. Compar. Physiol. B 173, 69–78. doi: 10.1007/s00360-002-0318-4
Martin, M. D., Chamecki, M., Brush, G. S., Meneveau, C., and Parlange, M. B. (2009). Pollen clumping and wind dispersal in an invasive angiosperm. Am. J. Bot. 96, 1703–1711. doi: 10.3732/ajb.0800407
McDonough, K. N. (2019). Middle Holocene menus: dietary reconstruction from coprolites at the Connley Caves, Oregon, USA. Archaeol. Anthropol. Sci. 11, 5963–5982. doi: 10.1007/s12520-019-00828-1
McDowell, M. C., David, B., Mullett, R., Fresløv, J., GunaiKurnai, L., Waters Aboriginal Corporation, et al. (2022). Interpreting the mammal deposits of Cloggs Cave (SE Australia), GunaiKurnai Aboriginal Country, through community-led partnership research. People Nat. 4, 1629–1643. doi: 10.1002/pan3.10410
McGarry, S. F., and Caseldine, C. (2004). Speleothem palynology: an undervalued tool in Quaternary studies. Quat. Sci. Rev. 23, 2389–2404. doi: 10.1016/j.quascirev.2004.06.007
McKay, G. M., and Winter, J. W. (1989). “Phalangeridae,” in Fauna of Australia: Mammalia, eds. D. W. Walton and B. J. Richardson (Canberra, ACT: Australian Government Publishing Service), 636–651.
Mercuri, A. M. (2008). Human influence, plant landscape evolution and climate inferences from the archaeobotanical records of the Wadi Teshuinat area (Libyan Sahara). J. Arid Environ. 72, 1950–1967. doi: 10.1016/j.jaridenv.2008.04.008
Mialanes, J., David, B., Stephenson, B., Fresløv, J., Mullett, R., GunaiKurnai, L., et al. (2022). “The mulla-mullung's bulk: GunaiKurnai perspectives on the stone artefacts of Cloggs Cave, GunaiKurnai Country, southeastern Australia,” in The Oxford Handbook of Global Indigenous Archaeologies, eds. C. Smith, J. Watkins, A. Kumar Kanungo, K. Pollard, S. Varela Lopez, and S. May (Oxford: Oxford University Press), 37.
Mullett, R., David, B., and Symonds, M. (2021). Cloggs Cave: A Temporary Hunting Camp or Secluded Retreat and Source of Magical Supplies? Current World Archaeology, 108. Available at: https://the-past.com/feature/cloggs-cave-a-temporary-hunting-camp-or-secluded-retreat-and-source-of-magical-supplies (accessed July 30, 2024).
O'Grady, J. G., McInnes, K. L., Colberg, F., Hemer, M., and Badanin, A. V. (2015). Longshore wind, waves and currents: climate and climate projections at Ninety Mile Beach, southeastern Australia. Int. J. Climatol. 35, 4079–4093. doi: 10.1002/joc.4268
Oliver, T. S., Kennedy, D. M., Tamura, T., Murray-Wallace, C. V., Konlechner, T. M., Augustinus, P. C., et al. (2018). Interglacial-glacial climatic signatures preserved in a regressive coastal barrier, southeastern Australia. Palaeogeogr. Palaeoclimatol. Palaeoecol. 501, 124–135. doi: 10.1016/j.palaeo.2018.04.011
Packer, J., Brouwer, N., Harrington, D., Gaikwad, J., Heron, R., Elders, Y. C., et al. (2012). An ethnobotanical study of medicinal plants used by the Yaegl Aboriginal community in northern New South Wales, Australia. J. Ethnopharmacol. 139, 244–255. doi: 10.1016/j.jep.2011.11.008
Pahl, L. I. (1987). Feeding-behavior and diet of the Common Ringtail Possum, Pseudocheirus peregrinus, in Eucalyptus woodlands and Leptospermum thickets in southern Victoria. Austral. J. Zool. 35, 487–506. doi: 10.1071/ZO9870487
Pepper, P. (1980). You Are What You Make Yourself to Be: the Story of a Victorian Aboriginal Family 1842–1980. Melbourne, VIC: Hyland House.
Pepper, P., and De Araugo, T. (1985). What Did Happen to the Aborigines of Victoria: the Kurnai of Gippsland. Melbourne, VIC: Hyland House.
Petherick, L., Bostock, H., Cohen, T. J., Fitzsimmons, K., Tibby, J., Fletcher, M.-S., et al. (2013). Climatic records over the past 30 ka from temperate Australia—a synthesis from the Oz-INTIMATE workgroup. Quat. Sci. Rev. 74, 58–77. doi: 10.1016/j.quascirev.2012.12.012
Pini, R., Furlanetto, G., Castellano, L., Saliu, F., Rizzi, A., and Tramelli, A. (2018). Effects of stepped-combustion on fresh pollen grains: morphoscopic, thermogravimetric, and chemical proxies for the interpretation of archeological charred assemblages. Rev. Palaeobot. Palynol. 259, 142–158. doi: 10.1016/j.revpalbo.2018.10.005
Piperno, D. R. (2006). Phytoliths: A Comprehensive Guide for Archaeologists and Paleoecologists. Lanham: AltaMira Press (Rowman & Littlefield).
