- 1Department of Archaeology, Max Planck Institute of Geoanthropology, Jena, Germany
- 2Department of History, Geography and Communication, Universidad De Burgos, Paseo de Comendadores, Burgos, Spain
- 3Extreme Events Research Group, Max Planck Institutes of Geoanthropology, Chemical Ecology, and Biogeochemistry, Jena, Germany
- 4Australian Research Centre for Human Evolution, Griffith University, Brisbane, QLD, Australia
- 5School of Social Science, The University of Queensland, St Lucia, QLD, Australia
- 6Department of Anthropology, University of Pittsburgh, Pittsburgh, PA, United States
- 7Department of Archaeology, The University of York, York, United Kingdom
- 8Department of Anthropology, Rice University, Houston, TX, United States
- 9Department of Anthropology, National Museum of Natural History, Smithsonian Institution, Washington, DC, United States
- 10Department of Anthropology and Archaeology, University of Calgary, Calgary, AB, Canada
- 11School of Archaeology, University of Oxford, Oxford, United Kingdom
- 12isoTROPIC Research Group, Max Planck Institute of Geoanthropology, Jena, Germany
Background: Climate change played a major role in shaping regional human-environment interactions in Africa during the late Pleistocene-Holocene, but this topic has not been exhaustively studied, particularly in eastern Africa. While there is growing evidence that the coastal and island settings in this region played a critical role in human evolution, combined archaeological and palaeoenvironmental studies have tended to focus on the arid interior and show the dominance of grasslands with patches of closed and open woodlands during the last 20,000 years.
Methods: Here, we present stable carbon and oxygen isotope analyses of zooarchaeological remains (n = 229) recovered from Kuumbi Cave, Zanzibar Island, spanning the last glacial period and the Holocene (20,000 to 500 cal. BP).
Results: Our data demonstrate that the vicinity of Kuumbi Cave was consistently covered by mosaic habitats, dominated by forests and small patches of open woodland and grassland. The inhabitants of Kuumbi Cave exploited these diverse tropical habitats even after the regional arrival of agriculture.
Discussion: We suggest that the stable coastal forest mosaic habitats acted as a refugium for foragers during glacial periods and that the Iron Age inhabitants of Kuumbi Cave were not food producers migrating from the interior, but rather Indigenous foragers interacting with food production.
1. Introduction
The impacts of late Pleistocene-Holocene ecological diversity and transformations in eastern Africa on human societies and adaptations is not well explored. This period is characterized by major climatic fluctuations dictated by orbital forces and compounded by orogenic landmarks (e.g., the Rift Valley, lakes and rivers, and volcanic mountains and highlands) (Bonnefille et al., 1990; Kiage and Liu, 2006; Stager and Johnson, 2008). Ecological diversity supported a wide range of subsistence systems (Marean, 1992a; Ambrose, 1998; Kusimba, 1999; Prendergast, 2009). Lake core records from the interior of eastern Africa reflect intense periods of aridity during glacial and interglacial periods (Stager and Johnson, 2000, 2008; Kiage and Liu, 2006; De Cort et al., 2013; Gelorini and Verschuren, 2013; Stager et al., 2017), under which human populations would have faced impacts of frequent water shortages on subsistence strategies (Marean, 1992b; Kusimba, 2013; Tryon and Faith, 2016). However, recent multidisciplinary studies suggest that the coast experienced more subtle changes in precipitation and forest extent, which led to very different trajectories of human-environment interactions (Shipton et al., 2018; Roberts et al., 2020). Eastern Africa's islands remain almost completely unexplored from this multidisciplinary perspective.
Palaeoenvironmental change presented different opportunities and challenges to late Pleistocene to late Holocene human populations in eastern Africa. Holocene foragers with Later Stone Age (LSA) technologies exploited different animal species, from the large game of open savannas to smaller, forest-adapted taxa (Ambrose, 1984a; Gifford-Gonzalez and Kimengich, 1984; Mehlman, 1989; Shipton et al., 2018). Although much attention has focused on the complex Middle Stone Age (MSA) to LSA transition in eastern Africa, there has been relatively little discussion as to how LSA-using communities interacted with environmental variability across space and time. Later, from ~5,000 years ago, pastoralists coming from northeastern Africa (Prendergast et al., 2016; Skoglund et al., 2017; Wang et al., 2020) brought domesticated animals such as cattle (Bos taurus), goats (Capra hircus), and sheep (Ovis aries) to eastern Africa (Ambrose, 1984b, 1998; Marshall et al., 1984; Barthelme, 1985; Lane, 2013). Another wave of domesticated animals, and crops that included sorghum (Sorghum bicolor), pearl millet (Pennisetum glaucum), lablab beans (Lablab purpureus) and cowpea (Vigna unguiculata) from western Africa followed from ~2000 years ago (Ambrose et al., 1984; Giblin and Fuller, 2011; Crowther et al., 2018), with potential ramifications for human demography, culture, and ecological impacts (Gelorini and Verschuren, 2013; Marchant and Lane, 2014; Marchant et al., 2018; Sirak et al., 2022; Vicente and Schlebusch, 2022).
Past technological and economic changes, including the uptake of food production, have commonly been suggested to have coincided with climate change in eastern Africa (Marshall, 1990; Marshall and Hildebrand, 2002; Tryon and Faith, 2016). However, a rarity of stratified archaeological sequences with multi-proxy palaeoenvironmental data has often made it difficult to test hypotheses relating to human-environment interactions across this critical period of human history. This is particularly the case for eastern Africa's coastal islands, where the impacts of climate change and varying human adaptations on local ecosystems might also be expected to have had the most significant biotic and ecological ramifications (Horton, 2017; Crowther et al., 2018; Wynne-Jones and Laviolette, 2018). While mainland Africa has often been highlighted as an example of where late Pleistocene megafaunal extinctions were relatively muted when compared to other parts of the world (Martin, 1966; Barnosky et al., 2004; Faith, 2014; Stuart, 2015), a number of large mammals are known to have disappeared from eastern Africa's Zanzibar Island between the terminal Pleistocene and late Holocene (Prendergast et al., 2016; Shipton et al., 2016). This raises the question as to which factors (e.g. climate change, geographic island isolation, human hunting) had the most significant role in this island's faunal turnover (Prendergast et al., 2016; Shipton et al., 2016).
Here, we apply stable carbon (δ13C) and oxygen (δ18O) isotope analysis to human and faunal tooth enamel from the site of Kuumbi Cave (KC) on Zanzibar Island, Tanzania. The site records late Pleistocene to late Holocene (20,000 to 500 cal. BP) human occupation, covering periods of glacial-to-interglacial climate change as well as the island's connection to the mainland and subsequent isolation (Figure 1) (Shipton et al., 2016). The site also records the extirpation of at least six medium to large mammals on the island following the Pleistocene, those being the Thomson's gazelle (Eudorcas thomsonii: reported by Prendergast et al., 2016 as Gazella thomsoni), buffalo (Syncerus caffer), waterbuck [Kobus ellipsiprymnus, reported by Prendergast et al. (2016) as K. defassa], reedbuck (Redunca redunca), zebra (Equus quagga), bushbuck (Tragelaphus scriptus), and two small bovids: klipspringer (Oreotragus oreotragus) and bush duiker (Sylvicapra grimmia) (Prendergast et al., 2016). Enamel δ13C and δ18O values reliably reflect consumer diets and environments in the region (Roberts et al., 2020). We combine stable isotope (n = 229, SI1) and complementary archaeological datasets to explore how humans at this site responded to climatic fluctuations, the arrival of food production, and how they might have impacted past prey populations. We put our data in regional context through comparisons with published stable isotope records (n = 756, SI2) from the Pleistocene-Holocene coastal cave site of Panga ya Saidi (PYS), Kenya and a series of sites in the interior of eastern Africa.
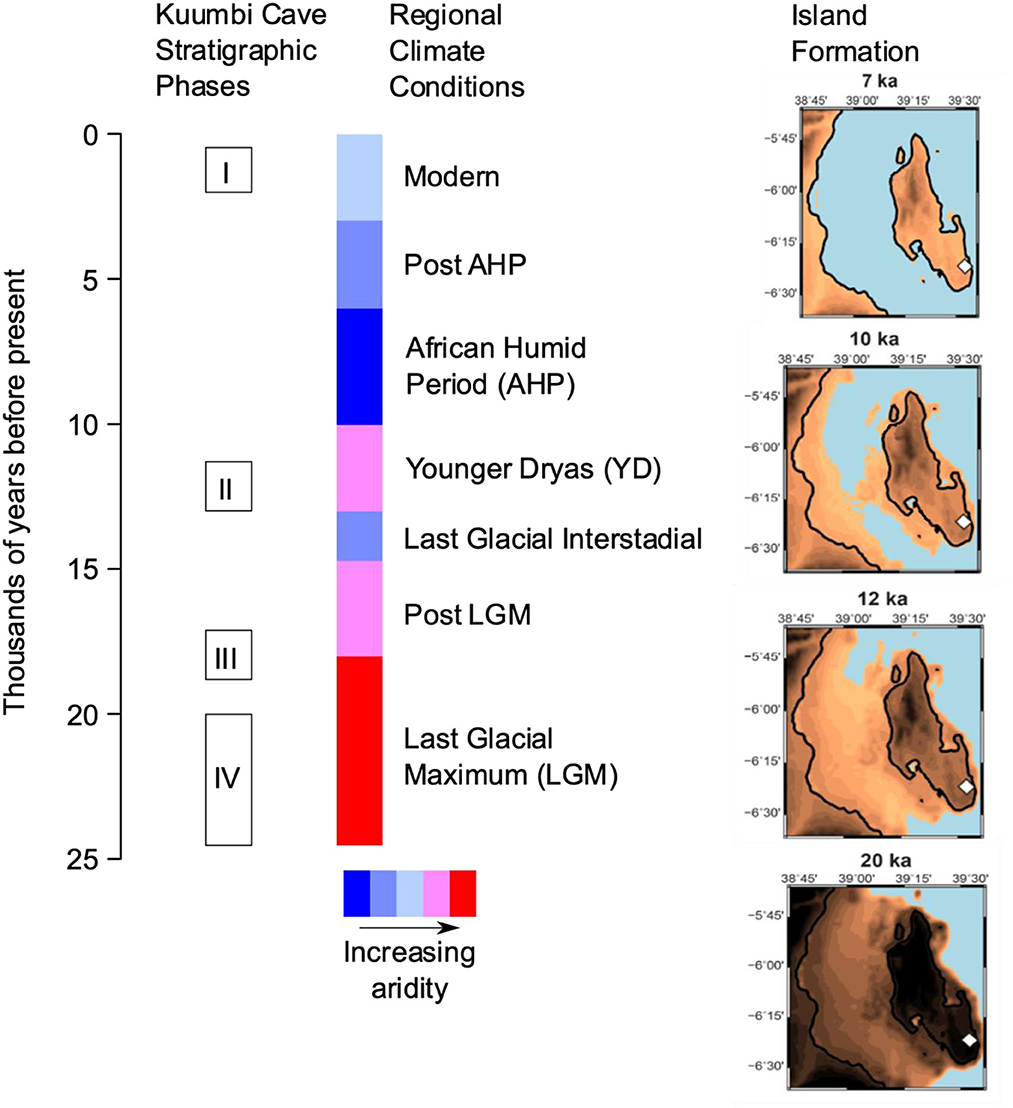
Figure 1. Chronology of the KC stratigraphic sequence against regional climate conditions and Zanzibar Island formation. The different shades of brown indicate different stages of island formation, blue represents the ocean, and the white diamond represents the location of KC. The island was formed between 12 to 10 ka. Island reconstructions by H. Rouby and adapted from Prendergast et al. (2016).
2. Background to the site and methodology
2.1. Human environments in eastern Africa during the last 20,000 years BP
Sedimentary records indicate that, during the late Pleistocene to the late Holocene, interior eastern Africa was characterized by dramatic climate-driven ecological transformations linked to changes in the Earth's orbit (Figure 1) (Talbot and Lærdal, 2000; Kiage and Liu, 2006; Foerster et al., 2015; Revel et al., 2015; Schaebitz et al., 2021). Palaeoclimatic discussions of eastern Africa focus on precipitation as the major climatic parameter (Bonnefille et al., 1990; Stager and Johnson, 2000). However, the majority of these discussions, and the above-mentioned climatic changes, are based almost entirely on lacustrine records from the arid interior.
The environmental manifestations of eastern Africa's climate history are, by contrast, poorly known for coastal, and especially island settings (Figure 2). There are several near-coastal archipelagoes in eastern Africa, notably Lamu, Zanzibar, Mafia, and Kilwa. During the last glacial period, Zanzibar (Unguja) Island of the eponymous archipelago was connected to mainland eastern Africa due to lower sea-level (For reconstruction of the topography of Zanzibar between 20–7 ka see Prendergast et al., 2016: Figures 4, 5) (Moreau and Pakenham, 1940, 1941; Burgess and Clarke, 2000; Prendergast et al., 2016). At this time, open grasslands apparently dominated the interior of eastern Africa thus connecting arid lands in northern and southern Africa while disconnecting the equatorial Congo-forest and the coastal forests of eastern Africa (Kingdon, 1984b, 2001a). During the Holocene, sea-level rise created Zanzibar Island, and closed woodlands and forests expanded as a result of increased precipitation in the interior (Burgess and Clarke, 2000; Prendergast et al., 2016). While these changes may have had major influences on human behaviour and migration in the interior (Ambrose, 1984a, 1998; Marshall and Hildebrand, 2002), the degree to which these palaeoenvironmental changes influenced the fauna and vegetation of islands such as Zanzibar remains relatively untested.
