- 1Department of Anthropology, Washington University in St. Louis, St. Louis, MO, United States
- 2Department of Archaeology, Max Planck Institute of Geoanthropology, Jena, Germany
- 3Domestication and Anthropogenic Evolution Research Group, Max Planck Institute of Geoanthropology, Jena, Germany
- 4School of Archaeology and Anthropology, Australian National University, Canberra, ACT, Australia
- 5Department of Archaeology, Center for Archaeological Science, Sichuan University, Chengdu, China
- 6Institute of Archaeology, Chinese Academy of Social Sciences, Beijing, China
- 7School of Cultural Heritage, Northwest University, Xi'an, China
High-altitude conditions on the Tibetan Plateau are often depicted as an inhospitable environment for conventional farming, yet evidence shows that communities in western Tibet grew ecologically hardy crops such as 6-row barley (Hordeum vulgare) by at least the 1st millennium BCE, at locations above 4,000 meters above sea level (masl). However, little is known about the specific cultivation strategies and culinary traditions that these agropastoral communities developed. Stable carbon and nitrogen isotope compositions of grains inform growing conditions and provide much needed insight into the cultivation strategies in such a unique environment. We use δ13C and δ15N values of archaeologically recovered barley remains to investigate past watering and soil-management strategies. Our results infer high labor investment in manuring and watering in barley farming. This suggests an intensive cultivation system in Western Tibet, 1,000 BCE −1,000 CE, despite the high-altitude pastoral landscape.
1 Introduction
We present a stable isotope study on ancient plant remains from the Tibetan Plateau to explore the cultivation strategies employed by herding communities during the 1st millennium BCE and 1st millennium CE. Through carbon and nitrogen stable isotope analyses of 6-row barley (Hordeum vulgare) grains recovered from three sites, representing some of the oldest evidence of grain cultivation in the region, we inquire whether these early highlanders developed intensive cultivation activities in environments optimal for transhumant animal pasturing. We further investigate the influence of growing conditions on barley grain size. Our results from Piyang, Dingdong, and Jiweng provide critical insight into early barley cultivation and labor strategies in western Tibet.
Research shows that this region was at a major crossroad of the trans-Eurasian exchange of crops and livestock, among other commodities, situated along a southern route of the eastern dispersal of the Fertile Crescent cereals, particularly barley (Hordeum spp.) (Liu et al., 2017; Lister et al., 2018; Gao et al., 2021). This route parallels (albeit a millennium later) the well-documented northern route through the Inner Asian Mountain Corridor, a series of foothill locations linking today's eastern Kyrgyzstan, Uzbekistan, and Kazakhstan and western Xinjiang, which were intensively utilized by ancient communities between the 3rd and 1st millennium BCE (Frachetti et al., 2010; Jones et al., 2016; Liu et al., 2019). The Eurasian food globalization process involved agropastoral communities across Inner Asia cultivating and transporting Southwest Asian crops such as free-threshing wheat (Triticum aestivum/durum) and barley and managing livestock such as sheep (Ovis aries), goat (Capra hircus), horse (Equus caballus), and cattle (Bos taurus). The spread of broomcorn (Panicum miliaceum) and foxtail millet (Setaria italica) from their domestication center in northern China westwards to Central Asia and beyond occurred during the 3rd and 2nd millennium BCE (Liu et al., 2018; Hermes et al., 2019; Dal Corso et al., 2022; Endo et al., 2023). Recently published stable isotope results, ceramic impressions, and genetic analyses on related materials are beginning to reveal evidence signifying the incorporation of these newly imported crops and livestock into the indigenous culinary and cultural traditions (Liu and Reid, 2020; Vaiglova et al., 2021; Li et al., 2022; Murakami et al., 2022; Ritchey et al., 2022; Tian et al., 2022; Endo et al., 2023; Sun et al., 2024a).
In recent years, there has been an increase in research in the western and central Tibetan Plateau that includes archaeobotanical recovery as one of the primary objectives of study. This resulted in new insight into human and plant interactions that manifested themselves in distinct subsistence strategies, cooking traditions, and ecological interactions. While maintaining these distinct traditions, communities in western and central Tibet were interconnected with broader Eurasia (e.g., Chen et al., 2023; Gao et al., 2021; Tang et al., 2021; d'Alpoim Guedes et al., 2014; Lu et al., 2021; Song et al., 2018, 2021). The Tibetan Plateau provides a unique window into understanding human ingenuity and persistence, as its high elevation presents particular challenges to human occupation, such as limited growing seasons, harsh weather systems, and reduced oxygen availability.
2 Plant cultivation on the Plateau
Indeed, humans utilized varying and innovative subsistence strategies to succeed in the high elevations of the Tibetan Plateau. Pastoralism and arable agriculture were first introduced to the eastern Tibetan Plateau between the 4th and 2nd millennium BCE. Domestic pig (Sus scrofa) and millet farming spread into the region from the Loess Plateau and were first concentrated along the northeastern margins of the Plateau (Aldenderfer, 2011; d'Alpoim Guedes et al., 2014; Chen et al., 2015; d'Alpoim Guedes and Aldenderfer, 2020; Ma et al., 2023). Archaeobotanical assemblages from before 1,500 BCE are dominated by foxtail and broomcorn millet, which predate the introduction of wheat (Triticum sp.) and barley (Hordeum sp.) into the region (Chen et al., 2015; d'Alpoim Guedes, 2015). A different strategy was used in southeastern Tibet. At the sites of Karou and Xiaoenda, for example, there has been evidence of mixed hunting and millet farming (Zhang et al., 2019; Song et al., 2021; Lu, 2023). Questions remain as to whether millet grains could be brought to high elevations from lowland farms via trading networks. Macrobotanical remains from the sites of Zongri and Karou support both sides of the argument (Ren et al., 2020; Song et al., 2021). However, human isotope values from Zongri show clear evidence of substantial millet consumption, likely occurring daily (Cui et al., 2006). While grain trade cannot be ruled out as an explanation, it is unlikely that such trade could sustain human food at a population level, raising questions about local cultivation. By the mid-second millennium BCE, the Southwest Asian domesticates such was wheat, barley, cattle and sheep/goats were introduced to various Tibetan regions (Chen et al., 2015; d'Alpoim Guedes, 2015). The hardiness of barley and ecological flexibilities of sheep/goats, yak (Bos grunniens) and yak-cattle hybrids likely contributed to the flourishing of agropastoral communities in regions higher than 2,500 meters above sea level (masl hereafter) (Chen et al., 2015, 2023; Zhang et al., 2022; Tang et al., 2023).
On the other side of the Plateau, communities in western Tibet were likely closely connected with cultural groups in the Kashmir region as well other regions on the Plateau (Spengler, 2015; Spate et al., 2017; Chen et al., 2024). As early as 2,600 BCE, Harrapan communities cultivated a diverse crop package including hulled 6-row barley (Horduem vulgare var. vulgare), wheat (Triticum aestivum and sphaerococcum), field-pea (Pisum arvense), and rice (Oryza sativa) (Pokharia and Saraswat, 2002; Pokharia et al., 2011). In northern Nepal (3,000–4,000 masl), between 1,000 BCE and 1,000 CE, there was an early 6-row barley and buckwheat (Fagopyrum esculentum/tatarieum) dominated agropastoral system, with later inclusions of bread wheat (Triticum aestivum), broomcorn millet, and pea (Pisum sativum) (Knörzer, 2000). Similar ceramic traditions of cord decorations found across the Himalayas may also support this interconnectivity (Chen et al., 2024). By 1,500 BCE in central Tibet, agropastoral communities at Changguogou (1,400–800 BCE, 2,750 masl) and Bangga (1,000–800 BCE, 3,750 masl) had hulled and naked (Hordeum vulgare var. nudum) barley, bread wheat, pea, and probably buckwheat (Fu, 2001; d'Alpoim Guedes et al., 2014; Liu et al., 2017; Tang et al., 2021). In southeast Tibet, Wang et al. (2021) show that the Nyingchi Region was connected to agropastoralist communities to the west, through the presence of bread wheat, 6-row barley, and peas dated to the 1st millennium BCE. In addition to the three western Tibetan sites presented in this study, the large site of Kaerdong (455–700 cal. CE, 4,300 masl) has evidence for a barley-dominated agropastoral system, with the addition of bread wheat and buckwheat (Song et al., 2018). This rapidly growing dataset suggests a possible southern introduction of domesticates into central and western Tibet (Laurent, 2015; Stevens et al., 2016; Liu et al., 2017; Lister et al., 2018; Gao et al., 2021). This paper begins to address the actual methods used by communities to successfully grow Southwest Asian crop domesticates on the Plateau by examining the labor choices involved in plant cultivation, as measured through stable isotope values of the barley grains themselves at three small agropastoral sites in the Tibetan highland.
3 Beyond the grain: labor strategies and culinary traditions
Crop management, such as irrigation and manuring, require high labor investment and integration with other tasks, especially in mixed agropastoral economies where mobility for at least some of the population is necessary (Lees and Bates, 1974). There is a potential opposition between the labor strategies necessary for plant cultivation and those of animal pastoralism, particularly in landscapes that lend themselves to herding activities, such as the Tibetan Plateau highlands. Pastoral systems use extensive labor strategies, where productivity is measured by the access to and quality of expansive pasture and the size of the herds grazed on them. Risks are mitigated through herd and grazing land management (Boserup, 1965; Khazanov, 1983; Barfield, 1999; Halstead, 2000; Kradin, 2015). In contrast, with arable farming, production is limited by the amount of suitable land available and its fertility (Boserup, 1965). In this context, intensification is measured by the amount of labor and resources invested per unit area of land through activities such as irrigation, plowing, and fertilizing to buffer against future food shortages (Boserup, 1965; Morrison, 1994; Halstead, 2006, p. 45). Extensification, within the farming context, uses larger plots of land, spreading labor, energy, and time across increasing area (Halstead, 1995, 2000). Macrobotanical remains and plant stable isotope values are useful proxies for measuring labor strategies and provide an exceptional opportunity to examine the under investigated character of plant cultivation in Western Tibet.
The trans-regional movements brought exotic food items to not only novel environments but also new cultural settings with their own food preparation techniques. Research across Eurasia identifies distinct cuisines and associated cooking traditions that were formulated before domestication and within which domestication occurred (Sakamoto, 1996; Fuller and Rowlands, 2009, 2011). As the cereal crops moved across Eurasia and into new cuisines, selective pressures modified traits apparent in the grains. For example, during the eastward movement of bread wheat and barley into ancient China, there was a dramatic decrease in grain size as the crops entered a boiling-and-steaming tradition that favored smaller grains (Liu et al., 2016; Ritchey et al., 2022). The Asian millets, on the other hand, grew in seed size as they move westward into new banking and grinding culinary traditions (Sun et al., 2024b). Ritchey et al. (2022) argue that the 6-row barley in Tibet, when compared to the other regions in Central and East Asia, are notably larger and attribute this to a historical tradition of a boiling-free roasting zone in high-elevation Tibet. Boiling whole grains is inefficient in time, labor, and fuel at such high altitudes where low vapor pressure reduces the boiling point of water to 86°C at 4,600 masl. A similar trend is seen in foxtail millet on the Plateau (Sun et al., 2024b).
An alternative hypothesis to increased grain size could be increasing investment in growing conditions to produce a higher yield with larger grains. Anthropogenically enriched growing conditions can enlarge grain sizes (Savin and Nicolas, 1996; Altenbach et al., 2003; Dupont and Altenbach, 2003; van Bommel et al., 2021; Larsson and Bergman, 2023). Poor ecological conditions, on the other hand, have been hypothesized to limit plant development and grain size (Fuller et al., 2017; Motuzaite-Matuzeviciute et al., 2018; Motuzaite Matuzeviciute et al., 2021). Experimental work shows variable but generally positive correlations between increased water availability and soil nutrition and grain size in various cereals (van Bommel et al., 2021; Larsson and Bergman, 2023). Within the context of this growing research, we consider this hypothesis through the analysis of grain size and stable isotope values from three Tibetan Sites in the larger Tibetan dataset from Ritchey et al. (2022).
