- Department of Geography, California State University, Sacramento, Sacramento, CA, United States
Paleoecology and paleolandscape modeling have the potential to differentiate cultural burning from climatic fires, improving interpretations of past fire histories and vegetation resource management practices. People have conducted variations of traditional fire management to increase terrestrial resources for hundreds of millennia, commonly in fire-prone areas where vegetation is adapted to frequent fire events. Over time, these cultural fires influenced regrowth and led to an anthropogenically-modified landscape. For some non-agrarian, semi-nomadic societies, such as the pre-Colonial groups within what is now known as California, identifying anthropogenic landscapes is difficult because of a lack of domesticated plant remains in the environmental record to indicate where human impacts occurred. This paper uses case studies from the central and southern Sierra Nevada range in California to explore the potential of paleoecology, specifically pollen and sedimentary charcoal, and spatially-explicit paleolandscape modeling to identify and distinguish periods of cultural burning in mountainous forests to improve archaeological interpretations of human-fire dynamics. Specifically, I use climate-vegetation dynamics and cluster analysis to look at temporal relationships of change between sites. These case studies are ideal because (1) the region is naturally fire-prone, (2) study sites are typically well-dated and analyzed at a sub-centennial resolution, (3) study sites are associated with archaeological sites, and (4) indigenous groups were proto-agricultural, balanophagy societies known to practice cultural burning. These case study sites show a strong potential to identify periods of cultural burning that help better inform archaeological interpretations and show synchronous evidence for cultural burning during the Little Ice Age (1250-1850). Furthermore, these studies provide better dated timelines of human influence at each site than nearby archaeological studies, indicating that in certain locales, paleoecological studies with high temporal resolutions could be used to inform the timing of archaeological activities and shifts.
1. Introduction
Humans have engaged in forms of traditional fire management for countless millennia, particularly in areas prone to wildfires where vegetation has evolved to withstand frequent fire occurrences (Stepka et al., 2022). World-wide, there is no doubt that these cultural fires shaped regrowth dynamics and ultimately resulted in landscapes that bears unmistakable imprint of human influence. And yet, debate continues as to whether cultural burning in pre-Colonial California altered landscapes beyond climatic expectations (Vachula et al., 2019; Knight et al., 2022).
Accounts by colonial Europeans indicate that indigenous fire management was a long-standing cultural practice. Historic Spanish accounts from the 1500s recount smoke resulting from the burning of grasslands by indigenous populations (Clar, 1959, p. 7; Bolton, 1967, p. 24, 33; Lewis, 1973). Ethnographies from the early 20th Century provide evidence for the regular use of low-intensity fires to clear corridors and remove underbrush, promote seed germination and resprouting, drive game and aid in hunting, and generally increase economic resource yields, but questions remain about the extent and intensity of cultural burning by pre-Colonial indigenous groups (Kroeber, 1925; Voegelin, 1938; Gayton, 1948).
Depending on the proxy data used, some scientists interpret support of cultural burning creating human-influenced landscapes in the pre-Colonial United States (Denevan, 1992; Lightfoot and Parrish, 2009; Abrams and Nowacki, 2020) while others argue in support of climate-driven ecosystems (Vale, 2002; Vachula et al., 2019; Oswald et al., 2020). The challenge then is finding and analyzing appropriate proxy records in a given environ that may help distinguish ecological changes resulting from cultural burning vs. climatic fires. Paleoecology may offer valuable insights into distinguishing cultural burning from climatic fires, thereby enhancing our understanding of fire patterns and vegetation resource management practices.
Recent paleoecologic studies in California have hypothesized that persistence or expansion of open-canopy, fire-tolerant taxa during cooler, wetter climatic periods is indicative of cultural burning (Crawford et al., 2015; Klimaszewski-Patterson and Mensing, 2016, 2020; Knight et al., 2022). This is because open-canopy, fire-tolerant taxa, such as oaks, are food resources and indigenous people would have preferentially preserved these economic habitats with fire. Because cultural burning typically consists of low intensity fires, it is more likely to produce ash rather than charcoal; therefore, these fires may not be captured as charcoal in the sedimentary record. Rather, sedimentary charcoal may be indicative of climatic wildfires and instead it is pollen-based vegetation changes that better elucidates cultural burning.
In this paper I look at existing environmental-focused paleoecological studies from forests in the southern and central Sierra Nevada range in California to explore paleoecological inferences to the timing and periodicity of cultural burning. I limit the study to the southern and central Sierra Nevada because the indigenous groups in this area are considered mobile with similar lifeways. I use a two pollen-based approaches to identify periods of cultural burning: (1) open/closed canopy pollen taxa relationships relative to climatic expectations, and (2) cluster analysis, which does not restrict pollen analysis to open/closed forest canopy taxa. My goal is to examine the current state of cultural burning effects via the environmental record and whether a regional synthesis is possible using existing datasets. I focus on stratigraphically-constrained environmental archives and compare results against known archaeological interpretations in the vicinity of paleoecologic sites. Finally, I summarize published explorations using paleolandscape modeling to test hypotheses of cultural burning.
1.1. Population context
Indigenous populations in the central and southern Sierra Nevada, including but not limited to, the Tubatulabal, Foothill Yokuts, Western Mono, and Sierra Miwok, were traditionally mobile. Mobile groups are generally considered to have had lower population densities because they occupied more extensive territories (Binford, 2001) and needed to leverage seasonal resources among diverse ecosystems in the Sierra Nevada within their territorial range (Simpson and Jones, 2020). Indigenous groups of the central and southern Sierra Nevada were, and remain, balanophagus (acorn-eating) and are associated with the utilization and management of acorn resources. Stone pestles, bedrock slicks, and bedrock mortars demonstrate a long-term investment in developing permanent facilities to process seeds and acorns (Stevens et al., 2019). Archaeological evidence suggests a seasonal fission-fusion pattern, with Sierran groups coming together (fusion) in the late fall and winter, and groups dispersing (fissioning) across their range from Spring to early Autumn for hunting, fishing, and harvesting of plant resources (Voegelin, 1938; Gayton, 1948; Harvey, 2018). Because these mobile groups are presumed to have lower population densities and less consistently intense use of resources, there is still debate as to whether the cultural burning they practiced was extensive and/or intensive enough to notably alter ecosystems.
1.2. Ecological context
Fire is both a natural disturbance process and a tool used by people world-wide for at least 800,000 years (Stepka et al., 2022). Where vegetation is fire-adapted, modern and historically-known hunter-gatherer groups are more likely to use fire for land and resource management (Coughlan et al., 2018). Many ecosystems in present-day California, such as chaparral and redwood forests, are fire adapted, and archaeological and paleoecological evidence demonstrates that humans have utilized fire for resource management in California for hundreds, if not thousands of years (Baumhoff, 1963).
Paleoecology is utilized to investigate the extent and impact of ecological changes over time, providing a framework for distinguishing between climatic and anthropogenic drivers of change. Tree-rings can be used for climate reconstructions (Cook et al., 2007), while proxies such as pollen, spores, and charcoal are used to reconstruct vegetation and fire histories. These latter proxies are obtained from sediments extracted from environmental archives such as lakes, ponds, and sedge meadows. These archives offer several advantages: (1) they have high sedimentation rates, capturing years to decades per centimeter, (2) when cored, the extracted sediments preserve continuous stratigraphy, and (3) bioturbation can be minimal in ideal locations due to the absence of burrowing animals or hydrologic disturbances, and sedge meadows have dense root masses that help maintain stratigraphic integrity. Because the radiometric dates are stratigraphically-controlled, the statistical age models for these proxies may allow for more granularity of temporal land use than Sierran archaeological excavations alone.
Though fire is a vital factor in shaping the structure and composition of vegetation in California, its extent and intensity are modulated by climate, weather, and season. Wet winters allow for rapid growth of annuals and grasses, while long, dry summers convert the annuals and grasses into fine fire fuels. Periodic late summer and autumn lightning storms ignite dried fuels and create wildfires. The greater the fuel load and number of lightning strikes, the greater the opportunity for wildfires.
California's climate is conducive to wildfires and vegetation has adapted accordingly (Sugihara et al., 2018). Many conifers, such as Pinus spp. (pines) and Sequoiadendron giganteum (giant sequoia), have serotinous cones and fire-resistant bark. Numerous Quercus spp. (oaks), such as Q. kelloggii (California black oak), Q. wislizeni (interior live oak), and Q. chrysolepis (canyon live oak) resprout after fire, chaparral plants such as Ceanothus spp. produce heat-resistant seeds that only germinate after intense fires. These sample species are drought-tolerant, fire-adapted, and because they thrive in open forest canopy conditions, shade-intolerant. Conversely, fir trees develop a thick, fire-resistant trunk as they age but are fire-sensitive and tender graze for deer when young. Firs grow rapidly and are shade tolerant, quickly leading to more closed forest canopies once tall enough to out-compete many shade-intolerant species (Tubbesing et al., 2020).
