- 1Department of Geosciences, University of Cincinnati, Cincinnati, OH, United States
- 2Department of Anthropology, University of Cincinnati, Cincinnati, OH, United States
Isotopic analysis is destructive and requires that a specimen retains its original (biogenic) chemical composition. A specimen's relative abundance of calcium and phosphorous (Ca/P) or carbonate and phosphate (CO3/PO4) is often used to assess preservation. If a specimen's Ca/P or CO3/PO4 is similar to modern specimens, a specimen's isotopic composition may be biogenic. However, most methods for measuring these proxies are destructive. Moreover the relationships between Ca/P, CO3/PO4 and isotopic preservation are poorly established. In this study, we assessed the ability of handheld X-ray fluorescence (hXRF) to non-destructively evaluate a specimen's preservation by characterizing the calcium to phosphorous ratio (Ca/P). We first established that surface Ca/P (Ca/Psurface) for modern specimens was consistent with expectations for unaltered bone (1.3–2.3). Several specimens had slightly larger ratios, suggesting the currently accepted range may need to be expanded. Second, we tested the ability of Ca/Psurface to detect alteration using twenty Quaternary mammal teeth from Big Bone Lick, Kentucky. Ten specimens had Ca/Psurface between 1.3 and 2.3 and ten had larger ratios, suggesting alteration. Because most methods measure Ca/P in powder (Ca/Ppowder), we compared Ca/Psurface, Ca/Ppowder, and the enamel subsurface (Ca/Psubsurface). With two exceptions, Ca/Psubsurface and Ca/Ppowder were below 2.3, regardless of Ca/Psurface, suggesting that Ca/Ppowder and Ca/Psubsurface underestimate alteration. We next compared Ca/Psurface, CO3/PO4, and carbon (δ13C) and oxygen (δ18O) isotope values for the fossil teeth. Fourteen specimens were identified as altered or unaltered by both proxies, but six specimens only had one altered proxy. Specimens with both proxies altered had lower, less variable δ13C values than specimens with both proxies unaltered. Median δ18O values were similar between these groups. Individuals with altered Ca/Psurface but unaltered CO3/PO4 isotopically resembled specimens with both proxies altered. Conversely, specimens with unaltered Ca/Psurface and altered CO3/PO4 were similar to specimens with both proxies unaltered. Notably, all individuals with both proxies altered had relatively low δ13C values, including a horse and mammoth, which are normally considered grazers (and therefore should have higher δ13C values). These and other altered specimens may be isotopically compromised. Overall, our results suggest that Ca/Psurface is effective at detecting alteration non-destructively, quickly, and affordably, making it an attractive approach for analyzing unique specimens.
1. Introduction
Isotopic and elemental analyses of the mineral matrix of bones and teeth (called bioapatite) can elucidate invaluable details about the life histories of animals and their environments (Wopenka and Pasteris, 2005). However, these analytical approaches require that specimens are chemically unaltered and retain biogenic values that reflect an animal's behavior at the time the tissue mineralized. Determining if values are biogenic or altered is particularly a concern for archeological and paleontological specimens that have been buried for long periods of time (Trueman and Benton, 1997; Pfretzschner, 2004; Kremer et al., 2012). Diagenesis, which includes both deposition of exogenous minerals and recrystallization of bioapatite, can irreversibly modify the isotopic or elemental composition of a specimen (Trueman et al., 2008). It is thus important to be able to assess if specimens have been altered, ideally prior to destructively sampling them for isotopic or elemental analysis.
There are several widely used methods for evaluating specimen preservation. These include: the isotope data themselves (Sponheimer and Lee-Thorp, 1999; Schoeninger et al., 2003; Trueman et al., 2003); concentration of rare earth elements and halides (Pate et al., 1994); relative abundances of calcium and phosphorous (Ca/P; Zaichick and Tzaphlidou, 2002; Roche et al., 2010; Sotiropoulou et al., 2015), or carbonate and phosphate (CO3/PO4; Sponheimer and Lee-Thorp, 1999; Roche et al., 2010; Piga et al., 2011); and carbonate crystallinity (e.g., Sponheimer and Lee-Thorp, 1999; Surovell and Stiner, 2001; Dal Sasso et al., 2018; however, see Trueman et al., 2008). The degree to which these proxies relate to each other has not been validated. Unfortunately, most of these methods also tend to be destructive, expensive, and time consuming. However, non-destructive handheld X-ray fluorescence (hXRF) has the unique ability to measure the abundances of elements from magnesium to uranium in the surface of a specimen, and may be able to non-destructively assess bioapatite preservation (Byrnes and Bush, 2016).
We conducted a two-part study examining the potential of hXRF for non-destructively assessing preservation of bioapatite in bones and teeth. First, we scanned a variety of modern skeletal elements from humans and other vertebrate taxa to confirm that the Ca/P of a specimen's surface (Ca/Psurface) measured by hXRF is consistent with expectations based on stoichiometry and previously published Ca/P for modern human femoral heads obtained with other methods (1.3–2.3; Zaichick and Tzaphlidou, 2002). Second, we evaluated the efficacy of Ca/Psurface and CO3/PO4 at detecting diagenetic alteration. While hXRF has the unusual ability to measure a specimen's surface, Ca/P is typically measured from powder, and it is unknown how sample form might impact Ca/P measurements. We compared Ca/Psurface, Ca/P in powdered enamel (Ca/Ppowder), and Ca/P of the enamel subsurface (Ca/Psubsurface) exposed by removing powdered sample for a selection of Quaternary mammal cheek teeth. After confirming that Ca/Psurface is better equipped for detecting alteration than either Ca/Ppowder or Ca/Psubsurface, we analyzed CO3/PO4, and carbon (δ13C), and oxygen (δ18O) stable isotope values for the Quaternary specimens and explored the degree to which Ca/Psurface and CO3/PO4 align in their identification of altered specimens.
2. Background
2.1. Diagenetic alteration and methods of detection
Although its composition can vary slightly, bioapatite is generally composed of calcium, phosphate, and a hydroxy group [Ca10(PO4)6(OH)2; Pate et al., 1994]. While an organism is alive, the compounds and elements of bioapatite are readily substituted by others (such as carbonates, strontium, barium, and magnesium), reflecting nutritional intake and conditions experienced by the individual as its bones and teeth matured (Pate et al., 1994; Pfretzschner, 2004; Wopenka and Pasteris, 2005; Kremer et al., 2012). Elements and compounds in bioapatite are also easily exchanged after burial (Wopenka and Pasteris, 2005; Keenan, 2016). The extent of diagenetic alteration to bones and teeth depends heavily on the timing and environment of burial (Trueman and Benton, 1997; Trueman et al., 2003; Piga et al., 2011). Biological alteration, generally caused by bacteria, leads to the loss of organics. Removal of organics leaves space for potential replacement and infilling by exogenous phosphates, carbonates, and other minerals (Shemesh, 1990; Smith et al., 2002; Kremer et al., 2012). Diagenesis also results from abiotic processes, such as the dissolution of bioapatite under acidic conditions.
As noted above, there are several widely used methods for evaluating specimen preservation. The amount of rare earth elements (e.g., uranium), or halogens (e.g., fluorine; chlorine) in bioapatite has been frequently used as an indicator of alteration (Pate et al., 1994; Trueman, 1999; Smith et al., 2002). These elements, which substitute for other elements in bioapatite, are only incorporated in enamel and bones in relatively small concentrations during life from diet and drinking water. However, they can be considerably more concentrated in archeological and paleontological specimens due to the replacement of biogenic material and the infilling of voids after the loss of organics (Alonzi et al., 2019). In some cases, researchers compare the elemental composition of a specimen to the surrounding sediment or rock. If the composition of a specimen closely resembles that of its matrix (and diverges from values observed in modern bones or teeth), then the specimen is likely altered (Roche et al., 2010).
Carbonates and phosphates precipitate into gaps left by the loss of organics. They readily replace compounds in bioapatite both in life and post-mortem (Wopenka and Pasteris, 2005). This can change both the relative abundance of calcium and phosphorous (Ca/P) and CO3 and PO4. Ca/P in unaltered bioapatite is 2.2 based on stoichiometry (Sotiropoulou et al., 2015), but values ranging from 1.3 to 2.3 have been measured for modern human femoral heads (Zaichick and Tzaphlidou, 2002). Researchers typically assume that altered specimens exhibit Ca/P outside of this expected range (Pate et al., 1994; Berna et al., 2004; Zaichick and Zaichick, 2010). Researchers also generally assume that CO3/PO4 for unaltered bioapatite will be lower than 0.5 based on stoichiometry and observation. A lower bound is not well established (Piga et al., 2011; Dal Sasso et al., 2018). In theory, Ca/P and CO3/PO4 for a specimen should covary. However, this expected relationship breaks down if a carbonate other than CaCO3 is precipitated (e.g., MgCO3, FeCO3). Further, the addition of secondary phosphate minerals, which generally also contain calcium, would lower CO3/PO4 while potentially maintaining or even increasing Ca/P.
Crystallinity measures the size of carbonate crystals within a specimen. Biogenic carbonate crystals are relatively small in comparison to the more stable diagenetic carbonates and apatites (e.g., fluorapatite) that can replace them (Posner, 1969; Shemesh, 1990; Surovell and Stiner, 2001).