4 Archaeological plant stable isotope principles
Plant stable isotope values are currently the most direct method for investigating past growing conditions of plants. In semi-arid/arid environments such as in our study area (< 450 mm/annual rainfall), non-native water-demanding cereals are expected to experience water stress to a certain degree (Li et al., 2022). Carbon isotope values (δ13C) of plant remains, particularly C3 plants like barley, reflect the water availability during plant growth and the grain-filling period (Farquhar et al., 1989; Araus et al., 1997, 1999; Wallace et al., 2013). δ15N values allow for the assessment of soil 15N enrichment processes during plant growth caused by natural and cultural factors including aridity, soil denitrification, and fertilization through middening and manuring (Bogaard et al., 2007; Fraser et al., 2011; Styring et al., 2018). Together, these measurements allow for a direct assessment of past crop agricultural activities of watering and soil maintenance, which have been used to investigate early crop management strategies in the Middle East, Europe, East Asia (Bogaard et al., 2013; Vaiglova et al., 2014a; Styring et al., 2016b, 2018; Li et al., 2022), and recently in the eastern Tianshan Mountains of Inner Asia (Tian et al., 2022). In this study, we use this method to analyze 55 total barley grains from the sites of Dingdong, Piyang, and Jiweng, with each sample representing a single grain (Table 1).
Water availability during photosynthesis directly influences a C3 plant's ability to assimilate CO2, which exists in lighter 12CO2 and heavier 13CO2 forms. When plants are well watered, there is free passage of CO2 through the open stomata on the leaves and the lighter 12CO2 is preferentially assimilated while the heavier 13CO2 is discriminated against (O'Leary, 1988; Girolamo et al., 2014). When growing in water-limited soils, plants periodically close the stomata to preserve plant moisture, causing CO2 to be recycled, resulting in higher assimilation of the heavier 13CO2 (O'Leary, 1988; Girolamo et al., 2014). A more negative δ13C value of an archaeological grain thus reflects higher water availability during the grain-filling growth period compared to a crop that grew in drier soils (Farquhar et al., 1989; Araus et al., 1999; Wallace et al., 2013). Stable carbon isotope values are typically reported as δ13C. However, to compare the values across distinct chronological periods (when the carbon isotope composition of atmospheric CO2 differed) a conversion to Δ13C is needed. This value captures the degree to which a plant discriminated against 13C irrespective of the atmospheric composition (Farquhar et al., 1989). We use Δ13Cplant−air values as the calculated 13C discrimination independent of source CO2 computed with the equation developed by Farquhar et al. (1989):
After the conversion of results into Δ13C, the directionality of the water availability changes, with higher Δ13C values corresponding to wetter soils. Elevated Δ13C values may reflect the use of watering practices such as man-made irrigation, terracing, or strategic planting in wetter soils (Araus et al., 1999; Ferrio et al., 2005; Wallace et al., 2013).
The productivity of the cereal crop can also be improved through the management of soil nutrition. In particular, anthropogenic fertilization of soils, accomplished in ancient times primarily through dung manuring but also through the application of guano and marine biofertilizers, can be detected using δ15N values (Szpak et al., 2012; Gröcke et al., 2021). Plant nitrogen isotope values (δ15N) reflect the isotopic composition of the nitrogen (N) source. For N non-fixers like the barley analyzed in this study, nitrogen is primarily absorbed from the soil. Any enhancements made to the soil, whether of natural or anthropogenic causes, are reflected in higher δ15N values of the plant remains (Ambrose, 1991; Bogaard et al., 2007; Fraser et al., 2011; Girolamo et al., 2014; Szpak, 2014). Natural causes for heightened δ15N values include aridity, salinity, and denitrification (Ambrose, 1991; Hartman and Danin, 2010; Szpak et al., 2013). Anaerobic soil conditions and high temperatures can cause soil denitrification which leads to soil bacteria consuming the available oxygen from nitrates, resulting in an increased δ15N value of the soil (Farrell et al., 1996; Hartman and Danin, 2010). Temporary waterlogging in floodplains or wadi slopes can result in elevated δ15N values of plants grown there (Hartman and Danin, 2010). High-elevation environments, such as the Tibetan Plateau, have unique impacts on δ15N values of both the soil and vegetation (see below). Anthropogenic activities to improve fields elevate plant δ15N values above the natural baseline, with values above 6 ‰ in European and southwest Asian contexts reflecting intensive manure application (Bogaard et al., 2007, 2013; Fraser et al., 2011; Szpak, 2014).
Regionally specific nitrogen isotope baselines can be estimated using archaeological remains of animals that subsist on natural vegetation (such as wild herbivores) (Vaiglova et al., 2022). Based on an estimated trophic offset between diet and consumer tissue, herbivore tissues are approximately 3‰−5‰ higher compared to the values of their diet (Deniro and Epstein, 1981; Minagawa and Wada, 1984). Thus, measured δ15N values from bone collagen of archaeological herbivores in the region can provide a local baseline against which the archaeological plant δ15N values can be compared. There are limitations to using local archaeological herbivores to reconstruct baselines, as the δ15N values can vary between individuals, species, and habitat (e.g., hot/dry vs. cool/wet habitats) but similar limitations exist when using modern plant isotope values (environments change over time and are a product of ancient human and natural processes that can influence soil compositions) (Deniro and Epstein, 1981; Ambrose, 2000; Vaiglova et al., 2022). Unpublished data on archaeological Tibetan deer provide a mean δ15N of 4.3 ‰ (n = 12) (Tang, 2024). When calculated with the known offset between trophic levels, this provides a baseline plant δ15N threshold of 0‰−1.3‰. Reported δ15N values of modern wild vegetation record a mean of 2.4‰ (Yang et al., 2013). We expect the natural non-manured soil δ15N values to be approximately between 0‰ and 2.4‰.
4.1 Environmental factors for stable isotopes values on the Tibetan Plateau
The extreme ecology and elevation of the Tibetan highlands engenders additional factors to consider before interpreting anthropogenic influences on δ13C and δ15N values, chiefly atmospheric pressure and aridity. Wallace et al. (2013) provide an interpretive framework for interpreting Δ13C watering thresholds. This incorporates the known difference in carbon assimilation in barley grown under the same watering regimes. Studies show that atmospheric pressure at high elevations reduces carbon discrimination, therefore increasing δ13C (and subsequently, calculated Δ13C values) in the plants (Zhou et al., 2011; Szpak et al., 2013). Szpak et al. (2013) find a positive linear correlation with elevation and δ13C values in Peruvian C3 plants ranging from lowland (approximately 10 masl) to highland (>4,000 masl) areas (Spearman's r = 0.879, p = 0.001). There is a positive offset of approximately 2‰−5 ‰ between values from the lowest elevation plants and those at the highest. These findings correlate with those on the Tibetan Plateau, where there is a mean positive increase of 1‰−2‰ across a variety of C3 plants between 2,500–5500 masl (Zhou et al., 2011). Thus, to accommodate for the atmospheric pressure influence on δ13C values on the plant remains from the Tibetan Plateau (>4,000 masl), we adjust the calculated δ13C ratios for watering bands by a conservative average of +2‰ difference (Table 2). We also use modified water categories that follow Li et al. (2022)'s divisions which provide a numeric boundary for Wallace et al. (2013)'s original watering threshold gradient. This provides a more accurate baseline for interpreting water stress in the Tibetan barley (Hordeum vulgare). Considering these factors, the Optimal Watering Threshold (OWT) for Δ13C is 15.0‰, reflecting the lowest threshold for non-growth restricting watering. Grains that receive more water than necessary are categorized above the Superfluous Watering Threshold (SWT) of Δ13C 16.5‰.
Environmental factors, such as soil aridity, precipitation, and soil denitrification, can influence the δ15N values of the plants. Experiments in Peru show that with increasing elevation, δ15N of modern foliage reduces by 2‰−6‰ (Szpak et al., 2013). This is compounded with a nearly identical negative relationship of δ15N values with rainfall, where increased rainfall causes lower δ15N values. This relationship is generally understood as driven by soil aridity and annual rainfall rather than solely by altitude. High soil aridity (as opposed to plant water uptake) from low annual precipitation can potentially increase δ15N values by 2‰−6‰ due to the openness of the nitrogen cycle in arid climates (Szpak et al., 2013). In the Peruvian context, the high elevations have increased rainfall, causing the lower δ15N values when compared to the arid, coastal deserts that have higher δ15N values (4‰−8‰). On the Tibetan Plateau, Yang et al. (2013) find that δ15N of modern vegetation increases with decreasing mean annual precipitation. Located within the Plateau temperate monsoon climate zone, the three sites in this study have a mean annual precipitation of < 450 mm/year (Tang et al., 2022). Based upon the model proposed by Szpak et al. (2013), this high soil aridity would positively impact δ15N values and thus we interpret the results presented in this study accordingly. Yang et al. (2013) and Szpak et al. (2013) both find that mean annual temperature does not seem to influence δ15N values at high elevations.
Additionally, the Tibetan Plateau has a high natural abundance of 15N in soil and vegetation (alpine grasses) when compared to the mean global measurements of areas with similar climatic conditions (mean annual precipitation and temperature) (Yang et al., 2013). Yang et al. (2013) measured the mean δ15N of vegetation at 2.4‰, and the mean δ15N of soil at 4.1‰, both higher than the mean global measurements of similar climates. They attribute increased ammonia in soil from grazing animals and increased foliar N concentrations as potential mechanisms for this natural abundance. These experiments, while on modern samples, provide data to aid in the interpretations of archaeobotanical assemblages on the Tibetan Plateau. The future addition of archaeological δ15N values of low-level herbivores and wild foliage will help clarify these interpretations and provide archaeological baselines for natural δ15N.
5 Materials and methods
5.1 Excavations and archaeobotanical sampling
Dingdong (4,212 masl), Piyang (4,174 masl), and Jiweng (4,094 masl) are a group of sites located within the broader Piyang Dongga complex (Figure 1). The complex consists of a number of settlements, cemeteries, and Buddhist monuments that are considered the first examples of permanent occupation in the western Plateau (Fu, 2008; Lu, 2008; Tang et al., 2022). The sites are in Zhada County, Ngari prefecture, along a tributary of the Langqên Zangbo (Upper Sutlej River). Faunal remains of sheep/goats, yaks/cattle, and horses indicate long-term pastoral activities in the site area (Lu, 2008). Excavations in 2019 uncovered new evidence of occupation and agropastoral activities at the sites, coinciding with an agriculture system focused on 6-row barley cultivation (Tang et al., 2022). Evidence of likely local cultivation is evident through the abundant remains of grains, rachises, and culm nodes, indicating crop processing (Table 1). For isotopic sampling, we aimed to choose barley from contexts that had sufficient barley remains present (>10 grains).
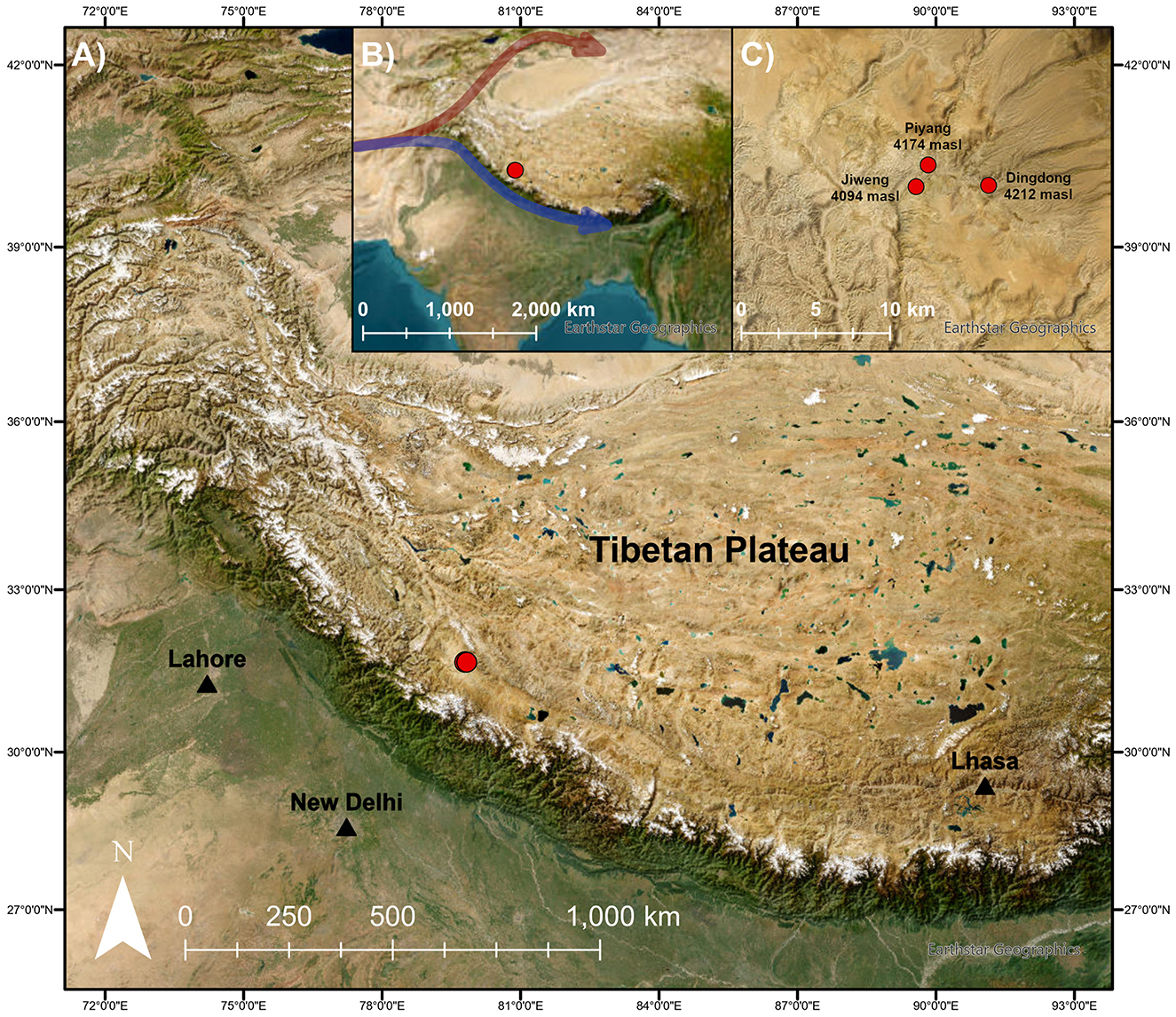
Figure 1. (A) Map of study area with (B) inset including hypothesized prehistoric food globalization routes: northern trajectory of wheat and barley (red), southern trajectory of barley (blue), and (C) an enlarged view of site locations. Map created using ArcGIS® software by Esri (2009). ArcGIS® and ArcMap™ are the intellectual property of Esri and are used herein under license. Copyright Esri. All rights reserved. For more information about Esri® software, please visit www.esri.com.
