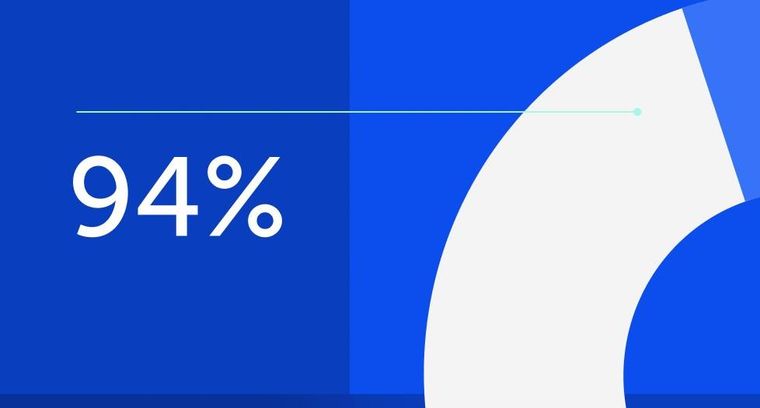
94% of researchers rate our articles as excellent or good
Learn more about the work of our research integrity team to safeguard the quality of each article we publish.
Find out more
METHODS article
Front. Energy Res., 02 April 2025
Sec. Hydrogen Storage and Production
Volume 13 - 2025 | https://doi.org/10.3389/fenrg.2025.1553134
This article is part of the Research TopicAdvanced Water Splitting Technologies Development: Best Practices and Protocols Volume IIView all 7 articles
This study presents the validation of protocols for measuring ion exchange capacity (IEC) and alkaline stability of anion exchange membranes (AEMs) for low-temperature water electrolysis. While protocols are often tested within individual laboratories, their results across multiple laboratories with varying equipment, environmental conditions, and personnel qualification remain unverified. The validation involved Los Alamos National Laboratory (LANL), National Renewable Energy Laboratory (NREL), and University of Oregon (UO) using the same commercially available AEM to assess reproducibility and reliability of the protocols under diverse conditions. For the IEC protocol, results across laboratories were consistent within ±10% of the NMR-determined reference value. The alkaline stability protocol could pose greater challenges due to factors such as variations in sample collection timing, preservation methods, and analytical techniques, but consistent test results for percentage IEC loss were demonstrated across institutions. These results highlight the reliability and applicability of the protocols, emphasizing the importance of validation to ensure consistency in diverse research environments.
Standard operating protocols (SOPs) have been developed for characterizing and benchmarking low-temperature electrolysis (LTE) materials, components, and sub-scale cells. These protocols offer valuable guidance on testing key properties of ion exchange membranes, such as ion exchange capacity (IEC) (Yan et al., 2023a), gas permeability (Park et al., 2022), water content (Capuano, 2023), conductivity (Yan et al., 2023b), and alkaline stability (Ramani and Meek, 2023). These protocols are designed to support the development of both proton exchange membranes (PEMs) and anion exchange membranes (AEMs). The goal of these protocols is to assist researchers, particularly those new to laboratory work or unfamiliar with material testing, by providing comprehensive, reproducible, and (largely) universal procedures. A carefully structured protocol incorporates insights gained from extensive testing and ensures reproducible results. By standardizing these procedures, researchers can minimize discrepancies in results and achieve greater consistency across different laboratories. Therefore, benchmarking these protocols is essential to reduce variability in reported results and ensure reliable comparisons, enabling more accurate evaluations within the research community.
A number of protocols have been developed and published for LTE materials over the last several years (Yan et al., 2023a; Park et al., 2022; Capuano, 2023; Yan et al., 2023b; Ramani and Meek, 2023; Meek, 2023a; Meek, 2023b; Arges et al., 2023; Alia and Danilovic, 2023; Serov, 2023; Morgan and Xu, 2023; Ouimet, 2023; Ouimet et al., 2022; Serov and Creel, 2021; Glenn, 2023; Ayers, 2023); however, they have not yet been cross-validated across multiple laboratories. While these protocols are typically tested and validated within the originating laboratory during development, they should be universal and adaptable for use in diverse settings to minimize result discrepancies between laboratories. Each laboratory may operate under unique conditions including variations in equipment from different manufacturers and with uncontrolled environmental factors. It is essential to assess whether the protocol remain consistent throughout these different settings. Identifying and documenting any necessary updates or adjustments to ensure the protocols work reliably in various laboratory environments is a critical step in achieving standardization across fields.
