- 1Muséum National d'Histoire Naturelle, Paris, France
- 2Institut National de Recherches en Archéologie Préventive, Passel, France
- 3Department of Crop and Forest Sciences, Universitat de Lleida, Lleida, Spain
The economic development of societies in Gaul during the Second Iron Age and the Roman period relied on agricultural system transformations. Hundreds of carpological studies carried out in the northern half of France over the last four decades have thoroughly documented the evolution of crop production and farming practices during this period. The increase in agricultural production required to sustain urbanization and trade expansion led to a diversification of cropping systems, resulting in the emergence of extensive systems. Weed ecological studies have shown how fertilization methods for cultivated soils changed between the earlier Gallic period, when highly fertile soils were common, and the Roman period, with a diversification of situations. This insight is only relevant on a global scale and required a more in-depth analysis. The present study investigates organic fertilization techniques at the crop scale, through nitrogen isotope analyses (δ15N), which are used as an indicator of soil fertility and the use of fertilizing organic matter (such as manure). The study was conducted on a sample of 124 crop remains, consisting of 6,490 archaeological cereal grains (emmer wheat, spelt wheat, free-threshing wheats and hulled barley) recovered from 68 archaeological sites located across the northern half of France, dating between the 6th century BC and the 5th century AD. To evaluate δ15N values of archaeological cereals in terms of fertilization, we compared published experimental data of modern cereals (grown under different fertilization regimes) for temperate Europe with published and new isotopic analyses of archaeological deer bone collagen from northern France as a proxy for wild unfertilised flora. Discriminant analysis and ordinal logistic regression were used for the classification of archaeological grain δ15N values by degree of fertilization. The results show that field fertilization was a common practice in Gaul, generally on a moderate scale. However, variations are observed in fertilization use within farms, with some intensively fertilized fields and others with less or no fertilization at all. In addition, regional differences emerged, particularly in the chalky Champagne region, where farmers used significantly less fertilizer than in other regions. Chronological trends over the studied period remain unclear. Disparities in the fertilization levels of different cereal species are noted. The δ15N values show considerable variations in soil fertility for barley and spelt, while fertilization regimes for free-threshing wheats and emmer only diversified during the 2nd and 1st centuries BC. These observations concur with the extensification of cultivation as inferred from carpological and weed ecology studies. Interspecific variations can also be seen in treatments applied to free-threshing wheats, which were fertilized much more commonly than the other three cereals from the 1st c. BC/1st c. AD, when these crops were in full expansion.
1 Introduction
1.1 The agrarian economy of the Second Iron Age and the Roman period in Northern France
1.1.1 Land use
The development of preventive archaeology since the 1980s−1990s, combined with bioarchaeological studies, renewed and extended our knowledge of the rural economy north of the Loire, from the Atlantic coast to the Rhine valley. Paleoenvironmental studies of the Second Iron Age show that, on a global scale, forest massifs largely retreated in favor of anthropogenic areas and agricultural activities (Blancquaert et al., 2012), accelerating the dynamic already observed since the Bronze Age (Gautier et al., 2018). Pollen and diaspores point to the marked expansion of grasslands (Zech-Matterne and Brun, 2016) attesting to the transformation of landscapes as rural societies developed.
Land use during the Second Iron Age was inherited from a system that had often been in place since the Bronze Age (Besnard-Vauterin et al., 2016). Ditch-enclosed settlements appeared in the west of the country around the 6th century BC and became widespread throughout Gaul by the 3rd century BC. They persisted into the Roman period, with some regional morphological variations (Bertrand et al., 2009; Malrain et al., 2013). The number of rural settlements varied throughout the Second Iron Age, with two peaks of new settlements in the 5th century BC and at the end of the 2nd century BC. The creation of oppida from this date onwards resulted in a decrease in the number of rural settlements (Malrain et al., 2013). These towns concentrated the elites, artisans and merchants, channeling a substantial proportion of the population and prefiguring the rise of Roman urbanity (Buchsenschutz, 2018). The impact of the Cesarean War on the occupation of the territory is not yet well defined. The number of settlements declined at this time, but a number were also newly built (Malrain, 2023a).
Developments in the Roman countryside continued into the Second Iron Age with no real break between the two periods. The main changes took place on the edges of the Rhine, beyond the scope of this study, in order to develop cereal production and supply the armies (Reddé, 2018a). Elsewhere, very little new land was exploited after the conquest, as most of it had already been occupied during the Second Iron Age. The environment continued to open up during the first centuries of our era, although woodland was still preserved or increased, and climatic conditions were mostly favorable to agriculture (Petit et al., 2018). One of the major changes in the social and economic organization of the region was the rise of urbanization under the impetus of the Roman administration, which redefined networks and markets (Van Andringa, 2015; Reddé et al., 2018). At the end of Antiquity, a decline in land use was observed, in connection with the invasions of the 3rd century AD, although the number of settlements did not diminish to the same extent in all regions (Reddé, 2018b).
1.1.2 Agricultural production
Archaeobotanical studies have significantly increased our knowledge of farming practices and the plants cultivated during the Second Iron Age and the Roman period in northern Gaul, as summarized just below (Matterne, 2001; Zech-Matterne et al., 2014, 2017a,b; Neveu, 2017; Toulemonde et al., 2017a,b; Neveu et al., 2021). Agriculture in the First and Early Second Iron Ages relied on a wide variety of crops. From the 4th century BC onwards, settlements began to specialize in the cultivation of one or two cereals, although the crop diversity was not significantly reduced. Einkorn and millets were relegated to a minor role in farming systems. Hulled barley and emmer wheat were common crops throughout Gaul for centuries to come, although there were regional variations, with free-threshing wheats and spelt playing a more prominent role in some areas. With the rise of free-threshing wheats, the importance of emmer crops declined during the Roman period. By choosing these species, farmers turned to high-performance cereals that could generate surpluses. Geographical variations in crops appear to be broadly adapted to soil types: the fertile soils of the Ile-de-France region are conducive to growing free-threshing wheats, while hulled barley and spelt are well suited to the less fertile soils of the east. Cereal growing evolved gradually, and the major changes in agricultural and dietary practices that occurred with the Romanisation of society consists of the adoption of fruit growing and the exploitation of the bread-making properties of certain types of wheat (free-threshing wheats and spelt).
Studies of weed flora provide information on plant-growing conditions in fields and help to define cultivation practices (Charles et al., 1997). Such studies have been carried out on a regional scale for the area of interest here, in particular for the Paris Basin (Ile-de-France, Picardie, chalky Champagne) (Zech-Matterne and Brun, 2016; Zech-Matterne et al., 2017b). The western regions (Massif Armorican, Caen Plain) are slightly less well documented (Neveu et al., 2021). At the transition between the First and Second Iron Ages, fields were located on nutrient-rich soils. They could be weeded, but the predominance of annual plants indicates that the soil was not significantly disturbed. During the Second Iron Age, archaeobotanical assemblages record the transformation of agriculture, and the same trend continued into the Roman period. Soil texture and fertility diversified, reflecting the exploitation of a variety of soils. These features are synchronous with palynological signals of deforestation (Blancquaert et al., 2012) and changes in the topographical location of settlement, which began to expand to plateaus and were no longer restricted to valley bottoms at the end of the 4th century BC (Malrain et al., 2015). These new sites indicate an increase in the farmed area. Alongside the adoption of cereal “monocultures,” these changes demonstrate a drive to increase the volume of agricultural production through a more extensive system (decrease in labor input per unit area, resulting in lower yields, compensated by an increase in cultivated area). Furthermore, grassland flora also took on an increasingly important role, pointing to the development of animal husbandry and improved livestock feed, which is manifest in the size of the animals (Duval and Clavel, 2018). Changes occurred in the tools used, with, for example, more and a greater variety of plowshares and sickles from the 3rd−2nd centuries BC onwards, indicating an increase in agricultural activity and tillage (Malrain, 2023b). Changes in farming practices must also have altered the intensity of the links between crops and livestock: better-fed livestock produce more manure, while larger fields dilute fertilization rates. The literary sources of the Latin Agronomists, which can be extrapolated for our study area, mention manure as the main source of fertilizer, but other materials are used in addition, such as the direct dejections of livestock on the fields or various composted plant materials (Ouzoulias, 2006). The use of urban latrines during the Roman period also made it possible to supply the countryside with fertilizer (Cordier, 2003).
1.2 Variations in the nitrogen isotopic composition of soil and plants and soil fertility management
The study of the fertilization of fields helps to characterize the techniques used to implement economic changes during the Iron Age and Roman periods. This may be undertaken through the analysis of the nitrogen isotope composition (δ15N) of cereal grains. Cereals take up nitrogen from the soil, largely derived from the decomposition of organic matter. The transformation of organic nitrogen into bioavailable minerals results in isotopic fractionation, and the soils δ15N values are subject to many environmental factors, such as climate, geology and erosion (Högberg, 1997; Amundson et al., 2003). Agriculture, causing disruption of the nitrogen cycle (export of crops and input of exogenous fertilizers), is a major factor of change in the soil and plants δ15N values, to an extent that may mask variations due to environmental factors (Amundson et al., 2003). In particular, the use of organic fertilizers, such as manure, leads to a relative enrichment in 15N (Bol et al., 2005; Choi et al., 2006). This enrichment results from the preferential loss of the light isotope (14N) through volatilisation in the form of ammonia, during manure storage and composting of organic matter. Other farming techniques can affect the isotopic composition of soil and plants, such as legume-based green manure, slash-and-burn farming and deep plowing, but their effect is either not yet well known or is relatively minimal (Szpak, 2014).
Several studies have been carried out to measure the effects of manure and animal excrement spreading on the δ15N values of cereals in different regions of the world. They have been conducted on cereals from long-term experiments (from several decades to more than a century) in agronomic stations, from short-term experiments over 1 or 2 years, or on cereals produced by working farms (practicing traditional agriculture). The most comprehensive studies in a European context are those by Fraser et al. (2011) and Larsson et al. (2019). These have shown that (i) there is a positive correlation between fertilization rates and δ15N values; (ii) the effect of fertilization is visible from the first years of application; (iii) when fertilization is stopped, δ15N values decrease slowly over time; (iv) inter-annual variations occur, without masking the difference between fertilized and control plots; (v) the effects of fertilization are similar for the different cereal species; (vi) the farm study shows that δ15N values vary considerably at the farm scale, due to the variability in the amount of fertilizer spread on the land. Threshold values have been defined to help determine the fertilization regime. In temperate Europe, cereals from unfertilised plots have δ15N values below 3‰. Values above this threshold result from human activities. δ15N values above 6‰ reflect long-term manuring with large quantities of fertilizer. High values (above 8‰−9‰) are due to very high levels of manuring (as in the case of intensive vegetable crops) or to the use of slurry, which is richer in ammonia than manure. δ15N values between 3 and 6‰ reflect either lower levels of manuring, or the first few years of manuring, or a residual effect after the cessation of intense or long-term manuring dating back more than a century. Although archaeological cereals are found charred and buried, they retain their original isotopic signal, which can be interpreted in terms of paleoenvironmental implications and agricultural practices (Fraser et al., 2013; Styring et al., 2013; Nitsch et al., 2015; Brinkkemper et al., 2018).