Dingdong is a collection of 11 stone structures and three semi-subterranean houses that represent a year-round agropastoral village (~150 m2), occupied between 348 cal BC to AD 541. Two flotation samples from fireplaces within these structures were analyzed for macrobotanical remains in 2022 (Tang et al., 2022). These samples included 413 naked (Hordeum vulgare var. nudum) (41.3 grains/L) 62 hulled (Hordeum vulgare var. vulgare) (6.2 grains/L), and 330 indeterminate naked/hulled barley (33 grains/L). These were accompanied by 36 barley rachises (3.6 rachises/L). For this isotopic analysis, we sampled 19 naked barley and 8 hulled grains from one of these fireplace contexts (Table 1).
Jiweng is a large stone enclosure (~960 m2), occupied between 391–208 cal BC. Three trenches from a small test excavation recovered only one artifact, an almost complete red-sand pot, and abundant macrobotanical remains. The layout and lack of artifacts recovered is similar to historical seasonal campsites in the region and suggest a seasonal agropastoral campsite. From three test trenches at Jiweng, 92 naked barley (2.59 grains/L), 3 hulled barley (0.8 grains/L), 84 indeterminate naked/hulled barley (2.37 grains/L), and 61 barley rachises (1.72 rachises/L) were recovered. In this study, we sampled 19 naked barley grains from a fireplace context located in Trench 4 (Table 1).
During construction in the village of Piyang, a soil section was exposed containing abundant ceramics, animal remains, and charcoal. Three subsections with dense deposits were sampled for floatation, totaling 6 samples. Due to the rescue nature of this sampling, it is unclear the site type of the archaeological deposits at Piyang, dated to 396–212 cal. BC. From these samples, 25 naked barley (4 grains/L), 1 hulled (0.16 grains/L), 10 indeterminate naked/hulled barley (1.6 grains/L), and 85 barley rachises (13.6 rachises/L) were recovered. The low number of overall grains recovered limited our sampling for isotopic analyses. As such, we sampled 9 naked barley grains from layer 2, which had the most grains (n = 20) recovered (Table 1).
Naked and hulled barley and indeterminate cereal grains (cerealia) were the only domesticated grains recovered from all three sites. The wild taxa were identified to the family level, with a few to the genus level, which limits our ability to determine if they are representative of arable weed taxa. The families identified include Amaranthaceae, Brassicaceae, Cyperaceae, Poaceae, Polygonaceae, Rosaceae, and Solanaceae. At the genus level, Chenopodium sp, Capsella type, and Bromus sp., may represent common arable weeds such as Chenopodium album, Capsella bursa-pastoris, and Bromus spp., if identification were possible to the species level. Some of the wild taxa, e.g., Chenopodium sp., Fabaceae, and Pontentilla/Fragaria spp., depending on the species, could have been collected as a source of wild food. Some of these taxa, particularly Chenopodium sp., are also consumed by livestock and could have entered the macrobotanical record through dung burning for fuel (Spengler, 2018). See Tang et al. (2022) for more detailed descriptions of the archaeological excavations and analysis of the macrobotanical assemblages from all three sites.
5.2 Grain preservation and charring
We analyzed barley grains that were charred through exposure to high temperatures and potentially directly to fire that caused the whole grain to undergo a chemical process that removes water vapor and volatile organic compounds from the grain matrix. This leaves a black carbon material, char, preserving the archaeological grain. In general, this charred grain maintains the carbon and nitrogen isotopic values from prior to charring, albeit with known charring offsets, varying with the temperature and duration of charring (Nitsch et al., 2015; Stroud et al., 2023b). The known charring offsets (averaged across temperatures and duration: δ13C by + 0.11‰ and δ15N by + 0.33‰ (Nitsch et al., 2015), are lower than the conservative 1‰ estimates used in most paleodietary studies (following Fraser et al., 2013) and consequently have little impact on our data interpretation. It is nonetheless important to consider the potential fractionation occurring during carbonization, particularly when reconstructing past agricultural practices using modern-day isotopic values or comparing the isotopic values from charred archaeological material to that of uncharred material.
The charring temperatures need to be considered, as they impact isotopic values at various rates depending on charring temperature and duration (Fraser et al., 2013; Nitsch et al., 2015; Stroud et al., 2023a). Well-preserved grains are those that have been charred within optimal charring conditions (Styring et al., 2013; Charles et al., 2015; Vaiglova et al., 2022; Stroud et al., 2023a). Following these guidelines, particularly those provided by Stroud et al. (2023a), we visually examined grain surface and cross sections, photographed, and categorized each grain as “poor,” “borderline,” and “good” preservation. Photo examples are provided in Supplementary Figure 1 and categorizations in Supplementary Table 1. We ran all grains for isotopic analysis, regardless of qualitative charring status. We then compared this qualification to the isotopic results to see if the charring status correlates with isotopic value. Visual inspection of grain charring and isotopic results by site shows no apparent clustering or pattern (Figure 2). We estimated 95% confidence intervals (CIs, reported as [lower level, upper level]) around the means of charring status group measurements to further interrogate a potential relationship between charring status and isotopic result (Supplementary Table 2, Supplementary Figure 2). The mean Δ13C values for each charring status are very similar, with nearly identical CIs: good 15.9 ‰ [15.3, 16.5], n = 10, borderline 16.0‰ [15.5, 16.5], n = 14, and poor 15.9‰ [15.6, 16.3], n = 31. The mean δ15N values for poor and borderline are similar: 14.5‰ [13.7, 15.3] and 15.10‰ [13.9, 16.3], respectively. The mean of the good category is higher at 17.1‰ [15.7, 18.5], with the lower CI overlapping with the upper CI of borderline. There is a difference between good and poor mean δ15N values, with a mean difference of −2.6‰ [−4.2, −1.0]. This suggests, given the small sample size of the data collected, the difference between the mean Δ13C values of good and poor grains is plausibly anywhere between −4.2‰ and −1.0‰. There is considerable overlap between CIs of good and poor grains, suggesting that the differences in means may not be notable. There is a broad spread in the poor grains (over a 10‰ difference between the lowest and highest values) compared to the good grains (~5‰). This could, in part, be influenced by the much higher sample size for poor grains (n = 31) than good grains (n = 10). If we were able to increase the good grain sample size, it is possible we would see a similar spread in the δ15N values. Based on the collected data, we conclude that there are no plausible differences between the Δ13C means of the different charring categories and that all isotopic measurements, regardless of charring status, are appropriate for our analyses. While there is a difference between the δ15N means of good and poor grains that should be considered, due to the limited materials available for additional analyses, we have included all grains within our study.
5.3 Grain pretreatment and isotopic analysis
We selected well-preserved barley grains (determined from surface texture and wholeness of the grain) for isotopic analysis. After cross-sectioning and photographing the grains for recording the charring status of the internal matrix, as previously discussed, we prepared the grains for individual grain isotopic analyses following Vaiglova et al. (2014b)'s pretreatment procedure using a gentle acid wash to remove any exogenous soil carbonates. Each grain was cleaned of any visible surface contaminants, crushed to a fine powder, and weighed. We then soaked each sample with 0.5 M HCl acid and heated them at 80°C for 30 min. After 3 washes with deionized water, samples were dried in the vacuum freezer for 8 h. 800 μg of the dried samples were placed into tin capsules. We conducted the carbon and nitrogen stable isotope analyses using an Elemental Analyzer coupled to a Thermo Delta V Plus Continuous Flow Isotope Ratio Mass Spectrometer (EA–IRMS) housed at the Department of Earth, Environmental, and Planetary Sciences at Washington University in St. Louis, USA. We normalized the raw δ13C and δ15N values relative to VPDB and AIR, respectively, with the international standards of USGS 40 δ13C = −26.4 ‰, δ15N = −4.5‰) and USGS41a (δ13C = +36.6‰, δ15N = −4.5‰). Two in-house standards were used for linearity and check standard: acetanilide (δ13C = −29.5‰, δ15N = +47.6‰) and BR millet (Bob's Red Mill millet flour; δ13C = −13.2‰, δ15N = +3.3‰). No replicates were conducted on the archaeological samples, due to the limited mass per grain sample after pre-treatment processing. Precision [u(Rw)] was determined to be ± 0.07‰ for δ13C and ± 0.08‰ for δ15N. Accuracy or systematic error [u(bias)] was determined to be ± 0.14‰ for δ13C and ± 0.20‰ for δ15N based on calibration standard and check standard measurements. Using the equations from Szpak et al. (2017), the total analytical uncertainty was determined to be ± 0.16‰ for δ13C and ± 0.22‰ for δ15N. One BR millet check standard produced unexpectedly different values (−21.75‰ compared to the expected −13.18‰ for δ13C and 15.04‰ compared to the expected 3.28‰) and was removed from uncertainty calculations. This anomalous check standard may be due to the possible slight heterogeneity of the sample or instrument drift (Szpak et al., 2017). If included in the calculations, the total analytical uncertainty is ± 2.1‰ for δ13C and ± 2.8‰ for δ15N.
We used R (version 4.3.1) to create graphs. R packages included ggplot2, esci, and EnvStats, with additional packages for aesthetics (Millard, 2013; Wickham, 2016; R Core Team, 2023; Calin-Jagemen, 2024). We used Jamovi (Version 2.4) to calculate confidence interval statistics and plots (The Jamovi Project, 2023).
6 Results
Results of Δ13C and δ15N analyses are reported in Table 3 and displayed as boxplots and CIs in Figure 3. We use a 95% confidence interval for all analyses. The Δ13C values of grains from Jiweng record the highest mean (16.7‰, [16.1, 16.9], n = 19), followed by Piyang (16.2‰, [15.7, 16.6], n = 9), and Dingdong (15.8‰, [15.5, 16.1], n = 27). With the adjusted watering bands for a high-elevation atmospheric effect, all three sites have grains that measure above the optimal watering threshold. Four grains from Dingdong measure below the OWT and four from Jiweng measure above the SWT. Overall, the most observable difference in Δ13C values between sites lies in the higher Δ13C values from Jiweng. The mean difference between Jiweng and the combined values of Dingdong and Piyang is −0.6‰, [−1.1, 0.0] (Supplementary Table 3, Supplementary Figure 3). This suggests that, given the small sample size of the data collected, the mean difference between the Δ13C values at Jiweng and the other two sites is plausibly anywhere between −1.1‰ and 0.0‰. There is some overlap between the CIs of the values from Jiweng and the Dingdong/Piyang data. This suggests that there is possible difference between the mean values of the two sets of groups. The difference is more notable between Jiweng and Dingdong than any other pairing, with a mean difference of 0.9‰, [0.3, 1.5]. This suggests, given the small sample size of the data collected, the difference between the mean Δ13C values at Jiweng and Dingdong is plausibly anywhere between 0.3‰ and 1.5‰. There is no overlap between the CIs of the values from Jiweng and Dingdong, suggesting that there is a notable difference between the higher mean values of Jiweng from that of Dingdong.