Los Alamos National Laboratory (LANL), the National Renewable Energy Laboratory (NREL), and the University of Oregon (UO) were selected to evaluate the SOPs LTE-P-7 AEM IEC (Yan et al., 2023a) and LTE-P-22 alkaline stability (Ramani and Meek, 2023). The goal was to assess the protocols’ applicability in multiple laboratories with varying conditions, equipment, chemicals, personnel, and environmental factors, while also adapting these protocols to best mitigate the potential discrepancies that arose. To minimize variability, a commercially available AEM sourced from the same batch was purchased and tested as a base material. LANL initiated the testing process, documenting any observations or updates needed while following the protocol. NREL and UO subsequently conducted the same protocols, using that laboratory’s distinct equipment and supplementary materials. The results from all three laboratories were compiled and analyzed to identify necessary refinements to the protocols. Based on these findings, the protocols were updated to enhance clarity and reliability. In this paper, the process of protocol validation, the results and discussion of the validation, and the subsequent updates made to improve the protocols are reported.
The purpose of this protocol is to provide guidance for validating procedures for SOPs LTE-P-7 (AEM IEC) and LTE-P-22 (alkaline stability).
A commercially available AEM, PiperION (PAP-TP-85-HCO3 by Versogen) (Wang et al., 2019; Yan et al., 2019), was distributed among institutions for the validation process. The selection of the membrane is not restricted to PiperION. The validation team can select a membrane of interest, preferably sourced from the same batch and roll, and proceed the validation. One institution began by reviewing, testing, and improving the protocols by updating details. The updates include enhanced instructions for equipment, supply, and chemical choices, additional procedure steps, and replacement of alternative titration methods and characterizing techniques to improve clarity and consistency. The revised protocols were then executed by other institutions, noting minor variations in equipment or supplementary materials. The results from all laboratories were compiled and analyzed to refine the protocols.
For SOP LTE-P-7 (AEM IEC), AEM samples (∼50.0 mg) in the bicarbonate counter-ion form were converted to the Br− form by soaking them in 4.00 M KBr solution with 0.0200 M KOH. After thoroughly rinsing with ultrapure water, the membranes were dried under vacuum, and their dry weight was measured. The samples were then exchanged with 1.00 M KNO3 solution twice for 24 h each with agitation. The exchange solutions were combined, and 2.00% HNO3 was added to adjust the pH ca. 3–4. An auto-titrator was used to titrate the solution with 0.0200 N AgNO3, and a silver/sulfide combination ion-selective electrode monitored the titration progress.
For SOP LTE-P-22 (alkaline stability), AEM samples (∼2.00 × 102 mg) were immersed in 1.00 M KOH at 80 °C for varied time intervals in polyethylene wide-mouth bottles. Samples were taken out from the bottles and washed with ultrapure water at regular time intervals (20, 50, 100, 200, 300, 400, and 500 h), and the procedure for IEC testing was carried out for each sample prior to IEC evaluation. For the associated ionic-conductivity measurements, the samples were converted to their chloride form by ion exchanging in a 1.00 M NaCl solution.
Operators are required to have basic laboratory safety training required by their respective institutions. They should also have knowledge of basic laboratory procedures, including an understanding of titration, conductivity measurements, and nuclear magnetic resonance (NMR) analysis. Additionally, operators must be trained in the proper use of relevant equipment and associated software.
All solutions must be handled with care, using appropriate personal protective equipment (PPE). Operators should review the safety data sheets (SDS) for all chemicals involved. Concentrated KOH is caustic and skin contact must be avoided.