1.3 Objectives and hypotheses
The dynamism of agricultural activities and the economic development of Gallic societies during the Second Iron Age and the Roman period shows that farmers used productive techniques to generate surpluses. Our aim is to identify the place of fertilization, its intensity and its differentiated use, in order to contribute to discussions on the agrarian system. We began by assessing the environmental variation in δ15N values from deer bone collagen, in order to obtain a baseline for archaeologically unfertilised plants. We then used the data from Fraser et al. (2011) in order to introduce ranges of uncertainty around the threshold values between levels of fertilization intensity. Following these methodological steps, we can approach variations in fertilization during the Second Iron Age and the Roman period in France from several scales of analysis. We assume that although fertilization was a common practice in these societies, in a context of extensive farming systems, the cultivated area is large and manure spreading cannot be uniform across all fields. We investigate variations within sites, depending on the period, the cereal species or the region.
2 Materials and methods
2.1 Material
2.1.1 Archaeological plant material
The archaeological cereals belong to the four most widely cultivated taxa during the Second Iron Age and the Roman period (Zech-Matterne et al., 2017a, 2020):
• Emmer wheat (Triticum turgidum ssp. dicoccum (Schrank) Thell.);
• Spelt wheat (Triticum aestivum ssp. spelta (L.) Thell.);
• Free-threshing wheats group (bread wheat–Triticum aestivum L. ssp. aestivum/durum wheat–T. turgidum ssp. durum (Desf.) Husn. /rivet wheat–T. turgidum L. ssp. turgidum);
• Hulled barley (Hordeum vulgare L.).
Hulled barley is identified by the characteristic shape of its grains. Emmer and spelt wheat species are distinguished by the morphology of their grains and their glume and spikelet bases. The species described as “free-threshing wheats” are difficult to distinguish solely on the basis of the morphology of their caryopses and in the absence of rachis elements bearing diagnostic characteristics of the genetic type (tetraploid-rivet wheat and durum wheat/hexaploid-bread wheat). As the husks of free-threshing wheats are so easy to separate, these plant parts are rarely found. Both types have been recognized in some Roman archaeobotanical assemblages from the Ile-de-France region, either in mixed or in isolated assemblages. Elsewhere, such determinations are exceptional and therefore, only the broad designation is retained here.
The studied cereals come from concentrated archaeobotanical assemblages, characterized by a high density of remains in the stratigraphic units. These correspond to once-off dumping event forming the archaeological layer. Various types of features were sampled, consisting mainly of negative features (pits, silos, wells, ditches, cellars) used as dumping grounds at the end of their primary function (Gransar, 2002). Other stratigraphic units correspond to fire levels (hearths and ovens) or accidental charring during the use of grain dryers. The remains found in post holes were trapped during the destruction of buildings. Grains of a given species in a concentration probably come from a unique harvest. However, as a certain amount of time and various events separate the harvest from the dumping of the grain (cleaning of the crop, storage, removal from storage, drying of the grain, carbonization), the different species contained in an assemblage, if not mixed crops, were most probably harvested at different times. They could have been harvested a few years apart and found mixed together in the dump after being stored separately. It is therefore impossible to determine whether the harvests were strictly contemporaneous. In almost all cases, the archaeological contexts were dated by relative chronology using the material contained in the fill. Where the reports use relative chronology and typochronological nomenclature, we have assigned calendar dates on the basis of a summarized chronology for the Second Iron Age (Barral and Fichtl, 2012, p. 16).
We sampled 30 or 50 grain of each species of each archaeological assemblage. The total cereal grains corpus consists in 124 groups (6,490 grains), from 95 features (68 sites, Figure 1), dated from the 6th century BC to the 5th century AD and includes the corpus previously published by Aguilera et al. (2018), which followed the same sampling strategy (Supplementary Data Sheet 1). Some of the contexts studied by Aguilera et al., were re-sampled to determine potential shifts between our two studies.
The geographical and plant species distribution of the corpus is disparate, reflecting the state of archaeological research in different regions and the material available, and partly reflecting past agricultural production. Fewer archaeobotanical studies have been carried out in the western regions of France (Massif Armorican, Normandy, with the exception of the Caen region) (Zech-Matterne et al., 2009; Neveu, 2017). Over thirty groups were sampled for emmer, free-threshing wheats and hulled barley (N = 34, N = 35, and N = 42, respectively), while only 13 groups were sampled for spelt, a species found mainly in the east of France. Free-threshing wheats were mainly grown in the Beauce region during the Second Iron Age (4th−2nd centuries BC) and then more widely in the Paris Basin during the Roman period (Zech-Matterne et al., 2014), while they were rarer elsewhere.
2.1.2 Archaeological faunal and experimental plant material
In order to estimate reference δ15N values for unfertilised flora, we use archaeological deer (Cervus elaphus) bone collagen as an indicator (Bogaard et al., 2013). Wild fauna remains are very rare in sites from the Second Iron Age and the Roman period in Gaul, accounting for only around 1% of faunal remains, and are not present at all sites (Lepetz and Morand, 2017). Although other studies have worked on a site scale (Bogaard et al., 2013; Styring et al., 2016; Aguilera et al., 2018; Alagich et al., 2018), the deer bones were sampled on a regional scale, by aggregating distant sites and therefore averaging the δ15N values over a larger area (Styring et al., 2017; Wang et al., 2018; Hart and Feranec, 2020).
In total, 41 deer bones were sampled from nine rural settlements dating from the Second Iron Age and the Roman period. To complete this corpus, we are using previously published data from Jouars-Pontchartrain “La Ferme d'Ithe,” Palaiseau “Les Trois Mares,” and Acy-Romance “La Warde” (Aguilera et al., 2018), Amiens “Ilot de la Boucherie” (Mion et al., 2016), Entzheim “Geispolsheim” (Schnitzler et al., 2018) and Basel “Gasfabrik” (Switzerland) (Knipper et al., 2017), bringing the corpus to a total of 79 deer bones spread over the entire study area (Figure 2, Supplementary Data Sheet 2).
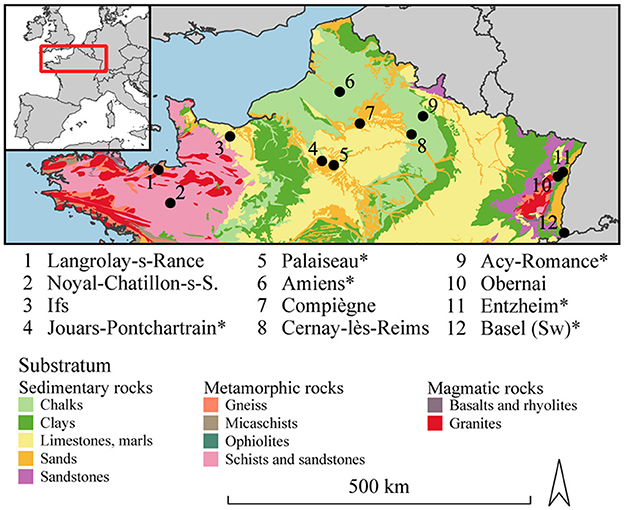
Figure 2. Location of archaeological sites with studied deer bones and geological context. *Previously published data. Geological data: BRGM.
The published data used to supplement the data in this paper all used the same collagen extraction protocol (Bocherens et al., 1991), which facilitates inter-laboratory comparisons.
To define baselines for manuring intensity levels, we used previously published δ15N values from cereals grown on long-term agronomic stations in Northern Europe (Fraser et al., 2011). The dataset consists of 62 groups of several dozen grains including several species, divided into three levels of fertilization: no manure, 10–15 t/ha/year of manure or ancient manuring and 20–35+ t/ha/year of manure (Supplementary Data Sheet 3).
2.2 Methods
2.2.1 Isotopic analysis of archaeological cereals
The grains were cleaned of sedimentary concretions and rootlets under a stereomicroscope, using a wooden pick. They were then treated to remove carbonates, following a protocol adapted from Vaiglova et al. (2014) and Aguilera et al. (2018): the 10-grain sub-samples were placed in 10 ml of 0.5 M HCl at 70°C for 1 h, then rinsed with distilled water at room temperature, renewing the water three times after 24 h. The ten grains were freeze-dried before being pulverized in an agate mortar and the powder was homogenized. Measurements were taken on 1 mg of powder using an elemental analyser (Thermo Flash 2000) interfaced with an isotope mass spectrometer (Thermo DeltaV Advantage) on the IRMS platform at the Muséum national d'Histoire naturelle in Paris (SSMIM).
Isotopic ratios are expressed by the notation δ15N relative to atmospheric N2 standard, relative nitrogen (%N) and carbon (%C) contents, and δ13C values (relative to VPDB standard) are presented in Supplementary Data Sheet 1. The accuracy and precision of the analyses were determined by the joint analysis of a laboratory standard (alanine), normalized to the IAEA-600 international standard (caffeine), and the NIST SRM 1547 standard (peach leaf). Over the course of all analyses (10 runs), the alanine standard (n = 80) yielded mean values of 0.73 ± 0.10‰ for δ15N (expected value = 0.60‰), −21.85 ± 0.11‰ for δ13C (expected value = −22.17‰), and 40.65 ± 0.45% for C content (expected value = 40.44%) and the peach leaf standard (n = 59) gave mean values of 2.87 ± 0.07% for N content (expected value = 2.97%). Analytical precision, determined within each run from eight alanine analyses, varied between ±0.07 and ± 0.17‰ for δ15N; ± 0.03 and ± 0.16‰ for δ13C; ±0.15% and ± 0.57% for C content. For the N content, analytical precision, determined from seven peach leaf analyses, ranges from ± 0.01% to ± 0.13%. Following Nitsch et al. (2015), we applied a correction of −0.31 and −0.11‰ to the δ15N and δ13C values of the carbonized grains, respectively, to take into account the effect of carbonization in archaeological interpretations.
In the following text, the term “cereal groups” refers to the average of 3–5 samples of ten grains of the same species from the same archaeological layers. Analysis by groups of 30–50 grains gives a more robust average isotopic signal than using only ten grains per context (Fraser et al., 2011).
2.2.2 Extraction and isotopic analysis of bone collagen from archaeological deer
A sample of each deer bone was extracted with a saw, the surface was cleaned by abrasion with a tungsten burr and then ground in a grinder (IKA M20, 20,000 rpm). The powder was sieved and the fraction between 0.3 and 0.7 mm was used for collagen extraction, following the protocol established by Bocherens et al. (1991): (i) Approximately 400 mg of powder was demineralised in 20 ml of 1 M HCl under magnetic stirring for 20 min at room temperature. (ii) The sample was then decontaminated of potential humic acids in 20 ml of 0.125 M NaOH for 20 h at room temperature. (iii) Gelatinisation of the collagen was carried out in ~10 ml of 10−2 M HCl hydrochloric acid (pH = 2) at 85°C for 17 h. After each step, the mixture was filtered through a 5 μm MF-Millipore membrane; after steps (i) and (ii), the residue was recovered and rinsed eight times with distilled water. After step (iii) the filtrate was recovered and freeze-dried and 0.3 mg of this collagen extract was weighed for isotopic analysis.