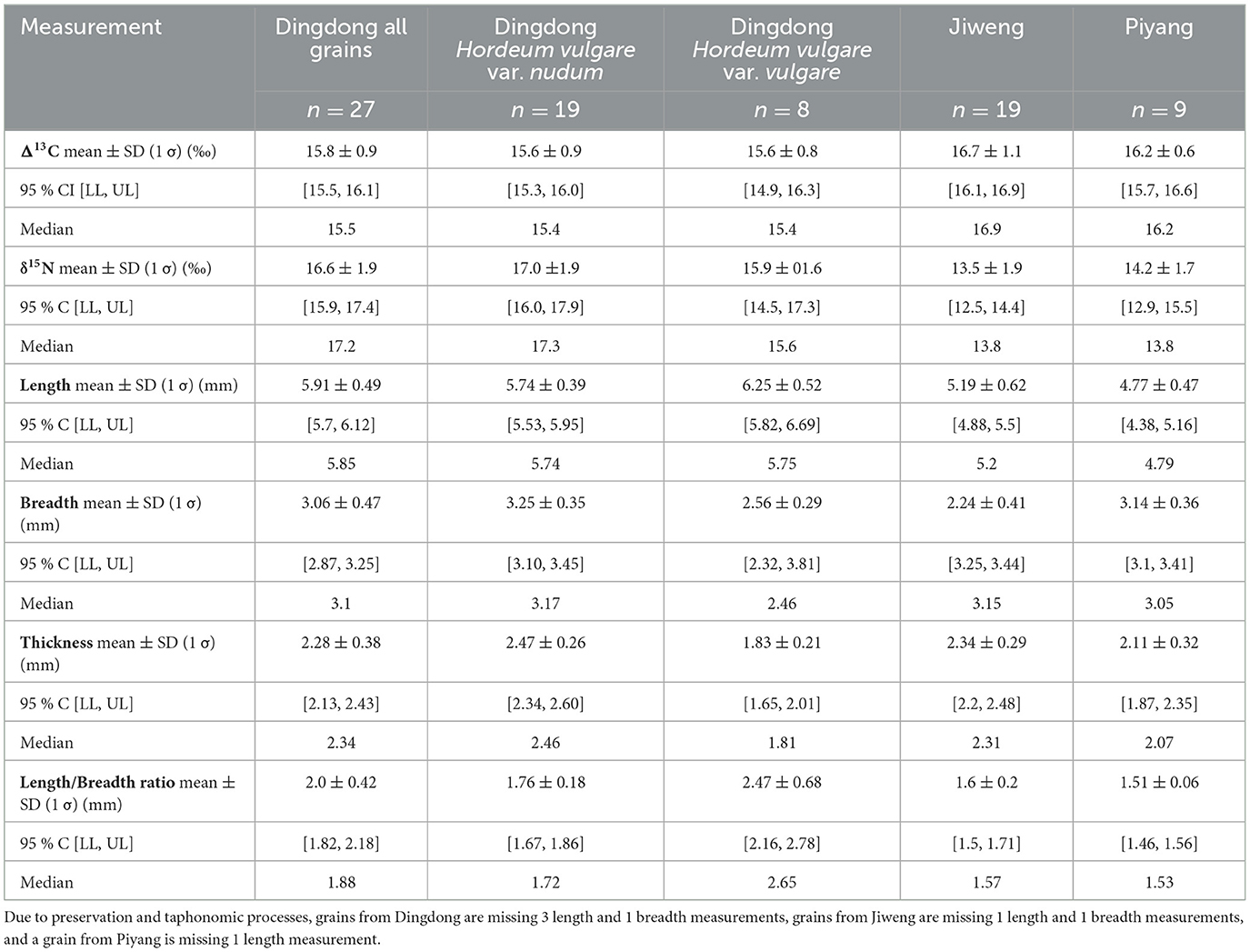
Table 3. A summary of Δ13C and δ15N values, mean, standard deviation (SD), 95% confidence intervals (CIs), median, and morphology metrics of barley grains (Hordeum vulgare) from Dingdong, Jiweng, and Piyang.
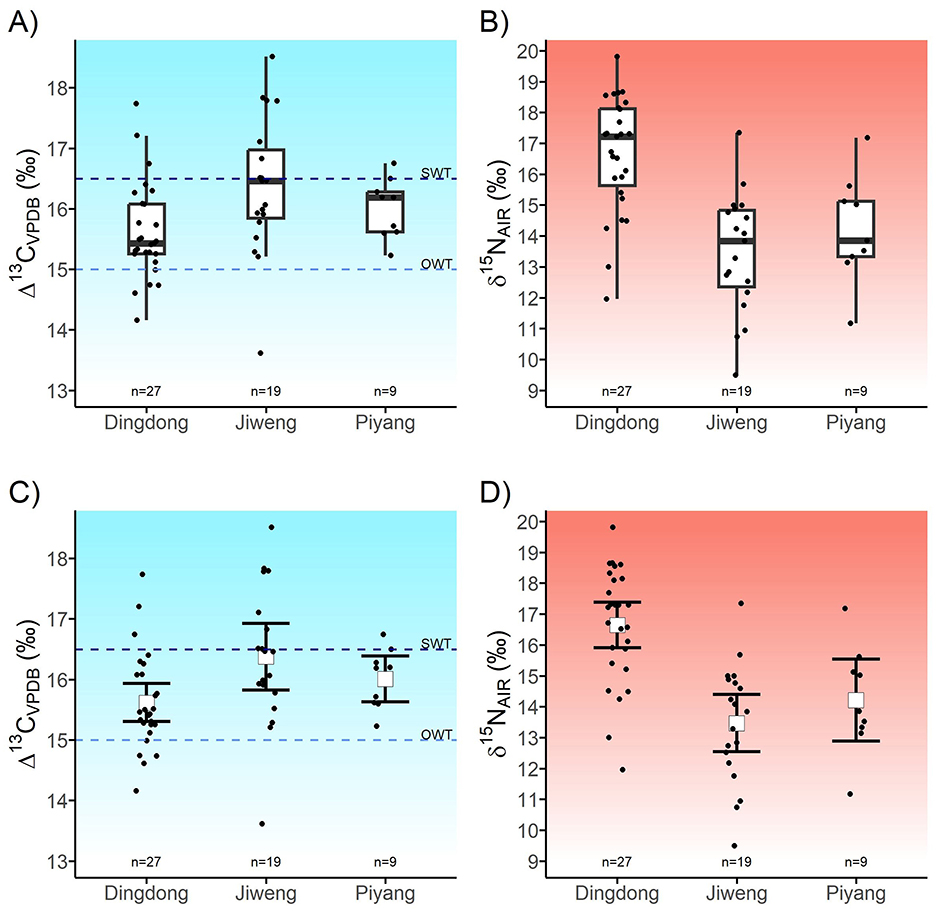
Figure 3. (A) Boxplots of Δ13C values and (B) δ15N values of barley grains from Piyang, Dingdong, and Jiweng. (C) Independent groups contrast figure comparing the 95 % confidence intervals (CIs) of Δ13C values and (D) δ15N values of barley grains from Dingdong, Jiweng, and Piyang; error bars represent CIs and squares represent group means. Horizontal lines in (A) and (C) represent high-elevation-adjusted (+2‰ Δ13C) optimal watering thresholds (OWT) and superfluous watering thresholds (SWT). Color gradients reflect increasing water input and soil nitrogen levels.
Dingdong grains have the highest mean δ15N of the group (16.6‰, [15.9, 17.4], n = 27), followed by Piyang (14.2‰, [12.9, 15.5], n = 9), and Jiweng (13.5‰, [12.5, 14.4], n = 19). All are well above the calculated natural baseline of 0‰−2.4‰ from archaeological herbivores and wild seed remains (Yang et al., 2013; Tang, 2024). When compared to experiments conducted in modern farm settings in England and Germany, where heavily manured (+35 tons of manure/ha) barley and wheat had a mean value of 8‰ δ15N, all barley grains from this study fall well above this highly-manured threshold (Bogaard et al., 2013). Overall, the most observable difference in δ15N values between sites is the increased mean of Dingdong. The mean difference between Dingdong and the combined values of Jiweng and Piyang is −2.8‰, [−3.9, −1.8] (Supplementary Table 4, Supplementary Figure 4). This suggests that, given the small sample size of the data collected, the mean difference between the δ15N values at Dingdong and the other two sites is plausibly anywhere between −3.9‰ and −1.8‰. As the lower limit of the CI for Dingdong is 15.9‰, and the upper limit of the CI for the combined Jiweng/Piyang data is 13.1‰, this difference is notable due to the lack of overlap between the groups. Further, when compared to a similar archaeological plant stable isotope study in Xinjiang, China, the samples from this study, while variable, fall above Li et al. (2022)'s calculated threshold for high nutrient-rich soil condition of 8.5‰ δ15N for a region (Zhuanglang county) that receives a mean annual precipitation of 550 mm.
At Dingdong, we analyzed both hulled and naked grains. CIs show no notable difference in the Δ13C values between naked (15.6‰, [15.3, 16.1], n = 19) and hulled grains (15.9‰, [14.5, 17.2], n = 8) with a mean difference of 0.1‰, [−0.6, 0.8] (Supplementary Table 5, Supplementary Figure 5). Mean δ15N values of naked barley (17.0‰, [16.1, 17.8), n = 19) are higher than those of hulled barley (15.9‰, [14.5, 17.2], n = 8), with a mean difference of 1.1‰, [−0.5, 2.7] (Supplementary Table 5, Supplementary Figure 5). Given the limited sample size, the difference between δ15N values of naked and hulled barley at Dingdong is plausibly anywhere between −0.5‰ and 2.7‰. However, there is considerable overlap in CIs between the two groups, suggesting there is not a meaningful difference for either Δ13C or δ15N.
Barley grain sizes at Piyang, Jiweng, and Dingdong are on average very large, in length and breadth, when compared to other archaeological grains in central and eastern Eurasia (see Ritchey et al., 2022 for a robust regional analysis). However, the individual grain metrics vary and span the spectrum of domesticated barley grain size, from the shortest at 4.03 mm to the longest at 7.01 mm (Supplementary Table 1). Figure 4 presents scatter plots of Δ13C and δ15N values vs. grain length, breadth, and thickness. We assessed linear correlations of length, breadth, thickness, and length/breadth ratio vs. stable isotope value using Pearson's r (Supplementary Table 6). However, due to the small sample size, correlation cannot be estimated with much precision. Overall, the results of the Pearson's r suggest poor correlation between grain metrics and isotope value. Two correlations of Piyang grains have Pearson's r that suggest moderate correlation:δ15N vs. thickness, r(7) = 0.55, n = 9 [−0.21, 0.88] and δ15N vs. breadth, r(7) = 0.46, n = 9 [−0.32, 0.85]. However, the wide confidence intervals suggest that the estimates of the true correlation have low precision and could be anywhere from −0.21 (suggesting poor negative correlation) to 0.88 (suggesting strong positive correlation) for δ15N vs. thickness and anywhere from −0.32 (suggesting poor negative correlation) to 0.85 (suggesting strong positive correlation) for δ15N vs. breadth. At Dingdong, both hulled and naked barley were analyzed. Hulled grains at Dingdong are generally narrower in breadth and shorter in thickness than the naked barley (Table 3). When analyzed separately, there are two correlations that suggest moderate positive correlations: δ15N vs. breadth, r(6) = 0.42, n = 8 [−0.43, 0.86] and Δ13C vs. breadth, r(6) = 0.47, n = 8 [−0.38, 0.87] (Supplementary Table 6). Additionally, one correlation suggests a moderate negative correlation: Δ13C vs. L/B ratio, r(6) = −0.51, n = 8 [−0.89, 0.33] (Supplementary Table 6). The large confidence intervals indicate that the true correlations have low precision for each of these analyses. All other correlations fall below a Pearson's r of 0.4.
