- 1Department of Archaeology, Max Planck Institute of Geoanthropology, Jena, Germany
- 2Institute of Oriental Studies, Indo-European Studies, Prehistoric and Early Historical Archaeology, Friedrich Schiller University, Jena, Germany
- 3Department of Archaeology, University of Aberdeen, Aberdeen, United Kingdom
- 4Institute for Prehistory and Early History, Friedrich-Alexander-University Erlangen-Nürnberg, Erlangen, Germany
- 5Department of Botany, University Museum of Norway, Tromsø, Norway
- 6Department of Geosciences, University of Cape Town, Cape Town, South Africa
- 7isoTROPIC Research Group, Max Planck Institute of Geoanthropology, Jena, Germany
- 8Institute of Prehistoric Archaeology, University of Cologne, Cologne, Germany
The application of high-resolution methods to reconstruct the ecology and behavior of large-herbivores within Late Pleistocene contexts in Europe has revealed increasing evidence for variability in diet, habitat preference, ranges and mobility patterns through time and space. This data has major implications for interpretations of hominin subsistence strategies in terms of prey-species selection, which rest to a large extent, on the spatial ecology of these animals and their resulting availability in the environment. To this end, multi-isotope analysis of faunal remains from archaeological sites has been shown to provide direct information in herbivore movements and ranges that move beyond assumptions of consistency in animal behavior through time. The Middle Paleolithic site of Salzgitter-Lebenstedt, Northern Germany, has been put forward as a prime example of specialized hunting of a single taxon – reindeer – by Neanderthals. However, questions remain around the number and season of hunting events. Here we employ strontium (87Sr/86Sr) isotope analysis in combination with stable oxygen (δ18O) and carbon (δ13C) isotope analysis of sequentially-sampled tooth enamel from reindeer (Rangifer tarandus) and horse (Equus sp.) to reconstruct the sub-annual dietary and ranging behaviors of these prey-species at Salzgitter. We find that reindeer exhibit parallel seasonal shifts in diet and likely experienced similar environmental conditions. While the majority of the reindeer display the potential for long-distance climate-induced migrations, mobility patterns and seasonal ranges appear to have differed between individuals. This may be indicative of behavioral flexibility, particularly in migratory behavior of reindeer, during this period in Northern Germany. Horses analyzed here likely consumed a graze-based diet year-round, while potentially undertaking more residential movements on a seasonal basis. We briefly discuss potential implications of these findings on Neanderthal hunting strategies at the site. Finally, we discuss the challenges to the application of 87Sr/86Sr as a provenancing tool in Central Europe, and the importance of multi-isotope approaches and development of additional spatial proxies.
1 Introduction
Increasingly high-resolution analyses of archaeofaunal remains have demonstrated the potential for variation and plasticity in large-herbivore behavior during the Late Pleistocene in Europe, including those species exploited by hominin groups (Britton et al., 2023a,b; Rivals and Lister, 2016; Rivals and Semprebon, 2017, 2011; Rivals and Solounias, 2007). This is significant as interpretations of subsistence strategies and adaptations of different hominins by archaeologists rest, in a large part, on the behavior of their prey (see Grayson and Delpech, 2002; Rendu, 2022 for discussion). In Europe, there exists a long-running debate framing opportunistic vs. selective hunting strategies for Neanderthals and Homo sapiens, respectively, with associated implications for cognitive ability and cultural and social complexity (e.g., Grayson and Delpech, 2002; Mellars, 1989, 1973, 2004; Straus, 1992). However, growing evidence for flexible and diverse subsistence strategies associated with Neanderthals, including single-taxon assemblages indicative of selective hunting, have problematized this dichotomy (Costamagno et al., 2006; Daujeard et al., 2019; Gaudzinski-Windheuser and Niven, 2009; Rendu, 2022; Rendu et al., 2012). Here, it is becoming increasingly critical to obtain direct insights into the behavior of particular prey species on a site-specific basis and in relation to specific ecological and environmental conditions in order to establish spatio-temporal distribution and availability of these animals in the local environment (Britton et al., 2023a, 2011; Burke and Pike-Tay, 1997; Julien et al., 2015). This is particularly important given the ongoing discussion as to whether animal behavior observed in the present is directly applicable into the past (Bocherens, 2003; Britton et al., 2023a; Guthrie, 2001; Parmesan, 2006).
Two important species in this regard are reindeer (Rangifer tarandus) and horses (Equus sp.). Both of which were heavily exploited by both Neanderthals and H. sapiens in Central Europe and whose ranging habits, and mobility patterns, and their variability through time, continue to be debated (e.g., Fontana, 2022, 2017; Grayson and Delpech, 2002; Kuntz and Costamagno, 2011). Interpretations of Late Pleistocene large-herbivore spatial ecology and biogeography within European contexts include hypotheses of both long-distance migratory reindeer (Bahn, 1977; Gordon, 1988; Lacorre, 1956) and non-migratory populations in Southern and Central France (Britton et al., 2023a; Fontana, 2017, 2012, 2000), to east-west migrations across the North European Plain (NEP) (Price et al., 2017) and smaller sale north-south movements between the Central Upland Zone and the NEP (Baales, 2021, 1999, 1996). Various lines of evidence including toothwear analyses (Rivals et al., 2009; Rivals and Semprebon, 2017), ecomorphometrics (López et al., 2022), osteometry (Weinstock, 2002), as well as isotope analyses (Britton et al., 2023a,b, 2011; Pryor et al., 2016a) point to spatio-temporal plasticity in ranging behaviors, migration patterns, as well as diet and habitat use. While relatively little data exists on the mobility patterns and biogeography of horses during this time evidence suggests European populations occupied relatively local ranges and were not wide-ranging (Britton et al., 2023a; Pederzani et al., 2021a; Pellegrini et al., 2008; Van Asperen, 2010). Late Pleistocene equids were primarily grazers (Bocherens, 2015, 2003; Britton et al., 2023b, 2012; Kovács et al., 2012; Rivals et al., 2015; Schwartz-Narbonne et al., 2019), although there is some evidence for plasticity in feeding behaviors through time (Bocherens et al., 1999; Pushkina et al., 2014; Rivals et al., 2009; Saarinen et al., 2016).
Given the potential variability in the ecology of these important prey species (Britton et al., 2023a; Pushkina et al., 2014; Rivals et al., 2008), it is essential to undertake site-specific analyses. Salzgitter-Lebenstedt is a Middle Paleolithic open-air site located ~50 km southeast of Hannover in Northern Germany (Figure 1). Archaeological excavations undertaken in 1952 (Tode et al., 1953) and again in 1977 (Grote and Preul, 1978) resulted in the recovery of about ~8,400 large mammal remains, dominated by R. tarandus (Gaudzinski, 2000, 1998; Gaudzinski and Roebroeks, 2000; Kleinschmidt, 1953; Krönneck and Staesche, 2017), ~4,200 of Middle Paleolithic stone artifacts (Grote and Preul, 1978; Pastoors, 1996, 2001, 2009), as well as Neanderthal remains (n = 5) (Gaudzinski, 1998; Hublin, 1984). Efforts to date the period of hominin occupation at Salzgitter are ongoing, and have been linked to an interstadial during a glacial period between Marine isotope Stage (MIS) 6 and MIS 3 (Bosinski, 1963; Pastoors, 2009, 2001; Pfaffenberg, 1991; Preul, 2017, 1991; Selle, 1991). Zooarchaeological evidence for hunting by Neanderthals at Salzgitter is indicative of specialized hunting of whole herds during autumn migration, and the processing of marrow-rich elements of adult individuals (Gaudzinski, 2000, 1999, 1998; Gaudzinski and Roebroeks, 2000). Despite certain aspects of this hypothesis being challenged (Munson and Marean, 2003), the site has remained a key example of specialized hunting of a single taxon by Neanderthals in Central Europe (Daujeard et al., 2019; Gaudzinski-Windheuser, 2021; Gaudzinski-Windheuser and Niven, 2009; Rendu, 2022; Rendu et al., 2012; White et al., 2016). However, recent evidence for winter (Kierdorf and Witzel, 2017) and spring (Staesche, 2017) season-of-death from the Salzgitter reindeer suggest individuals were present in the site vicinity at different times of the year. This raises questions around reindeer herd composition and the frequency of this species in the local environment, potentially challenging current interpretations of Neanderthal hunting strategy and seasonality at Salzgitter and highlighting the need to reconstruct seasonal mobility patterns and ranges of prey taxa at the site.
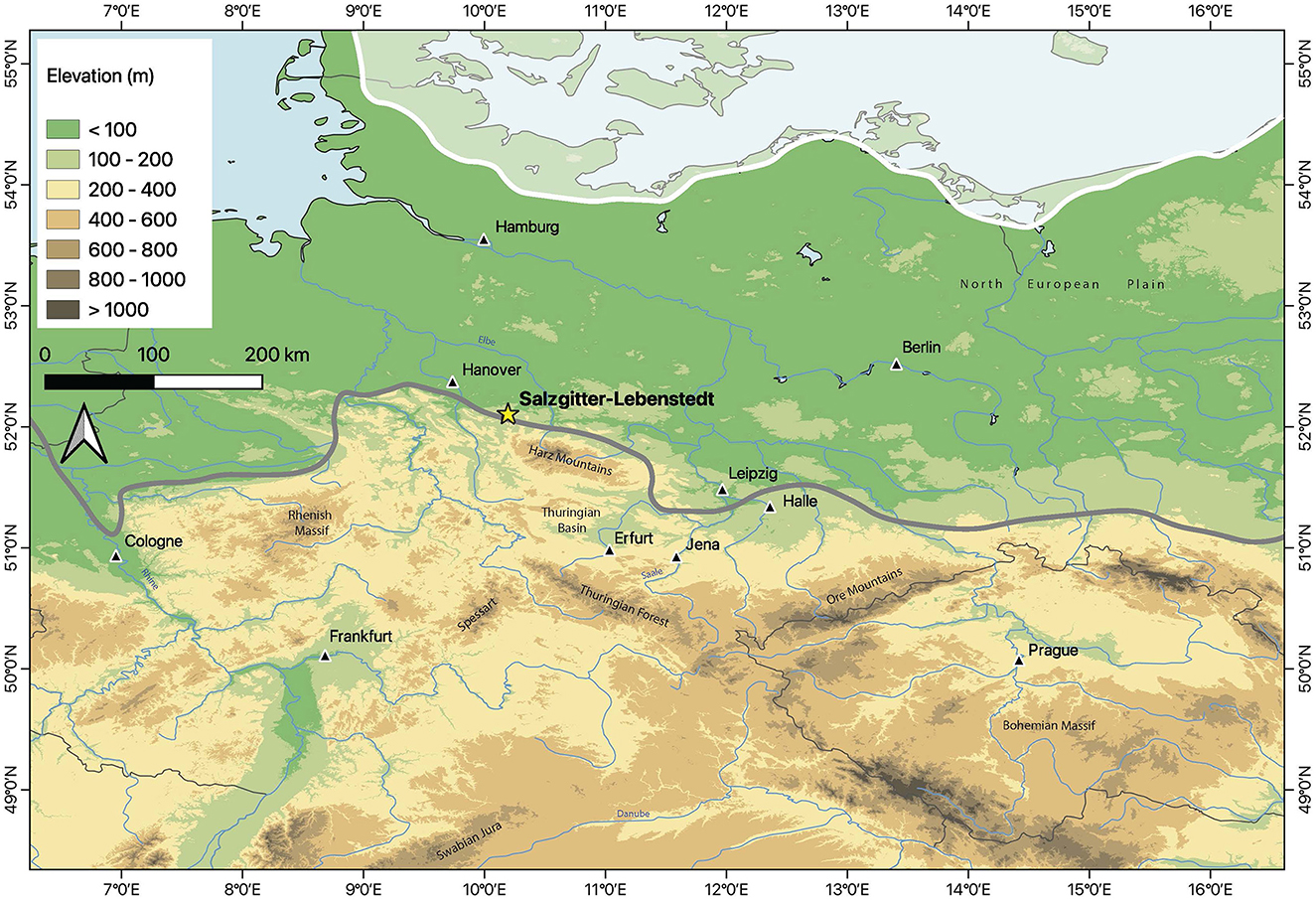
Figure 1. Elevation map of the study area, Central Europe, and location of Salzgitter-Lebenstedt (52°10′26″N, 10°19′42″E). Elevation map created using ETOPO1 data (Amante and Eakins, 2009). The location of the site is denoted by the yellow star, major cities are shown by black triangles. The approximate maximum southern extents of the Fennoscandian ice sheet advances into the study region between MIS 6 (gray line) and the end of MIS 3/MIS 2 (black line) (spanning the potential periods of site occupation based on different age models for Neanderthal presence at Salzgitter-Lebenstedt) (Batchelor et al., 2019; Eissmann, 2002; Lang et al., 2018; Toucanne et al., 2023).
Here, we utilize strontium isotope (87Sr/86Sr) analysis alongside stable oxygen (δ18Ocarbonate) and stable carbon (δ13Ccarbonate) isotope analysis of sequentially-sampled tooth enamel from Rangifer (n = 8) and Equus (n = 3) from the Lebenstedt I faunal assemblage from Salzgitter. Using this data our objective is to reconstruct the seasonal mobility patterns and biogeography of these taxa. Previous studies have demonstrated the efficacy of a combined isotopic approach to identifying large herbivore eco-ethology during the European Late Pleistocene (e.g., Britton et al., 2023a, 2011; Heddell-Stevens et al., 2024; Julien et al., 2012; Pellegrini et al., 2008). Large-herbivore enamel δ18O values predominantly reflect those of ingested environmental water derived from local precipitation and can therefore be used to identify seasonality in tooth enamel mineralization (e.g., Balasse, 2002; Barakat et al., 2023; Britton et al., 2009; Stevens et al., 2011). At temperate mid- to high-latitudes, higher δ18O values in enamel are typically indicative of the summer months, while lower δ18O values reflect winter conditions, due to the temperature effect in δ18Oprecipitation (Dansgaard, 1964; Kohn and Welker, 2005; Rozanski et al., 2013). As such, oxygen isotope data can ‘anchor' δ13C and 87Sr/86Sr isotope values within a seasonal framework (Britton, 2018; Britton et al., 2009; Hoppe et al., 2004; Pryor et al., 2024). Enamel δ13C values of herbivores predominantly reflect the type of plants consumed and their growing environment as a result of isotopic differences due to differences in photosynthetic pathway, light intensity, water availability and CO2 recycling among other factors (DeNiro and Epstein, 1978; Drucker et al., 2008; Tieszen, 1991; van der Merwe and Medina, 1991), allowing for the identification of seasonal shifts in diet and habitat preference (Julien et al., 2015; Pellegrini et al., 2008; Pryor et al., 2016a,b). Enamel 87Sr/86Sr values, which reflect local lithology of animal ranges during enamel mineralization, can be compared to regional bioavailable 87Sr/86Sr isoscapes to identify spatial distribution and movement patterns (Barakat et al., 2023; Britton et al., 2023a; Heddell-Stevens et al., 2024). Furthermore, by integrating the isotope data with the modeled isoscape and spatial assignment tools (Ma et al., 2020), we identified the probable winter and summer range locations for each animal (Ma et al., 2020). The multi-isotope data and geographic assignment of seasonal ranges undertaken here provide new insights into the spatiotemporal distribution of the two primary prey species from Salzgitter.
2 Reindeer and horse mobility patterns and Neanderthal hunting strategies in Central Europe
The existence and nature of seasonal migrations by reindeer during the Late Pleistocene has been long debated in the literature (see Fontana, 2017 and López et al., 2022 for overviews), and increasingly high-resolution data continue to complexify these discussions (Britton et al., 2023a; Price et al., 2017). Longstanding hypotheses include long distance north-south mobility through Central France (e.g., Bahn, 1977; Gordon, 1988; Lacorre, 1956) as well as smaller-scale east-west movements across southwest France (Burke and Pike-Tay, 1997; Delpech, 1987, 1983; Kuntz and Costamagno, 2011). Similar evidence for east-west seasonal movements across the NEP have also been identified in reindeer in Northern Germany during the Final Upper Paleolithic (Price et al., 2017). Lastly, arguments have been made for populations living locally year-round from Middle Paleolithic contexts in Southern France (Britton et al., 2023a; Fontana, 2017, 2012, 2000). This is supported by recent isotopic evidence for a diachronic shift from migratory to sedentary ecotypes at Abri du Maras, Southwest France (Britton et al., 2023a). Further evidence for plasticity in mobility patterns can be seen in the possible extension of Rangifer range size between Middle-Upper Paleolithic layers at Les Cottés (Britton et al., 2023a). This behavioral plasticity is highlighted by generalist feeding strategies of reindeer identified within Western and Central European contexts during the Late Pleistocene where individuals appear to have consumed a variety of plants year-round with lichens likely forming a significant component of the diet, particularly during winter (Bocherens, 2003; Britton et al., 2023b; Drucker et al., 2012, 2003; Fizet et al., 1995; Schwartz-Narbonne et al., 2019).
While there is a general absence of in-depth studies of equid spatial ecology during the Late Pleistocene in Europe, ecomorphological and isotope data indicate that populations most likely did not undertake long distance migrations (Britton et al., 2023a; Pederzani et al., 2024, 2021b; Pellegrini et al., 2008; Van Asperen, 2010). Although these horses appear to have occupied relatively small home ranges, potential for seasonal movements between territories has been identified during the UP in Poland (Pryor et al., 2016b). Evidence suggests that Late Pleistocene horses in Western and Central Europe were primarily grazers (Bocherens, 2015, 2003; Britton et al., 2023a; Kovács et al., 2012; Schwartz-Narbonne et al., 2019) displaying minimal inter-annual shifts in diet (Pryor et al., 2016b). However, individuals found in more northern locations appear to have consumed relatively higher amounts of browse (Pushkina et al., 2014; Rivals et al., 2009; Saarinen et al., 2016) suggestive of plasticity in feeding behavior traits.
While Neanderthals have long been established as capable hunters, research continues to reveal complexity in Neanderthal hunting strategies, demonstrating adaptation to different environmental and ecological conditions suggestive of flexibility in subsistence strategies (e.g., Blasco et al., 2022; Daujeard et al., 2019; Discamps et al., 2011; Moncel et al., 2021; Zilhão et al., 2020). Neanderthal subsistence practices have received close attention particularly as they relate to broader questions around the interplay of biological, cultural and environmental changes during the Middle to Upper Paleolithic cultural transition in Europe, and the subsequent decline of Neanderthal populations (Delagnes and Rendu, 2011; Grayson and Delpech, 2002; Mellars, 2004; Rendu, 2010; Rendu et al., 2012). However, the degree to which prey selection by hominins is an adaptation to the availability of different taxa in the local environment is still debated (Costamagno et al., 2006; Discamps et al., 2011; Grayson and Delpech, 2002; Mellars, 2004; Patou-Mathis, 2000; Rendu et al., 2012). For example, faunal assemblages dominated by a single taxon have been argued to reflect deliberate selection by hominin hunters from among a broader array of species available in the environment, often at a certain time of year (Daujeard et al., 2019; Gaudzinski, 2005; Gaudzinski and Roebroeks, 2000; Gaudzinski-Windheuser and Niven, 2009; Mellars, 2004; Niven et al., 2012). However, more often than not, the spatio-temporal distribution of large herbivore prey taxa in local the environment is assumed based on the modern-day ecological characteristics of a species, ignoring the potential for plasticity in past populations, and the presence of non-analog environments during the Late Pleistocene in Central Europe (Britton, 2018). As a result, questions remain around interpretations of specialized hunting and the significance of monospecific assemblages as an indicator of hominin cognitive and social capacities (see Rendu, 2022 for an overview).
3 Reconstructing faunal spatial paleoecology using multi-isotope analyses
Multi-isotope analysis of faunal remains from archaeological sites is an established method of reconstructing past animal ecology and seasonality directly from the remains themselves (e.g. Britton et al., 2011; Hoppe et al., 2004; Julien et al., 2012; Price et al., 2017). Tooth enamel is particularly applicable as it can preserve over millions of years (e.g., Lee-Thorp et al., 2010; Sponheimer and Lee-Thorp, 1999) and forms incrementally, providing time-series data corresponding to the period of enamel formation (Balasse, 2002; Bendrey et al., 2015; Hoppe et al., 2004; Passey and Cerling, 2002). Utilized in conjunction, intra-tooth δ18O, δ13C and 87Sr/86Sr data can reveal seasonal changes in diet related to movement between habitats and regions (e.g., Julien et al., 2012; Pellegrini et al., 2008; Pryor et al., 2016b). Together, these proxies have the ability to provide multi-scale insights into the seasonal mobility patterns of herbivore prey taxa.
At northern hemisphere mid- to high-latitudes, δ18O values of precipitation vary in relation to a number of factors including continentality, altitude, moisture source and transportation, rainfall amount and the temperature effect (see Pederzani and Britton, 2019 for an overview). The temperature effect describes the close relationship observed between air temperature and δ18O values in precipitation, resulting in a trend toward decreasing δ18Oprecipitation with colder temperatures and increasing δ18Oprecipitation with warmer temperatures (Dansgaard, 1964; Fricke and O'Neil, 1999; Gat, 1980; Kohn and Welker, 2005; Stumpp et al., 2014). As a result, seasonal temperature variation is typically reflected in δ18O values of precipitation-fed surface waters including rivers and lakes (Gat, 2010, 1995; Rozanski, 1985). However, de-coupling of δ18Osurfacewater from δ18Oprecipitation can occur in groundwaters, as well as in larger lakes and rivers, due to the effects of water residence time, transportation and evaporation (Gat, 2010; Gonfiantini, 1986; Jeelani et al., 2017; Rozanski, 1985). The δ18O values of large-herbivore tooth enamel are predominantly derived from those of ingested water during enamel mineralization, and appear as a semi-sinusoidal curve along the axis of growth (Hoppe et al., 2004). In obligate drinkers like horses, δ18Oenamel predominantly reflects their drinking water sources (Bryant and Froelich, 1995; Hoppe et al., 2004; Kohn, 1996; Kohn et al., 1996; Longinelli, 1984). As such, deviations from the expected seasonal amplitude in intra-tooth δ18O are likely related to one or more of the factors discussed above. Non-obligate drinkers, including reindeer, obtain a larger proportion of their ingested water from plant leafwater (Cain et al., 2006; Levin et al., 2006). The δ18O values of leafwater are closely correlated with those of the plant source water, which generally derives from local precipitation (Bowen and Good, 2015; Cernusak et al., 2016). However, plant δ18Oleafwater is typically higher than local δ18Oprecipitation due to enrichment of 18O during evapotranspiration processes in the leaves (Cernusak et al., 2016; Flanagan et al., 1991; Levin et al., 2006; Merlivat, 1978). In temperate environments, the evapotranspiration rates increase in C3 plants during the drier summer months, causing elevated leafwater δ18O values and amplifying seasonal variation (Dongmann et al., 1974; Gonfiantini et al., 1965). Consequently, while δ18Oenamel of non-obligate drinkers can reflect seasonal shifts in environmental water, these values are typically enriched relative to those of obligate drinkers, displaying greater intra-tooth amplitude (Kohn et al., 1996; Sponheimer and Lee-Thorp, 1999). In migratory animals, particularly those that undertake seasonal movements to avoid climatic extremes, seasonal amplitude in intra-tooth δ18O values may be reduced in comparison to that of local precipitation (Britton et al., 2009).
