- 1Department of Anthropology, University of Cincinnati, Cincinnati, OH, United States
- 2Department of Geography, University of Calgary, Calgary, AB, Canada
- 3W. S. Webb Museum of Anthropology, University of Kentucky, Lexington, KY, United States
Despite convincing archaeological arguments about the global effects of human pyrogeography and their evolutionary significance, many of the implicated data sources are unavailable in research contexts that lack significant accumulations of charcoal or stands of fire-scarred trees. In view of the strong likelihood that hominins routinely ignited small, low-intensity landscape fires for millennia, we explore the role of cultural burning for food-production in an area of the American Southwest where anthropogenic fire has not been considered. To illustrate the virtues of a multidisciplinary approach, informed by Formation Theory and time perspectivism, we focus on the returns from macrobotanical and palynological analyses of samples recovered from a variety of archaeological and geoarchaeological contexts in the Upper Basin, a landform located south of the Grand Canyon in northern Arizona. Previous archaeobotanical studies of samples recovered from archaeological sites (ca. AD 500–1500) in the basin's pinyon-juniper woodlands are dominated by amaranth, chenopodium, and other economic ruderals. These findings support the “fire foodway” model that posits prehistoric Indigenous populations of the Upper Basin depended on these fire-following wild plants, rather than maize, by harvesting their abundant seeds and leaves from production locations that were created by low-intensity understory fires. In this paper, we present the results of new studies of archaeobotanical remains recovered from cut-back terraces and sedimentary contexts that (i) expand the evidence base for the fire-foodway model, (ii) provide a basis for proposing several types of prehistoric cultural burning practices, and (iii) introduce the outlines of the ruderal seed-bed hypothesis. Combined, these findings provide a new archaeological perspective on upland subsistence practices in the northern American Southwest. Our study also highlights biases of modern vegetation surveys that do not include archaeological data, and contributes to an appreciation of the extent to which biodiversity has declined because of widespread fire exclusion.
1 Introduction
Nowadays, “breaking news” cycles that populate all types of media are apt to feature shocking images of ferocious wildfires and alarming reports of barely effective fire-control measures. The reporting segments, accompanied by snappy graphics and videos, predictably pivot to reminding viewers how unprecedented these calamities are, assessing what it will take to rebuild charred infrastructure, and cautioning them to stay indoors to avoid breathing wildfire-smoke that originated thousands of miles from their smart phones (Pyne, 2021a, p. 13).
However, these and other ecological effects of landscape wildfire—whether ignited by lightning or humans—and the rapidity with which they have overwhelmed an unprepared modern world are hardly “breaking news” to those who study fire. Fire historians (Pyne, 2021b), forest ecologists (Bonnicksen, 2000), anthropologists (Scherjon et al., 2015), and Indigenous peoples (Adlam et al., 2021) know that cultural burning profoundly affects fire regimes and peoples' surroundings (Lake et al., 2017). Despite the persuasive arguments of these studies, it has proven difficult to extrapolate their implications to the investigation of landscape fires that were ignited for food-production purposes in pre-European-contact cultural pasts (Roos et al., 2022). The principal obstacle, when viewed through the lens of archaeology, is the Registration Problem, which refers to how the consequences of “economic fire” become incorporated in the archaeological record and how they can be identified unambiguously today (Smith, 2014; Reddy, 2016). Because of the global diversity and deep history of human pyrogeography (Bowman et al., 2011), attributable to the innumerable reasons humans ignite fires in dissimilar landscapes, the potential range of fire records is vast (Pyne, 1983, p. 10) and likely to be unresponsive to the application of monolithic analytical approaches. For instance, determining fire-scar frequencies is indispensable for some problems, such as inferring the effects of anthropogenic burning on recent fire regimes (Roos et al., 2023), but impractical for other questions, such as differentiating cultural from natural fires that burned millennia ago (Snitker, 2018; Klimaszewski-Patterson, 2023).
Here, we explore the potential of Formation Theory (Lucas, 2012) to advance understandings of the effectiveness of archaeobotany for revealing the consequences of economic fire (Snitker et al., 2022). Specifically, we intend to show that macrobotanical remains and pollen recovered from archaeological features and surfaces in the Grand Canyon area (ca. AD 500-1500) enable the development of evidence of cultural burning for food production rather than for some other fire-related practice, such as stimulating the growth of squawbush branches for the manufacture of the Grand Canyon's famous split-twig figurines (Bohrer, 1983). After reviewing the remarkably thin history of archaeological investigations of economic burning generally and in the northern American Southwest specifically, we summarize our previous work on ruderal agriculture in the Grand Canyon area and present the results of new investigations that serve to introduce several models for differentiating the disparate effects of anthropogenic fire. We conclude with a discussion of how archaeological considerations of human pyrogeography profoundly affect the content and scope of narratives about ancient Indigenous burning practices and their impacts on forest ecosystems today.
2 The uneven history of economic burning studies
Our study is designed to navigate between two factors that historically have hampered the archaeological investigation of economic fire in the northern American Southwest, especially in the region's vast pinyon-juniper woodlands that harbor disproportionately high numbers of archaeological sites compared to other forested biomes (Lanner, 1981, p. 66).
One factor is the lack of sustained disciplinary traction by the anthropological community on the general topic of cultural burning. With the exceptions of Omer C. Stewart, Henry T. Lewis, and Henry F. Dobyns, cultural anthropologists have rarely studied the ecological and economic effects of anthropogenic fire (however, see Coughlan, 2013; Fowler and Welch, 2018). A significant conse-quence of this inattention is that archaeologists have been hard pressed to develop testable hypotheses and interpretive analogies—a grand tradition in American anthropological archaeology (Gibbon, 1984; Trigger, 2006)—about the antiquity, diverse practices, and food-production potential of human-ignited fire (Mellars, 1976).
A second factor is that descriptions of Southwest subsistence economies (e.g., Jorgensen, 1983) rarely mention economic burning or anthropogenic fire ecology (e.g., Adams, 2011). For instance, chapters in Volumes 9 and 10 of the Handbook of North American Indians (Ortiz, 1979, 1983, respectively), authored by leading late 20th-century ethnobotanists, ethnographers, and archaeologists, thoroughly describe maize production practices; however, the economic roles of globally important wild plants, such as chenopodium and amaranth (Dobyns, 1972; Marx, 1977), are not considered. When the production of undomesticated plants is discussed, the treatment is abbreviated, focusing largely on the degree to which they may have been encouraged or tolerated as byproducts—“weeds”—of maize agriculture (Adams and Fish, 2011, p. 169). These highly nutritious ruderals thrive after fire (Huffman et al., 2013), yet inexplicably the importance of purposive burning as a traditional, stand-alone food-production method has been downplayed (Ford, 1984, p. 129; Minnis, 2000, p. 279), if it is considered at all (Fritz, 2007, p. 303; Myers and Doolittle, 2014).
The impacts of these factors are vividly expressed by the archaeology of the heavily forested and once densely occupied Grand Canyon province of northern Arizona (Mink, 2019). As we show, the investigation of cultural burning in this fire-prone region has been disadvantaged, ironically, by efforts to control landscape wildfires, which burn more intensely because of 20th century fire-suppression and other land-management policies (Pyne, 2016; Roos et al., 2020), and have only succeeded in promoting a transition in fire regimes (principally, the production of highly combustible fuel-loads; Margolis, 2014). Complicating matters, fire ecologists agree that the Southwest's pre-fire-suppression forests were compositionally quite different than today's (Covington and Moore, 1994; Guiterman et al., 2022), and were shaped by high-frequency/low-intensity fires that forest occupants created and managed, largely with understory burning for economic purposes (Bonnicksen, 2000). In contrast, some archaeologists claim that current forest composition (species richness) and structure (spatial distribution of species) are indicative of past forest conditions (e.g., Crabtree et al., 2017), which means that prehistoric forest occupants would have been igniting high-intensity, stand-destroying fires at great risk to themselves and the ecosystem's resilience (Kohler, 1992, p. 256; Redman, 1999, p. 65). In view of this situation, it is difficult to appreciate “the degree to which Indian economies were dependent on fire” (Pyne, 1982, p. 71; also, Anderson and Lake, 2013)—a state of affairs we aspire to rectify with the following case study.
3 Searching for clues of economic burning in the Upper Basin
3.1 Environmental background
Symptomatic of the aforementioned issues, the area known as the Upper Basin is located south of the eastern South Rim of the Grand Canyon (Figure 1) and administered by both the USDI National Park Service and the USDA Forest Service under different resource-management plans (Washam, 2014). Topographically, the Upper Basin consists of a series of north-to-south trending, gently-inclined plateaus, capped by Kaibab Limestone (Hopkins and Thompson, 2003), that are cut by narrow drainages. To the west and south, drainages that issue from the encircling Coconino Rim are deeply incised and end abruptly at Lee Canyon. Runoff in the Upper Basin that does not percolate through its porous limestone surface (Metzger, 1961) is directed into rills that merge into larger discontinuous, ephemeral streams (see Bull, 1997 for an overview of the geomorphology of these types of drainages).
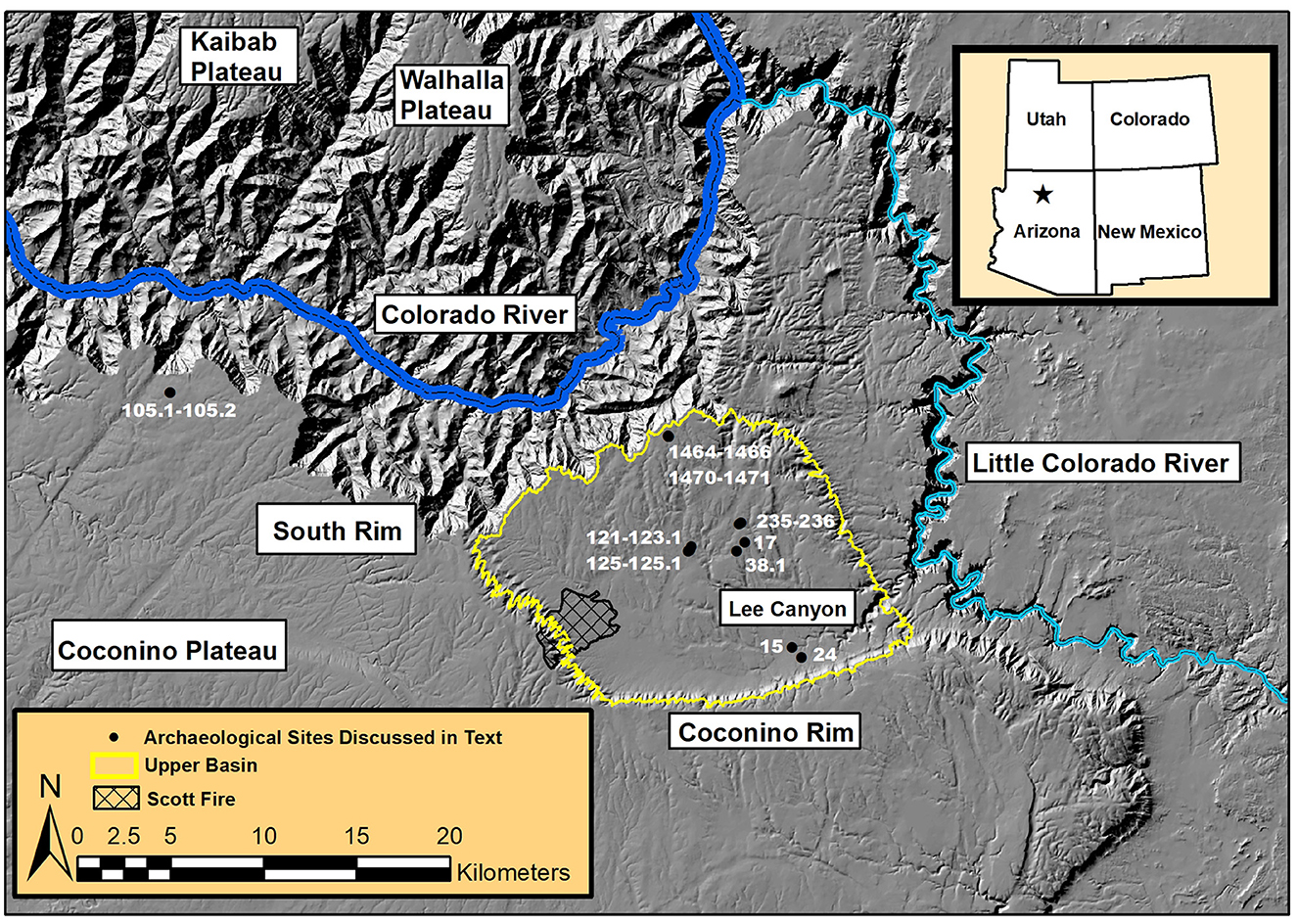
Figure 1. Regional map of northern Arizona showing prominent places, archaeological sites, and landscape features mentioned in the text, including the Coconino Plateau, the South Rim of the Grand Canyon, the Coconino Rim, the Scott Fire burned area, Lee Canyon, and the Upper Basin.
Pronounced bi-seasonal precipitation (mean = 40.9 cm ann-ually, standard deviation = 12.9 cm; Sullivan and Ruter, 2006, p. 185–186), with low-energy discharges in late autumn through early spring and high-energy discharges from mid-summer through early autumn (Darling, 1967), enables the transport of silts and sands and, ultimately, the development of rock-filled silty or sandy loams in drainages (Rand, 1965, p. 73). Between these periods of seasonal precipitation, pedogenic processes and vegetation growth contribute to landform stability and cumulic soil development. In general, the Upper Basin is devoid of water sources, and the soils that form from underlying limestone or sandstone tend to be shallow (25–35 cm) and rocky, resulting in a surface that is typified by significant exposures of fractured bedrock, decomposing bed-rock, and gravel (McNamee et al., 2021).
Today, the pinyon-juniper woodland that blankets most of the Upper Basin (Figure 2), which ranges in elevation between 2,255 masl at the South Rim to 1,859 masl at Lee Canyon, is so dangerously overstocked that inter-tree branch comingling makes the woodland highly fire-prone (Vankat, 2013, p. 278–281; Figure 3). In those settings that are covered by dense stands of ponderosa pine (western reaches of the Upper Basin; Merkle, 1952) or by grasslands (eastern reaches of the Upper Basin; Darling, 1967), understory biodiversity has declined significantly because of cattle grazing and elk management (Bye, 1985, p. 379), which has led to the expansion of bare soil patches and the onset of gullying (Williams and Baker, 2013). For the portion of the Upper Basin managed by Kaibab National Forest, uncontrolled recreational camping, permitted and illegal elk and deer hunting, and unpermitted woodcutting have contributed to the severity of erosion (Sullivan et al., 2002a; Uphus et al., 2006).