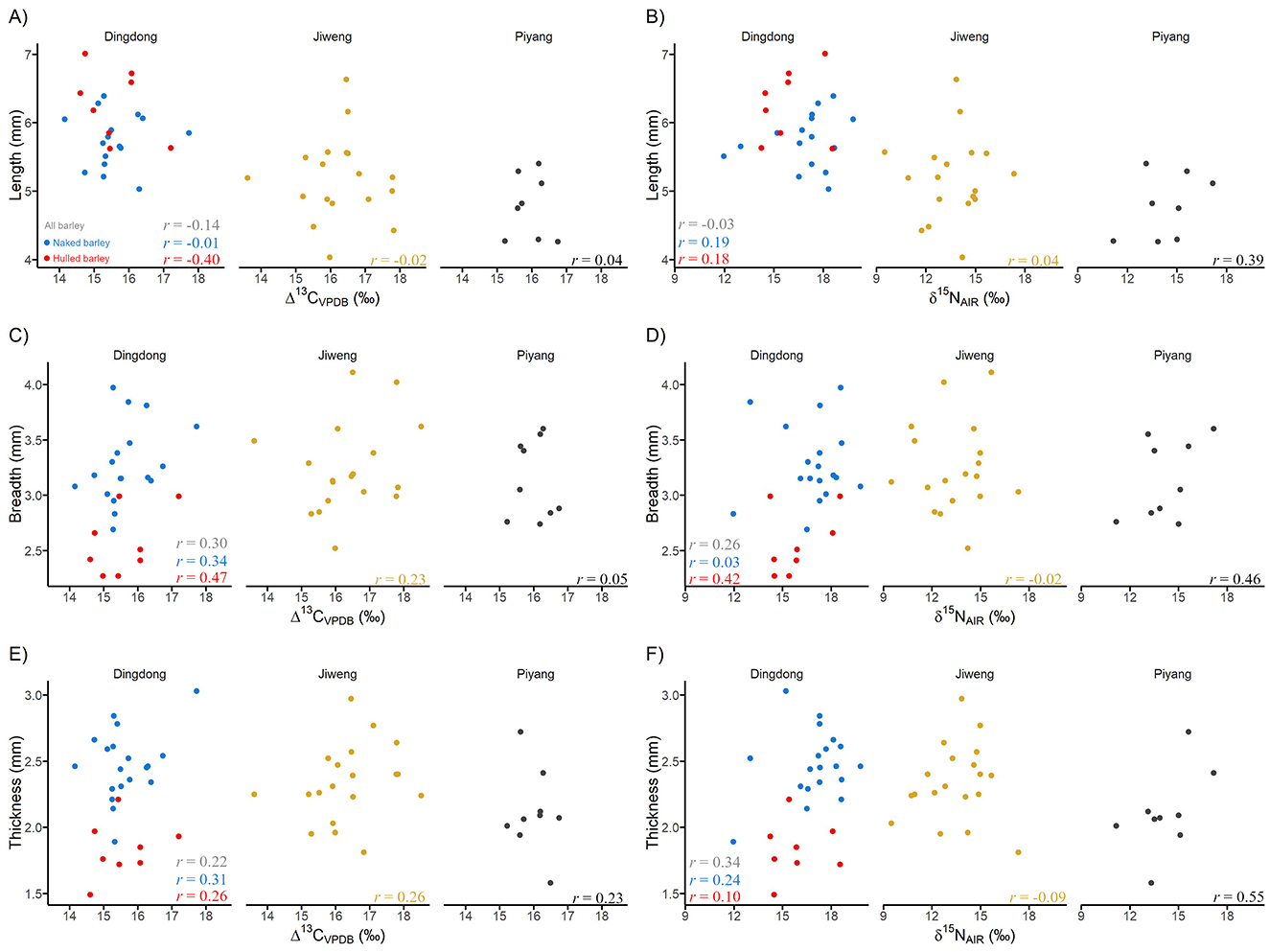
Figure 4. Scatterplots of grain measurements (in mm) vs. stable isotope value for each study site, with the calculated Pearson's r: (A) Δ13C vs. length; (B) δ15N vs. length; (C) Δ13C vs. breadth; (D) δ15N vs. breadth; (E) Δ13C vs. thickness; (F) δ15N vs. thickness. For Dingdong, red circles represent hulled barley (Hordeum vulgare var. vulgare) and blue circles represent naked barley (Hordeum vulgare var. nudum) grains; the gray Pearson's r is for all Dingdong grains combined.
7 Discussion
Stable isotope analyses of ancient crop remains allow for the assessment of their growing conditions (e.g., Ferrio et al., 2005; Fraser et al., 2011; Styring et al., 2015). In certain anthropogenic contexts, elevated carbon and nitrogen isotope values (Δ13C and δ15N) could indicate dedicated labor input to both watering (through irrigation activities) and manuring, which in normal circumstances, correspond to agricultural intensification rather than extensification (Bogaard et al., 2013). In an agropastoral setting, relatively lower labor input in watering and manuring could signify a de-emphasis on cultivation activities. Within communities focused more on herding practices for food production, crops may be grown opportunistically and/or extensively, as seen archaeologically in Tian et al. (2022). This study measured and calculated the Δ13C and δ15N of barley grains from the western Tibetan Plateau sites of Jiweng, Dingdong, and Piyang. The results indicate a managed crop cultivation system, where ancient herders invested time, energy, and labor into cultivating barley in the high-elevation western Tibetan Plateau. Furthermore, in considering the role that culinary traditions play in barley grain metrics (Ritchey et al., 2022), we presented a comparison between grain metrics and grain isotope values (Figure 4). No obvious relationship has been observed. Due to the small sample size, we are unable to make meaningful interpretations from the data. The relatively recent inclusion of systematic macrobotanical sampling on the Tibetan Plateau inhibits a larger analysis at this time. Future work will no doubt expand our understanding of early agricultural labor strategies when isotope analysis with a larger assemblage of macrofossils becomes available, which will increase the precision of mean Δ13C and δ15N estimates of ancient crop remains in the study area.
7.1 Ancient herders watered their barley crops
Δ13C values of barley grains from the three investigated sites indicate that the crop was grown in fairly well-watered soils. A few grains are above the optimal watering threshold while the remaining grains are above the superfluous watering threshold. It should be noted that barley is less water-demanding than other Southwest Asian domesticates, such as wheat, and can grow in more arid environments (Miller, 2003). It is, therefore, particularly interesting to observe elevated Δ13C ratios in the barley from all three sites when, ostensibly, they could have successfully grown without additional watering. Modern annual rainfall in the region is between 300–450 mm (Tang et al., 2022). Paleoclimate reconstructions of temperature and precipitation during the late Holocene, after 4,400–4,500 BP, suggest aridification in the region that stabilized into a climate that is similar to today (Gasse et al., 1991, 1996; Hou et al., 2012). There was a significant cooling period ca. 2,100 BP, with annual rainfall between 300–350 mm (Hou et al., 2012). Given such a semi-arid environment, our results support a cultivation system that utilized anthropogenic water sources. This could include irrigation of fields, as commonly applied today across the Plateau, or strategic planting of barley crops in naturally wetter areas. The lower Δ13C ratios at Dingdong, which was occupied a millennium later during the 5th century AD, might indicate a change in barley cultivation strategies with decreased investment in water-management systems through time. Our sample size is too small to permit such an inference, but such a scenario would resonate with what has been recently observed in eastern Tianshan and eastern Inner Mongolia where communities turned to extensification of plant cultivation corresponding with an increase in pastoralism (Tian et al., 2022; Sun et al., in review).
7.2 The question of manuring
As previously mentioned, plant δ15N values reflect soil conditions during plant growth. When compared to the average high-manured δ15N values in experiments or other studies in the surrounding regions, the Tibetan barley grains are extremely 15N enriched (Fraser et al., 2011; Kanstrup et al., 2011; Styring et al., 2016a; Tian et al., 2018). It is possible that the barley was grown in areas subject to seasonal flooding which may cause a waterlogged environment susceptible to denitrification and thus higher grain δ15N values. In an experiment in the eastern Mediterranean, Hartman and Danin (2010) find a mean increase of 3.5‰ δ15N in dry wash C3 plants (5.23 ± 2.4‰) as compared to C3 plants grown on exposed ridges (1.7 ± 3.2‰). Even when considering the highest 6‰ offset for aridity (Szpak et al., 2013), the naturally elevated soil δ15N of the Tibetan Plateau at 2.9‰ (Yang et al., 2013), and the influence of potential denitrification, the mean δ15N values range between 13.5–16.5‰ and are still well above the highly manured threshold proposed by Fraser et al. (2011) and that of the local baseline. The high values could indicate over-manuring that would limit yield but still successfully produce grains [as seen by Szpak et al. (2012) in maize experimentally manured with seabird guano]. In an experimental study by Styring et al. (2016a) of Moroccan farming plots, they record δ15N values of barley grains ranging between 12.5–15.4‰ from highly manured and irrigated fields. These data resonate with the results in our study, especially when considering the additional influences of natural soil conditions on the Plateau. This signifies that the ancient herders improved their cultivated fields, likely through animal manure. The δ15N values are variable, which could be the result of variation within a cultivation plot, cultivation in different soil conditions, over different growing seasons, different geographical locations and natural influences, or variable application of manure across the field(s) (Groffman and Hanson, 1997; Finlay and Kendall, 2008; Fraser et al., 2011; Styring et al., 2016a).
7.3 Tibetan cultivation in context
The prehistoric food globalization process likely triggered a series of transformations in subsistence strategies across Eurasia, resulting from the incorporation of non-locally domesticated crops and livestock into the indigenous systems. As part of this process, barley cultivation entered western Tibet, likely via the Kashmir along a southern dispersal route (Liu et al., 2017; Lister et al., 2018; Gao et al., 2021). In a separate, northern pathway, barley—along with wheat—also moved along the mountainous region of Inner Asia, eastwards to Eastern Tianshan and the Hexi Corridor (Liu et al., 2017; Lister et al., 2018). While currently there is no other research on crop labor investment available to compare to on the Tibetan Plateau, our findings can be contextualized with barley stable isotope research from regions connected to Tibet through this prehistoric food globalization process.
North of the Plateau, a recent study shows a similar pattern of early investment in barley cultivation (Tian et al., 2022). During the Bronze Age, communities living in the piedmont zones of the eastern Tianshan provided optimal watering conditions to their 6-row barley crops, whether through simple channel irrigation or opportunistic planting. Additionally, the fields were likely manured. This resonates with our findings in Western Tibet. During the later occupational phase, communities in eastern Tianshan experienced a transition in cultivation strategies from intensification to extensification. Such a trend is documented in crop Δ13C and δ15N values, signifying less water and manuring inputs associated with increased weed taxa (Tian et al., 2022). The researchers attribute this to an increasing reliance on nomadic pastoral lifeways resulting in a reduced labor budget for plant cultivation. Sheng et al. (2021) document a similar trend in the δ15N values of broomcorn and foxtail millet at Bronze Age Shimao, where early heavily manured millet cultivation transitioned to a more extensive system, incorporating more herded livestock (sheep/goats and cattle) as the climate changed and fields were possibly exhausted by the earlier intensive agriculture. The transition to extensive cultivation and pastoralism stands in contrast to the western Tibet data, which show a dedicated pastoral system coinciding with an intensive barley cultivation regime. Further east on the Loess Plateau in Zhuanglang County, isotopic analyses of multi-crop agricultural communities spanning 6,000 BCE to 1,800 CE indicate a separate management system for free-threshing wheat and 6-row barley, where wheat is intensively managed through watering and manuring, while barley is grown under water stress and no significant shifts over time were observed (Li et al., 2022).
Turning to Southwest Asia, research finds different drivers for changes seen in cultivation strategies. In northern Mesopotamia, there was a reduction in manuring through time that correlates with increased site size, from the 6th to 3rd millennium BCE. Additional factors that likely influenced this change were extensification from the increase of land under arable production and the development of a specialized pastoralist economy that reduced the availability of animal manure (Styring et al., 2017). In western Tibet, however, agropastoralists likely invested heavily in barley crops while simultaneously engaging in highland pastoralism. As discussed by Tang et al. (2022), communities at small sites (including the study sites in this paper) in western Tibet utilize dedicated monocropping of barley combined with livestock herding, whereas larger sites show more crop diversity. Zhang et al. (2024) show a unique pastoral system in central Tibet where high labor investment associated with foddering and water provisioning was common practice in high-altitude environments. The intensive cultivation signatures of barley from this study lend further support to the argument that these agropastoralists utilized a risk-reduction strategy of dedicated barley cultivation and intensified herding practice. These dissimilarities in the treatment of barley across regions complicates our notions of strict mobile pastoralism and settled agriculture as a dichotomy when investigating ancient subsistence patterns and call for further studies into the range of choice and possibilities available.
8 Conclusion
In this paper, we analyzed the stable carbon and nitrogen isotope values of barley grains recovered from three western Tibetan sites: Piyang, Jiweng, and Dingdong. The results suggest that when barley was first introduced into the region, the communities used a shared intensive cultivation strategy to successfully grow barley above 4,000 masl. Agropastoralists at Jiweng, Piyang, and Dingdong likely manured their fields for barley cultivation. Communities at Piyang and Jiweng provided additional water to the crops, either by irrigating the plants or strategically planting at locations with higher soil water retention. The community at Dingdong did not supplement watering for their barley as much as the other two sites but still ensured adequate water for their barley. Further work on the wild taxa to identify possible arable weeds to the genus and species level would add additional information regarding cultivation strategies that could be compared to the isotopic results.