Lastly, sometimes researchers use the δ13C or δ18O values of the specimens themselves to gauge if diagenetic alteration has occurred. The isotopic composition may be altered to reflect post-burial conditions (Zazzo et al., 2004b; Liu et al., 2008; Alonzi et al., 2019). In particular, extremely homogenous δ13C and δ18O values, or specimens with abnormally high or low isotope values, may be cause for concern (e.g., Koch et al., 1997; Sharp et al., 2000; Zazzo et al., 2004a). Carbon isotopes can be altered by deposition and replacement of carbonates (Koch et al., 1997; Lee-Thorp and Sponheimer, 2003; Trueman et al., 2003), and partial oxygen isotopic exchange can occur between bioapatite and groundwater early in diagenesis (Sharp et al., 2000; Zazzo et al., 2004a,b). Both of these processes are expected to decrease isotopic variability among specimens. However, because alteration may vary among specimens at a locality, some specimens may remain isotopically unaltered while others from the same locality may have abnormally high or low isotope values (Chillón et al., 1994; Zazzo et al., 2004a,b). Researchers typically rely on expected biology (diet and habitat) to determine if specimens with unusual δ13C or δ18O values have been isotopically altered (Zazzo et al., 2004b). It is also possible to use the offset in δ18O values between the carbonate and phosphate portions of bioapatite to assess preservation. The oxygen isotope composition of carbonate and phosphate can be impacted by different processes, leaving one of these compounds altered and the other untouched (Zazzo et al., 2004b; Martin et al., 2008).
2.2. Typical Instrumentation
All of the methods for checking preservation outlined above have some drawbacks due to the amount of sample required, the amount of time and training needed to conduct analyses, instrument availability, or the associated cost. Moreover, the instrumentation used to measure these preservation proxies is generally destructive to some degree. Researchers sometimes assess if specimens retain biogenic isotopic values by looking at the variability of the isotope values themselves using methods such as inductively coupled plasma mass spectrometry (ICP-MS). The most common analytical methods for measuring elemental abundance, including rare earth elements, halogens, and Ca/P, are benchtop XRF (bXRF), X-ray diffraction (XRD), and scanning electron microscopy (SEM). These methods, like hXRF, displace electrons and measure the energy difference that results to identify elements. Neutron activation analysis and flame atomic absorption spectroscopy were used to established the accepted range for unaltered Ca/P (Zaichick and Tzaphlidou, 2002; Roche et al., 2010; Sotiropoulou et al., 2015), but these methods are infrequently used in archeology and paleontology. Fourier Transfer Infrared spectroscopy (FTIR) is typically used to characterize CO3/PO4. This measures the intensities of carbonate and phosphate peaks. It can also characterize crystallinity because crystal size has a direct relationship with the relative intensities of peaks and valleys observed in FTIR spectra (Piga et al., 2011; Dal Sasso et al., 2018). These commonly used methods generally require powdered, homogenized samples or a small fragment of a specimen. FTIR can be used on whole specimens, but powdering samples aligns the orientation of the bioapatite crystals and gives considerably more accurate readings. Lastly, isotope ratio mass spectrometry (IRMS), which measures δ13C and δ18O values, also typically requires homogenization, and ultimately dissolution, of a sample. Laser ablation may also be able to measure elemental and isotopic composition. It is less destructive than other methods, but does cause a small amount of damage, takes a lot of time and resources, and has trouble accurately measuring some values, including oxygen isotopes (Passey and Cerling, 2006). It also only provides data about a tiny portion of a specimen, which will only capture a limited time window, and cannot remove any diagenetic material (Lewis et al., 2014; Limbeck et al., 2015; Willmes et al., 2016; Rey et al., 2022). To avoid sampling specimens with altered bioapatite, most researchers preferentially analyze tooth enamel (Cerling and Sharp, 1996; Lee-Thorp and Sponheimer, 2003). Enamel is more crystalline and has far less organic material than bone (roughly 5 vs. 30%), making it more resistant to alteration (Lear et al., 2008).
Benchtop XRF requires ~0.5 grams of homogenized sample pressed into a pellet with a binding agent such as cellulose powder, which prevents analyzing the same powdered sample for isotope data. Handheld XRF (hXRF) instead detects the composition of a specimen's surface (rather than homogenized powder; Byrnes and Bush, 2016) by exciting the specimen surface with primary X-rays and measuring the energy of the secondary X-rays emitted in approximately a minute. While FTIR does not have to be destructive, powdering sample randomizes the orientation of what is being measured. Unfortunately, even though only enough powder is needed to cover the detector eye (<10 mg) this powder can be difficult to recover without concern about contaminating samples prior to isotope analyses. In contrast to FTIR, the orientation of a specimen is not expected to matter with hXRF, giving hXRF the potential to assess the preservation of whole specimens without damaging their structural integrity. If there is a reason to measure powdered sample, hXRF also requires far less material than bXRF, and that powder is relatively easy to recover for further analyses, as we discuss below. The ability of hXRF to measure the surface of whole specimens, combined with the small size and portability of hXRF spectrometers, makes this method an attractive option for analyzing museum specimens that are rare or unique and may be difficult to move. Handheld XRF spectrometers also require minimal training and analytical time, and are inexpensive to use after initial purchase.
However, the capacity for hXRF to assess diagenetic alteration of fossil bones and teeth has not been previously tested. This tool was originally developed and used for in situ detection of the presence of uranium and other minerals in mining operations and other industries, but has also been used to identify metals and minerals and expanded into other arenas, including soil and waste analysis, art conservation, and food safety studies (Bosco, 2013). It has also been used to assess the burial history of modern human remains by measuring the composition of matrix on bone surfaces (Byrnes and Bush, 2016) and evaluate diet by measuring elemental abundance of tooth enamel (Hamilton et al., 2019, 2022). We suspect that it will be similarly useful for assessing alteration of fossil specimens. If Ca/Psurface (measured with hXRF) and other widely used methods, such as CO3/PO4 and δ13C and δ18O values, are comparable in their diagnoses of altered specimens, then measuring Ca/Psurface could reduce the time and cost associated with evaluating preservation and help prevent unnecessary damage to specimens that are too altered to have biogenic isotopic data.
3. Methods
3.1. Specimen selection and data collection
3.1.1. Part 1: Establishing Ca/Psurface for unaltered, modern skeletal elements using hXRF
To confirm that Ca/Psurface measured with hXRF is within the range established for human femoral heads using other methods (Zaichick and Tzaphlidou, 2002; Sotiropoulou et al., 2015), 30 points were measured on each of eight left modern human femora (Figure 1). For each specimen, we scanned a flat surface that covered as much of the hXRF window as possible. We then scanned 25 elements from each of three modern human skeletons to evaluate variability in Ca/Psurface among elements within the same individual. We also measured Ca/Psurface for a variety of single elements from modern non-human vertebrates, that represent two monotreme orders (Didelphimorphia, N = 2; and Macropoda, N = 1), four placental mammal orders (Artiodactyla, N = 16; Primates, N = 29; Carnivora, N = 16; and Rodentia, N = 4), and two reptile orders (Crocodilia, N = 1; Testudines, N = 1). Teeth and associated mandibles from a subset of these specimens (N = 20 teeth from eight mandibles) were also compared to verify that enamel has similar Ca/Psurface to bone. If the hXRF spectrometer could not measure a specimen, the measured abundance of calcium or phosphorous were below the detection limit or were too small relative to the margins of error (reported in Supplementary Tables 1–3), or values were outside of the expected range for Ca/P (1.3–2.3), the specimen was rotated and rescanned up to three times to ensure that the data reflected the composition of the bone, and not human or instrument error. All specimens were selected from the comparative osteology and zooarcheology teaching collections at the University of Cincinnati's Department of Anthropology.
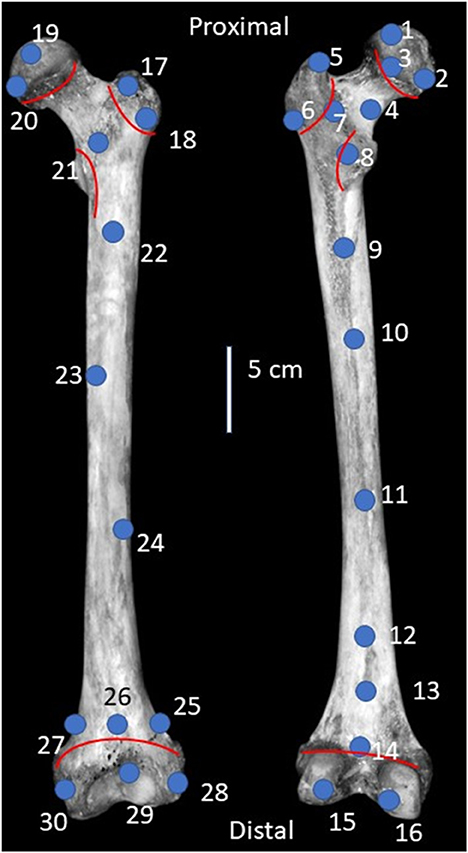
Figure 1. Diagram of a left modern human femur illustrating locations of scanned points on the anterior (left) and posterior (right) sides. Selected points are near recognizable landmarks for repeatability across femora, with an equal number of points on the anterior and posterior sides. In total, 30 points were measured: Ten on the proximal end, ten on the shaft, and ten on the distal end. Red lines indicate the locations of growth plates. Image adapted from Adams and Crabtree (2012).