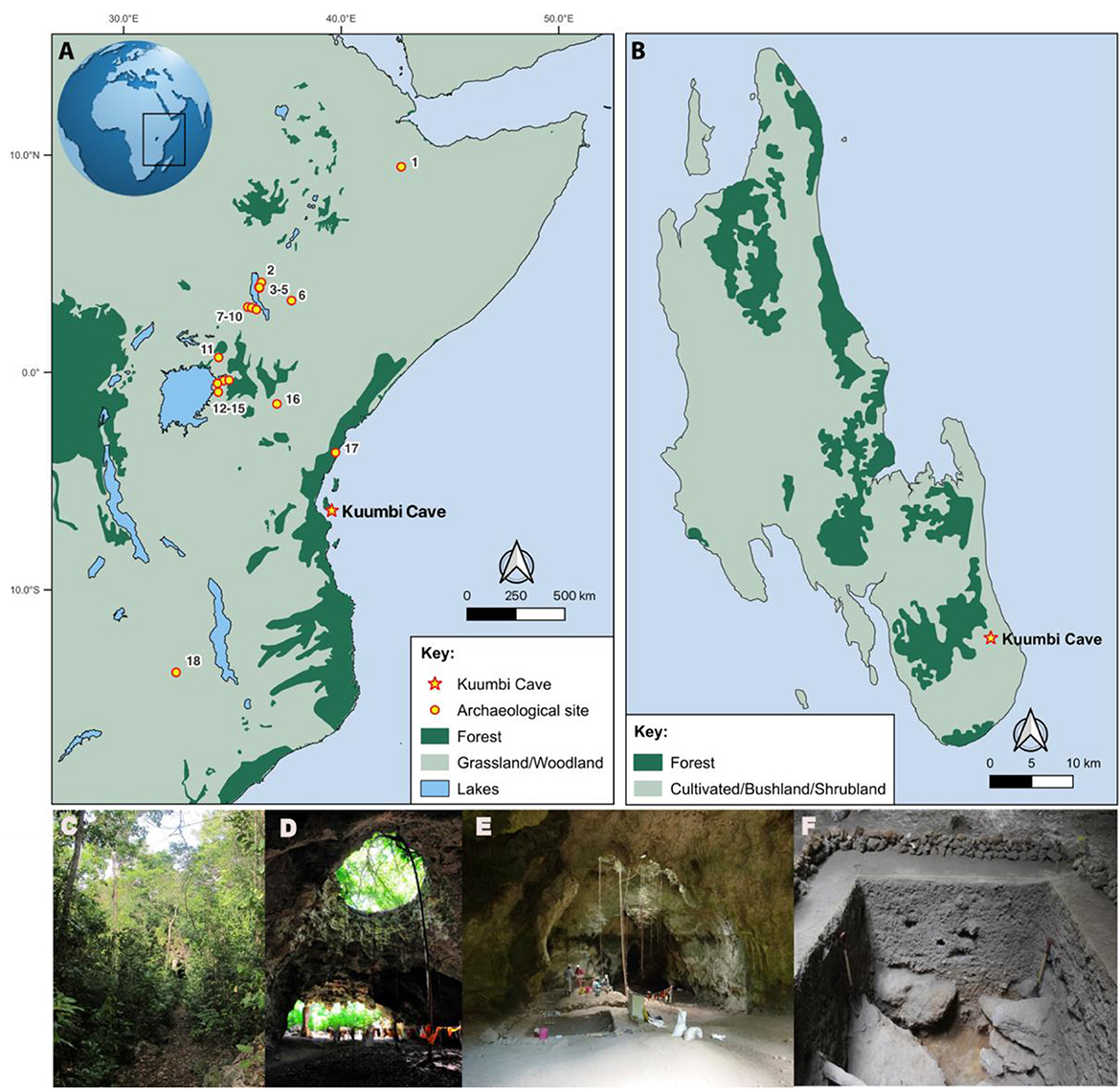
Figure 2. Map of (A) eastern Africa showing sites with stable isotope data cited in this paper: (1) Porc Epic, (2) FxJj12, (3) GaJj11, (4) GaJj2, (5) Dongodien, (6) Ele Bor, (7) “AW,” (8) “Equid site,” (9) “514”, (10) Lothagam Lokam, (11) Kakapel, (12) Wadh Lang'o, (13) Kanam East, (14) Luanda, (15) Gogo Falls, (16) Lukenya Hill, (17) Panga ya Saidi, (18) Kalemba Rockshelter; and (B) Unguja (Zanzibar) Island showing the location of Kuumbi Cave. Modern vegetation based on (A) White (1983) and (B) Hardy et al. (2015): classification of vegetation biomes developed from regional base maps validated through ground surveys. Photos of Kuumbi Cave view from (C) outside facing the entrance, (D) inside facing outside, (E) view of trench 10 excavation, and (F) Trench 10 cross section.
Palaeontological and zooarchaeological records from eastern African sites have long provided rich insights into the responses of human and faunal communities to climatic change (Marean, 1992a,b; Dale, 2007; Tryon and Faith, 2016; Prendergast and Beyin, 2018). In the late Pleistocene, fossils have documented major turnover of grazing fauna as arid grasslands expanded in the interior during glacial periods (Marean, 1992b; Kusimba, 2013; Tryon and Faith, 2016; Robinson, 2017, 2022). Meanwhile, the 78,000-year zooarchaeological record from PYS on the coast shows the persistence of forest- and grassland-adapted fauna throughout its record of occupation (Shipton et al., 2018; Roberts et al., 2020; Prendergast et al., 2023). Spatial differences in eastern African environments between these areas seem to have shaped human hunting and foraging behaviours well into the Holocene. From the middle to late Holocene, food production arrived in the form of domesticated animals and plants. Interior zooarchaeological records have shown complex variations in the use of wild and domesticate resources, with the gradual, non-linear adoption of cattle, sheep, goats, and crops (Gifford-Gonzalez, 1998; Marshall et al., 2011; Prendergast, 2011; Robertshaw, 2021). Despite the interest that island contexts have globally received with regards to forager impacts on environments, species extinction or extirpation, and the expansion of food production (Rick et al., 2013; Fitzpatrick et al., 2015; Braje et al., 2017), palaeoecological studies of humans and animals on eastern Africa's offshore islands remain rare.
2.2. Kuumbi Cave
Kuumbi Cave (KC) offers the unique opportunity to explore archaeological and zooarchaeological records from the late Pleistocene to late Holocene in an eastern African island context. The site is located on the southeastern side of Zanzibar Island (Figure 2). Excavations at KC by the Sealinks project exposed a stratigraphic sequence in Trench 10 that captures significant time periods during the last 20,000 cal. BP, including major climate change and island formation (Prendergast et al., 2016; Shipton et al., 2016). The sequence is divided into four Phases (Figure 1), with a hiatus in human occupation between Phases II and I (Shipton et al., 2016). The pre-food production Phases (IV, III, and II characterized by a lack of domesticates and associated material culture e.g. iron and pottery) are dominated by stone artefacts manufactured using the bipolar technique, and bone artefacts used as projectile points or awls (Langley et al., 2016). Small bovids, monkeys, hyrax, and giant rat dominate the faunal remains with a smaller percentage of larger animals that fluctuate over time (Prendergast et al., 2016). Fish bones, land snail, and marine shells are also present in these Phases. While a range of domesticates were identified by earlier projects (Sinclair et al., 2006; Chami, 2007, 2009), the Sealinks project has thus far only identified one goat specimen at the site from Phase 1 (Iron Age) (Prendergast et al., 2016; Shipton et al., 2016; Culley et al., 2021b).
The faunal assemblage at KC is characterized by two major trends through the four Phases (Prendergast et al., 2016; Shipton et al., 2016). There is consistent presence of, and increase in, forest-adapted species through time, with Phases I and II having the highest proportions. Concordantly, there is an increase over time in the rarity, and even extirpation, of open habitat adapted species. The forest-adapted species are dominated by primates, small bovids, and hyrax. Primates include frugivores and folivores such as vervet/blue monkeys (Cercopithecus or Chlorocebus spp.) and Zanzibar red colobus (Piliocolobus kirkii), as well as omnivores such as the greater galago (Otolemur crassicaudatus) and Zanzibar bush baby (Galago zanzibaricus). Bovids include the three dwarf antelopes: the frugivorous blue duiker [Philantomba monticola, reported by Prendergast et al. (2016) as Cephalophus monticola] and Ader's duiker (Cephalophus adersi), and the browser suni (Neotragus moschatus) that are dominant in all Phases. Other browsing bovids include bush duiker, klipspringer, and bushbuck. There is only one bovid mixed feeder (which tends more toward grazing), oribi (Ourebia ourebi). The browsing tree hyrax (Dendrohyrax validus) and omnivorous giant pouched rat (Cricetomys gambianus) are also conspicuous in all the Phases.
Highly flexible fauna include the omnivorous bush pig (Potamochoerus larvatus), elephant shrew (Macroscelidea), porcupine (Hystrix cristata), and mixed feeding hare (Lepus sp.). Open habitat adapted species are dominated by grazing taxa that include Thomson's gazelle, buffalo, waterbuck, reedbuck, and zebra. Eleven species in this assemblage are extirpated on Zanzibar Island today, among them eight large taxa: five grazers—Thomson's gazelle, buffalo, waterbuck, reedbuck, zebra; three browsers—bushbuck, klipspringer, and bush duiker; and three small mammals—hare, porcupine, and the carnivorous white-tailed mongoose (Ichneumia albicauda). Reedbuck and zebra are present in all the four Phases, but absent in the later/upper part of Phase I [reported as P1a in Prendergast et al. (2016)]. Thomson's gazelle and waterbuck are present in Phases II, III, and IV, and buffalo is only present in Phase III. Klipspringer is present in Phases II and III only, bushbuck is present only in Phase III, and bush duiker is present in Phases I, II and III. Hare is present in Phases I (early part) and II, porcupine in Phase II only and white-tailed mongoose is only present in Phases III and IV.
2.3. Stable isotope analysis and human palaeoecology in eastern Africa
Plants that use the C3 and C4 photosynthetic pathways have tissues with distinct δ13C values due to differences in fractionation during the fixation of CO2. C3 values range from about −36 to −24‰ (global mean −26.5‰) while C4 values range from approximately −17 to −9‰ (global mean −12‰) (Craig, 1953; Smith and Epstein, 1971; Farquhar et al., 1989). This distinction is transmitted through the food chain and recorded in the tissues of consumers, including durable tissues (e.g., bones) that are preserved in the archaeological record (DeNiro and Epstein, 1978; van der Merwe, 1982; Cerling and Harris, 1999). Based on the non-overlapping distinction between C3 and C4 resources, animal tissues have been isotopically analyzed to highlight ecological change through time in eastern Africa (Ambrose and DeNiro, 1986a; Cerling et al., 2003, 2010). A dominance of C3 consumers has been considered indicative of forest/woodland dominance, while C4 consumers are thought to be indicative of tropical grassland (Codron et al., 2012; Crowley, 2012; Roberts et al., 2020).
Within tropical forest settings, C3 vegetation growing beneath a closed forest canopy typically has lower δ13C values due to low light and recycled CO2 (van der Merwe and Medina, 1989, 1991; Cerling et al., 2004). This ‘canopy effect' also influences forest consumers, notably primates, and helps distinguish canopy and under canopy feeders. Prior to the industrial revolution and the impact of fossil fuel emissions on atmospheric CO2, enamel δ13C values less than or equal to −14‰ are thought to be indicative of reliance on closed-canopy forest vegetation, while average values for C3 and C4 reliance are c. −12‰ and 0‰, respectively (Lee-Thorp et al., 1989; Levin et al., 2008; Cerling et al., 2015). An average of −10‰ for C3 is expected to indicate reliance on open-canopy wood/shrub vegetation while values between −8‰ to −1‰ indicate mixing C3 and C4 vegetation (Cerling et al., 2015).
Stable oxygen isotope (δ18O) measurement of mammalian tissues provides additional palaeoecological information about feeding and drinking behaviours. In tropical ecosystems, δ18O of vegetation reflects either evaporative potential or the source-effect of rainfall (Buchmann et al., 1997; Buchmann and Ehleringer, 1998; Giraldo et al., 2022). The close association between plant δ18O and evaporative potential can, in turn, be used to infer forest canopy density. Vegetation δ18O has been shown to differ depending on height within the canopy, and among different plant parts (Silveira, 1989; Buchmann et al., 1997). Meanwhile, animals that obtain the majority of their water requirements from plants will most effectively record these environmental factors (Kohn et al., 1996, 1998; Levin et al., 2006; Carter and Bradbury, 2015).
It has been highlighted recently that stable isotope approaches to African fauna must consider the potential bias of taxonomic representation in the assemblage, with recent studies being careful to factor in feeding behaviours (e.g., browsers, grazers, mixed feeders, and omnivores) into their isotopic comparisons (Cerling et al., 2015; Robinson, 2017; Roberts et al., 2020). Here, we use zooarchaeological data to help interpret and analyze isotopic data from the faunal assemblage, as well as two human samples, to explore human palaeoecology at KC and the degree to which forest cover changed in the vicinity of the site between the late Pleistocene and Holocene.
3. Materials and methods
3.1. Zooarchaeology background and identification for isotope analysis
The faunal assemblage from KC Trench 10, from which all materials presented in this study are sourced, includes 6,667 individual specimens (Prendergast et al., 2016; Shipton et al., 2016). Most of the vertebrate remains are anthropogenically accumulated, with cutmarks and burning showing the persistent influence of humans on the assemblage. Faunal and human teeth were chosen for isotopic analysis on the basis of archaeological context, tooth completeness, and availability. A total of 229 faunal and 2 human enamel tooth samples were included in the δ13C and δ18O analysis. The tooth samples were systematically selected from the four Phases represented in the stratigraphical sequence. Only tooth samples that could be taxonomically identified were included in the analysis. Most of the samples came from fauna identified to species level (142) while the rest could be identified to genus (6), family (7), subfamily (63), tribe (7), or order (4) (Prendergast et al., 2016; Shipton et al., 2016). The two human molars that were sampled came from the Iron Age and terminal Pleistocene Phases. The terminal Pleistocene molar marks the earliest human remains recovered from eastern Africa's littoral islands. The sample set for stable isotope analysis also includes five extirpated large taxa: reedbuck, zebra, bushbuck, klipspringer, and bush duiker (Prendergast et al., 2016).
We use the basic ecological categories used by Roberts et al. (2020) based on collating ecological observation and isotope studies (Fleagle, 1999; Gagnon and Chew, 2000; Kingdon, 2001a; Cerling et al., 2003, 2004, 2010, 2015; Campbell et al., 2006) to categorize taxonomic groups into four broad feeding groups (browsers, grazers, mixed feeders, and omnivores) and five major habitat categories (open and closed forests, open and closed woodlands, and grasslands). Open habitat/environment is also used to refer to a mixture of open woodlands and grasslands. Browsers here refer to mammals that feed on plant parts that include leaves, fruits, soft shoots, and roots. In the isotope analysis, we include the frugivorous taxa blue duiker and Ader's duiker and vervet/blue monkeys, and the folivorous Zanzibar red colobus in the browser category.