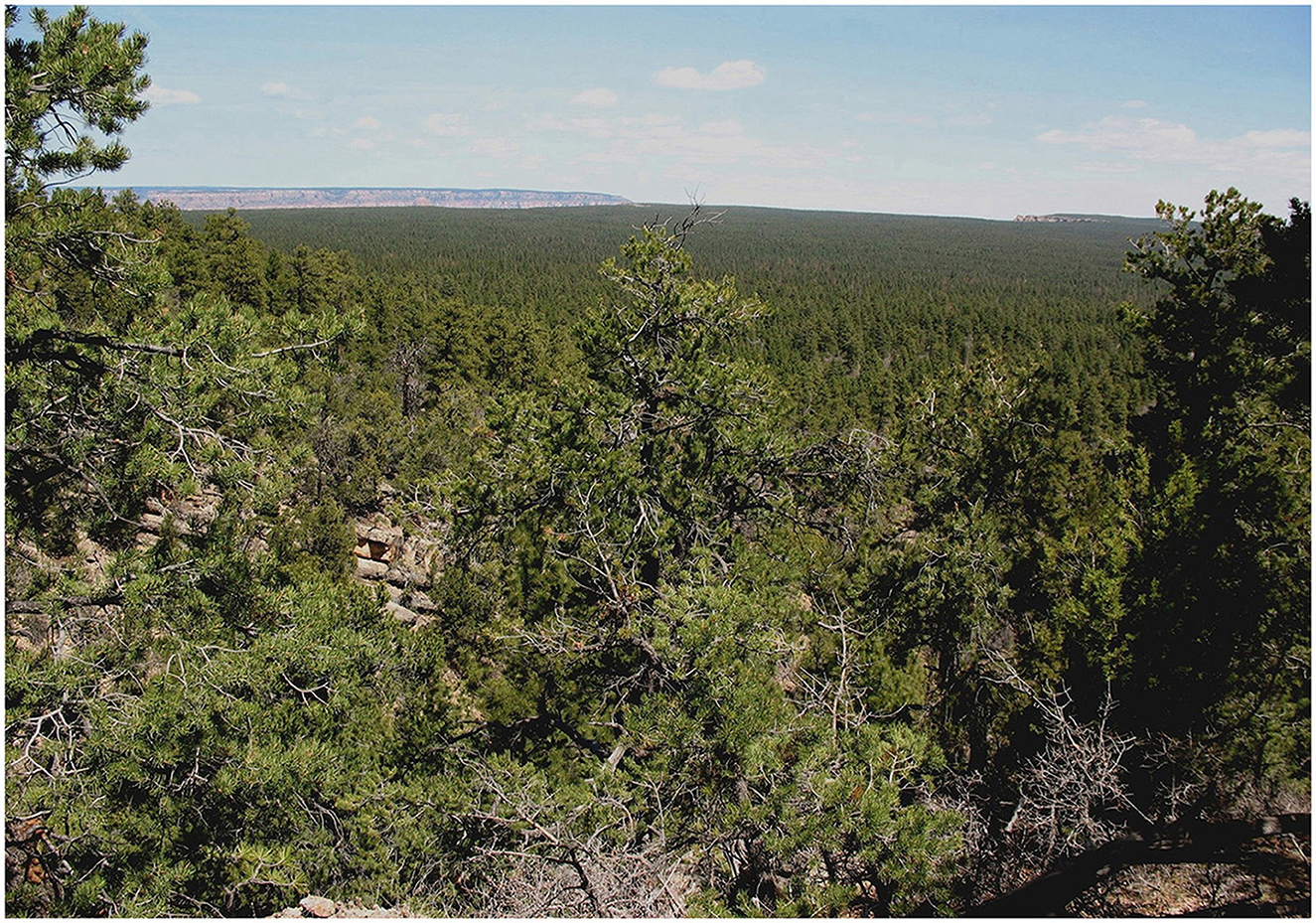
Figure 2. Panoramic view of the Upper Basin looking north from the Coconino Rim to the Walhalla Plateau on the North Rim showing the high density of ponderosa pine, pinyon, and juniper trees.
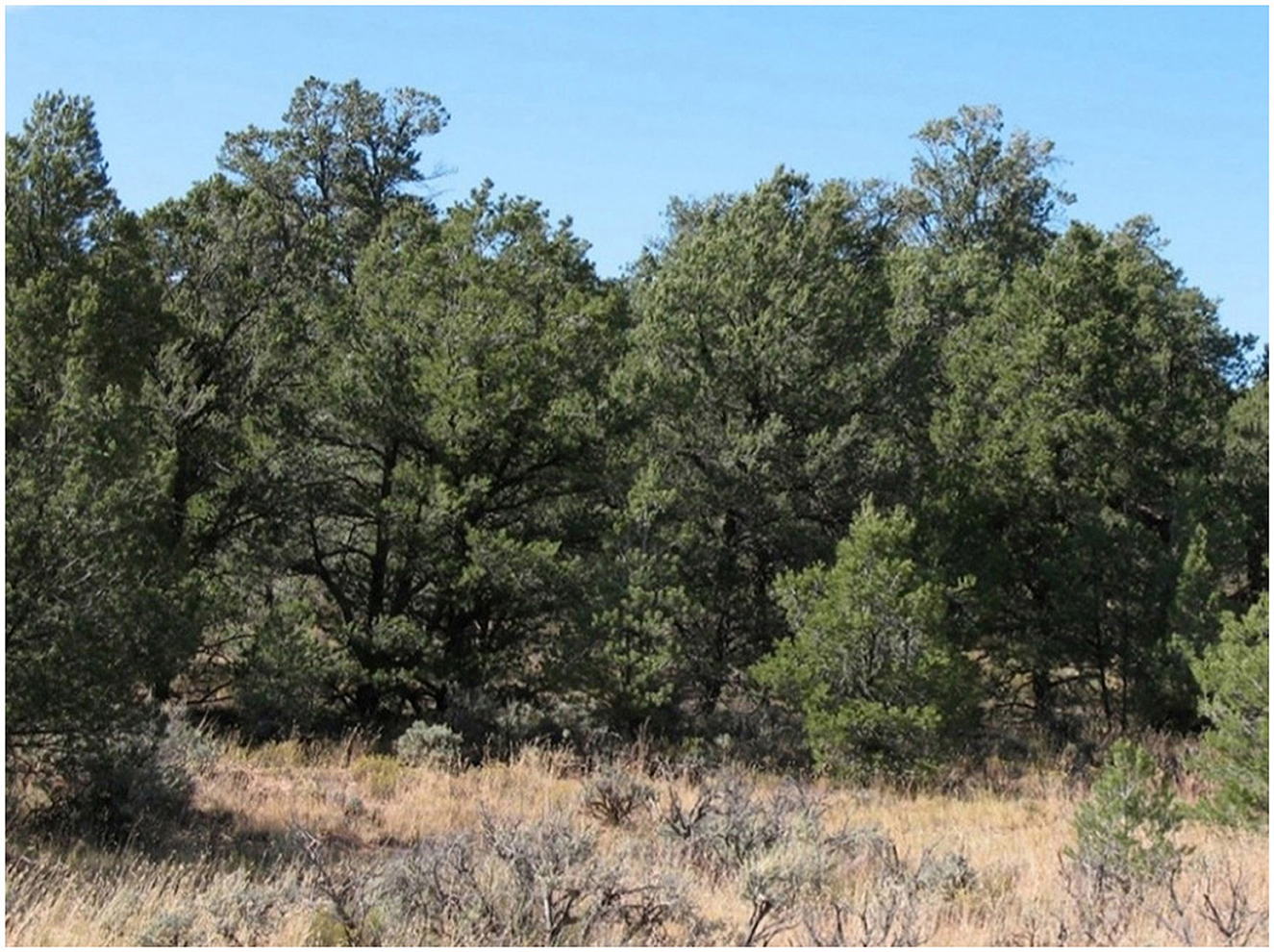
Figure 3. Over-stocked concentration of pinyon and juniper trees showing intermingling of branches and a dense understory, which creates a wildfire-vulnerable fire regime.
3.2 The hidden legacy of anthropogenic fire
One convincing measure of the effects of 20th-century fire suppression and grazing is provided by the results of two eco-system-wide vegetation surveys—one focusing on 1.5 million acres of Kaibab National Forest (Brewer et al., 1991) and the other on the entire Grand Canyon region (Alcoze and Hurteau, 2001). Despite producing allegedly exhaustive lists of plant species, neither survey reported occurrences of fire-following “disturbance” plants (a.k.a. therophytes), such as chenopodium and amaranth, whose seeds and pollen are common in archaeobotanical assemblages of the Grand Canyon area (Merkle, 1962; Sullivan, 2015; see Louderback and Pavlik, 2018 for southern Utah). A strikingly similar pat-tern has been noted by Cuthrell (2013, p. 276) on the central California coast where the abundance of the fire-following annual Phacelia spp. in an archaeobotanical assemblage (AD 1000–1300), attributable to frequent low-intensity cultural burning, is not reflected in modern vegetation communities that have sustained a century of fire suppression. These and other studies (e.g., Nabhan et al., 2004) highlight the effects of fire withdrawal on biodiversity and illustrate how incomplete our understandings are of vegetation histories that do no incorporate archaeological data—a situation best described as the Invisible Pyrophyte Paradox.
3.3 Occupation and land-use patterns through time
Although sporadic human activity in and around the Grand Canyon may have begun as early as 10,000 BC (Smiley, 2017), perennial occupation of the Grand Canyon region started around AD 550/700 (Downum and Vance, 2017; Mink, 2019) but was discontinued no later than AD 1250/1300 (Euler, 1969). This settlement history ensures that no fire-scarred trees (Fulé et al., 2003; Fulé, 2010) are available to track the frequency and extent of anthropogenic fire in the Upper Basin during the height of the area's occupation, roughly AD 900/950-1150/1200 (Sullivan et al., 2002b)—an exemplification of the Fading Record problem in historical ecology (Swetnam et al., 1999).
Protohistoric, ethnohistoric, and modern Indigenous use of the Upper Basin's woodlands by Havasupai and Navajo during the last 150 years is limited to short-term autumnal occupations when pinyon nuts can be gathered (Martin, 1985; Weber and Seaman, 1985; Hough and Brennan, 2008), and to ubiquitous firewood collecting, which entails extensive cutting of pinyon and juniper trees on a nearly continuous basis (Figure 4). Looking farther afield, consideration of J. W. Powell's late 19th century descriptions of Southern Paiute subsistence activities (Fowler and Fowler, 1969) and B. J. Lefler's (Lefler, 2014) study of modern Southern Paiute local ecological knowledge (LEK) yields no cases of fires being set in pinyon-juniper woodlands to produce edible wild plants. Overall, the broader Grand Canyon region—especially the Coconino Plateau and the Upper Basin—lacks specific ethnographic accounts of Indigenous cultural burning for economic purposes that might serve as baseline reference points for archaeological modeling of landscape-scale anthropogenic fire (Cleeland et al., 1990), which is indicative of the No Analog Problem in historical ecology (Swetnam et al., 1999; also, Maxwell and Anschuetz, 1992, p. 38–39).
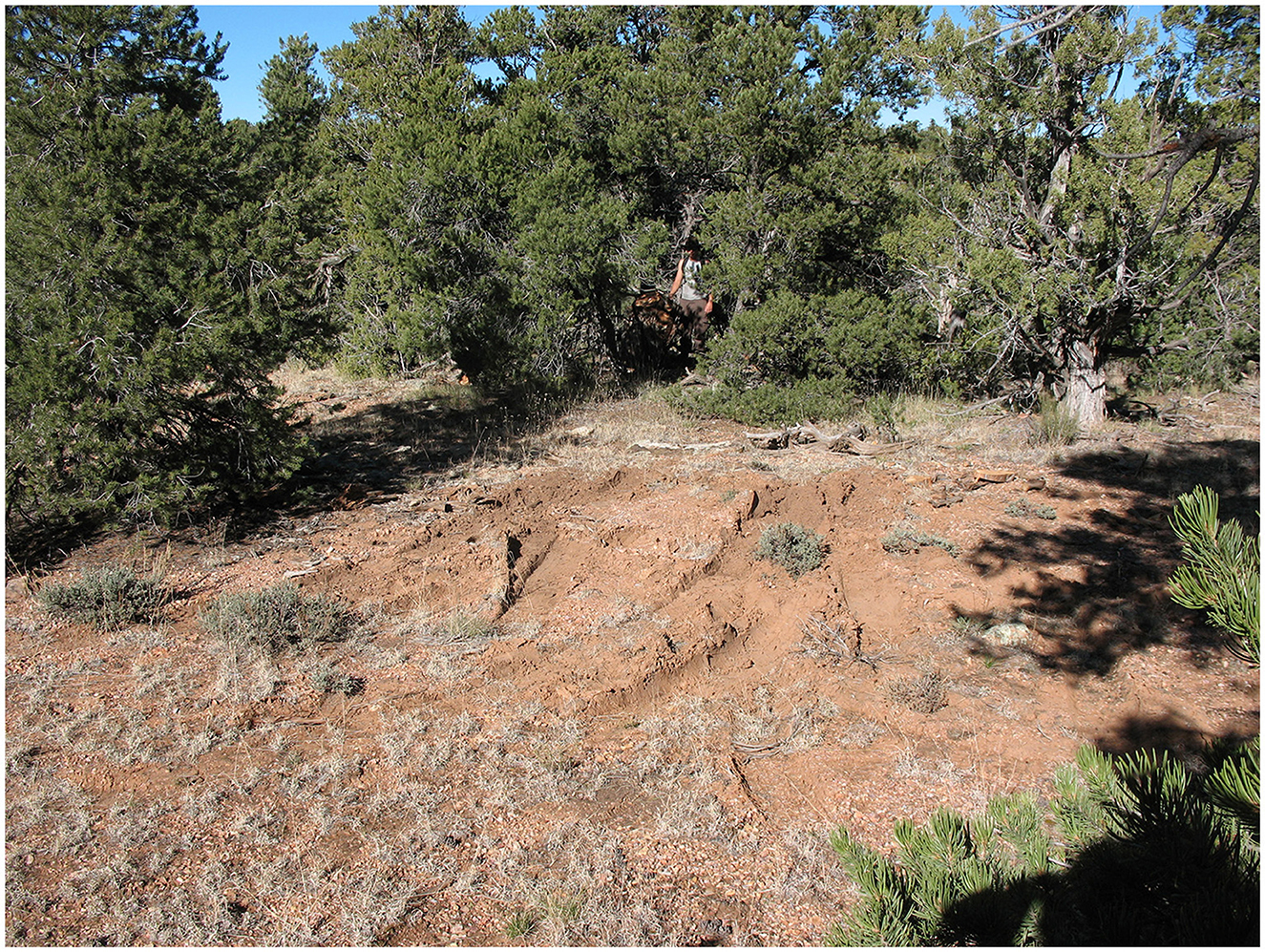
Figure 4. Evidence of recent illegal wood-cutting in Kaibab National Forest showing gullying attributable to unauthorized off-road vehicle traffic, slash from recently cut living trees, and damage to an archaeological site (indicated by the archaeologist gesturing to a site tag on freshly cut tree stump).
3.4 Framework for inquiry
The remedy we propose to overcome the Upper Basin's constraints on fire-based inquiries focuses on exploring the interpretive potential of palimpsests—archaeological phenomena that have systemically different formation histories and artifact accumulation cycles (Bailey, 2007). As noted above, Formation Theory provides a framework to consider how matter comes to be deposited on surfaces and incorporated dynamically into the archaeological record (Sullivan, 2008). This time perspectivism approach enables the development of inferences about cultural burning, particularly when the bulk of feature-derived and surface-occurring matter consists of charcoal, macrobotanical remains, and pollen that originated from the processing of fire-following therophytes that were produced and harvested during the first or “earliest” post-fire successional phase (West, 1984; Bohrer, 1992; Foxx, 1996).