3.1.2. Part 2: Evaluating relationships between Ca/P, CO3/PO4, and stable carbon and oxygen isotope values
We used hXRF to scan the surface of specimens housed at the Behringer-Crawford Museum in Covington, Kentucky, and selected 20 cheek teeth from large Quaternary herbivorous mammals from Big Bone Lick (BBL), Boone County, Kentucky (Bison, n = 12; Equus, n = 2; Mammuthus, n = 5; Mammut, n = 1) that had minimal consolidant or varnish coating. Ten of these specimens had Ca/Psurface between 1.3 and 2.3, and 10 had Ca/Psurface above 2.3. Because these specimens were collected between 1930 and 1950, the associated field notes are no longer available and stratigraphic context has been lost. However, based on published radiocarbon and optically stimulated luminescence dates for the deposits that yielded the specimens, the proboscidean and horse are likely 25,000 to 11,600 years old, while the bison teeth could date from that interval until 5,000 years ago or between 1,450 and the 1,800's CE (Tankersley et al., 2015).
Because hXRF can measure the elemental composition of a specimen's surface, and most methods for measuring Ca/P use powdered samples, we investigated potential differences in Ca/Psurface, Ca/P of enamel powder (Ca/Ppowder), and the enamel subsurface exposed by removal of powdered samples (Ca/Psubsurface). We first scanned a flat, non-occlusal enamel surface of each tooth before mechanically cleaning the enamel surface or removing powder. Using a handheld Dremel tool with a carbide dental bit, we then mechanically abraded a small area of the enamel surface (1 cm wide × 0.5 mm deep). After removing potentially contaminated surface enamel, we collected ~500 mg of homogenized, powdered enamel along a narrow (ca. 1 mm) strip between the crown and neck of each tooth. We scanned both the collected powder and the enamel subsurface exposed by sampling.
Next, we analyzed CO3/PO4 for the 20 cheek teeth and assigned each specimen to one of four alteration groups: (1) Altered Ca/Psurface and CO3/PO4; (2) Unaltered Ca/Psurface and altered CO3/PO4; (3) Altered Ca/Psurface and unaltered CO3/PO4; or (4) Unaltered Ca/Psurface and CO3/PO4. We then compared carbonate δ13C and δ18O values of chemically pretreated samples among these groups to look for possible similarities in average isotope values and isotopic variance among altered and unaltered specimens as defined by the two proxies. To chemically pretreat samples, we followed the standard method used in our lab (Crowley and Wheatley, 2014). Powdered samples were soaked in 0.5 mL of 30% H2O2 per 10 mg of sample for 24 hours at room temperature. Tube lids were left loose to release evolved gas, and samples were regularly agitated to ensure even reaction. Samples were rinsed five times with ultrapure water. They were then treated with 0.5 mL of 1M acetic acid buffered with calcium acetate (pH ~5) per 10 mg of sample at 4°C for 24 h with regular agitation. Samples were again rinsed five times with ultrapure water and freeze dried.
3.2. Instrumentation
We used a Bruker Tracer 5i hXRF spectrometer to measure Ca/Psurface, Ca/Psubsurface, and Ca/Ppowder in bones and teeth. The spectrometer was set to the mudrock duo calibration, and data were collected and viewed using the Bruker Instrument Tools program 1.7.0.128. For measuring Ca/Psurface, a flat surface of each specimen was scanned while in direct contact with the protective window over the detector of the hXRF spectrometer. When specimens were small enough to rest on the spectrometer's platform (4 inch-squared), the spectrometer was attached to a stand that directs the X-ray source and detector toward the ceiling. Specimens were placed directly on the platform over the detector window, and when possible, a lead cup was placed over them. If specimens were unable to be placed on top of the platform due to their large size or arrangement in a museum exhibit, they were set on a flat surface, and the hXRF spectrometer was removed from the stand and held by hand, directed downward.
Measuring Ca/Ppowder required some modifications to the setup described above (Figure 2). Powdered samples are generally measured with an hXRF spectrometer by placing them in plastic sample cups and scanning them through 4 μm-thick Prolene sample support windows. To reduce the amount of powdered enamel needed, we placed powdered sample between two Prolene sample support windows. This assemblage was then backed by a single, open-ended sample cup containing powdered cellulose (Figure 2). Having powdered cellulose behind the sample while scanning helped better cover the detector of the spectrometer, preventing the laser from scattering and allowing for more accurate measurements. The cellulose itself does not have heavy enough elements in high enough concentrations to be measured by hXRF, and thus did not interfere with measuring our samples. We found by iteratively measuring smaller amounts of an internal reference material (powdered bentonite) that spectrometer precision decreased for samples smaller than 250 mg. We therefore worked with 250 mg for powdered enamel samples.
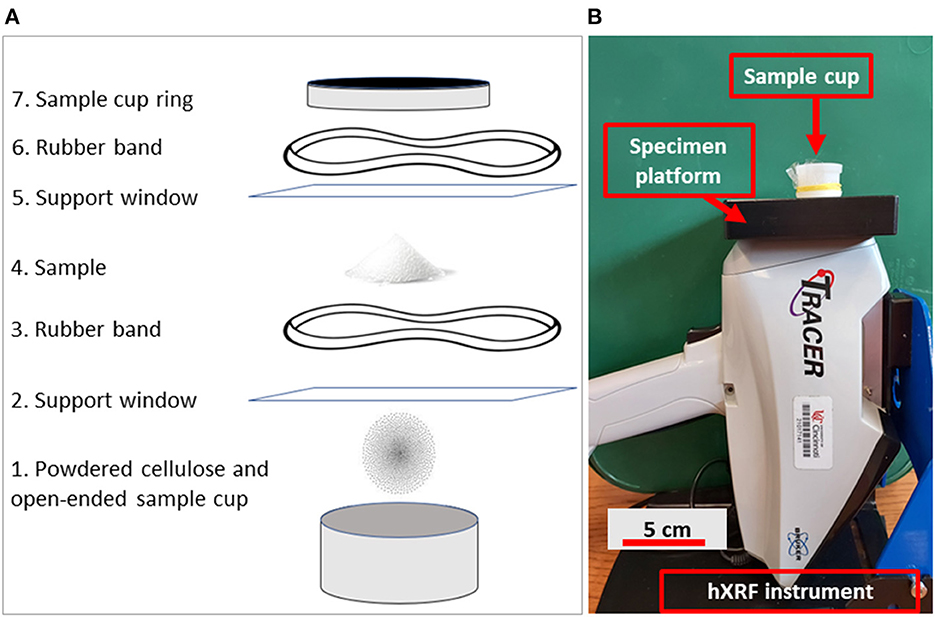
Figure 2. Diagram illustrating assembly of components for measuring powder with an hXRF instrument (A), and the final set up as it would appear after assembly (B). Numbered steps in (A) are as follows: (1) Powdered cellulose is placed in a single open-ended sample cup to provide a background to stop the laser from scattering and giving inaccurate results; (2) A 4 micron-thick Prolene® sample support window is placed over the powdered cellulose and cup to separate the powder from the sample; (3) The support window is secured to the cup with a rubber band to prevent loss of sample. The support window needs to be secure, but loose enough that the powder placed on it falls toward the center; (4) Powdered sample is placed on the sample support window; (5) A second support window is placed over the sample, which allows the sample to be recovered for future use; (6) A second rubber band is used to secure the second sample support window; and (7) Everything is secured with the sample cup's ring. The assembly is inverted and placed on the hXRF instrument (B). A lead cup (not pictured) would then be placed over the sample cup for safety.
We measured CO3/PO4 of untreated powder on a Nicolet™ iS™ 10 FTIR Spectrometer with OMNIC 9 software at the Chemistry Department at Northern Kentucky University. All values were measured relative to a baseline based on local minima for each peak, but additional spectra manipulation, such as peak deconvolution, was avoided (Supplementary Table 4). To characterize CO3/PO4, we divide the sum of the carbonate peaks at 1,400 and 1,500 nm−1 by the sum of the phosphate peaks at 1,000 and 604 nm−1. The 565 nm−1 phosphate peak is also frequently used to characterize CO3/PO4 (Dal Sasso et al., 2018); however, it was not considered in this analysis as the spectrometer was less accurate near and below 550 nm−1.
Lastly, we measured δ13C and δ18O values in pretreated enamel carbonate on a Finnigan-MAT 252 isotope ratio mass spectrometer (IRMS) equipped with a Thermo Scientific Kiel II carbonate device at the Light Stable Isotope Mass Spec Lab at the University of Florida. We report both δ13C and δ18O data relative to Vienna Pee Dee Belemnite (VPDB). Data were calibrated using the international standard NBS-19. Precision (based on 8 replicates of this standard), was 0.024‰ for carbon and 0.049‰ for oxygen. Accuracy was confirmed using NBS-18. Corrected values for δ13C (−5.01±0.02%) and δ18O (−23.08±0.02%) were very close to accepted values (−5.01% and −23.2%).
3.3. Data analysis
In Part 1, means and ranges for various groups were visually compared to the expected range for unaltered Ca/P. We used Wilcoxan matched pairs signed ranks test to compare Ca/Psurface for bone and associated teeth from individual mandibles.
In Part 2, we used Kruskal Wallis tests followed by Dunn Bonferonni post-hoc comparisons to compare Ca/Psurface, Ca/Psubsurface, and Ca/Ppowder for specimens identified as altered and unaltered based on Ca/Psurface, as well as δ13C and δ18O values among the four alteration groups (both altered Ca/Psurface and CO3/PO4, unaltered Ca/Psurface but altered CO3/PO4, altered Ca/Psurface but unaltered CO3/PO4, and both unaltered Ca/Psurface and CO3/PO4). We used linear regression to determine if a relationship exists between Ca/Psurface and CO3/PO4 and Levene's tests for homogeneity of variance to compare variances in δ13C and δ18O values among alteration groups. All statistical tests were run in SPSS 25 with significance set at a = 0.05.