Deer bone collagen was analyzed using the same instruments as archaeological cereals, and the accuracy and precision of the analyses were determined using the same alanine laboratory standard. Over the course of all analyses (two runs), the alanine standard (n = 19) gave mean values of 0.60 ± 0.16‰ for δ15N (expected value = 0.60‰), −21.63 ± 0.12‰ for δ13C (expected value = −22.17‰), and 40.51 ± 0.34% for C content (expected value = 40.44%) and 15.75 ± 0.17% for N content (expected value = 15.72%). Analytical precision, determined within each run from eight alanine analyses, varied between ±0.13 and ± 0.16‰ for δ15N; ± 0.09 and ± 0.12‰ for δ13C; ±0.30% and ± 0.37% for C content; ± 0.06% to ± 0.13% for N content.
Results are presented in Supplementary Data Sheet 2. The estimate of wild flora values, inferred from the deer diet, is calculated by subtracting 4‰ from the δ15N values of bone collagen (Bogaard et al., 2013).
2.3 Statistical analysis
To classify the δ15N values of cereals into levels of fertilization, the most common statistical method used in archaeology is linear discriminant analysis (Kovarovic et al., 2011), which is often applied to predict the taxonomic attribution of bioarchaeological remains on the basis of data measured on present-day, well-identified individuals (“training data”). Logistic regression is a competing model to discriminant analysis (Bellanger and Tomassone, 2014) but is more rarely used. Both methods are tested using supervised learning on cereals from the long-term experiments published by Fraser et al. (2011) with δ15N values as the unique predictor of fertilization levels in archaeological cereals. As the fertilization classes follow a gradient from the least to the most manured plots, ordinal logistic regression is used. Models are calculated using the leave-one-out cross-validation procedure to improve the accuracy of the classification, measured by the percentage of correctly classified individuals (Kovarovic et al., 2011). Classification predictions are associated with a posterior probability, depending on the individual's distance from the class mean. A probability threshold can be defined for accepting or rejecting a classification, which offers the opportunity to define ranges of uncertainty (Evin et al., 2013) and to shade the use of theoretical thresholds of 3 and 6‰, traditionally used in archaeological studies since Bogaard et al. (2013).
Statistical analyses were carried out using R version 4.3.2. Scripts are presented in Supplementary Data Sheet 5.
3 Results
3.1 Isotopic analyses of archaeological cereals
Overall, the new 105 groups of archaeological cereal grains, after correction for the charring effect, gave δ15N values ranging from 0.0‰ to 7.9‰ (mean = 3.9±1.6‰) and δ13C values ranging from −25.7‰ to −21.0‰ (mean = −23.5 ± 0.9‰; Figure 3). The results are listed extensively in Supplementary Data Sheet 1.
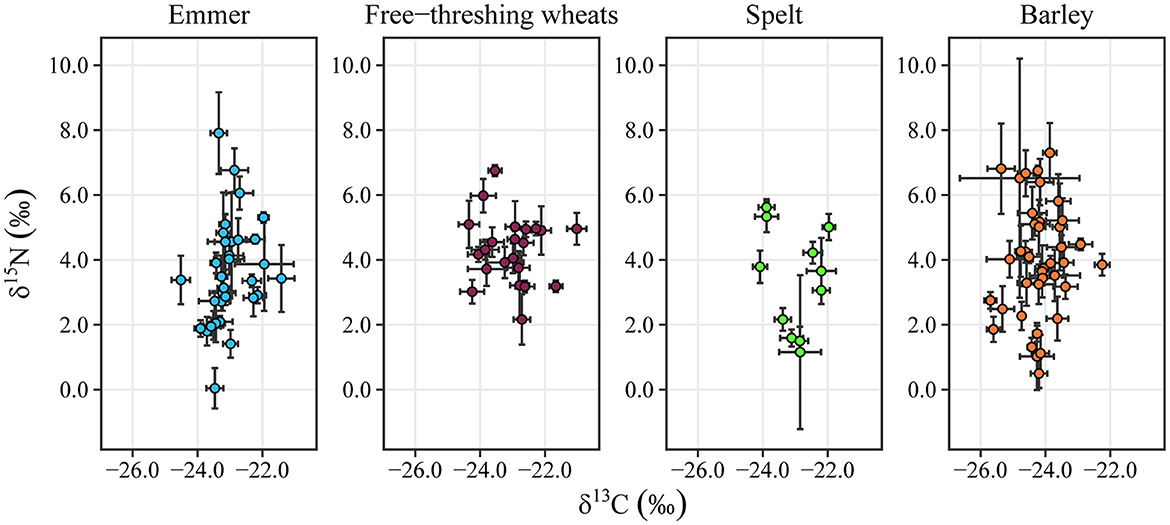
Figure 3. Stable nitrogen and carbon isotopic composition of archaeological cereal grains. Each point figures the average values of the 3–5 samples of 10 grains. Errorbars: 95% CI for each group.
3.2 Comparison with previously published data
The present study corpus and the one published in Aguilera et al. (2018) share common archaeological contexts. The pre-treatment protocol in the present study uses a more dilute acid wash and a shorter reaction time at a higher temperature than that used by Aguilera et al. (0.5 M HCl at 70°C for 1 h vs. 1–6 M HCl for 24 h at room temperature). Before joining these corpuses, we tested the reproducibility of the results between the two methods. Among cereals groups previously analyzed by Aguilera et al., seven were thus re-sampled and pretreated with the lighter acid wash. Figure 4 and Table 1 show that δ15N and δ13C values are globally similar for new and previously published data. Differences in δ15N values for spelt at Acy-Romance “La Warde” and free-threshing wheats at Houdan “Les Brosses” appear to be visible in Figure 4, but the reason remains unclear. The differences are not statistically significant according to the confidence interval (Table 1). Differences in the pretreatment protocol did not give rise to any systematic bias, as shown by Vaiglova et al. (2014) for moderate and slightly acid treatments and Aguilera et al. (2018) for higher concentration of acids. Therefore, no correction is necessary to combine the two datasets and Aguilera et al.' samples can be directly integrated into this study corpus.
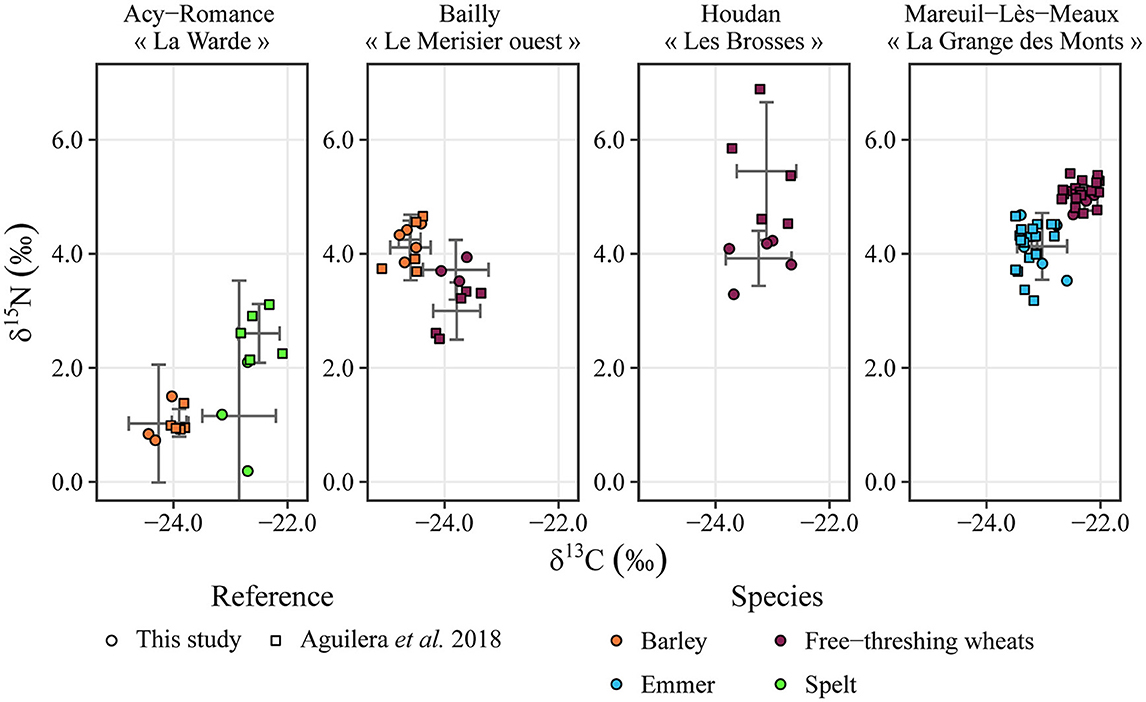
Figure 4. Stable nitrogen and carbon isotopic composition of samples common to both studies. Errorbars: 95% CI for each group.
3.3 Isotopic analyses of archaeological deer bone collagen
A total of 35 of the 41 analyzed archaeological deer bone collagen samples satisfied the established validity criteria (i.e., %N ≥ 4.8%, %C ≥ 13% and 2.9 ≤ atomic C:N ≤ 3.5; Ambrose, 1990; Van Klinken, 1999; Guiry and Szpak, 2021). The results are listed extensively in Supplementary Data Sheet 2 and are shown in Figure 5. The 35 deer bone collagen samples had collagen extraction yields ranging from 9.5 to 150.6 mg/g. Nitrogen contents ranged from 12.8 to 15.7%, carbon contents from 35.6 to 43.0% and C:N atomic ratios from 3.2 to 3.3. The δ15N values range from 3.6 to 7.9‰, with an average value of 5.6 ± 0.9‰. The δ13C values average of −22.1 ± 0.5‰, and range from −23.1 to −21.0‰.
3.4 Linear discriminant analysis
The discriminant analysis yielded promising results in classifying individuals into distinct manuring levels based on their δ15N values as unique predictor. The overall classification accuracy, i.e. the proportion of correct predictions, is 84%. Kappa is 0.76. Metric Kappa ranges from −1 to 1, with 0 indicating no better than random chance, and 1 indicating perfect agreement between predictions and true values. The confusion matrix (Table 1) illustrates the distribution of correct and incorrect classifications across different groups. The model achieved a high rate of true positives and true negatives, with minimal misclassifications.
3.5 Ordinal logistic regression
Ordinal logistic regression, computed in the same way as discriminant analysis, achieves greater accuracy (90%) and a better Kappa (0.85), indicating a higher level of predictive accuracy. The confusion matrix (Table 2) of ordinal logistic regression shows less misclassifications than discriminant analysis.
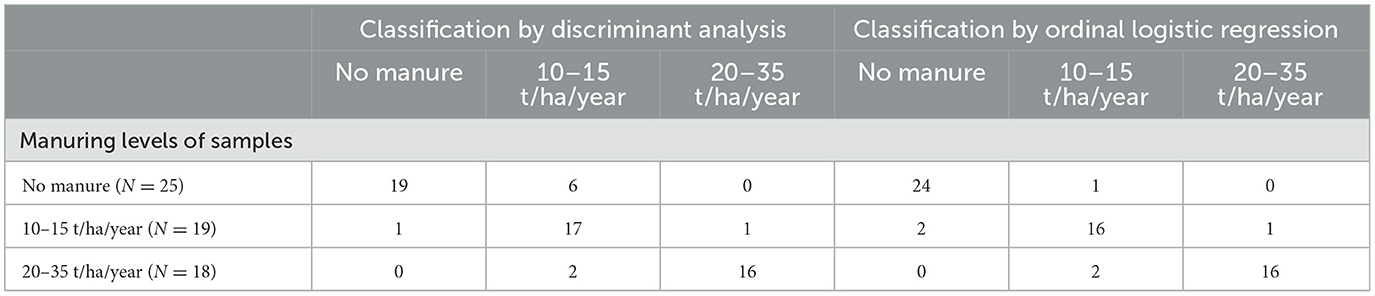
Table 2. Confusion matrix of linear discriminant analysis (left) and ordinal logistic regression (right).