For SOP LTE-P-7 (AEM IEC), the following materials and equipment are required: KBr (e.g., ACS reagent, ≥99.0%, Sigma Aldrich), ultrapure water (e.g., Milli-Q® Type 1 Ultrapure Water, 18.2 MΩ cm resistivity), AgNO3 (e.g., ACS reagent, ≥99.0%, Sigma Aldrich), KNO3 (e.g., ACS reagent ≥99.0%, Sigma Aldrich), HNO3 (e.g., ACS reagent, 70%, Sigma Aldrich), KOH (e.g., Semiconductor grade, 99.99%, Sigma Aldrich), and a titrator equipped with a silver/sulfide ion-selective electrode. The AgNO3 solution used in IEC titration must be stored away from light to prevent photodecomposition, which could affect the subsequent measurement. Suitable instruments include the Hanna HI931 with HI4115, the Thermo Scientific™ Orion Star T940 All-in-One Titrator paired with Orion™ Silver/Sulfide ISE Electrode 9416BNWP, the Mettler Toledo T90 Autotitrator with Silver Ring Electrode DM141-SC, or an equivalent equipment.
For SOP LTE-P-22 (alkaline stability), the required materials and equipment include an oven with temperature control (e.g., 25°C–95 °C), polyethylene wide-mouth bottles, inert gas (i.e., argon), ultrapure water (e.g., Milli-Q® Type 1 Ultrapure Water, 18.2 MΩ cm resistivity), KOH (e.g., Semiconductor grade, 99.99%, Sigma Aldrich), and NaCl (e.g., ReagentPlus®, ≥99.0%, Sigma Aldrich). Additional instrumentation, if available, includes NMR spectrometers for structural analysis, conductivity measuring equipment such as an electrochemical impedance spectroscopy (EIS) system and a frequency response analyzer (FRA).
Ion exchange capacity (IEC); anion exchange membrane (AEM); nuclear magnetic resonance (NMR); electrochemical impedance spectroscopy (EIS); frequency response analyzer (FRA).
To initiate the validation process among three institutions, LANL conducted an initial evaluation of the protocols in LTE-P-7 and the reference by Wang et al. (2022) Standard Operating Protocol for Ion-Exchange Capacity of Anion Exchange Membranes. Front. Energy Res.). The calculated IEC of the AEM of interest, determined using NMR analysis, was found to be 2.01 ± 0.02 meq/g in the Br− form and 2.30 ± 0.02 meq/g in the OH− form. These NMR-derived IEC values serve as a comparison to the titration-based IEC measurements and align with the previous report (Wang et al., 2019), which reported an IEC of 2.37 meq/g in the OH− form.
Both procedures begin by soaking the membrane (∼50.0 mg) in 4.00 M KBr with 0.0200 M KOH for 24 h, and then rinsing it thoroughly with ultrapure water. The membrane is subsequently dried and weighed immediately. The key difference in procedures from the two sources lies in the ion-exchange step. In Method 1 (Yan et al., 2023a), the dried membranes are exchanged twice with fresh 1.0 M KNO3 solutions for ion exchange over a total period of 2 days, and the solution is saved. In contrast, Method 2 (Wang et al., 2022) recommends using ∼50.0 mg of dry lithium triflate (LiOTf) mixed with ∼150 mL of ultrapure water to complete ion exchange in 30 min.
Following the ion-exchange step in both methods, the IEC titration is performed using 0.0200 N AgNO3 with the auto-titrator. The auto-titrator determines the titration endpoint by monitoring the inflection point in the potential vs titrant volume curve (Figure 1). Based on the titrant volume displayed on the auto-titrator screen, the IEC can be calculated using Equation 1:
Figure 1. Titration curve and first derivative of potential over volume. The endpoint of the titration is given by the vertical blue line.
VAgNO3 is the titrated volume of AgNO3 titrant (mL); CAgNO3 is the normality of AgNO3 (mmol/mL or meq/mL); and mdry is the weight of dry AEM sample (g).