Ecologically, when climate periods are drier or warmer, such as the Medieval Climate Anomaly (MCA; 900–1250) we expect more fires, less biomass, and more open forest canopies. This leads to increased trends of fire-adapted taxa such as oaks and Calocedrus decurrens (California incense-cedar). When climate periods are cooler or wetter, such as the Little Ice Age (LIA; 1250–1850) we expect fewer, smaller fires and more soil moisture, thus more biomass and more enclosed canopies. This leads toward increased trends of fire-sensitive and shade-tolerant taxa such as firs, Pseudotsuga menziesii (Douglas-fir), and Tsuga mertensiana (mountain hemlock). Climate-driven systems should follow these general trends due to vegetation life-histories.
Recent paleoecological works from environmental (not archaeological) contexts have looked specifically at disentangling cultural burning from climatic fire in mountainous California (Crawford et al., 2015; Klimaszewski-Patterson and Mensing, 2016, 2020; Knight et al., 2022). These studies were located near ecotones and used life history traits of vegetation to explore the relationship of taxa against their fire history traits and how they relate to climatic shifts over time.
2. Materials and methods
To synthesize and explore spatial and temporal cultural burning trends in the Sierra Nevada I identified existing pollen-based paleoecological sites from the Neotoma Paleoecology Database (www.neotomadb.org). The sites needed to meet the following criteria:
• Occur in the central or southern Sierra Nevada, California.
• Average < 100 years between samples (sub-centennial) within the last 1,100 years.
• Have at least one radiocarbon date within the last 2,000 years.
• Have published data in a peer-reviewed journal.
I downloaded pollen datasets for each site identified above and used R Statistical Software (R Core Team, 2023) for analysis. Datapoints are identified by the median date assigned to them from their study's statistical age model and are not representative of the range of dates possible within 95% confidence. To explore vegetation response in relation to climate I limited each dataset to the last 1,100 years because the climate reconstruction used is most reliable through this time. Unless otherwise stated, all dates are in calendar years C.E.
2.1. Climate-vegetation dynamics
I created a climate reconstruction and vegetation response index (VRI) for each site to explore the response of fire in/sensitive taxa in relation to climate (Figure 1). The climate reconstruction for the last 1,100 years comes from the North American Drought Atlas (Cook et al., 1999, 2004; Herweijer et al., 2007), a gridded 2.5° network of tree ring-derived climate reconstructions. Each year is reported with a value, where more positive values indicate wetter and/or cooler conditions and negative values indicate drier and/or warmer conditions. I created a 50-year smoothing spline to compare with VRI.
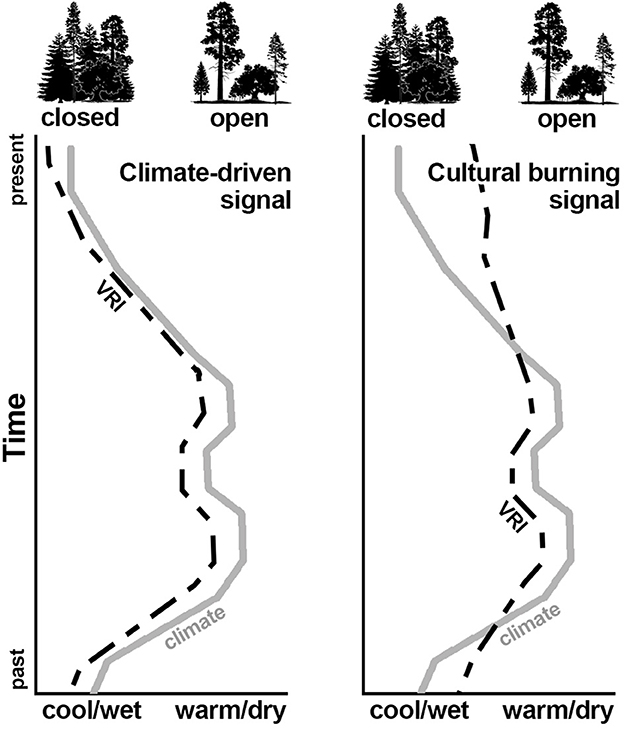
Figure 1. Conceptual model showing expected vegetation response (VRI) under climate-driven (left) vs. human-influenced (right) scenarios.
I calculated VRI for each site with an established formula (Klimaszewski-Patterson and Mensing, 2016; Klimaszewski-Patterson et al., 2021) using ecologically unambiguous pollen taxa to generate an index between indicators of closed (fir, Douglas-fir, mountain hemlock) and open (oak) canopy taxa for mid-elevation mixed conifer forests in the Sierra Nevada, such that
A positive VRI indicates a more closed-canopy and a negative VRI indicates a more open canopy.
In a climate-driven regime, I expect more firs, Douglas-fir, and mountain hemlock (closed canopy) during cooler, wetter periods and more oaks (open canopy) during warmer, drier periods. Persistence of oaks during climatically cool, wet periods is considered evidence for cultural burning. Cupressaceae (cypress family) is not used in the open canopy calculations because the pollen cannot be distinguished from other species in the family [e.g., Juniperus osteosperma (Utah juniper), California incense-cedar] that may be in the pollen rain but have confounding life history traits. It remains difficult to identify cultural burning during climatically warm, dry periods because climate is already conducive to economic resource habitats. For each site I generated a 50-year smoothing spline of VRI and normalized the data for plotting against the climate reconstruction. I use these plots to visually identify periods of cultural burning for each site and to look for general trends in cultural burning across sites.
2.2. Cluster analysis of vegetation over time
I performed a k-means cluster analysis of prevalent, non-correlated taxa as an alternative, statistically-based approach to identify pollen clusters and whether the clusters were more consistent with climate or cultural burning expectations. k-means clustering is a data reduction technique that iteratively processes data points into clusters based on their similarity by minimizing the sum of squares to the cluster centroid (Hartigan and Wong, 1979). In other words, k-means clustering minimizes variance within clusters and maximizes variance between clusters. To reduce signal noise produced by infrequently present taxa, I filtered each pollen dataset to include only taxa accounting for at least 4% of the terrestrial pollen sum and removed highly correlated taxa. I scaled the data and then used the “factoextra” R package v1.0.7 to determine the optimal number of clusters (Kassambara and Mundt, 2020). The quality of k-means partition value is a goodness of fit, calculated as percentage of between sum of squares divided by the total sum of squares of the clusters. I used the kmeans function in R to perform the cluster analysis (R Core Team, 2023) and “factoextra” to visualize the clusters using principal components on two axes. When graphed, each axis represents the percentage of variance on that dimension. Data points within clusters are considered more similar in n-dimensional space, and clusters closer together are more related than those further apart.
If pollen samples cluster together consistent with climate periods (e.g., LIA datapoints cluster separately from MCA datapoints) then climate is identified as the primary driving force; however, if an LIA datapoint clusters with MCA dates, then that datapoint shares a pollen composition more in common with the MCA and likely indicates a period of cultural burning. This approach is an alternative data analysis technique because it does not limit data to only specific open vs. closed canopy taxa like VRI.
3. Results
Five sites (Figure 2) were identified from the Neotoma Paleoecology Database that met all criteria, which all happen to occur on federally-protected lands. Two sites are located in in the southern Sierra Nevada within Sequoia National Forest: Holey Meadow (HLY; Klimaszewski-Patterson and Mensing, 2016) and Trout Meadow (TRT; Klimaszewski-Patterson and Mensing, 2020). Three sites are located in the central Sierra Nevada within Yosemite National Park: Wawona Meadow (WAM; Anderson and Stillick, 2013), Woski Pond (WOS; Anderson and Carpenter, 1991), and Swamp Lake (SWL; Smith and Anderson, 1992). All sites except for Wawona Meadow have records that extend the full 1,100-year period of analysis.