4 Discussion
4.1 δ15N values baseline for unfertilised plants
4.1.1 Variability in deer bone collagen δ15N values
The deer bone collagen δ15N values can be used to calculate their diet δ15N value, which we use as an estimate of the δ15N value of plants in relatively non-anthropised environments. Analysis of deer bones from across the study area thus allows us to observe potential variations in the isotopic composition of their diet caused by the different geological environments encountered.
The overlap of δ15N values in space and time (Figure 6) reflects a certain similarity in the nitrogen isotopic composition of these deer bones, for the covered geographical area during the Second Iron Age and the Roman period. Only two sites have relatively low values, around 4‰ at Ifs “ZAC Object'Ifs Sud” (n = 2) and Jouars-Pontchartrain “La Ferme d'Ithe” (n = 10), whereas all the other sites yield very similar mean δ15N values ranging from 5.0 to 6.2‰. However, the Jouars-Pontchartrain “La Ferme d'Ithe” site is located some 20 km from Palaiseau “Les Trois Mares,” which has higher δ15N values (5.7 ± 0.7‰) (Aguilera et al., 2018). These two sites are both located in identical geological environments, at the interface of shallow valleys and a silty plateau covering a substrate of sands and sandstones (Blin et al., 1999; Giorgi, 2016). The geological unity of this environment raises questions regarding the reasons for the difference in δ15N values.
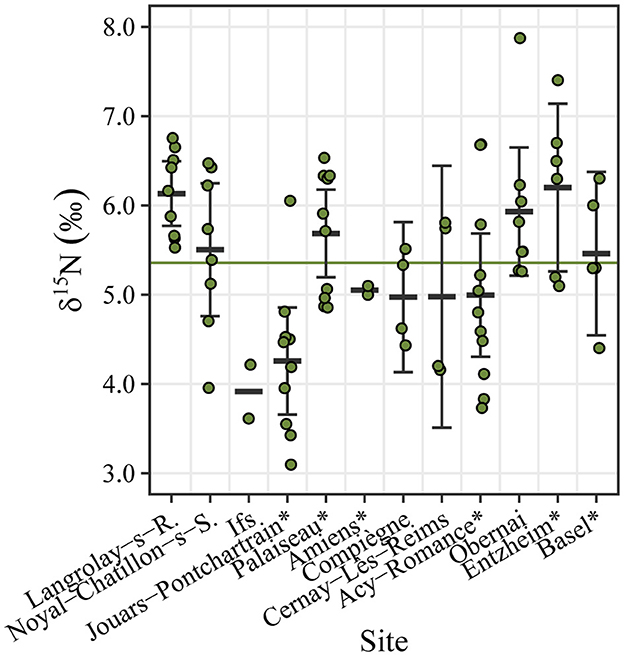
Figure 6. Stable nitrogen isotope composition of deer bone collagen per archaeological sites. Errorbars: 95% CI for each site. Green line: Average value of all samples. *Data from Mion et al. (2016), Knipper et al. (2017), Aguilera et al. (2018), and Schnitzler et al. (2018).
Even with several low values, the dispersion of δ15N values within each site is of the same magnitude as the variability between sites. The overlap of the archaeological values should not necessarily come as a surprise, as a similar finding has been made for present-day deer populations. Thirteen deer populations in Poland show the same or even greater dispersion of δ15N values and the overlap of values between sites (Sykut et al., 2020), for a study area similar in size to that of this study.
Given the overall dispersion in δ15N values in the corpus despite the large size of our study area (800 km between the westernmost and easternmost sites), we aggregated the 79 collagen samples, leading to an average δ15N value of 5.4 ± 1.0‰, or, after subtracting 4‰, a value of 1.4 ± 1.0‰ for diet, close to the δ15N values of modern unfertilised cereals (1.1 ± 1.5‰, Fraser et al., 2011). Thus, the experimental data from modern cereals are consistent with the δ15N values estimated for the archaeological wild flora in our study area and can therefore be used to model fertilization levels, since they show several well quantified manuring rates.
4.1.2 Classification of δ15N values by degree of fertilization
In order to assign a degree of fertilization to the archaeological cereal groups, we use ordinal logistic regression calculated from modern cereals, which produces better classification results than linear discriminant analysis. A break appears in the distribution of the posterior probability of classification, at 0.75 (Figure 7). We chose this somewhat arbitrary value as the threshold for accepting or rejecting the classification of δ15N values. A certain level of fertilization is assigned to cereal groups with a probability ≥0.75, whereas below this threshold classification is uncertain (Figure 8).
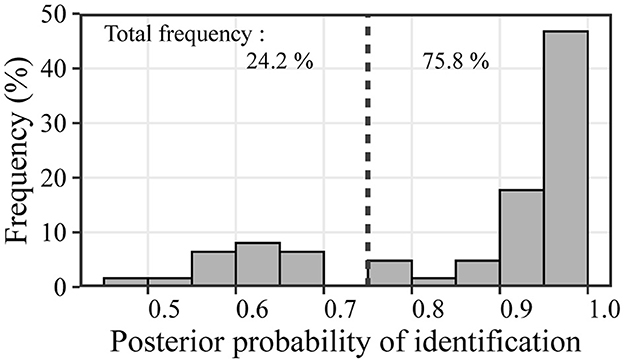
Figure 7. Histogram of posterior probabilities of classification based on the δ15N values of the experimental data (N = 62). The percentage of samples with a posterior probability above or below the acceptability threshold of 0.75 (dotted line) is given at the top of the figure. This percentage can be interpreted as the proportion of classifiable and unclassifiable samples, depending on the chosen threshold.
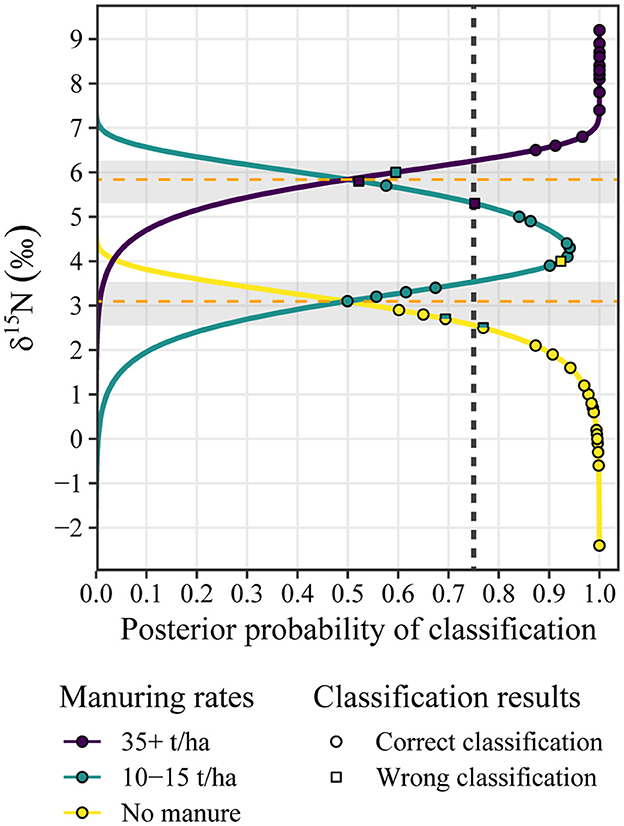
Figure 8. Distribution of posterior probabilities for each class, according to the predictor δ15N. Orange dotted line: class limits. Probabilities below the acceptance threshold (dotted line) define ranges of δ15N values for which assignment to a class is uncertain (gray bands).
The ordinal logistic regression gives threshold δ15N values equal to 3.1 and 5.8‰ discriminating the levels of fertilization intensity (Figure 8), very close to the habitually used referential values of 3 and 6‰ (since Bogaard et al., 2013). The uncertainty interval between no manuring and moderate or old manuring (defined by the probability line of 0.75) is between 2.6 and 3.5‰. The δ15N interval between 5.3 and 6.3‰ does not differentiate between moderate and high manuring.
Projecting the δ15N values estimated for wild flora (deer diet) alongside those of experimental modern cereals shows that the classification model can be applied to the archaeological record in Western Europe. As the overlap between the δ15N values of the experimental data shows, the allocation of a group of cereals to a level of manuring remains indicative, even if the introduction of the uncertainty ranges partially nuances the classification (Figure 9).
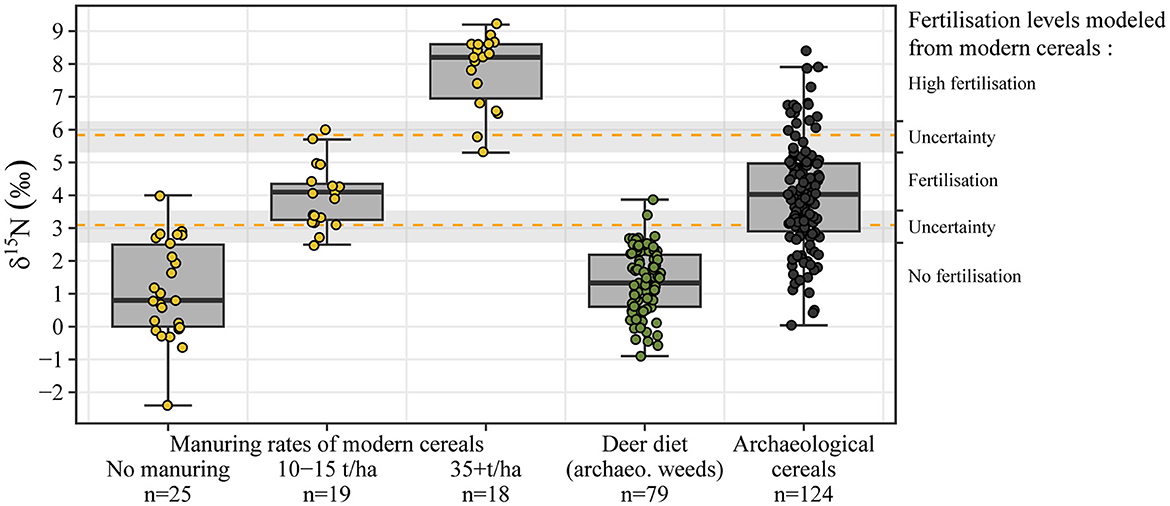
Figure 9. Stable nitrogen isotopic composition of modern cereals (yellow), archaeological wild flora (green) and archaeological cereals (black). Orange dotted line: class limits. Gray bands: Uncertainty intervals.
4.2 Fertilization during the Second Iron Age and the Roman period
4.2.1 Global fertilization intensity
Ordinal logistic regression was then used as a predictive model for the archaeological cereal groups (Supplementary Data Sheet 6). The distribution of results is shown in Figures 9, 10 (left). Ninteen percent of archaeological cereals are from non-fertilized fields (N = 24). A total of 74 (60%) groups shows fertilization use, mostly in the “Moderate fertilization” class (44%, N = 54), and a smaller proportion in the “Intense fertilization” class (10%, N = 13), with a few groups at the interface between these two levels of intensity (6%, N = 7). A large proportion of the groups show intermediate δ15N values between no fertilization and moderate fertilization (21%, N = 26), which indicates that manuring levels were generally relatively low for the studied periods.