At a broader regional level, our results resonate with the farming systems commonly seen in prehistoric northern China, where early farmers invested labor significantly into the cultivation of wheat, barley, and foxtail and broomcorn millet (Sheng et al., 2021; Li et al., 2022). Our results, however, differs from the extensification trend later seen in northern China and in eastern Tianshan that has been recently documented by isotope analysis (Tian et al., 2022). Additionally, we compared the isotopic results with the morphometrics of the barley grains to ascertain the role of growing conditions in grain size. We did not find any meaningful correlation between isotopically inferred growing conditions and barley grain metrics. Admittedly, the sample size is small, but already at the limit of destructive work allowed due to the limited number of grains recovered. When the opportunity arises, future isotopic and archaeobotanical work with a larger sample size can clarify the trends observed in this study.
Data availability statement
The original contributions presented in the study are included in the article/Supplementary material, further inquiries can be directed to the corresponding author/s.
Author contributions
MR: Data curation, Writing – review & editing, Writing – original draft, Visualization, Methodology, Investigation, Funding acquisition, Formal analysis, Conceptualization. LT: Resources, Writing – review & editing, Methodology, Investigation. PV: Supervision, Methodology, Formal analysis, Conceptualization, Writing – review & editing, Investigation. HL: Writing – review & editing, Resources, Investigation. YS: Writing – review & editing, Visualization, Methodology, Conceptualization. MF: Writing – review & editing, Supervision, Conceptualization. XL: Data curation, Writing – review & editing, Writing – original draft, Visualization, Supervision, Resources, Methodology, Investigation, Funding acquisition, Formal analysis, Conceptualization.
Funding
The author(s) declare financial support was received for the research, authorship, and/or publication of this article. This research was supported by a Doctoral Dissertation Improvement Research Grant from the United States' National Science Foundation (no. 2230527).
Acknowledgments
We would like to thank Melanie Suess and Jennifer Houghton for their work managing the EA Mass Spectrometers at the Bradley Laboratory and Fike Laboratory, Washington University in St. Louis.
Conflict of interest
The authors declare that the research was conducted in the absence of any commercial or financial relationships that could be construed as a potential conflict of interest.
The author(s) declared that they were an editorial board member of Frontiers, at the time of submission. This had no impact on the peer review process and the final decision.
Publisher's note
All claims expressed in this article are solely those of the authors and do not necessarily represent those of their affiliated organizations, or those of the publisher, the editors and the reviewers. Any product that may be evaluated in this article, or claim that may be made by its manufacturer, is not guaranteed or endorsed by the publisher.
Supplementary material
The Supplementary Material for this article can be found online at: https://www.frontiersin.org/articles/10.3389/fearc.2024.1398209/full#supplementary-material
References
Aldenderfer, M. S. (2011). Peopling the Tibetan Plateau: Insights from Archaeology. High Alt. Med. Biol. 12, 141–147. doi: 10.1089/ham.2010.1094
Altenbach, S. B., DuPont, F. M., Kothari, K. M., Chan, R., Johnson, E. L., and Lieu, D. (2003). Temperature, water and fertilizer influence the timing of key events during grain development in a US spring wheat. J. Cereal Sci. 37, 9–20. doi: 10.1006/jcrs.2002.0483
Ambrose, S. (2000). “Controlled diet and climate experiments on nitrogen isotope ratios of rats,” in Biogeochemical Approaches to Paleodietary Analysis, eds. S. H. Ambrose and M. A. Katzenberg (New York: Kluwer Academic/Plenum), 243–259. doi: 10.1007/0-306-47194-9_12
Ambrose, S. H. (1991). Effects of diet, climate and physiology on nitrogen isotope abundances in terrestrial foodwebs. J. Archaeol. Sci. 18, 293–317. doi: 10.1016/0305-4403(91)90067-Y
Araus, J. L., Febrero, A., Buxó, R., Rodríguez-Ariza, M. O., Molina, F., Camalich, M. D., et al. (1997). Identification of ancient irrigation practices based on the carbon isotope discrimination of plant seeds: a case study from the South-East Iberian Peninsula. J. Archaeol. Sci. 24, 729–740. doi: 10.1006/jasc.1997.0154
Araus, J. L., Febrero, A., Catala, M., Molist, M., Voltas, J., and Romagosa, I. (1999). Crop water availability in early agriculture: evidence from carbon isotope discrimination of seeds from a tenth millennium BP site on the Euphrates. Glob. Chang. Biol. 5, 201–212. doi: 10.1046/j.1365-2486.1999.00213.x
Bogaard, A., Fraser, R., Heaton, T. H. E., Wallace, M., Vaiglova, P., Charles, M., et al. (2013). Crop manuring and intensive land management by Europe's first farmers. PNAS 110, 12589–12594. doi: 10.1073/pnas.1305918110
Bogaard, A., Heaton, T. H. E., Poulton, P., and Merbach, I. (2007). The impact of manuring on nitrogen isotope ratios in cereals: archaeological implications for reconstruction of diet and crop management practices. J. Archaeol. Sci. 34, 335–343. doi: 10.1016/j.jas.2006.04.009
Calin-Jagemen, R. (2024). esci: Estimation Statistics with Confidence Intervals, Version 1.0.5. Available at: https://CRAN.R-project.org/package=esci. doi: 10.32614/CRAN.package.esci
Charles, M., Forster, E., Wallace, M., and Jones, G. (2015). “Nor ever lightning char thy grain”: establishing archaeologically relevant charring conditions and their effect on glume wheat grain morphology. Sci. Technol. Archaeol. Res. 1, 1–6. doi: 10.1179/2054892315Y.0000000008
Chen, F., Dong, G., Zhang, D., Liu, X., Jia, X., An, C. B., et al. (2015). Agriculture facilitated permanent human occupation of the Tibetan Plateau after 3600 B.P. Science 347, 248–250. doi: 10.1126/science.1259172
Chen, N., Zhang, Z., Hou, J., Chen, J., Gao, X., Tang, L., et al. (2023). Evidence for early domestic yak, taurine cattle, and their hybrids on the Tibetan Plateau. Sci. Adv. 9:eadi6857. doi: 10.1126/sciadv.adi6857
Chen, X., Lü, H., Liu, X., and Frachetti, M. D. (2024). Geospatial modelling of farmer – herder interactions maps cultural geography of Bronze and Iron Age Tibet, 3600 – 2200 BP. Sci. Rep. 14:2010. doi: 10.1038/s41598-023-50556-9
Cui, Y., Hu, Y., Chen, H., Dong, Y., Guan, L., Weng, Y., et al. (2006). Zongri yizhi rengu de wending tongweisu fenxi (Stable isotopic analysis on human bones from Zongri site). Disiji Yanjiu. 26, 604–611. doi: 10.3321/j.issn:1001-7410.2006.04.016
Dal Corso, M., Pashkevych, G., Filipović, D., Liu, X., Motuzaite Matuzeviciute, G., Stobbe, A., et al. (2022). Between Cereal Agriculture and Animal Husbandry: Millet in the Early Economy of the North Pontic Region. New York: Springer US. doi: 10.1007/s10963-022-09171-1
d'Alpoim Guedes, J. (2015). Rethinking the spread of agriculture to the Tibetan Plateau. Holocene 25, 1498–1510. doi: 10.1177/0959683615585835
d'Alpoim Guedes, J., and Aldenderfer, M. (2020). The Archaeology of the Early Tibetan Plateau: New Research on the Initial Peopling through the Early Bronze Age. New York: Springer US. doi: 10.1007/s10814-019-09137-6
d'Alpoim Guedes, J., Lu, H., Li, Y., Spengler, R. N., Wu, X., and Aldenderfer, M. S. (2014). Moving agriculture onto the Tibetan plateau: the archaeobotanical evidence. Archaeol. Anthropol. Sci. 6, 255–269. doi: 10.1007/s12520-013-0153-4
Deniro, M. J., and Epstein, S. (1981). Influence of diet on the distribution of nitrogen isotopes in animals. Geochim. Cosmochim. Acta 45, 341–351. doi: 10.1016/0016-7037(81)90244-1
Dupont, F. M., and Altenbach, S. B. (2003). Molecular and biochemical impacts of environmental factors on wheat grain development and protein synthesis. J. Cereal Sci. 38, 133–146. doi: 10.1016/S0733-5210(03)00030-4
Endo, E., Shoda, S., Frachetti, M., Kaliyeva, Z., Kiyasbek, G., Zhuniskhanov, A., et al. (2023). Pottery impressions reveal earlier westward dispersal of foxtail millet in inner Asian Mountain Corridor. Agronomy 13:1706. doi: 10.3390/agronomy13071706
Esri (2009). “World Imagery” [basemap]. Meters. “World Imagrery”. Available at: https://services.arcgisonline.com/ArcGIS/rest/services/World_Imagery/MapServer (accessed September 24, 2024).
Farquhar, G. D., Ehleringer, J. R., and Hubick, K. T. (1989). Carbon isotope discrimination and photosynthesis. Annu. Rev. Plant Physiol. Plant Mol. Biol. 40, 503–537. doi: 10.1146/annurev.pp.40.060189.002443
Farrell, R., Sandercock, P. J., Pennock, D. J., and Van Kessel, C. (1996). Landscape-scale variations in leached nitrate: relationship to denitrification and natural nitrogen-15 abundance. Soil Sci. Soc. Am. J. 60, 1410–1415. doi: 10.2136/sssaj1996.03615995006000050017x
Ferrio, J. P., Araus, J. L., Buxó, R., Voltas, J., and Bort, J. (2005). Water management practices and climate in ancient agriculture: inferences from the stable isotope composition of archaeobotanical remains. Veg. Hist. Archaeobot. 14, 510–517. doi: 10.1007/s00334-005-0062-2
Finlay, J. C., and Kendall, C. (2008). “Stable isotope tracing of temporal and spatial variability in organic matter sources to freshwater ecosystems,” in Stable Isotopes in Ecology and Environmental Science, eds. R. Michener and K. Lajtha (Hoboken: Blackwell Publishing Ltd.), 283–333. doi: 10.1002/9780470691854.ch10
Frachetti, M. D., Spengler, R. N., Fritz, G. J., and Mar'Yashev, A. N. (2010). Earliest direct evidence for broomcorn millet and wheat in the central Eurasian steppe region. Antiquity 84, 993–1010. doi: 10.1017/S0003598X0006703X
Fraser, R. A., Bogaard, A., Charles, M., Styring, A. K., Wallace, M., Jones, G., et al. (2013). Assessing natural variation and the effects of charring, burial and pre-treatment on the stable carbon and nitrogen isotope values of archaeobotanical cereals and pulses. J. Archaeol. Sci. 40, 4754–4766. doi: 10.1016/j.jas.2013.01.032
Fraser, R. A., Bogaard, A., Heaton, T., Charles, M., Jones, G., Christensen, B. T., et al. (2011). Manuring and stable nitrogen isotope ratios in cereals and pulses: Towards a new archaeobotanical approach to the inference of land use and dietary practices. J. Archaeol. Sci. 38, 2790–2804. doi: 10.1016/j.jas.2011.06.024
Fu, D. (2001). The discovery, identification and research on archaeobotanical remains from the Neolithic Site of Changguogou. Kaogu 3, 66–74.
Fu, D. (2008). Jiaoyubu Renwen Shehui Kexue Zhongdian Yanjiu Jidi, Sichuan Daxue Zangyansuo, Sichuan Daxue Lishi Wehuaxueyuan Kaoguxi and Xizang Zizhiqu Wenwu Shiye Guanliju [in Chinese] (The excavation report of the Piyang Dongga site). Chengdu: Sichuan Renmin Chubanshe.
Fuller, D. Q., Colledge, S., Murphy, C., and Stevens, C. J. (2017). “Sizing up cereal variation : patterns in grain evolution revealed in chronological and geographical comparisons,” in Miscelánea en homenaje a Lydia Zapata Peña (1965-2015), eds. J. Fernández Eraso, J. Antonio Mujika Alustiza, Á. Arrizabalaga Valbuena, and M. García Díez (Bilbao: Servicio Editorial Universidad Del País Vasco), 131–149.
Fuller, D. Q., and Rowlands, M. (2009). Towards a long-term macro-geography of cultural substances: food and sacrifice traditions in east, west and South Asia. Chinese Rev. Anthropol. 12, 1–37.