4. Results
4.1. Part 1: Establishing Ca/P for modern human skeletal elements and modern taxa using hXRF
Most of the 30 points scanned on the modern human femora had Ca/Psurface within the expected range of 1.3–2.3 (Figure 3, Supplementary Table 1). Measurements taken using the Brucker Tracer 5i used in this study generally have a 3σ limit of < 1%, and reported values for calcium and phosphorous were always >20,000 ppm, with a margin of error below 800 ppm, in this study. However, several points had values ranging from 2.3 to 2.8, especially those that were near metaphyses. Among the other modern human skeletal elements scanned, one cranium and one talus had Ca/Psurface >2.3 (2.4 and 2.6, respectively). All of the non-human specimens (both bones and teeth) had Ca/Psurface between 1.3 and 2.3 (Figure 3, Supplementary Tables 2, 3). There were no differences in Ca/Psurface between mandibles and their associated teeth (N = 8 individuals, W = 326, DF = 15, p = 0.9; Supplementary Table 5).
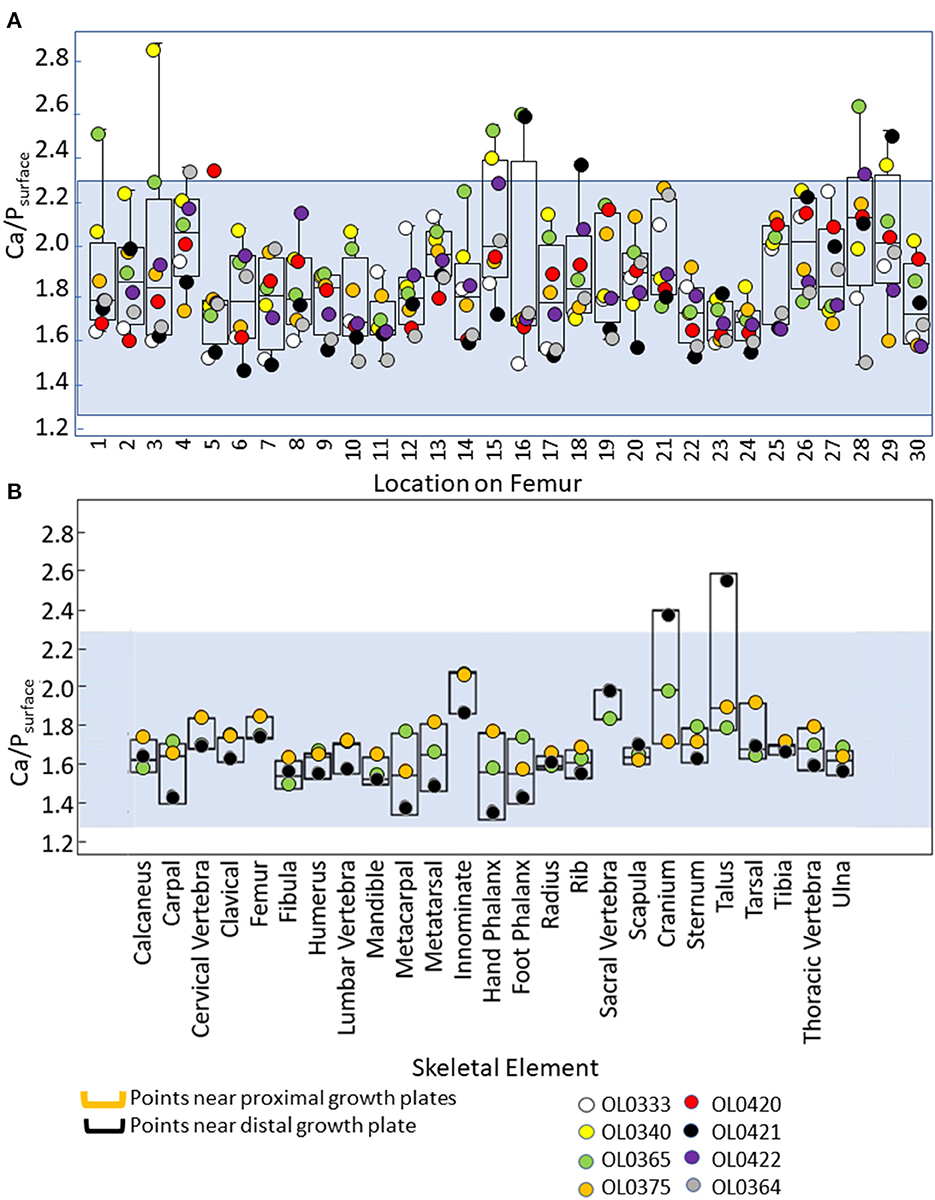
Figure 3. Box plots summarizing Ca/Psurface for various points along modern human femora (A), and modern human skeletal elements (B). The blue shading represents values within the expected range of unaltered Ca/P (1.3–2.3). Raw data are provided in Supplementary Tables 1, 2.
4.2. Part 2: Evaluating relationships between Ca/P, CO3/PO4, and stable carbon and oxygen isotope values
Ca/Psurface was higher than Ca/Psubsurface or Ca/Ppowder for both altered and unaltered specimens (altered Ca/Psurface: χ2 = 22.2, df = 2, p < 0.001; unaltered Ca/Psurface: χ2 = 8.0, df = 2, p = 0.02). With the exception of two specimens (2022-1950-57b and 2022-1950-23), which had Ca/Psubsurface values of 2.5 and 2.4, respectively, all specimens had Ca/Psubsurface and Ca/Ppowder below 2.3, regardless of the original Ca/Psurface value (Figure 4, Table 1).
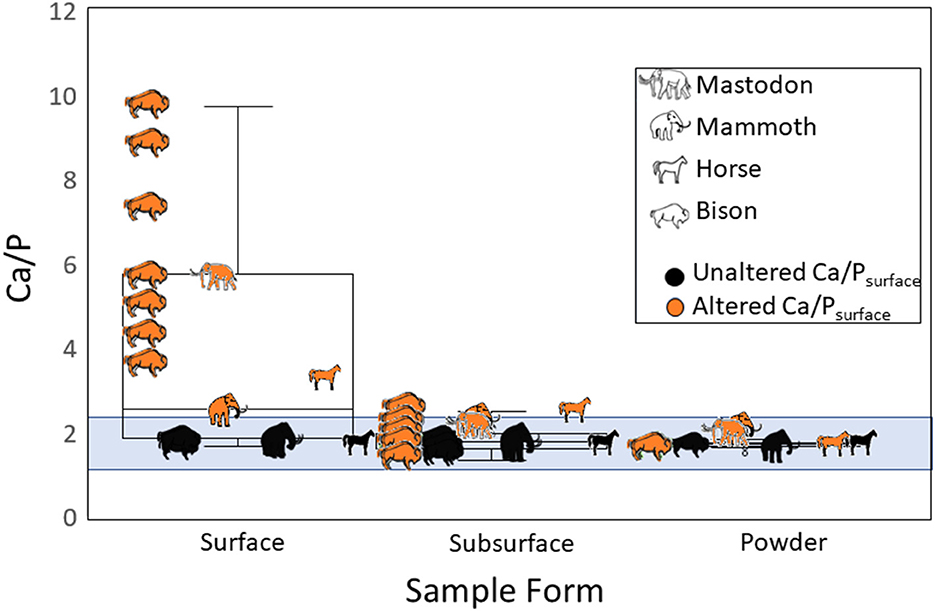
Figure 4. Ca/P values measured with hXRF for fossil herbivore cheek teeth from Big Bone Lick, Kentucky. Box plots compare surfaces of whole teeth (Ca/Psurface), the enamel subsurface after sampling (Ca/Psubsurface), and powdered enamel (Ca/Ppowder). The blue shading represents values within the expected range of unaltered Ca/P (1.3–2.3).