4 Sources of archaeological evidence for economic burning in the Upper Basin
An encouraging development in the application of the time perspectivism approach is that, since 1986, analyses conducted by archaeobotanists and palynologists from different universities (Davis, 1986; Smith, 2003) and research facilities (Scott, 1986; Bozarth, 1992; Huckell, 1992; Cummings and Puseman, 1995, 1997, 2010) have reported that chenopodium and amaranth dominate samples recovered from an assortment of Upper Basin contexts (Sullivan and Mink, 2018). Their widespread prevalence in the following types of archaeological phenomena, which are palimpsests that formed under disparate occupation and abandonment modalities (Sullivan and Ruter, 2006), provides the empirical foundation for our theoretical models that explain patterns of variation in economic burning (D'Andrea et al., 2023).
Multi-room structures [Site 17 (n = 4 rooms) and MU 125 (n = 6 rooms)], occupied continuously for no more than 10 years, have abundant plant-part catchment-traps and artifacts whose contents or enveloping sediments represent long-term (e.g., post holes), short-term (e.g., storage vessels), or last-use (e.g., hearths) accumulation cycles (van der Veen, 2007; Berkebile, 2014). These abandoned perennial habitations are characterized, as well, by dense concentrations of artifacts on structure floors and in exterior locations, and disclose on-site artifact-production facilities (Sullivan, 1988; Fugate, 2004).
In contrast, single-room structures [MU 38.1 (Becher, 1992); SRI 15 and SRI 24 (Whittlesey, 1992); GC 105 (Downum, 2010)] appear to have been used infrequently and reoccupied only on a seasonal basis (bi-monthly to multi-monthly, at most). Consequently, they have few plant-part catchment-traps, few artifacts on structure floors, and are accompanied by low to moderately dense artifact concentrations in exterior areas. Limited lithic and ceramic artifact production appears to have occurred episodically on-site and occasionally involved a dedicated feature (Sullivan et al., 1995), while artifact assemblage formation relied on low-level replenishment/restocking of ceramics and recycled ground-stone artifacts from nearby, off-site sources.
Fire-cracked-rock piles [MU 121, MU123.1, MU 235, MU 236 (Sullivan et al., 2001)], unaccompanied by structures of any kind, are characterized by very short-term occupations (daily to sub-weekly), typified by multiple, episodic accumulation cycles that involved intensive lithic-artifact production (Purtill, 1995), as well as significant provisioning and recycling of ground-stone artifacts (manos and metates) and ceramics from neighboring locations. Plant-part catchment-traps are rare; most samples were recovered from sediments lying beneath arrays of processing artifacts and from sediments surrounding dense, ashy, charcoal-rich concentrations of fire-cracked rock and burned surfaces (Cook, 1995).
Alignments of rocks placed on sloping surfaces [SRI 24 (Homburg, 1992); MU 125.1 (Sullivan, 2000)], presumably related to soil management, were characterized by unintensive, discontinuous seasonal (bi-monthly to multi-monthly) use histories that involved no artifact production and scant evidence of artifact accumulation cycles. No plant-part catchment-traps accompany these alignments; pollen samples were recovered from strata that had accumulated behind and upslope from the rock alignments.
As synthesized recently (Sullivan and Mink, 2018), the results of archaeobotanical and palynological analyses of samples recovered from sealed surfaces and features in burned and unburned structures, from bulk sediment samples obtained from occupation surfaces, from buried soil horizons in alluvial fans, and from exposed (open-air) artifact arrays, are unified in two respects – (i) they are dominated numerically by seeds and pollen from fire-responsive chenopodium and amaranth plants (cf. Carter et al., 2021, p. 4) and (ii) they contain few, if any, fire-intolerant domesticated plant remains, such as maize (Sullivan, 1987, 1992). It is no coincidence that, with the exception of the largely artifact-free soil management features, these archaeological phenomena are accompanied by numerous whole and fragmentary metates and manos [representing all types (basin, slab, and trough) and materials (sandstone, limestone, quartzite, and basalt); for ethnographic and ethnoarchaeological examples, see Fowler and Fowler (1969, p. 23) and Euler and Dobyns (1983), respectively] and thin sandstone “grinding slabs” that are indicative of small-seed and nut processing (Yoder et al., 2010; see also Mueller-Bieniek et al., 2018, p. 10).
5 Expanding archaeological evidence of economic burning in the Upper Basin
To gauge the reliability of our principal inference that low-intensity anthropogenic burning enabled the production of edible ruderals, two additional investigations were conducted recently—one involving a newly identified type of terrace complex, which consists of 14 “cut-back” terraces (Table 1; Figure 5A), and another featuring sedimentary sequences. In one respect, the cut-back terraces are similar to previously reported terrace complexes in the Upper Basin because they have no evidence of artifact production or accumulation, but are profoundly dissimilar in view of their manner of construction (Sullivan, 2000). That is, instead of the terrace builders placing rocks on a sloping surface to impound sediments entrained in slopewash (Homburg, 1992), we hypothesize that this newly identified type of terrace complex was created by people removing rocks from an unconsolidated bedrock slope—hence, the term “cut back”—thereby creating flat, linear surfaces that are arranged perpendicular to the slope's gradual pitch (Figure 5B; cf. Brown et al., 2023, p. 12–13).
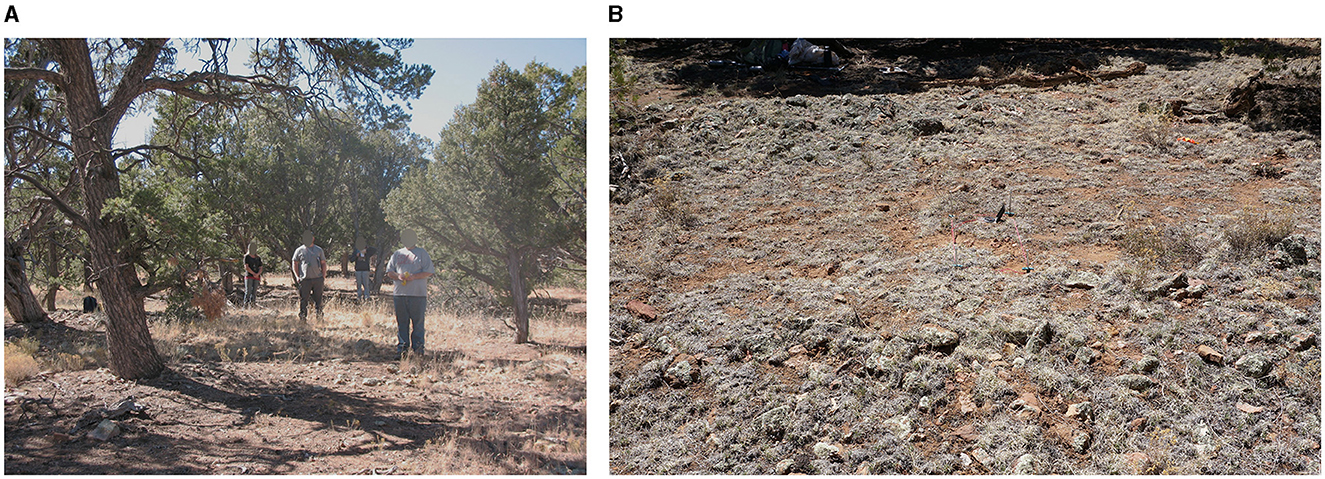
Figure 5. (A) Upslope view of archaeologists standing on the surfaces of four cut-back terraces in Grand Canyon National Park (bearded archaeologist [lower right] is standing on Terrace MU 1471 where test-trench excavations were conducted); (B) downslope view showing cut-back terrace surface (center, with field compass and staked test-trench [1.0 m × 0.5 m] in Terrace MU 1464) situated between two unconsolidated bedrock berms (foreground and top).
Test units (1.0 m × 0.5 m × 0.3–0.4 m) were placed in four terraces (Table 1) to reveal the nature of the contacts between adjoining surfaces (i.e., between a terrace surface and the face of the nearest upslope terrace; Figure 6) and to facilitate stratigraphic sampling of macrobotanical remains and pollen. A control unit was excavated on level ground at the top of the bedrock slope to ascertain natural depositional processes; pollen and macrobotanical samples were recovered from different soil horizons there, as well.
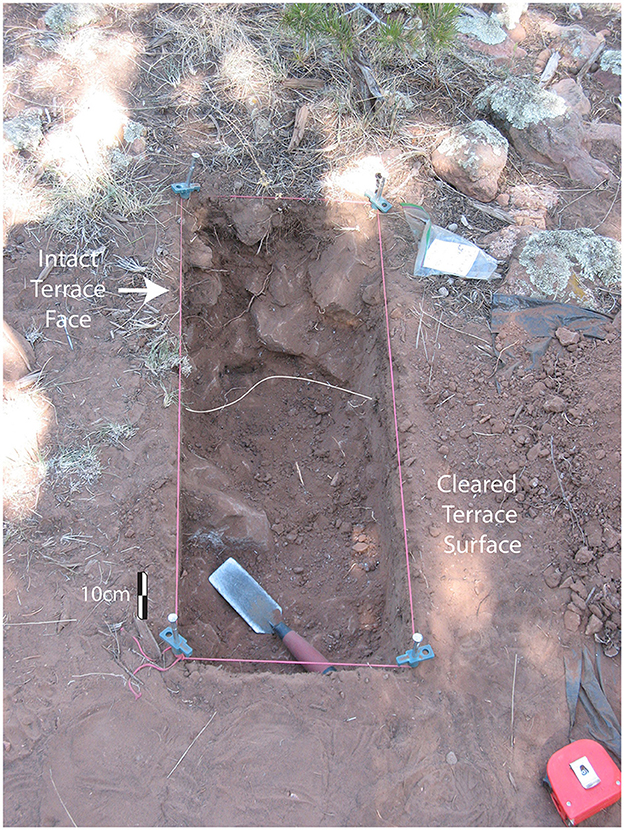
Figure 6. Test trench (1.0 m × 0.5 m × 0.3–0.4 m) excavated through the surface of Terrace MU 1464 showing the contact between the terrace's largely rock-free surface, from which rocks had been removed by people in the past, and the intact face of the next upslope terrace showing in situ rocks.
To determine the potential of the Upper Basin's sedimentary sequences for yielding evidence of burning, 14 auger cores were extracted from four areas (Figure 7). Coring locations were chosen to sample sediments from the highest to the lowest points in the basin-wide drainage system (i.e., from the South Rim to Lee Canyon, respectively), distributed among concentrations of masonry ruins and fire-cracked-rock piles (Coring Areas 1-3), and in an area devoid of known archaeological sites (Coring Area 4 along Lee Canyon near the base of the Coconino Rim). Although auger depth and sediment characteristics varied, evidence of cumulic soil development was present in 12 of 14 augers (Table 2). Variation in soil properties was recorded in the field by 10 cm-drives within each auger core; soil samples, which infrequently contained visible macrocharcoal fragments (6 of 14 augers; 34 of 150 drives) that are important indicators for understanding variation in fire regimes, were recovered from each auger drive.
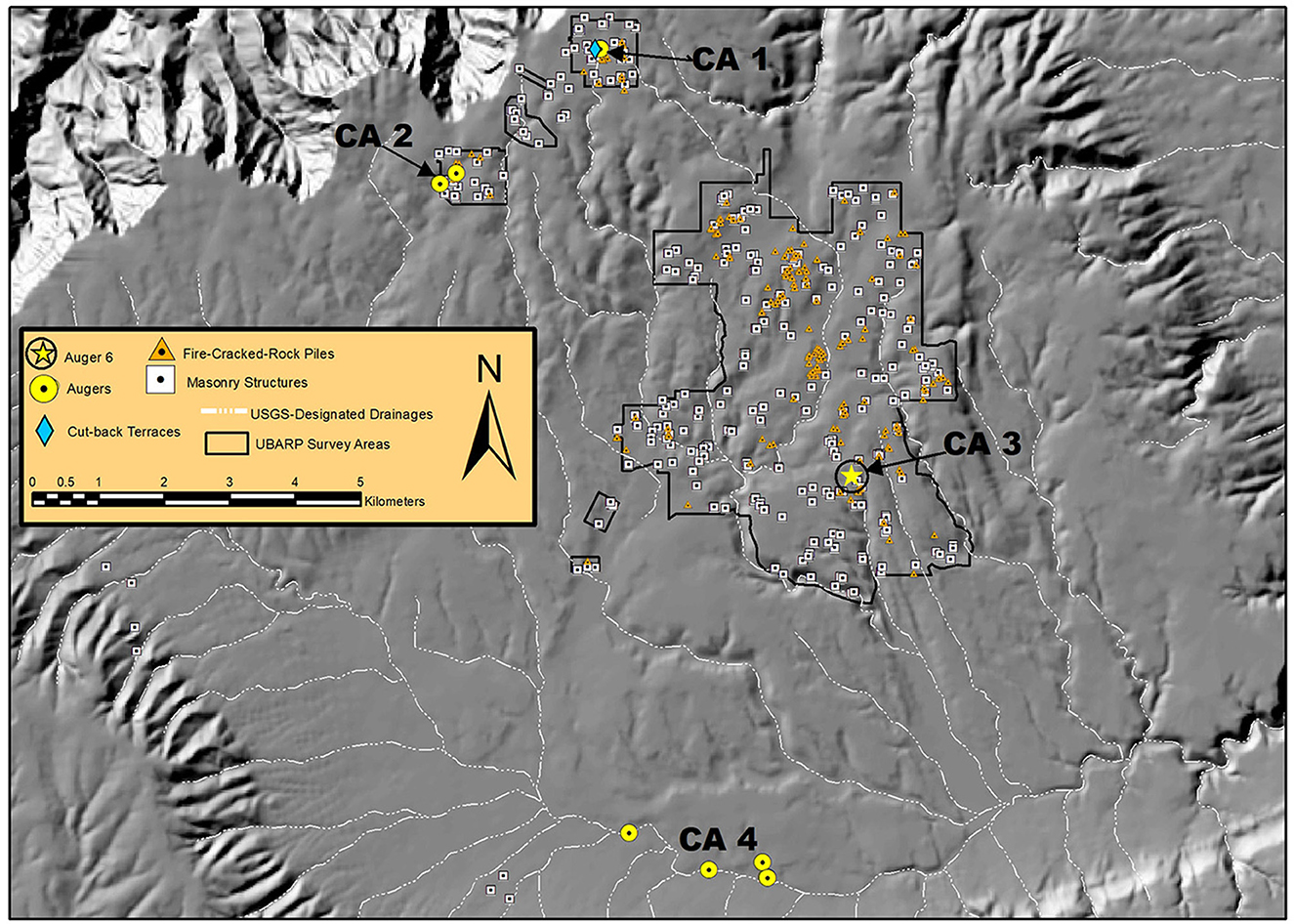
Figure 7. Map of the Upper Basin showing locations of Coring Areas (CA 1–4) and auger holes, cut-back terraces and control unit, masonry structures, and fire-cracked-rock piles.