Fuller, D. Q., and Rowlands, M. (2011). “Ingestion and food technologies: Maintaining differences over the long-term in West, South and East Asia,” in Interweaving Worlds – Systematic Interactions in Eurasia, 7th to 1st millennia BC (Essays from a conference in memory of Professor Andrew Sherratt), eds. J. Bennett, S. Sherratt, and T. Wilkinson (Oxford: Oxbow Books), 37–60. doi: 10.2307/j.ctvh1dr2k.9
Gao, Y., Yang, J., Ma, Z., Tong, Y., and Yang, X. (2021). New evidence from the Qugong site in the central Tibetan Plateau for the prehistoric Highland Silk Road. Holocene 31, 230–239. doi: 10.1177/0959683620941144
Gasse, F., Arnoldt, M., Fontes, J. C., Fortt, M., Qing, L., Meheres, F., et al. (1991). A 13,000-year climate record from western Tibet. Nature 3, 742–745. doi: 10.1038/353742a0
Gasse, F., Fontes, J. C., Van Campo, E., and Wei, K. (1996). Holocene environmental changes in Bangong Co basin (Western Tibet). Part 4: discussion and conclusions. Palaeogeogr. Palaeoclimatol. Palaeoecol. 120, 79–92. doi: 10.1016/0031-0182(95)00035-6
Girolamo, F., Ferrio, J. P., Bogaard, A., Araus, J. L., and Riehl, S. (2014). Stable isotopes in archaeobotanical research. Veg. Hist. Archaeobot. 24, 215–227. doi: 10.1007/s00334-014-0492-9
Gröcke, D. R., Treasure, E. R., Lester, J. J., Gron, K. J., and Church, M. J. (2021). Effects of marine biofertilisation on Celtic bean carbon, nitrogen and sulphur isotopes: implications for reconstructing past diet and farming practices. Rapid Commun. Mass Spectrom. 35, 1–17. doi: 10.1002/rcm.8985
Groffman, P. M., and Hanson, G. C. (1997). Wetland denitrification: influence of site quality and relationships with wetland delineation protocols. Soil Sci. Soc. Am. J. 61, 323–329. doi: 10.2136/sssaj1997.03615995006100010047x
Halstead, P. (1995). Plough and power: the economic and social significance of cultivation with the ox-drawn ard in the Mediterranean. Bull. Sumer. Agric. 8, 11–22.
Halstead, P. (2000). “Land use in postglacial greece: cultural causes and environmental effects,” in Landscape and land use in postglacial Greece, eds. P. Halstead and C. Frederick (Sheffield: Sheffield Academic Press), 110–128.
Halstead, P. (2006). “Sheep in the Garden: The Integration of Crop and Livestock Husbandry in Early Farming Regimes of Greece and Southern Europe,” in Animals in the Neolithic of Britain and Europe, eds. D. Serjeantson and D. Field (Oxford, UK: Oxbow Books), 42–55. doi: 10.2307/j.ctt1w1vjbn.12
Hartman, G., and Danin, A. (2010). Isotopic values of plants in relation to water availability in the Eastern Mediterranean region. Oecologia 162, 837–852. doi: 10.1007/s00442-009-1514-7
Hermes, T. R., Frachetti, M. D., Dupuy, P. N. D., Mar, A., Nebel, A., and Makarewicz, C. A. (2019). Early integration of pastoralism and millet cultivation in Bronze Age Eurasia. Proc. R. Soc. B 286:20191273. doi: 10.1098/rspb.2019.1273
Hou, G., Chongyi, E., and Xiao, J. (2012). Synthetical reconstruction of the precipitation series of the Qinghai-Tibet Plateau during the holocene (in Chinese). Prog. Geogr. 31, 1117–1123. doi: 10.11820/dlkxjz.2012.09.001
Jones, M. K., Hunt, H., Kneale, C., Lightfoot, E., Lister, D., Liu, X., et al. (2016). Food globalisation in prehistory: The agrarian foundations of an interconnected continent. J. Br. Acad. 4, 73–87. doi: 10.5871/jba/004.073
Kanstrup, M., Thomsen, I. K., Andersen, A. J., Bogaard, A., and Christensen, B. T. (2011). Abundance of 13C and 15N in emmer, spelt and naked barley grown on differently manured soils: towards a method for identifying past manuring practice. Rapid Commun. Mass Spectrom. 25, 2879–2887. doi: 10.1002/rcm.5176
Khazanov, A. M. (1983). Nomads and the Outside World., 2nd Edn. Madison: University of Wisconsin Press.
Knörzer, K. H. (2000). 3000 years of agriculture in a valley of the high Himalayas. Veg. Hist. Archaeobot. 9, 219–222. doi: 10.1007/BF01294636
Kradin, N. N. (2015). “The ecology of inner asian Pastoral nomadism,” in The Ecology of Pastoralism, ed. P. Nick Kardulias (Boulder: University Press of Colorado), 1–291. doi: 10.5876/9781607323433.c003
Larsson, M., and Bergman, J. (2023). Experimental approach to evaluate the effect of growing conditions on cereal grain size and its relevance for interpreting archaeological cereal grain assemblages. J. Archaeol. Sci. 152:105752. doi: 10.1016/j.jas.2023.105752
Laurent, Y. (2015). The Tibetans in the making - barley cultivation and cultural representations. Rev. d'Etudes Tibétaines 33, 73–108.
Lees, S. H., and Bates, D. G. (1974). The origins of specialized nomadic pastoralism: a systemic model. Am. Antiq. 39, 187–193. doi: 10.2307/279581
Li, H., Sun, Y., Yang, Y., Cui, Y., Ren, L., Li, H., et al. (2022). Water and soil management strategies and the introduction of wheat and barley to northern China: an isotopic analysis of cultivation on the Loess Plateau. Antiquity 96, 1478–1494. doi: 10.15184/aqy.2022.138
Lister, D. L., Jones, H., Oliveira, H. R., Petrie, C. A., Liu, X., Cockram, J., et al. (2018). Barley heads east: Genetic analyses reveal routes of spread through diverse Eurasian landscapes. PLoS ONE 13:e0196652. doi: 10.1371/journal.pone.0196652
Liu, X., Jones, P. J., Motuzaite-Matuzeviciute, G., Hunt, H. V., Lister, D. L., An, T., et al. (2019). From ecological opportunism to multi-cropping: mapping food globalisation in prehistory. Quat. Sci. Rev. 206, 21–28. doi: 10.1016/j.quascirev.2018.12.017
Liu, X., Lister, D. L., Zhao, Z., Petrie, C. A., Zeng, X., Jones, P. J., et al. (2017). Journey to the east: Diverse routes and variable flowering times for wheat and barley en route to prehistoric China. PLoS ONE 12:e187405. doi: 10.1371/journal.pone.0187405
Liu, X., Lister, D. L., Zhao, Z., Staff, R. A., Jones, P. J., Zhou, L., et al. (2016). The virtues of small grain size: Potential pathways to a distinguishing feature of Asian wheats. Quat. Int. 426, 107–119. doi: 10.1016/j.quaint.2016.02.059
Liu, X., Matuzevičiute, G. M., and Hunt, H. V. (2018). “From a fertile idea to a fertile arc: the origins of broomcorn millet 15 years on,” in Far from the Hearth: Essays in Honour of Martin K. Jones, eds. E. Lightfoot, X. Liu, and D. Q. Fuller (Cambridge, UK: Cambridge University Press), 155–164.
Liu, X., and Reid, R. E. B. (2020). The prehistoric roots of Chinese cuisines: mapping staple food systems of China, 6000 BC−220 AD. PLoS ONE 15, 1–25. doi: 10.1371/journal.pone.0240930
Lu, H. (2008). ???????????????? [in Chinese] (A Preliminary Study on Related Issues of Ali Dingdong Site in Western Tibet). Tibet. Stud. 4, 56–70.
Lu, H. (2023). Local millet farming and permanent occupation on the Tibetan Plateau. Sci. China Earth Sci. 66, 430–434. doi: 10.1007/s11430-022-1018-7
Lu, H., Chen, X., Zhang, Z., Tang, L., Lemoine, X., Wangdue, S., et al. (2021). Early agropastoral settlement and cultural change in central Tibet in the first millennium BC: excavations at Bangga. Antiquity 95, 955–972. doi: 10.15184/aqy.2020.185
Ma, M., Dong, J., Yang, Y., Jones, M. K., Wang, J., Chen, G., et al. (2023). Isotopic evidence reveals the gradual intensification of millet agriculture in Neolithic western Loess Plateau. Fundam. Res. 2023, 1–17. doi: 10.1016/j.fmre.2023.06.007
Millard, S. P. (2013). EnvStats: An R Package for Environmental Statistics. New York: Springer. doi: 10.1007/978-1-4614-8456-1
Miller, N. F. (2003). “The Use of Plants at Anau North,” in A Central Asian Village at the Dawn of Civilization: Excavations at Anau, Turkmenistan, eds. F. T. Hiebert and K. Kurbansakhatov (Philadelphia: University of Pennsylvania Museum).
Minagawa, M., and Wada, E. (1984). Stepwise enrichment of 15N along food chains: further evidence and the relation between δ15N and animal age. Geochim. Cosmochim. Acta 48, 1135–1140. doi: 10.1016/0016-7037(84)90204-7
Morrison, K. D. (1994). The intensification of production archaeological approaches. J. Archaeol. Method Theory 1, 111–159. doi: 10.1007/BF02231414
Motuzaite Matuzeviciute, G., Mir-Makhamad, B., and Spengler, R. N. (2021). Interpreting diachronic size variation in prehistoric central asian cereal grains. Front. Ecol. Evol. 9, 1–15. doi: 10.3389/fevo.2021.633634
Motuzaite-Matuzeviciute, G., Abdykanova, A., Kume, S., Nishiaki, Y., and Tabaldiev, K. (2018). The effect of geographical margins on cereal grain size variation: case study for highlands of Kyrgyzstan. J. Archaeol. Sci. Reports 20, 400–410. doi: 10.1016/j.jasrep.2018.04.037
Murakami, N., Onggaruly, A., Rakhimzhanova, S., Standall, E. A., Talbot, H. M., Lucquin, A., et al. (2022). Lipid residues in ancient pastoralist pottery from Kazakhstan reveal regional differences in cooking practices. Front. Ecol. Evol. 10:1032637. doi: 10.3389/fevo.2022.1032637
Nitsch, E. K., Charles, M., and Bogaard, A. (2015). Calculating a statistically robust δ13C and δ15N offset for charred cereal and pulse seeds. Sci. Technol. Archaeol. Res. 1, 1–8. doi: 10.1179/2054892315Y.0000000001
O'Leary, M. H. (1988). Carbon Isotopes in Photosynthesis: fraction techniques may reveal new aspects of carbon dynamics in plants. Bioscience 38, 328–336. doi: 10.2307/1310735
Pokharia, A. K., Kharakwal, J. S., Rawat, R. S., Osada, T., Nautiyal, C. M., and Srivastava, A. (2011). Archaeobotany and archaeology at Kanmer, a Harappan site in Kachchh, Gujarat: evidence for adaptation in response to climatic variability. Curr. Sci. 100, 1833–1846. Available at: http://www.jstor.org/stable/24077554
Pokharia, A. K., and Saraswat, K. (2002). Harappan plant economy at ancient Balu, Haryana. Pragdhara 12, 153–172.
R Core Team (2023). R: A language and environment for statistical computing. Available at: https://www.r-project.org/ (accessed September 25, 2024).