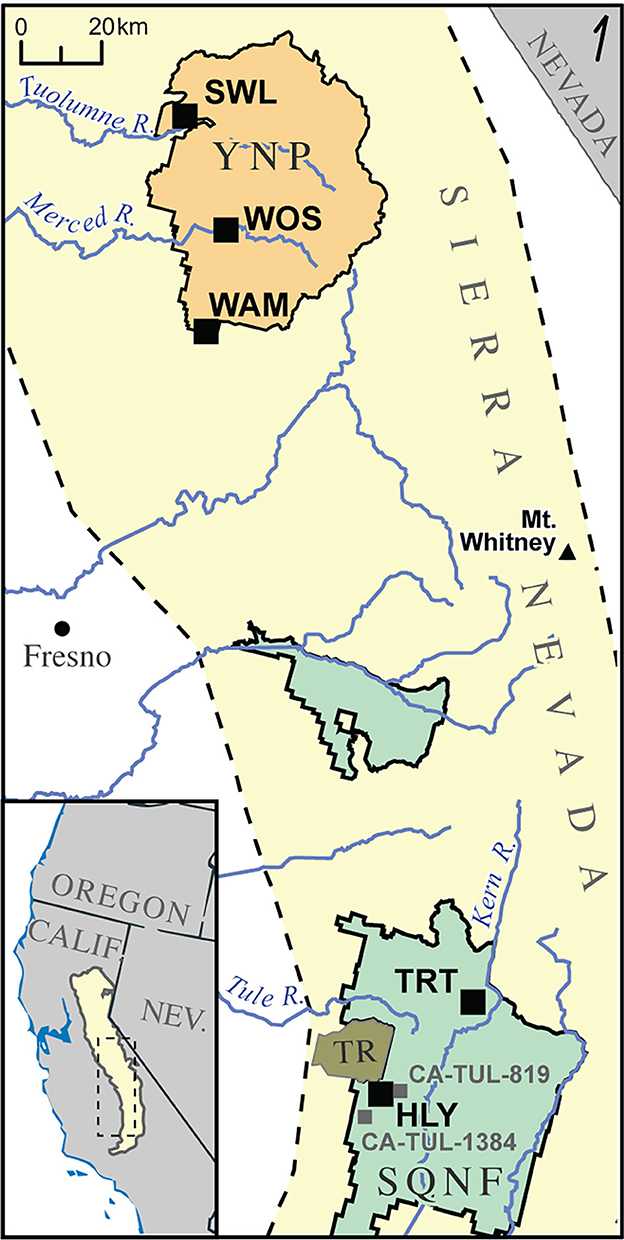
Figure 2. Map of paleoecologic sites (black squares) and archaeological sites (gray squares) discussed in this paper. Yellow background indicates the Sierra Nevada range. HLY, Holey Meadow; TRT, Trout Meadow; WAM, Wawona Meadow; WOS, Woski Pond; SWL, Swamp Lake; CA-TUL-1384, Hot Springs Station; CA-TUL-813, Johnsondale Work Station. SQNF, Sequoia National Forest (green area); TR, Tule River Reservation (olive area); YNP, Yosemite National Park (orange area).
3.1. Holey Meadow
The downloaded dataset for Holey Meadow (HLY; Figure 2) has 25 samples averaging 44.1 years between samples within the last 1,100 years. The original publication indicates a well-dated subset with five macrofossil radiocarbon dates in the top 1,800 years, three in the last 1,100 years.
Climate-vegetation dynamics analysis (Figure 3) indicates a general trend of human impacts from ca. 1400 to the present day. There are three possible periods of more cultural burning during the LIA (ca. 1290–1350, 1410–1750, and 1820–1850) and one possible period during the MCA (ca. 1090–1150). Variations in VRI during the Modern period (1850-present) capture known historical human impacts such as Euroamerican settlement, fire suppression, and lumber activities (R-Ranch in the Sequoias, 2022).
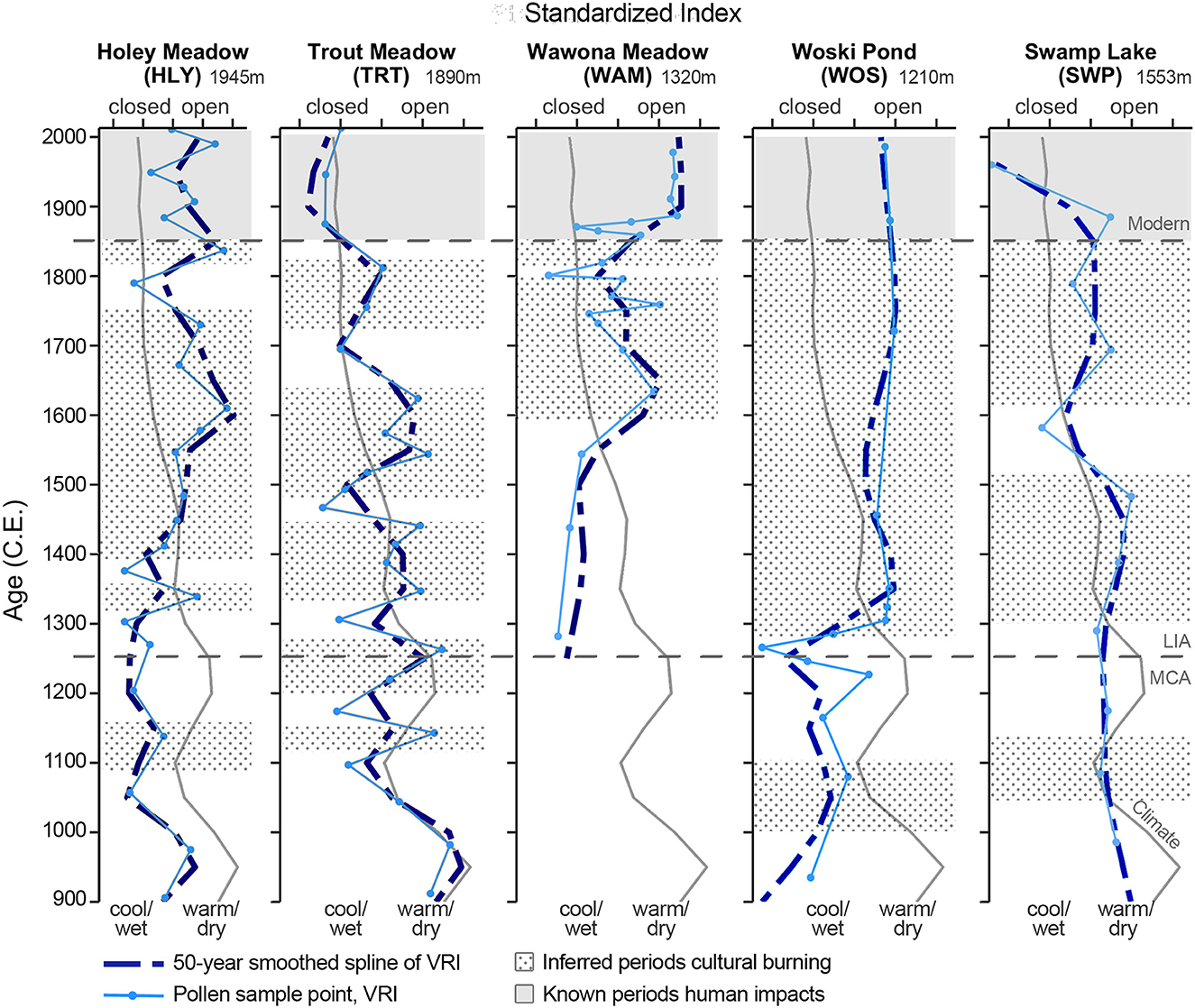
Figure 3. Comparisons of climate (gray line) vs. vegetation response index (VRI) for each site. Dotted backgrounds indicate inferred periods of cultural burning. LIA, Little Ice Age; MCA, Medieval Climate Anomaly.
Cluster analysis produced five clusters with a quality of k-means partition value of 72.3% (Figure 4). Six taxa contribute to the cluster analysis: pines, Cupressaceae, white fir [and possibly A. magnifica (red fir)], oak, Rosaceae (rose family), and Poaceae (grasses) (Table 1). The Modern period is entirely found within clusters 1 (1884–1928, 1990) and 4 (1949, 2011), with both high oak and fir, and low pine contributions. Cluster 2 (1057–1138, 1303, 1376) has moderate representation of taxa, with higher contribution of grass and pine. Cluster 3 (906–975, 1204–1270, 1339, 1412–1449, 1547–1578, 1837) has high pine, moderate fir, and low grass contributions. Cluster 5 (1484, 1672–1790) has high Cupressaceae and rose family contributions.