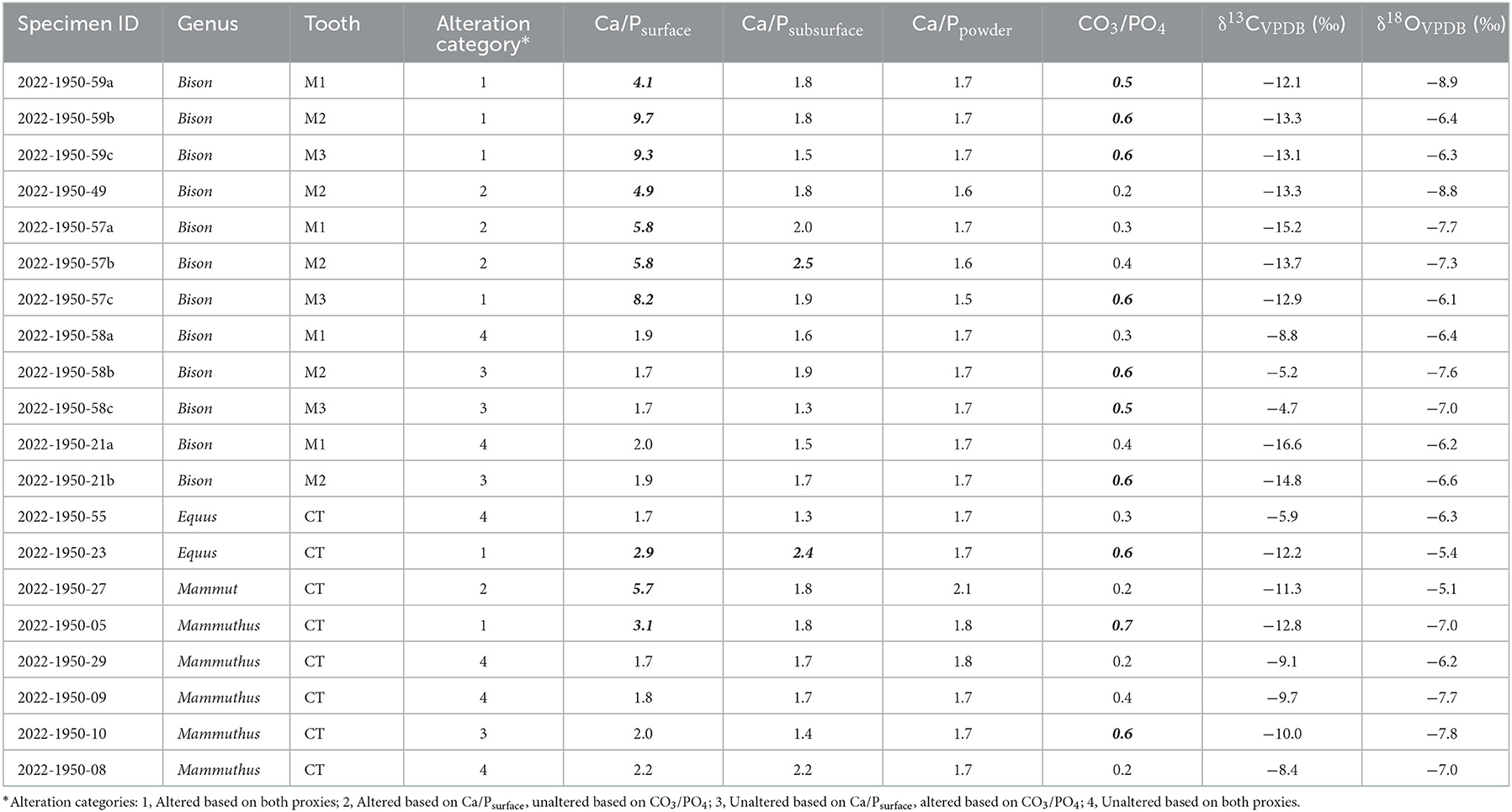
Table 1. Ca/Psurface, Ca/Psubsurface, Ca/Ppowder, CO3/PO4, and isotope data for fossil teeth from Big Bone Lick. Ca/Psurface and CO3/PO4 values that are considered altered (Ca/Psurface>2.3, CO3/PO4≥0.5) are bolded and italicized. Specimens that could not be identified as a specific premolar or molar are labeled as cheek teeth (CT).
The relationship between Ca/Psurface and CO3/PO4 was weak and insignificant (R2 = 0.1, p = 0.3; Figure 5). Of the twenty teeth scanned, eight were identified as altered and six as unaltered by both Ca/Psurface and CO3/PO4; four were identified as altered by CO3/PO4 despite being identified as unaltered by Ca/Psurface; and two were identified as altered by Ca/Psurface but unaltered by CO3/PO4 (Table 1, Figure 5). There were no significant differences in median δ13C or δ18O values among alteration groups (δ13C: χ2 = 5.7, df = 3, p = 0.1; δ18O: χ2 = 1.7, df = 3, p=0.6). There were also no differences in isotopic variance among these groups (Levene's test p > 0.05 for both isotopes). Nevertheless, there were apparent trends. Specimens identified as unaltered by both Ca/Psurface and CO3/PO4 had apparently higher, more variable δ13C values, and similar but apparently less variable δ18O values, than specimens identified as altered by both proxies. Specimens with altered Ca/Psurface but unaltered CO3/PO4 had a similar range in δ13C and δ18O values to specimens identified as altered by both proxies. Conversely, specimens identified as unaltered by Ca/Psurface but altered by CO3/PO4 had a similar range in δ13C and δ18O values to specimens that were identified as unaltered by both proxies (Figure 6).
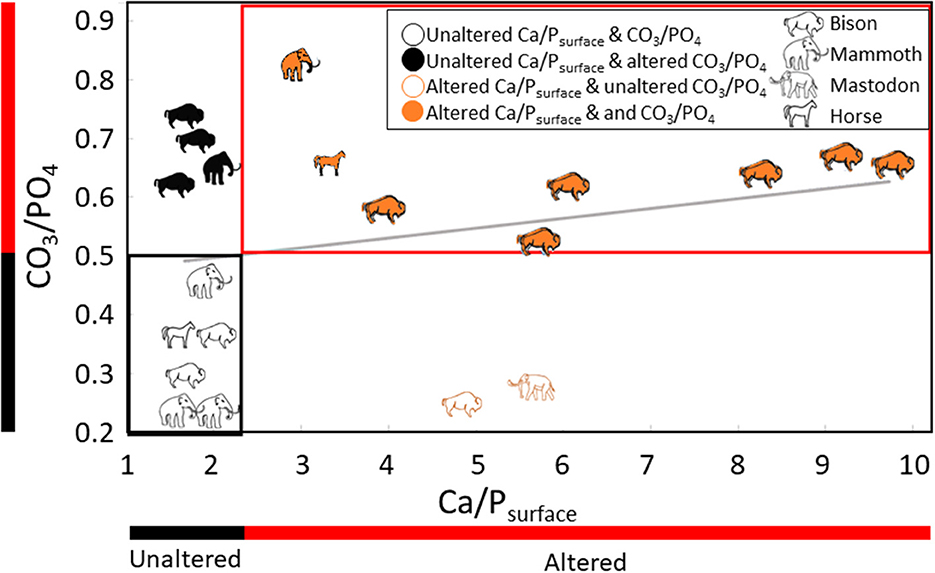
Figure 5. Bivariate scatter plot comparing Ca/Psurface and CO3/PO4 for fossil specimens from Big Bone Lick, Kentucky. Altered and unaltered ranges for each proxy are indicated by red and black bars, respectively. Specimens identified as altered by both proxies are outlined with a red box, and specimens identified as unaltered by both proxies are outlined with a black box. The diagonal gray line shows the apparent but insignificant relationship between Ca/Psurface and CO3/PO4 (R2 = 0.1, p = 0.3).
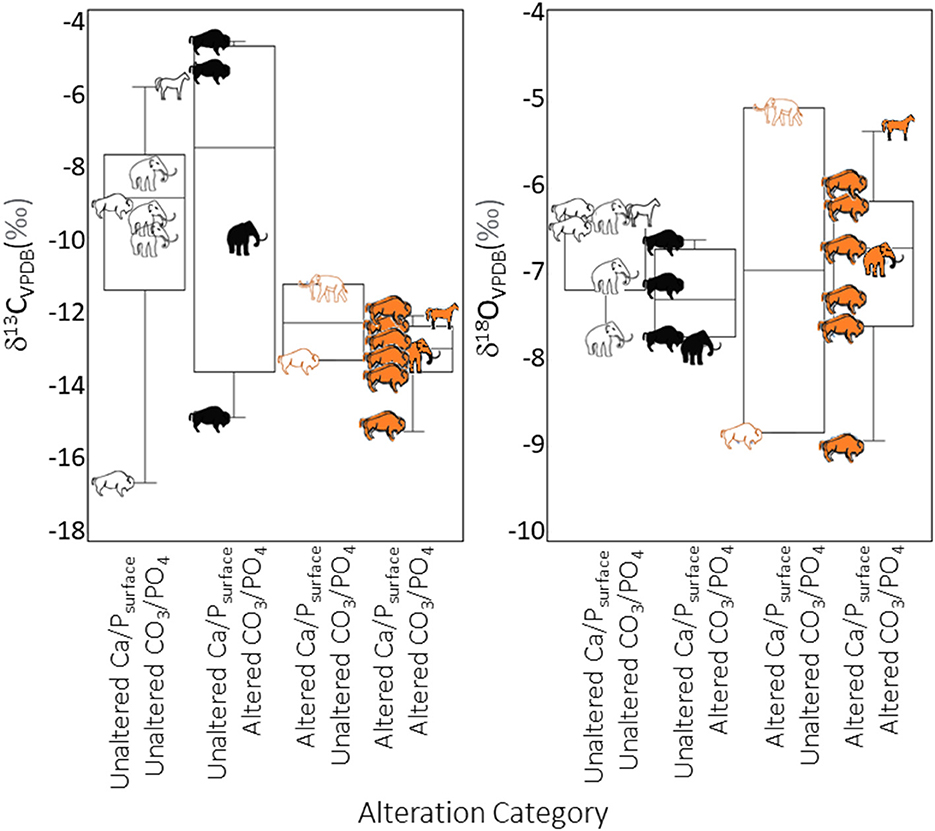
Figure 6. Box plots comparing δ13C and δ18O values for altered and unaltered pretreated powdered fossil enamel, with specimens defined as unaltered based on Ca/Psurface (1.3–2.3) or CO3/PO4 <0.5. Symbols for alteration categories and taxa are the same as in Figure 5.
To avoid the influence of taxon, we also compared isotope values among alteration groups for just bison. Using this smaller dataset (N = 12), isotope values among alteration groups remained statistically indistinguishable (δ13C: χ2 = 1.5, df = 3, p = 0.7; δ18O: χ2 = 3.6, df = 3, p = 0.3; Levene's test p > 0.05 for both isotopes). Apparent patterns in δ13C and δ18O values among the alteration groups were also somewhat similar to the whole dataset. Specimens identified as unaltered by both Ca/Psurface and CO3/PO4 had apparently more variable δ13C and less variable δ18O values than specimens identified as altered by both proxies (Figure 6). Specimens with unaltered Ca/Psurface and altered CO3/PO4 had similar trends in δ13C and δ18O values to specimens identified as unaltered by both proxies, but the range in δ13C values was higher. The single bison with altered Ca/Psurface but unaltered CO3/PO4 had a similar δ13C value to most of the specimens identified as altered by both proxies and a low δ18O value (comparable to one of the bison identified as altered by both proxies).