Using Method 1 (Yan et al., 2023a), where KNO3 was used for ion exchange, the average IEC obtained was 1.90 ± 0.01 meq/g in the Br− form (Table 1). The corresponding value in the OH− form was 2.16 ± 0.01 meq/g. In Method 2 (Wang et al., 2022), where LiOTf was used for ion exchange, the average IEC was 1.82 ± 0.08 meq/g in the Br− form (Table 1), and 2.06 ± 0.10 meq/g in the OH− form. Compared to the NMR-derived IEC of 2.30 ± 0.02 meq/g (OH− form), Method 1 (Yan et al., 2023a) using KNO3 produced values closer to the NMR-derived IEC and demonstrated a smaller standard deviation, indicating better consistency.
Table 1. IEC values obtained from titration using LTE-P-7 and the SOP from Frontiers in Energy Research.
Method 1 has the advantage of having the closest IEC value to the theoretical IEC value, better reproducibility, chemical availability, and lower cost although it takes 5 days for ion exchange with nitrate anions. Method 2 has the advantage of taking shorter time (∼3 days) due to the faster exchange rate of triflate ions, but the result came with larger standard deviation, indicating insufficient ion exchange with the given time. Extending the exchange time and performing multiple exchanges (e.g., decanting and refilling with fresh ion exchange solution, as in Method 1) can optimize the ion exchange process. Additionally, LiOTf is expensive with limited chemical availability than KNO3.
Based on the results obtained at LANL, Method 1, which uses KNO3 as the ion exchange solution, was selected for further testing. The protocol was subsequently revised and formalized into the updated LTE-P-7, incorporating the detailed procedures of Method 1, and further validation was conducted using the updated protocol by NREL and the UO. NREL and UO, reported average IEC values (Br− form) of 2.07 ± 0.01 meq/g and 1.94 ± 0.01 meq/g (Table 2). Comparing the LTE-P-7 IEC values across the three laboratories to the 1H NMR reference value determined by LANL, the percent differences between the measurements in the Br− form were -−5.47% for LANL, +2.99% for NREL, and −3.48% for the UO (Table 2). Because these differences are much larger than the standard deviations within samples measured at each site, there evidently remain small differences between protocol implementation and measurements resulting in the ±10% range in values.
The experiments were conducted in a normal lab setting (30%–60% RH, 25°C), minimizing the impact of environmental variations on the results. Potential errors from differences in chemical grades and sample handling methods were limited by specifying chemical grades and following the same procedure. A most significant source for variation in results could be using different autotitrators across laboratories, including the instrument precision, as well as calibration status and maintenance history. However, our results came reasonably consistent with an acceptable level of variation, falling within a ±10% range.
Initiating the validation process for LTE-P-22 (alkaline stability) required LANL to first test and compare the conditions outlined in the original LTE-P-22. LANL investigated whether 1.00 M or 4.00 M KOH provided adequate testing conditions. The evaluation also included time intervals for sample collection (20, 50, 100, 200, 300, 400, and 500 h) and the identification of critical steps for sample preparation and preservation.
After the alkaline stability test, the membranes were preserved in 1.00 M NaCl solution. In a subsequent trial, UO processed and measured each sample immediately after each timer interval, while the reproduction by NREL maintained the preservation method originally used, measuring all samples at once at the conclusion of the test. Prior to analysis, it was found crucial to completely dry the membranes and repeat the ion exchange process by immersing them in the appropriate solution. Without thorough drying, ion exchange appeared ineffective. Storage of AEMs for a short time scale of up to several weeks may be done in a neutral pH electrolyte solution such as NaCl. For long-term storage, a sealed container is recommended with approximately 100 ppm biocide (NaN3) or 1–3 wt% sodium sulfite (Na2SO3) to prevent biological fouling (Fuel Cell Store, 2025a; Fuel Cell Store, 2025b).
Using the updated LTE-P-7 protocol, LANL measured the IEC of membranes exposed to either 1.00 M or 4.00 M KOH at various time intervals (Figure 2; Table 3). Both conditions showed an initial decrease in IEC between 0 and 100 h, followed by a gradual decline for the remaining time intervals. Minimal differences in IEC were observed between the membranes treated with 1.00 M KOH and 4.00 M KOH, indicating that the harsher 4.00 M KOH condition did not result in significantly greater degradation. All IEC measurements were conducted in the Br− form. The retention percentage was calculated based on changes in the IEC at different time intervals, relative to the initial IEC value.