6 Results of the test-trenching program
6.1 Stratigraphic evidence
Horizons identified in the four terrace test-trenches revealed that the terraces are fairly uniform stratigraphically despite minor differences in strata thicknesses. The first 5–10 cm below surface are characterized by soft, loose, silty sand deposits that contain few, if any, rocks, and no ceramic, flaked stone, or ground stone artifacts (Figure 8). As depth below surface increases, so does soil compaction, and rock size and frequency (Merkle, 1952, p. 377). Unexpectedly, a feature (Feature 1) was discovered in Terrace MU 1471 that consisted of two 10 cm deep, ash-filled postholes accompanied by partially burned fragments of rotted juniper wood intruded through the floor of a post-hole trench (Figure 8).
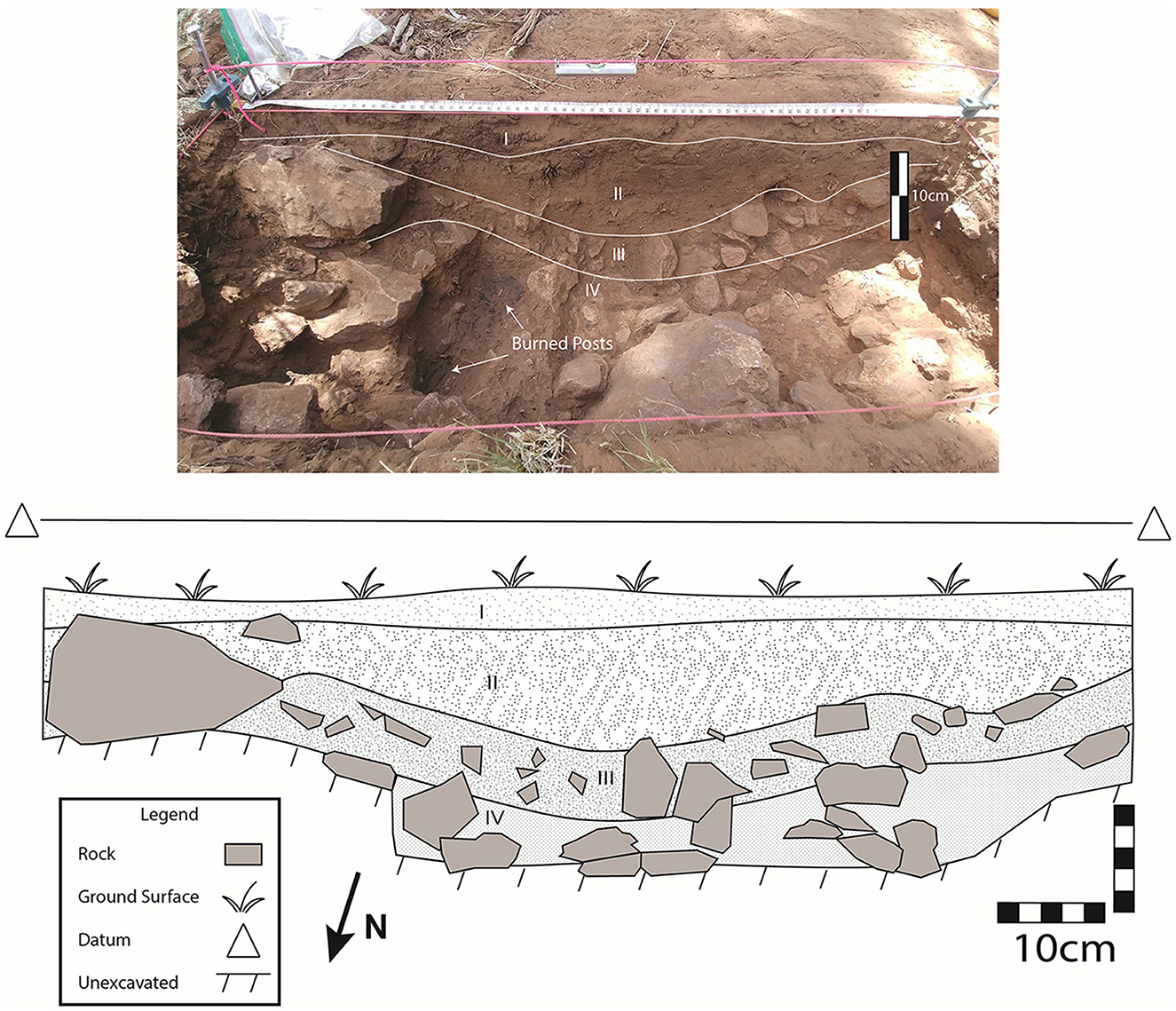
Figure 8. Profile views of test trench in Terrace MU 1471 showing rock-free zone (Strata I and II) of accumulated fine-grained, silty sand sediments (center, below tape measure), large rocks of adjoining downslope terrace (left center), and post-hole trench intruded through large rocks (left center) with burned remains of two posts in 10 cm-deep postholes.
The stratigraphy of the control test-trench differs importantly from the terrace units because of the presence of large rocks at the surface and in subsurface positions, and by thick (>10 cm) and heavily-compacted clayey sand that increased in hardness with depth (Figure 9). These findings are consistent with those reported for non-anthropogenic stratigraphic contexts in the Upper Basin and elsewhere in the Grand Canyon (Rand, 1965, p. 88).
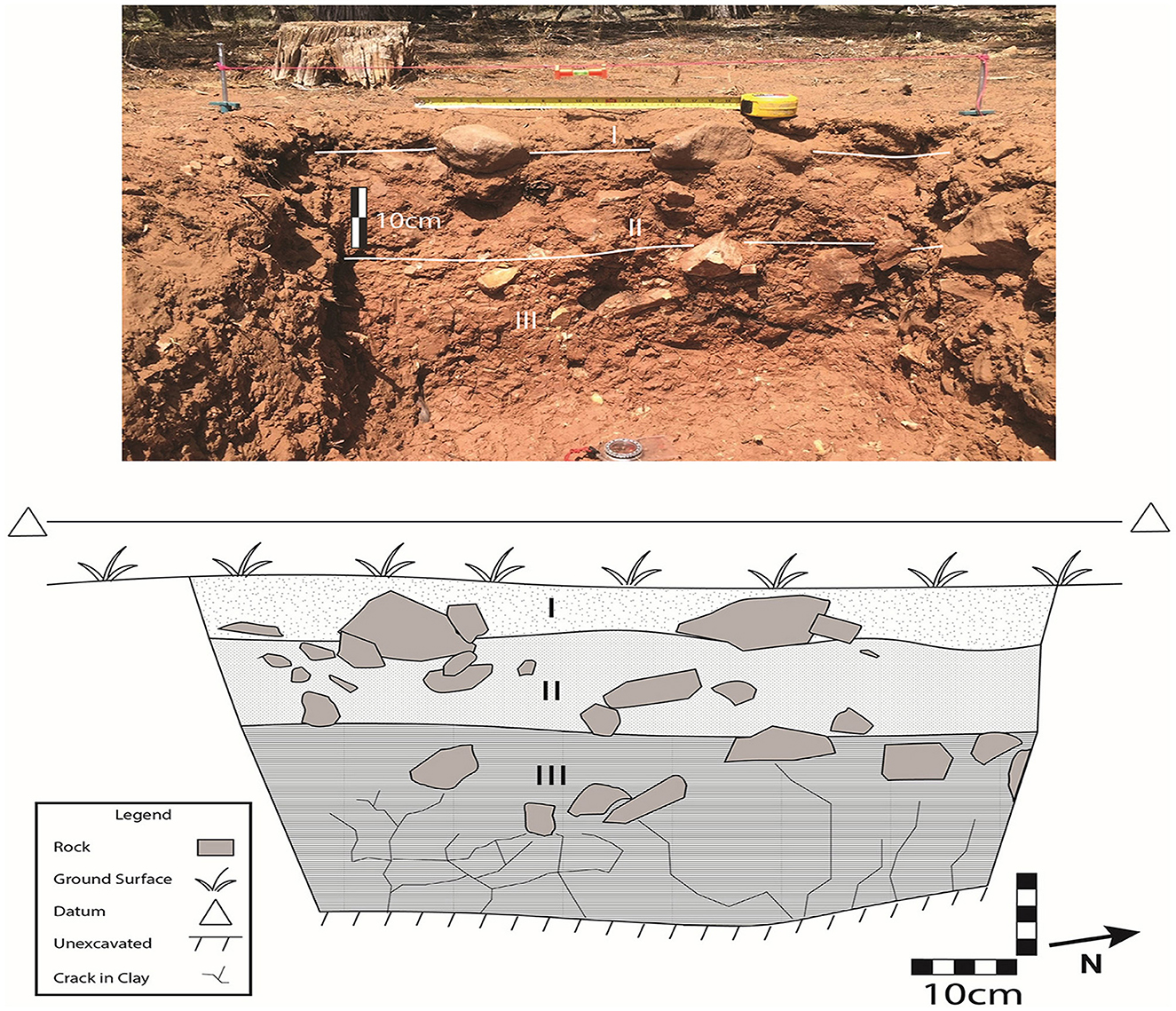
Figure 9. Profile views of control test-trench. Note the large rocks that range from the surface to the bottom of the exposure (Strata I-III). The tape is extended 50 cm.
Together, the stratigraphic and content differences between the terrace test-trenches and the control test-trench support the hypothesis that rocks were cleared from the unconsolidated bedrock slope on which they originally reposed and were deliberately placed aside to produce level surfaces of highly varying length (coefficient of variation = 73.2%) but tightly constrained width (coefficient of variation = 12.5%). Although the intentions of the people who created these surfaces are unclear, it is reasonable to conclude that the cut-back terraces may have served as stable landforms from which to concentrate the production or processing of economic plants (details below). Because of the limited exposure of Feature 1 in Terrace MU 1471, hypotheses regarding the circum-stances that led to its origination are decidedly speculative. That said, the feature may represent the remains of a double-post support for an unroofed shade or a small enclosure similar to those reported in 19th century Hopi agricultural fields (Mindeleff, 1891, p. 217–218). It is worth noting that one other alignment complex in the Upper Basin has disclosed surface indications of a small enclosure (MU 4030) situated on the surface of a cut-back terrace (MU 4029).
6.2 Archaeobotanical evidence
The two uppermost strata in the test trenches for Terraces MU 1464, MU 1466, and MU 1471 yielded highly variable quantities of chenopodium seeds (n = 45, n = 306, and n = 413 seeds, respectively per 0.25 liter; Table 3) accompanied by low numbers of seeds from several miscellaneous economic plants [Fabaceae; Portulaca; Polygonum; and Mammillaria (Cummings, 1994; Springer et al., 2009, p. 136–138; Ford, 2000, p. 217)]. In contrast, Terrace MU 1470 yielded just one economic plant seed (Polygonum), whereas the control test-trench yielded only 12 chenopodium seeds.
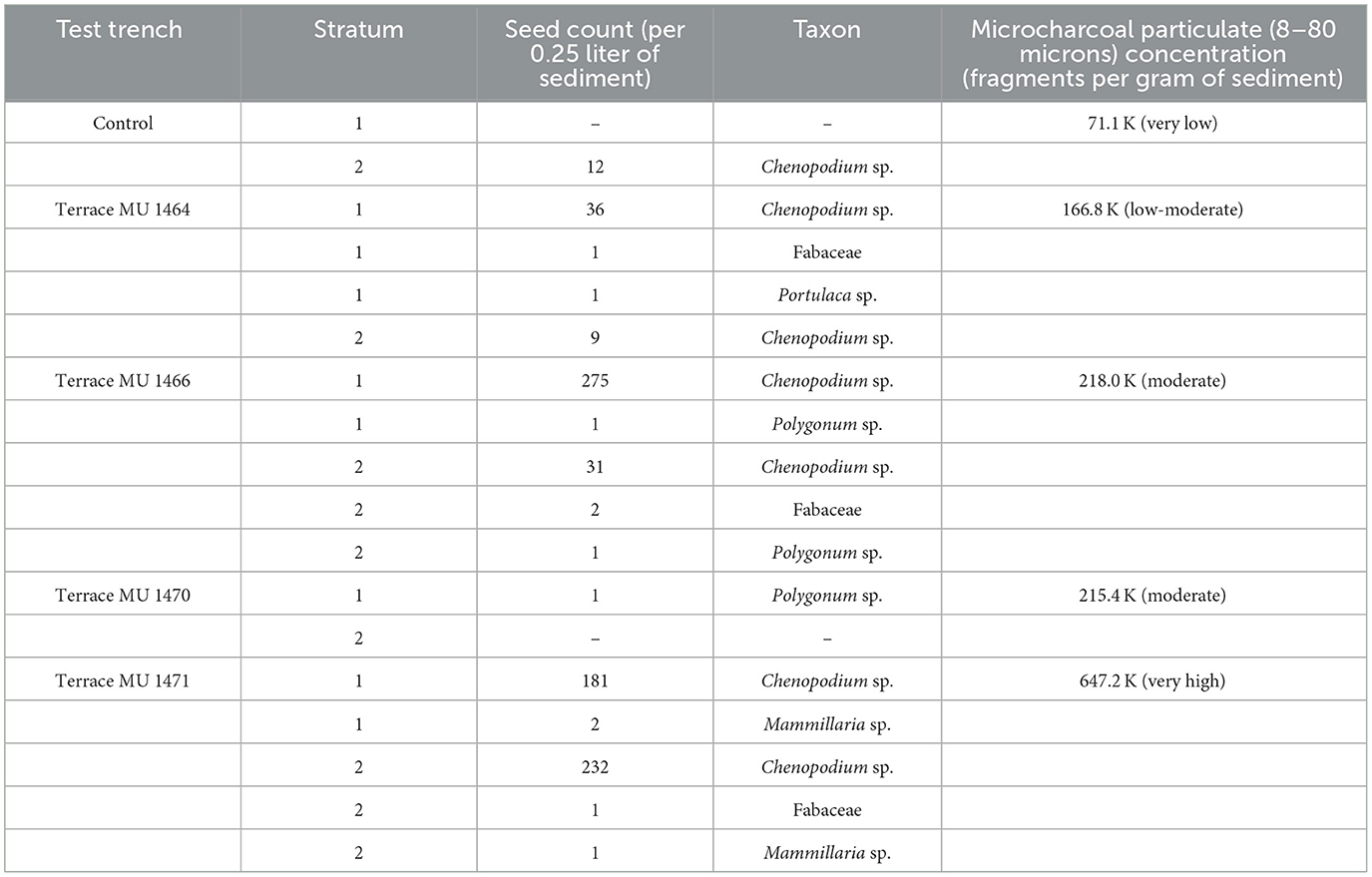
Table 3. Frequencies of identifiable seeds from economically-significant ruderal plants and of microcharcoal particulate concentrations for control and terrace test-trenches.
Results of the palynological analysis of samples recovered from the uppermost stratum of the four terrace test-trenches and the control test-trench (ranging 2–8 cm below ground surface) align with the aforementioned macrobotanical data (Jones, 2019). Because pollen preservation was excellent across samples, with concentrations ranging between 3.9 k and 104.7 k grains per gram of sediment (Jones, 2019, p. 6), the absence of domesticated plant pollen is particularly noteworthy because it reinforces findings and interpretations obtained from other excavated contexts in the Upper Basin noted earlier (Sullivan and Forste, 2014). Excluding arboreal pollen, which dominates all pollen assemblages, and numerically inconsequential “background” herbaceous taxa pollen, the samples have elevated frequencies of nonarboreal pollen from the disturbance-responsive Amaranthceae family, which includes chenopodium. The only exception to this generalization is the sample from Terrace MU 1471, which disclosed no cheno-am pollen even though its macrobotanical assemblage is dominated heavily by chenopodium seeds. One explanation for this discrepancy is that a “very local burning event” consumed new growth, including undispersed pollen still clinging to the plants, on the terrace surface but did not incinerate earlier deposited and protected seeds below the ground's surface (Jones, 2019, p. 8; also, Reed, 2021, p. 16).