All teeth were photographed prior to analysis and are currently housed either at the British Institute in Eastern Africa (BIEA) or have been repatriated to Zanzibar. Sampling took place at both National Museums of Kenya (NMK) and Max Planck Institute of Geoanthropology (MPI-GEA), where teeth or tooth fragments were cleaned using air-abrasion to remove any adhering external material. Enamel powder was obtained using gentle abrasion with a diamond tipped drill along the full length of the buccal surface in order to maximize the period of formation represented by the bulk sample. Enamel powders were pre-treated to remove any organic or secondary carbonate contaminates. This consisted of a wash in 1.5% sodium hypochlorite for 60 min, followed by three rinses in purified H2O and centrifugation, before 0.1 M acetic acid was added for 10 min, followed by another three rinses in purified H2O (Sponheimer et al., 2005; Lee-Thorp et al., 2012; Roberts et al., 2017). Following reaction with 100% phosphoric acid, gases evolved from the samples were analyzed for their stable carbon and oxygen isotope measurements using a Thermo Gas Bench 2 connected to a Thermo Delta V Advantage Mass Spectrometer at MPI-GEA.
Isotopic values are reported as the ratio of the heavier isotope to the lighter isotope (13C/12C or 18O/16O) as δ values in parts per mill (‰) relative to international standards, VPDB for δ13C and δ18O. δ13C and δ18O values were normalized using a three-point calibration against international standards (IAEA-603 (δ13C = 2.5‰; δ18O = −2.4‰); IAEA–CO−8 (δ13C = −5.8‰; δ18O = −22.7‰); and IAEA NBS 18 (δ13C = = −5.014‰; δ18O = −23.2‰)). USGS44 (δ13C= −42.2‰) was included as an in-house standard. Replicate analysis of USGS44 standards suggests that machine measurement error is c. ± 0.1‰ for δ13C and ± 0.2‰ for δ18O. Overall measurement precision was studied through the measurement of repeat extracts from a bovid tooth enamel standard (n = 20, ± 0.2‰ for δ13C and ± 0.3‰ δ18O).
3.2. Statistical analysis
The free R statistical software (R Core Team, 2013) was used for all statistical analyses. Two-way ANOVAs were performed to check the effect of Phase and family (given limited species-specific data) on both enamel δ13C and δ18O values. Levene's test and the Shapiro-Wilk test were used to check the quality of the models. Post-hoc comparisons using Tukey's method were done to determine which taxa or stratigraphic layers were distinct from each other. An alternative set of two-way ANOVAs checked the effect of Phase and dietary category (each category including multiple families) on both δ13C and δ18O values. Dietary categories here include “browsers (including folivores and frugivores),” “grazers,” “mixed feeders,” and “omnivores” and are based on existing ecological publications (Kingdon, 1984b, 2001a; Gagnon and Chew, 2000; Cerling et al., 2003, 2004, 2015). We performed taxon-specific regression on the three families that include the largest ranges of δ13C values (Bovidae, Equidae, and Procaviidae) to determine whether δ13C and δ18O values were positively associated. Aside from Equids, the taxa analyzed in abundance at KC are relatively scarce at sites in mainland eastern Africa, and taxon-specific samples from particular time periods are too small to permit statistical analysis. However, we used Kruskal-Wallis tests (and associated Wilcoxon tests with p values adjusted according to Bonferroni) to examine the effect of site on regional equid δ13C and browser δ13C data. The details of all statistical analyses are presented in the Supplementary material (SI).
4. Results
4.1. Faunal stable carbon and oxygen isotope results from Kuumbi Cave
4.1.1. Variation in taxa and dietary groups
The full dataset (n = 229) of δ13C and δ18O values of faunal teeth is shown in Supplementary material SI1. The taxa and dietary groups summary statistics, two-way ANOVAs, and post-hoc comparisons show similar δ13C and δ18O patterns (Supplementary material SI3:1-3). The range of faunal δ13C values (−20.4 to −1.9‰; mean −11.9‰, median −12.3‰) indicates that the fauna hunted by foragers at the site exploited diverse habitats (Figure 3 and Supplementary material SI3:1-2). Small, forest-adapted mammals weighing less than 25 kg make-up ~70% of the mammalian faunal remains. This includes five non-human primates, tree hyrax, giant pouched rat, and small bovids mainly from the Cephalophini and Neotragini forest-adapted tribes. Primates identified as vervet/blue monkeys, Zanzibar red colobus, Zanzibar bush baby, and greater galago, consistently have low δ13C values (−15.3 to −10.6‰). Tree hyrax and bovids blue duiker, suni, klipspringer, and bush duiker show the lowest values (−20.4 to −15‰). Oribi, reedbuck and zebra have the highest values in the dataset (−5.5 to −1.9‰). The other taxa (suid and small mammals) have an intermediate range (−13.2 to −9.2‰). Two-way ANOVAs show that family has a significant effect on δ13C values (model 1, p < 0.0001) (Supplementary material SI3:3). Subsequent post-hoc comparisons suggest that Equidae samples are significantly enriched in 13C relative to samples from all other taxa (model 1, p < 0.0001).
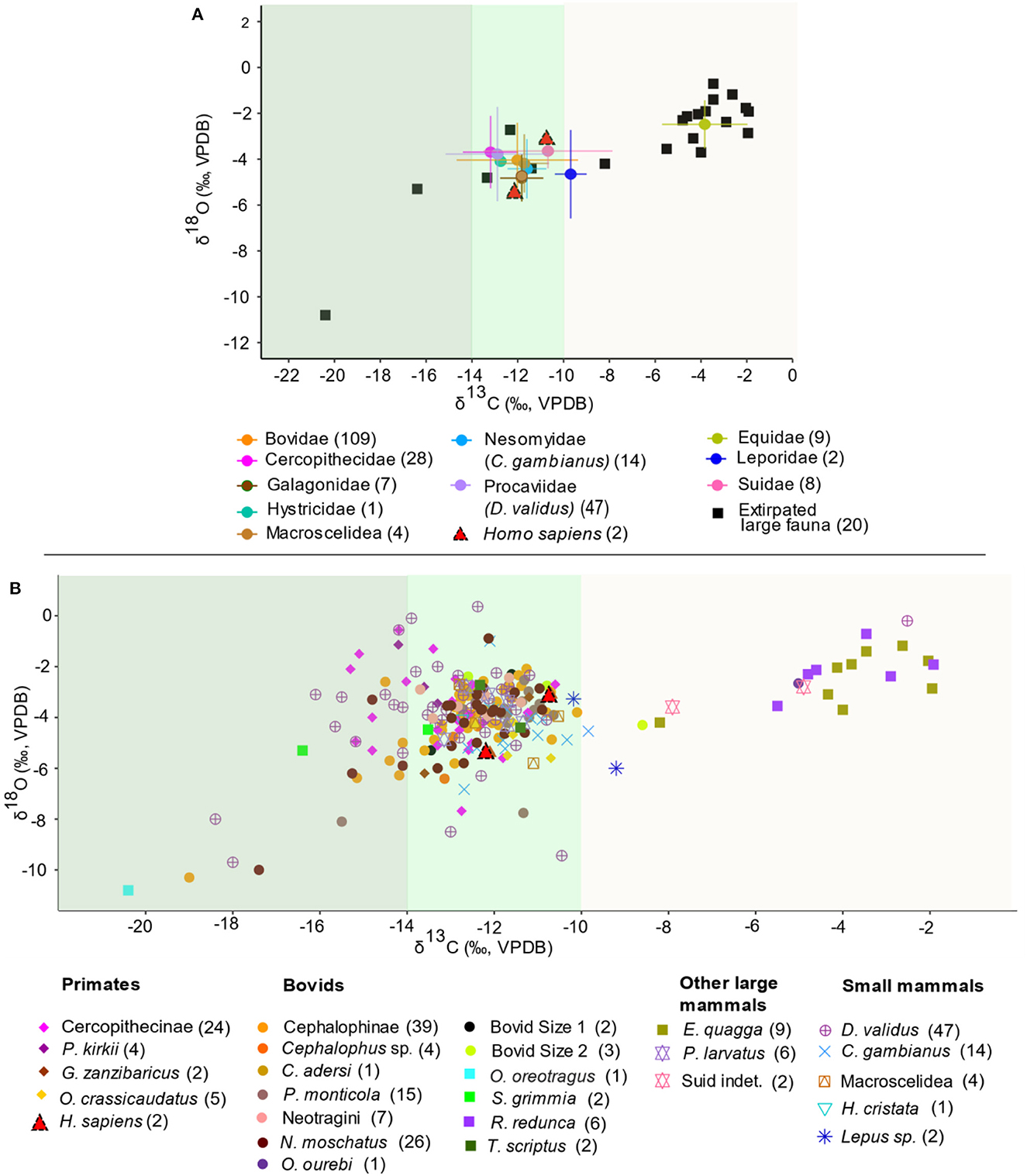
Figure 3. Scatterplots of δ13C and δ18O values (A) by family highlighting forest (closed and open canopy shaded dark and light green respectively) and mosaic grassland (light brown) adapted fauna, extirpated fauna in square symbols, and the two Homo sapiens specimens (red triangles), and (B) by taxa. Taxon-specific sample sizes are in brackets. Different faunal groups highlighted in (A, B) are represented by different symbols and colors: primates, bovids, other large mammals, and small mammals, except the five extirpated large fauna, which are represented by square symbols (O. oreotragus, S. grimmia, R. redunca, T. scriptus, and E. quagga). The error bars in (A) represents standard deviations.
The KC fauna also record a wide range in δ18O values (range −10.8 to 0.4‰; mean −3.9‰) (Figure 3 and Supplementary material SI3:1-2). Klipspringer has the lowest δ18O value (−10.8‰) and tree hyrax has the widest range and highest values (range −9.7 to 0.36‰; mean −3.8‰). Most of the arboreal and bovid taxa have relatively low values. Among the arboreal taxa (range −18.4 to −2.5‰), red colobus has the highest mean followed by tree hyrax, cercopithecines, Zanzibar bush baby, and greater galago. The forest-adapted terrestrial small mammals giant pouched rat and elephant shrew have similar values to the arboreal taxa. Zebra and reedbuck have the highest values among the ungulates (−8.2 to −1.9‰). Although two-way ANOVAs show that family has a significant effect on δ18O values (p = 0.03), subsequent post-hoc comparisons suggest there is no significant distinctions in δ18O values among families (model 2, p > 0.05) (Supplementary material SI3:3).
When the KC faunal dataset is divided into dietary groups, the δ13C values show significant distinctions. Browsers have the lowest values (mean −12.8‰), and grazers the highest values (mean −3.9‰). Values for mixed feeders (mean −8.1‰) and omnivores (mean −11.7‰) fall between browsers and grazers as expected. The δ18O values mirror the δ13C value patterns with browsers having the lowest values (mean −4.0‰) and grazers the highest values (mean −2.3‰) (Figure 4). Two-way ANOVAs showed that diet groups have a significant effect on δ13C values (model 3, p < 0.0001) and δ18O values (model 4, p = 0.02) (Supplementary material SI3:3). Post-hoc comparisons suggest that grazers, browsers, and omnivores tend to have significantly different δ13C values, with grazers most enriched in 13C, browsers most depleted in 13C, and omnivores between the two (model 3, p < 0.0001). Post-hoc comparisons also suggest that grazers are enriched in 18O relative to browsers (model 4, p = 0.03).
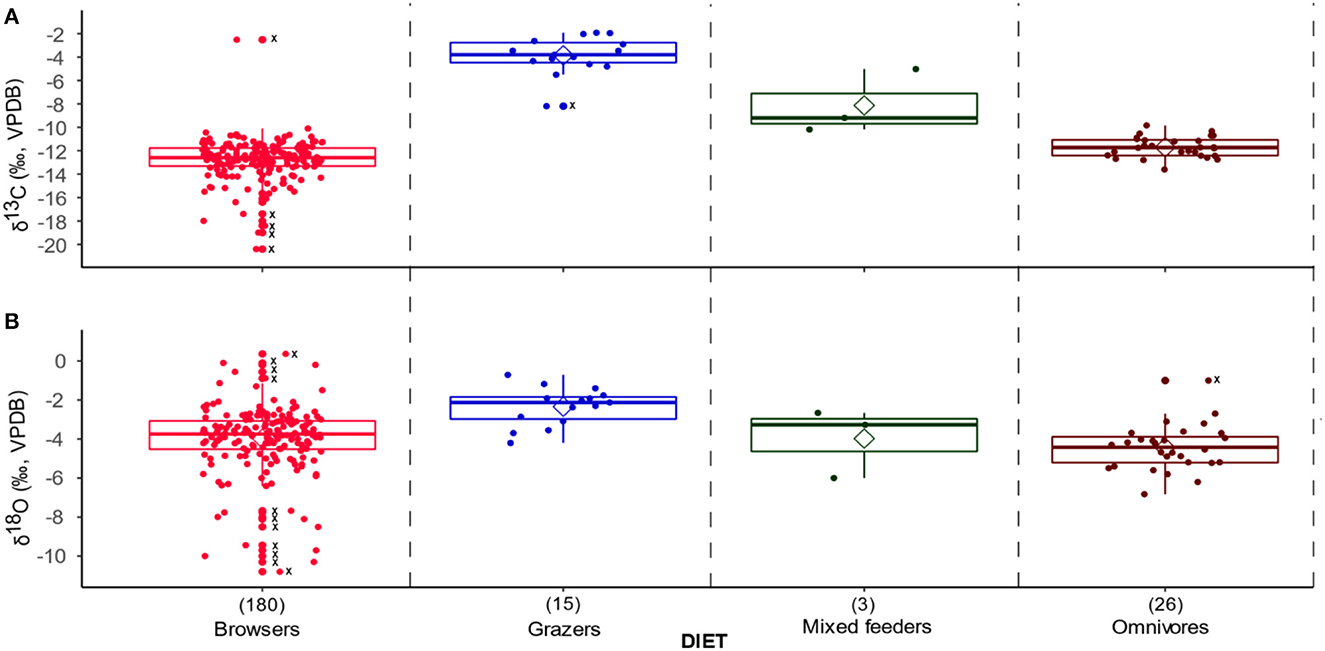
Figure 4. Box and jitter plots of (A) δ13C and (B) δ18O values classified by the faunal dietary category. Outliers are shown with dots and Xs along the midline of the plots, and the sample sizes are in brackets above the plot labels along the X-axis.