Herbivore enamel stable carbon isotope (δ13C) values reflect the dietary contributions of different plants during the period of enamel formation (Cerling and Harris, 1999; DeNiro and Epstein, 1978; Vogel, 1978). Enamel bioapatite δ13C values of large-herbivore are enriched in 13C by a consistent +14.1 ± 0.5‰ compared to consumed plants (Cerling and Harris, 1999). In C3 plants, δ13C values typically range from−22‰ to−35‰ (Ehleringer and Monson, 1993; Farquhar et al., 1989a; O'Leary, 1988). In plants that follow the C3 photosynthetic pathway, including those that dominated Central European environments during the Late Pleistocene, δ13C values reflect light intensity, nutrient and water availability, as well as recycling of CO2 in closed-canopy forests (Ehleringer and Monson, 1993; Farquhar et al., 1989a; Feranec and MacFadden, 2006; O'Leary, 1988). C3 plants growing in forest and woodlands exhibit lower δ13C values than those in open grasslands (Heaton, 1999; Tieszen, 1991). Isotopic variation also exists between different types of plants, for example lichens generally have higher δ13C values relative to vascular plants in the same ecosystem (e.g., Máguas and Brugnoli, 1996; Park and Epstein, 1960; Teeri, 1981). In C3 environments, herbivores consuming predominantly grasses or large amounts of lichen exhibit more positive δ13C values (>c.-9‰) which decrease with increased consumption of forage from more closed, i.e. woodland environments (typically < -12‰) (Bocherens, 2003). Mixed feeders are predicted to display intermediate values (c.−9‰ and −12‰) (Bocherens, 2003). Isotopic differences between plants can therefore be used to identify dietary preferences, including seasonal shifts, as well as resource partitioning among herbivore groups (e.g. Bocherens, 2003; Bocherens et al., 1997; Cerling and Harris, 1999; Drucker et al., 2003; Iacumin et al., 2000). While C3 plants may exhibit seasonal shifts in δ13C values due to water stress, for example (Farquhar et al., 1989b; Mook et al., 1983), these tend to be relatively small compared to isotopic differences between different types of plants and those growing in different environments (Bocherens, 2003).
Strontium isotope (87Sr/86Sr) analysis of tooth enamel bioapatite has been increasingly employed in mobility studies of modern and ancient terrestrial animals (Barakat et al., 2023; Britton et al., 2023a, 2011, 2009; Hoppe et al., 1999; Price et al., 2017). Bioavailable strontium, strontium that enters the food chain, is predominantly derived from underlying bedrock. Bedrock 87Sr/86Sr ratios are largely determined by the age of the rock, initial amount of 87Rb, mineral content, and weathering rates (Capo et al., 1998; Montgomery, 2010; Price et al., 2002). Strontium weathered into soil is taken up by plants and subsequently incorporated into herbivore tooth enamel via consumed forage (Bentley, 2006; Capo et al., 1998). Enamel 87Sr/86Sr ratios therefore predominantly reflect the values of local lithologies occupied during enamel formation, with minimal fractionation between diet and consumer, and may be used as a mobility proxy (Capo et al., 1998; Price et al., 2002). One of the challenges in utilizing this method particularly in Northern Central Europe, is the movement of sediment due to water, wind, and glacier action (Lehmkuhl et al., 2021) across potentially large areas resulting in bioavailable 87Sr/86Sr values derived from the local bedrock and to some extent from these imported sediments (Holt et al., 2021; Price et al., 2002). This has necessitated the development of 87Sr/86Sr isoscapes that model the spatial distribution of bioavailable 87Sr/86Sr across a landscape (e.g., Bataille et al., 2020, 2018; Evans et al., 2010; Kootker et al., 2016; Willmes et al., 2018). A growing number of different models are being used in this pursuit including statistical and mechanistic models that can incorporate empirical data as well as a range of variables known to influence strontium isotope values locally (see Holt et al., 2021 for a review). Here, machine learning with a random forest regression algorithm have demonstrated the ability to produce accurate results, which include multiple influential variables, across large areas (Bataille et al., 2020, 2018; Holt et al., 2021). By comparing 87Sr/86Sr values in herbivore enamel with the spatial distribution of bioavailable strontium, it is possible to identify local and non-local individuals, as well as seasonal ranges and mobility patterns of animals in the past (Bentley, 2006; Capo et al., 1998). Furthermore, the inclusion of ecological spatial assignment tools into archaeological analyses has enabled statistical approaches to identifying locations of these ranges (e.g., Barakat et al., 2023; Britton et al., 2023a; Le Corre et al., 2023; Ma et al., 2020).
4 Site background and faunal assemblage
The site of Salzgitter-Lebenstedt, Lower Saxony, Germany (52°10′26″N, 10°19′42″E) is located on the northern slope of the Krähenriede stream valley, close to its convergence with the Fuhse river valley (Figure 1). An area of ca. 150 m2 was excavated in 1952 (Lebenstedt I) (Tode et al., 1953) and in 1977 (Lebenstedt II) in an adjacent area of 220 m2 to the west (Figure 2) (Grote and Preul, 1978). The sample material for this study comes from the Lebenstedt I faunal assemblage. The Middle Paleolithic find horizon comprises fluvial gravel, sand and silt deposits < 2 m in thickness ~4–7 m below the current surface (Grote and Preul, 1978; Preul, 2017, 1991; Tode et al., 1953)(Grote and Preul, 1978; Preul, 2017, 1991; Tode et al., 1953), with a 30–60 cm thick core horizon consisting of the majority of finds (Pastoors, 2001, 1996). Complex depositional conditions characterize the site, at Lebenstedt I lithic and faunal material were concentrated in two backwater ponds (Gaudzinski and Roebroeks, 2000; Preul, 2017, 1991; Tode et al., 1953), while at Lebenstedt II, the finds were distributed throughout the excavation area with some higher concentrations discovered within palaeochannels in the southern part (Ludowici and Pöppelmann, 2017). The composition of the archaeological horizon, consisting of various sandy sediments and gravels, with humic peat lenses, deposited during smaller- and larger-scale events, has complicated efforts to determine specific periods of occupation and number of hunting events at the site. Excavation techniques and documentation of the 1950s and 1970s, although modern for their time, cannot do justice to the complex natural depositional conditions described. The challenge for Salzgitter is therefore to combine episodes identified in the faunal and lithic analysis at the microscale level (Gaudzinski and Roebroeks, 2000; Pastoors, 2009, 2001; Preul, 1991; Tode, 1982) into larger depositional events and occupation phases that ultimately subdivide the entire Salzgitter assemblage and to integrate them into the natural processes of sedimentation.
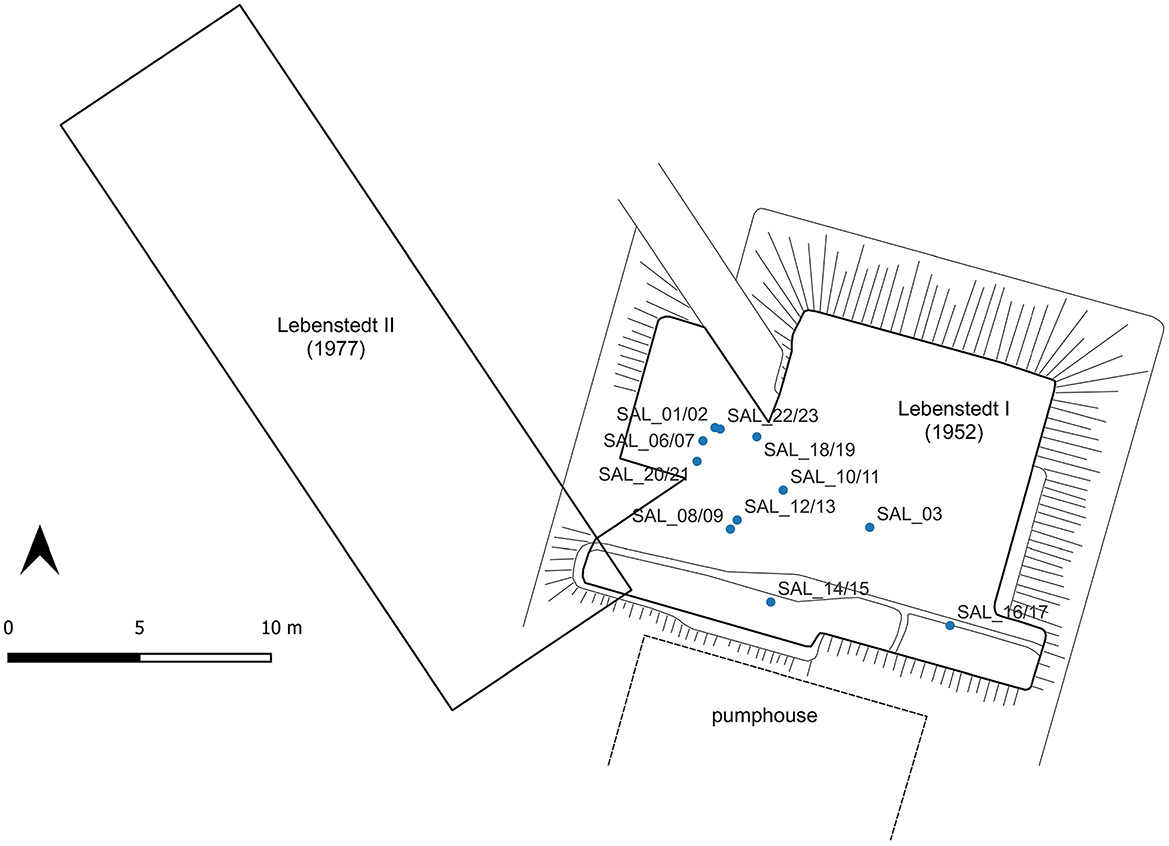
Figure 2. Relative location of the two excavation areas, Lebenstedt I (1952, excavations) and Lebenstedt II (1977 excavations) at Salzgitter-Lebenstedt and the spatial distribution of the specimens sampled in this study (Grote and Preul, 1978; Kleinschmidt, 1953; Pastoors, 2001, 1996; Preul, 1991).
The good preservation of the faunal material and the sharp edges of the stone tools (Pastoors, 2009, 2001) suggest only minor post-depositional disturbance despite some local freeze-thaw action. As such, the finds have been treated as a single assemblage by researchers (Gaudzinski, 1998; Gaudzinski and Roebroeks, 2000; Pastoors, 2009, 2001; Ruebens et al., 2023; Tode, 1982). The lithic assemblage has been attributed to the Weichselian Keilmessergruppen industry (Jöris, 2004; Jöris et al., 2022; Pastoors, 2009, 2001; Richter, 1997). Notable material culture finds from the site include a number of mammoth rib bones interpreted as having been modified by Neanderthals for use in stone tool manufacture (Gaudzinski, 1999; Tode, 1982; Tode et al., 1953). Age models based on palaeoecological material from the find horizon attribute Neanderthal occupation at Salzgitter to an interstadial between MIS 6 to MIS 3 (Bosinski, 1963; Pastoors, 2009, 2001; Pfaffenberg, 1991; Preul, 2017, 1991; Selle, 1991). Direct dating of archaeological material has produced a range of dates between 76 and 33 ka (Pastoors, 2001; Preul, 2017; Ruebens et al., 2023; Vogel and Zagwijn, 1967). Organic material from the Middle Paleolithic find horizon is indicative of a tree-less shrub tundra environment during this period (Schütrumpf, 1991; Selle, 1991), and relatively dry climate conditions (Stephan, 2017) while July temperatures during this time in Northern Germany were likely below ~10°C (Caspers and Freund, 2001).
The Lebenstedt I and II faunal assemblages combined consist of ~8,400 large mammal bones including a large number of identifiable skeletal elements (Supplementary Table 1) (Gaudzinski, 1998; Kleinschmidt, 1953; for an overview see Gaudzinski-Windheuser, 2021). Reindeer skeletal material dominates the assemblage and at least 86 individuals have been identified, while ~6% of the skeletal material exhibits bone surface modifications including cutmarks and evidence for the splitting of long bones (Supplementary Table 2) (Gaudzinski, 1998; Gaudzinski and Roebroeks, 2000; Kleinschmidt, 1953). A variety of other species, including horse (Equus sp.) are present in smaller numbers and also displaying evidence of anthropogenic accumulation (Gaudzinski, 1998; Gaudzinski and Roebroeks, 2000). A different taphonomic history has been identified for the reindeer material compared to other taxa in the assemblage, namely better preservation and similar patina (Gaudzinski and Roebroeks, 2000; Ruebens et al., 2023), and it has been suggested that these remains were deposited within a short time period (Gaudzinski, 1999; Gaudzinski and Roebroeks, 2000). Based on stages of tooth eruption, epiphyseal fusion, and antler growth Gaudzinski and Roebroeks (2000) identifed a catastrophic mortality profile with the majority of animals having died between late summer and autumn. Skeletal element representation indicates the presence of whole carcasses in most cases, suggesting these animals died or were killed close to the site. Cutmarks and percussion marks found on reindeer bones (Supplementary Table 2) have been interpreted as the targeted processing of marrow-rich elements from adults between 8 and 9 years of age by Neanderthals (Gaudzinski, 1999, 1998; Gaudzinski and Roebroeks, 2000). To date, ecological reconstructions of prey taxa at Salzgitter comprise dental microwear analysis of R. tarandus teeth reflect a broad range of browsing behavior, while meso- and microwear patterns of the Equus sp. individuals are indicative of long-term grazing and seasonal mixed feeding behavior (Rivals et al., 2009; Rivals and Solounias, 2007). Bison were also identified as exhibiting mixed feeding traits at Salzgitter (Rivals et al., 2009).
5 Materials and methods
5.1 Sample material and collection
The faunal material analyzed in this study is part of the Lebenstedt I (1952) assemblage currently stored at the Braunschweigisches Landesmuseum, Wolfenbüttel (Table 1). The Lebenstedt I faunal assemblage was chosen for sampling due to the excellent state of preservation including whole R. tarandus mandibles, compared to the high degree of fragmented of skeletal material from Lebenstedt II. The sample material was recovered from the main find horizon within the excavated area of Lebenstedt I (Figure 2) (Kleinschmidt, 1953; Preul, 1991). We sampled twenty-two R. tarandus teeth belonging to nine individuals including seven permanent second (M2) and third (M3) molars from the same mandible, one isolated M2 and one M3 from two additional individuals to provide a robust data framework for reindeer population analysis. For Equus sp. we studied an M2 and M3 tooth from one animal and an M3 tooth from a second individual. All elements were previously taxonomically identified by Kleinschmidt (1953). We targeted adult individuals with fully erupted M3 teeth (>2.5 years of age) and teeth were chosen based on availability, preservation and least wear. We selected teeth from the same side in order to avoid sampling the same individual twice. We preferentially selected later forming teeth to avoid any influence of an isotopic weaning signal (Hoppe et al., 2004; Skoog, 1968; Wright and Schwarcz, 1998). Furthermore, we sampled a number of individuals that were previously analyzed for tooth wear patterns by Rivals et al. (2009) to reconstruct dietary preference enabling us to compare our results with their study.
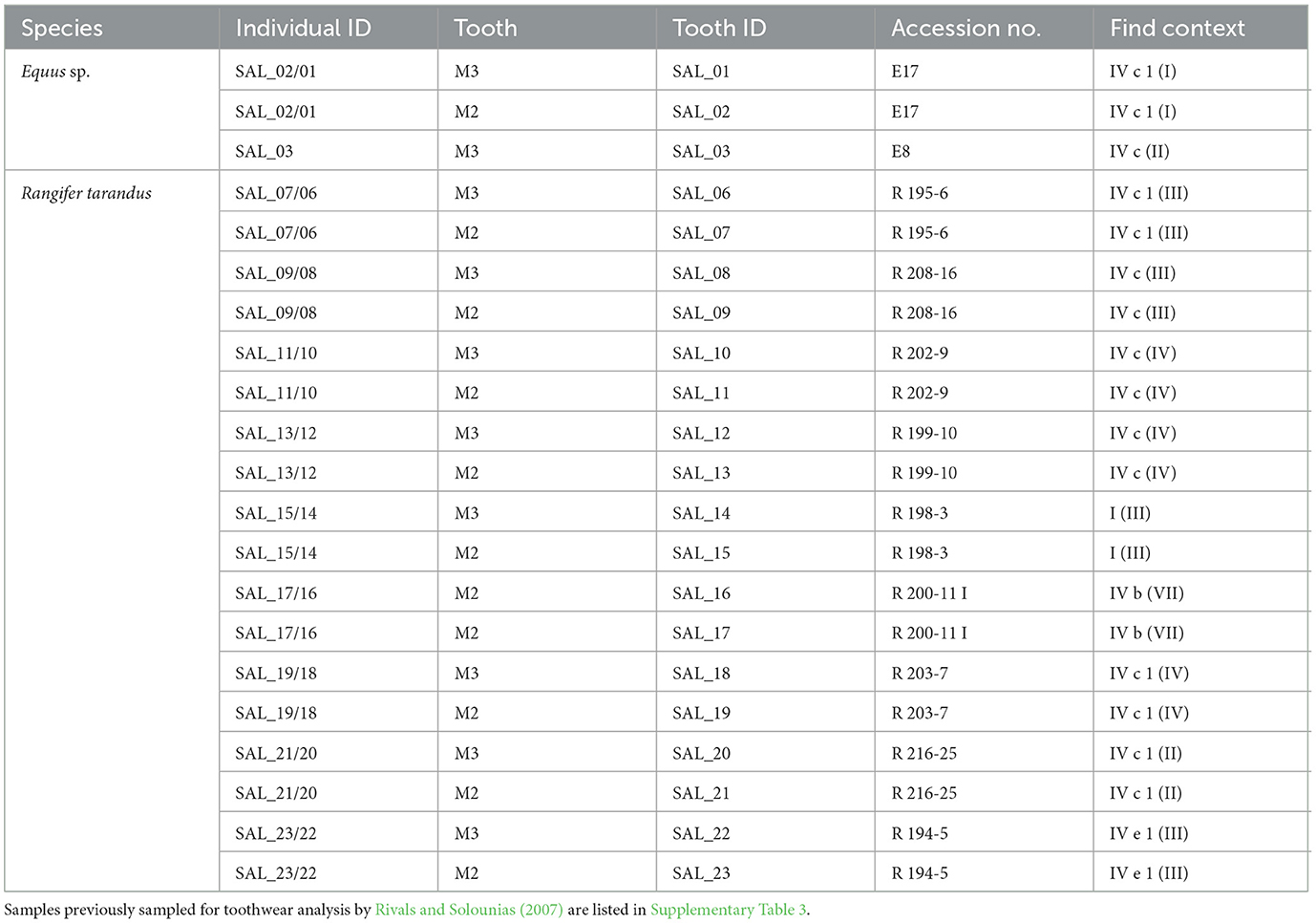
Table 1. Individual identification number and individual tooth identification number (this study), tooth analyzed, museum accession number and find context of specimens from Lebenstedt I (Kleinschmidt, 1953) sampled in this study.
5.1.1 Tooth mineralization and sequential sampling strategy
In hypsodont herbivores tooth enamel forms incrementally from the cusp to the apex over a period of months or years (Table 2) (Hillson, 2005). Once mineralized, enamel bioapatite is not replaced during an individual's lifetime and its dense crystalline structure means it is relatively resistant to diagenetic alteration compared to other skeletal tissues (Koch et al., 1997; Kohn et al., 1996; Lee-Thorp, 2000).
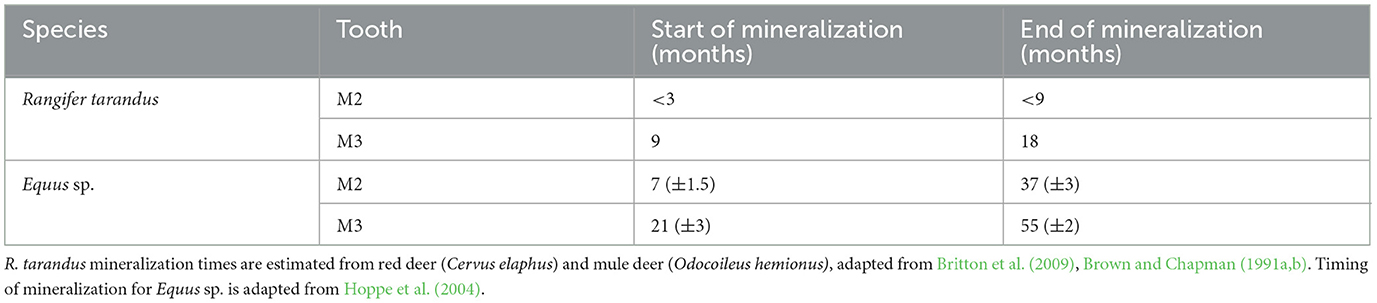
Table 2. Tooth enamel mineralization times of Rangifer tarandus and Equus sp. permanent molar teeth sampled in this study.