7 Results of the augering program
Soilscapes in this part of northern Arizona support numerous classifications (Hendricks, 1985; Brewer et al., 1991) but Homburg's (1992) designation of Upper Basin soils in the vicinity of alignment complex SRI 24 as Cumulic Haplustolls covers the range of pedological variation revealed among auger cores (e.g., see Table 4 for details of Auger 6). Importantly, six augers contained visible macrocharcoal fragments that were associated with periods of cumulic soil development (Table 2). When present, concentrations of small- to medium-sized fragments of charcoal (< 1 mm−4 mm) imply transport and deposition from nearby sources via alluvial or colluvial processes (cf. French et al., 2009, p. 669). In a few drives, larger charcoal fragments (>4 mm) were encountered, suggesting burning in-situ or near the auger location (cf. Herring et al., 2014).
8 Regional synchroneity of economic burning
Burning practices related to edible-resource production and processing are reflected in broad temporal patterns of calibrated radiocarbon dates that crosscut the formation histories of the Upper Basin's highly variable palimpsests (Table 5). An AMS determination of AD 541-602 (AA-112248), for instance, places the origin of microcharcoal recovered from Auger 6 (Drive 10) at the beginning of sustained human activity in the Upper Basin. This date range overlaps slightly with that of an early activity surface at MU 235 dedicated to seed and nut processing (AD 420-556; Pitt-1060), but aligns more completely with a date range of AD 549-757 (AA-17934) obtained from charcoal recovered from a fire-cracked-rock pile (MU 121).
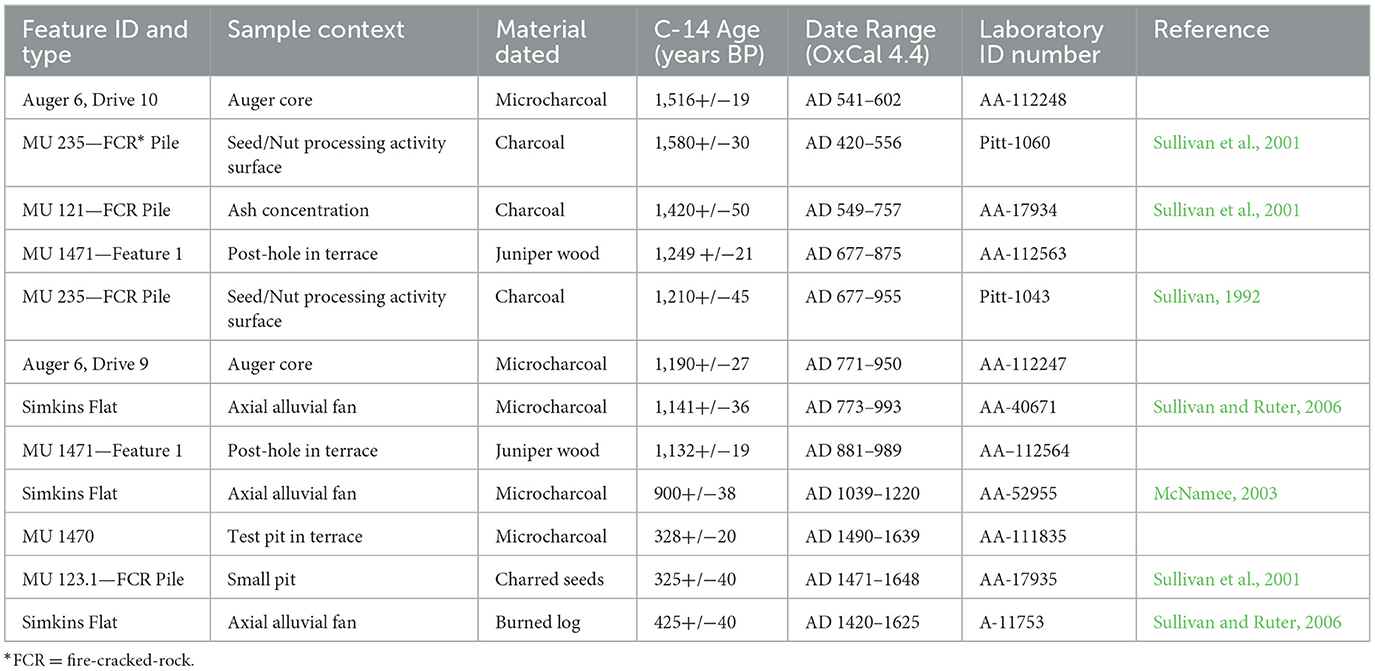
Table 5. Feature types, sample contexts, material dated, C-14 ages, two-sigma (95.4%) calibrated date ranges, and laboratory identification numbers for radiocarbon-dated samples from the Upper Basin.
The juniper wood samples recovered from Feature 1 at Terrace MU 1471 were submitted to the Laboratory of Tree-Ring Research at the University of Arizona but could not be dated because of their deteriorated condition. AMS determinations on the same wood samples returned date ranges of AD 677-875 (AA-112563) and AD 881-989 (AA-112564). Because both adjoining wood samples originate from the same surface, the second date range (AD 881–989) likely indicates the construction period of Terrace MU 1471, with the slightly earlier date range representing the use of dead wood—a common practice in the Upper Basin (Whittlesey, 1992).
Both wood sample date-ranges overlap significantly with a conventional radiocarbon date range of AD 677–955 (Pitt-1043) obtained from charcoal recovered from a seed and nut processing activity-surface adjacent to a fire-cracked-rock pile (MU 235; Sullivan et al., 2001, p. 370). They also overlap partially with an AMS-determined date range of AD 773–993 (AA-40671) returned for microcharcoal recovered from an axial alluvial fan (Simkins Flat; Sullivan and Ruter, 2006, p. 199), and another of AD 771-950 (AA-112247) based on microcharcoal recovered from Auger 6 (Drive 9).
A microcharcoal sample recovered from Terrace MU 1470 yielded an AMS-determined date range of AD 1490–1639, which overlaps largely with a conventional radiocarbon determination of AD 1471–1648 (AA-17935) that was obtained from charred seeds recovered from a pit on the edge of a fire-cracked-rock pile (MU 123.1; Sullivan et al., 2001, p. 370). It also overlaps partially with a date range of AD 1420–1625 (A-11753) returned from a buried and partially burned log discovered in the axial alluvial fan (Simkins Flat) noted above (Sullivan and Ruter, 2006).
Despite the likelihood that dead wood was used for fuel and construction material, we suspect that much of the dated material recovered from the terrace test-trenches, Auger 6, and previously investigated contexts brackets the range of economic burning practices during the bulk of the radiocarbon-determined span of human occupation of the Upper Basin, which is no earlier than AD 420–556 and no later than AD 1471–1648 (Table 5). Although additional quantification of charcoal accumulation rates would provide a more fine-grained picture of variation in the frequency and impacts of natural and anthropogenic burning events, the totality of current evidence, especially robust co-occurrences of charcoal and ruderal archaeobotanical remains recovered from a variety of contexts, strongly indicates anthropogenic manipulation of the Upper Basin's fire regimes for food-production purposes. In contrast, some other contexts examined here, such as the uppermost drives of the auger cores and the uppermost stratum of the control test-trench, which do not correspond to the dated occupation span of the area, are devoid of economic ruderal pollen or seeds, yield little or no charcoal, and disclose very low concentrations of microcharcoal particulates, likely arose in circumstances that did not involve frequent, low-intensity fires and intentional ruderal production (D'Andrea et al., 2023). Collectively, and importantly, these findings provide a foundation for modeling variation in ancient human pyrogeography, as follows.
9 Modeling variation of economic burning practices
Here, we illustrate the role of Formation Theory and the value of time perspectivism in providing plausible explanations for how evidence of anthropogenic fire is expressed in the archaeological record. First, as noted above, no pollen or macrobotanical remains of domesticated plants were identified; with the exception of Terrace MU 1470, all samples are dominated by chenopodium seeds, and in the case of pollen, by cheno-am and low-spine Asteraceae [these plants are all indicative of disturbance (King, 1994, p. 200–201) in post-fire settings (Damick et al., 2022, p. 9)]. These findings suggest that differences among samples are attributable to variation in formation histories of the terraces. For example, Terrace MU 1470 may have been built but rarely used, if it was used at all. A similar pattern has been inferred for structures in the Upper Basin (Sullivan, 2008), and for alignments and terraces at nearby Wupatki National Monument (Sullivan and Downum, 1991), that disclose few if any surface artifacts. Similarly, Terrace 1464 may have been used briefly, perhaps only once. In contrast, Terraces 1466 and 1471, which were heavily used as measured by the high frequencies of seeds identified in their samples, appear to have different formation histories, with the former having the bulk of its seeds recovered from Stratum 1 (near-surface context) whereas the latter terrace's seeds were nearly equally divided between Stratum 1 (near-surface context) and Stratum 2 (subsurface context).
Second, it is worth reiterating that all the plants in Table 3 are therophytes—disturbance-related economic ruderals that have been recovered in abundance in disparate prehistoric contexts around the Grand Canyon province (Balsom et al., 2017, p. 223). Whether their ubiquity is attributable to natural disturbances in the Upper Basin, such as wildfires that create openings in overstocked stands, or floods that create fresh exposures in alluvial flats, or winds that uproot trees and create basins for sediment accumulation and subsequent seed germination (Karkanas and Goldberg, 2019), or to anthropogenic burning, these plants are “obligate initial community species” (United States Department of Agriculture Fire Effects Information System., 2023), which bestows competitive advantages on their survivability and spread. Hence, it is not surprising that these species register collectively as highly variable assemblages of macrobotanical and microcharcoal remains that arose from differences in fuel loads, ignition sources, and time since the last burning event (Mellars, 1976, p. 28–29, 35).
With these factors in mind (Table 3), we advance the following models to account for the range of variability of cultural burning, and its archaeological consequences, in the Upper Basin.
Model 1: stratigraphically discontinuous occurrences of low amounts of microcharcoal unaccompanied by ruderal seeds are indicative of charcoal transport from naturally-ignited fires (e.g., Auger 6);
Model 2: low frequencies of ruderal seeds accompanied by exceptionally low amounts of microcharcoal are indicative of unintended/incomplete combustion that occurs at the edges of or beyond the perimeters of economic burn patches (e.g., Control Test Trench);
Model 3: stratigraphically continuous occurrences of moderate amounts of microcharcoal accompanied by very few ruderal seeds are indicative of largely unproductive economic burning (e.g., Terrace MU 1470);
Model 4: stratigraphically discontinuous occurrences of low to moderate amounts of microcharcoal accompanied by low numbers of ruderal seeds are indicative of sporadic economic burning (> 6 years; e.g., Terrace MU 1464; Pyne, 2021b, p. 71);
Model 5: stratigraphically continuous occurrences of moderate amounts of microcharcoal accompanied by high numbers of ruderal seeds are indicative of repeated economic burning at low-frequency rotation intervals (ca. 4–6 years; e.g., Terrace MU 1466; Roos et al., 2023; Springer et al., 2024, p. 29);
Model 6: stratigraphically continuous occurrences of extremely high amounts of microcharcoal accompanied by high numbers of ruderal seeds are indicative of sustained economic burning at high-frequency rotation intervals (ca. 1–3 years; e.g., Terrace MU 1471; Coughlan, 2013, p. 95; Wu et al., 2023, p. 3).
These proposed archaeological outcomes of anthropogenic patch-burning make sense ecologically because landscape fires do not yield symmetrical patches of burned material (Damick et al., 2022). To the contrary, the extent and boundaries of cultural burning are difficult to control (Steward, 2018) and, consequently, produce highly irregular spatial distributions of burned vegetation and scorched earth accompanied by heterogeneously-sized exposures of ash and charcoal—in short, spatial jumbles of heavily, lightly, and unburned areas emerge during the same cultural burning event (also, Roos et al., 2010, p. 158–159; Bates and Davies, 2016).
10 Time, economic burning, and the ruderal seed-bed hypothesis
The widespread and long-term synergy between anthropogenic fire and ruderal production that we infer is recorded in the Upper Basin's archaeological and archaeobotanical records is reflected in the successional aftermaths of several 21st century lightning-caused fires. These conflagrations, of varying severity and coverage (Sullivan and Mink, 2018, p. 630), activated near-surface accumulations of dormant ruderal seeds, resulting in pyrophyte “blooms” (or “flourishes”) that grow on and around archaeological sites. For example, ruderal pyrophytes, such as fetid goosefoot (chenopodium), amaranth, and tobacco sprouted more than a year after the Scott Fire torched 1,076.5 ha of the Upper Basin's pinyon-juniper woodland in 2016 (Figure 10). This phenomenon is not an isolated occurrence as similar post-fire ruderal blooms have been recorded on several archaeological sites in ponderosa pine forests southwest of the Upper Basin near Sitgreaves Mountain in northern Arizona (Figure 11; cf. Yarnell, 1965, p. 668–669).
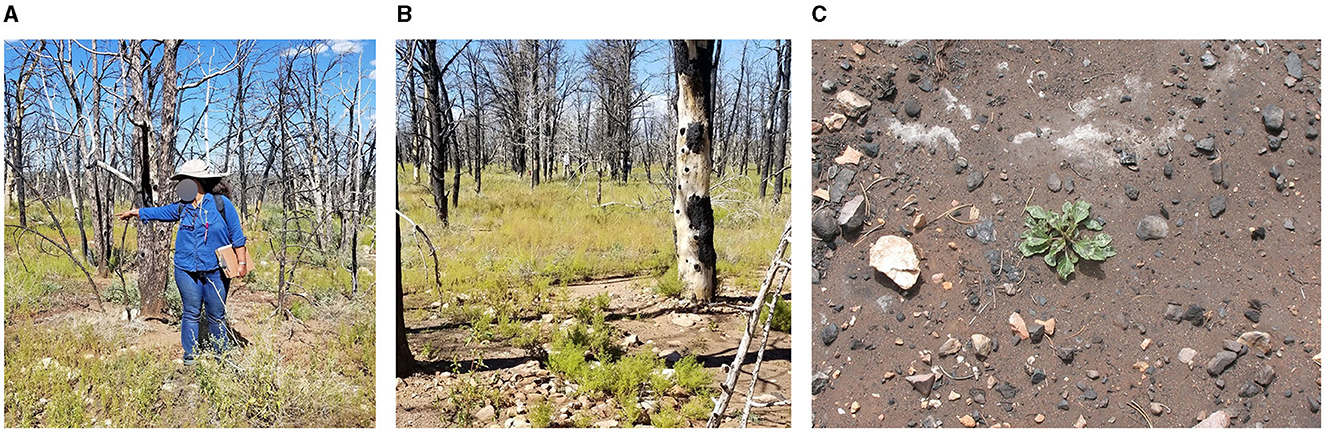
Figure 10. Expanses of fire-following ruderals 1 year after the 2016 Scott Fire in the Upper Basin, Kaibab National Forest: (A) tobacco sprouts (in front of the gesturing archaeologist; image and botanical identification courtesy of N. Weintraub); (B) fetid goosefoot (chenopodium; image and botanical identification courtesy of N. Weintraub); (C) amaranth sprout (image by A. P. Sullivan; botanical identification by J. D. Springer).