Rowe, C., O'Connor, S., and Balme, J. (2020). An examination of rockshelter palynology: Carpenter's Gap 1, northwestern Australia. Holocene 30, 1142–1154. doi: 10.1177/0959683620913921
Sengupta, S. (1975). Experimental alterations of the spores of Lycopodium clavatum as related to diagenesis. Rev. Palaeobot. Palynol. 19, 173–192. doi: 10.1016/0034-6667(75)90039-1
Shulmeister, J., Kemp, J., Fitzsimmons, K. E., and Gontz, A. (2016). Constant wind regimes during the Last Glacial Maximum and early Holocene: evidence from Little Llangothlin Lagoon, New England Tablelands, eastern Australia. Clim. Past 12, 1435–1444. doi: 10.5194/cp-12-1435-2016
Smyth, R. B. (1878). The Aborigines of Victoria, with Notes Relating to the Habits of the Natives of Other Parts of Australia and Tasmania. Melbourne, VIC: John Ferres, Government Printer.
Solecki, R. S. (1975). Shanidar IV, a Neanderthal Flower Burial in Northern Iraq. Science 190, 880–881. doi: 10.1126/science.190.4217.880
Stanley, S., and De Deckker, P. (2002). A Holocene record of allochthonous, aeolian mineral grains in an Australian alpine lake; implications for the history of climate change in southeastern Australia. J. Paleolimnol. 27, 207–219. doi: 10.1023/A:1014249404845
Stephenson, B., David, B., Fresløv, J., Arnold, L. J., GunaiKurnai, L., Waters Aboriginal Corporation, et al. (2020). 2000 Year-old Bogong moth (Agrotis infusa) Aboriginal food remains, Australia. Sci. Rep. 10:22151. doi: 10.1038/s41598-020-79307-w
Thom, B., Hesp, P., and Bryant, E. (1994). Last glacial “coastal” dunes in eastern Australia and implications for landscape stability during the Last Glacial Maximum. Palaeogeogr. Palaeoclimatol. Palaeoecol. 111, 229–248. doi: 10.1016/0031-0182(94)90065-5
Victoria State Government Department of Sustainability and Environment (2004). EVC/Bioregion Benchmark for Vegetation Quality Assessment: East Gippsland Uplands Bioregion. Available at: https://www.environment.vic.gov.au/__data/assets/pdf_file/0027/48564/EGU_EVCs_combined.pdf (accessed August 08, 2023).
Wadley, L., Sievers, C., Bamford, M., Goldberg, P., Berna, F., and Miller, C. (2011). Middle Stone Age bedding construction and settlement patterns at Sibudu, South Africa. Science 334, 1388–1391. doi: 10.1126/science.1213317
Ward, I. A., and Friesem, D. (2021). Many words for fire: an etymological and micromorphological consideration of combustion features in Indigenous archaeological sites of Western Australia. J. Royal Soc. West. Austr. 104, 11–24.
Weinstein-Evron, M. (1994). Biases in archaeological pollen assemblages: case studies from Israel. AASP Contribut. Ser. 29, 193–205.
Whitau, R., Vannieuwenhuyse, D., Dotte-Sarout, E., Balme, J., and O'Connor, S. (2018). Home is where the hearth is: anthracological and microstratigraphic analyses of Pleistocene and Holocene combustion features, Riwi Cave (Kimberley, Western Australia). J. Archaeol. Method Theory 25, 739–776. doi: 10.1007/s10816-017-9354-y
Wigley, T. M. L., and Brown, M. C. (1976). “The physics of caves,” in The Science of Speleology, eds. T. D. Ford and C. H. D. Cullingford (London: Academic Press), 239–347.
Xia, Q. K., Zhao, J. X., and Collerson, K. D. (2001). Early-Mid Holocene climatic variations in Tasmania, Australia: multi-proxy records in a stalagmite from Lynds Cave. Earth Planet. Sci. Lett. 194, 177–187. doi: 10.1016/S0012-821X(01)00541-6
Keywords: anthropic pollen, archaeobotany, cave pollen, Cloggs Cave, GunaiKurnai, pollen clumps, pollen taphonomy
Citation: Grono E, David B, Stevenson J, Fresløv J, Mullett R, Keaney B, Graham C, Ash J, GunaiKurnai Land and Waters Aboriginal Corporation, McDowell MC, Petchey F, Delannoy J-J, Rogers AJ and Kennedy DM (2024) Cloggs Cave pollen sequences, GunaiKurnai Country, East Gippsland (SE Australia): 25,000 years of cultural plant use and changing environments. Front. Environ. Archaeol. 3:1488477. doi: 10.3389/fearc.2024.1488477
Received: 30 August 2024; Accepted: 11 November 2024;
Published: 05 December 2024.
Edited by:
Alison Crowther, The University of Queensland, AustraliaReviewed by:
Ian Moffat, Flinders University, AustraliaMelanie Fillios, University of New England, Australia
Copyright © 2024 Grono, David, Stevenson, Fresløv, Mullett, Keaney, Graham, Ash, GunaiKurnai Land and Waters Aboriginal Corporation, McDowell, Petchey, Delannoy, Rogers and Kennedy. This is an open-access article distributed under the terms of the Creative Commons Attribution License (CC BY). The use, distribution or reproduction in other forums is permitted, provided the original author(s) and the copyright owner(s) are credited and that the original publication in this journal is cited, in accordance with accepted academic practice. No use, distribution or reproduction is permitted which does not comply with these terms.
*Correspondence: Elle Grono, ZWxsZS5ncm9ub0BhbnUuZWR1LmF1
†ORCID: Elle Grono orcid.org/0000-0002-3607-6686
Bruno David orcid.org/0000-0002-8567-6135
Janelle Stevenson orcid.org/0000-0001-9640-7275
Jeremy Ash orcid.org/0000-0003-0306-6307
Matthew C. McDowell orcid.org/0000-0001-8009-4171
Fiona Petchey orcid.org/0000-0002-3308-9983
Jean-Jacques Delannoy orcid.org/0000-0002-2815-4920
Ashleigh J. Rogers orcid.org/0000-0003-0455-9381
David M. Kennedy orcid.org/0000-0002-4878-7717