Ren, L., Dong, G., Liu, F., d'Alpoim-Guedes, J., Flad, R. K., Ma, M., et al. (2020). Foraging and farming: archaeobotanical and zooarchaeological evidence for Neolithic exchange on the Tibetan Plateau. Antiquity 94, 637–652. doi: 10.15184/aqy.2020.35
Ritchey, M. M., Sun, Y., Motuzaite Matuzeviciute, G., Shoda, S., Pokharia, A. K., Spate, M., et al. (2022). The wind that shakes the barley: the role of East Asian cuisines on barley grain size. World Archaeol. 53, 287–304. doi: 10.1080/00438243.2022.2030792
Sakamoto, S. (1996). “Glutinous-endosperm starch food culture specific to Eastern and Southeastern Asia,” in Redefining Nature: Ecology, Culture and Domestication, eds. R. Ellen and K. Fukui (Berg, Oxford: Routledge), 215–231. doi: 10.4324/9781003135746-10
Savin, R., and Nicolas, M. E. (1996). Effects of short periods of drought and high temperature on grain growth and starch accumulation of two malting barley cultivars. Aust. J. Plant Physiol. 23, 201–210. doi: 10.1071/PP9960201
Sheng, P., Shang, X., Zhou, X., Storozum, M., Yang, L., Guo, X., et al. (2021). Feeding Shimao: archaeobotanical and isotopic investigation into early urbanism (4200-3000 BP) on the Northern Loess Plateau, China. Environ. Archaeol. 29, 425–439. doi: 10.1080/14614103.2021.2009995
Song, J., Gao, Y., Tang, L., Zhang, Z., Hayashi Tang, M., Xu, H., et al. (2021). Farming and multi-resource subsistence in the third and second millennium BC: archaeobotanical evidence from Karuo. Archaeol. Anthropol. Sci. 13, 1–16. doi: 10.1007/s12520-021-01281-9
Song, J., Lu, H., Zhang, Z., and Liu, X. (2018). Archaeobotanical remains from the mid-first millennium AD site of Kaerdong in western Tibet. Archaeol. Anthropol. Sci. 10, 2015–2026. doi: 10.1007/s12520-017-0521-6
Spate, M., Zhang, G., Yatoo, M., and Betts, A. (2017). New evidence for early 4th millennium BP agriculture in the Western Himalayas: Qasim Bagh, Kashmir. J. Archaeol. Sci. Reports 11, 568–577. doi: 10.1016/j.jasrep.2016.12.038
Spengler, R. N. (2015). Agriculture in the central Asian bronze age. J. World Prehistory 28, 215–253. doi: 10.1007/s10963-015-9087-3
Spengler, R. N. (2018). Dung burning in the archaeobotanical record of West Asia: where are we now? Veg. Hist. Archaeobot. 28, 215–227. doi: 10.1007/s00334-018-0669-8
Stevens, C. J., Murphy, C., Roberts, R., Lucas, L., Silva, F., and Fuller, D. Q. (2016). Between China and South Asia: a Middle Asian corridor of crop dispersal and agricultural innovation in the Bronze Age. Holocene 26, 1541–1555. doi: 10.1177/0959683616650268
Stroud, E., Charles, M., Bogaard, A., and Hamerow, H. (2023a). Turning up the heat: Assessing the impact of charring regime on the morphology and stable isotopic values of cereal grains. J. Archaeol. Sci. 153:105754. doi: 10.1016/j.jas.2023.105754
Stroud, E., Charles, M., Bogaard, A., Nitsch, E., and Hamerow, H. (2023b). The experimental heating of rye, oat, spelt, wheat and barley between 215 and 300 °C: the stable carbon and nitrogen isotope data and the photographic evidence of changes to the morphology of the grains. Data Br. 50:109544. doi: 10.1016/j.dib.2023.109544
Styring, A. K., Ater, M., Hmimsa, Y., Fraser, R., Miller, H., Neef, R., et al. (2016a). Disentangling the effect of farming practice from aridity on crop stable isotope values: a present-day model from Morocco and its application to early farming sites in the eastern mediterranean. Anthr. Rev. 3, 2–22. doi: 10.1177/2053019616630762
Styring, A. K., Charles, M., Fantone, F., Hald, M. M., McMahon, A., Meadow, R. H., et al. (2017). Isotope evidence for agricultural extensification reveals how the world's first cities were fed. Nat. Plants 3, 1–11. doi: 10.1038/nplants.2017.76
Styring, A. K., Hmimsa, Y., Ater, M., and Bogaard, A. (2015). “The potential of crop isotopes to identify past manuring practices in arid regions,” in 8th International Workshop for African Archaeobotany, (Modena and Reggio Emilia, Italy: Associato alla Unione Stampa Periodica Italiana), 77–79.
Styring, A. K., Knipper, C., Müller-Scheeßel, N., Grupe, G., and Bogaard, A. (2018). The proof is in the pudding: crop isotope analysis provides direct insights into agricultural production and consumption. Environ. Archaeol. 27, 61–72. doi: 10.1080/14614103.2018.1497832
Styring, A. K., Maier, U., Stephan, E., Schlichtherle, H., and Bogaard, A. (2016b). Cultivation of choice: new insights into farming practices at Neolithic lakeshore sites. Antiquity 90, 95–110. doi: 10.15184/aqy.2015.192
Styring, A. K., Manning, H., Fraser, R. A., Wallace, M., Jones, G., Charles, M., et al. (2013). The effect of charring and burial on the biochemical composition of cereal grains: Investigating the integrity of archaeological plant material. J. Archaeol. Sci. 40, 4767–4779. doi: 10.1016/j.jas.2013.03.024
Sun, Y., Jia, X., Reid, R. E. B., Sun, Y., and Liu, X. (in review). Agricultural extensification corresponding to the increase of pastoral activities in prehistoric Chifeng, Northeast China. Archaeol. Anthropol. Sci.
Sun, Y., Ritchey, M., Zhong, H., and Tang, L. (2024b). Variation of millet grain size and cooking techniques across Asia between the late fourth and fi rst millennia BC. Antiquity 98, 401–416. doi: 10.15184/aqy.2024.31
Sun, Y., Ritchey, M. M., Zhong, H., Tang, L., Sergusheva, E., Shi, T., et al. (2024a). Grain size variations of millets and cooking techniques across Asia between the late fourth and first millennium BCE. Antiquity. 98. doi: 10.15184/aqy.2024.31
Szpak, P. (2014). Complexities of nitrogen isotope biogeochemistry in plant-soil systems: implications for the study of ancient agricultural and animal management practices. Front. Plant Sci. 5, 1–19. doi: 10.3389/fpls.2014.00288
Szpak, P., Longstaffe, F. J., Millaire, J. F., and White, C. D. (2012). Stable isotope biogeochemistry of seabird guano fertilization: Results from growth chamber studies with maize (Zea Mays). PLoS ONE 7:e33741. doi: 10.1371/journal.pone.0033741
Szpak, P., Metcalfe, J. Z., and Macdonald, R. A. (2017). Best practices for calibrating and reporting stable isotope measurements in archaeology. J. Archaeol. Sci. Reports 13, 609–616. doi: 10.1016/j.jasrep.2017.05.007
Szpak, P., White, C. D., Longstaffe, F. J., Millaire, J. F., and Vásquez Sánchez, V. F. (2013). Carbon and Nitrogen Isotopic Survey of Northern Peruvian Plants: Baselines for Paleodietary and Paleoecological Studies. PLoS ONE 8:e53763. doi: 10.1371/journal.pone.0053763
Tang, L. (2024). High-altitude dietary adaptations on the interior Tibetan Plateau in prehistory: Archaeobotanical, paleoproteomic, and stable isotopic evidence (Doctoral Diss). University of Kiel, Kiel.
Tang, L., Lu, H., Chen, X., Xu, H., Boivin, N., Storozum, M., et al. (2022). Prehistoric agricultural decision making in the western Himalayas: ecological and social variables. Antiquity 96, 1214–1231. doi: 10.15184/aqy.2022.80
Tang, L., Lu, H., Song, J., Wangdue, S., Chen, X., Zhang, Z., et al. (2021). The transition to a barley-dominant cultivation system in Tibet: first millennium BC archaeobotanical evidence from Bangga. J. Anthropol. Archaeol. 61:101242. doi: 10.1016/j.jaa.2020.101242
Tang, L., Wilkin, S., Richter, K. K., Bleasdale, M., Fernandes, R., He, Y., et al. (2023). Paleoproteomic evidence reveals dairying supported prehistoric occupation of the highland Tibetan Plateau. Sci. Adv. 9, 1–13. doi: 10.1126/sciadv.adf0345
The Jamovi Project (2023). jamovi. Version 2.4. Available at: https://www.jamovi.org (accessed September 25, 2024).
Tian, D., Ma, J., Wang, J., Pilgram, T., Zhao, Z., and Liu, X. (2018). Cultivation of naked barley by early iron age agro-pastoralists in Xinjiang, China. Environ. Archaeol. 23, 416–425. doi: 10.1080/14614103.2017.1415121
Tian, D., Sun, Y., Ritchey, M. M., Xi, T., Ren, M., Ma, J., et al. (2022). Varying cultivation strategies in eastern Tianshan corresponded to growing pastoral lifeways between 1300 BCE and 300 CE. Front. Ecol. Evol. 10:966366. doi: 10.3389/fevo.2022.966366
Vaiglova, P., Bogaard, A., Collins, M., Cavanagh, W., Mee, C., Renard, J., et al. (2014a). An integrated stable isotope study of plants and animals from Kouphovouno, southern Greece: a new look at Neolithic farming. J. Archaeol. Sci. 42, 201–215. doi: 10.1016/j.jas.2013.10.023
Vaiglova, P., Lazar, N. A., Stroud, E. A., Loftus, E., and Makarewicz, C. A. (2022). Best practices for selecting samples, analyzing data, and publishing results in isotope archaeology. Quat. Int. 650, 86–100. doi: 10.1016/j.quaint.2022.02.027
Vaiglova, P., Reid, R. E. B., Lightfoot, E., Pilaar Birch, S. E., Wang, H., Chen, G., et al. (2021). Localized management of non-indigenous animal domesticates in Northwestern China during the Bronze Age. Sci. Rep. 11:15764. doi: 10.1038/s41598-021-95233-x
Vaiglova, P., Snoeck, C., Nitsch, E., Bogaard, A., and Lee-Thorp, J. (2014b). Impact of contamination and pre-treatment on stable carbon and nitrogen isotopic composition of charred plant remains. Rapid Commun. Mass Spectrom. 28, 2497–2510. doi: 10.1002/rcm.7044
van Bommel, D., Bruins, H. J., Lazarovitch, N., and van der Plicht, J. (2021). Effect of dung, ash and runoff water on wheat and barley grain sizes and stable isotope ratios: experimental studies in ancient desert agriculture (Negev, Israel). J. Archaeol. Sci. Rep. 39:103172. doi: 10.1016/j.jasrep.2021.103172
Wallace, M., Jones, G., Charles, M., Fraser, R., Halstead, P., Heaton, T. H. E., et al. (2013). Stable carbon isotope analysis as a direct means of inferring crop water status and water management practices. World Archaeol. 45, 388–409. doi: 10.1080/00438243.2013.821671
Wang, Y., Gao, Y., Yang, J., Tan, Y., Shargan, W., Zhang, S., et al. (2021). New evidence for early human habitation in the Nyingchi Region, Southeast Tibetan Plateau. Holocene 31, 240–246. doi: 10.1177/0959683620970255
Wickham, H. (2016). ggplot2: Elegant Graphics for Data Analysis. New York: Springer-Verlag. doi: 10.1007/978-3-319-24277-4
Yang, Y., Ji, C., Robinson, D., Zhu, B., Fang, H., Shen, H., et al. (2013). Vegetation and soil 15N natural abundance in alpine grasslands on the tibetan plateau: patterns and implications. Ecosystems 16, 1013–1024. doi: 10.1007/s10021-013-9664-1
Zhang, Z., Chen, Z., Marshall, F., Lü, H., Lemoine, X., Wangyal, T., et al. (2019). The importance of localized hunting of diverse animals to early inhabitants of the Eastern Tibetan Plateau at the Neolithic site of Xiaoenda. Quat. Int. 529, 38–46. doi: 10.1016/j.quaint.2019.09.019
Zhang, Z., Lu, H., Wangdue, X., Chen, L., Tang, H., Song, J., et al. (2024). Sequential isotope analyses of enamel bioapatite on the Tibetan Plateau reveal sheep and goat provisioning at high elevation environment, 3000-2200 BP. Antiquity 2024, 1–17. doi: 10.15184/aqy.2024.137
Zhang, Z., Xu, H., Wangdue, S., Gao, X., Hongliang, L., Liu, X., et al. (2022). High lamb natural mortality versus specialized culling at Bangga on the southern Tibetan Plateau 3000 BP. Int. J. Osteoarchaeol. 33, 6–15. doi: 10.1002/oa.3167
Keywords: barley cultivation, stable isotope analysis, cultivation strategies, archaeobotany, Tibetan Plateau
Citation: Ritchey MM, Tang L, Vaiglova P, Lu H, Sun Y, Frachetti MD and Liu X (2024) Into thin air: prehistoric intensive crop management in high altitude western Tibet. Front. Environ. Archaeol. 3:1398209. doi: 10.3389/fearc.2024.1398209
Received: 09 March 2024; Accepted: 14 October 2024;
Published: 01 November 2024.
Edited by:
Rosie Bishop, University of Stavanger, NorwayReviewed by:
Robert C. Power, University College Dublin, IrelandJade D'Alpoim Guedes, University of California, San Diego, United States
Darren R. Gröcke, Durham University, United Kingdom
Copyright © 2024 Ritchey, Tang, Vaiglova, Lu, Sun, Frachetti and Liu. This is an open-access article distributed under the terms of the Creative Commons Attribution License (CC BY). The use, distribution or reproduction in other forums is permitted, provided the original author(s) and the copyright owner(s) are credited and that the original publication in this journal is cited, in accordance with accepted academic practice. No use, distribution or reproduction is permitted which does not comply with these terms.
*Correspondence: Melissa M. Ritchey, bW1yaXRjaGV5QHd1c3RsLmVkdQ==; Xinyi Liu, bGl1eGlueWlAd3VzdGwuZWR1