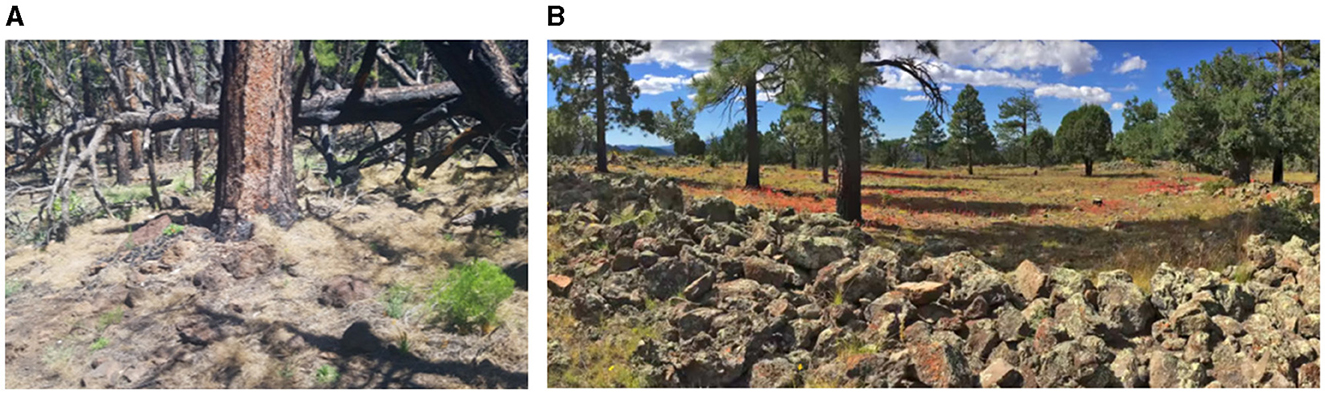
Figure 11. Fire-following fetid goosefoot (chenopodium) growing on and around archaeological sites: (A) 1 year after a wildfire on Sitgreaves Mountain, Kaibab National Forest (2014); (B) 1 year after a prescribed fire on Walavudu, Kaibab National Forest (2017); images and botanical identifications, courtesy of N. Weintraub.
The aforementioned data and interpretations provide the evidential foundation for the ruderal seed-bed hypothesis (Smith, 1998). Essentially, we propose that the repeated application of low-intensity anthropogenic fire to understory vegetation results in the establishment of self-perpetuating, viable seed beds of pyrophytic ruderals, such as chenopodium and amaranth (Abella and Springer, 2008). In this model, humans control the location and extent of food production activities that depend on fuel-load management rather than on uncontrollable factors, such as poor soil conditions or erratic rainfall amounts that plague corn farming, which are widespread in the Upper Basin today (Sullivan, 2015).
With regard to ecological dynamics, low-intensity burning of understory vegetation has six predictable effects: it reduces com-petition from non-economic ruderals, activates the germination cycle of the near-surface seed bed (Reed, 2021, p. 3), maintains the growing temperature of the seed bed by changing the surface albedo from reflective to absorptive, releases nutrients into the seed bed (Lewis, 1972, p. 199), enhances photosynthesis by thinning the canopy (Lefler, 2014, p. 70–71; Pyne, 1998, p. 77), and with repeated ignitions makes “the pinyon-juniper woodlands look like patchy savannas” (Bonnicksen, 2000, p. 239; also Romme et al., 2009). By encouraging the production of pyrophytes and their prodigious amounts of seeds (Turner et al., 2021), humans confer a selective advantage upon these fire-adapted ruderals (e.g., Cuthrell, 2013, p. 277; Mueller-Bieniek et al., 2019, p. 8), which goes far to explain their widespread distribution across the greater Southwest (Fritz et al., 2017). And, because of their abundance and longevity (Mueller-Bieniek et al., 2018; Bisbing et al., 2023; upwards of 50 years), long-dormant seeds of fire-following plants can be activated by lightning-caused fires that occur long after humans have abandoned the archaeological landscapes that shelter them (Springer et al., 2024, p. 26).
11 Summary and conclusions
Archaeological and geoarchaeological investigations have revealed that macrobotanical and pollen assemblages recovered from a variety of accumulation contexts in the Upper Basin are dominated by disturbance-based, fire-following [“fire-stimulated” (Nabhan et al., 2004, p. 17–21)] ruderals. These assemblages, largely devoid of domesticated plant remains, support the hypothesis that the prehistoric occupants of the Upper Basin regularly ignited and managed “food fires,” which constitute a sustainable food-production practice that has no ethnographic referent in the northern American Southwest. From the perspective of long-term ecosystem dynamics, the effects of these fire foodways still register in the region as “ruderal blooms” once fire is reintroduced to the landscape. This pattern means that after ruderal seed beds had been established in and around settlements and activity areas prehistorically, presumably by the application of fire to understory plant communities, they persisted long afterwards (Wilke et al., 1972, p. 206).
The prevalence of pyrophytic ruderals in assemblages formed under a variety of accumulation circumstances cannot be explained by sampling bias or preservation variability. To the contrary, the economic centrality of these plants in prehistoric food-production systems attests to persistent understory burning. Importantly, members of the Amaranthceae and Asteraceae families, in particular (Springer et al., 2024, p. 32), produce abundant seeds and leaves that are highly nutritious (James, 2009) and can be produced in conditions that are unfavorable to maize cultivation (Sullivan et al., 2015, p. 49). In essence, anthropogenic fire in the service of ruderal production made pinyon-juniper woodlands widely habitable (Sullivan and Forste, 2014).
With these understandings, our emerging model of the behavioral-ecological dynamics associated with the establishment of ruderal seed-beds hardly differs from those proposed by fire anthropologist Henry T. Lewis (1972) and archaeobotanist Bruce D. Smith (1998) for wheat domestication in the Near East. That is, the repeated application of fire to understory vegetation increases the density of nutritious seed- and leaf-bearing ruderals, thereby favorably altering the selective pressures that influence their growth and reproduction (Oryokot et al., 1997). Additionally, those seeds not captured during harvesting create an enriched and persistently viable near-surface seed bed that keeps on producing economic plants whenever activated by either anthropogenic fires, with short rotation intervals (multi-year) during human occupation, or by natural fires, with long rotation intervals (multi-decadal) following abandonment. Because these seed beds are refreshed whenever touched by fire, ruderal production can persist long after the cessation of the human occupation that created them in the first place.
Such ecological dynamics help explain why three AMS dates of seeds recovered from Feature 1 at Terrace MU 1471 (AA-113503; Beta-661553; Beta-661554) returned post-bomb ages (>AD 1955). It seems that in the late 20th century lightning-ignited fires consumed understory vegetation on the cut-back terraces and activated dormant seeds in the underlying soil (Koniak and Everett, 1982). If correct, this inference contributes to our understanding of the Invisible Pyrophyte Paradox, namely, that there are no reported instances of cheno-ams in areas that have not sustained fire for at least 30–50 years (Brewer et al., 1991; Alcoze and Hurteau, 2001). With sufficiently combustible fuel loads, the Upper Basin's prehistoric occupants burned when they wanted and where they could—sometimes successfully, other times not. The byproducts of this style of rolling economic burning, particularly how they register and are expressed today in the archaeological record, are what you would expect—patchy exposures of fire-following therophytes whose occurrences are difficult to predict.
Investigating the archaeological consequences of anthro-pogenic burning on forest ecosystem structure and vegetation community composition is advanced by the macrobotanical and pollen analysis of archaeological and geoarchaeological contexts that arise from a variety of palimpsest formation and assemblage accumulation cycles. In addition to providing antidotes to persistent problems in archaeology (e.g., the Registration Problem) and historical ecology (e.g., the Fading Record and No Analog Problems), the adoption of a time perspectivism theoretical framework enables archaeologists and paleoecologists an opportunity to appreciate the “hidden legacy” of pyrophytic ruderals, their economic significance in prehistoric foodways, and the extent to which anthropogenic fire, and its withdrawal, continues to profoundly affect the health and vitality of forested landscapes, and their human occupants, worldwide.
Data availability statement
The data reported in this paper are available by contacting the corresponding author (YWxhbi5zdWxsaXZhbkB1Yy5lZHU=).
Author contributions
AS: Conceptualization, Investigation, Methodology, Supervision, Writing – original draft, Writing – review & editing, Resources. CM: Investigation, Supervision, Writing – review & editing, Resources, Validation, Visualization. MW: Investigation, Writing – review & editing. PM: Writing – review & editing, Investigation, Software, Visualization. SA: Writing – review & editing, Resources, Supervision, Validation, Visualization.
Funding
The author(s) declare financial support was received for the research, authorship, and/or publication of this article. Financial support for the Upper Basin Archaeological Research Project fieldwork reported in this paper was provided by the C. P. Taft Research Center (University of Cincinnati), the University Research Council (University of Cincinnati), and the Matthew Van Pelt Memorial Fund (Department of Anthropology, University of Cincinnati). Support for CM's independent research in the wider Southwestern region was provided by the Wenner-Gren Foundation (Grant #8774).
Acknowledgments
We thank Josh Conver, Christina Hahn, and Emily Phillips for their tireless efforts in excavating and recording their rock-filled test-trenches at Terraces MU 1470, MU 1471, and MU 1466, respectively. Emily Phillips also provided substantial logistical support. Dr. Kathleen M. Forste (Joukowsky Institute for Archaeology and the Ancient World, Brown University), Dr. Christopher I. Roos (Department of Anthropology, Southern Methodist University), and Dr. Sissel Schroeder (Department of Anthropology, University of Wisconsin, Madison) provided invaluable comments that improved the organization and conclusions of this paper. Fieldwork permits were issued by Grand Canyon National Park (GRCA-2018-SCI-0032) and Kaibab National Forest (KAI135). We thank R. Newton and E. Brennan of Grand Canyon National Park and M. Hangan of Kaibab National Forest for securing these research permits for us.
Conflict of interest
The authors declare that the research was conducted in the absence of any commercial or financial relationships that could be construed as a potential conflict of interest.
Publisher's note
All claims expressed in this article are solely those of the authors and do not necessarily represent those of their affiliated organizations, or those of the publisher, the editors and the reviewers. Any product that may be evaluated in this article, or claim that may be made by its manufacturer, is not guaranteed or endorsed by the publisher.
References
Abella, S. R., and Springer, J. D. (2008). Estimating Soil Seed Bank Characteristics in Ponderosa Pine Forests Using Vegetation and Forest-Floor Data. RMRS-RN-35. Fort Collins, CO: USDA Forest Service Rocky Mountain Research Station.
Adams, K. R. (2011). “Anthropogenic ecology in the American Southwest,” in Movement, Connectivity, and Landscape Change in the Ancient Southwest, eds. M. C. Nelson and C. Strawhacker (Boulder, CO: University Press of Colorado), 119–140.
Adams, K. R., and Fish, S. K. (2011). “Subsistence through time in the Greater Southwest,” in The Subsistence Economies of Indigenous North American Societies: A Handbook, ed. B. D. Smith (Washington, DC: Smithsonian Institution Scholarly Press), 147–183.
Adlam, C., Almendariz, D., Goode, R. W., Martinez, D. J., and Middleton, B. R. (2021). Keepers of the flame: supporting the revitalization of Indigenous cultural burning. Soc. Nat. Resour. 35, 575–590. doi: 10.1080/08941920.2021.2006385
Alcoze, T., and Hurteau, M. (2001). “Implementing the archaeo-environmental reconstruction technique: rediscovering the historic ground layer of three plant communities in the greater Grand Canyon region,” in The Historical Ecology Handbook: A Restorationist's Guide to Reference Ecosystems, eds. D. Egan and E. A. Howell (Washington, DC: Island Press), 413–424.
Anderson, M. K., and Lake, F. K. (2013). California Indian ethnomycology and associated forest management. J. Ethnobiol. 33, 33–85. doi: 10.2993/0278-0771-33.1.33
Bailey, G. N. (2007). Time perspectives, palimpsests, and the archaeology of time. J. Anthropol. Archaeol. 26, 198–223. doi: 10.1016/j.jaa.2006.08.002
Balsom, J., Brennan, E., Dieker, J., and Hough, I. (2017). “Life along the Colorado River,” in The Archaeology of Grand Canyon: Ancient Peoples, Ancient Places, eds. F. E. Smiley, C. E. Downum, and S. G. Smiley (Grand Canyon, AZ: Grand Canyon Association), 201–239.
Bates, J. D., and Davies, K. W. (2016). Seasonal burning of juniper woodlands and spatial recovery of herbaceous vegetation. For. Ecol. Manage. 361, 117–130. doi: 10.1016/j.foreco.2015.10.045
Becher, M. E. (1992). Archaeological investigations at a Pueblo II Kayenta Anasazi ruin located in the Tusayan Ranger District, Kaibab National Forest, Arizona (B.A. thesis). University of Cincinnati, Cincinnati, OH, United States.
Berkebile, J. N. (2014). Investigating subsistence diversity in the Upper Basin: a second look at archaeobotanical remains from MU 125, a Late Pueblo II settlement (Master's thesis). University of Cincinnati, Cincinnati, OH, United States.
Bisbing, S. M., Urza, A. K., York, R. A., Hankin, L. E., and Putz, R. R. (2023). Persistent, viable seedbank buffers serotinous bishop pine over a broad fire return interval. Fire Ecol. 19:35. doi: 10.1186/s42408-023-00194-3
Bohrer, V. L. (1983). New life from ashes: the tale of the burnt bush (Rhus trilobata). Desert Plants 5, 122–124.
Bonnicksen, T. M. (2000). America's Ancient Forests: From the Ice Age to the Age of Discovery. New York, NY: John Wiley and Sons.
Bowman, D. M. J. S., Balch, J., Artaxo, P., Bond, W. J., Cochrane, M. A., D'Antonio, C. M., et al. (2011). The human dimension of fire regimes on Earth. J. Biogeogr. 38, 2223–2236. doi: 10.1111/j.1365-2699.2011.02595.x
Bozarth, S. (1992). “Fossil pollen and phytolith analysis,” in Archaeological Investigations at Lee Canyon: Kayenta Anasazi Farmsteads in the Upper Basin, Coconino County, Arizona, ed. S. M. Whittlesey (Tucson, AZ: Statistical Research), 135–144.
Brewer, D. G., Jorgensen, R. K., Munk, L. P., Robbie, W. A., and Travis, J. L. (1991). Terrestrial Ecosystems Survey of the Kaibab National Forest. Albuquerque, NM: USDA Forest Service.
Brown, A. G., Fallu, D., Cucchiaro, S., Alonso, M., Albert, R. M., Walsh, K., et al. (2023). Early to Middle Bronze Age agricultural terraces in north—east England: morphology, dating and cultural implication. Antiquity 97, 348–366. doi: 10.15184/aqy.2023.1
Bye, R. A. Jr. (1985). Botanical perspectives of ethnobotany of the Greater South-west. Econ. Bot. 39, 375–386.