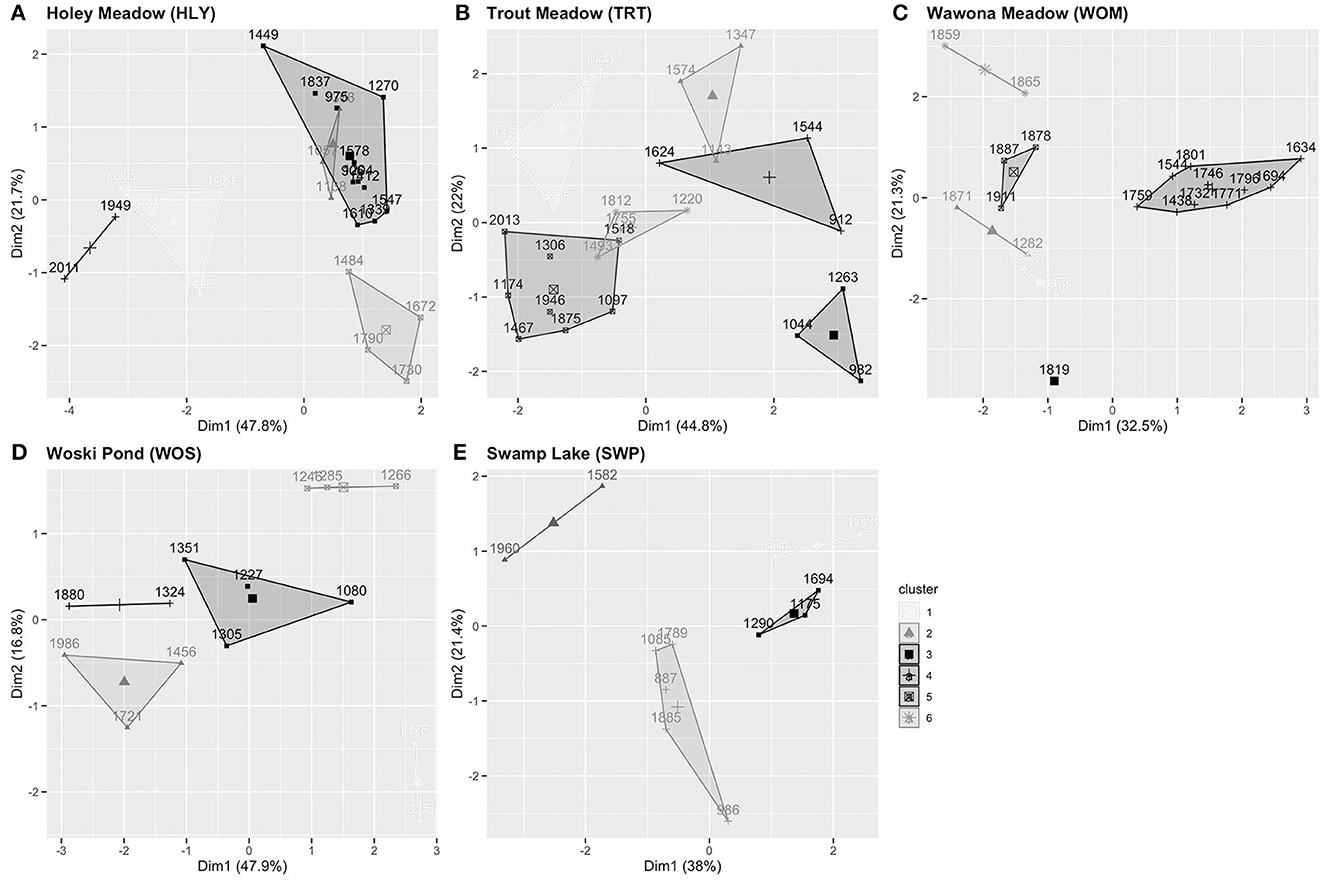
Figure 4. k-means clustering labeled by year. Observations use principal components, with axes indicating how much variance is accounted for in that dimension. Cluster information is based on unsupervised classification within a site and cluster/symbology information does not transfer between sites. (A) Holey Meadow, (B) Trout Meadow, (C) Wawona Meadow, (D) Woski Pond, (E) Swamp Lake.
3.2. Trout Meadow
Trout Meadow (TRT; Figure 2) has 25 samples averaging 43.7 years between samples within the last 1,100 years. Original publication indicates a well-dated subset with seven macrofossil radiocarbon dates in the top 1,700 years, four in the last 700 years.
Anomalies in the climate-vegetation dynamics analysis (Figure 3) indicate sporadic cultural burning since 1100. There are three periods of more cultural burning during the LIA (ca. 1320–1470, 1490–1610, and 1710–1810), one possible period during the MCA-LIA transition (ca. 1200–1270), and one possible during the MCA (ca. 1110–1150).
Cluster analysis produced four clusters with a quality of k-means partition value of 70.7% (Figure 4). Seven taxa contribute to the cluster analysis: pine, Cupressaceae, (white) fir, oaks, rose family, Asteraceae (sunflower family, typically open areas, disturbance indicator), and grasses (Table 2). Cluster 1 (1388–1441, 1695) shows higher contributions of pine and rose family, and low Cupressaceae and grass. Clusters 2 (1143, 1347, 1574), 3 (982–1044, 1263), and 4 (912, 1544) indicate open forest canopies, with different contributions of taxa distinguishing between the clusters. Cluster 5 (1097, 1174, 1306, 1467, 1518, 1875–2013) has high contributions of pine and fir, and low oak and rose family. Cluster 6 (1263, 1493, 1755–1812) has moderate contributions of fir and Cupressaceae, low Asteraceae, and high rose family.
3.3. Wawona Meadow
Wawona Meadow (WAM; Figure 2) has 20 samples averaging 34.1 years between samples over the last 800 years. Original publication indicates a well-dated dataset with three macrofossil radiocarbon dates and lead-210.
Anomalies in the climate-vegetation dynamics analysis (Figure 3) indicate cultural burning beginning ca. 1590–1850, and known settlement and logging activity from ca. 1860 to present-day. A rapid closed signal in VRI briefly occurs around 1800 and just after 1850. The record only encompasses the LIA.
Cluster analysis produced six clusters with a quality of k-means partition value of 71.7% (Figure 4). Nine taxa contribute to the cluster analysis: pines, Cupressaceae, firs, oaks, Alnus spp. (alders, grow along streams), Salix spp. (willows, grow along margins of lakes and meadows), rose family, Asteraceae, and grasses (Table 3). Clusters 1 (1943–1978), 5 (1878–1911), and 6 (1859–1865) occur during the Modern period, all with pine contributions notably lower than the pre-Modern period. Cluster 2 (1282, 1871) has a high contribution of fir, and low contributions of oak and Cupressaceae. Cluster 3 (1819) has high contributions of alder and pine. Cluster 4 (1438–1801) has high contributions of willow and Cupressaceae, moderate Asteraceae and pine, and moderately low oak, fir, and grass contributions.
3.4. Woski Pond
Woski Pond (WOS; Figure 2) has 14 samples averaging 69.9 years between samples. Original publication indicates three bulk radiocarbon dates in the last 1,500 years, three in the last 1,100 years.
Anomalies in the climate-vegetation dynamics analysis (Figure 3) generally indicate a rapid signal toward cultural burning starting at ca. 1290 to 1850s, and known settlement and human activity from ca. 1850 to present-day. There is a possible period of cultural burning during the MCA from ca. 1000 to 1100.
Cluster analysis produced five clusters with a quality of k-means partition value of 71.0% (Figure 4). Eight taxa contribute to the cluster analysis: pines, Cupressaceae, firs, oaks, alders, Populus spp. (poplar family, includes aspen and cottonwoods, typically along streambanks), rose family, and Ceanothus spp. (buckthorn family, includes whitethorn ceanothus, typically chaparral or open conifer forests) (Table 4). Cluster 1 (935, 1165) has high contributions of pine, fir, alder, and Populus, with low oak, Cupressaceae, and Ceanothus. Cluster 2 (1456–1721, 1986) has low pine and fir, and high oak and rose family contributions. Cluster 3 has low fir and rose family, and moderate Ceanothus contributions. Cluster 4 (1324, 1880) contains low pine, fir, and Ceanothus, and high oak and Cupressaceae contributions. Cluster 5 (1246–1285) has high pine and fir, with low oak, alder, rose family, and Cupressaceae contributions.
3.5. Swamp Lake
Swamp Lake (SWP; Figure 2) has 12 samples averaging 87.8 years between samples. Original publication indicates one bulk radiocarbon date in the last 1,800 years.