In both R. tarandus and Equus sp. teeth in the jaw form roughly in sequence, with varying degrees of overlap. As a result, sampling multiple teeth from one individual can provide a continuous record of isotopic data (Hoppe et al., 2004). The exact timing of enamel mineralization is currently unknown for reindeer and is estimated based on data from other cervids (Brown and Chapman, 1991a,b) and supported by intra-tooth isotope studies of modern caribou populations (Britton, 2010; Britton et al., 2009). The M2 and M3 are expected to form in sequence, with M2 mineralization taking place between < 3.5 and 9 months, between the first summer and the first winter of the animal's life, and M3 mineralization occurring between 9 and < 18 months, from the second spring to second winter of life (Table 2) (Britton et al., 2009; Brown and Chapman, 1991a,b). In contrast, timing of enamel mineralization in modern equids is relatively well documented (Bryant et al., 1996; Hoppe et al., 2004; Soana et al., 1999). Mineralization in the M2 occurs between 7 (±1.5) and 37 (±3) months, and between 21 (±3) and 55 (±2) months in M3 teeth (Table 2) (Bendrey et al., 2015; Hoppe et al., 2004).
Prior to sampling, dirt from the burial environment and dental calculus was removed from the tooth sampling surface using a Dremel drill. Sequential samples of powdered enamel were obtained using a handheld diamond-tipped Dremel drill along the length of the tooth crown from cusp to cervix (Figure 3). Samples were obtained between ~2 mm below the occlusal surface (OS) to ~2 mm above the enamel root junction (ERJ) to prevent breakage, with a width of ~ 1 mm and a breadth of ~3 mm. Approximately 10 mg of enamel powder was taken for stable oxygen and carbon isotope analyses and an additional ~15 mg for strontium isotope analysis, in order to accommodate for sample loss during pre-treatment or during analysis. Our sampling strategy was based on the specific timing of enamel mineralization of each tooth in order to best capture intra-annual variation in the enamel isotope signal (Britton et al., 2009; Hoppe et al., 2004; Price et al., 2017). From the equid molars, enamel samples for δ18O and δ13C analyses were taken at ~3 mm intervals. For 87Sr/86Sr analysis, samples were obtained at evenly spaced intervals along the crown corresponding with approximately 6 months intervals of enamel mineralization in order to capture sub-annual fluctuations in the intra-tooth isotope signal. Due to the shorter period of enamel mineralization in reindeer molars, we sampled as close to the previous sample as possible along the length of the crown for δ18O, δ13C and 87Sr/86Sr isotope analysis.
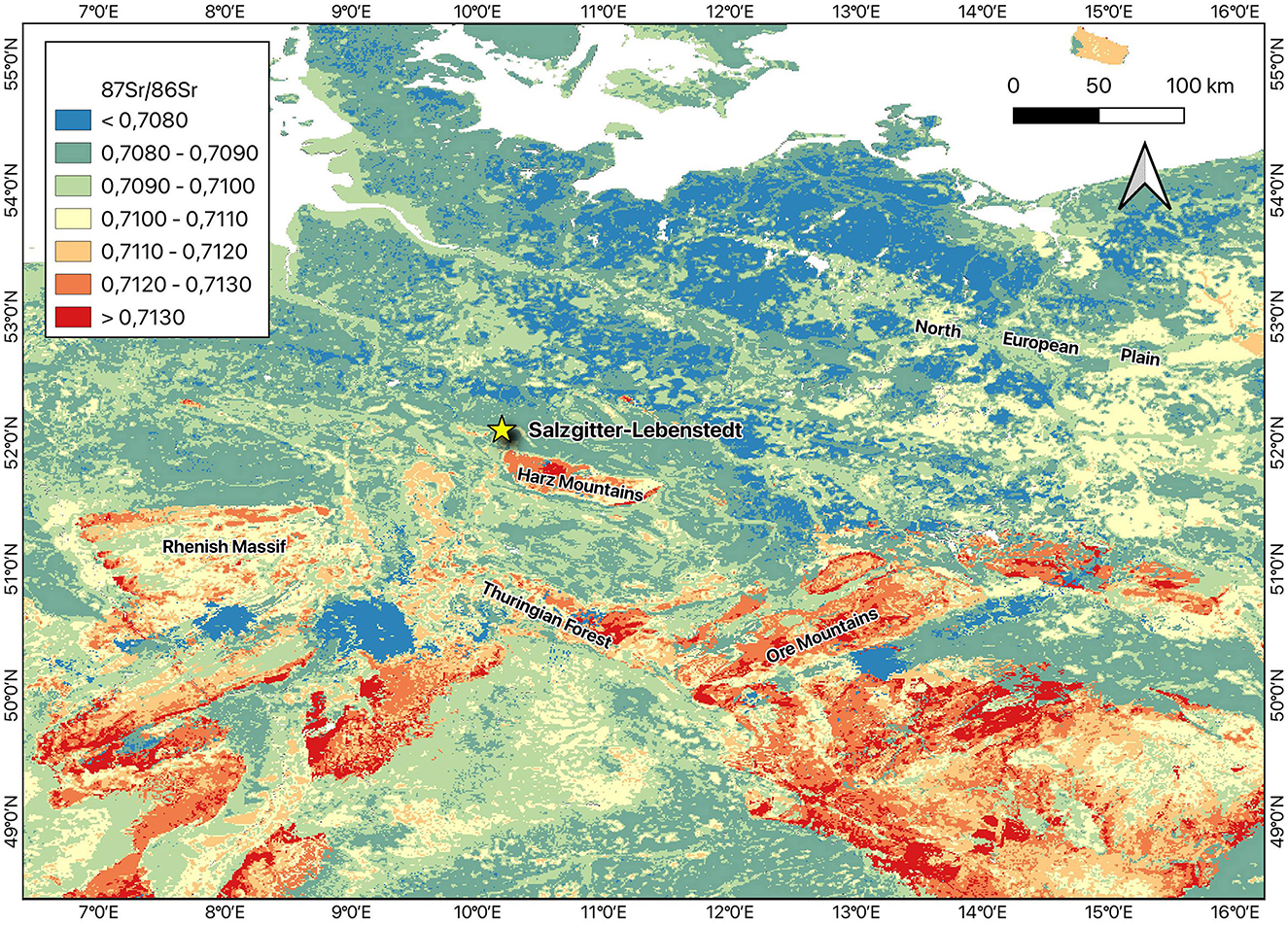
Figure 3. Bioavailable environmental 87Sr/86Sr isoscape from Bataille et al. (2020) cropped to Central Europe. See Supplementary Figure 2 for standard error map.
5.2 Isotope analysis
5.2.1 suple carbon (δ13C) and oxygen (δ18O) isotope analysis
Stable carbon and oxygen isotope analysis of R. tarandus and Equus sp. tooth enamel carbonate was carried out at the Stable Isotope laboratory, Max Planck Institute of Geoanthropology, Jena, Germany. In order to remove any organic or exogenous carbonate contaminates the powdered enamel samples were chemically pretreated prior to analysis following the protocol described in Sponheimer et al. (2005) and Lee-Thorp et al. (2012). This protocol comprised of a wash in 1 mL of 1% bleach solution (NaClO) for 60 minutes. Enamel samples were centrifuged and rinsed three times in ultra-pure H2O. Following this, 1 mL of 0.1 M acetic acid was added to each enamel sample for a period of 10 min, after which each sample was again centrifuged and rinsed three times in ultra-pure H2O. In preparation for analysis samples were freeze dried for 4 h, or until fully dry, after which 3–3.5 mg of the enamel powder was weighed out for analysis by mass spectrometry. Samples were then reacted with 100% phosphoric acid, and gases evolved from the samples were analyzed for their stable carbon and oxygen isotopic composition using a Thermo GasBench 2 connected to a Thermo Delta V Advantage Mass Spectrometer (Thermo Fisher Scientific).
These stable carbon and oxygen isotope values were then compared against international standards registered by the International Atomic Energy Agency. The results are reported as delta (δ) values as parts per thousand (per mil, ‰) relative to the international standard Vienna Pee Dee Belemnite for 13C/12C and 18O/16O. Where δ(‰) = [(Rsample/Rstandard)−1] x 1,000, and R is the 13C/12C or 18O/16O ratio. The δ18O and δ13C values were normalized using a three-point calibration against the international standards mIAEA-603 (δ13C = 2.5‰; δ18O = −2.4‰); IAEA–CO−8 (δ13C = −5.8‰; δ18O = −22.7‰); and IAEA NBS 18 (δ13C = −5.04‰; δ18O = −23.2‰)] and USGS44 (δ13C= −42.2‰) was used as the in-house standard. Replicate analysis of the USGS44 standard shows that the instrument measurement error is c. ± 0.1‰ for δ13C and ± 0.2‰ for δ18O. Finally, measurement of repeat extracts from an equid enamel standard (ID: MAXIMUS) (n = 3, ± 0.2‰ for δ13C and ± 0.3‰ δ18O) to calculate overall measurement precision.
5.2.2 Strontium isotope (87Sr/86Sr) analysis
Preparation and analysis of enamel for strontium isotope (87Sr/86Sr) ratios was carried out in the Department of Geological Sciences, University of Cape Town following established procedure described in Copeland et al. (2008). Following chemical preparation, powdered samples were weighed into 7 mL Savillex PFA beakers and 2–3 m of 65% HNO3 was added, after which the beakers were closed and kept at 140°C for 1 h. After complete dissolution of the sample, they were dried before being taken up in 1.5 ml of 2M HNO3. Following this, the strontium (Sr) fraction was then isolated using TrisKem International Sr Spec resin and dried down before being re-dissolved in 0.2% HNO (Pin et al., 1994). The isolated Sr fractions were then analyzed as 200 ppb Sr solutions using the Nu Plasma HR MC-ICP-MS. The enamel samples were processed in parallel with repeat analysis of the in-house carbonate reference material (NM95) which produced an average 87Sr/86Sr value of 0.7089015 ± 0.000028 [2σ] (n = 24). This value is in good agreement with the long-term average of this reference material at this facility of 0.708911 ± 0.000040 [2σ]; n = 414. The 87Sr/86Sr data presented in the current study are referenced to bracketing analyses of the international strontium isotope standard NIST SRM987 (87Sr/86Sr reference value of 0.710255), and enamel values were corrected for instrumental mass fractionation using the exponential law and an 86Sr/88Sr value of 0.1194. Correction of isobaric 87Rb interferences was undertaken using the measured 85Rb signal alongside the natural 85Rb/87Rb. Total procedural blanks processed with samples at this laboratory produced background Sr levels < 250 pg, and were therefore negligible.
5.3 Bioavailable strontium (87Sr/86Sr) isoscape and spatial assignment
We used the global bioavailable 87Sr/86Sr isoscape raster produced by Bataille et al. (2020), cropped to Central Europe, to interpret the faunal enamel isotope data in terms of individual movements and range locations on the landscape (Figure 3). Throughout the study area, variation in modeled ranges of bioavailable strontium values broadly corresponds with variation in the regional lithology (Supplementary Figure 1). Higher bioavailable 87Sr/86Sr values are seen in volcanic mountainous areas, while lower values characterize the sedimentary basins including the Subhercynian Basin, in which Salzgitter is located, as well as the NEP. This trend is supported by 87Sr/86Sr values of locally sampled water sources (Käßner et al., 2023) and modern plants (Heddell-Stevens et al., 2024). Modeled ranges of bioavailable environmental 87Sr/86Sr in the area of the site are between 0.7080 and 0.7100 (~5 km radius). Using the cropped 87Sr/86Sr isoscape from Bataille et al. (2020) we performed spatial assignment on 87Sr/86Sr values correlating with the winter and summer (end members) based on the maximum δ18O intra-tooth values (summer) and the minimum intra-tooth δ18O values (winter) for each individual using the assignR package v.2.2.0 in R (Figure 4) (Ma et al., 2020). The assignR package then uses a Bayesian statistical approach to assign geographical origin of enamel 87Sr/86Sr values to the bioavailable 87Sr/86Sr isoscape within a ~400 km radius of the site.
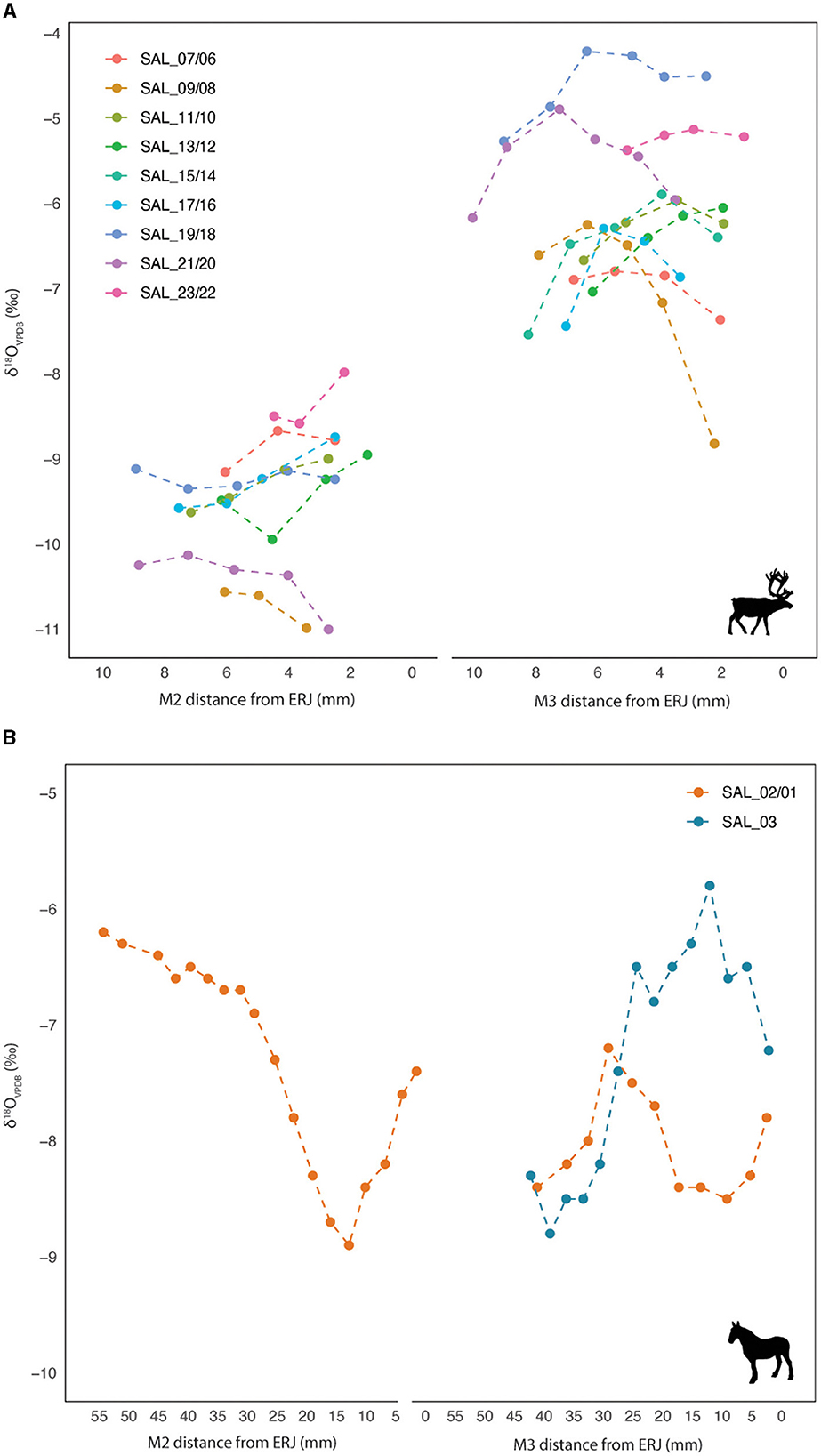
Figure 4. Intra-tooth δ18O results for (A) Rangifer tarandus M2-M3 teeth from individuals SAL_07/06, SAL_09/08, SAL_11/10, SAL_13/12, SAL_17/16, SAL_19/18, SAL_21/20 and SAL_23/22 and isolated M3 tooth from SAL_15/14 and (B) Equus sp. M2-M3 (SAL_01/02) and isolated M3 (SAL_03) teeth at Salzgitter-Lebenstedt.
6 Results
6.1 Oxygen (δ18O) isotope results
The intra-tooth δ18O values from the nine Salzgitter reindeer display a combined range of −11.0 to −4.2‰ (Supplementary Table 4, Figure 4). All individuals exhibit a semi-sinusoidal inter-tooth pattern in M2-M3 δ18O values and this pattern is likely reflective of seasonal fluctuations in environmental waters during enamel mineralization. Taking into account a degree of tooth wear and loss of isotope signal, the intra-tooth δ18O values of the Salzgitter individuals correlate well with predicted growth rates for R. tarandus molars (Britton et al., 2009; Brown and Chapman, 1991a) with M2 mineralization taking place between winter and early spring, and M3 mineralization between early spring and autumn. Based on this pattern, the combined M2-M3 isotopic signal represents ~1 year of life. (Britton et al., 2009; Brown and Chapman, 1991a). The Salzgitter reindeer all display greater differences in δ18O values between teeth than within teeth, while, within the teeth, M3 intra-tooth δ18O values display greater inter-individual variation compared to that seen in the M2 teeth. Differences in the position of maximum summer (peaks) and minimum winter (troughs) along the tooth crown likely derive from a combination of factors including differences in the amount of wear, as well as small-scale differences in climate conditions between years and/or differences in migratory behaviors, such as the timing of spring migration, of these animals.
The two equids also display semi-sinusoidal curves in intra-tooth δ18O values exhibiting a combined range of−8.9 to−5.8‰, with an intra-tooth amplitude of 2.7‰ in the M2 tooth (SAL_02/01) and 1.3‰ and 3.0‰ in the M3 teeth (SAL_02/01 and SAL_05 respectively) (Figure 4). When compared to the predicted timing of enamel mineralization (Hoppe et al., 2004), the amplitude and spacing of intra-tooth δ18O peaks and troughs along the tooth crown likely reflect seasonal fluctuations in the δ18O values of meteoric water (Balasse, 2002; Hoppe et al., 2004). On the basis of the intra-tooth δ18O signal and taking into account wear on the teeth, SAL_02/01 M2-M3 teeth represent a combined period of ~18 months of life with the M2 tooth likely incorporates the period between mid-summer through winter into spring, while timing of M3 mineralization corresponds to a ~1 year period between two winters (Balasse, 2002; Hoppe et al., 2004). The M3 isotopic signal of SAL_03 represents ~7 months of isotopic signal, from winter to mid-summer. The M2 and M3 teeth belonging to individuals SAL_02/01 and SAL_03 respectively, exhibit a similar range in δ18O values (between−6‰ and−9‰). In contrast the M3 tooth of SAL_02/01 displays a smaller range in intra-tooth δ18Oenamel, due to lower warm season values.
6.2 Carbon (δ13C) isotope results
Intra-tooth δ13C values of the Salzgitter reindeer (n = 9) have a combined range of −10.7 to −7.6‰ (Figure 5). A decrease in δ13C values is seen between the M2 and M3 teeth resulting in a semi-sinusoidal intra-tooth pattern (Supplementary Table 4, Figure 5). All analyzed individuals display higher δ13C values in the M2 teeth compared to the M3 with no overlap in values between the two molars. This is indicative of seasonal shifts in diet, coupled with smaller-scale seasonal changes in plant leaf δ13C values related to environmental factors (Cernusak et al., 2016; Farquhar et al., 1989a; Hoppe et al., 2004; Mook et al., 1983). It is likely higher δ13C values in M2 teeth (during the cold season) reflect consumption of lichens at this time of the year (Bocherens, 2003; Fizet et al., 1995), a trend that is widely observed in modern R. tarandus populations (Ben-David et al., 2001; Drucker et al., 2001). The same pattern is seen across all individuals, suggestive of similar dietary traits among these animals. Inter-individual range in δ13C values is smallest at the M2 ERJ sample position and largest at the M3 ERJ sample position. The combined range in intra-tooth δ13C values of the two Salzgitter horses is −12.3‰ to −11.4‰, with a large degree of overlap between the individuals (Figure 5). These values are indicative of a graze-based diet during the period of enamel mineralization, indicative of grazing in grassland and steppic environments (Bocherens, 2003). While all three teeth display minimal intra-tooth variation in δ13C ranges, the M2 of individual SAL_01/02 exhibits the largest range in δ13C values (0.9‰) and the δ13C values in the M3 teeth (SAL_02/01 and SAL_03) fall within this range.