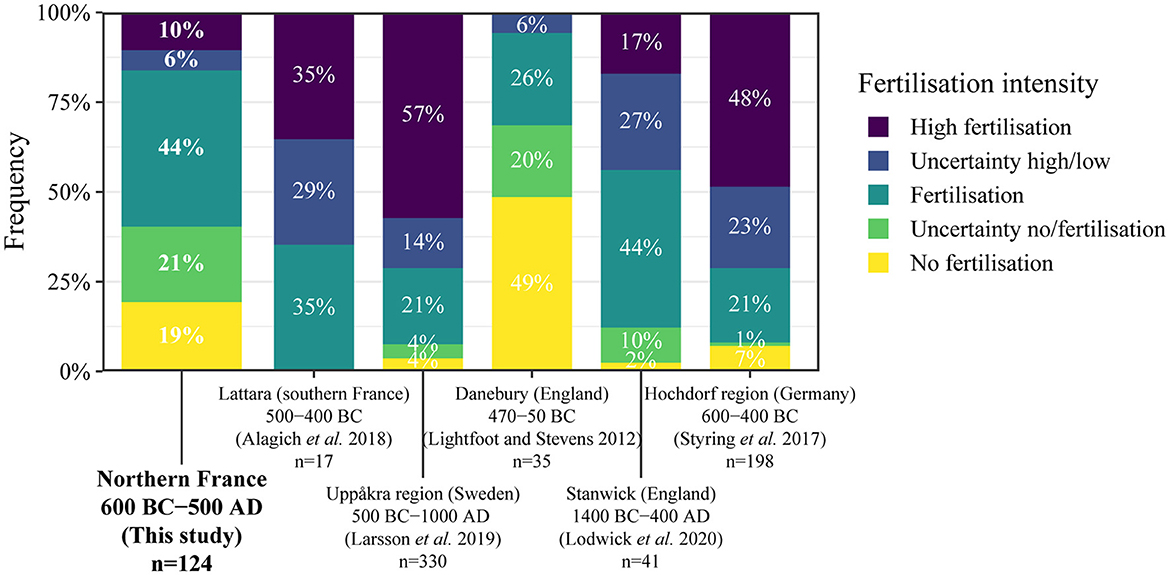
Figure 10. Distribution of fertilization intensity levels in this study (left) and different studies in Europe (right), for the Iron Age and Roman periods.
Other studies investigated fertilization regimes for the same protohistoric and antique periods in other European regions (Lightfoot and Stevens, 2012; Styring et al., 2017; Alagich et al., 2018; Larsson et al., 2019; Lodwick et al., 2020; Supplementary Data Sheet 7). Figure 10 (right) shows the fertilization levels modeled by ordinal logistic regression using the δ15N values published for these studies. In most cases, the δ15N values are higher than in our corpus, indicating that fertilization was more frequent than in the north of Gaul, with the exception of the site at Danebury (England), where the majority of cereals did not come from manured fields. The other sites around Danebury during Iron Age and Roman period seem to follow the same levels of fertilization intensity, reflecting the extensification of South Britain agriculture (Lodwick, 2023). The cause of these differences could be sought in variations in environmental conditions, but the social organization and types of agriculture (e.g. intensive vs. extensive agriculture) in these regions potentially generate stronger effects to explain the different fertilization regimes practiced. For example, the high δ15N values observed at Lattara, in the Uppåkra region and at Hochdorf can be achieved by an agropastoral society with sufficient livestock to produce the amount of manure required for intensive fertilization.
4.2.2 Inter-species comparison within archaeological feature
The results obtained for different cereals from the same archaeological feature are compared in Figure 11. These layers were probably deposited over a very short period of time and the cereals they contain are contemporaneous to within a few years of each other. This study therefore provides information on very short-term field fertilization practices, as well as practices in the life of a farm.
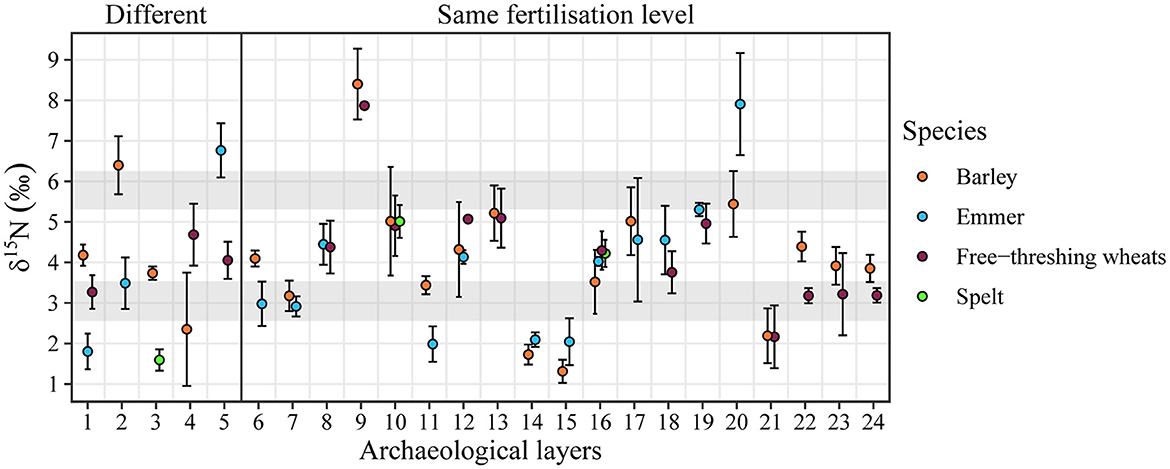
Figure 11. Stable nitrogen isotopic composition of cereal groups by archaeological layers. Different fertilization levels in one layer (left), same fertilization level in one layer (right). Layer identifier refers to Supplementary Data Sheet 8. Error bars: 95%CI for each cereal group.
Five features showed δ15N values reflecting different levels of manuring, indicating that the cereals come from different fields and that not all the fields in the farmland were subject to the same fertilization regime. The other 19 layers show similar δ15N values for cereals and it is not currently possible to determine whether the cereals were grown together as mixed crops in the same field, or cultivated in different fields with the same degree of fertilization.
4.2.3 Chronological and species variations
The projection of δ15N values for cereal groups according to their dates (Figure 12) shows some slight evolutions. Increasingly high δ15N values for certain groups of emmer and free-threshing wheats can be observed throughout the Second Iron Age. The highest values were reached in the 1st century BC and 1st century AD, for emmer, free-threshing wheats and hulled barley. For the first two species, the lowest values are also recorded during the same period. In other words, δ15N values are more dispersed for the 2nd century BC, 1st century BC and 1st century AD. This illustrates the diversity of cultivated soils with different manuring rates. These changes occur at the same time (in the second half of the Second Iron Age) as the transformation of agricultural production, from a certain diversification to specialization in one or two dominant cereals per rural settlement (Zech-Matterne et al., 2014).
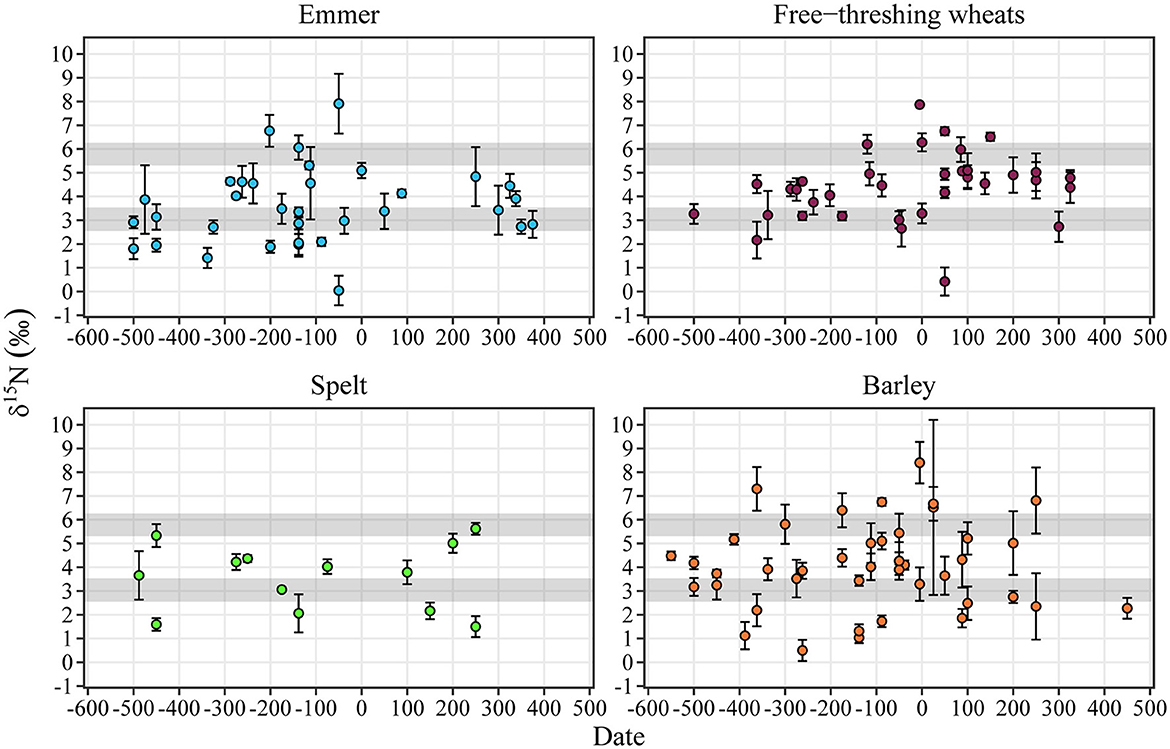
Figure 12. Stable nitrogen isotopic composition by chronology. Error bars: 95%CI for each cereal group.
δ15N values are less scattered after the 2nd century AD, particularly for emmer and free-threshing wheats, and remain stable during the Roman period, with perhaps a slight decrease in the 4th century AD. This could indicate a reduction in the contrast between fields and a standardization of fertilization across the farmland. In comparison, the dispersion of values for hulled barley is on average greater and remains very constant over the centuries. For spelt, the small number of groups shows no change between the 4th century BC and the 3rd century AD.
These slight variations can be compared with the ecological analyses of the weeds preserved with cereal harvests, which were carried out for the regions of the Massif Armorican, the Caen Plain, the center of the Paris Basin/Picardy/Champagne and a large eastern region (Zech-Matterne and Brun, 2016; Neveu, 2017; Zech-Matterne et al., 2017b). During the transition from the First to the Second Iron Age, most weeds were dependent on nutrient-rich soil. During the Second Iron Age, the flora was more tolerant to less nutrient-rich soils. The situation during the Roman period reveals the use of diversified soils of varying quality.
The lower δ15N values at the beginning of the Second Iron Age do not necessarily contradict the relatively high fertility of cultivated soils highlighted by weed assemblages. Indeed, this method, based on the ecology of archaeobotanical weeds, cannot distinguish between “natural” fertility and fertility maintained by soil fertilizers. Rural settlements during this period were mainly located in valley bottoms where the rich alluvial soils were cultivated. Fertilizer was not necessarily essential, which would explain the relatively lower values of emmer and free-threshing wheats from the first centuries of the Second Iron Age. Later, the dispersion of δ15N values for these two species reflects the diversification of soil quality. With the cultivation of plateau soils, the extensification of farming practices and the likely expansion of cultivated areas, soil types were more diversified and manure inputs were more unevenly distributed across farmlands. In the Paris Basin, weeds showed a decline in soil fertility from the 3rd century AD onwards and during Late Antiquity (Zech-Matterne et al., 2017b), which is consistent with the slight drop in δ15N values in the 4th century AD, due to the end of intense fertilization levels. Emmer cultivation declined during Antiquity, but fertilization continued on the few remaining fields.