Anomalies in the climate-vegetation dynamics analysis (Figure 3) generally indicate cultural burning initiates at least ca. 1300. Two periods during the LIA may indicate more cultural burning: ca. 1300–1500 and ca. 1610–1880. The consistency of signal during the pluvial period of the MCA may indicate some cultural burning preserving oak habitat during that time.
Cluster analysis produced four clusters with a quality of k-means partition value of 60.7% (Figure 4). Seven taxa contribute to the cluster analysis: pines, Cupressaceae, firs, oaks, alders, rose family, and grasses (Table 5). Cluster 1 (1388–1483) has high rose family, oak, and Cupressaceae, low fir, pine, and grass contributions. Cluster 2 (1582, 1960) has high contributions of fir and pine, and low Cupressaceae, oak, and grass. Cluster 3 (1175–1290, 1694) has low pine and rose family, and high Cupressaceae contributions. Fir, oak, and grass contributions are moderately low. Cluster 4 (986–1085, 1789–1885) has overall moderate contributions, with the highest values from grass and alder.
4. Discussion
Each paleoecologic site analyzed in this paper indicates periods of cultural burning via VRI, and the interpretation is supported by k-means cluster analysis via LIA datapoints appearing in non-closed canopy clusters. These paleoecologic inferences broadly correspond with regional or local archaeological temporal inferences, and in some cases may help refine archaeological interpretations.
4.1. Southern Sierra Nevada site summaries and archaeological contexts
The southern Sierra Nevada region includes Holey Meadow and Trout Meadow paleoecologic sites, both managed by Sequoia National Forest (established 1908). Both sites are long, spring-fed sedge meadows. Holey Meadow, located near the Tule River Reservation (established 1873), is situated on the western slope of the western Sierra Crest (Klimaszewski-Patterson and Mensing, 2016). Trout Meadow is located within the double crest of the southern Sierra Nevada in the Golden Trout Wilderness Area (Klimaszewski-Patterson and Mensing, 2020). Both of these paleoecologic studies were originally designed specifically to examine the effects to cultural burning within the sedimentary record. These sites were intentionally located near archaeological sites and at the upper ecotone of California black oak, a tree that produces a highly prized acorn known for its sweet flavor. Holey Meadow is located along the southern third of traditionally-defined Tubatulabal and Foothill Yokuts territory (Harvey, 2018) while Trout Meadow is located at the traditionally-defined northwestern periphery of Tubatulabal territory.
4.1.1. Holey Meadow
Holey Meadow (elev. 1,945 m) shows the strongest signal for continuous cultural burning during the LIA with a nearly continuous elevated VRI from 1400 to 1850. The cluster analysis showed ecologically unexpected overlap in vegetation communities between the MCA and LIA that are consistent with anomalies in the climate-vegetation dynamics analysis. Clusters 1 and 4 (1884–2013) represent the Modern period and are distinct from any times preceding. Cluster 2 contributions likely indicate more closed forest canopy and are consistent with changes in VRI. Clusters 3 and 5 (906–975, 1204–1270, 1339, 1412–1837) occur during both the MCA and the LIA, and indicate open forest canopy.
The reported sedimentary charcoal record trends in agreement with climate reconstructions and not VRI; therefore charcoal-producing fire is explained by climate, and neither climate nor charcoal explain the variation in vegetation. The openness of forest canopy is best explained by low-intensity cultural burning and not climatic fire.
Two archaeological sites occur within 8 km of Holey Meadow (Figure 2): CA-TUL-1384 (Hot Springs Station; Moskowitz et al., 1998; Kraus, 2016) and CA-TUL-819 (Johnsondale Work Center; Ramirez et al., 2010). The Hot Springs Station site (elev. 1,150 m) is located 7.8 km southwest of Holey Meadow in foothill woodlands while the Johnsondale Work Center site (elev. 1,425 m) is roughly four miles east of Holey Meadow on the eastern side of the western crest. Holey Meadow is debated as used by both Foothill Yokuts and Tubatulabal groups, with the Hot Springs Station site presumed to be Foothill Yokut (Moskowitz et al., 1998) and the Johnsondale Work Center site as Tubatulabal (Ramirez et al., 2010). Both sites indicate site use within two phases during this period of analysis: Sawtooth Phase (600–1300) and Chimney Phase (1300–1850). The Johnsondale Work Center site has a cluster of obsidian hydration (OH) dates between 1208 and 1453, which center along with VRI-inferred cultural burning at Holey Meadow from ca. 1300 to 1350. Inferred cultural burning (via negative VRI) from ca. 1425 to 1750 overlaps with OH dates during the Chimney Phase at the Hot Springs Station site. Given its location, it is possible that Holey Meadow was used by both groups over time, and that this may have led to the observed signal of consistent cultural burning from 1400 at Holey Meadow.
4.1.2. Trout Meadow
Trout Meadow (elev. 1,890 m) shows episodic periods of cultural burning in VRI that coincide with OH dates taken from archaeological excavations (CA-TUL-2027/2077) adjacent to the meadow and previously reported by Kraus (2016). Of the 68 OH dates calculated at Trout Meadow, 20 occur during the last 1,100 years and all coincide with inferred periods of cultural burning. While I recognize the controversy in converting OH dates to calendar years (Stevenson et al., 2000; Rogers, 2010), and that the OH sample size for this period of analysis is small, it is interesting that the OH dates by happenstance occur during the same periods of time when I interpret cultural burning from the pollen record.
Charcoal in the original publication was processed using a different method (chemical digestion vs. quantification) than the other sites in this analysis and is not readily comparable. The previously published results indicate no relationship of charcoal with either vegetation or climate.
Episodic evidence of cultural burning at Trout Meadow supports archaeological inferences of mobile groups having large territories used intermittently at the periphery (Voegelin, 1938; Harvey, 2018). Per Voegelin (1938, p. 39), the northern two-thirds of the territory was predominantly used as hunting range, though evidence of bedrock mortars adjacent to Trout Meadow indicate the site was also used for resource procurement and processing (Kraus, 2016). Both southern Sierran sites show consistency in interpretation between anomalies in climate-vegetation dynamics and cluster analysis.
4.2. Northern Sierra Nevada site summaries and archaeological contexts
The northern Sierra Nevada region includes Woski Pond, Wawona Meadow, and Swamp Lake, all located in Yosemite National Park (established 1890). Woski Pond was small oxbow depression of the Merced River in a meadow within Yosemite Valley (Anderson and Carpenter, 1991). Wawona Meadow is a long, wet meadow, similar to Holey and Trout Meadows, located on the western slope near the southern border of the park (Anderson and Stillick, 2013). Swamp Lake is a mid-elevation lake located on the western slope near the northern border of the park. It is situated just west of Hetch Hetchy Reservoir, which lies along the Tuolumne River, and just south of Lake Eleanor (Smith and Anderson, 1992). These three studies were originally conducted as paleoenvironmental reconstructions and were not initially designed to test effects of cultural burning.
4.2.1. Woski Pond
Woski Pond (elev. 1,210 m) shows the strongest signal of cultural burning during the LIA of the three northern Sierra sites. VRI indicates continuous human impacts from 1300 to present-day. k-means cluster analysis is consistent with VRI interpretations. Clusters 1 and 5 (935, 1165, 1246–1285) indicate a closed canopy forest, while contributions to clusters 2, 3, and 4 indicate open canopy forests. I should note that the cluster analysis also responds on the extra-local relationship between the oxbow lake and the stream, as cluster 1 includes high contributions of alder and Populus, which typically grow along streams.
Anderson and Carpenter (1991) report greatest charcoal abundance from 400 to 1300, with maximum abundance at 1300. Maximum charcoal accumulation at 1300 explains the rapid shift in VRI but does not explain the prevalence of open-canopy taxa during the LIA; therefore, the pollen record is indicative of cultural burning during the LIA.
The original publication indicates that there are several archaeological sites in the immediate vicinity of Woski Pond dating to 2,000 to 3,000 years ago. The authors note a change in culture phase from Tamarack to Mariposa occurs around 1300–1350, correlating to the maximum charcoal and pollen signal shift to oak dominated. The Mariposa phase is associated with increased population and increase in resource procurement and economic development and corresponds with migration of the Miwok south into the Yosemite area (Anderson and Carpenter, 1991; Moratto, 1999; Gassaway, 2009). Given that Yosemite Valley in considered to have some of the highest population density in modern-day Yosemite National Park (Anderson and Moratto, 1996; Hull, 2005), I find archaeological and paleoecologic evidence are in agreement with the timing of events for increased populations, resource intensification, and cultural burning at Woski Pond.