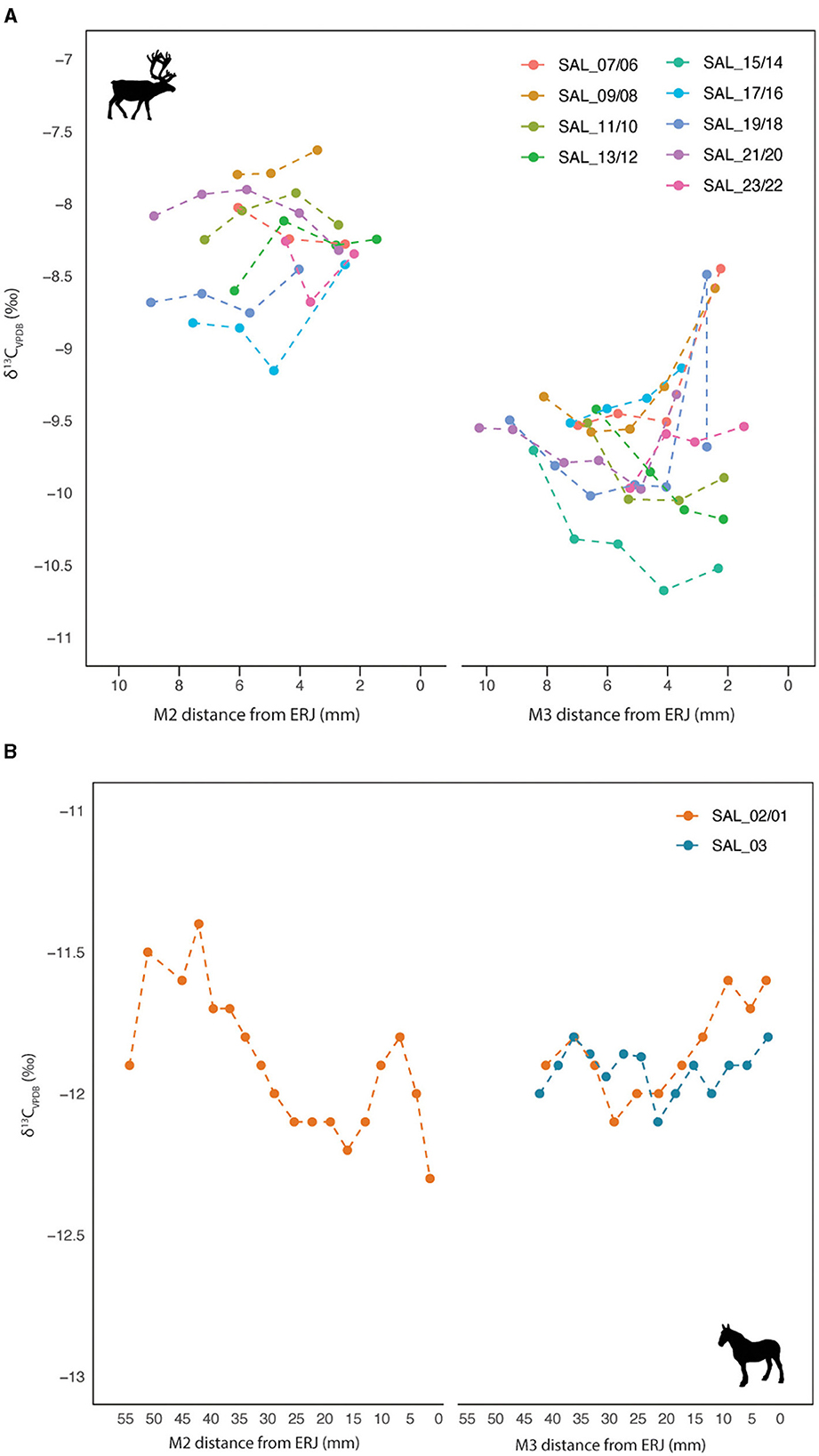
Figure 5. Intra-tooth δ13C results for (A) Rangifer tarandus M2-M3 teeth from individuals SAL_07/06, SAL_09/08, SAL_11/10, SAL_13/12, SAL_17/16, SAL_19/18, SAL_21/20 and SAL_23/22 and isolated M3 tooth from SAL_15/14 and (B) Equus sp. M2-M3 (SAL_01/02) and isolated M3 (SAL_03) teeth at Salzgitter-Lebenstedt.
6.3 Strontium (87Sr/86Sr) isotope results
The combined range of intra-tooth 87Sr/86Sr values of Salzgitter reindeer (n = 9) is 0.7100-0.7136, with the values of the majority of individuals (n=6) plotting between 0.7110 and 0.7118 (Supplementary Table 4, Figure 6). The 87Sr/86Sr values of SAL_07/06, SAL_11/10 and SAL_13/12 lie in the same range as those of the bioavailable 87Sr/86Sr found within a ~2km radius of the site (0.7100-0.7107). No clear inter- or intra-tooth trends in 87Sr/86Sr values can be identified among individuals, and summer peaks and winter troughs in intra-tooth 87Sr/86Sr values are located at different points along the M2-M3 tooth crowns. Three reindeer exhibit higher 87Sr/86Sr values in M2 teeth compared to the M3 (SAL_15/14, SAL_19/18 and SAL_21/20), while the opposite trend is observed for the remaining four individuals (SAL_07/06, SAL_11/10, SAL_17/16 and SAL_23/22). Two of the nine reindeer (SAL_09/08 and SAL_13/12) exhibit an overlap in M2 and M3 87Sr/86Sr values, displaying opposite cyclical trends in inter-/intra-tooth (M2-M3 teeth) 87Sr/86Sr values. The largest difference in values is seen at the ERJ sample position in M2 teeth, corresponding with first winter to early spring timing of mineralization, while the greatest amount of overlap among individuals is at the M3 mid-crown (through to ERJ) sample position corresponding with enamel mineralization during the second summer to early autumn of life.
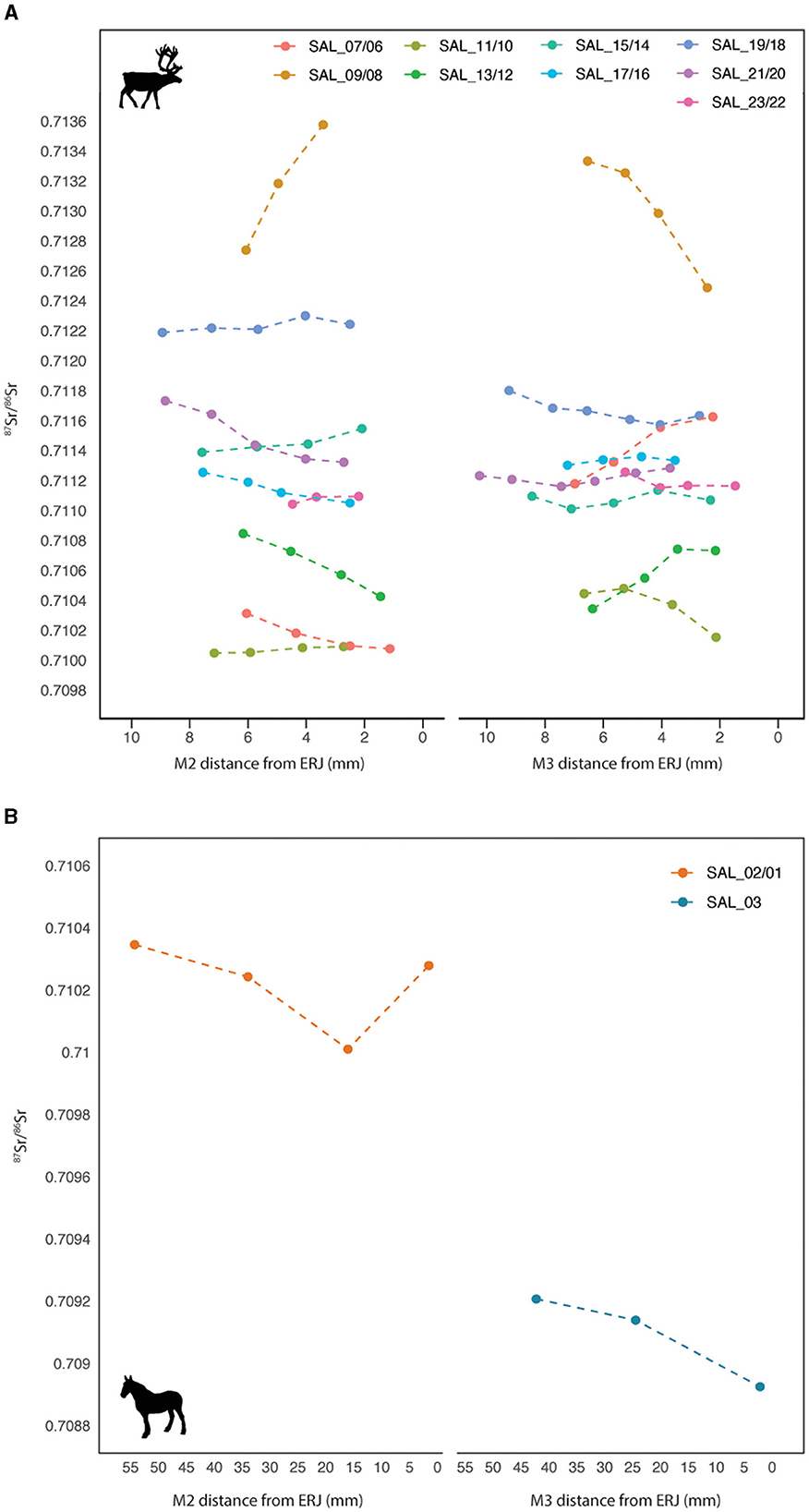
Figure 6. Intra-tooth 87Sr/86Sr results for (A) M2-M3 teeth of Rangifer tarandus individuals SAL_07/06, SAL_09/08, SAL_11/10, SAL_13/12, SAL_15/14, SAL_17/16, SAL_19/18, SAL_21/20 and SAL_23/22 and (B) Equus sp. M2 (SAL_02/01) and M3 (SAL_03) teeth at Salzgitter-Lebenstedt.
The combined range of 87Sr/86Sr values for the two Salzgitter horses is 0.7089-0.7103 (Figure 6). SAL_02/01 has a combined M2-M3 intra-tooth range of 0.7100-0.7103 with 87Sr/86Sr intra-tooth values decreasing mid-crown during the colder months, as indicated by trends in the intra-tooth δ18O values, then increasing again toward the ERJ, during spring-summer. The second equid (SAL_03) exhibits comparatively lower 87Sr/86Sr values with a greater intra-tooth range (0.7089-0.7092). These values overlap with the range of modeled bioavailable 87Sr/86Sr values for site lithology. The 87Sr/86Sr intra-tooth values in this animal exhibit a slight decrease (0.0003) from OS to ERJ corresponding with winter to summer period of enamel mineralization identified on the basis of intra-tooth δ18O values.
6.4 Spatial assignment results
Spatial assignment was used to identify the location of winter and summer ranges (90% probability) of the Salzgitter reindeer (Figure 7) and horses (Figure 8) analyzed in this study. Overlap in these ranges is shown in purple while probable winter ranges are given in blue and summer ranges are in yellow.
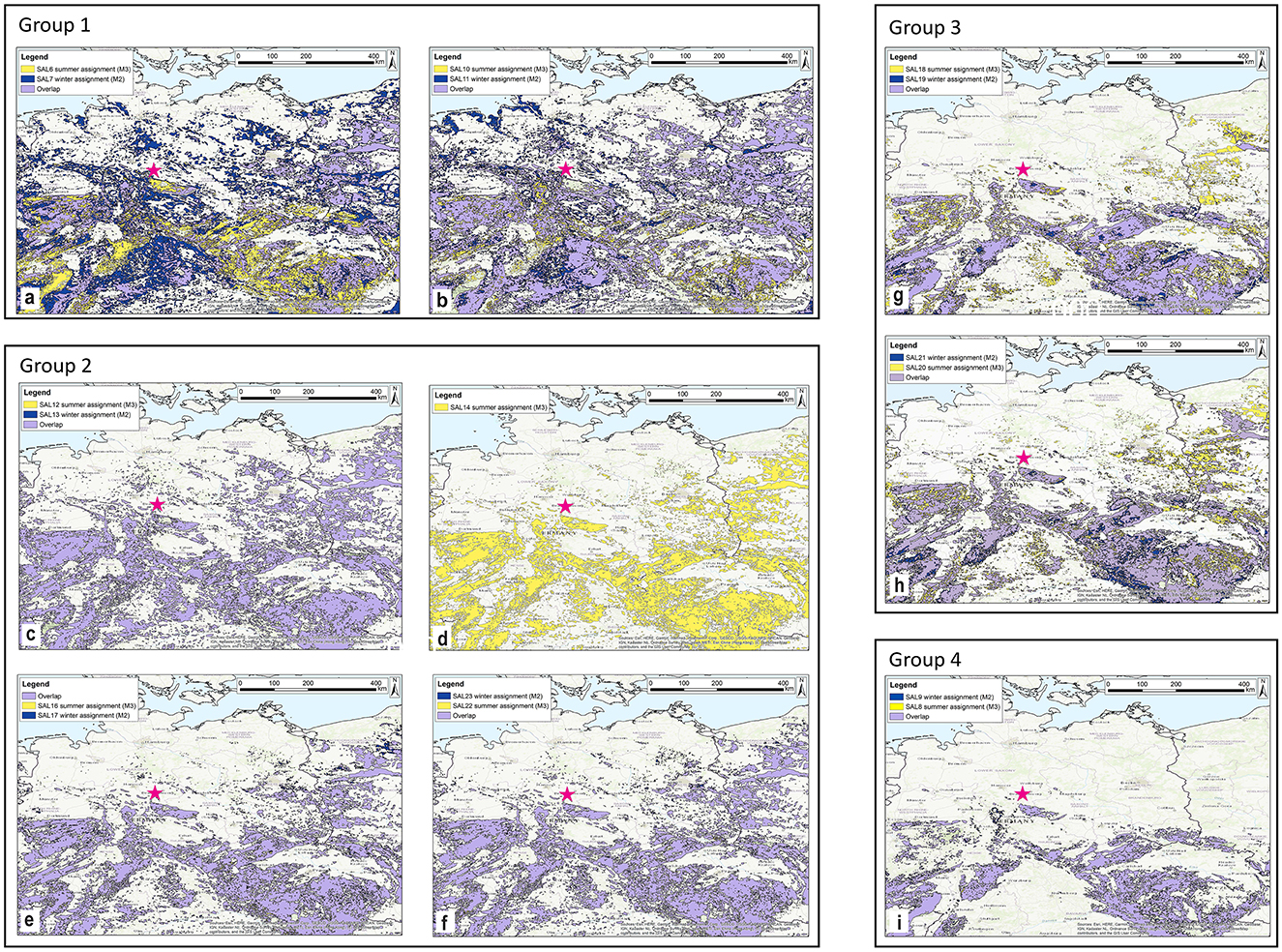
Figure 7. Spatial assignment of winter and summer ranges for Rangifer tarandus at Salzgitter with a 90% probability of origin. Blue denotes winter ranges, yellow denotes summer ranges and overlap between likely winter and summer ranges is shown in purple. The pink star gives the location of the site. The individual reindeer are grouped according to the four major trends in probably (90%) winter and summer ranges identified in the spatial assignment. Group 1 includes individuals SAL_07/06 (A) and SAL_11/10 (B), group 2 includes SAL_13/12 (C), SAL_14 (M3 only) (D), SAL_17/16 (E) and SAL_23/22 (F), group 3 comprises of SAL_19/18 (G) and SAL_21/20 (H) and group 4 contains SAL_09/08 (I).
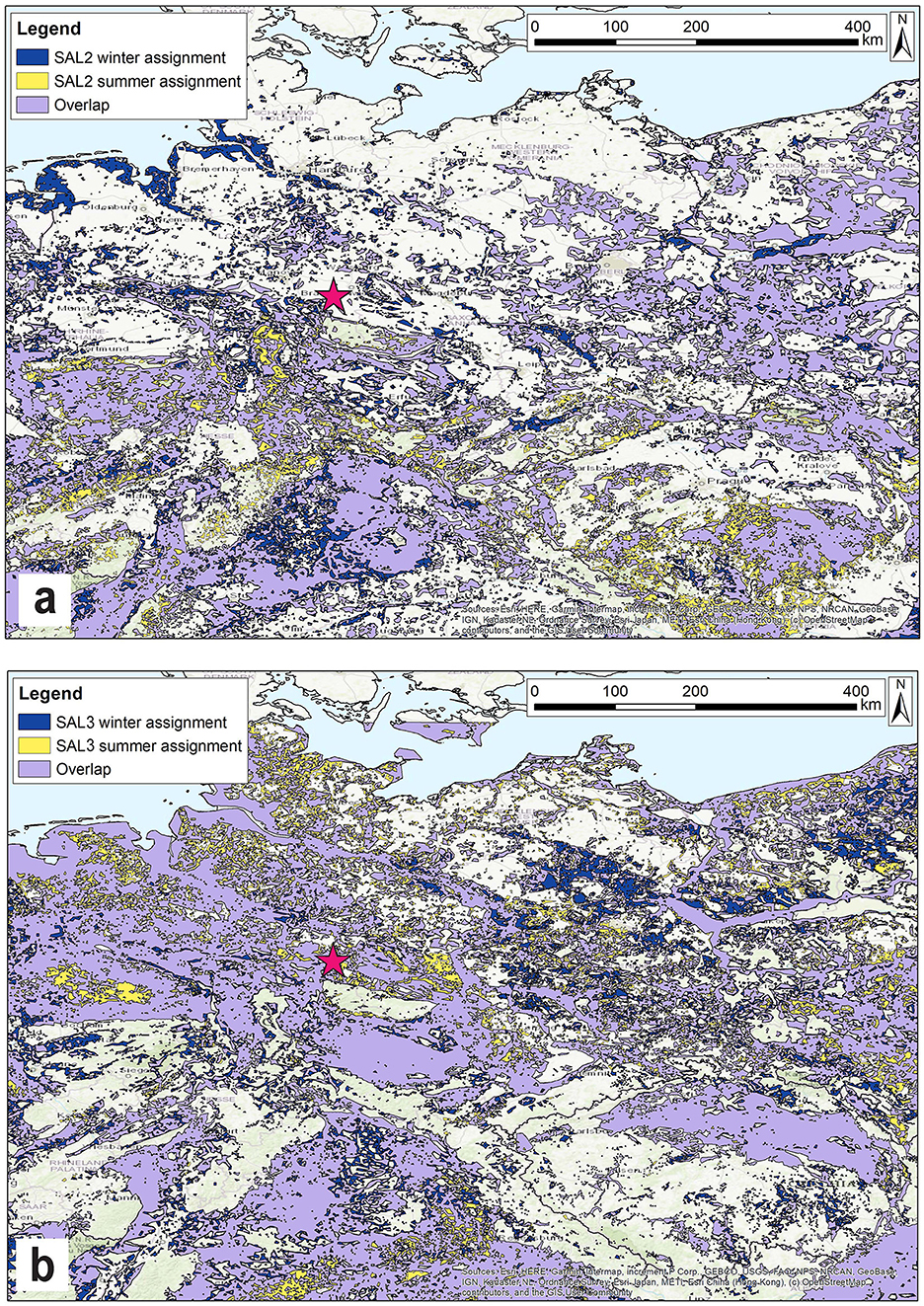
Figure 8. Spatial assignment of the Salzgitter Equus sp. individuals, (A) SAL_02/01, (B) SAL_03, winter and summer ranges with a 90% probability of origin. Blue denotes winter range, yellow/orange denotes summer ranges and winter/summer range overlap is shown in purple. The pink star gives the location of the site.
Multiple areas are identified as probable winter and summer ranges for the Salzgitter reindeer. It is important to note that the spatial assignment highlights all areas with 87Sr/86Sr values that match those identified in the reindeer winter (minimum δ18O values) and summer (maximum δ18O values) enamel sample positions. This does not, however, mean that these individuals occupied the entirety of the highlighted regions, rather they could have undertaken smaller or larger scale movements within these areas. However, a number of trends in space use by the Salzgitter reindeer analyzed here are still apparent. There is a notable lack of identified ranges in the immediate vicinity of the site, and to the north and northwest. The spatial assignment identifies a large degree of overlap (>67%) in likely winter and summer ranges of all reindeer, although the potential size of the ranges may have varied between individuals. We identified four major trends (groups 1–4) (Figure 7) in potential sizes of winter/summer ranges, range overlap and associated topographies in the spatial assignment results of the Salzgitter reindeer. Group 1 comprises of SAL_07/06 (Figure 7A) and SAL_11/10 (Figure 7B), group 2 is made up of SAL_13/12 (Figure 7C), SAL_14 (Figure 7D), SAL_17/16 (Figure 7E) and SAL_23/22 (Figure 7F), group 3 includes SAL_19/18 (Figure 7G) and SAL_21/20 (Figure 7H) and group 4 consists solely of SAL_09/08 (Figure 7I). Individual SAL_07/06 exhibits the largest potential range with the least amount of overlap (68%) between winter and summer ranges. For this individual, areas identified as exclusive to summer ranges are associated with areas [above ~400 m above sea level (asl)] in the Central European Upland Zone, while potential winter ranges are identified in lower laying areas on the NEP and in Southern Germany. Seasonal ranges identified for SAL_11/12, display a greater degree of overlap between winter and summer ranges (88%) associated with areas < 400 m asl. For individuals SAL_13/12, SAL_17/16 and SAL_23/22 slightly smaller ranges are identified, as well as a larger degree of overlap in likely winter and summer ranges of 100%, 95% and 97% respectively, associated with elevated areas up to ~800 m asl in the Upland Zone, as well as lower laying areas to the west of the site and Southern Germany. Individuals SAL_19/18 and SAL_21/20 exhibit similar sized potential ranges, however, smaller areas at elevated areas may have been occupied exclusively during winter, while some low laying are exclusively associated with summer ranges. Individual SAL_09/08 exhibits the smallest potential home range, associated almost exclusively with areas above ~400 m asl, and displays a high degree of overlap (95%) between winter and summer ranges. For individual SAL_15/14, only spatial assignment of summer range was possible, due to a lack of M2 enamel preservation. The probable winter range of this individual, is almost identical to those of SAL_13/12 and SAL_23/22.
Spatial assignment of winter and summer ranges was also undertaken for the two Salzgitter equid individuals, SAL_02/01 (M2) (Figure 8A) and SAL_03 (M3) (Figure 8B). The results indicate different potential winter and summer ranges for the two individuals. Both animals display a large degree of overlap between their respective winter and summer ranges of 79% (SAL_02/01) and 87% (SAL_03). For individual SAL_02/01 probable ranges include foothills and lower mountain slopes of the Central Upland Zone, including the Harz Mountains, and low laying areas within the Thuringian Basin, Southern Germany and to the Northeast on the NEP. They do not include the area in the immediate vicinity of the site (2–5 km radius). Areas exclusively highlighted as potential summer ranges are scattered across the region to the south of the site while exclusive likely winter ranges are seen in small areas across the region. Overlap in probable winter and summer ranges of SAL_03 corresponds closely with low lying areas, including in the site lithology. Potential winter/summer range of this individual also includes parts of the NEP north of the site and to the west into Belgium and the Netherlands, as well the Thuringian Basin and Southern Germany. Small areas of potentially exclusive winter and summer ranges are seen scattered throughout the region.
7 Discussion
7.1 Reindeer behavior at Salzgitter
As outlined above, large-herbivore enamel δ18O values can be used to identify the seasonality of animal movements and can also inform on the scale of migrations (e.g., Barakat et al., 2023; Britton et al., 2009; Glassburn et al., 2018; Pellegrini et al., 2008). Intra-group similarity in enamel δ18O values among the Salzgitter reindeer suggests that these individuals experienced broadly similar climatic conditions during enamel mineralization. These reindeer exhibit greater variation in δ18O values between teeth than within them, a trend which has been shown to reflect seasonal long-distance (e.g., hundreds of kilometers) thermo-stress migrations between distinct winter and summer ranges in modern caribou herds in Alaska (Britton et al., 2009). These movements by Alaskan caribou (Rangifer tarandus) correspond with changing seasons and are undertaken to avoid climatic extremes at the different ends of these animals' ranges (Alerstam et al., 2003; Avgar et al., 2014) resulting in reduced seasonal amplitude in the intra-tooth δ18O signal relative to local precipitation (Britton et al., 2009). This same trend in the Salzgitter reindeer enamel oxygen isotope values may therefore also be indicative of similar, longer distance migrations between winter and summer ranges in response to seasonal weather patterns.