5. Discussion
We set out to test the capacity of hXRF to non-destructively measure Ca/Psurface of bones and teeth. Collectively, our results help substantiate the use of Ca/Psurface as an effective means of evaluating specimen preservation. Ca/Psurface appears to be at least as effective as CO3/PO4, and considerably more sensitive than Ca/Ppowder at identifying altered specimens. However, there are several caveats and interesting exceptions. Below we discuss some of our unexpected findings and note where additional work is warranted.
Based on previous work, we had anticipated that modern bones and teeth would exhibit Ca/Psurface between 1.3 and 2.3, and that this would be true regardless of element or taxon. Overall, Ca/Psurface for modern specimens was within this expected range. These results, though preliminary, help support that the commonly accepted (but untested) range for Ca/P is applicable beyond human femoral heads, and validate the use of hXRF for non-destructively evaluating Ca/Psurface. Several human elements had Ca/Psurface between 2.3 and 2.8. Most of these elevated measurements were for points near metaphyses in the human femora (Figure 2). These elevated readings may reflect bone that was still in the process of mineralizing, which would affect the chemical composition (Pate et al., 1994; Zaichick and Tzaphlidou, 2002; Zaichick and Zaichick, 2010). However, immature bioapatite and bone subjected to nutritional stress generally have lower calcium concentrations than mature bone, which would be expected to reduce Ca/P. Moreover, most of the individuals we analyzed were mature adults; the only sub-adult individual included (OL0364) had Ca/Psurface values consistently between 1.3 and 2.3. Instead, discrepancies in measurements near the metaphyses may result from these being regions having thinner, more porous bone (Byrnes and Bush, 2016). We caution other researchers to be aware of possible issues with measuring Ca/Psurface near metaphyses but suggest that a more likely explanation for elevated Ca/Psurface is that the currently accepted range for unaltered bioapatite needs to be updated. Given that the stoichiometry of bioapatite would require a Ca/P of 2.2, it is conceivable that the currently accepted upper boundary for unaltered Ca/P should be increased (possibly as high as 2.8).
We did not observe any Ca/Psurface values below 1.3 in either our modern or fossil datasets. To our knowledge, just one previous study reported values below 1.3 (Berna et al., 2004), and these authors experimentally evaluated the solubility of modern and fossil bone. We suspect that the conditions necessary to yield low Ca/P (e.g., acidic or anoxic) are rarer than those that result in elevated Ca/P, and more importantly, are less likely to preserve fossils.
We were surprised to find that nearly all of the fossil teeth we scanned had Ca/Psubsurface and Ca/Ppowder within the expected range for unaltered bioapatite (1.3–2.3), even when Ca/Psurface was much larger than 2.3 (Figure 4, Table 1). The two specimens with slightly elevated Ca/Psubsurface (2022-1950-57b and 2022-1950-23) were identified as altered based on their Ca/Psurface, but did not have particularly elevated values (Table 1). They also did not have unusual CO3/PO4 or isotopic values compared to other altered specimens (Table 1). It is possible that small cracks or microfractures in the enamel allowed alteration deeper in the enamel than for other specimens.
Because low Ca/Ppowder and Ca/Psubsurface were observed for specimens with high Ca/Psurface, it would be easy to conclude that the subsurface of specimens with altered Ca/Psurface were pristine and that removing the outer enamel surface before sampling a specimen is sufficient to avoid analyzing altered enamel. For example, elevated Ca/Psurface may be the result of calcium-rich minerals precipitating closer to the outside of the specimens, or the preferential dissolution of phosphates closer to the enamel surface (Berna et al., 2004). It would be interesting to be able to check Ca/P for the powder that was removed when we mechanically cleaned the surface of specimens, but unfortunately, there was not enough material to measure with our current methods.
However, isotope values and CO3/PO4 (which were both measured from powder) suggest that the interiors of teeth with altered Ca/Psurface were also compromised. There were clear apparent isotopic differences between specimens identified as altered and unaltered by Ca/Psurface, and most of the specimens identified as altered by Ca/Psurface also had elevated CO3/PO4. These results suggest that removing powder from a specimen compromises our ability to use Ca/P as a proxy for diagenesis, which may, in part, account for a lack of reported Ca/Ppowder values above 2.3 in the literature. We recommend scanning specimens before removing powdered sample or abrading the surface.
Twelve of the fossil cheek teeth from BBL were identified as altered or unaltered by both Ca/Psurface and CO3/PO4. However, we did not find a significant relationship between these two proxies. Roche et al. (2010) also found no relationship between Ca/Ppowder and CO3/PO4, although they were working with a powder, and the Ca/P values they reported were all between 1.3 and 2.3. As we have noted above, powdered enamel may yield Ca/P between 1.3 and 2.3 regardless of Ca/Psurface or CO3/PO4. The fossils we worked with were deposited in a salt lick with periodic fluvial input during the late Pleistocene and Holocene. All of them were exposed to CaCl2-rich water from springs and subsurface brine derived from the Middle Ordovician St. Peters Sandstone that sits below the Quaternary deposits at Big Bone Lick (McCartney et al., 2005; Tankersley et al., 2015). The lack of correlation between Ca/Psurface and CO3/PO4 may be explained by the precipitation of phosphate minerals (e.g., Ca5(PO4)Cl3), which would decrease CO3/PO4 while also increasing Ca/Psurface. There could also be an influence of non-calcium-bearing carbonates (e.g., BaCO3), which would only affect CO3/PO4 (Panno et al., 2022). However, given the prevalence of Ca2+ ions in the burial environment, this possibility seems less likely.
The degree to which altered Ca/P and CO3/PO4 relate to isotopic alteration is poorly understood. Chemical pretreatment is expected to remove exogenous carbonates (Verbruggen et al., 2010; Grimes and Pellegrini, 2013). However, it can only remove exogenous minerals that adsorbed onto crystal surfaces or infilled voids (Grimes and Pellegrini, 2013). If the chemical composition of bioapatite itself has been altered (e.g., via exchange or replacement of ions), then it is not possible to remove diagenetic material. Our results suggest that the interiors of specimens identified as altered with Ca/Psurface have been isotopically altered, even though their Ca/Ppowder and Ca/Psubsurface values are low (and in some cases even though they have low CO3/PO4). It is possible that Ca/Psurface and isotope values are affected earlier than other proxies during diagenesis, with isotopic exchange between the original bioapatite and burial environment also occurring before Ca/P or CO3/PO4 of the tooth interior are impacted. We had expected that altered specimens would have either less variable δ13C and δ18O values than unaltered specimens, or perhaps unusually high or low values that contradict expected foraging ecology. Specimens identified as unaltered by both proxies had a larger range in δ13C values (Figure 6), spanning expected values for both C4 and C3 consumers (>-2‰, and <-8‰, respectively; Cerling and Harris, 1999). However, all of the individuals identified as altered by both proxies had relatively low δ13C values (Figure 6). This included a horse and mammoth, which are normally considered grazers (and therefore would be expected to have higher δ13C values; Koch et al., 1998). Bison are often considered grazers. However, modern woodland bison (Bison bison athabascae) consume a mix of grass, C3 herbs, and woody vegetation (Blackburn et al., 2021). Tooth wear and previous isotopic analyses of BBL bison have also suggested that individuals from this locality had mixed diets of C3 and C4 foods (Widga, 2006; Tankersley et al., 2015). Thus, low δ13C values for some of the individuals we analyzed do not conflict with the expected foraging ecology for bison.
In contrast, the δ18O values for specimens identified as unaltered by both proxies were apparently more homogenous than specimens identified as altered by both proxies (Figure 6). The range in δ18O values for specimens with altered Ca/Psurface (ca. 4‰) was similar to has previously been reported for modern and recent co-occurring taxa within a community (Higgins and MacFadden, 2004; Crowley et al., 2008). Specimens with unaltered Ca/Psurface had a narrower range of δ18O (2‰). Stratigraphic information is unknown for these specimens. It is possible that only some of them have altered δ18O (i.e., the horse with an elevated δ18O value, 2022-1950-23, and the bison with low δ18O compared to other individuals, 2022-1950-59a), both of which had altered Ca/Psurface and CO3/PO4; Table 1). It is also possible that diagenetic δ18O was similar to biogenic δ18O in this particular burial environment, or that none of the specimens have altered δ18O, and that the apparent differences among groups reflect our limited sample size and shifts in meteoric δ18O over time (Baumann and Crowley, 2015). Further work in other taphonomic environments is needed. It would also be fruitful to evaluate if Ca/Psurface reflects alteration of δ18O values in hydroxyapatite phosphate. Future work assessing Ca/Psurface should include both hydroxyapatite carbonate and phosphate δ18O values to test this possibility.
In conclusion, we suggest that Ca/Psurface is considerably more effective than Ca/Ppowder, and at least as indicative as CO3/PO4 at identifying specimens with altered carbon or oxygen isotope data. In addition to its low cost, hXRF has the unique benefit of being able to measure Ca/Psurface quickly and non-destructively, possibly without even removing a specimen from a collection or display. Being able to non-destructively exclude specimens that are potentially too altered to be worth sampling would be invaluable to both researchers and institutions.