4.2.2. Wawona Meadow
Wawona Meadow (elev. 1,320 m) shows a general shift to more open forest canopy than expected at around 1550. Specific VRI shows a slowly closing canopy from 1625 to 1750, possibly due to reduced levels of cultural burning nearby, and a rapidly closed canopy around 1800 which could be from a lack of nearby burning.
Clusters 1, 5, and 6 encompass the Modern period, from 1859 to 1978, with low pine but moderate fir signals, possibly a result of mechanical clearing. This is consistent with historical accounts of several homesteaders and a hotel in the area by 1890 when the meadow was incorporated into Yosemite National Park. Homesteaders sold timber rights to a lumber company which operated in the area from 1897 to the 1930s (Anderson and Stillick, 2013). A golf course opened in 1918, and the meadow continued to be developed with a sewage pond, tank, and fencing in the 1970s (Whittaker and Huckell, 1981).
Contributions to clusters 2 and 3 (1282, 1819–1871) indicate a closed canopy forest, while cluster 4 (1438–1801) indicates open canopy, primarily via disturbance taxa. The low pollen contributions of oak and fir in this cluster likely explain the difference in timing of cultural burning between the climate-vegetation dynamics analysis (1550) and cluster analysis (1438). Anderson and Stillick (2013) report low charcoal influx prior to 1375 with 12 fire events between 1375 and 1800.
Charcoal does not explain changes in vegetation at Wawona Meadow. The closing canopy signal in the VRI between 1625 and 1750 does not correspond with reported charcoal, which indicates five events during this period. Anderson and Stillick note that the charcoal reconstruction corresponds well with regional fire scar studies (Swetnam, 1993; Swetnam et al., 2009), further supporting my argument that charcoal likely reflects climate and does not necessarily capture established cultural burning. Therefore, both pollen-based methods find evidence for cultural burning from 1550 to 1800.
Unfortunately, Anderson and Stillick do not provide more detailed archaeological information than already established at Woski Pond. An undated application for the National Register of Historic Places of Wawona Archaeological District (http://npshistory.com/publications/yose/nr-wawona-ad.pdf) indicates that archaeological investigations have been limited to surface inventory surveys. Diagnostic artifacts in the district are mostly characteristic of the Tamarask-Mariposa culture phase shift (Moratto, 1980; Whittaker and Huckell, 1981), which pre-dates the cultural burning signal by ~200 years. Thus, at Wawona Meadow, paleoecology may provide missing temporal data via interpretations of the pollen record that can be used for archaeological interpretations because of the paucity of archaeological excavations.
4.2.3. Swamp Lake
Swamp Lake (elev. 1,540 m) shows two general periods of cultural burning, from ca. 1300–1500 and ca. 1600–1880. The canopy closed slightly around 1550, potentially indicating a brief hiatus in burning, but in general VRI during the LIA is comparable to, or indicates more open conditions, than during the MCA. The sharp closing trend in forest canopy during the Modern period likely demonstrates fire suppression policy since 1910 (Silcox, 1910).
Cluster analysis produces four clusters. Contributions to clusters 1, 3, and 4 (887–1483, 1694–1885) indicate periods of open canopy. Cluster 2 (1582, 1960), with its high fir and pine contributions indicates a closed canopy. Again, cluster analysis is consistent with anomalies in the climate-vegetation dynamics analysis.
I have synthesized the minimal publicly available archaeological interpretations in the vicinity of Swamp Lake to provide context because the original study focused on reconstructing 16,000 years of vegetation. Bennyhoff (1956, p. 7) reports that the Big Creek Miwok and Paiutes disputed the territory of Hetch Hetchy Valley in the late 1800s, indicating pre-Modern site use surrounding Swamp Lake. Although archaeological sites are present near Lake Eleanor and Hetch Hetchy, I am unable to find more recent and available temporal data associated with the sites, as information is broadly synthesized throughout the park. As with Wawona Meadow, paleoecology might provide evidence of cultural burning, and could potentially provide inferences of occupation that are missing from the archaeological record due to limited excavations and dating materials from archaeological contexts.
4.3. Case studies in testing qualitative hypotheses using paleolandscape modeling
The previous sections discussed paleoecology-based interpretations of cultural burning by indigenous peoples for the last 1,100 years against archaeological interpretations of site use. Like archaeology, paleoecology is an empirical science and while these interpretations are well-informed, they are based on qualitative hypotheses. This section examines the potential for paleolandscape modeling to test drivers of change with respect to paleoenvironmental hypotheses, and whether modeling results support or fail to support earlier inferences of cultural burning at two of the sites discussed above.
Two previously published paleolandscape modeling studies at Holey Meadow (Klimaszewski-Patterson et al., 2018) and Trout Meadow (Klimaszewski-Patterson and Mensing, 2020) provide additional support for cultural burning. These studies used LANDIS-II, a spatially-explicit stochastic landscape change model (Scheller and Mladenoff, 2004; Scheller et al., 2011) used by forest managers and researchers to simulate forest growth, disturbance, management policies, and land use change. While LANDIS-II is typically used to forecast change, these studies utilized the model to test the drivers of forest change over the last 1,500 years. In other words, whether climate alone or the addition of cultural burning at each site best approximated the pollen record. All modeled scenarios included 20–40 iterations that were averaged for analysis, and each scenario allowed for climatic, lightning-caused fire, with the number of potential lightning strikes scaled linearly with climate. Earlier state-wide fire history research indicated that 6–16% of pre-Columbian California burned annually (Kilgore and Taylor, 1979; Martin and Sapsis, 1992). Because the southern Sierra Nevada were considered to have had low population densities given that the Foothill Yokuts and Tubatulabal were mobile, scenarios that tested cultural burning allowed up to 6% area cumulatively burned, inclusive of climatic, lightning-caused fires.
At Holey Meadow, Klimaszewski-Patterson et al. (2018) tested three hypotheses in the model using the follow scenarios: (1) no cultural burning (climate only), (2) consistent cultural burning throughout the MCA and LIA, and (3) only during the wet periods such as the LIA, from 1200 to 1850. They found that modeled results of no cultural burning (climate only) provided the worst fit to empirical VRI, and that burning throughout the MCA and LIA has moderately significantly negative correlations. Strong statistical significance and the best fit to empirical pollen data came from applying up to 6% cultural burning only during wet climatic periods, specifically preceding the MCA and during the LIA.
At Trout Meadow, Klimaszewski-Patterson and Mensing (2020) tested three hypotheses in the model using the follow scenarios: (1) no cultural burning (climate only), (2) cultural burning only during the wetter pre-MCA and LIA when VRI exceeded climate expectations (400–700, 850–900, 1200–1250, 1300–1400, 1500–1600, 1700–1800) and (3) cultural burning during all periods when VRI exceeded climate expectations (all dates as #2 including 850–950 and 1100–1150). Unlike Holey Meadow they did not test consistent cultural burning throughout the MCA and LIA because the pollen reconstruction at Trout Meadow did not support such a pattern. They found that while climate alone was not a poor model fit, scenarios 1 (no cultural burning) and 3 (cultural burning during all periods where VRI exceeded expectation) were not correlated at statistical significance with the observed pollen reconstruction. Scenario 2 (cultural burning only during climatically wet periods) produced modeled results that had statistically significant correlation with empirical pollen data.
Both published studies demonstrated the potential for paleolandscape modeling to test hypotheses and inferences of climatic fires and cultural burning against the observed pollen record. Both paleolandscape modeling studies demonstrated that climate alone could not explain the observed changes in forest composition, and both studies support the inferences made by climate-vegetation dynamics via VRI and k-means clustering approaches. The modeling approach allowed for synthetic hypothesis testing, which is uncommon in empirical disciplines such as paleoecology and archaeology. Further work needs to be conducted in this realm to test cultural burning hypotheses at sites outside Sequoia National Forest, but the method has shown promise in further elucidating and detangling human influence from climate drivers in the environmental record.
4.4. Limitations
In the Neotoma Paleoecology Database, only five out of 14 central and southern Sierra Nevadan paleoecology sites met the requirement of published sub-centennial vegetation reconstructions in the last 1,100 years with a radiocarbon date within the last 2,000 years. This dearth of high-temporal, reasonably dated paleoecologic reconstructions limits interpretations of this approach across the Sierran range and thus cannot provide insight as to whether indigenous groups extensively altered ecosystems via cultural burning. Additional well-dated sites are needed to provide interpretations both across different culture groups and across the Sierran range.