Figure 2. IEC values as a function of time for membranes in alkaline solutions: (A) 1.00 M KOH and (B) 4.00 M KOH.
Table 3. IEC values and percent changes for membranes after exposure to 1.00 M and 4.00 M KOH alkaline solutions.
Chloride conductivity was measured using a two-probe cell with membranes ion-exchanged into Cl− form. The procedure included drying the membranes, ion-exchanging them in 1.00 M NaCl for 2 days, and equilibrating them in ultrapure water for 1 day. Conductivity measurements were performed at 25 °C and 80°C, with membranes equilibrated at the respective temperatures before measurement. Minimal variations in chloride conductivity were observed between membranes treated with 1.00 M KOH and 4.00 M KOH (Figure 3; Table 4). While there was a slight decrease in chloride conductivity for membranes treated with 4.00 M KOH, the difference remained minimal. Interestingly, for membranes with 1.00 M KOH, a slight increase in conductivity was noted during the first 50 h, which may indicate morphological changes in the alkaline stability environment. A similar trend was observed for 4.00 M KOH.
Figure 3. Chloride conductivity values as a function of time for membranes in alkaline solutions: (A) 1.00 M KOH and (B) 4.00 M KOH.
Table 4. Chloride conductivity values and percent changes for membranes after exposure to 1.00 M and 4.00 M KOH alkaline solutions.
LANL compared the alkaline stability results after exposure to 1.00 M KOH using the updated LTE-P-22 protocol (Table 5). While UO started the sample preparation for IEC measurements immediately after removing the samples from KOH solution, LANL and NREL stored their samples in 1.00 M NaCl prior to IEC testing. This difference enabled the evaluation of whether the two different sample-handling methods resulted in variations in membrane degradation profiles. After approximately 50 h of exposure, the percentage of IEC loss was 11.2% for LANL, 10.1% for NREL, and 11.0% for the UO, with a maximum difference of 1.1% between the values. After 500 h of exposure, the percentage of IEC loss was 14.6% for LANL, 13.7% for NREL, and 15.1% for the UO, with a maximum difference of 1.4% between the values. Across all three institutions, the validation showed consistent results in terms of percentage of IEC loss. The consistent sample results across institutions highlights the reliability of the alkaline stability testing method, validating the updated LTE-P-22 as a reliable method for assessing alkaline stability of AEMs. Additionally, storing the samples in a NaCl solution until ready for IEC testing was found to effectively prevent further degradation of the membranes, given the similarity of the results to those obtained when the membranes were immediately tested.
The protocol validation processes for LTE-P-7 and LTE-P-22 were successfully conducted across multiple institutions. A consistent and detailed procedure was provided to the participating labs. However, there were slight variations in equipment, such as the titrator or conductivity measurement setup, which provides information on the universality of the protocols and the typical variations introduced across laboratories. These variations allowed us to determine whether the protocols remained reliable across different setups and conditions.
The validation process for LTE-P-7 (AEM IEC) focused solely on measuring IEC, simplifying the comparison of results across participating labs. With the availability of a reliable reference, the NMR-determined IEC, the validation process was further streamlined. As a result, the IEC values across the labs were consistent and reliable within ±10% of the NMR-determined reference.
Validating LTE-P-22 (alkaline stability) posed challenges due to the variety of characterization methods available and the greater number of samples involved. The process required careful attention to variables such as sample collection timing, preservation methods, and the specific analytical techniques employed, which varied depending on each lab’s capabilities. While IEC is a critical parameter for validation, additional methods like conductivity and NMR may be utilized if available and relevant. However, these methods should not be universally required, as the primary objective is to confirm that the protocol functions effectively within the constraints of different laboratories. LANL, NREL, and UO compared the percentage of IEC loss and demonstrated consistent results, validating the reliability of the LTE-P-22 protocol.