We emphasize the importance of following best recommended practices for using hXRF, including covering the X-ray beam as much as possible with a specimen for the most accurate measurement. Bones and teeth are not always ideal, simple shapes. Specimens that are too small, or curved in a way that prevents them from entirely covering the detector window, can scatter the x-ray beam and are less likely to be measured reliably. We stress that finding a flat surface on a specimen is important for being able to safely and accurately measure Ca/Psurface; if this is not possible the remaining portion of the spectrometer's detector needs to be covered with something that will block the x-ray beam and has a composition that will not interfere with sample measurement (e.g., cellulose powder).
While measuring Ca/Psurface with hXRF holds promise as a low-cost, non-destructive method for detecting alteration, we acknowledge that we only analyzed a limited number of fossil specimens from one locality and one taphonomic environment. More work is needed to test the efficacy of Ca/Psurface as an indicator of alteration in other depositional environments. We also suspect that the higher end of the range of Ca/Psurface needs to be expanded to include values >2.3. However, better constraining variability in unaltered Ca/Psurface will require additional work and is beyond the scope of this study. Nevertheless, our results demonstrate the potential of the method, and we recommend the inclusion of hXRF data in the process of specimen selection.
Data availability statement
The original contributions presented in the study are included in the article/Supplementary material, further inquiries can be directed to the corresponding author.
Ethics statement
Ethical approval was not required for the study involving human remains in accordance with the local legislation and institutional requirements. All specimens were selected from the comparative osteology and zooarcheology teaching collections at the University of Cincinnati's Department of Anthropology.
Author contributions
ES conceived the project and collected and analyzed the data. ES and BC wrote the manuscript. DS developed the analytical method and provided editorial advice. All authors contributed to the article and approved the submitted version.
Funding
This project was funded by a Geological Society of America Graduate Student Research Grant (to ES).
Acknowledgments
We thank Jim Wilkinson, Lili Ma, and Kristen Mason, for access and training on the FTIR instrument at Northern Kentucky University, Andy Czaja for help interpreting FTIR data, and Jason Curtis for analyzing carbon and oxygen isotopes. We also thank Jason French at the Behringer Crawford Museum for allowing us to scan and destructively sample specimens from Big Bone Lick. Finally, we thank the reviewers for their feedback prior to publication.
Conflict of interest
The authors declare that the research was conducted in the absence of any commercial or financial relationships that could be construed as a potential conflict of interest.
Publisher's note
All claims expressed in this article are solely those of the authors and do not necessarily represent those of their affiliated organizations, or those of the publisher, the editors and the reviewers. Any product that may be evaluated in this article, or claim that may be made by its manufacturer, is not guaranteed or endorsed by the publisher.
Supplementary material
The Supplementary Material for this article can be found online at: https://www.frontiersin.org/articles/10.3389/fearc.2022.1098403/full#supplementary-material
References
Adams, B., Crabtree, P. (2012). “Femur- An Overview,” in Comparative Osteology, eds B. Adams and P. Crabtree (Academic Press), 73–74.
Alonzi, E., Daly, N., Gordon, G., Scott, R. E., Knudson, K. J. (2019). Traveling monastic paths: mobility and religion at medieval Irish monasteries. J. Anthropol. Archaeol. 55, 101077. doi: 10.1016/j.jaa.2019.101077
Baumann, E. J., Crowley, B. E. (2015). Stable isotopes reveal ecological differences amongst now-extinct proboscideans from the Cincinnati region, USA. Boreas 44, 240–254. doi: 10.1111/bor.12091
Berna, F., Matthews, A., Weiner, S. (2004). Solubilities of bone mineral from archaeological sites : the recrystallization window. J. Archaeol. Sci. 31, 867–882. doi: 10.1016/j.jas.2003.12.003
Blackburn, R. C., Barber, N. A., Jones, H. P. (2021). Reintroduced bison diet changes throughout the season in restored prairie. Restor. Ecol. 29, 1–12. doi: 10.1111/rec.13161
Bosco, G. L. (2013). Development and application of portable, hand-held X-ray fluorescence spectrometers. Trends Anal. Chem. 45, 121–134. doi: 10.1016/j.trac.2013.01.006
Byrnes, J. F., Bush, P. J. (2016). Practical considerations in trace element analysis of bone by portable X-ray fluorescence. J. Forensic Sci. 61, 1041–1045. doi: 10.1111/1556-4029.13103
Cerling, T. E., Harris, J. M. (1999). Carbon isotope fractionation between diet and bioapatite in ungulate mammals and implications for ecological and paleoecological studies. Oecologia 120, 347–363. doi: 10.1007/s004420050868
Cerling, T. E., Sharp, Z. D. (1996). Stable carbon and oxygen isotope analysis of fossil tooth enamel using laser ablation, Palaeogeogr. Palaeoclimatol. Palaeoecol. 126, 173–186. doi: 10.1016/S0031-0182(96)00078-8
Chillón, B. S., Alberdi, M. T., Leone, G., Bonadonna, F. P., Stenni, B., Longinelli, A., et al. (1994). Oxygen isotopic composition of fossil equid tooth and bone phosphate: an archive of difficult interpretation. Palaeogeogr. Palaeoclimatol. Palaeoecol. 107, 317–328. doi: 10.1016/0031-0182(94)90103-1
Crowley, B. E., Koch, P. L., Davis, E. B. (2008). Stable isotope constraints on the elevation history of the Sierra Nevada Mountains. California. Bull. Geol. Soc. Am. 120, 588–598. doi: 10.1130/B26254.1
Crowley, B. E., Wheatley, P. V. (2014). To bleach or not to bleach? Comparing treatment methods for isolating biogenic carbonate. Chem. Geol. 381, 234–242. doi: 10.1016/j.chemgeo.2014.05.006
Dal Sasso, G., Asscher, Y., Angelini, I., Nodari, L., Artioli, G. (2018). A universal curve of apatite crystallinity for the assessment of bone integrity and preservation. Sci. Rep. 8, 1–13. doi: 10.1038/s41598-018-30642-z
Grimes, V., Pellegrini, M. (2013). A comparison of pretreatment methods for the analysis of phosphate oxygen isotope ratios in bioapatite. Rapid Commun. Mass Spectrom. 27, 375–390. doi: 10.1002/rcm.6463
Hamilton, M. I., Drake, B. L., Galloway, A., Dzhinenko, E., Nelson, S. V. (2022). How inter-species variation in strontium-calcium ratios of leaves and fruit informs dietary reconstructions. Am. J. Biol. Anthropol. 177 (S73), 78–79.
Hamilton, M. I., Drake, B. L., Nelson, S. V. (2019). “Differentiating between frugivory and folivory in primates using non-destructive XRF measurements of strontium/calcium ratios: A case study from Kibale National Park,” in Program of the 88th Annual Meeting of the American Association of Physical Anthropologists.
Higgins, P., MacFadden, B. J. (2004). “Amount effect” recorded in oxygen isotopes of Late Glacial horse (Equus) and bison (Bison) teeth from the Sonoran and Chihuahuan deserts, southwestern United States. Palaeogeogr. Palaeoclimatol. Palaeoecol. 206, 337–353. doi: 10.1016/j.palaeo.2004.01.011
Keenan, S. W. (2016). From bone to fossil: a review of the diagenesis of bioapatite. Am. Mineral. 101, 1943–1951. doi: 10.2138/am-2016-5737
Koch, P. L., Hoppe, K. A., Webb, S. D. (1998). The isotopic ecology of late Pleistocene mammals in North America Part 1. Florida. Chem. Geol. 152, 119–138. doi: 10.1016/S0009-2541(98)00101-6
Koch, P. L., Tuross, N., Fogel, M. L. (1997). The effects of sample treatment and diagenesis on the isotopic integrity of carbonate in biogenic hydroxylapatite. J. Archaeol. Sci. 24, 417–429. doi: 10.1006/jasc.1996.0126
Kremer, B., Owocki, K., Królikowska, A., Wrzosek, B., Kazmierczak, J. (2012). Mineral microbial structures in a bone of the Late Cretaceous dinosaur Saurolophus angustirostris from the Gobi Desert, Mongolia—A Raman spectroscopy study. Palaeogeogr. Palaeoclimatol. Palaeoecol. 358–360, 51–61. doi: 10.1016/j.palaeo.2012.07.020
Lear, C. H., Bailey, T. R., Pearson, P. N., Coxall, H. K., Rosenthal, Y. (2008). Cooling and ice growth across the Eocene–Oligocene transition. Geology. 251–254. doi: 10.1130/G24584A.1
Lee-Thorp, J., Sponheimer, M. (2003). Three case studies used to reassess the reliability of fossil bone and enamel isotope signals for paleodietary studies. J. Anthr. 22, 208–216. doi: 10.1016/S0278-4165(03)00035-7
Lewis, J., Coath, C. D., Pike, A. W. G. (2014). An improved protocol for 87Sr/86Sr by laser ablation multi-collector inductively coupled plasma mass spectrometry using oxide reduction and a customised plasma interface. Chem. Geol. 173–181. doi: 10.1016/j.chemgeo.2014.10.021
Limbeck, A., Galler, P., Bonta, M., Bauer, G., Nischkauer, W., Vanhaecke, F., et al. (2015). Recent advances in quantitative LA-ICP-MS analysis: challenges and solutions in the life sciences and environmental chemistry. Anal. Bioanal. Chem. 407, 6593–6617. doi: 10.1007/s00216-015-8858-0
Liu, A. G. S. C., Seiffert, E. R., Simons, E. L. (2008). Stable isotope evidence for an amphibious phase in early proboscidean evolution. Proc. Natl. Acad. Sci. USA. 105, 5786–5791. doi: 10.1073/pnas.0800884105
Martin, C., Bentaleb, I., Kaandorp, R., Iacumin, P., Chatri, K. (2008). Intra-tooth study of modern rhinoceros enamel δ18O: Is the difference between phosphate and carbonate δ18O a sound diagenetic test?. Palaeogeogr. Palaeoclimatol. Palaeoecol. 266, 183–189. doi: 10.1016/j.palaeo.2008.03.039
McCartney, D. M., Finney, M. A., Maynard, J. B. (2005). Sources of the Salt in the Big Bone Lick Springs, Northern Kentucky Geological Society of America Abstracts with Programs, Vol. 37. p. 1–34.