The similar ranges and patterning in δ13C values of the Salzgitter reindeer are indicative of analogous seasonal feeding behavior during enamel mineralization. These data display parallel trends to those seen in the inter-tooth δ18O values, further indicating that these individuals likely had similar habitat preferences. Lichens appear to have made a substantial contribution to the diets of the reindeer, particularly during the cold season when enamel δ13C values are highest. Evidence for lichen consumption has previously been identified in R. tarandus at other Late Pleistocene sites in Europe (Bocherens, 2003; Britton et al., 2018; Fizet et al., 1995; Rivals and Semprebon, 2017). During the warmer months, lichen intake by the Salzgitter reindeer appears to have decreased in favor of vascular plants (i.e., graminoids and shrubs), most likely in response to their increased availability during spring-summer (Ben-David et al., 2001; Klein, 1992; Webber et al., 2022). These δ13C values of these reindeer are not indicative of browsing behaviors (Bocherens, 2003) as previously identified for a number of these individuals via dental microwear analysis (Supplementary Table 3) (Rivals and Solounias, 2007). However, as microwear patterns reflect forage consumed immediately prior to death, it may be that these reindeer consumed greater amounts of browse in later years, subsequent to the period of during enamel mineralization.
Owing to the potential for long distance migrations in this species and the equifinality in bioavailable 87Sr/86Sr values across the region we were unable to determine the exact locations of the seasonal ranges of the reindeer analyzed in this study. However, in comparing the reindeer enamel 87Sr/86Sr signal with the modeled isoscape it appears that some areas can be ruled out as potential ranges, including most of Northern Germany and the lowlands to the west, as well as the Thuringian Basin. Rather, ranges of the Salzgitter reindeer are more likely to have been located within the Central Uplands, including parts of Southern Germany, and/or further to the east on the NEP. Due to the considerable size of these identified areas, individual range size and migration distance could have varied substantially.
The lack of overlap between 87Sr/86Sr values in the Salzgitter reindeer enamel and bioavailable 87Sr/86Sr values found in the vicinity of the site (ca. 5 km radius) are potentially due to a number of factors. Firstly, the reindeer may not have spend time in the immediate site vicinity, and by extension, large parts of the NEP, during the ~1 year period of enamel formation. Secondly, due to the presence of small discrete areas with higher bioavailable strontium values within a ~20 km of the site it is also possible that these animals moved through the area, across the different lithologies, relatively quickly (i.e. faster than the rate of enamel mineralization) producing an averaged 87Sr/86Sr signal in their enamel. Modern R. tarandus tend to be highly mobile, even within summer and winter habitats, moving continuously to find forage, and avoid predators and insect harassment (Ehlers et al., 2024; Joly et al., 2024; Theoret et al., 2022). It is therefore possible that the site was located within the seasonal ranges of these reindeer without producing a visible “local” strontium signal in the enamel. A further explanation here could be that the incremental sampling by hand was not at a high enough resolution to pick up brief periods on the local geology.
Given the range in 87Sr/86Sr values found in the Salzgitter reindeer (0.7100-0.7136) and the relatively low values modeled for most of the NEP (< 0.7100), it appears that the majority of these individuals spent time on lithologies with substantially higher values, such as those found in the Central Uplands (>0.7110). The closest values in this range are found in the Harz Mountains, some 20 kms to the south of Salzgitter. If the site was located on a reindeer migration route, as hypothesized (Gaudzinski, 1999; Gaudzinski and Roebroeks, 2000), it appears likely that migrations were undertaken on a roughly east-west axis across – potentially along the northern edge of the Central Uplands. East-west migrations in Northern Central Europe have previously been identified in reindeer from the Late Upper Paleolithic site of Stellmoor, Northern Germany, during the Younger Dryas cold stage using multi-isotope data (Price et al., 2017). It is possible that reindeer undertook east-west directional migrations at different times during glacial periods in Northern Europe.
The intra-group variation in enamel δ18O and 87Sr/86Sr values of the Salzgitter reindeer are indicative of differences in biogeography and mobility patterns between these animals. The four major trends identified via the spatial assignment point to differences spatial ecology of the Salzgitter reindeer. Taking into account the timing of enamel formation and wear on the teeth, inter-individual variation in δ18O values also point to slight differences the seasonal timing of enamel formation, suggestive of difference in climate conditions, and/or, the timing of migrations among these animals (Britton et al., 2009; Gigleux et al., 2019). Evidence for inter-annual variability in the timing, route, and speed of migration is well-documented in modern caribou herds (Ferguson and Elkie, 2004; Gurarie et al., 2019; Joly et al., 2021b; Le Corre et al., 2014; Theoret et al., 2022). These aspects are dependent on a wide range of factors, including climate and weather conditions, the timing of spring green-up, body condition and predation risk (Bergerud and Luttich, 2003; Joly et al., 2021a; Le Corre et al., 2016; Mallory et al., 2020). The Salzgitter reindeer may have exhibited flexibility in movement ecology in response to a similar range of factors. For example, the larger body size of a number of carnivore species during glacial periods in Europe (Bocherens, 2015) may have resulted in increased predation of ungulates during this period Therefore, predator avoidance may have been a main contributing factor in the variability of reindeer movements at Salzgitter.
While we could not to identify the exact ranges and migration routes of the Salzgitter reindeer, we were able to identify some intra-group level trends in space use/biogeography, potentially associated with topography. The combined results of the spatial assignment and intra-tooth 87Sr/86Sr data indicate a high probability that parts of the Central Upland Zone as potential winter and summer range locations for all of the reindeer analyzed here. While no clear seasonal preference toward higher or lower elevation could be identified, at least four of the reindeer (SAL_07/06, SAL_11/10, SAL_19/18 and SAL_21/20) may have moved altitudinally between seasons. Within these individuals however, opposite tends are observed, with two of these animals (SAL_07/06 and SAL_11/10) potentially moving occupying summer ranges at higher elevation while for SAL_19/18 and SAL_21/20 higher elevation is associated with winter ranges. Seasonal altitudinal movements have been observed in different sub-species of extant R. tarandus, and here too, both winter and summer ranges can be found at elevation, as well as intra-seasonal movements between elevations (Bergerud, 1967; Brown et al., 1986; Cichowski et al., 2004; Oosenbrug and Theberge, 1980). In these modern populations altitudinal movements are driven predominantly by climate, but also snow cover and forage availability as well as insect avoidance (Cichowski et al., 2004; Stevens et al., 2001). Salzgitter is located on the northern edge of the Central European Upland Zone, and the closest potential ranges found at elevation to the site at elevation are seen ~30 km to the south in the Harz Mountains. Large areas throughout the Central Uplands are also identified as likely seasonal ranges. Fontana (2017) argues that diverse range of habitats provided by the topographic contrast found between the foothills of the Massif Central (Southwestern France) and adjacent areas, supported herds of non-migratory reindeer at the end of the Late Pleistocene. Perhaps the Central Uplands and contiguous basins provided a similarly diverse range of environments and access to different habitats earlier in the Late Pleistocene, making this region attractive to the Salzgitter reindeer.
Depending on the number of depositional events and period of accumulation of the reindeer remains, these differences in spatial ecology between the reindeer analyzed here may indicate considerable flexibility in migration routes as well as varying degrees of seasonal range fidelity in Salzgitter reindeer. While arguments for a single or small number of hunting events have been made on the basis of zooarchaeological data (Gaudzinski, 1998; Gaudzinski and Roebroeks, 2000), the complex depositional conditions outlined above have ultimately hampered efforts to identify the exact number of events. The isotopic data presented in this study points to differences in ranges and mobility patterns these animals, however, due to the high potential for considerable migratory plasticity in modern R. tarandus (e.g. Gurarie et al., 2019; Joly et al., 2021b,a; Severson et al., 2021), it is not currently possible to identify whether they belonged to a single herd on the basis of these results. The behavioral variation may reflect the presence of animals a single herd or population with a high degree variation in mobility patterns and seasonal range use, or alternatively, the existence of different groups in the region with different movement ecologies. Variation in spatial ecology of reindeer through time at a single location have also been identified between Middle Paleolithic contexts in Southwestern France, evidenced by a shift from migratory to sedentary ecotypes (Britton et al., 2023a). More recently, inter-individual variation in summer ranges was identified for reindeer during early MIS 4 at Königsaue, eastern Central Germany, ~100 km to the southeast of Salzgitter (Heddell-Stevens et al., 2024), further demonstrating the potential for behavioral plasticity of R. tarandus during the Last Glacial.
7.2 Equid behavior at Salzgitter
Given the similarity in intra-tooth trends in δ18Oenamel in the Salzgitter horses we suggest that climate conditions experienced by these individuals were broadly similar. Intra-tooth amplitudes in δ18O values also correlate well with those of two further equids (δ18Ophosphate) from the site obtained during a previous study (Stephan, 2017). Given the seasonal-scale variation in δ18Oenamel of the individuals analyzed here, we argue that these animals most likely drank from surface water source/s fed predominantly by local precipitation (Pederzani and Britton, 2019). This may have included a source within the nearby Fuhse river valley, as the current course of the river is located approximately 300 m to the west of the site. The horses may have come to this area to fulfill daily drinking water requirements as access to water sources is a key determinate of range use in modern equids (Hinton, 1978; Scheibe et al., 1998; Schoenecker et al., 2016). Seasonal amplitude in δ18Oenamel may further indicate that these horses are unlikely to have undertaken long-distance movements across climatic gradients during the period of enamel mineralization. Rather, they appear more likely to have been more residential, with ranges that incorporated the region around Salzgitter. Relatively sedentary behavior has been suggested for other equids within European contexts during the Last Glacial, in which similar intra-tooth δ18O amplitudes (1.5–5‰, enamel carbonate and phosphate) have been identified (Heddell-Stevens et al., 2024; Pederzani et al., 2024, 2021b; Pryor et al., 2016b). Interestingly, analysis of equid (n = 2) enamel from the Middle Paleolithic site of Königsaue (Layer A), also located on the northern edge of the Central Uplands, display a very similar range (between −10.0‰ and −6‰) and amplitude (1.9–2.5‰) in intra-tooth δ18O values. This is suggestive of similarities in drinking behaviors and similar climate conditions experienced by horses at both sites. The slight decrease in M3 intra-tooth amplitude displayed in SAL_02/01 may be explained by a degree of averaging in the isotopic signal, expected in later forming teeth and enamel (i.e., closer to the ERJ) owing to an increase in formation time (Bendrey et al., 2015). The lower warm season δ18O values in this tooth may also reflect enamel formation under cooler summer conditions, as a result of inter-annual fluctuations in temperature.
The two Salzgitter horses most likely consumed a graze-based diet during the period of enamel mineralization. Year-round grazing is consistent with the shrub tundra environment in the vicinity of the site (Schütrumpf, 1991; Selle, 1991; Sirocko et al., 2016) and dietary trends identified in equids at other glacial sites in central Eastern Germany (Heddell-Stevens et al., 2024) and Southwestern Germany (Pushkina et al., 2014), and Southern France (Britton et al., 2023a). The lack of intra-tooth variation in δ13C values in the Salzgitter horses is further indicative of a relatively stable diet during the period of enamel formation. In the majority of wild horses today, seasonal dietary shifts largely reflect availability of forage (Crane et al., 1997; Girard et al., 2013; Salter and Hudson, 1979). The persistence of grazing year-round by the Salzgitter horse therefore most likely reflect the grass-dominated steppe tundra environment of Northern Germany during the Last Glacial. The Salzgitter equids exhibit a much more restricted seasonal diet compared to the reindeer at the site, however, dietary spacing between these two species is similar overall to that identified at other Late Pleistocene glacial sites in Europe (Figure 9) (Drucker, 2022).
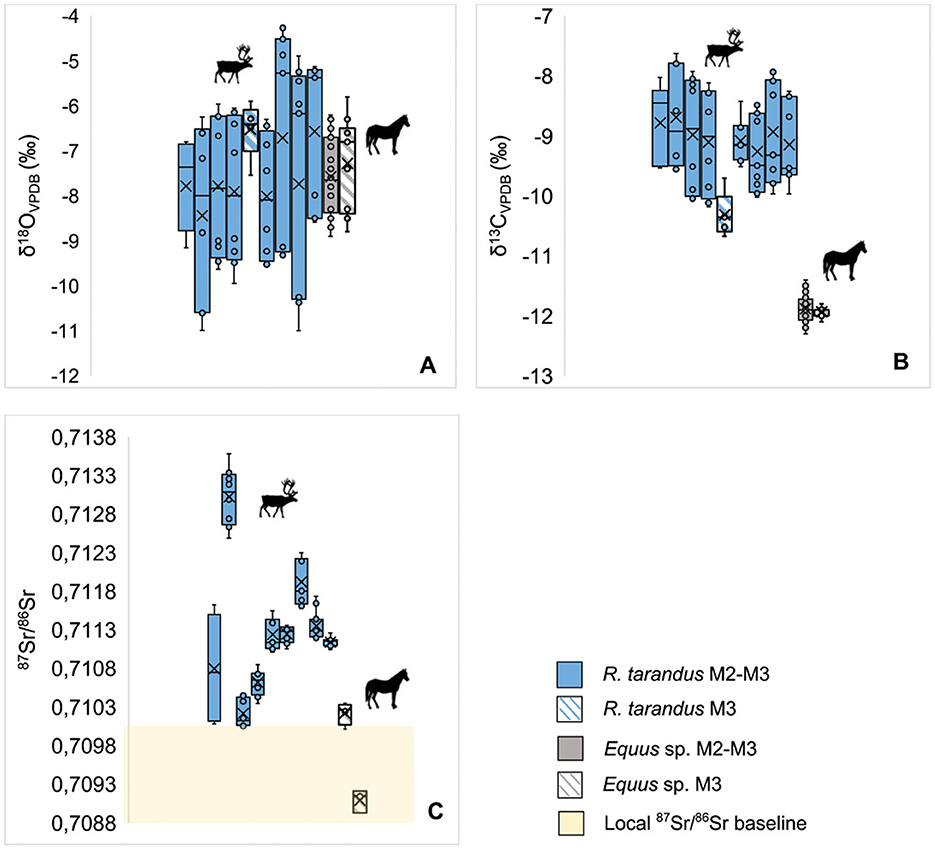
Figure 9. Combined enamel δ18O (A), δ13C (B) and 87Sr/86Sr (C) data for Rangifer tarandus and Equus sp. individuals at Salzgitter-Lebenstedt. Each box represents one individual. Blue boxes denote reindeer M2-M3 teeth and blue and white striped boxes represent isolated teeth. Gray box denotes equid M2-M3 teeth (SAL_02/01) and the gray and white striped box represents the isolated M3 tooth (SAL_03). The yellow box represents the local bioavailable 87Sr/86Sr baseline within a 5km radius of the site.
The 87Sr/86Sr values of both equids overlap with modeled bioavailable 87Sr/86Sr values within a ~5 km radius of Salzgitter, suggesting that these animals spent time in the site vicinity or on similar lithologies during the period of enamel mineralization. The seasonal-scale variation in δ18O intra-tooth signals and the presence of these animals' remains at the site suggests that they may have lived relatively local to the area during their lifetimes. The absence of long-distance migrations and relatively residential mobility has also been identified in equids within the Last Glacial contexts from central Eastern Germany (Pederzani et al., 2024; Heddell-Stevens et al., 2024) and Southwestern France (Britton et al., 2023a). These findings are consistent with smaller range sizes of wild extant equids in modern mesic steppe grasslands (0.75–12 km2) (King, 2002). In contrast to the reindeer, the spatial assignment results suggests that winter and summer ranges of the Salzgitter horses were located in lower laying areas, which may further point to differences in the ecological niches of these species in terms of topography as the reindeer likely spent time at elevation (Figure 7). Differences in 87Sr/86Sr values between the two Salzgitter horses does indicate that they moved over different lithologies during enamel mineralization, highlighting differences in individual biogeography. Differences in home ranges and mobility patterns may be due to differences in sex between these individuals, as wild extant bachelor bands are typically wider ranging than harem groups (Bahloul et al., 2001; King, 2002). Differences in spatial ecology may also be the result of differences in birth years and inter-annual differences in forage and water availability. Parallel trends in intra-tooth variation in 87Sr/86Sr and δ18O values, it appears that both animals undertook movements on a seasonal basis. This is likely reflective of seasonal availability of graze and drinking water sources, which are major drivers of seasonal movements in extant wild equids (Petersen et al., 2023; Schoenecker et al., 2023, 2016). During the period of site occupation Salzgitter was on a small stream alongside backwater ponds, which may have been exploited by horses as a drinking source at this time.
7.3 Strontium isotopes as a proxy for large-herbivore mobility patterns in Late Pleistocene Central Europe
As discussed above, the presence of similar underlying geologies and lithologies and resulting equifinality in bioavailable 87Sr/86Sr across the Central Europe can conceal individual ranging patterns within these areas and therefore pose a challenge when utilizing strontium isotopes as a provenancing tool in this region. Laser ablation sampling of tooth enamel for strontium isotope analysis could potentially reveal smaller scale movements or more specific areas that individuals moved across on the landscape between different geologies and may be a useful approach for future studies in this region (e.g., Le Corre et al., 2023). However, it would not enable us to discern between areas with the same bioavailable strontium values. The use of additional spatial proxies such as sulfur isotope (δ34S) analysis of bone collagen may provide another line of evidence in mobility studies (Bataille et al., 2021; Nehlich, 2015). Sulfur values reflect distance to the coast, and initial applications to reindeer remains from Late Pleistocene contexts in France have shown the efficacy of this method (Britton et al., 2023b). A major consideration when using 87Sr/86Sr isoscapes of Central and Northern Europe is that 87Sr/86Sr isoscapes are modeled from predominantly modern environmental samples and include a comparatively small number of archaeological samples, resulting in predominantly modern-day bioavailable 87Sr/86Sr baselines (Crowley et al., 2017; Holt et al., 2021). It is therefore important to consider the potential impact of the introduction of more recent depositions such as glacial tills and aeolian sediments into the region, subsequent to the period of Middle Paleolithic occupation at Salzgitter (Haase et al., 2007; Koster, 2005; Lehmkuhl et al., 2021). However, as bedrock age has been determined a key predictor of local bioavailable 87Sr/86Sr (Bataille et al., 2020, 2018; Bataille and Bowen, 2012; Hoogewerff et al., 2019; Käßner et al., 2023) we argue that the isoscape utilized in this study (Bataille et al., 2020) is applicable. However, future work should, where possible, incorporate sampling material from archaeological sites to ensure the 87Sr/86Sr isoscape reflects the bioavailable strontium values for the study time period as closely as possible.
7.4 Potential implications for Neanderthal reindeer hunting strategies at Salzgitter-Lebenstedt
Neanderthal hunting practices at Salzgitter have been interpreted as the targeted exploitation of reindeer herd/s during autumn migration (Gaudzinski, 2000; Gaudzinski and Roebroeks, 2000). It has been suggested that the assemblage was likely accumulated relatively rapidly, possibly as the result of a single hunting event (Gaudzinski, 2000; Gaudzinski and Roebroeks, 2000). In light of inter-individual variation in the biogeography and mobility patterns during enamel mineralization of the Salzgitter reindeer identified in the current study, it appears unlikely that these animals were from the same herd killed during a single event. We suggest that these animals likely belonged to different herds, or possibly to one herd that visited the site in different years. If these individuals were introduced to the site as a result of Neanderthal exploitation, then it appears likely that they represent multiple hunting episodes. However, we cannot rule out that they were members of a single herd, born in different years, with a high degree of inter-annual variation in mobility patterns, in which case they may have been hunted at the same time. Trends in the isotope data presented here cannot be explained by the spatial distribution, either vertical or horizontal, of the fauna material within Lebenstedt I, as such, further interpretation of Neanderthal hunting strategy at the site requires greater knowledge of depositional processes and the relationship between the finds. Ultimately, further dating of the faunal remains is needed to clarify the hunting events and Neanderthal occupation at the site. Whether these reindeer belonged to one or multiple herds, our results point to a potentially high degree of plasticity in reindeer mobility patterns and seasonal ranges during the Last Glacial Period in Central Europe which may have made reindeer movements and ranges less predictable for hominin hunters, which could point to flexibility in Neanderthal hunting strategies and the ability to adapt to changes in prey-species ecology. While the small sample size of the Salzgitter horses precludes in-depth interpretation of hunting strategy for this species at the site, these animals may have been relatively local to the site environment, if so, they could have been available to hunters throughout the year.
8 Conclusion
We analyzed δ18O, δ13C and 87Sr/86Sr isotope compositions of sequentially-sampled enamel of R. tarandus and Equus sp. from the Middle Paleolithic site of Salzgitter-Lebenstedt, Northern Germany, in order to reconstruct individual seasonal ranges and mobility patterns. Parallel trends in enamel δ18O and δ13C data point to similarity in seasonal feeding behavior in the Salzgitter reindeer. This suggests these individuals experienced similar environmental conditions during enamel formation. While inter-tooth patterns in δ18O values are potentially reflective of climate-induced migrations, 87Sr/86Sr values and spatial assignment of probable winter and summer ranges point to differences in mobility patterns and seasonal ranges of these animals. Although we were unable to identify the exact location of these ranges, most likely due to equifinality in bioavailable 87Sr/86Sr across Central Europe, the results of the spatial assignment suggest that the majority of the reindeer spent time at elevation and largely avoided the area to the north of the site. In contrast, the two equids from the site analyzed here appear to have preferentially occupied low laying areas, and while they may have undertaken smaller-scale movements on a seasonal basis. Similarity with the local 87Sr/86Sr values indicates they may have been relatively local to the site environment. We have discussed the challenges of using 87Sr/86Sr to identify exact ranges and migration routes of large-herbivores in Late Pleistocene Central Europe. Here we call for future studies to increase local sampling efforts, and these should ideally include sampling of archaeological material to establish bioavailable 87Sr/86Sr baselines that are contemporaneous with the period of hominin activity. The incorporation of other spatial proxies, currently under development, could provide an additional line of evidence for animal mobility behavior. Based on the isotope data generated in this study we suggest that the Salzgitter reindeer likely represent multiple herds with different annual ranges and mobility patterns, or, were members of the same herd with a high degree of inter-annual variation in movement ecology. Either way, our data point to varying degrees of plasticity and potentially flexibility in ranging behavior of reindeer during this time in Central Europe. This is consistent with the results of similar analysis of R. tarandus from other Middle Paleolithic contexts in Western and Central Europe (Britton et al., 2023a,b; Heddell-Stevens et al., 2024). While variation in reindeer spatial ecology would have played a role in Neanderthal hunting strategies at Salzgitter, further work is required to determine the seasonality and number of hunting events at the site.