In contrast, throughout the chronological sequence, the dispersion of hulled barley δ15N values, as well as their balanced distribution within the three levels of manuring intensity, reflects the ubiquitous status of this species in agricultural production during the Second Iron Age and the Roman period. Hulled barley can adapt to a wide range of growing conditions (resistance to cold, moisture or drought, and low nutrient requirements). Although less present in the samples, spelt seems to follow the same pattern. Spelt is also very flexible, but requires little fertilizer because of the risk of lodging if the soil is too rich (Toulemonde, 2013). Barley and spelt may have been grown more extensively than free-threshing wheats and emmer on a wide range of soils, but also included in rotation cycles, benefiting from the manuring of certain fields. On the other hand, emmer and free-threshing wheats, with less dispersed δ15N values, were cultivated on better prepared soils and in a rather similar way throughout the country, at least during the first centuries of the Second Iron Age and the last centuries of the Roman period. The extensification of their cultivation in the 2nd century BC-1st century AD certainly accompanied the economic development of Gaul, which was identified in many areas of society (Olmer, 2018).
4.2.4 Regional variations
The maps of the different sub-periods (Figure 13) confirm that for emmer and free-threshing wheats, the proportion of fertilized fields increased between the Second Iron Age and the Roman period. In the case of spelt and hulled barley, however, the situation varied throughout the country.
The situation in chalky Champagne (white circled dots) is different from that in other regions, where δ15N values for cereal groups are much lower than elsewhere: half of the groups were not fertilized (N = 7/12). This is particularly the case for hulled barley, the main cereal in the Champagne agropastoral system (Boulen et al., 2012; Toulemonde et al., 2017a,b), which is rarely grown on manured soils (N = 1/6). In this region with major soil constraints, the high calcium concentration, thinness and relative dryness of the soils limit crop potential (Boulen et al., 2012). The most widely grown cereals were resistant, easily adaptable species with low nutrient requirements (hulled barley and spelt). The absence of fertilization during most of the Second Iron Age could indicate that the constraints were such that livestock feeding also had to be limited and manure production capacities reduced. For pre-industrial societies, farming was difficult in this area. In the 18th century, the production limits encountered by rural settlements resulted in manure production capacities in Champagne being almost five times lower than elsewhere in Northern France (Meuvret, 1977).
Few Roman contexts were sampled in the Champagne region (N = 3). Two of them reflect the use of fertilizers on sites conductive to fertilizer production. The first is the villa at Cuperly “La Perte,” a large settlement in contrast to the size of previous farms, which was able to centralize a number of livestock and a certain quantity of manure. The second is difficult to interpret as it is an urban context (Reims “Rue Rockefeller,” Roman city of Durocortorum) and not a production site. The group of free-threshing wheats in question may have come from a farm close to the city, which may have benefited from the use of urban latrines and other organic wastes (Cordier, 2003).
5 Conclusion
The statistical treatment of the experimental data from Fraser et al. (2011) allowed us to redefine threshold values between fertilization levels. We propose considering δ15N values of European cereals in the ranges 2.6‰-3.5‰ and 5.3‰-6.3‰ as indeterminate manuring levels.
This global study shows that field fertilization was used throughout the studied area, during the Second Iron Age and the Roman period, mostly at relatively moderate levels. The wide range of δ15N values for cereals (from 0.0 to 8.4‰) reflects a diversity of manure inputs and field history. Differences in practices appear between regions and periods, where different cereal species may have been grown on more or less enriched soils. These observations are consistent with an extensive agropastoral system, where cultivated areas are spread out. In a social context where fertilizer resources and transport capacities are limited, fertilizer is either diluted over the entire farmland, or is concentrated on the fields closest to the farm, with less fertilization of the more distant fields. This extensive system emerged during the Second Iron Age and its development remains unclear beyond the first few centuries of the Roman period.
Data availability statement
The datasets presented in this study can be found in online repositories. The names of the repository/repositories and accession number(s) can be found in the article/Supplementary material.
Ethics statement
Ethical approval was not required for the study involving animals in accordance with the local legislation and institutional requirements because the fauna studied consists of archaeological remains.
Author contributions
SB: Data curation, Formal analysis, Methodology, Software, Visualization, Writing – original draft, Writing – review & editing. MB: Funding acquisition, Supervision, Writing – review & editing, Writing – original draft. DF: Data curation, Writing – review & editing. FM: Funding acquisition, Supervision, Writing – review & editing, Writing – original draft. MA: Data curation, Formal analysis, Methodology, Writing – original draft, Writing – review & editing. VM: Funding acquisition, Supervision, Writing – review & editing, Writing – original draft.
Funding
The author(s) declare financial support was received for the research, authorship, and/or publication of this article. This research has received funding from the Agence nationale de la recherche (French national research agency), MICA project-ANR-22-CE27-0026. This research was a part of a PhD funded by the DIM Région - Île-de-France “Matériaux Anciens et Patrimoniaux” (“AGROFER” project, dir. VM, MB, and FM). All analytical costs were supported by the MNHN ATM (“AgroFertil” project 2020; dir VM and MB) and by the Sorbonne Université OPUS programm (“AGALITT” project 2019, dir. MB and VM). Stable isotope analyses were conducted at the SSMIM (MNHN Paris).
Acknowledgments
We thank VM, Bénédicte Pradat, Françoise Toulemonde, Elsa Neveu, Geneviève Daoulas, Philippe Marinval and Caroline Schaal for studying the archaeobotanical assemblages. We thank Benoit Clavel, Karyne Debue, Svenja Höltkemeier, Sébastien Lepetz, Nicolas Morand, Élodie Sauvage and Rose-Marie Arbogast for identifying the deer bones. We thank Louise Byrne for English editing.
Conflict of interest
The authors declare that the research was conducted in the absence of any commercial or financial relationships that could be construed as a potential conflict of interest.
Publisher's note
All claims expressed in this article are solely those of the authors and do not necessarily represent those of their affiliated organizations, or those of the publisher, the editors and the reviewers. Any product that may be evaluated in this article, or claim that may be made by its manufacturer, is not guaranteed or endorsed by the publisher.
Supplementary material
The Supplementary Material for this article can be found online at: https://www.frontiersin.org/articles/10.3389/fearc.2024.1440714/full#supplementary-material
Supplementary Data Sheet 1. Archaeological cereal samples data and sites.
Supplementary Data Sheet 2. Archaeological deer samples data.
Supplementary Data Sheet 3. Archaeological cereal data by group.
Supplementary Data Sheet 4. Analysis of laboratory standards.
Supplementary Data Sheet 5. R scripts.
Supplementary Data Sheet 6. Data_cereals_groups.
Supplementary Data Sheet 7. Data_other studies in Europe.
Supplementary Data Sheet 8. Cereals_groups from a same context.
References
Aguilera, M., Zech-Matterne, V., Lepetz, S., and Balasse, M. (2018). Crop fertility conditions in North-Eastern Gaul during the La Tène and Roman periods: a combined stable isotope analysis of archaeobotanical and archaeozoological remains. Environ. Archaeol. 23, 323–337. doi: 10.1080/14614103.2017.1291563
Alagich, R., Gardeisen, A., Alonso, N., Rovira, N., and Bogaard, A. (2018). Using stable isotopes and functional weed ecology to explore social differences in early urban contexts: the case of lattara in Mediterranean France. J. Archaeol. Sci. 93, 135–149. doi: 10.1016/j.jas.2018.03.006
Ambrose, S. H. (1990). Preparation and characterization of bone and tooth collagen for isotopic analysis. J. Archaeol. Sci. 17, 431–451. doi: 10.1016/0305-4403(90)90007-R
Amundson, R., Austin, A. T., Schuur, E. A. G., Yoo, K., Matzek, V., Kendall, C., et al. (2003). Global Patterns of the isotopic composition of soil and plant nitrogen. Glob. Biogeochem. Cycles 17, 1–11. doi: 10.1029/2002GB001903
Barral, P., and Fichtl, S. (2012). “Regards sur la chronologie de la fin de l'âge du Fer, IIIe-Ier siècle avant J.-C.,” en Gaule non méditerranéenne: actes de la table ronde tenue à Bibracte, Glux-en-Glenne, 15-17 octobre 2007. Collection Bibracte 22. Bibracte: Glux-en-Glenne.
Bellanger, L., and Tomassone, R. (2014). Exploration de données et méthodes statistiques: data analysis and data mining avec le logiciel R. Références sciences. Paris: Ellipses.
Bertrand, I., Duval, A., Gomez de Soto, J., and Maguer, P., (eds.) (2009). Habitats et Paysages Ruraux En Gaule et Regards Sur d'autres Régions Du Monde Celtique: Actes Du XXXIe Colloque International de l'Association Française Pour l'étude de l'âge Du Fer, 17-20 Mai 2007, Chauvigny (Vienne F). Mémoire 35. Chauvigny: Association des publications chauvinoises.
Besnard-Vauterin, C.-C., Giraud, P., Lepaumier, H., and Giazzon, D. (2016). “Genèse d'un Réseau de Fermes Du Second Âge Du Fer En Plaine de Caen, in Évolution Des Sociétés Gauloises Du Second Âge Du Fer, Entre Mutations Internes et Influences Externes. Actes Du 38e Colloque International de l'AFEAF, Amiens, 29 Mai - 1er Juin 2014, eds. Ge. Blancquaert, and F. Malrain, 61–81. Revue Archéologique de Picardie, N° Spécial 30-2016. Revue archéologique de Picardie.
Blancquaert, G., Leroyer, C., Lorho, T., Malrain, F., and Zech-Matterne, V. (2012). “Rythmes de créations et d'abandons des établissements ruraux du second âge du fer et interactions environnementales,” in Variabilités Environnementales, Mutations Sociales: Nature, Intensités, Échelles et Temporalités Des Changements, XXXIIe Rencontres Internationales d'archéologie et d'histoire d'Antibes, eds. F. Bertoncello, and F. Braemer (Antibes: Association pour la Promotion et la Diffusion des Connaissances archéologiques), 233–245.
Blin, O., Bernard, V., Dietrich, A., Gebhardt, A., Marguerie, D., Michel Morin, J., et al. (1999). Impact Anthropique et Gestion Du Milieu Durant l'Antiquité. L'approche paléo-environnementale pluridisciplinaire du site de ‘la ferme d'ithe' à jouars-pontchartrain (Yvelines). Nouv. Aarchéol. 78, 45–56.