The validation of LTE-P-7 and LTE-P-22 was successful and demonstrated the protocols’ reliability across different institutions and equipment setups. This process highlights the importance of universal applicability in protocol design. Based on these results, similar validation efforts should be conducted for other protocols to ensure consistency and reliability across diverse research environments.
The original contributions presented in the study are included in the article/supplementary material, further inquiries can be directed to the corresponding authors.
SA: Formal Analysis, Investigation, Methodology, Validation, Visualization, Writing–original draft, Writing–review and editing. AB: Formal Analysis, Investigation, Methodology, Validation, Visualization, Writing–original draft, Writing–review and editing. AE: Formal Analysis, Investigation, Methodology, Validation, Visualization, Writing–original draft, Writing–review and editing. AP: Formal Analysis, Investigation, Methodology, Validation, Visualization, Writing–original draft, Writing–review and editing. OV: Validation, Visualization, Writing–review and editing. MK: Formal Analysis, Investigation, Methodology, Validation, Visualization, Writing–original draft, Writing–review and editing. YK: Conceptualization, Methodology, Writing–review and editing. SB: Supervision, Writing–review and editing. EP: Conceptualization, Methodology, Supervision, Visualization, Writing–original draft, Writing–review and editing. GR: Conceptualization, Project administration, Writing–review and editing. SP: Project administration, Writing–review and editing. KA: Conceptualization, Project administration, Writing–review and editing.
The author(s) declare that financial support was received for the research and/or publication of this article. The US Department of Energy (US DOE), Office of Energy Efficiency and Renewable Energy (EERE), and Hydrogen and Fuel Cell Technologies Office (HFTO) supported this work through the H2NEW (Hydrogen from Next-generation Electrolyzers of Water) Consortium.
Authors GR, SP, and KA were employed by the Nel Hydrogen.
The remaining authors declare that the research was conducted in the absence of any commercial or financial relationships that could be construed as a potential conflict of interest.
The author(s) declare that no Generative AI was used in the creation of this manuscript.
All claims expressed in this article are solely those of the authors and do not necessarily represent those of their affiliated organizations, or those of the publisher, the editors and the reviewers. Any product that may be evaluated in this article, or claim that may be made by its manufacturer, is not guaranteed or endorsed by the publisher.
Arges, C. G., Ramani, V., Wang, Z., and Ouimet, R. (2023). “LTE-P-23 AEM alkaline-oxidative stability SOP,”. United States. Available online at: https://datahub.h2awsm.org/dataset/a4f6ba8f-873d-4787-8063-aa1900c3b1c2/resource/e3377065-d1d1-4829-bc62-aae7de57c3bb/download/lte-p-23-aem-alkaline-oxidative-stability-sop-rev-3.pdf (Accessed December 2, 2024).
Ayers, K. (2023). LTE-P-19 comparison metrics and terms for low temperature electrolysis. United States. doi:10.17025/1983656
Fuel Cell Store (2025a). Fumasep® FAB-PK-130. Available online at: https://www.fuelcellstore.com/fumasep-fab (Accessed February 13, 2025).
Fuel Cell Store (2025b). Fumasep® FAM. Available online at: https://www.fuelcellstore.com/fumasep-fam (Accessed February 13, 2025).
Glenn, J. (2023). LTE-P-21 SOP for post-operation cell component disassembly and observation of benchtop water electrolyzer testing. Available online at: https://datahub.h2awsm.org/dataset/a4f6ba8f-873d-4787-8063-aa1900c3b1c2/resource/75ebe7c8-5102-41f4-b5e5-e97a6329e667/download/lte-p-21-post-test-teardown-analysis-rev-2.pdf (Accessed December 2, 2024).
Ouimet, R. (2023). LTE-P-17 PTL resistance, porosity, and porosimetry measurements. United States. Available online at: https://datahub.h2awsm.org/dataset/a4f6ba8f-873d-4787-8063-aa1900c3b1c2/resource/339d3853-5188-48dd-a59c-fc88b13956c5/download/lte-p-17-ptl-resistance-and-water-properties_rev2.pdf (Accessed December 2, 2024).