Carter, V. A., Brunelle, A., Power, M. J., DeRose, R. J., Bekker, M. F., Hart, I., et al. (2021). Legacies of Indigenous land use shaped past wildfire regimes in the Basin-Plateau Region, USA. Nat. Commun. Earth Environ. 2:72. doi: 10.1038/s43247-021-00137-3
Cleeland, T., Hanson, J., Lesko, L., and Weintraub, N. (1990). Ethnographic Use of the South Kaibab. Williams, AZ: Kaibab National Forest.
Cook, R. A. (1995). Long-term upland wild-resource subsistence technology: evidence from fire-cracked rock piles in the Upper Basin, Kaibab National Forest, northern Arizona (Master's thesis). University of Cincinnati, Cincinnati, OH, United States.
Coughlan, M. R. (2013). Errakina: pastoral fire use and landscape memory in the Basque region of the French western Pyrenees. J. Ethnobiol. 33, 86–104. doi: 10.2993/0278-0771-33.1.86
Covington, W. W., and Moore, M. M. (1994). Southwestern ponderosa forest structure. J. For. 92, 39–47.
Crabtree, S. A., Vaughn, L. J. S., and Crabtree, N. T. (2017). Reconstructing ancestral Pueblo food webs in the southwestern United States. J. Arch. Sci. 81, 116–127. doi: 10.1016/j.jas.2017.03.005
Cummings, L. S. (1994). “Anasazi diet: variety in the Hoy House and Lion House coprolite record and nutritional analysis,” in Paleonutrition: The Diet and Health of Prehistoric American, ed. K. D. Sobolik (Carbondale, IL: Southern Illinois University Press), 134–150.
Cummings, L. S., and Puseman, K. (1995). Pollen and Macrofloral Analysis at Sites MU 123, MU 125, and MU 235 in Kaibab National Forest, North-Central Arizona. Report 95-10. Denver, CO: PaleoResearch Laboratories.
Cummings, L. S., and Puseman, K. (1997). Pollen and Macrofloral Analyses at Sites MU 125 and MU 125A, Arizona. Report 97-45. Denver, CO: PaleoRe-search Laboratories.
Cummings, L. S., and Puseman, K. (2010). “Pollen, macrofloral, and organic residue (FTIR) analysis for site AZ B:16:105, Northern Arizona,” in Archaeological Excavations at Site B:16:105, Grand Canyon National Park, ed. C. E. Downum (Flagstaff, AZ: Northern Arizona University), 83–115.
Cuthrell, R. Q. (2013). Archaeobotanical evidence for Indigenous burning practices and foodways at CA-SMA-113. Calif. Archaeol. 5, 265–290. doi: 10.1179/1947461X13Z.00000000015
Damick, A., Krause, S., and Rosen, A. (2022). Building resilient landscapes in a semi-arid watershed: anthropogenic and natural burning histories in late Holocene Tesuque Creek, northern New Mexico. Holocene 32, 1437–1449. doi: 10.1177/09596836221121771
D'Andrea, R. M., Anderson, R. S., Cole, K. L., and Zweifel, M. K. (2023). Climate and human impact of vegetation and fire in an arid Colorado Plateau ecosystem in Western North America. Quarter. Sci. Rev. 321, 1–19. doi: 10.1016/j.quascirev.2023.108374
Darling, M. L. S. (1967). Structure and productivity of pinyon-juniper woodland in northern Arizona (Dissertation). Duke University, Durham, NC, United States.
Davis, O. K. (1986). “Pollen analysis from AZ:I:1:17 (ASM),” in Prehistory of the Upper Basin, Coconino County, Arizona, ed. A. P. Sullivan III (Tucson, AZ: University of Arizona), 333–338.
Downum, C. E. (ed.)., (2010). Archaeological Excavations at Site B:16:105, Grand Canyon National Park. Flagstaff, AZ: Northern Arizona University.
Downum, C. E., and Vance, M. M. (2017). “The formative and protohistoric periods in Grand Canyon,” in The Archaeology of Grand Canyon: Ancient Peoples, Ancient Places, eds. F. E. Smiley, C. E. Downum, and S. G. Smiley (Grand Canyon, AZ: Grand Canyon Association), 89–136.
Euler, R. C. (1969). “The archaeology of the Canyon Country,” in John Wesley Powell and the Anthropology of the Canyon Country, eds. D. D. Fowler, R. C. Euler, and C. S. Fowler (Washington, DC: United States Government Printing Office), 8–20.
Euler, R. C., and Dobyns, H. F. (1983). “The ethnoarchaeology of Pai milling stones,” in Collected Papers in Honor of Charlie R. Steen Jr., ed. N. L. Fox (Albuquerque, NM: Archaeological Society of New Mexico), 253–267.
Ford, R. I. (1984). “Ecological consequences of early agriculture in the Southwest,” in Papers on the Archaeology of Black Mesa, Arizona, Vol. II, eds. S. Plog and S. Powell (Carbondale, IL: Southern Illinois University Press), 127–138.
Ford, R. I. (2000). “Human disturbance and biodiversity: a case study from northern New Mexico,” in Biodiversity and Native America, eds. P. E. Minnis and W. J. Elisens (Norman, OK: University of Oklahoma Press), 207–222.
Fowler, C. T., and Welch, J. R. (2018). Fire Otherwise: Ethnobiology of Burning for a Changing World. Salt Lake City, UT: University of Utah Press.
Fowler, D. D., and Fowler, C. S. (1969). “The ethnography of the Canyon Country,” in John Wesley Powell and the Anthropology of the Canyon Country, eds. D. D. Fowler, R. C. Euler, and C. S. Fowler (Washington, DC: United States Government Printing Office), 20–30.
Foxx, T. S. (1996). “Vegetation succession after the La Mesa Fire at Bandelier National Monument,” in Fire Effects in Southwestern Forests: Proceedings of the Second La Mesa Fire Symposium, ed. C. D. Allen (Fort Collins, CO: USDA Forest Service), 47–69.
French, C., Perriman, R., Cummings, L. S., Hall, S., Goodman-Elgar, M., and Boreham, J. (2009). Holocene alluvial sequences, cumulic soils, and fire signatures in the Middle Rio Puerco Basin at Guadalupe Ruin, New Mexico. Geoarchaeology 24, 638–676. doi: 10.1002/gea.20278
Fritz, G. J. (2007). “Pigweeds for the ancestors: cultural identities and arch-aeobotanical identification methods,” in The Archaeology of Food and Identity, ed. K. C. Twiss (Carbondale, IL: Southern Illinois University Press), 288–307.
Fritz, G. J., Bruno, M. C., Langlie, B. S., Smith, B. D., and Kistler, L. (2017). “Cultigen chenopods in the Americas: a hemispherical perspective,” in Social Perspectives on Ancient Lives from Paleoethnobotanical Data, eds. M. P. Sayre and M. C. Bruno (Dordrecht, NL: Springer International Publishing), 55–75.
Fugate, T. I. (2004). Inferring settlement formation patterns: a GIS-based Harris Matrix analysis of a prehistoric masonry ruin in the Upper Basin, Kaibab National Forest, Northern Arizona (Master's thesis). University of Cincinnati, Cincinnati, OH, United States.
Fulé, P. Z. (2010). “Wildfire ecology and management at Grand Canyon, AZ: tree-ring applications in forest fire history and modeling,” in Tree Rings and Natural Hazards: A State-of-the-Art, eds. M. Stoffel, M. Bollschweiler, D. R. Butler, and B. H. Luckman (New York, NY: Springer), 365–381.
Fulé, P. Z., Heinlein, T. A., Covington, W., and Moore, M. M. (2003). Assessing fire regimes on Grand Canyon landscapes with fire-scar and fire-record data. Int. J. Wildland Fire 12, 129–145. doi: 10.1071/WF02060
Guiterman, C. H., Gregg, R. M., Marshall, L. A. E., Beckmann, J. J., van Mantgem, P. J., Falk, D. A., et al. (2022). Vegetation type conversion in the US Southwest: frontline observations and management responses. Fire Ecol. 18:6. doi: 10.1186/s42408-022-00131-w
Herring, E. M., Anderson, R. S., and San Miguel, G. L. (2014). Fire, vegetation, and ancestral Puebloans: a sediment record from Prater Canyon in Mesa Verde National Park, Colorado, USA. Holocene 24, 853–863. doi: 10.1177/0959683614530440
Homburg, J. A. (1992). “Soil fertility study,” in Archaeological Investigations at Lee Canyon: Kayenta Anasazi Farmsteads in the Upper Basin, Coconino County, Arizona, ed. S. M. Whittlesey (Tucson, AZ: Statistical Research), 145–161.
Hopkins, R. L., and Thompson, K. L. (2003). “Kaibab formation,” in Grand Canyon Geology, 2nd Edn, eds. S. S. Beus and M. Morales (New York, NY: Oxford University Press), 196–211.
Hough, I., and Brennan, E. (2008). “Architectural documentation and preservation of Havasupai and Navajo wooden pole structures in Grand Canyon National Park,” in Reflections of Grand Canyon Historians: Ideas, Arguments, and First-Person Accounts, ed. T. R. Berger (Grand Canyon, AZ: Grand Canyon Association), 81–88.
Huckell, L. W. (1992). “Plant remains,” in Archaeological Investigations at Lee Canyon: Kayenta Anasazi Farmsteads in the Upper Basin, Coconino Country, Arizona, ed. S. M. Whittlesey (Tucson, AZ: Statistical Research), 119–133.
Huffman, D. W., Stoddard, M. T., Springer, J. D., Crouse, J. E., and Chancellor, W. W. (2013). Understory plant community responses to hazardous fuels reduction treatments in pinyon-juniper woodlands of Arizona, USA. For. Ecol. Manage. 289, 478–488. doi: 10.1016/j.foreco.2012.09.030
James, L. E. A. (2009). Quinoa (Chenopodium quinoa Willd): composition, chemistry, nutritional, and functional properties. Adv. Food Nutr. Res. 58, 1–31. doi: 10.1016/S1043-4526(09)58001-1
Jones, J. G. (2019). Analysis of Pollen from Northern Arizona. Tempe, AZ: Archaeological Consulting Servies.
Jorgensen, J. G. (1983). “Comparative traditional economies and ecological adaptations,” in Handbook of North American Indians, Vol. 9, Southwest, ed. A. Ortiz (Washington, DC: Smithsonian Institution), 684–710.
Karkanas, P., and Goldberg, P. (2019). Reconstructing Archaeological Sites: Understanding the Geoarchaeological Matrix. Hoboken, NJ: John Wiley and Sons.
King, F. B. (1994). “Interpreting wild plant foods in the archaeological record,” in Eating on the Wild Side: The Pharmacologic, Ecologic, and Social Implications of Using Noncultigens, ed. N. L. Etkin (Tucson, AZ: University of Arizona Press), 185–209.
Klimaszewski-Patterson, A. (2023). Potential of paleoecology and paleolandscape modeling to identify pre-Colonial cultural burning in montane forests: case studies in California. Front. Environ. Archaeol. 2:1251149. doi: 10.3389/fearc.2023.1251149
Kohler, T. A. (1992). Prehistoric human impact on the environment in the upland North American Southwest. Popul. Environ. 13, 255–268.
Koniak, S., and Everett, R. L. (1982). Seed reserves in soils of successional stages of pinyon woodlands. Am. Midland Nat. 108, 295–303.
Lake, F. K., Wright, V., Morgan, P., McFadzen, M., McWethy, D., and Stevens-Rumann, C. (2017). Returning fire to the land – celebrating traditional knowledge and fire. J. For. 115, 343–353. doi: 10.5849/jof.2016-043R2
Lanner, R. M. (1981). The Piñon Pine: A Natural and Cultural History. Reno, NV: University of Nevada Press.
Lefler, B. J. (2014). Nuwuvi (Southern Paiute) ecological knowledge of pinyon-juniper woodlands: implications for conservation and sustainable resource use in two southern Nevada protected areas (Master's thesis). Portland State University, Portland, OR, United States.
Lewis, H. T. (1972). The role of fire in the domestication of plants and animals in southwest Asia: a hypothesis. Man. 7, 195–222.
Louderback, L. A., and Pavlik, B. M. (2018). Integrating modern vegetation and ethnographic data to understand dietary choices in the past: the case of the Southern Paiute, Utah. Hum. Ecol. 46, 897–908. doi: 10.1007/s10745-018-0040-9
Lucas, G. (2012). Understanding the Archaeological Record. Cambridge, MA: University of Cambridge Press.
Margolis, E. Q. (2014). Fire regime shift linked to increased forest density in a pinyon-juniper savanna landscape. Int. J. Wildland Fire. 23, 234–245. doi: 10.1071/WF13053
Martin, J. F. (1985). The prehistory and ethnohistory of Havasupai-Hualapai relations. Ethnohistory 32, 135–153.
Maxwell, T. D., and Anschuetz, K. F. (1992). “The Southwestern ethnographic record and prehistoric agricultural diversity,” in Gardens of Prehistory: The Archaeology of Settlement Agriculture in Greater Mesoamerica, ed. T. W. Killion (Tuscaloosa, AL: University of Alabama Press), 35–68.
McNamee, C. (2003). A geoarchaeological investigation of Simkins Flat, Upper Basin, northern Arizona (Master's thesis). University of Calgary, Calgary, AB, Canada.
McNamee, C., Sullivan, A. P. III., Marketou, T., Vitale, S., and Michailidou, M. (2021). Managing environmental challenges with anthropogenic bedrock modification: archaeological survey evidence from the Upper Basin (USA) and the Island of Kos (Greece). Quat. Int. 597, 76–86. doi: 10.1016/j.quaint.2020.12.023
Mellars, P. (1976). Fire ecology, animal populations and man: a study of some ecological relationships in prehistory. Proc. Pre. Soc. 42, 15–45.
Merkle, J. (1952). An analysis of a pinyon-juniper community at Grand Canyon, Arizona. Ecology 33, 375–384.
Metzger, D. G. (1961). Geology in Relation to Availability of Water along the South Rim, Grand Canyon National Park, Arizona. Washington, DC: Government Printing Office.
Mindeleff, V. (1891 [reprinted 1989]). A Study of Pueblo Architecture in Tusayan and Cibola. Washington, DC: Smithsonian Institution.
Mink, P. B. II. (2019). Eastern Virgin hinterlands: formative period (AD 700–AD 1225) settlement practices in Grand Canyon National Park. Kiva. 85, 412–433. doi: 10.1080/00231940.2019.1690319
Minnis, P. E. (2000). “Prehistoric agriculture and anthropogenic ecology of the North American Southwest,” in The Archaeology of Drylands: Living at the Margin, eds. G. Barker and D. Gilbertson (New York, NY: Routledge), 272–287.
Mueller-Bieniek, A., Bogucki, P., Pyzel, J., Kapcia, M., Hoyo, M. M., and Nalepka, D. (2019). The role of Chenopodium in the subsistence economy of pioneer agriculturalists on the northern frontier of the Linear Pottery culture in Kuyavia, central Poland. J. Arch. Sci. 111:105027. doi: 10.1016/j.jas.2019.105027
Mueller-Bieniek, A., Pyzel, J., and Kapcia, M. (2018). Chenopodium seeds in open-air archaeological sites – how to not throw the baby out with the bathwater. Environ. Archaeol. 25, 69–81. doi: 10.1080/14614103.2018.1536500
Myers, M. D., and Doolittle, W. E. (2014). “The new narrative on native landscape transformations,” in North American Odyssey: Historical Geographies for the Twenty-First Century, eds. C. E. Colton and G. L. Buckley (Lanham, MD: Rowan and Little-field), 9–26.