Panno, S. V., Kelly, W. R., Askari, Z., Hackley, K. C., Krothe, J. (2022). Stratigraphic and structural controls on the occurrence of saline springs within the Illinois Basin, U.S. J. Hydrol. 610, 127823. doi: 10.1016/j.jhydrol.2022.127823
Passey, B. H., Cerling, T. E. (2006). In situ stable isotope analysis (δ13C, δ18O) of very small teeth using laser ablation GC/IRMS. Chem. Geol. 235, 238–249. doi: 10.1016/j.chemgeo.2006.07.002
Pate, F. D., Journal, S., Jun, N., Donald, F. (1994). Bone chemistry and paleodiet. J. Archaeol. Method Theory 1, 161–209. doi: 10.1007/BF02231415
Pfretzschner, H. U. (2004). Fossilization of Haversian bone in aquatic environments. Comptes Rendus Pale 3, 605–616. doi: 10.1016/j.crpv.2004.07.006
Piga, G., Santos-Cubedo, A., Brunetti, A., Piccinini, M., Malgosa, A., Napolitano, E., et al. (2011). A multi-technique approach by XRD, XRF, FT-IR to characterize the diagenesis of dinosaur bones from Spain. Palaeogeogr. Palaeoclimatol. Palaeoecol. 310, 92–107. doi: 10.1016/j.palaeo.2011.05.018
Posner, A. S. (1969). Crystal chemistry of bone mineral. Physiol. Rev. 49, 760–792. doi: 10.1152/physrev.1969.49.4.760
Rey, L., Tacail, T., Santos, F., Rottier, S., Goude, G., Balter, V., et al. (2022). Disentangling diagenetic and biogenic trace elements and Sr radiogenic isotopes in fossil dental enamel using laser ablation analysis. Chem. Geol. 587, 120608. doi: 10.1016/j.chemgeo.2021.120608
Roche, D., Ségalen, L., Balan, E., Delattre, S. (2010). Preservation assessment of Miocene-Pliocene tooth enamel from Tugen Hills (Kenyan Rift Valley) through FTIR, chemical and stable-isotope analyses. J. Archaeol. Sci. 37, 1690–1699. doi: 10.1016/j.jas.2010.01.029
Schoeninger, M. J., Hallin, K., Reeser, H., Valley, J. W., Fournelle, J. (2003). Isotopic alteration of mammalian tooth enamel. Int. J. Osteoarchaeol. 13, 11–19. doi: 10.1002/oa.653
Sharp, Z. D., Atudorei, V., Furrer, H. (2000). The effect of diagenesis on oxygen isotope ratios of biogenic phosphates. Am. J. Sci. 300, 222–237. doi: 10.2475/ajs.300.3.222
Shemesh, A. (1990). Crystallinity and diagenesis of sedimentary apaties. Geochim. Cosmochim. Acta. 54, 2433–2438. doi: 10.1016/0016-7037(90)90230-I
Smith, C. I., Nielsen-Marsh, C. M., Jans, M. M. E., Arthur, P., Nord, A. G., Collins, M. J., et al. (2002). The strange case of Apigliano: early “fossilization” of medieval bone in southern Italy. Archaeometry 44, 405–415. doi: 10.1111/1475-4754.t01-1-00073
Sotiropoulou, P., Fountos, G., Martini, N., Koukou, V., Michail, C., Kandarakis, I., et al. (2015). Bone calcium/phosphorus ratio determination using dual energy X-ray method. Phys. Medica. 31, 307–313. doi: 10.1016/j.ejmp.2015.01.019
Sponheimer, M., Lee-Thorp, J. (1999). Alteration of enamel carbonate environments during fossilization. J. Archaeol. Sci. 26, 143–150. doi: 10.1006/jasc.1998.0293
Surovell, T. A., Stiner, M. C. (2001). Standardizing infra-red measures of bone mineral crystallinity: an experimental approach. J. Archaeol. Sci. 28, 633–642. doi: 10.1006/jasc.2000.0633
Tankersley, K. B., Murari, M. K., Crowley, B. E., Owen, L. A., Storrs, G. W., Mortensen, L., et al. (2015). Quaternary chronostratigraphy and stable isotope paleoecology of Big Bone Lick, Kentucky, USA. Quat. Res. 83, 479–487. doi: 10.1016/j.yqres.2015.01.009
Trueman, C. N. (1999). Rare earth element geochemistry and taphonomy of terrestrial vertebrate assemblages. Palaios 14, 555. doi: 10.2307/3515313
Trueman, C. N., Benton, M. J. (1997). A geochemical method to trace the taphonomic history of reworked bones in sedimentary settings. Geology 25, 263–266.
Trueman, C. N., Chenery, C., Eberth, D. A., Spiro, B. (2003). Diagenetic effects on the oxygen isotope composition of bones of dinosaurs and other vertebrates recovered from terrestrial and marine sediments. J. Geol. Soc. London. 160, 895–901. doi: 10.1144/0016-764903-019
Trueman, C. N., Privat, K., Field, J. (2008). Why do crystallinity values fail to predict the extent of diagenetic alteration of bone mineral?. Palaeogeogr. Palaeoclimatol. Palaeoecol. 266, 160–167. doi: 10.1016/j.palaeo.2008.03.038
Verbruggen, F., Heiri, O., Reichart, G. J., Leeuw, d. e., Nierop, J. W. K.G.J., Lotter, A.F. (2010). Effects of chemical pretreatments on δ18O measurements, chemical composition, and morphology of chironomid head capsules. J. Paleolimnol. 43, 857–872. doi: 10.1007/s10933-009-9374-z
Widga, C. (2006). Niche variability in late Holocene bison: a perspective from Big Bone Lick, KY. J. Archaeol. Sci. 33, 1237–1255. doi: 10.1016/j.jas.2005.12.011
Willmes, M., Kinsley, L., Moncel, M. H., Armstrong, R. A., Aubert, M., Eggins, S., et al. (2016). Improvement of laser ablation in situ micro-analysis to identify diagenetic alteration and measure strontium isotope ratios in fossil human teeth. J. Archaeol. Sci. 70, 102–116. doi: 10.1016/j.jas.2016.04.017
Wopenka, B., Pasteris, J. D. (2005). A mineralogical perspective on the apatite in bone. Mater. Sci. Eng. C. 25, 131–143. doi: 10.1016/j.msec.2005.01.008
Zaichick, S., Zaichick, V. (2010). The effect of age and gender on 38 chemical element contents in human femoral neck investigated by instrumental neutron activation analysis. Biol. Trace Elem. Res. 137, 1–12. doi: 10.1007/s12011-009-8554-z
Zaichick, V., Tzaphlidou, M. (2002). Determination of calcium, phosphorus, and the calcium/phosphorus ratio in cortical bone from the human femoral neck by neutron activation analysis. Appl. Radiat. Isot. 56, 781–786. doi: 10.1016/S0969-8043(02)00066-0
Zazzo, A., Lécuyer, C., Mariotti, A. (2004a). Experimentally-controlled carbon and oxygen isotope exchange between bioapatites and water under inorganic and microbially-mediated conditions. Geochim. Cosmochim. Acta. 68, 1–12. doi: 10.1016/S0016-7037(03)00278-3
Zazzo, A., Lécuyer, C., Sheppard, S. M. F., Grandjean, P., Mariotti, A. (2004b). Diagenesis and the reconstruction of paleoenvironments: a method to restore original δ18O values of carbonate and phosphate from fossil tooth enamel. Geochim. Cosmochim. Acta. 68, 2245–2258. doi: 10.1016/j.gca.2003.11.009
Keywords: diagenesis, Ca/P, CO3/PO4, carbon isotopes, oxygen isotopes, bioapatite, X-ray fluorescence, enamel
Citation: Simpson EMB, Crowley BE and Sturmer DM (2023) Is the damage worth it? Testing handheld XRF as a non-destructive analytical tool for determining biogenic bone and tooth chemistry prior to destructive analyses. Front. Environ. Archaeol. 1:1098403. doi: 10.3389/fearc.2022.1098403
Received: 14 November 2022; Accepted: 13 December 2022;
Published: 09 January 2023.
Edited by:
Patrick Roberts, Max Planck Institute for the Science of Human History (MPI-SHH), GermanyReviewed by:
Eric Bartelink, California State University, Chico, United StatesSarah Pederzani, University of La Laguna, Spain
Copyright © 2023 Simpson, Crowley and Sturmer. This is an open-access article distributed under the terms of the Creative Commons Attribution License (CC BY). The use, distribution or reproduction in other forums is permitted, provided the original author(s) and the copyright owner(s) are credited and that the original publication in this journal is cited, in accordance with accepted academic practice. No use, distribution or reproduction is permitted which does not comply with these terms.
*Correspondence: Emily M. B. Simpson, c2ltcHNvZXlAbWFpbC51Yy5lZHU=