Ouimet, R. J., Young, J. L., Schuler, T., Bender, G., Roberts, G. M., and Ayers, K. E. (2022). Measurement of resistance, porosity, and water contact angle of porous transport layers for low-temperature electrolysis Technologies. Front. Energy Res. 10. doi:10.3389/fenrg.2022.911077
Park, E. J., Komini Babu, S., and Kim, Y. S. (2022). Gas permeability test protocol for ion-exchange membranes. Front. Energy Res. 10. doi:10.3389/fenrg.2022.945654
Ramani, V., and Meek, K. (2023). LTE-P-22 alkaline stability of AEMs. United States. doi:10.17025/1983654
Serov, A., and Creel, E. (2021). LTE-P-13 3-electrode cell for screening OER/HER electrocatalyst activity. Available online at: https://datahub.h2awsm.org/dataset/a4f6ba8f-873d-4787-8063-aa1900c3b1c2/resource/63250c2e-b76f-4de5-90c2-de84e571251d/download/lte-p-13-3-electrode-cell-for-screening-oerher-electrocatalyst-activity.pdf (Accessed December 2, 2024).
Wang, J., Zhao, Y., Setzler, B. P., Rojas-Carbonell, S., Ben Yehuda, C., Amel, A., et al. (2019). Poly(aryl piperidinium) membranes and ionomers for hydroxide exchange membrane fuel cells. Nat. Energy 4 (5), 392–398. doi:10.1038/s41560-019-0372-8
Wang, L., Rojas-Carbonell, S., Hu, K., Setzler, B. P., Motz, A. R., Ueckermann, M. E., et al. (2022). Standard operating protocol for ion-exchange capacity of anion exchange membranes. Front. Energy Res. 10. doi:10.3389/fenrg.2022.887893
Yan, Y., Rojas-Carbonell, S., Setzler, B., Ueckermann, M., and Meek, K. (2023a). LTE-P-7 IEC AEM SOP. United states. doi:10.17025/1983663
Yan, Y., Rojas-Carbonell, S., Setzler, B., Ueckermann, M., and Meek, K. (2023b). “LTE-P-6 AEM conductivity,”. United States. doi:10.17025/1983666
Keywords: green hydrogen, water splitting, low-temperature water electrolysis, anion exchange membrane, ion exchange capacity, alkaline stability, membrane property, protocol validation
Citation: Ahn SM, Boudreau A, Ekennia AC, Palau A, Vulpin OT, Kwak M, Kim YS, Boettcher SW, Park EJ, Roberts GM, Perez Bakovic SI and Ayers KE (2025) Anion exchange membrane test protocol validation. Front. Energy Res. 13:1553134. doi: 10.3389/fenrg.2025.1553134
Received: 30 December 2024; Accepted: 18 March 2025;
Published: 02 April 2025.
Edited by:
Chenxiao Jiang, University of Science and Technology of China, ChinaCopyright © 2025 Ahn, Boudreau, Ekennia, Palau, Vulpin, Kwak, Kim, Boettcher, Park, Roberts, Perez Bakovic and Ayers. This is an open-access article distributed under the terms of the Creative Commons Attribution License (CC BY). The use, distribution or reproduction in other forums is permitted, provided the original author(s) and the copyright owner(s) are credited and that the original publication in this journal is cited, in accordance with accepted academic practice. No use, distribution or reproduction is permitted which does not comply with these terms.
*Correspondence: Su Min Ahn, c2FobkBsYW5sLmdvdg==; Eun Joo Park, ZXBhcmtAbGFubC5nb3Y=; George M. Roberts, Z3JvYmVydHNAbmVsaHlkcm9nZW4uY29t
†These authors have contributed equally to this work and share first authorship
Disclaimer: All claims expressed in this article are solely those of the authors and do not necessarily represent those of their affiliated organizations, or those of the publisher, the editors and the reviewers. Any product that may be evaluated in this article or claim that may be made by its manufacturer is not guaranteed or endorsed by the publisher.
Research integrity at Frontiers
Learn more about the work of our research integrity team to safeguard the quality of each article we publish.