A general linear model (GLM) clarifies the general correlation between δ13C and δ18O values (p < 0.0001) (Supplementary material SI3:4), which is particularly visible through the regression between δ13C and δ18O values within both Bovidae and Procaviidae (Figure 5). Data for families with the largest ranges of δ13C values (Bovidae from −20.4 to −1.9 ‰, Equidae from −8.2 to −2.0 ‰, and Procaviidae from −18.4 to −2.5 ‰) were used for the taxon-specific regression (Figure 5). Though δ13C values explain a small fraction of the variation in δ18O data, a 3‰ increase in δ13C values is typically matched by an ~1‰ increase in δ18O values.
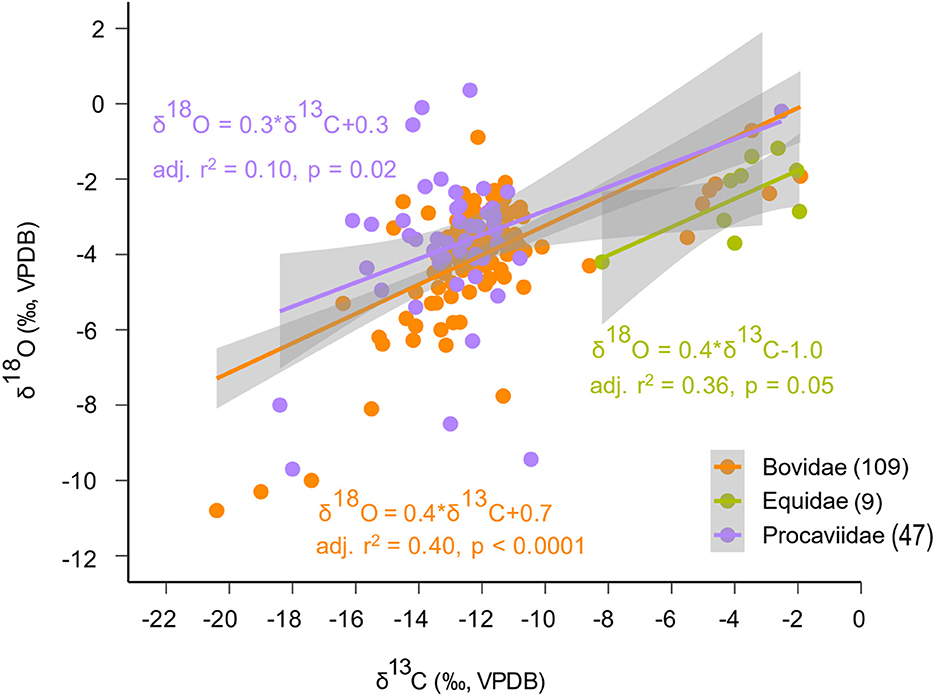
Figure 5. Taxon-specific regression between δ13C and δ18O data for the three families that include the largest ranges of δ13C values (Bovidae from −20.4 to −1.9‰, Equidae from −8.2 to −2.0‰, and Procaviidae from−18.4 to −2.5‰) show that there is a significant regression between δ13C and δ18O data within each family. The grey bands mark the 95% confidence intervals associated with each regression line. Taxon-specific sample sizes are in brackets.
4.1.2. Variation over time
δ13C and δ18O summary statistics, two-way ANOVAs and post-hoc comparisons show there is variation through time with contrasting patterns between Phases I and II on the one hand, and III and IV on the other (Figure 6 and Supplementary material SI3:2-3). δ13C values show that Phase III (mean −10.8‰) and IV (mean −10.0‰) have higher values than Phase II (−13.3‰) (Figure 6 and Supplementary material SI3:2 Figure S2). The Phase I δ13C values (mean −12.5‰) are slightly higher than Phase II, but the Phase I δ18O values (mean −4.6‰) are the lowest of the four Phases. Two-way ANOVAs show that Phase has a significant effect on δ13C values (p < 0.0001) and δ18O values (p < 0.0001) (Supplementary material SI3:3). Post-hoc comparisons show samples from Phases III and IV tend to be significantly higher in 13C relative to samples from Phases I and II (p < 0.0004). Samples from Phase III are consistently higher in 18O relative to samples from Phases I and II (p ≤ 0.01) (Supplementary material SI3:3).
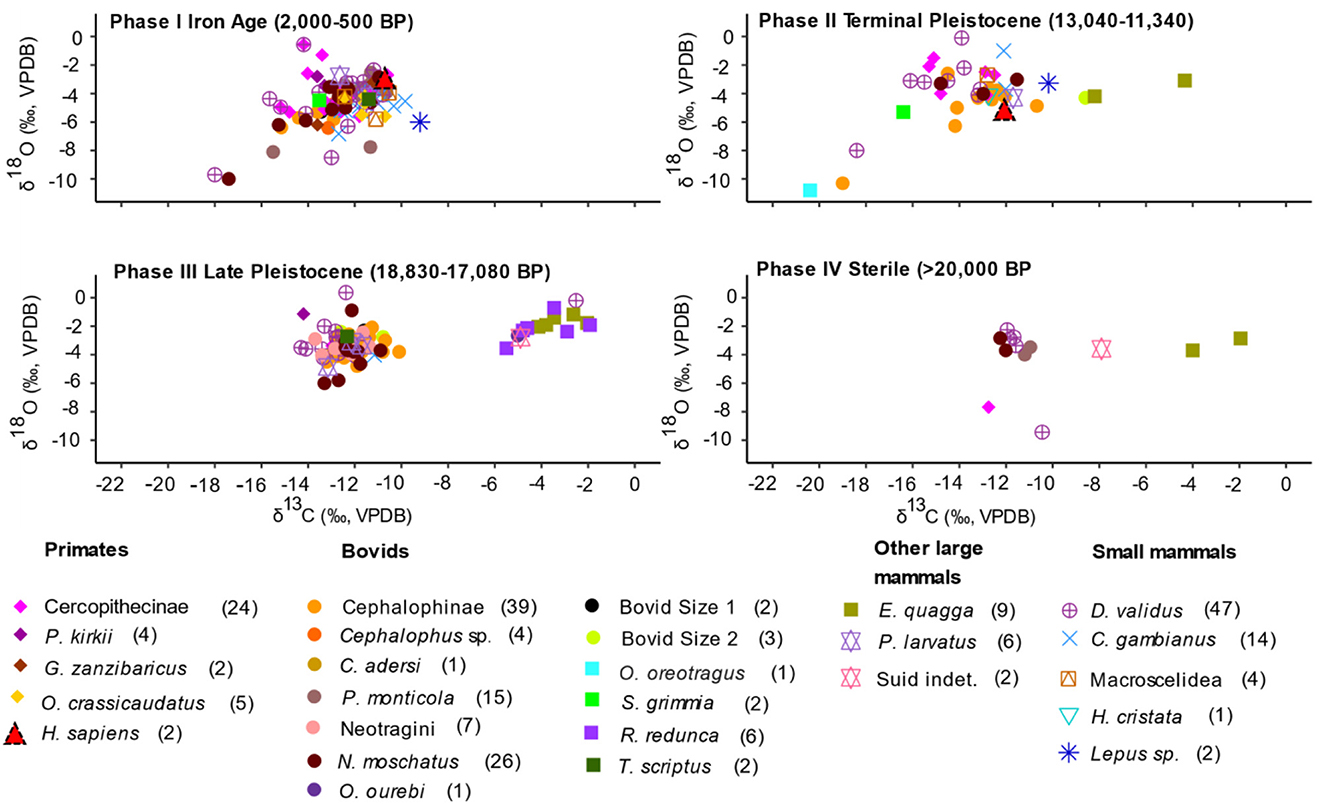
Figure 6. Scatterplots of δ13C and δ18O values by taxa in Phases I-IV. Different faunal groups are represented by different symbols and colors: primates, bovids, other large mammals, and small mammals, except the five extirpated large fauna which are represented by square symbols (O. oreotragus, S. grimmia, R. redunca, T. scriptus, and E. quagga). The two Homo sapiens specimens are represented by red triangles. Taxon-specific sample sizes are in brackets.
Evaluation of the δ13C and δ18O taxa values based on the temporal Phases indicates a trend towards lower values and increased variation (Figure 6 and Supplementary material SI3:2 Figure S2). This trend is particularly conspicuous for arboreal fauna represented in all the four Phases. There are lower values and less variation in cercopithecines and tree hyrax from Phases I and II compared to Phases III and IV. Values for forest adapted terrestrial fauna indicate lower values and increased variability through time (Figure 6 and Supplementary material SI3:2 Figure S2). The bovid tribes that are represented in the four Phases (Cephalophini and Neotragini) also illustrate this observation. Values for other bovid categories which include the other named tribes and species not represented in all the four Phases amplify this variation: klipspringer, (Phase II), bush duiker (Phase I and II), bushbuck (Phase III and I), oribi (Phase III), Bovid size 1 (Phase I and III), and Bovid size 2 (Phase II and III).
When the dietary groups are analyzed through time, both δ13C and δ18O values show significant distinctions (Supplementary material SI3:3). Two-way ANOVAs suggest that the interaction between the effects of phase and family has an insignificant effect on δ13C values (model 1, F(13,199) = 0.49, p = 0.93) and on δ18O values (model 2, F(13,199) = 1.51, p = 0.11). The same is true when taxa are grouped according to dietary category (δ13C model 3, F(4,213) = 0.35, p = 0.84, δ18O model 4 F(4,213) = 0.62, p = 0.65) (Supplementary material SI3:3). Phase I and II δ13C values are the lowest for most of the dietary groups. Browsers also show consistently low δ13C values through the four phases, and grazers have lower values in Phase II, but consistently high values in Phase III and Phase IV (No values for Phase I). The δ18O values for browsers show that the lowest values and the widest ranges are in Phases I and II (Figure 6 and Supplementary material SI3:2 Figure S2). Grazers have relatively low values in Phase II, and high values in Phase III and IV. Omnivores have relatively low values in Phases I and III, but high values in Phase II. Mixed feeders have a small sample size: Phases I, II, and III have one sample each and Phase IV has no samples. Two-way ANOVAs and post-hoc comparisons for δ13C and 18O values also highlight contrasting variability patterns between browsers, grazers, and omnivores in the different phases (Supplementary material SI3:3). Two-way ANOVAs shows that phase has a significant effect on δ13C values (p < 0.0001) and δ18O values (p < 0.0001) of dietary groups (Supplementary material SI3:3). These patterns among phases hold regardless of whether taxa are grouped according to family (models 1–2) or dietary category (models 3–4). The value pattern in Phase III is driven by relatively high numbers of equids and reduncines (Figure 6).
4.2. Human values
The two measured human individuals δ 13C and δ18O values are within the range of the other omnivorous taxa: Terminal Pleistocene −12.1‰ and −5.4‰, and Iron Age −10.7‰ and −3.1‰.
4.3. Kuumbi Cave and mainland eastern African sites
The summary statistics for δ13C and δ18O values (n = 985) show that KC has the lowest values in eastern Africa followed by PYS and Kalemba (Table 1 and Figure 7). The other mainland sites have high values. Equids and browsers have representative sample sizes to allow δ13C values statistical comparisons between sites (Figures 7, 8 and Supplementary material SI3:5). Equids are sufficiently well represented in six regional sites (KC, Lake Victoria, Lake Turkana, Kalemba, Lukenya, and Porc Epic). A one-way ANOVA suggests that there are significant differences in equid values among these sites (F(4,216) = 115.4, p < 0.0001) (Supplementary material SI3:5). The δ13C model fails Levene's test for equal variance (F = 7.75, p = 0.007), but significant differences in δ13C values among sites are still supported by a Kruskal-Wallis test (H(4) = 180.14, p < 0.0001). Post-hoc Wilcoxon test suggest that KC equid δ13C values are significantly different than those at all other sites (p < 0.0001 according to Bonferroni correction), that there are significant differences in median equid δ13C values between Lake Victoria and Lukenya and Kalemba (p < 0.02), and that there is a significant difference in median δ13C values between Kalemba and Lake Turkana (p = 0.006, see Supplementary material SI3:5 for full details).