Bocherens, H., Fizet, M., Mariotti, A., Lange-Badre, B., Vandermeersch, B., Borel, J. P., et al. (1991). Isotopic biogeochemistry (13C,15N) of fossil vertebrate collagen: application to the study of a past food web including neandertal man. J. Hum. Evol. 20, 481–492. doi: 10.1016/0047-2484(91)90021-M
Bogaard, A., Fraser, R., Heaton, T. H. E., Wallace, M., Vaiglova, P., Charles, M., et al. (2013). Crop manuring and intensive land management by europe's first farmers. Proc. Natl. Acad. Sci. 110, 12589–12594. doi: 10.1073/pnas.1305918110
Bol, R., Eriksen, J., Smith, P., Garnett, M. H., Coleman, K., Christensen, B. T., et al. (2005). The natural abundance of 13C, 15N, 34S and 14C in archived (1923–2000) Plant and soil samples from the askov long-term experiments on animal manure and mineral fertilizer. Rapid Commun. Mass Spectrom. 19, 3216–3226. doi: 10.1002/rcm.2156
Boulen, M., Créteur, Y., Fechner, K., Loicq, S., and Zech-Matterne, V. (2012). “Environnement et exploitation des sols autour des sites protohistoriques champenois. Première confrontation des résultats archéobotaniques, malacologiques et pédologiques,” in L'Âge du fer entre la Champagne et la Vallée du Rhin: 34e colloque international de l'Association française pour l'étude de l'âge du fer du 13 au 16 mai 2010 à Aschaffenburg, eds. M. Schönfelder, and S. Sievers (Mainz: Verl. des Römisch-Germanisches Zentralmuseums), 41–76. RGZM-Tagungen, Band 14..
Brinkkemper, O., Braadbaart, F., van Os, B., van Hoesel, A., van Brussel, A. A. N., Fernandes, R., et al. (2018). Effectiveness of different pre-treatments in recovering pre-burial isotopic ratios of charred plants. Rapid Commun. Mass Spectrom. 32, 251–261. doi: 10.1002/rcm.8033
Buchsenschutz, O. (2018). “Les habitats de l'âge du fer en france du IVe au ier avant notre ère,” in La Protohistoire de La France, Actes Du XVIIIe Congrès UISPP Tenu à Paris, 4-9 Juin 2017, eds. J. Guilaine, and D. Garcia (Paris: Hermann), 441–454. doi: 10.3917/herm.garci.2018.01.0404
Charles, M., Jones, G., and Hodgson, J. (1997). FIBS in archaeobotany: functional interpretation of weed floras in relation to husbandry practices. J. Archaeol. Sci. 24, 1151–1161. doi: 10.1006/jasc.1997.0194
Choi, W. J., Arshad, M. A., Chang, S. X., and Kim, T. H. (2006). Grain 15N of crops applied with organic and chemical fertilizers in a four-year rotation. Plant Soil 284, 165–174. doi: 10.1007/s11104-006-0038-8
Cordier, P. (2003). “Le destin urbain du stercus et de l'urine,” in La ville et ses déchets dans le monde romain: rebuts et recyclages, eds. P. Ballet, P. Cordier, and N. Dieudonné-Glad (Montagnac: Édition M. Mergoil), 51–60.
Duval, C., and Clavel, B. (2018). Boeufs gaulois et boeufs français: morphologies animales et dynamiques économiques au cours de La Tène et des périodes historiques. Gallia. Archéologie des Gaules 75, 141–171. doi: 10.4000/gallia.3904
Evin, A., Cucchi, T., Cardini, A., Vidarsdottir, U. S., Larson, G., Dobney, K., et al. (2013). The Long and winding road: identifying pig domestication through molar size and shape. J. Archaeol. Sci. 40, 735–743. doi: 10.1016/j.jas.2012.08.005
Fraser, R. A., Bogaard, A., Charles, M., Styring, A. K., Wallace, M., Jones, G., et al. (2013). Assessing natural variation and the effects of charring, burial and pre-treatment on the stable carbon and nitrogen isotope values of archaeobotanical cereals and pulses. J. Archaeol. Sci. 40, 4754–4766. doi: 10.1016/j.jas.2013.01.032
Fraser, R. A., Bogaard, A., Heaton, T., Charles, M., Jones, G., Christensen, B. T., et al. (2011). Manuring and stable nitrogen isotope ratios in cereals and pulses: towards a new archaeobotanical approach to the inference of land use and dietary practices. J. Archaeol. Sci. 38, 2790–2804. doi: 10.1016/j.jas.2011.06.024
Gautier, É., Marcigny, C., and Barral, P. (2018). “Le paysage anthropisé et son évolution de la fin du iiie millénaire au début de notre ère en France Métropolitaine,” in La Protohistoire de La France, Actes Du XVIIIe Congrès UISPP Tenu à Paris, 4-9 Juin 2017, eds. J. Guilaine, and D. Garcia (Paris: Hermann), 355–370. doi: 10.3917/herm.garci.2018.01.0356
Giorgi, C. (2016). Île-de-France, Essone (91), Palaiseau, Lieu-Dit ≪ Les Trois Mares ≫, Avenue de La Vauve. Rapport final d'opération, Fouille archéologique. Paris: Inrap, SRA Île-de-France.
Gransar, F. (2002). Le Stockage Alimentaire à l'âge Du Fer En Europe Tempérée. Thèse de doctorat en Archéologie, sous la direction de Buchsenschutz Olivier, Paris: Paris 1.
Guiry, E. J., and Szpak, P. (2021). Improved quality control criteria for stable carbon and nitrogen isotope measurements of ancient bone collagen. J. Archaeol. Sci. 132:105416. doi: 10.1016/j.jas.2021.105416
Hart, J. P., and Feranec, R. S. (2020). Using maize δ15N values to assess soil fertility in Fifteenth- and Sixteenth-Century ad Iroquoian Agricultural Fields. PLOS ONE 15:e0230952. doi: 10.1371/journal.pone.0230952
Högberg, P. (1997). Tansley review No. 95 15N natural abundance in soil-plant systems. New Phytol. 137, 179–203. doi: 10.1046/j.1469-8137.1997.00808.x
Knipper, C., Pichler, S. L., Rissanen, H., Stopp, B., Kühn, M., Spichtig, N., et al. (2017). What is on the menu in a Celtic Town? Iron age diet reconstructed at Basel-Gasfabrik, Switzerland. Archaeol. Anthropol. Sci. 9, 1307–1326. doi: 10.1007/s12520-016-0362-8
Kovarovic, K., Aiello, L. C., Cardini, A., and Lockwood, C. A. (2011). Discriminant function analyses in archaeology: are classification rates too good to be true? J. Archaeol. Sci. 38, 3006–3018. doi: 10.1016/j.jas.2011.06.028
Larsson, M., Bergman, J., and Lagerås, P. (2019). Manuring practices in the first millennium AD in Southern Sweden Inferred from isotopic analysis of crop remains. PLOS ONE 14:e0215578. doi: 10.1371/journal.pone.0215578
Lepetz, S., and Morand, N. (2017). “Archéozoologie Des Territoires Du Nord-Est de La France, du second âge du fer à l'antiquité tardive: banque de données et éléments de synthèse,” in Productions Agro-Pastorales, Pratiques Culturales et Élevage Dans Le Nord de La Gaule Du Deuxième Siècle Avant J.-C. à La Fin de La Période, Actes de La Table Ronde Internationale Des 8 et 9 Mars 2016 à l'Institut National d'histoire de l'art (Paris), eds. S. Lepetz, and V. Zech-Matterne (Quint-Fonsegrives: Édition M. Mergoil), 43–62. Archéologie Des Plantes et Des Animaux.
Lightfoot, E., and Stevens, R. E. (2012). Stable isotope investigations of Charred Barley (Hordeum Vulgare) and wheat (Triticum Spelta) grains from danebury hillfort: implications for palaeodietary reconstructions. J. Archaeol. Sci. 39, 656–662. doi: 10.1016/j.jas.2011.10.026
Lodwick, L. (2023). Cultivating villa economies: archaeobotanical and isotopic evidence for iron age to roman agricultural practices on the chalk downlands of Southern Britain. Eur. J. Archaeol. 26, 445–466. doi: 10.1017/eaa.2022.47
Lodwick, L., Campbell, G., Crosby, V., and Müldner, G. (2020). Isotopic evidence for changes in cereal production strategies in iron age and Roman Britain. Environ. Archaeol. 2020:1718852. doi: 10.1080/14614103.2020.1718852
Malrain, F. (2023a). Les domaines ruraux du nord de la Gaule, une archéologie historique du second âge du Fer. CNRS Éditions. Available at: https://inrap.hal.science/hal-04107142
Malrain, F. (2023b). “L'outillage agricole dans les établissements ruraux laténiens en Gaule du Nord,” in L'outillage agropastoral en Gaule: IIe av.-VIe s. ap, eds. M. Kasprzyk, and N. Tisserand (Dijon: Éditions universitaires de Dijon), 37–72. Art, archéologie and patrimoine.
Malrain, F., Blancquaert, G., and Lorho, T. eds. (2013). L'habitat rural du second âge du Fer: rythmes de création et d'abandon au nord de la Loire. Paris: CNRS Éditions, Inrap.
Malrain, F., Zech-Matterne, V., Blanquaert, G., Auxiette, G., Leroyer, C., Lorho, T., et al. (2015). “Apprehending continuity and discontinuity in iron age soil occupation and rural landscape through a collective database,” in Persistant Economic Ways of Living: Production, Distribution and Consumption in Late Préhistory and Early History, eds. A. Danielisova, and M. Fernandez-Götz (Budapest: Archaeolingua Alapitvany), 137–144.
Matterne, V. (2001). Agriculture et alimentation végétale durant l'âge du fer et l'époque gallo-romaine en France septentrionale. Montagnac: Édition M. Mergoil.
Meuvret, J. (1977). Le Problème Des Subsistances à l'époque Louis XIV: I La Production Des Céréales Dans La France Du XVIIe et Du XVIII Siècle. Civilisations et Sociétés 50. Paris-La Haye: Mouton. doi: 10.1515/9783111608662
Mion, L., Herrscher, E., Blondiaux, J., Binet, E., and Andre, G. (2016). Comportements alimentaires en gaule du nord: étude isotopique du site de l'îlot de la boucherie (IIIe–Ve Siècles Apr. J.-C.) à Amiens. Bull. Mém. Soc. Anthropol. Paris 28, 155–175. doi: 10.1007/s13219-016-0164-7
Neveu, E. (2017). Évolution Des Agricultures Dans Le Nord-Ouest de La France de l'âge de Bronze à l'époque Gallo-Romaine [Thèse en Archéologie, sous la direction de Serge Cassen], Nantes: Université de Nantes.
Neveu, E., Zech-Matterne, V., Brun, C., Dietsch-Sellami, M.-F., Durand, F., Toulemonde, F., et al. (2021). New insights into agriculture in northwestern france from the bronze age to the late iron age: a weed ecological approach. Veg. Hist. Archaeobot. 30, 47–61. doi: 10.1007/s00334-020-00817-8
Nitsch, E., Charles, M., and Bogaard, A. (2015). Calculating a statistically ROBUST δ13C and δ15N offset for charred cereal and pulse seeds. Sci. Technol. Archaeol. Res. 1, 1–8. doi: 10.1179/2054892315Y.0000000001
Olmer, F. (2018). “Le commerce et les importations en gaule au second âge du fer,” in La Protohistoire de La France, Actes Du XVIIIe Congrès UISPP Tenu à Paris, 4-9 Juin 2017, eds. J. Guilaine, and D. Garcia (Paris: Hermann), 453–469.