In summary, this study highlights the importance of multi-isotope analysis when studying the movements of past animal populations. We have demonstrated the efficacy of this approach to obtain new information on the seasonal biogeography of two prevalent large-herbivore species during the Late Pleistocene in Northern Germany. Our results highlight the importance of reconstructing prey-taxa behavior and ecology on a site-by-site basis, particularly as this information plays a major role in interpretations of hominin subsistence strategies and environmental adaptations during the Paleolithic in Europe.
Data availability statement
The original contributions presented in the study are included in the article/Supplementary material, further inquiries can be directed to the corresponding author.
Ethics statement
Ethical approval was not required for the study involving animals in accordance with the local legislation and institutional requirements because archaeological faunal remains of deceased Pleistocene specimens were sampled for this study with full permission from the Braunschweigisches Landesmuseum, Wolfenbüttel.
Author contributions
PH-S: Conceptualization, Formal analysis, Investigation, Methodology, Project administration, Visualization, Writing – original draft, Writing – review & editing. SB: Formal analysis, Investigation, Software, Visualization, Writing – review & editing. AP: Visualization, Writing – review & editing. ML: Formal analysis, Writing – review & editing. ES: Formal analysis, Writing – review & editing. PL: Formal analysis, Methodology, Writing – review & editing. PR: Conceptualization, Investigation, Methodology, Resources, Supervision, Writing – review & editing.
Funding
The author(s) declare financial support was received for the research, authorship, and/or publication of this article. The project was funded by the Max Planck Society.
Acknowledgments
We thank B. Ludowici, Braunschweigisches Landesmuseum, Wolfenbüttel, for permission to study this material and M. Scheffler for providing access to the collections. We thank C. Pasda, Friedrich Schiller University, for his comments on the manuscript.
Conflict of interest
The authors declare that the research was conducted in the absence of any commercial or financial relationships that could be construed as a potential conflict of interest.
The author(s) declared that they were an editorial board member of Frontiers, at the time of submission. This had no impact on the peer review process and the final decision.
Publisher's note
All claims expressed in this article are solely those of the authors and do not necessarily represent those of their affiliated organizations, or those of the publisher, the editors and the reviewers. Any product that may be evaluated in this article, or claim that may be made by its manufacturer, is not guaranteed or endorsed by the publisher.
Supplementary material
The Supplementary Material for this article can be found online at: https://www.frontiersin.org/articles/10.3389/fearc.2024.1458040/full#supplementary-material
References
Alerstam, T., Hedenström, A., and Åkesson, S. (2003). Long-distance migration: evolution and determinants. Oikos 103, 247–260. doi: 10.1034/j.1600-0706.2003.12559.x
Amante, C., and Eakins, B. W. (2009). “ETOPO1 1 arc-minute global relief model: procedures, data sources and analysis [WWW Document],” in NOAA Technical Memorandum NESDIS NGDC-24.
Avgar, T., Street, G., and Fryxell, J. M. (2014). On the adaptive benefits of mammal migration. Can. J. Zool. 92, 481–490. doi: 10.1139/cjz-2013-0076
Baales, M. (1996). “Umwelt und Jagdökonomie der Ahrensburger Rentierjäger im Mittelgebirge,” in Monographien Des RGZM 38. Mainz: Verlag des Römisch-Germanischen Zentralmuseums.
Baales, M. (1999). “Economy and Seasonality in the Ahrensburgian,” in Tanged Points Cultures in Europe. Lubelskie Materialy Archeologiczne, eds. S. K. Kozłowski, J. Gruba, L. L. Zaliznya, L. L.Wydawwnictwo (Lublin: Uniwersytetu Marii Curie-Skłodowskiej), 64–75.
Baales, M. (2021). “Tracking the reindeer: the upper and final pleistocene record of reindeer in the northern uplands and its seasonal implications,” in The Beef behind All Possible Pasts. The Tandem Festschrift in Honour of Elaine Turner and Martin Street (Monographien Des RGZM, Band 157.2), eds. S. Gaudzinski-Windheuser, O. Jöris (Heidelberg: Propylaeum), 609–638.
Bahloul, K., Pereladova, O. B., Soldatova, N., Fisenko, G., Sidorenko, E., and Sempéré, A. J. (2001). Social organization and dispersion of introduced kulans (Equus hemionus kulan) and Przewalski horses (Equus przewalski) in the Bukhara Reserve, Uzbekistan. J. Arid Environ. 47, 309–323. doi: 10.1006/jare.2000.0714
Bahn, P. G. (1977). Seasonal migration in South-west France during the late glacial period. J. Archaeol. Sci. 4, 245–257. doi: 10.1016/0305-4403(77)90092-9
Balasse, M. (2002). Reconstructing dietary and environmental history from enamel isotopic analysis: time resolution of intra-tooth sequential sampling. Int. J. Osteoarchaeol. 12, 155–165. doi: 10.1002/oa.601
Barakat, S., Le Corre, M., Willmes, M., Cohen, J., Vuillien, M., Desclaux, E., et al. (2023). Laser ablation strontium isotopes and spatial assignment show seasonal mobility in red deer (Cervus elaphus) at Lazaret Cave, France (MIS 6). Front. Ecol. Evol. 11, 1–14. doi: 10.3389/fevo.2023.988837
Bataille, C. P., and Bowen, G. J. (2012). Mapping 87Sr/86Sr variations in bedrock and water for large scale provenance studies. Chem. Geol. 304–305, 39–52. doi: 10.1016/j.chemgeo.2012.01.028
Bataille, C. P., Crowley, B. E., Wooller, M. J., and Bowen, G. J. (2020). Advances in global bioavailable strontium isoscapes. Palaeogeogr. Palaeoclimatol. Palaeoecol. 555:109849. doi: 10.1016/j.palaeo.2020.109849
Bataille, C. P., Jaouen, K., Milano, S., Trost, M., Steinbrenner, S., Crubézy, É., et al. (2021). Triple sulfur-oxygen-strontium isotopes probabilistic geographic assignment of archaeological remains using a novel sulfur isoscape of western Europe. PLoS ONE 16:e0250383. doi: 10.1371/journal.pone.0250383
Bataille, C. P., von Holstein, I. C. C., Laffoon, J. E., Willmes, M., Liu, X. M., and Davies, G. R. (2018). A bioavailable strontium isoscape for Western Europe: a machine learning approach. PLoS ONE 13:e0197386. doi: 10.1371/journal.pone.0197386
Batchelor, C. L., Margold, M., Krapp, M., Murton, D. K., Dalton, A. S., Gibbard, P. L., et al. (2019). The configuration of Northern Hemisphere ice sheets through the quaternary. Nat. Commun. 10, 3713. doi: 10.1038/s41467-019-11601-2
Ben-David, M., Shochat, E., and Adams, L. G. (2001). Utility of stable isotope analysis in studying foraging ecology of herbivores: examples from moose and caribou. Alces 37, 421–434. doi: 10.1644/11-MAMM-S-166.1
Bendrey, R., Vella, D., Zazzo, A., Balasse, M., and Lepetz, S. (2015). Exponentially decreasing tooth growth rate in horse teeth: implications for isotopic analyses. Archaeometry 57, 1104–1124. doi: 10.1111/arcm.12151
Bentley, R. A. (2006). Strontium isotopes from the earth to the archaeological skeleton: a review. J. Archaeol. Method Theory. 13, 135–187.
Bergerud, A. T. (1967). Management of labrador caribou. J. Wildl. Manage. 31:621. doi: 10.2307/3797966
Bergerud, A. T., and Luttich, S. N. (2003). Predation risk and optimal foraging trade-off in the demography and spacing of the George River Herd, 1958 to 1993. Rangifer 23:169. doi: 10.7557/2.23.5.1699
Blasco, R., Cochard, D., Colonese, A. C., Laroulandie, V., Meier, J., Morin, E., et al. (2022). Small animal use by Neanderthals, in Updating Neanderthals, eds. F. Romagnoli, F. Florent Rivals, S. Benazzi (London: Elsevier), 123–143.
Bocherens, H. (2003). Isotopic biogeochemistry and the palaeoecology of the mammoth steppe fauna. Deinsea 9, 57–76.
Bocherens, H. (2015). Isotopic tracking of large carnivore palaeoecology in the mammoth steppe. Quat. Sci. Rev. 117, 42–71. doi: 10.1016/j.quascirev.2015.03.018
Bocherens, H., Billiou, D., Mariotti, A., Patou-Mathis, M., Otte, M., Bonjean, D., et al. (1999). Palaeoenvironmental and palaeodietary implications of isotopic biogeochemistry of last interglacial neanderthal and mammal bones in Scladina Cave (Belgium). J. Archaeol. Sci. 26, 599–607. doi: 10.1006/jasc.1998.0377
Bocherens, H., Billiou, D., Patou-Mathis, M., Bonjean, D., Otte, M., and Mariotti, A. (1997). Paleobiological implications of the isotopic signatures (13C, 15N) of fossil mammal collagen in Scladina Cave (Sclayn, Belgium). Quat. Res. 48, 370–380. doi: 10.1006/qres.1997.1927
Bosinski, G. (1963). Eine mittelpalaeolithische formengruppe und das problem ihrer geochronologischen einordnung. Eiszeitalter und Gegenwart 14, 124–140. doi: 10.3285/eg.14.1.14
Bowen, G. J., and Good, S. P. (2015). Incorporating water isoscapes in hydrological and water resource investigations. WIREs Water 2, 107–119. doi: 10.1002/wat2.1069
Britton, K. (2010). Multi-Isotope Analysis and the Reconstruction of Prey Species Palaeomigrations and Palaeoecology (PhD thesis).
Britton, K. (2018). “Prey species movements and migrations in ecocultural landscapes: reconstructing late Pleistocene herbivore seasonal spatial behaviours,” in Multi-Species Archaeology, S. Pilaar-Birch (London: Routledge), 347–367. doi: 10.4324/9781315707709-21
Britton, K., Gaudzinski-Windheuser, S., Roebroeks, W., Kindler, L., and Richards, M. P. (2012). Stable isotope analysis of well-preserved 120,000-year-old herbivore bone collagen from the Middle Palaeolithic site of Neumark-Nord 2, Germany reveals niche separation between bovids and equids. Palaeogeogr. Palaeoclimatol. Palaeoecol. 333–334, 168–177. doi: 10.1016/j.palaeo.2012.03.028
Britton, K., Grimes, V., Dau, J., and Richards, M. P. (2009). Reconstructing faunal migrations using intra-tooth sampling and strontium and oxygen isotope analyses: a case study of modern caribou (Rangifer tarandus granti). J. Archaeol. Sci. 36, 1163–1172. doi: 10.1016/j.jas.2009.01.003
Britton, K., Grimes, V., Niven, L., Steele, T. E., McPherron, S., Soressi, M., et al. (2011). Strontium isotope evidence for migration in late Pleistocene Rangifer: Implications for Neanderthal hunting strategies at the Middle Palaeolithic site of Jonzac, France. J. Hum. Evol. 61, 176–185. doi: 10.1016/j.jhevol.2011.03.004
Britton, K., Jimenez, E.-L., Le Corre, M., Pederzani, S., Daujeard, C., Jaouen, K., et al. (2023a). Multi-isotope zooarchaeological investigations at Abri du Maras: the paleoecological and paleoenvironmental context of Neanderthal subsistence strategies in the Rhône Valley during MIS 3. J. Hum. Evol. 174:103292. doi: 10.1016/j.jhevol.2022.103292
Britton, K., Jimenez, E.-L., Le Corre, M., Renou, S., Rendu, W., Richards, M. P., et al. (2023b). Multi-isotope analysis of bone collagen of Late Pleistocene ungulates reveals niche partitioning and behavioural plasticity of reindeer during MIS 3. Sci. Rep. 13:15722. doi: 10.1038/s41598-023-42199-7
Britton, K., McManus-Fry, E., Nehlich, O., Richards, M., Ledger, P. M., and Knecht, R. (2018). Stable carbon, nitrogen and sulphur isotope analysis of permafrost preserved human hair from rescue excavations (2009, 2010) at the precontact site of Nunalleq, Alaska. J. Archaeol. Sci. Rep. 17, 950–963. doi: 10.1016/j.jasrep.2016.04.015
Brown, W. A. B., and Chapman, N. G. (1991a). The dentition of red deer (Cervus elaphus): a scoring scheme to assess age from wear of the permanent molariform teeth. J. Zool. 224, 519–536. doi: 10.1111/j.1469-7998.1991.tb03783.x
Brown, W. A. B., and Chapman, N. G. (1991b). Age assessment of fallow deer (Dama dama): from a scoring scheme based on radiographs of developing permanent molariform teeth. J. Zool. 224, 367–379. doi: 10.1111/j.1469-7998.1991.tb06031.x
Brown, W. K., Huot, J., Lamothe, P., Luttich, S., Par,é, M., Martin, G., et al. (1986). The distribution and movement patterns of four woodland caribou herds in Quebec and Labrador. Rangifer 6:43. doi: 10.7557/2.6.2.581
Bryant, J. D., and Froelich, P. N. (1995). A model of oxygen isotope fractionation in body water of large mammals. Geochim. Cosmochim. Acta 59, 4523–4537. doi: 10.1016/0016-7037(95)00250-4
Bryant, J. D., Froelich, P. N., Showers, W. J., and Genna, B. J. (1996). A tale of two quarries: biologic and taphonomic signatures in the oxygen isotope composition of tooth enamel phosphate from modern and miocene equids. Palaios 11:397. doi: 10.2307/3515249
Burke, A., and Pike-Tay, A. (1997). “Reconstructing “l'Age du Renne,”” in Caribou/Reindeer Hunters of the Northern Hemisphere (London Avebury Press), 69–81.
Cain, J. W., Krausman, P. R., Rosenstock, S. S., and Turner, J. C. (2006). Mechanisms of thermoregulation and water balance in desert ungulates. Wildl. Soc. Bull. 34, 570–581. doi: 10.2193/0091-7648(2006)34[570:MOTAWB]2.0.CO;2
Capo, R. C., Stewart, B. W., and Chadwick, O. A. (1998). Strontium isotopes as tracers of ecosystem processes: theory and methods. Geoderma 82, 197–225. doi: 10.1016/S0016-7061(97)00102-X
Caspers, G., and Freund, H. (2001). Vegetation and climate in the Early- and Pleni-Weichselian in northern Central Europe. J. Quat. Sci. 16, 31–48. doi: 10.1002/1099-1417(200101)16:1<31::AID-JQS577>3.0.CO;2-3
Cerling, T. E., and Harris, J. M. (1999). Carbon isotope fractionation between diet and bioapatite in ungulate mammals and implications for ecological and paleoecological studies. Oecologia 120, 347–363. doi: 10.1007/s004420050868
Cernusak, L. A., Barbour, M. M., Arndt, S. K., Cheesman, A. W., English, N. B., Feild, T. S., et al. (2016). Stable isotopes in leaf water of terrestrial plants. Plant Cell Environ. 39, 1087–1102. doi: 10.1111/pce.12703
Cichowski, D., Kinley, T., and Churchill, B. (2004). Caribou, Rangifer tarandus: Identified Wildlife Management Strategy Accounts and Measures for Managing Identified Wildlife Northern Interior Forest Region. British Columbia.
Copeland, S. R., Sponheimer, M., le Roux, P. J., Grimes, V., Lee-Thorp, J. A., de Ruiter, D. J., et al. (2008). Strontium isotope ratios (87Sr/86Sr) of tooth enamel: a comparison of solution and laser ablation multicollector inductively coupled plasma mass spectrometry methods. Rapid Commun. Mass Spectrom. 22, 3187–3194. doi: 10.1002/rcm.3717
Costamagno, S., Liliane, M., Cédric, B., Bernard, V., and Bruno, M. (2006). Les Pradelles (Marillac-le-Franc, France): a mousterian reindeer hunting camp? J. Anthropol. Archaeol. 25, 466–484. doi: 10.1016/j.jaa.2006.03.008
Crane, K. K., Smith, M. A., and Reynolds, D. (1997). Habitat selection patterns of feral horses in Southcentral Wyoming. J. Range Manage. 50:374. doi: 10.2307/4003303
Crowley, B. E., Miller, J. H., and Bataille, C. P. (2017). Strontium isotopes (87Sr/86Sr) in terrestrial ecological and palaeoecological research: empirical efforts and recent advances in continental-scale models. Biol. Rev. 92, 43–59. doi: 10.1111/brv.12217
Dansgaard, W. (1964). Stable isotopes in precipitation. Tellus 16, 436–468. doi: 10.1111/j.2153-3490.1964.tb00181.x
Daujeard, C., Vettese, D., Britton, K., Béarez, P., Boulbes, N., Crégut-Bonnoure, E., et al. (2019). Neanderthal selective hunting of reindeer? The case study of Abri du Maras (south-eastern France). Archaeol. Anthropol. Sci. 11, 985–1011. doi: 10.1007/s12520-017-0580-8
Delagnes, A., and Rendu, W. (2011). Shifts in Neandertal mobility, technology and subsistence strategies in western France. J. Archaeol. Sci. 38, 1771–1783. doi: 10.1016/j.jas.2011.04.007
Delpech, F. (1987). “L'environnement animal des magdaléniens,” in Le Magdalénien En Europe. (Liège: Actes Du Colloque de Mayence, XI° Congrès U.I.S.P.P. Études et Recherches Archéologiques de l'Université de Liège), 5–30.
DeNiro, M. J., and Epstein, S. (1978). Influence of diet on the distribution of carbon isotopes in animals. Geochim. Cosmochim. Acta 42, 495–506. doi: 10.1016/0016-7037(78)90199-0
Discamps, E., Jaubert, J., and Bachellerie, F. (2011). Human choices and environmental constraints: deciphering the variability of large game procurement from Mousterian to Aurignacian times (MIS 5-3) in southwestern France. Quat. Sci. Rev. 30, 2755–2775. doi: 10.1016/j.quascirev.2011.06.009
Dongmann, G., Nürnberg, H. W., Förstel, H., and Wagener, K. (1974). On the enrichment of H2 18O in the leaves of transpiring plants. Radiat. Environ. Biophys. 11, 41–52. doi: 10.1007/BF01323099
Drucker, D., Bocherens, H., Pike-Tay, A., and Mariotti, A. (2001). Isotopic tracking of seasonal dietary change in dentine collagen: preliminary data from modern caribou. Earth Planetary Sci. 333, 303–309. doi: 10.1016/S1251-8050(01)01640-8
Drucker, D. G. (2022). The isotopic ecology of the mammoth steppe. Annu. Rev. Earth Planet. Sci. 50, 395–418. doi: 10.1146/annurev-earth-100821-081832
Drucker, D. G., Bocherens, H., and Billiou, D. (2003). Evidence for shifting environmental conditions in Southwestern France from 33 000 to 15 000 years ago derived from carbon-13 and nitrogen-15 natural abundances in collagen of large herbivores. Earth Planet. Sci. Lett. 216, 163–173. doi: 10.1016/S0012-821X(03)00514-4
Drucker, D. G., Bridault, A., and Cupillard, C. (2012). Environmental context of the Magdalenian settlement in the Jura Mountains using stable isotope tracking (13C, 15N, 34S) of bone collagen from reindeer (Rangifer tarandus). Quat. Int. 272–273, 322–332. doi: 10.1016/j.quaint.2012.05.040
Drucker, D. G., Bridault, A., Hobson, K. A., Szuma, E., and Bocherens, H. (2008). Can carbon-13 in large herbivores reflect the canopy effect in temperate and boreal ecosystems? Evidence from modern and ancient ungulates. Palaeogeogr. Palaeoclimatol. Palaeoecol. 266, 69–82. doi: 10.1016/j.palaeo.2008.03.020
Ehleringer, J. R., and Monson, R. K. (1993). Evolutionary and ecological aspects of photosynthetic pathway variation. Annu. Rev. Ecol. Syst. 24, 411–439. doi: 10.1146/annurev.es.24.110193.002211
Ehlers, L., Palm, E., Herriges, J., Bentzen, T., Suitor, M., Joly, K., et al. (2024). A taste of space: Remote animal observations and discrete-choice models provide new insights into foraging and density dynamics for a large subarctic herbivore. J. Anim. Ecol. 93, 891–905. doi: 10.1111/1365-2656.14109
Eissmann, L. (2002). Quaternary geology of eastern Germany (Saxony, Saxon–Anhalt, South Brandenburg, Thu.ringia), type area of the Elsterian and Saalian Stages in Europe. Quat. Sci. Rev. 21, 1275–1346. doi: 10.1016/S0277-3791(01)00075-0
Evans, J. A., Montgomery, J., Wildman, G., and Boulton, N. (2010). Spatial variations in biosphere 87Sr/86Sr in Britain. J. Geol. Soc. London. 167, 1–4. doi: 10.1144/0016-76492009-090
Farquhar, G. D., Ehleringer, J. R., and Hubick, K. T. (1989a). Carbon isotope discrimination and photosynthesis. Annu. Rev. Plant Physiol. Plant Mol. Biol. 40, 503–537. doi: 10.1146/annurev.pp.40.060189.002443
Farquhar, G. D., Hubick, K. T., Condon, A. G., and Richards, R. A. (1989b). Carbon Isotope Fractionation and Plant Water-Use Efficiency. New York: Springer.