These analyses are limited to published sub-centennial reconstructions, and not all sites are reconstructed at comparable temporal resolutions or situated in locations to test the effects and extent of cultural burning. The average time between samples varies from 34.5 to 87.8 years, with longer and shorter gaps between specific samples. Furthermore, the types of prevalent vegetation vary between the sites, with some sites more prolific in riparian vegetation which influence clustering analysis, while others have minimal fir or oak pollen percentages.
5. Summary
This paper explores the potential of paleoecology, specifically pollen, in identifying periods of cultural burning used by mobile populations over the last 1,100 years. To achieve this, I used five existing paleoecologic studies from the southern and central portions of the Sierra Nevada range in California that are currently available in the Neotoma Paleoecology Database. In this study area, the Little Ice Age (LIA) climate should have favored closed-canopy forest taxa such as fir, Douglas-fir, and mountain hemlock, over open-canopy taxa such as oak. Yet each of the five sites shows the preservation and greater persistence than expected of open-canopy forest taxa. I argue that this is because low-intensity cultural burning was used to intentionally maintain the oak woodlands of the Medieval Climate Anomaly (MCA), and that cultural burning is not captured via sedimentary charcoal, but rather in the pollen record.
A second goal of this paper was to explore methods that could help improve archaeological interpretations of human-fire dynamics via paleoecologic inferences. As case studies, these sites indicate that climate-vegetation dynamics via VRI and k-means clustering approaches show the ability to identify periods of cultural burning that may supplement or support archaeological data. Further, paleolandscape modeling at two of the case study sites shows promise at testing the veracity of hypothesized periods of cultural burning, and whether people or climate best explain the empirical pollen and charcoal records. In some places paleoenvironmental reconstructions may provide more robust dating that can be applied to archaeological interpretations, especially where excavations are limited.
Data availability statement
The datasets presented in this study can be found in online repositories. The names of the repository/repositories and accession number(s) can be found in the article/supplementary material.
Author contributions
AK-P contributed to conception, design, statistical analysis of the study, wrote, revised, and approved the submitted manuscript version.
Conflict of interest
The author declares that the research was conducted in the absence of any commercial or financial relationships that could be construed as a potential conflict of interest.
The handling editor AS declared a past co-authorship with the author.
Publisher's note
All claims expressed in this article are solely those of the authors and do not necessarily represent those of their affiliated organizations, or those of the publisher, the editors and the reviewers. Any product that may be evaluated in this article, or claim that may be made by its manufacturer, is not guaranteed or endorsed by the publisher.
References
Abrams, M. D., and Nowacki, G. J. (2020). Native American imprint in palaeoecology. Nat. Sustain. 3, 896–897. doi: 10.1038/s41893-020-0578-6
Anderson, M. K., and Moratto, M. J. (1996). “Native American land-use practices and ecological impacts,” in Sierra Nevada Ecosystem Project: final report to Congress (Davis: University of California, Centers for Water and Wildland Resources), 187–206.
Anderson, R. S., and Carpenter, S. L. (1991). Vegetation change in Yosemite Valley, Yosemite National Park, California, during the Protohistoric period. Madroño 38, 1–13.
Anderson, R. S., and Stillick, R. D. (2013). 800 years of vegetation change, fire and human settlement in the Sierra Nevada of California, USA. Holocene 23, 823–832. doi: 10.1177/0959683612471985
Baumhoff, M. A. (1963). Ecological determinants of Aboriginal California populations. Am. Archaeol. Ethnol. 49, 155–236.
Bennyhoff, J. A. (1956). Reports of the University of California Archaeological Survey: An Appraisal of the Archaeological Resources of Yosemite National Park. Berkeley, CA: The University of California Archaeological Survey.
Binford, L. R. (2001). Constructing Frames of Reference: An Analytical Method for Archaeological Theory Building Using Hunter-Gatherer and Environmental Data Sets. Berkeley, CA: University of California Press.
Bolton, H. E. (1967). Spanish exploration in the Southwest, 1542-1706. New York, NY: Barnes and Noble, Inc.
Clar, C. R. (1959). California Government and Forestry: From Spanish Days Until the Creation of the Department of Natural Resources in 1927. Sacramento, CA: Division of Forestry; Department of Natural Resources; State of California.
Cook, E. R., Meko, D. M., Stahle, D. W., and Cleaveland, M. K. (1999). Drought reconstructions for the continental United States. J. Clim. 12, 1145–1163. doi: 10.1175/1520-0442(1999)012<1145:DRFTCU>2.0.CO
Cook, E. R., Seager, E., Cane, M. A., and Stahle, D. W. (2007). North American drought: reconstructions, causes, and consequences. Earth Sci. Rev. 81, 93–134. doi: 10.1016/j.earscirev.2006.12.002
Cook, E. R., Woodhouse, C. A., Eakin, C. M., Meko, D. M., and Stahle, D. W. (2004). Long-term aridity changes in the western United States. Science 306, 1015–1018. doi: 10.1126/science.1102586
Coughlan, M. R., Magi, B., and Derr, K. (2018). A global analysis of hunter-gatherers, broadcast fire use, and lightning-fire-prone landscapes. Fire 1, 41. doi: 10.3390/fire1030041
Crawford, J. N., Mensing, S. A., Lake, F. K., and Zimmerman, S. R. (2015). Late Holocene fire and vegetation reconstruction from the western Klamath Mountains, California, USA: a multi-disciplinary approach for examining potential human land-use impacts. Holocene 25, 1341–1357. doi: 10.1177/0959683615584205
Denevan, W. M. (1992). The pristine myth: the landscape of the Americas in 1492. Ann. Assoc. Am. Geogr. 82, 269–285. doi: 10.1111/j.1467-8306.1992.tb01965.x
Gassaway, L. (2009). Native American fire patterns in Yosemite Valley: archaeology, dendrochronology, subsistence, and culture change in the Sierra Nevada. SCA Proceed. 22, 1–19.
Gayton, A. H. (1948). Yokuts and western mono ethnography I: Tulare Lake, Southern Valley, and Central Foothill Yokuts. Anthropol. Rec. 10, 1–142.
Hartigan, J. A., and Wong, M. A. (1979). Algorithm AS 136: a K-means clustering algorithm. J. R. Stat. Soc. Ser. C 28, 100–108. doi: 10.2307/2346830
Harvey, D. C. (2018). Core-periphery dynamics in the Kern River watershed. Hunter Gatherer Res. 4, 557–587. doi: 10.3828/hgr.2018.33
Herweijer, C., Seager, R., Cook, E. R., and Emile-Geay, J. (2007). North American droughts of the last millennium from a gridded network of tree-ring data. J. Clim. 20, 1353–1376. doi: 10.1175/JCLI4042.1
Hull, K. L. (2005). Process, perception, and practice: time perspectivism in Yosemite native demography. J. Anthropol. Archaeol. 24, 354–377. doi: 10.1016/j.jaa.2005.06.003
Kassambara, A., and Mundt, F. (2020). Factoextra: Extract and Visualize the Results of Multivariate Data Analyses. Available online at: https://CRAN.R-project.org/package=factoextra
Kilgore, B. M., and Taylor, D. (1979). Fire history of a sequoia mixed-conifer forest. Ecology 60, 129–142. doi: 10.2307/1936475
Klimaszewski-Patterson, A., and Mensing, S. A. (2016). Multi-disciplinary approach to identifying Native American impacts on Late Holocene forest dynamics in the southern Sierra Nevada range, California, USA. Anthropocene 15, 37–48. doi: 10.1016/j.ancene.2016.04.002
Klimaszewski-Patterson, A., and Mensing, S. A. (2020). Paleoecological and paleolandscape modeling support for pre-Columbian burning by Native Americans in the Golden Trout Wilderness Area, California, USA. Landsc. Ecol. 35, 2659–2678. doi: 10.1007/s10980-020-01081-x
Klimaszewski-Patterson, A., Morgan, C., and Mensing, S. A. (2021). Identifying a pre-Columbian Anthropocene in California: a paleoecological and archaeological study of fire, vegetation change, and human settlement. Ann. Am. Assoc. Geogr. 11, 784–794. doi: 10.4324/9781003208211-18
Klimaszewski-Patterson, A., Weisberg, P. J., Mensing, S. A., and Scheller, R. M. (2018). Using paleolandscape modeling to investigate the impact of Native American–set fires on pre-Columbian forests in the southern Sierra Nevada, California, USA. Ann. Am. Assoc. Geogr. 4452, 1–20. doi: 10.1080/24694452.2018.1470922
Knight, C. A., Anderson, L., Bunting, M. J., Champagne, M., Clayburn, R. M., Crawford, J. N., et al. (2022). Land management explains major trends in forest structure and composition over the last millennium in California's Klamath Mountains. Proc. Natl. Acad. Sci. U. S. A. 119, e2116264119. doi: 10.1073/pnas.2116264119
Kraus, G. (2016). Ever upward?: lithics and intensification on the Western Divide (Thesis). University of Nevada Reno.