Ouzoulias, P. (2006). L'économie agraire de la Gaule: aperçus historiographiques et perspectives archéologiques [Thèse de doctorat en Archéologie, sous la direction de François Favory]. Besançon: Université de Franche-Comté. Available at: https://tel.archives-ouvertes.fr/tel-00011567
Petit, C., Bernigaud, N., Binois, A., Camizuli, E., Fajon, P., Fechner, K., et al. (2018). “Conditions environnementales de l'exploitation des espaces ruraux en Gaule du Nord,” in Gallia rvstica 2: les campagnes du nord-est de la Gaule, de la fin de l'âge du Fer à l'Antiquité tardive, ed. M. Reddé (Bordeaux: Ausonius Éditions), 31–82. Mémoires 50.
Reddé, M. (2018a). “De La Tène finale à l'Empire, la dynamique d'occupation du sol,” in Gallia rvstica 2: les campagnes du nord-est de la Gaule, de la fin de l'âge du Fer à l'Antiquité tardive, ed. M. Reddé (Bordeaux: Ausonius Éditions), 485–500. Mémoires 50.
Reddé, M., (ed.). (2018b). Gallia rvstica 2: les campagnes du nord-est de la Gaule, de la fin de l'âge du Fer à l'Antiquité tardive. Mémoires 50. Bordeaux: Ausonius Éditions.
Reddé, M., Bernigaud, N., Lepetz, S., and Zech-Matterne, V. (2018). “Les conditions du développement économique. II. Les marchés,” in Gallia rvstica 2: les campagnes du nord-est de la Gaule, de la fin de l'âge du Fer à l'Antiquité tardive, edi. M. Reddé (Bordeaux: Ausonius Éditions), 519–584. Mémoires 50.
Schnitzler, A., Granado, J., Putelat, O., Arbogast, R.-M., Drucker, D., Eberhard, A., et al. (2018). Genetic diversity, genetic structure and diet of ancient and contemporary red deer (Cervus Elaphus L.) from North-Eastern France. PLOS ONE 13:e0189278. doi: 10.1371/journal.pone.0189278
Styring, A., Maier, U., Stephan, E., Schlichtherle, H., and Bogaard, A. (2016). Cultivation of choice: new insights into farming practices at Neolithic lakeshore sites. Antiquity 90, 95–110. doi: 10.15184/aqy.2015.192
Styring, A., Rösch, M., Stephan, E., Stika, H.-P., Fischer, E., Sillmann, M., et al. (2017). Centralisation and long-term change in farming regimes: comparing agricultural practices in Neolithic and Iron Age south-west Germany. Proc. Prehist. Soc. 83, 357–381. doi: 10.1017/ppr.2017.3
Styring, A. K., Manning, H., Fraser, R. A., Wallace, M., Jones, G., Charles, M., et al. (2013). The Effect of charring and burial on the biochemical composition of cereal grains: investigating the integrity of archaeological plant material. J. Archaeol. Sci. 40, 4767–4779. doi: 10.1016/j.jas.2013.03.024
Sykut, M., Pawełczyk, S., Borowik, T., Pokorny, B., Flajšman, K., Niedziałkowska, M., et al. (2020). Intraindividual and interpopulation variability in carbon and nitrogen stable isotope ratios of bone collagen in the modern red deer (Cervus Elaphus). J. Archaeol. Sci. Rep. 34:102669. doi: 10.1016/j.jasrep.2020.102669
Szpak, P. (2014). Complexities of nitrogen isotope biogeochemistry in plant-soil systems: implications for the study of ancient agricultural and animal management practices. Front. Plant Sci. 5:288. doi: 10.3389/fpls.2014.00288
Toulemonde, F. (2013). Economie Végétale et Pratiques Agricoles Au Bronze Final et Au Premier Âge Du Fer, de La Côte de l'Île-de-France à La Côte de Champagne [Thèse de doctorat en Archéologie et Environnement, sous la direction de Stéphanie Thiébault]. Paris: Université Paris 1 Panthéon-Sorbone. Available at: http://www.theses.fr/2013PA010657
Toulemonde, F., Zech-Matterne, V., and Bandelli, A. (2017a). Diversité des productions végétales et animales dans les campagnes champenoises et leur capitale de cité: études archéobotaniques et archéozoologiques récentes à et autour de reims/durocortorum,” in Productions Agro-Pastorales, Pratiques Culturales et Élevage Dans Le Nord de La Gaule Du Deuxième Siècle Avant J.-C. à La Fin de La Période, Actes de La Table Ronde Internationale Des 8 et 9 Mars 2016 à l'Institut National d'histoire de l'art (Paris), eds. S. Lepetz, and V. Zech-Matterne (Quint-Fonsegrives: Édition M. Mergoil), 91–102. Archéologie Des Plantes et Des Animaux.
Toulemonde, F., Zech-Matterne, V., Wiethold, J., Brun, C., Malrain, F., Riquier, V., et al. (2017b). “Reconstitution Des pratiques agricoles du Ier Millénaire a.C. En France Orientale, d'après Le croisement des données carpologiques et archéologiques,” in Production et Proto-Industrialisation Aux Âges Du Fer. Perspectives Sociales et Environnementales, Actes Du 39e Colloque International de l'Association Française Pour l'Étude de l'Âge Du Fer (Nancy 14-17 Mai 2015), Vol. 47 eds. by S. Marion, S. Deffressigne-Tikonoff, J. Kaurin, and G. Bataille (Bordeaux: Ausonius Éditions), 29–50. Mémoires. Available at: https://hal.archives-ouvertes.fr/hal-03176627
Vaiglova, P., Snoeck, C., Nitsch, E., and Bogaard, A. J Lee-Thorp. (2014). Impact of contamination and pre-treatment on stable carbon and nitrogen isotopic composition of charred plant remains. Rapid Commun. Mass Spectrom. 28, 2497–2510. doi: 10.1002/rcm.7044
Van Andringa, W. (2015). Le cens, l'autel et la ville chef-lieu: auguste et l'urbanisation des Trois Gaules. Gallia. Archéol. Gaules 72, 19–33. doi: 10.4000/gallia.2637
Van Klinken, G. J. (1999). Bone collagen quality indicators for palaeodietary and radiocarbon measurements. J. Archaeol. Sci. 26, 687–695. doi: 10.1006/jasc.1998.0385
Wang, X., Fuller, B. T., Zhang, P., Hu, S., Hu, Y., Shang, X., et al. (2018). Millet manuring as a driving force for the late neolithic agricultural expansion of North China. Sci. Rep. 8:5552. doi: 10.1038/s41598-018-23315-4
Zech-Matterne, V., Bonnaire, E., Daoulas, G., Derreumaux, M., Durand, F., Rousselet, O., et al. (2017a). “Diversité et évolution des productions céréalières et fruitières dans le quart nord-est de la france, d'après les données carpologiques (IIe s. Av. J.-C. - Ve s. Ap. J.-C.),” in Productions Agro-Pastorales, Pratiques Culturales et Élevage Dans Le Nord de La Gaule Du Deuxième Siècle Avant J.-C. à La Fin de La Période, Actes de La Table Ronde Internationale Des 8 et 9 Mars 2016 à l'Institut National d'histoire de l'art (Paris), eds. S. Lepetz, and V. Zech-Matterne (Quint-Fonsegrives: Édition M. Mergoil), 43–62. Archéologie Des Plantes et Des Animaux.
Zech-Matterne, V., Bouby, L., Bouchette, A., Cabanis, M., Derreumaux, M., Durand, F., et al. (2009). “L'agriculture Du VIe Au Ier Siècle Avant J.-C. En France: État Des Recherches Carpologiques Sur Les Établissements Ruraux,” in Habitats et Paysages Ruraux En Gaule et Regards Sur d'autres Régions Du Monde Celtique: Actes Du XXXIe Colloque International de l'Association Française Pour l'Etude de l'Âge Du Fer, eds. I. Bertrand, A. Duval, and J. Gomez de Soto (Chauvigny: Association des publications chauvinoises), 383–416.
Zech-Matterne, V., and Brun, C. (2016). “Vers une agriculture extensive ? Etude diachronique des productions végétales et des flores adventices associées, au cours de la période Laténienne, En France Septentrionale,” in Évolution Des Sociétés Gauloises Du Second Âge Du Fer, Entre Mutations Internes et Influences Externes, Actes Du 38e Colloque International de l'AFEAF, Amiens, 29 Mai - 1er Juin 2014, eds. G. Blancquaert, and F. Malrain (Amiens: Revue Archéologique de Picardie), 623–638.
Zech-Matterne, V., Brun, C., Toulemonde, F., and Wiethold, J. (2017b). “Essai d'approche Du Mode d'exploitation Des Terres et Des Pratiques Agricoles Dans Le Nord-Est de La France, à Partir de l'évolution Des Flores Adventices (IIe s. Av. J.-C. - Ve s. Ap. J.-C.),” in Productions Agro-Pastorales, Pratiques Culturales et Élevage Dans Le Nord de La Gaule Du Deuxième Siècle Avant J.-C. à La Fin de La Période Romaine, Actes de La Table Ronde Internationale Des 8 et 9 Mars 2016 à l'Institut National d'histoire de l'art (Paris), eds. S. Lepetz, and V. Zech-Matterne (Quint-Fonsegrives: Édition M. Mergoil), 63–74. Archéologie Des Plantes et Des Animaux.
Zech-Matterne, V., Malrain, F., Bossard, S., Lorho, T., Derreumaux, M., Neveu, E., et al. (2020). Crop Productions, Forms and Sustainability of the Iron Age Farmsteads in Northern France: Differences and Convergences. Beiträge Zur Frühgeschichte Mitteleuropas 93, 9–22.
Zech-Matterne, V., Wiethold, J., and Pradat, B. (2014). “L'essor des blés nus en france septentrionale: systèmes de culture et commerce céréalier autour de la conquête césarienne et dans les siècles qui suivent,” in Consommer Dans Les Campagnes de La Gaule Romaine, Actes Du Xe Congrès de l'Association AGER, eds. X. Deru, and R. González Villaescusa (Lille: Revue du Nord), 23–49, Hors-série, 21.
Keywords: manuring, crop stable isotopes, rural settlements, France, iron age, Roman period
Citation: Ben Makhad S, Balasse M, Fiorillo D, Malrain F, Aguilera M and Matterne V (2024) The fertilization of fields in the northern half of France (600 BCE−500 CE), approached by N isotope analyses of cereal remains. Front. Environ. Archaeol. 3:1440714. doi: 10.3389/fearc.2024.1440714
Received: 29 May 2024; Accepted: 25 July 2024;
Published: 16 August 2024.
Edited by:
Amy Styring, University of Oxford, United KingdomReviewed by:
Alessandra Varalli, UMR7269 Laboratoire méditerranéen de préhistoire Europe Afrique (LAMPEA), FranceCharlotte Diffey, University of Oxford, United Kingdom
Copyright © 2024 Ben Makhad, Balasse, Fiorillo, Malrain, Aguilera and Matterne. This is an open-access article distributed under the terms of the Creative Commons Attribution License (CC BY). The use, distribution or reproduction in other forums is permitted, provided the original author(s) and the copyright owner(s) are credited and that the original publication in this journal is cited, in accordance with accepted academic practice. No use, distribution or reproduction is permitted which does not comply with these terms.
*Correspondence: Sammy Ben Makhad, c2FtbXkuYmVubWFraGFkJiN4MDAwNDA7Z21haWwuY29t