Feranec, R. S., and MacFadden, B. J. (2006). Isotopic discrimination of resource partitioning among ungulates in C 3 -dominated communities from the Miocene of Florida and California. Paleobiology 32, 191–205. doi: 10.1666/05006.1
Ferguson, S. H., and Elkie, P. C. (2004). Seasonal movement patterns of woodland caribou (Rangifer tarandus caribou). J. Zool. 262, 125–134. doi: 10.1017/S0952836903004552
Fizet, M., Mariotti, A., Bocherens, H., Lange-Badr,é, B., Vandermeersch, B., Borel, J. P., et al. (1995). Effect of diet, physiology and climate on carbon and nitrogen stable isotopes of collagen in a late pleistocene anthropic palaeoecosystem: Marillac, Charente, France. J. Archaeol. Sci. 22, 67–79. doi: 10.1016/S0305-4403(95)80163-4
Flanagan, L. B., Bain, J. F., and Ehleringer, J. R. (1991). Stable oxygen and hydrogen isotope composition of leaf water in C3 and C4 plant species under field conditions. Oecologia 88, 394–400. doi: 10.1007/BF00317584
Fontana, L. (2000). La chasse au renne au Paléolithique supérieur dans le sud-ouest de la France : nouvelles hypothèses de travail/Reindeer hunting in the Upper Palaeolithic in south-west France : demography and seasonality. Paléo 12, 141–164. doi: 10.3406/pal.2000.1600
Fontana, L. (2012). “Les relations homme-animal dans les sociétés de chasseurs-collecteurs,” in L'archéologie à Découvert (Paris: CNRS Éditions), 72–78.
Fontana, L. (2017). The four seasons of reindeer: non-migrating reindeer in the Dordogne region (France) between 30 and 18 k? Data from the Middle and Upper Magdalenian at La Madeleine and methods of seasonality determination. J. Archaeol. Sci. Rep. 12, 346–362. doi: 10.1016/j.jasrep.2017.02.012
Fontana, L. (2022). Reindeer Hunters of the Ice Age in Europe. Cham: Springer International Publishing.
Fricke, H. C., and O'Neil, J. R. (1999). The correlation between 18O/16O ratios of meteoric water and surface temperature: its use in investigating terrestrial climate change over geologic time. Earth Planet. Sci. Lett. 170, 181–196. doi: 10.1016/S0012-821X(99)00105-3
Gat, J. R. (1980). “The isotopes of hydrogen and oxygen in precipitation,” in Handbook of Environmental Isotope Geochemistry, eds. P. Fritz, and J. C. Fontes (Elsevier, Amsterdam), 21–47. doi: 10.1016/B978-0-444-41780-0.50007-9
Gat, J. R. (1995). “Stable isotopes of fresh and Saline Lakes,” in Physics and Chemistry of Lakes (Berlin: Springer Berlin Heidelberg), 139–165.
Gat, J. R. (2010). Isotope Hydrology: A Study of the Water Cycle, Environmental Science and Management. London: Imperila College Press.
Gaudzinski, S. (1998). Knochen und Knochengeräte der mittelpaläolithischen Fundstelle Salzgitter-Lebenstedt (Deutschland). Jahrbuch des Römisch-Germanischen Zentralmuseums 45, 163–220.
Gaudzinski, S. (1999). Middle Palaeolithic bone tools from the open-air site Salzgitter-Lebenstedt (Germany). J. Archaeol. Sci. 26, 125–141. doi: 10.1006/jasc.1998.0311
Gaudzinski, S. (2000). On the variability of Middle Palaeolithic procurement tactics: the case of Salzgitter-Lebenstedt, Northern Germany. Int. J. Osteoarchaeol. 10, 396–406.3. doi: 10.1002/1099-1212(200009/10)10:5<396::AID-OA559>3.0.CO;2-Z
Gaudzinski, S. (2005). “Monospecific or species-dominated faunal assemblages during the Middle Paleolithic in Europe,” in Transitions Before the Transition. Evolution and Stability in the Middle Palaeolithic and Middle Stone Age, eds. E. Hovers, and S. Kuhn (New York: Springer), 137–147. doi: 10.1007/0-387-24661-4_8
Gaudzinski, S., and Roebroeks, W. (2000). Adults only. Reindeer hunting at the Middle Palaeolithic site Salzgitter Lebenstedt, northern Germany. J. Hum. Evol. 38, 497–521. doi: 10.1006/jhev.1999.0359
Gaudzinski-Windheuser, S. (2021). Recapitulating zoo archaeology at Salzgitter-Lebenstedt: Current state of research and perspectives, in The Beef behind All Possible Pasts. The Tandem-Festschritt in Honour of Elaine Turner and Martin Street, S. Gaudzinski-Windheuser, and O. Jöris (Mainz: Monographien Des RGZM 157), 137–172.
Gaudzinski-Windheuser, S., and Niven, L. (2009). “Hominin subsistence patterns during the middle and late paleolithic in Northwestern Europe,” in Vertebrate Paleobiology and Paleoanthropology (Cham: Springer), 99–111.
Gigleux, C., Grimes, V., Tütken, T., Knecht, R., and Britton, K. (2019). Reconstructing caribou seasonal biogeography in Little Ice Age (late Holocene) Western Alaska using intra-tooth strontium and oxygen isotope analysis. J. Archaeol. Sci. Rep. 23, 1043–1054. doi: 10.1016/j.jasrep.2017.10.043
Girard, T. L., Bork, E. W., Nielsen, S. E., and Alexander, M. J. (2013). Seasonal variation in habitat selection by free-ranging feral horses within Alberta's forest reserve. Rangel Ecol. Manag. 66, 428–437. doi: 10.2111/REM-D-12-00081.1
Glassburn, C. L., Potter, B. A., Clark, J. L., Reuther, J. D., Bruning, D. L., and Wooller, M. J. (2018). Strontium and oxygen isotope profiles of sequentially sampled modern bison (Bison bison bison) teeth from interior alaska as proxies of seasonal mobility. Arctic 71, 183–200. doi: 10.14430/arctic4718
Gonfiantini, R. (1986). “Environmental isotopes in lake studies,” in Handbook of Environmental Isotope Geochemistry; The Terrestrial Environment, B, P. Fritz, and J. C. Fontes (New York: Elsevier Science Publishing Company Incorporated), 113–168.
Gonfiantini, R., Gratziu, S., and Tongiorgi, E. (1965). “Oxygen isotopic composition of water in leaves,” in Use of Isotopes and Radiation in Soil–Plant Nutrition Studies (Vienna: IAEA), 405–410.
Gordon, B. G. (1988). Of Men and Reindeer Herds in French Magdalenian Prehistory. Oxford: BAR International Series 390.
Grayson, D. K., and Delpech, F. (2002). Specialized early Upper Palaeolithic hunters in southwestern France? J. Archaeol. Sci. 29, 1439–1449. doi: 10.1006/jasc.2002.0806
Grote, K., and Preul, F. (1978). Der mittelpaläolithische Lagerplatz in Salzgitter-Lebenstedt. Vorbericht über die Grabung und die geologische Untersuchung 1977 I. Archäologischer Vorbericht. Nachrichten aus Niedersachsens Urgeschichte 47, 77–100.
Gurarie, E., Hebblewhite, M., Joly, K., Kelly, A. P., Adamczewski, J., Davidson, S. C., et al. (2019). Tactical departures and strategic arrivals: divergent effects of climate and weather on caribou spring migrations. Ecosphere 10:2971. doi: 10.1002/ecs2.2971
Guthrie, R. D. (2001). Origin and causes of the mammoth steppe: a story of cloud cover, woolly mammal tooth pits, buckles, and inside-out Beringia. Quat. Sci. Rev. 20, 549–574. doi: 10.1016/S0277-3791(00)00099-8
Haase, D., Fink, J., Haase, G., Ruske, R., Pecsi, M., Richter, H., et al. (2007). Loess in Europe- its spatial distribution based on a European Loess Map, scale 1:2,500,000. Quat. Sci. Rev. 26, 1301–1312. doi: 10.1016/j.quascirev.2007.02.003
Heaton, T. H. E. (1999). Spatial, species, and temporal variations in the 13 C/12 C ratios of C 3 plants: implications for palaeodiet studies. J. Archaeol. Sci. 26, 637–649. doi: 10.1006/jasc.1998.0381
Heddell-Stevens, P., Britton, K., Jöris, O., Matthies, T., Lucas, M., Scott, E., et al. (2024). Multi-isotope reconstruction of Late Pleistocene large-herbivore biogeography and mobility patterns. Commun. Biol. 7, 1–14. doi: 10.1038/s42003-024-06233-2
Hinton, M. (1978). On the watering of horses: a review. Equine Vet. J. 10, 27–31. doi: 10.1111/j.2042-3306.1978.tb02210.x
Holt, E., Evans, J. A., and Madgwick, R. (2021). Strontium (87Sr/86Sr) mapping: a critical review of methods and approaches. Earth Sci. Rev. 216. doi: 10.1016/j.earscirev.2021.103593
Hoogewerff, J. A., Reimann, C., Ueckermann, H., Frei, R., Frei, K. M., van Aswegen, T., et al. (2019). Bioavailable 87 Sr/86 Sr in European soils: a baseline for provenancing studies. Sci. Total Environm. 672, 1033–1044. doi: 10.1016/j.scitotenv.2019.03.387
Hoppe, K. A., Koch, P. L., Carlson, R. W., and Webb, S. D. (1999). Tracking mammoths and mastodons: Reconstruction of migratory behavior using strontium isotope ratios. Geology 27, 439.
Hoppe, K. A., Stover, S. M., Pascoe, J. R., and Amundson, R. (2004). Tooth enamel biomineralization in extant horses: Implications for isotopic microsampling. Palaeogeogr. Palaeoclimatol. Palaeoecol. 206, 355–365. doi: 10.1016/j.palaeo.2004.01.012
Hublin, J. J. (1984). The fossil man from Salzgitter-Lebenstedt (FRG) and its place in human evolution during the Pleistocene in Europe. Z. Morphol. Anthropol. 75, 45–56. doi: 10.1127/zma/75/1984/45
Iacumin, P., Nikolaev, V., and Ramigni, M. (2000). C and N stable isotope measurements on Eurasian fossil mammals, 40 000 to 10 000 years BP: Herbivore physiologies and palaeoenvironmental reconstruction. Palaeogeogra. Palaeoclimatol Palaeoecol. 163, 33–47. doi: 10.1016/S0031-0182(00)00141-3
Jeelani, G., Shah, R. A., Jacob, N., and Deshpande, R. D. (2017). Estimation of snow and glacier melt contribution to Liddar stream in a mountainous catchment, western Himalaya: an isotopic approach. Isotopes Environ. Health Stud. 53, 18–35. doi: 10.1080/10256016.2016.1186671
Joly, K., Cameron, M. D., and White, R. G. (2024). Behavioral adaptation to seasonal resource scarcity by Caribou (Rangifer tarandus) and its role in partial migration. J. Mammal. 1–9. doi: 10.1093/jmammal/gyae100
Joly, K., Gunn, A., Côté, S. D., Panzacchi, M., Adamczewski, J., Suitor, M. J., et al. (2021a). Caribou and reindeer migrations in the changing Arctic. Animal Migration 8, 156–167. doi: 10.1515/ami-2020-0110
Joly, K., Gurarie, E., Hansen, D. A., and Cameron, M. D. (2021b). Seasonal patterns of spatial fidelity and temporal consistency in the distribution and movements of a migratory ungulate. Ecol. Evol. 11, 8183–8200. doi: 10.1002/ece3.7650
Jöris, O. (2004). Zur chronostratigraphischen Stellung der spätmittelpaläolithischen Keilmessergruppen. Der Versuch einer kulturgeographischen Abgrenzung einer mittelpaläolithischen Formengruppe in ihrem europäischen Kontext. Berichte der Römisch- Germanischen Kommission 84, 49–153.
Jöris, O., Neruda, P., Wiśniewski, A., and Weiss, M. (2022). The late and final middle palaeolithic of Central Europe and its contributions to the formation of the regional upper palaeolithic: a review and a synthesis. J. Paleolithic Archaeol. 5, 1–55. doi: 10.1007/s41982-022-00126-8
Julien, M. A., Bocherens, H., Burke, A., Drucker, D. G., Patou-Mathis, M., Krotova, O., et al. (2012). Were European steppe bison migratory? 18O, 13C and Sr intra-tooth isotopic variations applied to a palaeoethological reconstruction. Quat. Int. 271, 106–119. doi: 10.1016/j.quaint.2012.06.011
Julien, M. A., Rivals, F., Serangeli, J., Bocherens, H., and Conard, N. J. (2015). A new approach for deciphering between single and multiple accumulation events using intra-tooth isotopic variations: application to the Middle Pleistocene bone bed of Schöningen 13 II-4. J. Hum. Evol. 89, 114–128. doi: 10.1016/j.jhevol.2015.02.012
Käßner, A., Kalapurakkal, H. T., Huber, B., and Tichomirowa, M. (2023). A new water-based 87Sr/86Sr isoscape map of central and ne germany, with special emphasis on mountainous regions. Aquat. Geochem. 29, 95–125. doi: 10.1007/s10498-023-09412-5
Kierdorf, H., and Witzel, C. (2017). “Bestimmung der Todessaison durch Analyse der Zementzonierung an Rentierzähnen der paläolithischen Fundstätte Salzgitter- Lebenstedt, Norddeutschland,” in Die Tierknochenfunde Der Mittelpaläolithischen Jägerstation von Salzgitter- Lebenstedt, eds. B. Ludovici, and H. Pöppelmann (Braunschweig: Forschungen und Berichte des Braunschweigischen Landesmuseums Neue Folge 1), 197–200.
King, S. R. B. (2002). Home range and habitat use of free-ranging Przewalski horses at Hustai National Park, Mongolia. Appl. Anim. Behav. Sci. 78, 103–113. doi: 10.1016/S0168-1591(02)00087-4
Klein, R. (1992). Comparative ecological and behavioral adaptations of Ovibos moschatus and Rangifer tarandus. Rangifer 12, 47–55. doi: 10.7557/2.12.2.1016
Kleinschmidt, A. (1953). Die Zoologischen Funde der Grabung Salzgitter-Lebenstedt. Eiszeitalter und Gegenwart 3, 166–188.
Koch, P. L., Tuross, N., and Fogel, M. L. (1997). The effects of sample treatment and diagenesis on the isotopic integrity of carbonate in biogenic hydroxylapatite. J. Archaeol. Sci. 24, 417–429. doi: 10.1006/jasc.1996.0126
Kohn, M. J. (1996). Predicting animal δ18O: accounting for diet and physiological adaptation. Geochim. Cosmochim. Acta 60, 4811–4829. doi: 10.1016/S0016-7037(96)00240-2
Kohn, M. J., Schoeninger, M. J., and Valley, J. W. (1996). Herbivore tooth oxygen isotope compositions: effects of diet and physiology. Geochim. Cosmochim. Acta 60, 3889–3896. doi: 10.1016/0016-7037(96)00248-7
Kohn, M. J., and Welker, J. M. (2005). On the temperature correlation of δ18O in modern precipitation. Earth Planet. Sci. Lett. 231, 87–96. doi: 10.1016/j.epsl.2004.12.004
Kootker, L. M., van Lanen, R. J., Kars, H., and Davies, G. R. (2016). Strontium isoscapes in The Netherlands. Spatial variations in 87Sr/86Sr as a proxy for palaeomobility. J. Archaeol. Sci. Rep. 6, 1–13. doi: 10.1016/j.jasrep.2016.01.015
Koster, E. A. (2005). Recent advances in luminescence dating of Late Pleistocene (cold-climate) aeolian sand and loess deposits in western Europe. Permafr. Periglac Proc. 16, 131–143. doi: 10.1002/ppp.512
Kovács, J., Moravcová, M., Újvári, G., and Pintér, A.G. (2012). Reconstructing the paleoenvironment of East Central Europe in the Late Pleistocene using the oxygen and carbon isotopic signal of tooth in large mammal remains. Quat. Int. 276–277, 145–154. doi: 10.1016/j.quaint.2012.04.009
Krönneck, P., and Staesche, U. (2017). “Die Jagdstation der Neandertaler von Salzgitter-Lebenstedt, Norddeutschland. Entdeckung, Untersuchung, Dokumentation 13–40,” in Die Tierknochenfunde Der Mittelpaläolithischen Jägerstation von Salzgitter-Lebenstedt. Forschungen Und Berichte Des Braunschweigischen Landesmuseum – Neu Folge – Band 1, B. Ludowici, and H. Pöppelmann (Braunschweig: Braunschweigischen Landesmuseum), 13–40.
Kuntz, D., and Costamagno, S. (2011). Relationships between reindeer and man in southwestern France during the Magdalenian. Quat. Int. 238, 12–24. doi: 10.1016/j.quaint.2010.10.023
Lacorre, F. (1956). Les migrations des Rennes dans la Province Préhistorique des Eyzies. Bulletin de la Société préhistorique de France 53, 302–310. doi: 10.3406/bspf.1956.3336
Lang, J., Lauer, T., and Winsemann, J. (2018). New age constraints for the Saalian glaciation in northern central Europe: implications for the extent of ice sheets and related proglacial lake systems. Quat. Sci. Rev. 180, 240–259. doi: 10.1016/j.quascirev.2017.11.029
Le Corre, M., Dussault, C., and Côté, S. D. (2014). Detecting changes in the annual movements of terrestrial migratory species: using the first-passage time to document the spring migration of caribou. Mov Ecol 2. doi: 10.1186/s40462-014-0019-0
Le Corre, M., Dussault, C., and Côté, S. D. (2016). Weather conditions and variation in timing of spring and fall migrations of migratory caribou. J. Mammal. 98:gyw177. doi: 10.1093/jmammal/gyw177
Le Corre, M., Grimes, V., Lam, R., and Britton, K. (2023). Comparison between strip sampling and laser ablation methods to infer seasonal movements from intra-tooth strontium isotopes profiles in migratory caribou. Sci. Rep. 13:3621. doi: 10.1038/s41598-023-30222-w
Lee-Thorp, J., Likius, A., Mackaye, H. T., Vignaud, P., Sponheimer, M., and Brunet, M. (2012). Isotopic evidence for an early shift to C4 resources by Pliocene hominins in Chad. Proc. Nat. Acad. Sci. 109:20369–20372. doi: 10.1073/pnas.1204209109
Lee-Thorp, J. A. (2000). Preservation of Biogenic Carbon Isotopic Signals in Plio-Pleistocene Bone and Tooth Mineral, in: Ambrose, S.H., Katzenberg, A.M. (Eds.), Biogeochemical Approaches to Paleodietary Analysis. Kluwer Academic/Plenum Publishers, New York, pp. 89–115. doi: 10.1007/0-306-47194-9_5
Lee-Thorp, J. A., Sponheimer, M., Passey, B. H., De Ruiter, D. J., and Cerling, T. E. (2010). Stable isotopes in fossil hominin tooth enamel suggest a fundamental dietary shift in the Pliocene. Philos. Trans. Royal Soc. B: Biol. Sci. 365, 3389–3396. doi: 10.1098/rstb.2010.0059
Lehmkuhl, F., Nett, J. J., Pötter, S., Schulte, P., Sprafke, T., Jary, Z., et al. (2021). Loess landscapes of Europe – mapping, geomorphology, and zonal differentiation. Earth Sci. Rev. 215:103496. doi: 10.1016/j.earscirev.2020.103496
Levin, N. E., Cerling, T. E., Passey, B. H., and Ehleringer, J. R. (2006).A stable isotope ariditu index for terrestrial environments. Proc. Natl. Acad. Sci. U.S.A. 103, 11201–11205. doi: 10.1073/pnas.0604719103
Longinelli, A. (1984). Oxygen isotopes in mammal bone phosphate: a new tool for paleohydrological and paleoclimatological research? Geochlmrca d Cosmochimrca Acla 48, 385–390. doi: 10.1016/0016-7037(84)90259-X
López, A. B. G., Costamagno, S., and Burke, A. (2022). Osteometric study of metapodial bones and phalanges as indicators of the behavioural ecology of modern reindeer (Rangifer tarandus) and implications for reconstruction of paleo mobility. Open Quat. 8:106. doi: 10.5334/oq.106
Ludowici, B., and Pöppelmann, H. (2017). “Die Tierknochenfunde der mittelpaläolithischen Jägerstation von Salzgitter-Lebenstedt,” in Forschungen und Berichte des Braunschweigischen Landesmuseums Neue Folge 1 (Braunschweig: Verlag Uwe Krebs).
Ma, C., Vander Zanden, H. B., Wunder, M. B., and Bowen, G. J. (2020). assignR: an R package for isotope-based geographic assignment. Methods Ecol. Evol. 11, 996–1001. doi: 10.1111/2041-210X.13426
Máguas, C., and Brugnoli, E. (1996). Spatial variation in carbon isotope discrimination across the thalli of several lichen species. Plant Cell Environ. 19, 437–446. doi: 10.1111/j.1365-3040.1996.tb00335.x
Mallory, C. D., Williamson, S. N., Campbell, M. W., and Boyce, M. S. (2020). Response of barren-ground caribou to advancing spring phenology. Oecologia 192, 837–852. doi: 10.1007/s00442-020-04604-0
Mellars, P. (1973). “The character of the Middle-Upper Paleolithic transition in Southwest France,” in The Explanation of Culture Change, Duckworth, C. Renfrew (London: De Gruyter), 255–276.