Nabhan, G. P., Coder, M., and Smith, S. J. (2004). Woodlands in Crisis: A Legacy of Lost Diversity on the Colorado Plateau. Flagstaff, AZ: Northern Arizona University.
Ortiz, A. (ed.)., (1979). Handbook of North American Indians, Vol. 9, Southwest. Washington, DC: Smithsonian Institution.
Ortiz, A. (ed.)., (1983). Handbook of North American Indians, Vol. 10, Southwest. Washington, DC: Smithsonian Institution.
Oryokot, J. O. E., Murphy, S. D., Thomas, A. G., and Swanton, C. J. (1997). Temperature-and moisture-driven models of seed germination and shoot elongation in green and redroot pigweed (Amaranthus powelli, A. retroflexus). Weed Sci. 45, 488–496.
Purtill, M. P. (1995). Analysis and interpretation of chipped-stone tool assemblages from the Upper Basin, Kaibab National Forest, northern Arizona (Master's thesis). University of Cincinnati, Cincinnati, OH, United States.
Pyne, S. J. (1982). Fire in America: A Cultural History of Wildland and Rural Fire. Princeton, NJ: Princeton University Press.
Pyne, S. J. (1998). “Forged in fire: history, land, and anthropogenic fire,” in Advances in Historical Ecology, ed. W. L. Balee (New York, NY: Columbia University Press), 64–103.
Pyne, S. J. (2021b). The Pyrocene: How We Created an Age of Fire, and What Happens Next. Oakland, CA: University of California Press.
Rand, P. J. (1965). Factors related to the distribution of ponderosa and pinyon pines at Grand Canyon, Arizona (Dissertation). Duke University, Durham, NC, United States.
Reddy, S. (2016). Changing palates and resources: regional and diachronic trends in plant use in prehistoric California. J. Calif. Great Basin Anthropol. 36, 225–242.
Redman, C. L. (1999). Human Impact on Ancient Environments. Tucson, AZ: University of Arizona Press.
Reed, S. C. (2021). Joining Laboratory, Greenhouse, and Field Approaches to Improve Our Understanding of Fire Effects on Seed Germination in Great Basin, Colorado Plateau, and Sonoran Deserts. Joint Fire Science Program Project ID: 17-1-04-17. U.S. Geological Survey.
Romme, W. H., Allen, C. D., Bailey, J. D., Baker, W. L., Bestelmeyer, B. T., Brown, P. M., et al. (2009). Historical and modern disturbance regimes, stand structures, and landscape dynamics in pinyon-juniper vegetation of the western United States. Rangeland Ecol. Manage. 62, 203–222. doi: 10.2111/08-188R1.1
Roos, C. I., Guiterman, C. H., Margolis, E. Q., Swetnam, T. W., Laluk, N. C., Thompson, K. F., et al. (2022). Indigenous fire management and cross-scale fire-climate relationships in the Southwest United States from 1500 to 1900 CE. Sci. Adv. 8:49. doi: 10.1126/sciadv.abq3221
Roos, C. I., Laluk, N. C., Reitze, W., and Davis, O. K. (2023). Stratigraphic evidence for culturally variable Indigenous fire regimes in ponderosa pine forests of the Mogollon Rim area, east-central Arizona. Quat. Res. 113, 69–86. doi: 10.1017/qua.2022.61
Roos, C. I., Rittenour, T. M., Swetnam, T. W., Loehman, R. A., Hollenback, K. L., Liebmann, M. J., et al. (2020). Fire suppression impacts on fuels and fire intensity in the western U.S.: insights from archaeological luminescence dating in northern New Mexico. Fire 3:3. doi: 10.3390/fire3030032
Roos, C. I., Sullivan, A. P. III., and McNamee, C. (2010). “Paleoecological evidence for systematic Indigenous burning in the upland Southwest,” in The Archaeology of Anthropogenic Environments, ed. R. M. Dean (Carbondale, IL: Southern Illinois University Press), 142–171.
Scherjon, F., Berkels, C., MacDonald, K., and Roebroeks, W. (2015). Burning the land: an ethnographic study of off-site fire use by current and historically documented foragers and implications for the interpretation of past fire practices in the landscape. Curr. Anthropol. 56, 299–326. doi: 10.1086/681561
Scott, L. J. (1986). “Analysis of selected macrofloral samples from AZ:I:1:17 (ASM),” in Prehistory of the Upper Basin, Coconino County, Arizona, ed. A. P. Sullivan III (Tucson, AZ: University of Arizona), 339–346.
Smiley, F. E. (2017). “The ancient ones: ten thousand years of hunting and gathering at Grand Canyon,” in The Archaeology of Grand Canyon: Ancient Peoples, Ancient Places, eds. F. E. Smiley, C. E. Downum, and S. G. Smiley (Grand Canyon, AZ: Grand Canyon Association), 27–60.
Smith, B. D. (2014). “Documenting human niche construction in the archaeological record,” in Method and Theory in Paleoethnobotany, eds. J. C. Marston, J. D. Guedes, and C. Warinner (Boulder, CO: University Press of Colorado), 355–370.
Smith, S. J. (2003). Simkins Flat Pollen Analysis. Flagstaff, AZ: Laboratory of Paleoecology; Northern Arizona University.
Snitker, G. (2018). Identifying natural and anthropogenic drivers of prehistoric fire regimes through simulated charcoal records. J. Arch. Sci. 95, 1–15. doi: 10.1016/j.jas.2018.04.009
Snitker, G., Roos, C. I., Sullivan, A. P. III., Maezumi, S. Y., Bird, D. W., Coughlan, M. R., Derr, K. M., et al. (2022). A collaborative agenda for archaeology and fire science. Nat. Ecol. Evol. 12:1759. doi: 10.1038/s41559-022-01759-2
Springer, J. D., Daniels, M. L., and Nazaire, M. (2009). Field Guide to Forest and Mountain Plants of Northern Arizona. Flagstaff, AZ: Northern Arizona University.
Springer, J. D., Stoddard, M. T., Rodman, K. C., Huffman, D. W., Fornwalt, P. J., Pedersen, P. J., et al. (2024). Increases in understory plant cover and richness persist following restoration treatments in Pinus ponderosa forests. J. Appl. Ecol. 61, 25–35. doi: 10.1111/1365-2664.14538
Steward, A. M. (2018). “Fire use among swidden farmers in Central Amazonia: reflections on practice and conservation policies,” in Fire Otherwise: Ethnobiology of Burning for a Changing World, eds. C. T. Fowler and J. R. Welch (Salt Lake City, UT: University of Utah Press), 104–127.
Sullivan, A. P. III. (1987). Seeds of discontent: implications of a ‘Pompeii' botanical assemblage for Grand Canyon Anasazi subsistence models. J. Ethnobiol. 7, 137–153.
Sullivan, A. P. III. (1988). Prehistoric southwestern ceramic manufacture: the limi-tations of current evidence. Am. Antiq. 53, 23–35. doi: 10.2307/281152
Sullivan, A. P. III. (1992). “Pinyon nuts and other wild resources in Western Anasazi subsistence economies,” in Long-Term Subsistence Change in Prehistoric North America, eds. D. R. Croes, R. A. Hawkins, and B. L. Isaac (Greenwich, CT: JAI Press), 195–240.
Sullivan, A. P. III. (2000). Effects of small-scale prehistoric runoff agriculture on soil fertility: the developing picture from upland terraces in the American Southwest. Geoarchaeology 15, 291–314. doi: 10.1002/(SICI)1520-6548(200004)15:4<291::AID-GEA1>3.0.CO;2-K
Sullivan, A. P. III. (2008). “Time perspectivism and the interpretive potential of palimpsests: theoretical and methodological considerations of assemblage formation history and contemporaneity,” in Time in Archaeology: Time Perspectivism Revisited, eds. S. Holdaway and L. Wandsnider (Salt Lake City, UT: University of Utah Press), 31–45.
Sullivan, A. P. III. (2015). “The archaeology of ruderal agriculture,” in Traditional Arid Lands Agriculture: Understanding the Past for the Future, eds. S. E. Ingram and R. C. Hunt (Tucson, AZ: University of Arizona Press), 273–305.
Sullivan, A. P. III., Becher, M. E., and Downum, C. E. (1995). Tusayan whiteware chronology: new archaeological and dendrochronological evidence. Kiva 61, 175–188.
Sullivan, A. P. III., Berkebile, J. N., Forste, K. M., and Washam, R. M. (2015). Disturbing developments: an archaeobotanical perspective on pinyon-juniper wood-lands fire ecology, economic resource production, and ecosystem history. J. Ethnobiol. 35, 37–59. doi: 10.2993/0278-0771-35.1.37
Sullivan, A. P. III., Cook, R. A., Purtill, M. P., and Uphus, P. M. (2001). Economic and land-use implications of prehistoric fire-cracked-rock piles, northern Arizona. J. Field Arch. 28, 367–382. doi: 10.1179/jfa.2001.28.3-4.367
Sullivan A. P. III. and Downum C. E. (1991). Aridity, activity, and volcanic ash agriculture: a study of short-term prehistoric cultural-ecological dynamics. World Arch. 22, 271–287.
Sullivan A. P. III. and Forste K. M. (2014). Fire-reliant subsistence economies and anthropogenic coniferous ecosystems in the pre-Columbian northern American Southwest. Veg. Hist. Archaeobot. 23, 35–151. doi: 10.1007/s00334-014-0434-6
Sullivan A. P. III. and Mink P. B. II (2018). Theoretical and socioecological consequences of fire foodways. Am. Antiq. 83, 619–638. doi: 10.1017/aaq.2018.32
Sullivan A. P. III. Mink P. B. II. and Uphus P. M. (2002b). “From John W. Powell to Robert C. Euler: testing models of Grand Canyon's prehistoric Puebloan settlement history,” in Culture and Environment in the American Southwest: Essays in Honor of Robert C. Euler, eds. D. A. Phillips Jr., and J. A. Ware (Phoenix, AZ: SWCA), 49–68.
Sullivan A. P. III. and Ruter A. H. (2006). “The effects of environmental fluctuations on ancient livelihoods: implications of paleoeconomic evidence from the Upper Basin, Northern Arizona,” in Environmental Change and Human Adaptation in the Ancient American Southwest, eds. D. E. Doyel and J. S. Dean (Salt Lake City, UT: University of Utah Press), 180–203.
Sullivan, A. P. III., Uphus, P. M., Roos, C. I., and Mink, P. B. II (2002a). Inadvertent vandalism: the hidden challenge for heritage resource management. Cult. Res. Manage. 25, 42–46.
Swetnam, T. W., Allen, C. D., and Betancourt, J. L. (1999). Applied historical ecology: using the past to manage the future. Ecol. Appl. 9, 1189–1206.
Trigger, B. G. (2006). A History of Archaeological Thought, 2nd Edn. New York, NY: Cambridge University Press.
Turner, M. I., Adams, K. R., Berkebile, J. N., and Dockter, A. R. (2021). Ancient grains: new evidence for Ancestral Puebloan use of domesticated amaranth. Am. Antiq. 86, 815–832. doi: 10.1017/aaq.2021.57
United States Department of Agriculture and Fire Effects Information System. (2023). Available online at: https://www.feis-crs.org/feis/ (accessed September 13, 2023).
Uphus, P. M., Sullivan, A. P. III., and Mink, P. B. II (2006). Identifying at-risk heritage resources with GIS: modeling the impact of recreational activities on the archaeological record. Int. J. Risk Assess. Manage. 6, 330–343. doi: 10.1504/IJRAM.2006.009550
van der Veen, M. (2007). Formation processes of desiccated and carbonized plant remains—the identification of routine practice. J. Arch. Sci. 34, 968–990. doi: 10.1016/j.jas.2006.09.007
Vankat, J. L. (2013). Vegetation Dynamics on the Mountains and Plateaus of the American Southwest. Dordrecht, NL: Springer-Verlag.
Washam, R. (2014). Archaeology in distress: federal land management and archaeological vulnerability (Master's thesis). University of Cincinnati, Cincinnati, OH, United States.
Weber, S. A., and Seaman, P. D. (1985). Havasupai Habitat: A. F. Whiting's Ethnography of a Traditional Indian Culture. Tucson, AZ: University of Arizona Press.
West, N. E. (1984). “Successional patterns and productivity potentials of pinyon-juniper ecosystems,” in Developing Strategies for Rangeland Management, ed. National Research Council/National Academy of Sciences (Boulder, CO: Westview Press), 1301–1332.
Whittlesey, S. M. (1992). “Synthesis and interpretations,” in Archaeological Investigations at Lee Canyon: Kayenta Anasazi Farmsteads in the Upper Basin, Coconino County, Arizona, ed. S. M. Whittlesey (Tucson, AZ: Statistical Research), 163–189.
Wilke, P. J., Bettinger, R., King, T. F., and O'Connell, J. F. (1972). Harvest selection and domestication in seed plants. Antiquity. XLVI, 203–209. doi: 10.1017/S0003598X0005362X
Williams, M. A., and Baker, W. L. (2013). Variability of historical forest structure and fire across ponderosa pine landscapes of the Coconino Plateau and South Rim of Grand Canyon National Park, Arizona, USA. Landscape Ecol. 28, 297–310. doi: 10.1007/s10980-012-9835-z
Wu, X., Sverdrup, E., Mastrandrea, M. D., Wara, M. W., and Wager, S. (2023). Low-intensity fires mitigate the risk of high-intensity wildfires in California's forests. Sci. Adv. 9, 1–9. doi: 10.1126/sciadv.adi4123
Yarnell, R. A. (1965). Implications of distinctive flora on Pueblo ruins. Am. Anthropol. 67, 662–674. doi: 10.1525/aa.1965.67.3.02a00030
Keywords: economic fire, ruderals, archaeobotany, terraces, seedbeds, Grand Canyon
Citation: Sullivan AP III, McNamee C, Wendel M, Mink PB II and Allen SE (2024) Archaeological evidence of anthropogenic burning for food production in forested uplands of the Grand Canyon province, northern Arizona. Front. Environ. Archaeol. 3:1302604. doi: 10.3389/fearc.2024.1302604
Received: 26 September 2023; Accepted: 29 March 2024;
Published: 04 September 2024.
Edited by:
Laurent Lespez, Université Paris-Est Créteil Val de Marne, FranceReviewed by:
Debora Zurro, Spanish National Research Council (CSIC), SpainJade D'Alpoim Guedes, University of California, San Diego, United States
Copyright © 2024 Sullivan, McNamee, Wendel, Mink and Allen. This is an open-access article distributed under the terms of the Creative Commons Attribution License (CC BY). The use, distribution or reproduction in other forums is permitted, provided the original author(s) and the copyright owner(s) are credited and that the original publication in this journal is cited, in accordance with accepted academic practice. No use, distribution or reproduction is permitted which does not comply with these terms.
*Correspondence: Alan P. Sullivan III, YWxhbi5zdWxsaXZhbkB1Yy5lZHU=