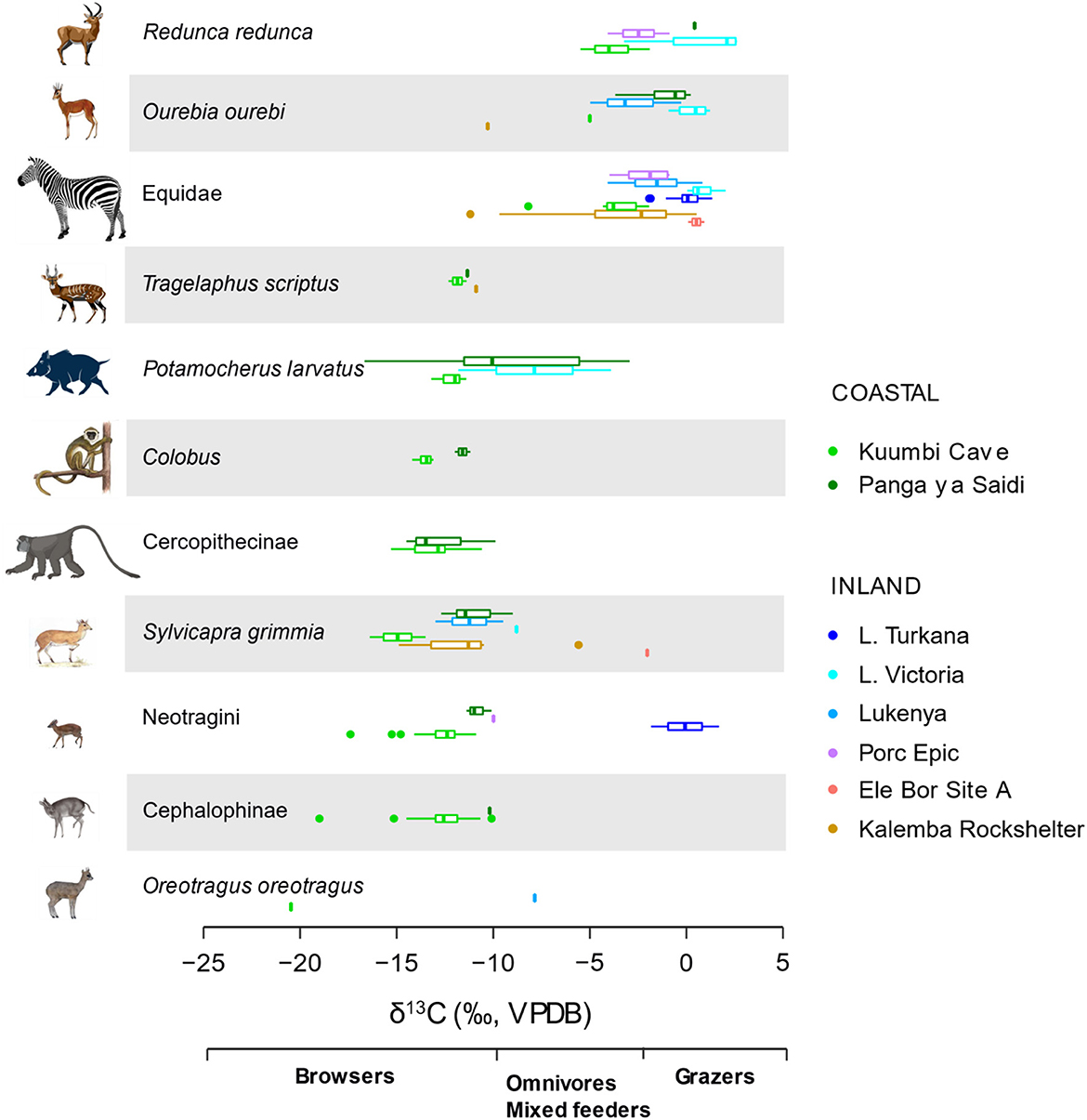
Figure 7. Box and jitter plots of δ13C values of taxa represented in most of the sites in eastern Africa. KC distribution is mostly nonoverlapping with those from inland sites.
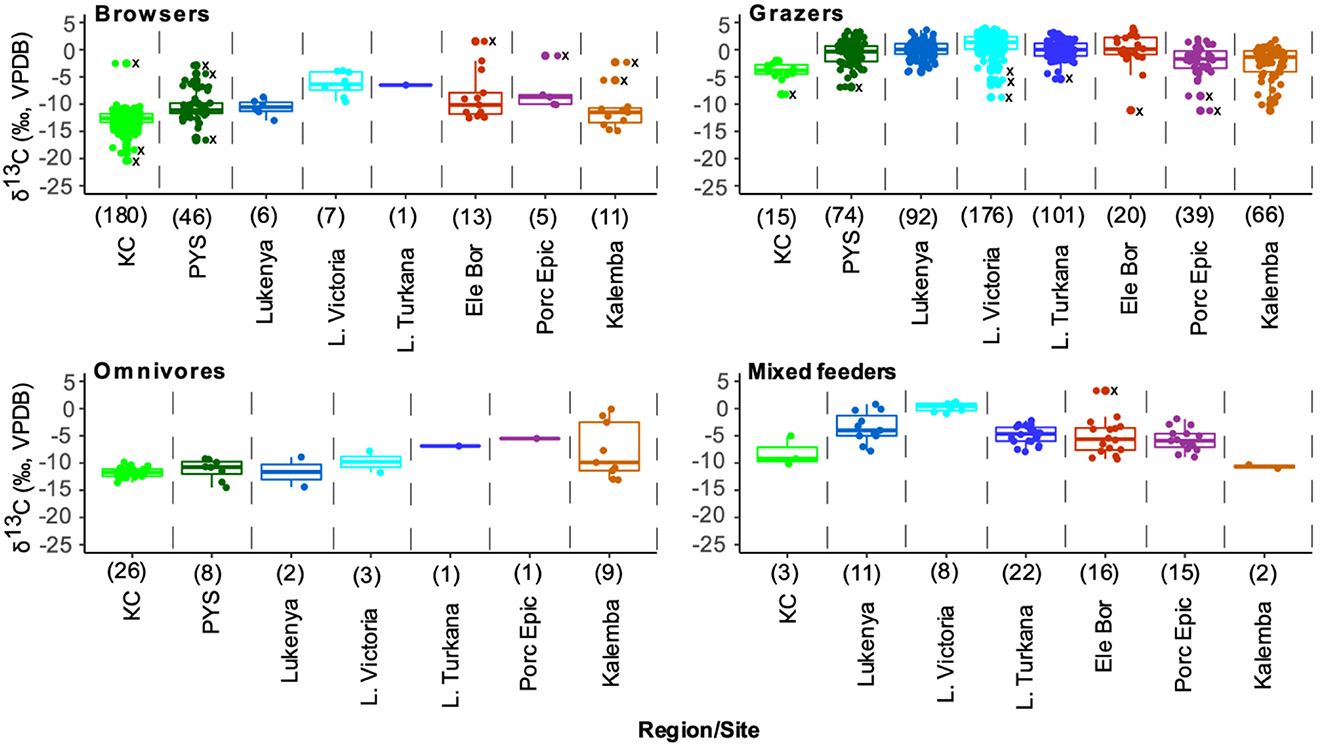
Figure 8. Box and jitter plots of δ13C values of feeding group by region and site in eastern Africa. Outliers are shown with dots and Xs along the midline of the plots. Sample sizes from each site or region are in brackets.
Browsers are sufficiently well represented in all sites except Turkana region to permit statistical comparisons (Figure 8 and Supplementary material SI3:5). A one-way analysis of variance suggests that there are significant differences in browser δ13C values among these sites (F(6,853) = 221.6, p < 0.0001). The δ13C model fails Levene's test for equal variance (F = 28.24, p < 0.0001), but significant differences in δ13C values among sites are still supported by a Kruskal-Wallis test (H(6) = 509.4, p < 0.0001). Post-hoc Wilcoxon test suggest that browser δ13C values from KC, Lake Victoria, and Lukenya are distinct from each other and from those of all other sites (p < 0.03 according to Bonferroni correction, see R output for full details). Post-hoc tests also suggest that the median Kalemba Rockshelter browser δ13C value is distinct from those at KC, Lake Victoria, and Lukenya, see Supplementary material SI3:5 for full details).
5. Discussion
5.1. Late Pleistocene and late holocene palaeoecology at Kuumbi Cave
The isotopic data are supported by previous zooarchaeological work at KC, which showed that the assemblage was comprised of animals living in habitats ranging from closed to open forests, woodlands, and grasslands but with an overall dominance of forest and woodland habitats [Supplementary material SI3:1-2 and Prendergast et al. (2016)]. The primate and hyrax species that are ubiquitous and abundant throughout are arboreal and adapted to dense forest cover (Kingdon, 1984b, 2001a; Fleagle, 1999; Burgess and Clarke, 2000). Most of the bovids represented are endemic to closed forested habitats, but some, such as klipspringer, bush duiker, and bushbuck, are found in more mixed mosaic habitats (Gagnon and Chew, 2000; Cerling et al., 2003). Bush pigs, giant pouched rat, and porcupine are found in varied habitats from forests to woodland (Kingdon, 1984b, 2001a). Grassland adapted species are few, rare in numbers, and are not present in the later part of Phase I (reported as P1a in Prendergast et al., 2016). The isotope data similarly highlights the dominance of dense forests and woodlands as environments exploited by humans at the site, with small patches of open forests and woodlands, thickets, bushlands, and grasslands throughout the sequence. Significantly, as at PYS, the δ13C and δ18O values of faunal remains from KC are effective at discerning among forest, woodland, and grassland conditions (Roberts et al., 2020), with perhaps even greater resolution being possible at the level of forest niches as per Cerling et al. (2004).
Stable isotope and zooarchaeological data indicate that KC inhabitants exploited wild fauna adapted to diverse vegetation communities (Kingdon, 1984b, 2001a; Prendergast et al., 2016). The majority of terrestrial fauna are from closed and open forested niches (δ13C values between −15 and −9‰, n = 121). A small fraction of fauna seems to come from dense forest habitats (−21 to −15‰, n = 7). These δ13C values are consistent with sub canopy to canopy habitats within the forest (between −15 to −12‰), and the understory (−19 to −15‰). A small proportion of the fauna have values consistent with open woodland and grassland settings (−9 to 2‰, n = 19). Temporal comparison of δ13C values suggest that hunters may have focused on more open/peripheral parts of forests and woodlands during Phases IV and III when grasslands expanded from the mainland to the island (−15 to 1‰ for primates and hyrax, and −14 to −10‰ for browsing bovids, and −6 to −2‰ for grazers). However, during the time of islandisation (Phases II and I), hunters focused more on forest-dwelling prey (−19 to −10 ‰ for primates and hyrax and −21 to −8‰ for bovids, and −9 to −4 ‰ for zebra).
Apart from island formation and Iron Age anthropogenic landscape modification (Prendergast et al., 2016), expansion of forest cover may have contributed to the rarity and extirpation of grassland-adapted fauna at KC. This is likely a result of overall wetter Holocene climate conditions as well as the geographical context of islandisation leading to more mesic conditions (Prendergast et al., 2016; Shipton et al., 2016), particularly given the fact that foragers do not seem to show an unbalanced preference for grassland fauna in Phases IV and III. Although the sample size is small, grazer δ13C (n = 13, mean −3‰) and δ18O (mean −2‰) isotope values in Phases IV and III are relatively high and narrower in range than δ13C (n = 2, mean −6.3‰) and δ18O (mean −3.6‰) values in Phase II. The relatively low δ13C and δ18O values in Phase II may reflect contracting grassland habitat that pushed grazers away from the KC. Precise times of extirpation are not well established, but faunal occurrence data show that buffalo are only present in Phase III, and waterbuck are present in Phases II, III, and IV. Reedbuck and zebra are rare in all the phases and disappear in later Phase I. Indeterminate mammal size 3–4 (which are the appropriate size for waterbuck, zebra, and buffalo) remains are also rare in Phases II and I. The influx of Iron Age farmers may have exacerbated defaunation by contributing to the disappearance of reedbuck and zebra in the KC sequence.
Several stable isotope studies have highlighted that δ18O values may reflect food resource partitioning among arboreal primates, but that δ13C values do not (Krigbaum et al., 2013; Roberts et al., 2017; Lowry et al., 2021). The KC primates and hyrax δ13C and δ18O values seemingly capture intraspecies vertical food resource partitioning, however (Figure 9). The values reflect animals within each given taxon exploiting resources along a gradient between the forest floor and upper canopy (this could be considered partitioning between individuals within a given taxon). The cercopithecines, which includes vervet and blue monkeys, are versatile and generalized in habitat choice (Fleagle, 1999; Kingdon, 2001a; Campbell et al., 2006). They can inhabit the canopy and subcanopy, and even the understory of forested habitats, open and closed woodlands, and thickets (Kingdon, 2001b). Tree hyrax roam in between the understory to the canopy parts of a forest and are also common in evergreen thickets and forest mosaics (Kingdon, 2001a). Greater galago and Zanzibar bush baby are omnivorous and common in the understory to the subcanopy part of a forest. Members of these faunal taxa also adapt to alternative niches based on existing competitors (Kingdon, 2001b). Zanzibar colobus are exclusively canopy inhabitants, which explains the low range in their δ13C values and high δ18O values. The δ13C and δ18O values (see summary statistics) for primates and hyrax reflect these eclectic behaviors.
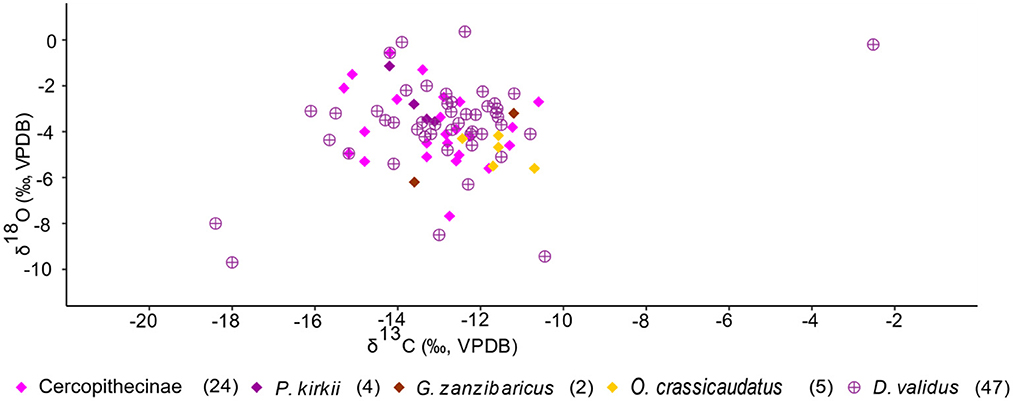
Figure 9. Scatter plot for arboreal fauna at KC (primates and hyrax). The values reflect animals within each given taxon exploiting resources along a gradient between the forest floor and upper canopy.
The extreme outlier (hyrax, Figures 5, 9) with high δ13C (−2.52‰) and δ18O (−0.2‰) values that reflect reliance on C4 resources is perplexing. No studies that we know have reported a similar observation from hyrax (Kingdon, 1984b, 2001a; Ambrose and DeNiro, 1986a,b; Cerling et al., 2004; la Grange, 2015).
While the zooarchaeological analyses and faunal stable isotope values provide broad insights into the niches being exploited by humans at KC, the two human δ13C and δ18O values (terminal Pleistocene −12.1 and −5.4‰, and Iron Age −10.7 and −3.1‰) provide direct insights into forager diet. In general, the human δ13C values are consistent with a dominance of forest/woodland C3 resources in their diets, overlapping with the faunal omnivore values at the site (δ13C −13.6 to −9.8‰ and δ18O −6.8 to −1‰). The Iron Age sample has slightly higher δ13C and δ18O values than the Pleistocene sample, though the small sample size precludes drawing any meaningful conclusions from this. These human and faunal values suggest that humans primarily subsisted on forest/woodland resources, but with varying contributions from denser canopied areas and more open settings. The high numbers of arboreal and other forest adapted taxa dominated by primates, hyrax, and the dwarf antelopes (Neotragini, Cephalophini) in the archaeological record supports the forest/woodland subsistence interpretation. The persistence of stone tools, and the near-absence of domesticated animals, crops, and iron amplify the likelihood of this adaptation (Prendergast et al., 2016; Shipton et al., 2016; Culley et al., 2021b). Ancient DNA also shows that the KC Iron Age human is most closely related to ancient and present-day coastal foragers (Skoglund et al., 2017). This means a lack of direct biological interaction with food producing groups who have more mixed genetic signatures.