Mellars, P. (2004). Reindeer specialization in the early Upper Palaeolithic: the evidence from south west France. J. Archaeol. Sci. 31, 613–617. doi: 10.1016/j.jas.2003.10.010
Merlivat, L. (1978). Molecular diffusivities of H2 16O, HD16O, and H2 18O in gases. J. Chem. Phys. 69, 2864–2871. doi: 10.1063/1.436884
Moncel, M., Chacón, M. G., Vettese, D., Courty, M., and Daujeard, C. (2021). Late Neanderthal short-term and specialized occupations at the Abri du Maras (South-East France, level 4.1, MIS 3). Archaeol. Anthropol. Sci. 5, 2–28. doi: 10.1007/s12520-021-0285-5
Montgomery, J. (2010). Passports from the past: Investigating human dispersals using strontium isotope analysis of tooth enamel. Ann. Hum. Biol. 37, 325–346. doi: 10.3109/03014461003649297
Mook, W. G., Koopmans, M., Carter, A. F., and Keeling, C. D. (1983). Seasonal, latitudinal, and secular variations in the abundance and isotopic ratios of atmospheric carbon dioxide: 1. Results from land stations. J. Geophys. Res. Oceans 88, 10915–10933. doi: 10.1029/JC088iC15p10915
Munson, P. J., and Marean, C. W. (2003). Adults only? A reconsideration of Middle Paleolithic prime-dominated reindeer hunting at Salzgitter Lebenstedt. J. Hum. Evol. 44, 263–273. doi: 10.1016/S0047-2484(02)00163-X
Nehlich, O. (2015). The application of sulphur isotope analyses in archaeological research: A review. Earth Sci. Rev. 142, 1–17. doi: 10.1016/j.earscirev.2014.12.002
Niven, L., Steele, T. E., Rendu, W., Mallye, J. B., McPherron, S. P., Soressi, M., et al. (2012). Neandertal mobility and large-game hunting: the exploitation of reindeer during the Quina Mousterian at Chez-Pinaud Jonzac (Charente-Maritime, France). J. Hum. Evol. 63, 624–635. doi: 10.1016/j.jhevol.2012.07.002
O'Leary, M. H. (1988). Carbon isotopes in photosynthesis. Bioscience 38, 328–336. doi: 10.2307/1310735
Oosenbrug, S. M., and Theberge, J. B. (1980). Altitudinal movements and summer habitat preferences of Woodland Caribou in the Kluane Ranges, Yukon Territory. Arctic 33, 59–72. doi: 10.14430/arctic2548
Park, R., and Epstein, S. (1960). Carbon isotope fractionation during photosynthesis. Geochim. Cosmochim. Acta 21, 110–126. doi: 10.1016/S0016-7037(60)80006-3
Parmesan, C. (2006). Ecological and evolutionary responses to recent climate change. Annu. Rev. Ecol. Evol. Syst. 37, 637–669. doi: 10.1146/annurev.ecolsys.37.091305.110100
Passey, B. H., and Cerling, T. E. (2002). Tooth enamel mineralization in ungulates: implications for recovering a primary isotopic time-series. Geochim. Cosmochim. Acta 66, 3225–3234. doi: 10.1016/S0016-7037(02)00933-X
Pastoors, A. (1996). Die Steinartefakte von Salzgitter-Lebenstedt (Doctoral dissertation). Cologne: University of Cologne.
Pastoors, A. (2001). Die mittelpaläolithische Freilandstation von Salzgitter-Lebenstedt – Genese der Fundstelle und Systematik der Steinbearbeitung. Salzgitter: Salzgitter-Forschungen. Herausgegeben von Archiv der Stadt Salzgitter.
Pastoors, A. (2009). Blades? – Thanks, no interest! – Neanderthals in Salzgitter-Lebenstedt. Quartär 56, 105–118. doi: 10.7485/QU56_05
Patou-Mathis, M. (2000). Neanderthal subsistence behaviours in Europe. Int. J. Osteoarchaeol. 10, 379–395.
Pederzani, S., Aldeias, V., Dibble, H. L., Goldberg, P., Hublin, J. J., Madelaine, S., et al. (2021a). Reconstructing late Pleistocene paleoclimate at the scale of human behavior: an example from the Neandertal occupation of La Ferrassie (France). Sci. Rep. 11:1419. doi: 10.1038/s41598-020-80777-1
Pederzani, S., and Britton, K. (2019). Oxygen isotopes in bioarchaeology: principles and applications, challenges and opportunities. Earth Sci. 188, 77–107. doi: 10.1016/j.earscirev.2018.11.005
Pederzani, S., Britton, K., Aldeias, V., Bourgon, N., Fewlass, H., Lauer, T., et al. (2021b). Subarctic climate for the earliest Homo sapiens in Europe. Sci. Adv. 7:eabi4642. doi: 10.1126/sciadv.abi4642
Pederzani, S., Britton, K., Trost, M., Fewlass, H., Bourgon, N., McCormack, J., et al. (2024). Stable isotopes show Homo sapiens dispersed into cold steppes ~45,000 years ago at Ilsenhöhle in Ranis, Germany. Nat. Ecol. Evol. 8, 578–588. doi: 10.1038/s41559-023-02318-z
Pellegrini, M., Donahue, R. E., Chenery, C., Evans, J., Lee-Thorp, J., Montgomery, J., et al. (2008). Faunal migration in late-glacial central Italy: implications for human resource exploitation. Rapid Commun. Mass Spectrom. 22, 1714–1726. doi: 10.1002/rcm.3521
Petersen, S. L., Scasta, J. D., Schoenecker, K. A., and Hennig, J. D. (2023). “Feral equids,” Rangeland Wildlife Ecology and Conservation, in L. B. McNew, D. K. Dahlgren, and J. L. Beck (Cham: Springer International Publishing), 735–757.
Pfaffenberg, K. (1991). “Die Vegetationsverhaltnisse waehrend und nach der Sedimentation der Fundschichten von Salzgitter-Lebenstedt,” in Der Altsteinzeitliche Fundplatz Salzgitter-Lebenstedt, R. Busch, and H. Schwabedissen (Köln: Böhlau), 183–210.
Pin, C., Briot, D., Bassin, C., and Poitrasson, F. (1994). Concomitant separation of strontium and samarium-neodymium for isotopic analysis in silicate samples, based on specific extraction chromatography. Anal. Chim. Acta 298, 209–217. doi: 10.1016/0003-2670(94)00274-6
Preul, F. (1991). “Die Fundschichten im Klarwerksgelande von Salzgitter- Lebenstedt und ihre Einordnung in die Schichtenfolge des Quartär,” in Der Altsteinzeitliche Fundplatz Salzgitter-Lebenstedt, R. Busch, and H. Schwabedissen (Köln: Böhlau), 9–99.
Preul, F. (2017). “Die Schichtenfolge Der Ausgrabungen des mittelpaläolithischen Jägerlagers vom Salzgitter-Lebenstedt, Norddeutschland,” in Die Tierknochenfunde Der Mittelpaläolithischen Jägerstation von Salzgitter-Lebenstedt. Forschungen Und Berichte Des Braunschweigischen Landesmuseums - Neue Forge - Band 1 (Braunschweigesches Landmuseum, Braunschweig), 41–70.
Price, T. D., Burton, J. H., and Bentley, R. A. (2002). The characterization of biologically available strontium isotope ratios for the study of prehistoric migration. Archaeometry 44, 117–135. doi: 10.1111/1475-4754.00047
Price, T. D., Meiggs, D., Weber, M. J., and Pike-Tay, A. (2017). The migration of late Pleistocene reindeer: isotopic evidence from northern Europe. Archaeol. Anthropol. Sci. 9, 371–394. doi: 10.1007/s12520-015-0290-z
Pryor, A. J. E., Nesnídalová, T., Šída, P., Lengyel, G., Standish, C. D., Milton, J. A., et al. (2024). Reindeer prey mobility and seasonal hunting strategies in the late Gravettian mammoth steppe. Archaeol. Anthropol. Sci. 16:123. doi: 10.1007/s12520-024-02019-z
Pryor, A. J. E., Sázelová, S., and Standish, C. (2016a). “Season of death and strontium/oxygen isotope data for seasonal mobility of three reindeer prey,” in Dolní Vestonice II: Chronology, Paleoethnology, Paleoanthropology, eds. J. Svoboda (Brno: Institute of Archaeology), 147–159.
Pryor, A. J. E., Stevens, R. E., and Pike, W. G. (2016b). “Seasonal mobility of the adult horse killed by hunters at Klementowice,” in Klementowice. A Magdalenian Site in Eastern Poland, T. Wiśniewski (Lublin: Institute of Archaeology, Maria Curie-Skłodowska University in Lublin), 298–304.
Pushkina, D., Bocherens, H., and Ziegler, R. (2014). Unexpected palaeoecological features of the Middle and Late Pleistocene large herbivores in southwestern Germany revealed by stable isotopic abundances in tooth enamel. Quat. Int. 339–340, 164–178. doi: 10.1016/j.quaint.2013.12.033
Rendu, W. (2010). Hunting behavior and Neanderthal adaptability in the Late Pleistocene site of Pech-de-l'Azé I. J. Archaeol. Sci. 37, 1798–1810. doi: 10.1016/j.jas.2010.01.037
Rendu, W. (2022). Selection versus opportunism: a view from Neanderthal subsistence strategies, in: updating Neanderthals. Understanding behavioural complexity in the Late Middle Palaeolithic (London: Elsevier), 109–122. doi: 10.1016/B978-0-12-821428-2.00013-5
Rendu, W., Costamagno, S., Meignen, L., and Soulier, M. C. (2012). Monospecific faunal spectra in Mousterian contexts: implications for social behavior. Quat. Int. 247, 50–58. doi: 10.1016/j.quaint.2011.01.022
Richter, J. (1997). Der G-Schichten-Komplex der Sesselfelsgotte - Zum Verständnis des Micoquien. Saarbrücken: SDV.
Rivals, F., Julien, M.-A., Kuitems, M., Van Kolfschoten, T., Serangeli, J., Drucker, D. G., et al. (2015). Investigation of equid paleodiet from Schöningen 13 II-4 through dental wear and isotopic analyses: archaeological implications. J. Hum. Evol. 89, 129–137. doi: 10.1016/j.jhevol.2014.04.002
Rivals, F., and Lister, A. M. (2016). Dietary flexibility and niche partitioning of large herbivores through the Pleistocene of Britain. Quat. Sci. Rev. 146, 116–133. doi: 10.1016/j.quascirev.2016.06.007
Rivals, F., Schulz, E., and Kaiser, T. M. (2008). Climate-related dietary diversity of the ungulate faunas from the middle Pleistocene succession (OIS 14-12) at the Caune de l'Arago (France). Paleobiology 34, 117–127. doi: 10.1666/07023.1
Rivals, F., Schulz, E., and Kaiser, T. M. (2009). Late and middle Pleistocene ungulates dietary diversity in Western Europe indicate variations of Neanderthal paleoenvironments through time and space. Quat. Sci. Rev. 28, 3388–3400. doi: 10.1016/j.quascirev.2009.09.004
Rivals, F., and Semprebon, G. M. (2011). Dietary plasticity in ungulates: insight from tooth microwear analysis. Quat. Int. 245, 279–284. doi: 10.1016/j.quaint.2010.08.001
Rivals, F., and Semprebon, G. M. (2017). Latitude matters: an examination of behavioural plasticity in dietary traits amongst extant and Pleistocene Rangifer tarandus. Boreas 46, 254–263. doi: 10.1111/bor.12205
Rivals, F., and Solounias, N. (2007). Differences in tooth microwear of populations of caribou ( Rangifer tarandus, Ruminantia, Mammalia) and implications to ecology, migration, glaciations and dental evolution. J. Mammal. Evol. 14, 182–192. doi: 10.1007/s10914-007-9044-8
Rozanski, K. (1985). Deuterium and oxygen-18 in European groundwaters—Links to atmospheric circulation in the past. Chem. Geol.: Isotope Geosci. Section 52, 349–363. doi: 10.1016/0168-9622(85)90045-4
Rozanski, K., Araguás-Araguás, L., and Gonfiantini, R. (2013). Isotopic Patterns in Modern Global Precipitation (Washington, DC: American Geophysical Union), 1–36.
Ruebens, K., Smith, G. M., Fewlass, H., Sinet-Mathiot, V., Hublin, J. J., and Welker, F. (2023). Neanderthal subsistence, taphonomy and chronology at Salzgitter-Lebenstedt (Germany): a multifaceted analysis of morphologically unidentifiable bone. J. Quat. Sci. 38, 471–487. doi: 10.1002/jqs.3499
Saarinen, J., Eronen, J., Fortelius, M., Sepp,ä, H., and Lister, A. M. (2016). Patterns of diet and body mass of large ungulates from the Pleistocene of Western Europe, and their relation to vegetation. Palaeontol. Electron. 19, 1–58. doi: 10.26879/443
Salter, R. E., and Hudson, R. J. (1979). Feeding ecology of feral horses in western Alberta. J. Range Managem. 32, 221–225. doi: 10.2307/3897127
Scheibe, K. M., Eichhorn, K., Kalz, B., Streich, W. J., and Scheibe, A. (1998). Water consumption and watering behavior of Przewalski horses (Equus ferus przewalskii) in a semireserve. Zoo Biol. 17, 181–192.
Schoenecker, K. A., Esmaeili, S., and King, S. R. B. (2023). Seasonal resource selection and movement ecology of free-ranging horses in the western United States. J. Wildl. Manage. 87:22341. doi: 10.1002/jwmg.22341
Schoenecker, K. A., King, S. R. B., Nordquist, M. K., Nandintsetseg, D., and Cao, Q. (2016). “Habitat and diet of equids,” in Wild Equids: Ecology, Management, and Conservation, J. I. Ransom, and P. Kaczensky (Baltimore: John Hopkins University Press), 41–57.
Schütrumpf, R. (1991). “Kommentar zu dem vorstehenden Beitrag von W. Selle über Salzgitter-Lebenstedt nach dem Forschungsstand der achtziger Jahre,” in Der Altsteinzeitliche Fundplatz Salzgitter-Lebenstedt. Teil II, eds. R. Busch, and H. Schwabedissen (Naturwissenschaftliche Untersuchungen; Böhlau, Köln), 162–182.
Schwartz-Narbonne, R., Longstaffe, F. J., Kardynal, K. J., Druckenmiller, P., Hobson, K. A., Jass, C. N., et al. (2019). Reframing the mammoth steppe: insights from analysis of isotopic niches. Quat. Sci. Rev. 215, 1–21. doi: 10.1016/j.quascirev.2019.04.025
Selle, W. (1991). “Die palynologischen Untersuchungen am palaeolithischen Fundplatz Salzgitter–Lebenstedt,” in Der Altsteinzeitliche Fundplatz Salzgitter- Lebenstedt. Teil II, Naturwissenschaftliche Untersuchungen, R. Busch, and H. Schwabedissen (Köln: Böhlau), 149–161.
Severson, J. P., Johnson, H. E., Arthur, S. M., Leacock, W. B., and Suitor, M. J. (2021). Spring phenology drives range shifts in a migratory Arctic ungulate with key implications for the future. Glob. Chang. Biol. 27, 4546–4563. doi: 10.1111/gcb.15682
Sirocko, F., Knapp, H., Dreher, F., Förster, M. W., Albert, J., Brunck, H., et al. (2016). The ELSA-Vegetation-Stack: Reconstruction of Landscape Evolution Zones (LEZ) from laminated Eifel maar sediments of the last 60,000 years. Glob. Planet. Change 142, 108–135. doi: 10.1016/j.gloplacha.2016.03.005
Skoog, R. O. (1968). Ecology of the caribou (Rangifer tarandus granti) in Alaska. Berkley: University of California.
Soana, S., Gnudi, G., and Bertoni, G. (1999). The teeth of the horse: evolution and anatomo-morphological and radiographic study of their development in the foetus. Anat. Histol. Embryol. 28, 273–280. doi: 10.1046/j.1439-0264.1999.00204.x
Sponheimer, M., Lee-Thorp, J., de Ruiter, D., Codron, D., Codron, J., Baugh, A. T., et al. (2005). Hominins, sedges, and termites: new carbon isotope data from the Sterkfontein valley and Kruger National Park. J. Hum. Evol. 48, 301–312. doi: 10.1016/j.jhevol.2004.11.008
Sponheimer, M., and Lee-Thorp, J. A. (1999). Isotopic evidence for the diet of an early hominid, Australopithecus africanus. Science. 283, 368–370. doi: 10.1126/science.283.5400.368
Staesche, U. (2017). “Das Ren (Rangifer tarandus) aus den mittelpaläolithischen Fundschichten von Salzgitter- Lebenstedt (Niedersachsen, Deutschland),” in Die Tierknochenfunde Der Mittelpaläolithischen Jägerstation von Salzgitter-Lebenstedt. Forschungen und Berichte des Braunschweigischen Landesmuseums Neue Folge 1, eds. B. Ludovici, H. Pöppelmann (Braunschweig: Verlag Uwe Krebs), 99–194.
Stephan, E. (2017). “Paläotemperaturbestimmungen anhand von Sauerstoffisotopenverhältenissen in Pferde- und Rentierfunden aus der mittelpaläolitischen Fundstelle Salzgitter-Lebenstedt, Norddeutschland,” in Die Tierknochenfunde Der Mittelpaläolithischen Jägerstation von Salzgitter-Lebenstedt. Forschungen Und Berichte Des Braunschweigischen Landesmuseums - Neue Forge - Band 1, 287–301.
Stevens, R. E., Balasse, M., and O'Connell, T. C. (2011). Intra-tooth oxygen isotope variation in a known population of red deer: Implications for past climate and seasonality reconstructions. Palaeogeogr. Palaeoclimatol. Palaeoecol. 301, 64–74. doi: 10.1016/j.palaeo.2010.12.021
Stevens, S. K., Armleder, H., Jull, M., King, D., McLellan, B., and Coxson, D. (2001). Mountain Caribou in Managed Forests: Recommendations for Managers. Wildlife report No. R-26. Victoria, BC: Ministry of Environment, Lands and Parks.
Stumpp, C., Klaus, J., and Stichler, W. (2014). Analysis of long-term stable isotopic composition in German precipitation. J. Hydrol. 517, 351–361. doi: 10.1016/j.jhydrol.2014.05.034
Teeri, J. A. (1981). Stable carbon isotope analysis of mosses and lichens growing in Xeric and moist habitats. Bryologist. 84, 82–84.
Theoret, J., Cavedon, M., Hegel, T., Hervieux, D., Schwantje, H., Steenweg, R., et al. (2022). Seasonal movements in caribou ecotypes of Western Canada. Mov. Ecol. 10:12. doi: 10.1186/s40462-022-00312-x
Tieszen, L. L. (1991). Natural variations in the carbon isotope values of plants: implications for archaeology, ecology, and paleoecology. J. Archaeol. Sci. 18, 227–248. doi: 10.1016/0305-4403(91)90063-U
Tode, A. (1982). “Der altsteinzeitliche Fundplatz Salzgitter-Lebenstedt. Teil I,” in Archäologisher Teil, A. Tode (Köln: Böhlau Verlag).
Tode, A., Preul, F., Richter, K., Sell, W., Pfaffenberg, K., Kleinschmidt, A., et al. (1953). Die Untersuchung der paläolithischen Freilandstation von Salzgitter-Lebenstedt. E&G Quat. Sci. J. 3, 144–220. doi: 10.3285/eg.03.1.14
Toucanne, S., Rodrigues, T., Menot, G., Soulet, G., Cheron, S., Billy, I., et al. (2023). Marine Isotope Stage 4 (71–57 ka) on the Western European margin: Insights to the drainage and dynamics of the Western European Ice Sheet. Glob. Planet. Change 229:104221. doi: 10.1016/j.gloplacha.2023.104221
Van Asperen, E. N. (2010). Ecomorphological adaptations to climate and substrate in late Middle Pleistocene caballoid horses. Palaeogeogr. Palaeoclimatol. Palaeoecol. 297, 584–596. doi: 10.1016/j.palaeo.2010.09.007
van der Merwe, N. J., and Medina, E. (1991). The canopy effect, carbon isotope ratios and foodwebs in amazonia. J. Archaeol. Sci. 18, 249–259. doi: 10.1016/0305-4403(91)90064-V
Vogel, J. C. (1978). Isotopic assessment of the dietary habits of ungulates. S. Afr. J. Sci. 74, 298–301.
Vogel, J. C., and Zagwijn, W. H. (1967). Groningen radiocarbon dates VI. Radiocarbon 9, 63–106. doi: 10.1017/S0033822200000485
Webber, Q. M. R., Ferraro, K. M., Hendrix, J. G., and Vander Wal, E. (2022). What do caribou eat? A review of the literature on caribou diet. Can. J. Zool. 100, 197–207. doi: 10.1139/cjz-2021-0162
Weinstock, J. (2002). Reindeer hunting in the upper palaeolithic: sex ratios as a reflection of different procurement strategies. J. Archaeol. Sci. 29, 365–377. doi: 10.1006/jasc.2002.0716
White, M., Pettitt, P., and Schreve, D. (2016). Shoot first, ask questions later: Interpretative narratives of Neanderthal hunting. Quat. Sci. Rev. 140, 1–20. doi: 10.1016/j.quascirev.2016.03.004
Willmes, M., Bataille, C. P., James, H. F., Moffat, I., McMorrow, L., Kinsley, L., et al. (2018). Mapping of bioavailable strontium isotope ratios in France for archaeological provenance studies. Appl. Geochem. 90, 75–86. doi: 10.1016/j.apgeochem.2017.12.025
Wright, L. E., and Schwarcz, H. P. (1998). Stable carbon and oxygen isotopes in human tooth enamel: Identifying breastfeeding and weaning in prehistory. Am. J. Phys. Anthropol. 106, 1–18.
Keywords: palaeoecology, reindeer, enamel, isotope analysis, Late Pleistocene, Neanderthal, Paleolithic
Citation: Heddell-Stevens P, Barakat S, Pastoors A, Lucas M, Scott E, Le Roux P and Roberts P (2024) Spatial palaeoecology of large-herbivore hominin prey-species at Salzgitter-Lebenstedt, Lower Saxony, Germany: multi-isotope analysis of sequentially-sampled tooth enamel from Rangifer tarandus and Equus sp.. Front. Environ. Archaeol. 3:1458040. doi: 10.3389/fearc.2024.1458040
Received: 01 July 2024; Accepted: 04 December 2024;
Published: 24 December 2024.
Edited by:
Sally Christine Reynolds, Bournemouth University, United KingdomReviewed by:
Sarah Pederzani, University of La Laguna, SpainChris Baumann, Senckenberg Research Centre for Human Evolution and Palaeoenvironment (S-HEP), Germany
Copyright © 2024 Heddell-Stevens, Barakat, Pastoors, Lucas, Scott, Le Roux and Roberts. This is an open-access article distributed under the terms of the Creative Commons Attribution License (CC BY). The use, distribution or reproduction in other forums is permitted, provided the original author(s) and the copyright owner(s) are credited and that the original publication in this journal is cited, in accordance with accepted academic practice. No use, distribution or reproduction is permitted which does not comply with these terms.
*Correspondence: Phoebe Heddell-Stevens, aGVkZGVsbHN0ZXZlbnNAZ2VhLm1wZy5kZQ==