Kroeber, A. L. (1925). Handbook of the Indians of California. Washington, DC: Bureau of American Ethnology Bulletin No. 78.
Lewis, H. T. (1973). “Patterns of Indian burning in California: ecology and ethnohistory,” in Anthropological Papers No. 1, ed L. J. Bean (Ramona, CA: Ballena Press). Available online at: www.jstor.org/stable/673478 (accessed August 18, 2023).
Lightfoot, K. G., and Parrish, O. (2009). The First Fire Managers. 95th Edn. Berkeley, CA: University of California Press.
Martin, R. E., and Sapsis, D. B. (1992). “Fires as agents of biodiversity: pyrodiversity promotes biodiversity,” in Proceedings of the Symposium on Biodiversity of Northwestern California, 150–157.
Moratto, M. J. (1980). An Archaeological Research Program for Yosemite National Park, Part 1, Research Design. Tucson, AZ: Western Archeological and Conservation Center; National Park Service.
Moskowitz, K., Ptomey, S., and Garcia, J. (1998). Archaeological Investigations at CA-TUL-1384: The Uhl Station Site. Sequoia National Forest USDA Forest Service.
Oswald, W. W., Foster, D. R., Shuman, B. N., Chilton, E. S., Doucette, D. L., and Duranleau, D. L. (2020). Conservation implications of limited Native American impacts in pre-contact New England. Nat. Sustain. 3, 241–246. doi: 10.1038/s41893-019-0466-0
R Core Team (2023). R: A Language and Environment for Statistical Computing. Available online at: http://www.r-project.org (accessed August 18, 2023).
Ramirez, R. S., Murray, S., Dietler, J., and Steely, J. W. (2010). “Cultural resources inventory and eligibility assessment of the Johnsondale work center site, CA-TUL-819 (FS 05-13-53-08),” in SWCA Cultural Resources Report Database No. 2009-320 (South Pasadena, CA; Porterville, CA: USDA Forest Service; Sequoia National Forest; SWCA Environmental Consultants).
Rogers, A. K. (2010). Accuracy of obsidian hydration dating based on obsidian–radiocarbon association and optical microscopy. J. Archaeol. Sci. 37, 3239–3246. doi: 10.1016/j.jas.2010.07.023
R-Ranch in the Sequoias (2022). Building Johnsondale. R-Ranch in the Sequoias. Available online at: https://rranchinthesequoias.com/building-johnsondale/ (accessed August 18, 2023).
Scheller, R. M., and Mladenoff, D. J. (2004). A forest growth and biomass module for a landscape simulation model, LANDIS: design, validation, and application. Ecol. Model. 180, 211–229. doi: 10.1016/j.ecolmodel.2004.01.022
Scheller, R. M., Spencer, W. D., Rustigian-Romsos, H., Syphard, A. D., Ward, B. C., and Strittholt, J. R. (2011). Using stochastic simulation to evaluate competing risks of wildfires and fuels management on an isolated forest carnivore. Landsc. Ecol. 26, 1491–1504. doi: 10.1007/s10980-011-9663-6
Silcox, F. A. (1910). “Fire prevention and control on national forests,” in Yearbook of Department of Agriculture (Washington, DC: Government Printing Office).
Simpson, L. M., and Jones, T. L. (2020). Sedentism and settlement in native California: research progress and prospects. Calif. Archaeol. 12, 59–92. doi: 10.1080/1947461X.2020.1755188
Smith, S. J., and Anderson, R. S. (1992). Late Wisconsin paleoecologic record from Swamp Lake, Yosemite National Park, California. Quat. Res. 38, 91–102. doi: 10.1016/0033-5894(92)90032-E
Stepka, Z., Azuri, I., Kolska Horwitz, L., Chazan, M., and Natalio, F. (2022). Hidden signatures of early fire at Evron Quarry (1.0 to 0.8 Mya). Proc. Natl. Acad. Sci. 119, e2123439119. doi: 10.1073/pnas.2123439119
Stevens, N. E., Whitaker, A. R., and Rosenthal, J. S. (2019). Bedrock mortars as indicators of territorial behavior in the Sierra Nevada. Q. Int. 518, 57–68. doi: 10.1016/j.quaint.2017.11.008
Stevenson, C. M., Gottesman, M., and Macko, M. (2000). Redefining the working assumptions of obsidian hydration dating. J. Calif. Great Basin Anthropol. 22, 223–236.
Sugihara, N. G., Keeler-Wolf, T., and Barbour, M. G. (2018). “Introduction: fire and California vegetation,” in Fire in California's Ecosystems, eds J. W. van Wagtendonk, N. G. Sugihara, S. L. Stephens, A. E. Thode, K. E. Shaffer, and J. A. Fites-Kaufman (Berkeley, CA: University of California Press), 1–8.
Swetnam, T. W. (1993). Fire history and climate change in giant sequoia groves. Science 262, 885–889. doi: 10.1126/science.262.5135.885
Swetnam, T. W., Baisan, C. H., Caprio, A. C., Brown, P. M., Touchan, R., Anderson, R. S., et al. (2009). Multi-millennial fire history of the Giant Forest, Sequoia National Park, California, USA. Fire Ecol. 5, 120–150. doi: 10.4996/fireecology.0503120
Tubbesing, C. L., York, R. A., Stephens, S. L., and Battles, J. J. (2020). Rethinking fire-adapted species in an altered fire regime. Ecosphere 11, e03091. doi: 10.1002/ecs2.3091
Vachula, R. S., Russell, J. M., and Huang, Y. (2019). Climate exceeded human management as the dominant control of fire at the regional scale in California's Sierra Nevada. Environ. Res. Lett. 14. doi: 10.1088/1748-9326/ab4669
Vale, T. R. (2002). “The pre-European landscape of the United States,” in Fire, Native Peoples, and the Natural Landscape, ed T. R. Vale (Washington, DC: Island Press), 1–39.
Whittaker, J. C., and Huckell, L. (1981). Archeology in Yosemite National Park: the Wawona Testing Project. Tucson, AZ: Western Archeological and Conservation Center National Park Service.
[DATASET] Klimaszewski-Patterson, A., and Mensing, S. (2016) Neotoma dataset 48945: Holey Meadow pollen dataset. Neotoma Paleoecol. Database. doi: 10.21233/CQ9Q-5983
[DATASET] Klimaszewski-Patterson, A., and Mensing, S. (2020) Neotoma dataset 48964: Trout Meadow pollen dataset. Neotoma Paleoecol. Database. doi: 10.21233/SYJT-DJ16
[DATASET] Anderson, R. S., and Stillick, R. D. (2013) Neotoma dataset 48549: Wawona Meadow pollen dataset. Neotoma Paleoecology Database. doi: 10.21233/DTAC-5E13
[DATASET] Anderson, R. S. (1987) Neotoma dataset 3039: Woski Pond pollen dataset. Neotoma Paleoecology Database. doi: 10.21233/n3fj5g
Keywords: California, Sierra Nevada, paleoecology, fire, traditional ecological knowledge, cultural burning
Citation: Klimaszewski-Patterson A (2023) Potential of paleoecology and paleolandscape modeling to identify pre-Colonial cultural burning in montane forests: case studies in California. Front. Environ. Archaeol. 2:1251149. doi: 10.3389/fearc.2023.1251149
Received: 30 June 2023; Accepted: 11 October 2023;
Published: 14 November 2023.
Edited by:
Alan P. Sullivan III, University of Cincinnati, United StatesReviewed by:
Andrew Somerville, Iowa State University, United StatesDaniel Alexander Contreras, University of Florida, United States
Copyright © 2023 Klimaszewski-Patterson. This is an open-access article distributed under the terms of the Creative Commons Attribution License (CC BY). The use, distribution or reproduction in other forums is permitted, provided the original author(s) and the copyright owner(s) are credited and that the original publication in this journal is cited, in accordance with accepted academic practice. No use, distribution or reproduction is permitted which does not comply with these terms.
*Correspondence: Anna Klimaszewski-Patterson, anna.kp@csus.edu