5.2. Kuumbi Cave in the wider eastern African context
Comparison of the zooarchaeological assemblage and isotopic dataset from KC with PYS and other interior eastern African sites highlights spatial variation in forager subsistence in eastern Africa (Robinson, 2017, 2019; Prendergast, 2020; Roberts et al., 2020; Jones and Brandt, 2022; Prendergast et al., 2023). Of the known late Pleistocene and late Holocene sites, KC has the highest proportion in NISP and MNI of forest-adapted species: primates, hyrax, bovids (Cephalophini and Neotragini) and rodent species, for any eastern Africa zooarchaeological assemblage (Gifford-Gonzalez and Kimengich, 1984; Mehlman, 1989; Marean, 1992a; Prendergast et al., 2007, 2013; Robinson, 2017, 2019; Chritz et al., 2019). The nearby coastal Kenyan sequence of PYS also documents a high number of forest-adapted species, such as suni, klipspringer, tree hyrax, vervet/blue monkeys, Colobus sp. (colobus monkey), and giant pouched rat, however, there is a greater persistence of open woodland and grassland fauna at the site, such as kongoni/topi (Alcelaphus/Damaliscus), waterbuck, blue wildebeest (Connochaetes taurinus), buffalo, warthog (Phacochoerus sp.), and reedbuck (Shipton et al., 2018; Roberts et al., 2020; Culley et al., 2021a; Faulkner et al., 2021; Prendergast et al., 2023).
Apart from Enkapune ya Muto (EYM) rock shelter and Gamble's Cave, Central Rift Valley, Kenya, sites in the interior dating to the Pleistocene and Holocene have yielded fauna generally focused on grassland environments, indicative of much more open conditions in these portions of eastern Africa. Sites in the Lake Eyasi basin, Mbulu Plateau and Serengeti in Tanzania, Lake Victoria and Lake Turkana region in Kenya, and other related sites such as Ele Bor, Lukenya Hill in Kenya, Porc Epic in Ethiopia, and Kalemba in Zambia (Figure 2) record high numbers of hyper-grazers that include blue wildebeest, hartebeest, waterbuck, topi, reedbucks (R. redunca, R. arundinum, R. fulvorufula), buffalo (S. caffer, S. cf. antiquus), beisa oryx (Oryx beisa), common and Grevy's zebra (E. quagga and grevyi), and warthog (Phacochoerus africanus) (Mehlman, 1989; Marean, 1992b; Gifford-Gonzalez, 2003; Dale, 2007; Prendergast et al., 2007, 2013; Prendergast, 2010; Robinson, 2017, 2019; Grillo et al., 2018; Chritz et al., 2019). Primates, hyrax, and rodents are rare in the interior eastern African sites. The faunal δ13C and δ18O values from the mainland eastern African sites are relatively higher than KC, confirming the persistence of grasslands and open woodlands at the former (Figures 7, 8, Table 1, and Supplementary material SI3:5) (Robinson, 2017, 2019; Chritz et al., 2019; Roberts et al., 2020). Comparative statistical analysis of equid and browser δ13C values highlight the ecological uniqueness of KC (Supplementary material SI3:5). Statistical analysis also shows that foragers at KC hunted animals from the most diverse ecological niches, and the deepest or most forested parts of the forest when compared to other existing eastern African datasets (Figures 7, 8 and Supplementary material SI3:5 Tables S2a-d) (Robinson, 2017, 2019; Chritz et al., 2019; Roberts et al., 2020).
Comparison of human δ13C and δ18O enamel values from KC, PYS, and Kakapel documents a similar spatial diversity (Roberts et al., 2020; Wang et al., 2020). The PYS human δ13C (−11.4 and −11.1‰; MSA and Iron Age, respectively) and δ18O (−4.3 and −3.2‰; MSA and Iron Age, respectively) are very similar to the KC humans and document a focus on tropical forest and woodland resources. Unlike PYS and KC, Kakapel human isotope values from two individuals show a clear dietary shift throughout that sequence with Individual 1 (~4,000 BP) having lower δ13C and δ18O values (−10.6‰ and −1.4‰). The higher δ13C and δ18O values (−4.4‰ and −1‰) in Individual 2 (< 2300 BP) from this site have been associated with high consumption of finger millet and sorghum, with long-term cultivation of millet being proposed for this site (Mueller et al., 2022). Some collagen values from Kakapel (below −8‰ when normalized to enamel level) also reflect the dramatic changes associated with high consumption of domesticated C4 crops. The Kakapel case illustrates what might be expected to happen should domesticated C4 crops have been taken up meaningfully in the coastal regions. Similarly, humans reliant on cattle, sheep, and goat in eastern Africa have also been associated with more “C4” values (Ambrose, 1986; Bleasdale et al., 2020). The fact that this change is not seen at KC or PYS perhaps indicates a persistence of forest and woodland focused dietary strategies in these areas. These persistently low δ13C values indicate that KC and PYS humans did not significantly adopt domestic crops, such as sorghum and finger millet, as a dietary resource.
5.3. A climatic and economic “refugium”
The apparent stability of the eastern Africa coastal forest mosaic may have made it an important refugium for foragers during the Pleistocene glacial cycles, something argued for the MIS5-MIS1 sequence of PYS Cave (Shipton et al., 2018; Roberts et al., 2020). Most of the present-day biodiversity found in the coastal forests are relict species of the once connected Congo-Zanzibar-Inhambane forest mosaic (Kingdon, 1984b, 2001a; Burgess and Clarke, 2000). These include members of the faunal assemblage from KC (primates, bovids, suid, and rodent taxa) (Prendergast et al., 2016; Shipton et al., 2016). Climatic, palaeontological, and archaeological records suggest that the interior of eastern Africa was dominated by open woodlands and grasslands during glacial periods, but, during the interglacial periods, patches of forests and closed woodlands expanded (Kingdon, 1984a; Kiage and Liu, 2006; Bonnefille, 2010; Werdelin and Sanders, 2010). These dramatic changes seem to have been buffered in the coastal forest zone of PYS in Kenya and on Zanzibar, with coastal climate perhaps providing mesic conditions for the persistence of forests and woodlands. Interestingly, however, some subtle changes are visible. In the case of KC, connectivity to the mainland in the late Pleistocene may have resulted in more widespread grasslands, with subsequent isolation leading to an increase of forest and the relative decline of grazing taxa.
The relative stability of forest habitat at KC and PYS may also have initially contributed to foragers choosing to limit the uptake of agriculture, at least of C4 crops such as millet and sorghum or grazing domesticates, during the Iron Age. Human δ13C and δ18O values at Iron Age KC, as at PYS, suggest that these populations continued to focus on forest and woodland resources for their subsistence (Roberts et al., 2020). The dearth of domesticated animals at KC therefore may indicate a community of foragers agentively experimenting with the adoption of food production, rather than incoming food producers. Zooarchaeological and material culture evidence further support this point (Langley et al., 2016; Prendergast et al., 2016; Shipton et al., 2016). This includes a continuation of similar patterns of hunting and faunal diversity during the Iron Age, a dearth of domesticated fauna, a stone tool assemblage, and an absence of iron which is common in sites associated with established food production (Shipton et al., 2016). The site has also produced a crude version of local Tana Tradition (TT) and Triangular Incised Ware (TIW) ceramics. Compared to other Zanzibar Island sites, such as Unguja Ukuu and Fukuchani, KC ceramics differ in fabric: they contain much less sand temper, are more heavily fired, and have thicker body sherds (Shipton et al., 2016). Preliminary analyses of archaeobotanical assemblages from the site have also found no unequivocal crop remains thus far, but this work is still ongoing (Crowther et al., 2016).
A recent genetic study shows close affinity between ancient South African foragers and the KC Iron Age individual (Skoglund et al., 2017). Furthermore, they highlight that three island and coastal individuals–Makangale Cave, KC, and PYS—are closely related, with those from KC and PYS closest to one another (Skoglund et al., 2017; Lipson et al., 2022). These three individuals have ancestry components most closely related to eastern, southern, and, to a lesser extent, central African foragers. There is little genetic contribution to KC foragers from pastoralists. This further supports the use of the coastal region by foragers from interior Africa as a refugium during dry glacial periods, and the existence of a long-term resident forager population in this coastal region interacting with food producers during the Iron Age.
6. Conclusions
We present a multiproxy palaeoecological study on finds from KC, a stratified island site in coastal eastern Africa with four major phases spanning the late Pleistocene to the late Holocene. Using published zooarchaeological and new stable isotope data from the site, we highlight the persistence of forest-dominated mosaic habitats in the vicinity of KC during the last 20,000 years BP, and the stability of a specialized hunting strategy focused on forest-adapted taxa from different ecological niches. We argue that the eastern African coastal region and islands' stable forest mosaic habitats served as a refugium for foragers during ecological transformations dictated by orbital forcing, although forest expansion following island formation seems to have contributed to the extirpation of some fauna. It is possible that the spread of food production further exacerbated this latter turnover, although we argue that, overall, the Iron Age inhabitants of KC were foragers experimenting with food production, with long-term strategies of multi-habitat foraging dictating the uptake of novel economic and social forms.
Data availability statement
The original contributions presented in the study are included in the article/Supplementary material, further inquiries can be directed to the corresponding authors.
Author contributions
Conceived and designed the experiments: VI, PR, MS, SH, SG, AJ, ML, JI, MP, and NB. Performed the experiments: VI, PR, MS, SH, AC, AJ, SG, MB, CC, ML, JI, MP, and NB. Analyzed the data: VI, PR, SH, SG, and MS. Contributed reagents/materials/analysis tools: PR, SH, ML, JI, MP, and NB. Wrote the paper: VI, PR, MS, SH, SG, AC, AJ, MB, CC, ML, JI, MP, and NB. All authors contributed to the article and approved the submitted version.
Funding
Funding was provided by the SEALINKS project under a European Research Council (ERC) grant (no. 206148) awarded to NB and the Max Planck Society (MPG).
Acknowledgments
We thank the Office of Chief Government Statistician, Zanzibar Research Committee, and the Department of Archives, Museums and Antiquities (DAMA), for permission to excavate. We are particularly indebted to Amina Issa and Abdallah Ali from DAMA for supporting our research on Zanzibar. We are grateful for the involvement of Ruth Tibesasa on the excavations and for the permission and collaboration of the cave's local custodians, particularly: Bandari Ame Haji, Asha Ali Makame, Meja Haji Nyonje, Nyonje Pandu Nyonje, and Hassan Ali. MP thanks Ogeto Mwebi and staff of the Osteology Unit, National Museums of Kenya, as well as Humphrey Mathenge and the staff of the British Institute in Eastern Africa.
Conflict of interest
The authors declare that the research was conducted in the absence of any commercial or financial relationships that could be construed as a potential conflict of interest.
Publisher's note
All claims expressed in this article are solely those of the authors and do not necessarily represent those of their affiliated organizations, or those of the publisher, the editors and the reviewers. Any product that may be evaluated in this article, or claim that may be made by its manufacturer, is not guaranteed or endorsed by the publisher.
Supplementary material
The Supplementary Material for this article can be found online at: https://www.frontiersin.org/articles/10.3389/fearc.2023.1080785/full#supplementary-material
Supplementary Table 1. KC faunal isotope dataset.
Supplementary Table 2. Mainland EA sites faunal isotope dataset.
References
Ambrose, S. (1984a). Holocene Environments and Human Adaptations in the Central Rift Valley: PhD University of California.
Ambrose, S. (1984b). “THE Introduction of pastoral adaptations to the highlands of East Africa,” in From Hunters to Farmers: The Causes and Consequences of Food Production in Africa, eds. D. Clark and S.A. Brandt. (London: University of California Press) 212–421.
Ambrose, S. (1986). Stable carbon and nitrogen isotope analysis of human and animal diet in Africa. J. Human Evolut. 15, 707–731.
Ambrose, S. (1998). Chronology of the Later Stone Age and food production in East Africa. J. Archaeol. Sci. 25, 377–392. doi: 10.1006/jasc.1997.0277
Ambrose, S. H., Collett, D., Collett, D., Marshall, F. (1984). Excavations at Deloraine, Rongai, 1978. Azania: Archaeol. Res. Africa 19, 79–104. doi: 10.1080/00672708409511329
Ambrose, S. H., DeNiro, M. J. (1986a). The isotopic ecology of East African mammals. Oecologia 69, 395–406. doi: 10.1007/bf00377062
Ambrose, S. H., DeNiro, M. J. (1986b). Reconstruction of African human diet using bone collagen carbon and nitrogen isotope ratios. Nature 319, 321–324.
Barnosky, A. D., Koch, P. L., Feranec, R. S., Wing, S. L., Shabel, A. B. (2004). Assessing the causes of late Pleistocene extinctions on the continents. Science 306, 70–75. doi: 10.1126/science.1101476
Barthelme, J. (1985). Fisher-Hunters and Neolithic Pastoralists in East Turkana, Kenya. Oxford: Cambridge Monographs in African Archaeology 13 (B.A.R. International Series 254).
Bleasdale, M., Wotzka, H-. P., Eichhorn, B., Mercader, J., Styring, A., Zech, J., et al. (2020). Isotopic and microbotanical insights into Iron age agricultural reliance in the Central African rainforest. Commun. Biol. 3, 619. doi: 10.1038/s42003-020-01324-2
Bonnefille, R. (2010). Cenozoic vegetation, climate changes and hominid evolution in tropical Africa. Global Planetary Change 72, 390–411. doi: 10.1016/j.gloplacha.2010.01.015
Bonnefille, R., Roeland, J. C., Guiot, J. (1990). Temperature and rainfall estimates for the past 40,000 years in equatorial Africa. Nature 346, 347–349. doi: 10.1038/346347a0
Braje, T. J., Leppard, T. P., Fitzpatrick, S. M., Erlandson, J. M. (2017). Archaeology, historical ecology and anthropogenic island ecosystems. Environ. Conserv. 44, 286–297. doi: 10.1017/S0376892917000261
Buchmann, N., Ehleringer, J. R. (1998), CO2. concentration profiles, carbon oxygen isotopes in C3 C4 crop canopies. Agricult. Forest Meteorol. 89, 45–58. doi: 10.1016/S0168-1923(97)00059-2
Buchmann, N., Guehl, J. M., Barigah, T. S., Ehleringer, J. R. (1997). Interseasonal comparison of CO2 concentrations, isotopic composition, and carbon dynamics in an Amazonian rainforest (French Guiana). Oecologia 110, 120–131. doi: 10.1007/s004420050140
Burgess, N. D., Clarke, G. P. (2000). Coastal Forests of Eastern Africa. Cambridge: IUCN - The World Conservation Union.
Campbell, C., Fuentes, C., Mackonnon, K. M., Panger, M., Bearder, S. K. (2006). Primates in Perspective. New York: Oxford University Press.
Carter, M., Bradbury, M. (2015). Oxygen isotope ratios in Primate bone carbonate reflect amount of leaves and vertical stratification in the diet. Am. J. Primatol. 78, 2432. doi: 10.1002./ajp.22432
Cerling, T., Andanje, S. A., Blumenthal, S. A., Brown, F. H., Chritz, K. L., Harris, J. M., et al. (2015). Dietary changes of large herbivores in the Turkana Basin, Kenya from 4 to 1 Ma. Proceed. Nat. Acad. Sci. 112, 11467–11472. doi: 10.1073/pnas.1513075112
Cerling, T., Harris, J. M., Leakey, M. G., Passey, B. H., Levin, N. (2010). “Stable Carbon and Oxygen Isotopes in East African Mammals: Modern and Fossil,” in Cenozoic Mammals of Africa, eds. L. Werdelin and W.J. Sanders. (Los Angeles: University of California Press) 949–960.
Cerling, T. E., Harris, J. M. (1999). Carbon isotope fractionation between diet and bioapatite in ungulate mammals and implications for ecological and paleoecological studies. Oecologia 120, 347–363. doi: 10.1007/s004420050868
Cerling, T. E., Harris, J. M., Passey, B. H. (2003). Diets of East African Bovidae based on stable isotope analysis. J. Mammol. 84, 456–470. doi: 10.1644/1545-1542(2003)084<0456:DOEABB>2.0.CO;2
Cerling, T. E., Hart, J. A., Hart, T. B. (2004). Stable isotope ecology in the Ituri forest. Oecologia 138, 5–12. doi: 10.1007/s00442-003-1375-4
Chami, F. A. (2007). Diffusion in the studies of the African past: reflections from new archaeological findings. Af. Archaeol. Rev. 24, 1–14. doi: 10.2307./40645410
Chami, F. A. (2009). Zanzibar and the Swahili Coast from c.30, 000. Years Ago. Dar es Salaam: EandD Vision Publishing.
Chritz, K. L., Cerling, T. E., Freeman, K. H., Hildebrand, E. A., Janzen, A., Prendergast, M. E., et al. (2019). Climate, ecology, and the spread of herding in eastern Africa. Quarter. Sci. Rev. 204, 119–132. doi: 10.1016/j.quascirev.11029
Codron, D., Sponheimer, M., Codron, J., Newton, I., Lanham, J., Clauss, M., et al. (2012). The confounding effects of source isotopic heterogeneity on consumer-diet and tissue-tissue stable isotope relationships. Oecologia 169, 939–953. doi: 10.1007/s00442-012-2274-3
Craig, H. (1953). The geochemistry of the stable carbon isotopes. Geochimica et Cosmochim. Acta 3, 53–92. doi: 10.1016/0016-7037(53)90001-5
Crowley, B. (2012). Stable isotope techniques and applications for primatologists. Int. J. Primatol. 33, 7. doi: 10.1007./s10764-012-9582-7
Crowther, A., Lucas, L., Helm, R., Horton, M., Shipton, C., Wright, H. T., et al. (2016). Ancient crops provide first archaeological signature of the westward Austronesian expansion. Proceed. Nat. Acad. Sci. USA. 113, 6635–6640. doi: 10.1073/pnas.1522714113
Crowther, A., Prendergast, M. E., Fuller, D. Q., Boivin, N. (2018). Subsistence mosaics, forager-farmer interactions, and the transition to food production in eastern Africa. Quarter. Int. 30, 1–20. doi: 10.1016/j.quaint.2017.01.014
Culley, C., Janzen, A., Brown, S., Prendergast, M. E., Shipton, C., Ndiema, E., et al. (2021a). Iron Age hunting and herding in coastal eastern Africa: ZooMS identification of domesticates and wild bovids at Panga ya Saidi, Kenya. J. Archaeol. Sci. 130, 105368. doi: 10.1016/j.jas.2021.105368
Culley, C., Janzen, A., Brown, S., Prendergast, M. E., Wolfhagen, J., Abderemane, B., et al. (2021b). Collagen fingerprinting traces the introduction of caprines to island Eastern Africa. Royal Soc. Open Sci. 8, 202341. doi: 10.1098/rsos.202341
Dale, D. D. (2007). An Archaeological Investigation of the Kansyore, Later Stone Age Hunter-Gatherers in East Africa. PhD Washington University in Saint Louis.
De Cort, Bessems, G., Keppens, I., Mees, E., Cumming, F. (2013). B., and Verschuren, D. (2013). Late-Holocene and recent hydroclimatic variability in the central Kenya Rift valley: the sediment record of hypersaline lakes Bogoria, Nakuru and Elementeita. Palaeogeography Palaeoclimatol. Palaeoecol. 388, 69–80. doi: 10.1016/j.palaeo.07029
DeNiro, M. J., Epstein, S. (1978). Influence of diet on the distribution of carbon isotopes in animals. Geochimica et Cosmochimica Acta 42, 495–506. doi: 10.1016/0016-7037(78)90199-0
Faith, J. T. (2014). Late pleistocene and holocene mammal extinctions on continental Africa. Earth-Sci. Rev. 128, 105–121. doi: 10.1016/j.earscirev.10009
Farquhar, G. D., Ehleringer, J. R., Hubick, K. T. (1989). Carbon isotope discrimination and photosynthesis. Ann. Rev. Plant Physiol. Plant Mol. Biol. 40, 503–537. doi: 10.1146/annurev.pp.40.060189.002443
Faulkner, P., Miller, J. M., Quintana Morales, E. M., Crowther, A., Shipton, C., Ndiema, E., et al. (2021). 67, 000. years of coastal engagement at Panga ya Saidi, eastern Africa. PLoS ONE 16, e0256761. doi: 10.1371/journal.pone.0256761
Fitzpatrick, S. M., Rick, T. C., Erlandson, J. M. (2015). Recent progress, trends, and developments in island and coastal archaeology. J. Island Coastal Archaeol. 10, 3–27. doi: 10.1080/15564894.2015.1013647
Foerster, V., Vogelsang, R., Junginger, A., Asrat, A., Lamb, H. F., Schaebitz, F., et al. (2015). (2015). Environmental change and human occupation of southern Ethiopia and northern Kenya during the last 20,000 years. Quat. Sci. Rev. 129, 333–340. doi: 10.1016/j.quascirev.10026
Gagnon, M., Chew, A. E. (2000). Dietary preferences in extant African Bovidae. J. Mammal. 81, 490–511. doi: 10.1644/1545-1542(2000)081<0490:DPIEAB>2.0.CO;2
Gelorini, V., Verschuren, D. (2013). Historical climate-human-ecosystem interaction in East Africa: a review. Af. J. Ecol. 51, 409–421. doi: 10.1111/aje.12045
Giblin, J., Fuller, D. (2011). First and second millennium AD agriculture in Rwanda: archaeobotanical finds and radiocarbon dates from seven sites. Veget. History Archaeob. 20, 253–265. doi: 10.1007/s00334-011-0288-0
Gifford-Gonzalez, D. (1998). Early pastoralists in East Africa: ecological and social dimensions. J. Anthropol. Archaeol. 17, 166–200. doi: 10.1006/jaar.1998.0322
Gifford-Gonzalez, D. (2003). The fauna from Ele Bor: evidence for the persistence of foragers into the later Holocene of arid North Kenya. Af. Archaeol. Rev. 20, 81–119. doi: 10.1023/A:1024410814287
Gifford-Gonzalez, D. P., Kimengich, J. (1984). “Faunal Evidence for Early Stock-keeping in the Central Rift of Kenya: Preliminary Finds,” in Origin and Early Development of Food-Producing Cultures in North-Eastern Africa, eds. L. Krzyzaniak and M. Kobusiewicz. (Poznan: Polish Academy of Sciences and Poznan Archaeological Museum) 457–471.
Giraldo, J., del Valle, J., González-Caro, S., Sierra, C. (2022). Intra-annual isotope variations in tree rings reveal growth rhythms within the least rainy season of an ever-wet tropical forest. Trees. 4, 7. doi: 10.1007./s00468-022-02271-7
Grillo, K., Prendergast, M., Contreras, D., Fitton, T., Gidna, A., Goldstein, S., et al. (2018). Pastoral Neolithic settlement at Luxmanda, Tanzania. J. Field Archaeol. 43, 102–120. doi: 10.1080/00934690.2018.1431476
Hardy, A., Mageni, Z., Dongus, S., Killeen, G., Macklin, M. G., Majambare, S., et al. (2015). Mapping hotspots of malaria transmission from pre-existing hydrology, geology and geomorphology data in the pre-elimination context of Zanzibar, United Republic of Tanzania. Parasites Vect. 8, 41. doi: 10.1186/s13071-015-0652-5
Horton, M. (2017). “Zanzibar and Pemba: the archaeology of an Indian Ocean Archipelago”. (British Institute in Eastern Africa).
Jones, M. B., Brandt, S. A. (2022). 20, 000. years of small game hunting in southern Somalia. Quat. Int. 3, 4. doi: 10.1016/j.quaint.2022.06.004
Kiage, L. M., Liu, K. (2006). Late Quaternary paleoenvironmental changes in East Africa: a review of multiproxy evidence from palynology, lake sediments, and associated records. Prog. Phys. Geograp. 30, 633–658. doi: 10.1177/0309133306071146
Kingdon, J. (1984a). “East African Environment,” in East African Mammals: an Atlas of Evolution in Africa, ed. J. Kingdon. (Chicago: The University of Chicago Press) 8–21.
Kingdon, J. (1984b). East African Mammals: An Atlas of Evolution in Africa. Chicago: The University of Chicago Press.
Kingdon, J. (2001b). “Primates,” in The Kingdon Field Guide to African Mammals, ed. J. Kingdon. (San Diego: Academic Press) 8–111.
Kohn, M. J., Schoeninger, M. J., Valley, J. W. (1996). Herbivore tooth oxygen isotope compositions: effects of diet and physiology. Geochim. et Cosmochim. Acta 60, 3889–3896. doi: 10.1016/0016-7037(96)00248-7
Kohn, M. J., Schoeninger, M. J., Valley, J. W. (1998). Variability in oxygen isotope compositions of herbivore teeth: reflections of seasonality or developmental physiology? Chem. Geol. 152, 97–112.
Krigbaum, J., Berger, M. H., Daegling, D. J., McGraw, W. S. (2013). Stable isotope canopy effects for sympatric monkeys at Tai Forest Cote d'Ivoire. Biol. Lett. 9, 20130466. doi: 10.1098/rsbl.2013.0466
Kusimba, S. B. (1999). Hunter–gatherer land use patterns in Later Stone Age East Africa. J. Anthropol. Archaeol. 18, 165–200. doi: 10.1006/jaar.1998.0335
Kusimba, S. B. (2013). “Hunter-Gatherer-Fishers of Eastern and South-Central Africa Since 20, 000. Years Ago”, in: The Oxford Handbook of African Archaeology. (eds.) P. Mitchell and P.J. Lane. (Oxford: Oxford University Press).
la Grange, L. (2015). Rock Hyraxes (Procavia capensis) and their Environments: δ 13Ccollagen and δ 15Ncollagen as Environmetal Indicators. Master of Philosophy, University of Cape Town.
Lane, P. (2013). “The Archaeology of Pastoralism and Stock-Keeping in East Africa”, in: The Oxford Handbook of African Archaeology. (eds.) P. Mitchell and P. Lane. (Oxford: Oxford University Press).
Langley, M. C., Prendergast, M. E., Shipton, C., Quintana Morales, E. M., Crowther, A., Boivin, N., et al. (2016). Poison arrows and bone utensils in late Pleistocene eastern Africa: evidence from Kuumbi Cave, Zanzibar. Azania: Archaeol. Res. Africa 51, 155–177. doi: 10.1080/0067270X.2016.1173302
Lee-Thorp, J., Likius, A., Mackaye, H. T., Vignaud, P., Sponheimer, M., Brunet, M., et al. (2012). Isotopic evidence for an early shift to C4 resources by Pliocene hominins in Chad. Proceed. Nat. Acad. Sci. 109, 20369–20372. doi: 10.1073/pnas.1204209109
Lee-Thorp, J. A., van der Merwe, N. J., Brain, C. K. (1989). Isotopic evidence for dietary differences between two extinct baboon species from Swartkrans. J. Human Evolut. 18, 183–189.
Levin, N., Simpson, S. W., Quade, J., Cerling, T. E., Frost, S. (2008). Herbivore enamel carbon isotopic composition and the environmental context of Ardipithecus at Gona, Ethiopia. Geol. Soc. Am. 446, 251–234. doi: 10.1130/2008.2446(10)
Levin, N. E., Cerling, T. E., Passey, B. H., Harris, J. M., Ehleringer, J. R. (2006). A Stable isotope aridity index for terrestrial environments. Proceed. Nat. Acad. Sci. 103, 11201–11205. doi: 10.1073/pnas.0604719103
Lipson, M., Sawchuk, E. A., Thompson, J. C., Oppenheimer, J., Tryon, C. A., Ranhorn, K. L., et al. (2022). Ancient DNA and deep population structure in sub-Saharan African foragers. Nature 4, 9. doi: 10.1038./s41586-022-04430-9
Lowry, B. E., Wittig, R. M., Pittermann, J., Oelze, V. M. (2021). Stratigraphy of stable isotope ratios and leaf structure within an African rainforest canopy with implications for primate isotope ecology. Scient. Reports 11, 14222. doi: 10.1038/s41598-021-93589-8
Marchant, R., Lane, P. (2014). Past perspectives for the future: foundations for sustainable development in East Africa. J. Archaeol. Sci. 51, 12–21. doi: 10.1016/j.jas.07005
Marchant, R., Richer, S., Boles, O., Capitani, C., Courtney-Mustaphi, C. J., Lane, P., et al. (2018). Drivers and trajectories of land cover change in East Africa: Human and environmental interactions from 6,000 years ago to present. Earth-Sci. Rev. 178, 322–378. doi: 10.1016/j.earscirev.12010
Marean, C. W. (1992a). Hunter to herder: large mammal remains from the hunter-gatherer occupation at Enkapune Ya Muto rock-shelter, central Rift, Kenya. Af. Archaeol. Rev. 10, 65–127. doi: 10.1007/BF01117697
Marean, C. W. (1992b). Implications of late Quaternary mammalian fauna from Lukenya Hill (South-central Kenya) for paleoenvironmental change and faunal extinctions. Quat. Res. 37, 239–255. doi: 10.1016/0033-5894(92)90085-W
Marshall, F. (1990). (1990). Origins of specialized pastoral production in East Africa. Am. Anthropol. 92, 873–894. doi: 10.1525/aa.924.02a00020
Marshall, F., Grillo, K., Arco, L. (2011). “Prehistoric pastoralists and social responses to climatic risk in East Africa,” in Sustainable lifeways: cultural persistence in an ever-changing environment, eds. N. Miller, K. Moore and K. Ryan. (Philadelphia: University of Pennsylvania Press).
Marshall, F., Hildebrand, E. (2002). Cattle before crops: the beginnings of food production in Africa. J. World Prehistory 16, 99–143. doi: 10.1023/A:1019954903395
Marshall, F., Stewart, K., Barthelme, J. (1984). Early domestic stock at Dongodien in northern Kenya. Azania: Archaeol. Res. Af.19, 120–127. doi: 10.1080/00672708409511332
Mehlman, M. J. (1989). Later Quaternary Archaeological Sequences in Northern Tanzania. PhD University of Illinois at Urbana-Champaign.
Moreau, R. E., Pakenham, R. H. W. (1940). Faunistics and Ages of the East African Islands. Nature 146, 462–462. doi: 10.1038/146462a0
Moreau, R. E., Pakenham, R. H. W. (1941). The land vertebrates of Pemba, Zanzibar, and Mafia: zoogeographical study. Proceed. Zool. Soc. London 110, 97–128.
Mueller, N., Goldstein, S., Odeny, D., Boivin, N. (2022). Variability and preservation biases in the archaeobotanical record of Eleusine coracana (finger millet): evidence from Iron Age Kenya. Vegetat. History Archaeobot. 31, 853. doi: 10.1007./s00334-021-00853-y
Prendergast, M. (2010). Kansyore fisher-foragers and transitions to food production in East Africa: the view from Wadh Lang'o, Nyanza Province, Western Kenya. Azania: Archaeol. Res. Af. 45, 83–111. doi: 10.1080/00672700903291765
Prendergast, M. (2011). “Hunters and Herders at the Periphery: The Spread of Herding in Eastern Africa,” in People and Animals in Holocene Africa: Recent Advances in Archaeozoology, eds. H. Jousse and J. Lesur. (Frankfurt: Africa Magna Verlag).
Prendergast, M., Luque, L., Dominguez-Rodrigo, M., Diez-Martín, F., Mabulla, A. Z. P., Barba, R., et al. (2007). New excavations at Mumba Rockshelter, Tanzania. J. African Archaeol. 5, 217–243. doi: 10.3213/1612-1651-10093
Prendergast, M., Rouby, H., Punnwong, P., Marchant, R., Crowther, A., Kourampas, N., et al. (2016). Continental island formation and the archaeology of defaunation on Zanzibar, eastern Africa. PLoS ONE 11, e0149565. doi: 10.1371/journal.pone.0149565
Prendergast, M. E. (2009). Forager variability and transitions to food production in secondary settings: Kansyore and Pastoral Neolithic economies in East Africa. Azania: Archaeol. Res. Af. 44, 276–277. doi: 10.1080/00671990903052330
Prendergast, M. E. (2020). “The History of Eastern African Foragers”. (Oxford: Oxford University Press).
Prendergast, M. E., Beyin, A. (2018). Fishing in a fluctuating landscape: terminal Pleistocene and early Holocene subsistence strategies in the Lake Turkana Basin, Kenya. Quat. Int. 471, 203–218. doi: 10.1016/j.quaint.04022
Prendergast, M. E., Mabulla, A. Z. P., Grillo, K. M., Broderick, L. G., Seitsonen, O., Gidna, A. O., et al. (2013). Pastoral Neolithic sites on the southern Mbulu Plateau, Tanzania. Azania Archaeol. Res. Af.48, 498–520. doi: 10.1080/0067270X.2013.841927
Prendergast, M. E., Miller, J., Mwebi, O., Ndiema, E., Shipton, C., Boivin, N., et al. (2023). Small game forgotten: late pleistocene foraging strategies in eastern Africa, and remote capture at Panga ya Saidi, Kenya. Quat. Sci. Rev. 305, 108032. doi: 10.1016/j.quascirev.2023.108032
R Core Team. (2013). R: A language and environment for statistical computing. R Foundation for Statistical Computing [Online]. Available online at: http://www.R-project.org/
Revel, M., Ducassou, E., Skonieczny, C., Colin, C., Bastian, L., Bosch, D., et al. (2015). 20, 000. years of Nile River dynamics and environmental changes in the Nile catchment area as inferred from Nile upper continental slope sediments. Quat. Sci. Rev. 130, 200–221. doi: 10.1016/j.quascirev.2015.10.030
Rick, T. C., Kirch, P. V., Erlandson, J. M., Fitzpatrick, S. M. (2013). (2013). Archeology, deep history, and the human transformation of island ecosystems. Anthropocene 4, 33–45. doi: 10.1016/j.ancene.08002
Roberts, P., Perera, N., Wedage, O., Deraniyagala, S., Perera, J., Eregama, S., et al. (2017). (2017). Fruits of the forest: human stable isotope ecology and rainforest adaptations in Late Pleistocene and Holocene (~36 to 3 ka) Sri Lanka. J. Human Evolut. 106, 102–118. doi: 10.1016/j.jhevol.01015
Roberts, P., Prendergast, M. E., Janzen, A., Shipton, C., Blinkhorn, J., Zech, J., et al. (2020). Late Pleistocene to Holocene human palaeoecology in the tropical environments of coastal eastern Africa. Palaeogeography Palaeoclimatol. Palaeoecol. 537, 109438. doi: 10.1016/j.palaeo.2019.109438
Robertshaw, P. (2021). “Archaeology of Early Pastoralism in East Africa”. (Oxford: Oxford University Press).
Robinson, J. R. (2017). Thinking locally: environmental reconstruction of middle and later stone age archaeological sites in Ethiopia, Kenya, and Zambia based on ungulate stable isotopes. J. Hum. Evol. 106, 19–37. doi: 10.1016/j.jhevol.01013
Robinson, J. R. (2019). A Holocene paleoenvironmental record based on ungulate stable isotopes from Lukenya Hill, Kenya. J. Archaeol. Sci. Reports 28, 102016. doi: 10.1016/j.jasrep.2019.102016
Robinson, J. R. (2022). Investigating habitat heterogeneity of Late Pleistocene archaeological sites in eastern Africa from stable isotopes. Historical Biol. 34, 674–693. doi: 10.1080/08920211942465
Schaebitz, F., Asrat, A., Lamb, H. F., Cohen, A. S., Foerster, V., Duesing, W., et al. (2021). Hydroclimate changes in eastern Africa over the past 200,000 years may have influenced early human dispersal. Commun. Earth Environ. 2, 123. doi: 10.1038/s43247-021-00195-7
Shipton, C., Crowther, A., Kourampas, N., Prendergast, M. E., Horton, M., Douka, K., et al. (2016). Reinvestigation of Kuumbi Cave, Zanzibar, reveals later stone age coastal habitation, early Holocene abandonment and Iron Age reoccupation. Azania Archaeol. Res. iAfrica 51, 197–233. doi: 10.1080/0067270X.2016.1173308
Shipton, C., Roberts, P., Archer, W., Armitage, S. J., Bita, C., Blinkhorn, J., et al. (2018). 78, 000.-year-old record of middle and later stone age innovation in an East African tropical forest. Nat. Commun. 9, 1832. doi: 10.1038/s41467-018-04057-3
Silveira, d. a. L.S.L. (1989). ”Oxygen and Hydrogen Isotope Ratios in Plant Cellulose: Mechanisms and Applications,” in Stable Isotopes in Ecological Research Conference, eds. P.W. Rundel, J.R. Ehleringer and K.A. (Nagy: Springer New York), 124–141.
Sinclair, P., Juma, A., Chami, F. (2006). Excavations at Kuumbi Cave on Zanzibar 2005. Dar es Salaam: Dar es Salaam University Press.
Sirak, K. A., Sawchuk, E. A., Prendergast, M. E. (2022). “Ancient Human DNA and African Population History”. (Oxford University Press).
Skoglund, P., Thompson, J. C., Prendergast, M. E., Mittnik, A., Sirak, K., Hajdinjak, M., et al. (2017). (2017). Reconstructing prehistoric African population structure. Cell 171, 59–71.e21. doi: 10.1016/j.cell.08049
Smith, B. N., Epstein, S. (1971). Two categories of 13C/12C ratios for higher plants Plant Physiol. 47, 380–384. doi: 10.1104/pp.47.3.380
Sponheimer, M., Lee-Thorp, J., Ruiter, D. d., Codron, D., Codron, J., Baugh, A.T., et al. (2005). Hominins, sedges, and termites: new carbon isotope data from the Sterkfontein Valley and Kruger National Park. J. Human Evolut. 48, 301–312. doi: 10.1016/j.jhevol.2004.11.008
Stager, J. C., Cumming, B. F., Meeker, L. D. (2017). A 10,000-year high-resolution diatom record from Pilkington Bay, Lake Victoria, East Africa. Quat. Res. 59, 172–181. doi: 10.1016/s0033-5894(03)00008-5
Stager, J. C., Johnson, T. C. (2000). A 12,400 14c yr offshore diatom record from East central Lake Victoria, East Africa. J. Paleolimno. 23, 373–383. doi: 10.1023/A:1008133727763
Stager, J. C., Johnson, T. C. (2008). The Late Pleistocene desiccation of Lake Victoria and the origin of its endemic biota. Hydrobiologia 596, 5–16. doi: 10.1007/s10750-007-9158-2
Stuart, A. J. (2015). Late Quaternary megafaunal extinctions on the continents: a short review. Geol. J. 50, 338–363. doi: 10.1002/gj.2633
Talbot, M. R., Lærdal, T. (2000). The Late Pleistocene - Holocene palaeolimnology of Lake Victoria, East Africa, based upon elemental and isotopic analyses of sedimentary organic matter. J. Paleolimnol. 23, 141–164. doi: 10.1023/A:1008029400463
Tryon, C. A., Faith, J. T. (2016). A demographic perspective on the middle to later stone age transition from Nasera rockshelter, Tanzania. Philosoph. Transact. Royal Soc. B: Biol. Sci. 371, 20150238. doi: 10.1098/rstb.2015.0238
van der Merwe, N. J. (1982). Carbon isotopes, photosynthesis, and archaeology: different pathways of photosynthesis cause characteristic changes in carbon isotope ratios that make possible the study of prehistoric human diets. Am. Sci.70, 596–606.
van der Merwe, N. J., Medina, E. (1989). Photosynthesis and 13C/12C ratios in Amazonian rain forests. Geochim. et Cosmochim. Acta 53, 1091–1094.
van der Merwe, N. J., Medina, E. (1991). The canopy effect, carbon isotope ratios and foodwebs in Amazonia. J. Archaeol. Sci. 18, 249–259.
Vicente, M., Schlebusch, C. (2022). “Ancient DNA Studies and African Population History,” in Africa, The Cradle of Human Diversity: Cultural and Biological Approaches to Uncover African Diversity, eds. C. Schlebusch, C. Fortes-Lima and E. Mtetwa. (Leiden: Brill) 236–256.
Wang, K., Goldstein, S., Bleasdale, M., Clist, B., Bostoen, K., Bakwa-Lufu, P., et al. (2020). Ancient genomes reveal complex patterns of population movement, interaction, and replacement in sub-Saharan Africa. Sci. Adv. 6, eaaz0183. doi: 10.1126./sciadv.aaz0183
Werdelin, L., Sanders, W. J. (2010). Cenozoic Mammals of Africa. Berkeley: University of California Press.
White, F. (1983). The Vegetation of Africa: A Descriptive Memoir to Accompany the UNESCO/AETFAT/UNSO Vegetation Maps of Africa (3 plates), 1, 5. 000,000. Paris: UNESCO.
Keywords: climate change, human-environment interactions, stable carbon and oxygen isotopes, glacial period, holocene
Citation: Iminjili V, Stewart M, Culley C, Hixon S, Goldstein S, Bleasdale M, Jesús Sanchez Flores A, Lucas M, Ilgner J, Prendergast ME, Crowther A, Boivin N and Roberts P (2023) Late Pleistocene to late Holocene palaeoecology and human foraging at Kuumbi Cave, Zanzibar Island. Front. Environ. Archaeol. 2:1080785. doi: 10.3389/fearc.2023.1080785
Received: 26 October 2022; Accepted: 30 March 2023;
Published: 18 April 2023.
Edited by:
Xinyi Liu, Washington University in St. Louis, United StatesReviewed by:
Helina Woldekiros, Washington University in St. Louis, United StatesRachel Reid, Virginia Tech, United States
Copyright © 2023 Iminjili, Stewart, Culley, Hixon, Goldstein, Bleasdale, Jesús Sanchez Flores, Lucas, Ilgner, Prendergast, Crowther, Boivin and Roberts. This is an open-access article distributed under the terms of the Creative Commons Attribution License (CC BY). The use, distribution or reproduction in other forums is permitted, provided the original author(s) and the copyright owner(s) are credited and that the original publication in this journal is cited, in accordance with accepted academic practice. No use, distribution or reproduction is permitted which does not comply with these terms.
*Correspondence: Victor Iminjili, iminjili@shh.mpg.de; Patrick Roberts, roberts@shh.mpg.de